- 1University of Science and Technology of Hanoi, Vietnam Academy of Science and Technology, Hanoi, Vietnam
- 2LMI DRISA, IRD-USTH, Hanoi, Vietnam
- 3Institute of Biotechnology, Vietnam Academy of Science and Technology, Hanoi, Vietnam
- 4UMR MIVEGEC, University of Montpellier-IRD-CNRS, Montpellier, France
Introduction: In the past decades, extended-spectrum beta-lactamase (ESBL)-producing and carbapenem-resistant (CR) Escherichia coli isolates have been detected in Vietnamese hospitals. The transfer of antimicrobial resistance (AMR) genes carried on plasmids is mainly responsible for the emergence of multidrug-resistant E. coli strains and the spread of AMR genes through horizontal gene transfer. Therefore, it is important to thoroughly study the characteristics of AMR gene-harboring plasmids in clinical multidrug-resistant bacterial isolates.
Methods: The profiles of plasmid assemblies were determined by analyzing previously published whole-genome sequencing data of 751 multidrug-resistant E. coli isolates from Vietnamese hospitals in order to identify the risk of AMR gene horizontal transfer and dissemination.
Results: The number of putative plasmids in isolates was independent of the sequencing coverage. These putative plasmids originated from various bacterial species, but mostly from the Escherichia genus, particularly E. coli species. Many different AMR genes were detected in plasmid contigs of the studied isolates, and their number was higher in CR isolates than in ESBL-producing isolates. Similarly, the blaKPC-2, blaNDM-5, blaOXA-1, blaOXA-48, and blaOXA-181 β-lactamase genes, associated with resistance to carbapenems, were more frequent in CR strains. Sequence similarity network and genome annotation analyses revealed high conservation of the β-lactamase gene clusters in plasmid contigs that carried the same AMR genes.
Discussion: Our study provides evidence of horizontal gene transfer in multidrug-resistant E. coli isolates via conjugative plasmids, thus rapidly accelerating the emergence of resistant bacteria. Besides reducing antibiotic misuse, prevention of plasmid transmission also is essential to limit antibiotic resistance.
1. Introduction
The rapid increase of antibiotic-resistant bacteria, which is the consequence of excessive and inappropriate use of antibiotics (Kahlmeter et al., 2003; Costelloe et al., 2010), is a tremendous public health issue because it hinders the proper treatment of infections, thus increasing morbidity and mortality and also healthcare costs (Cosgrove and Carmeli, 2003). It was estimated that in 2019, antimicrobial resistance (AMR) was associated with ~4.95 million deaths worldwide and that AMR bacteria directly caused 1.27 million deaths. Escherichia coli is one of the six leading AMR bacteria associated with more than 800,000 deaths (Antimicrobial Resistance Collaborators, 2022). E. coli, a Gram-negative bacterial species, is found in the lower intestine of humans and plays a vital role as commensal bacterium, but can also cause urinary tract infections, sepsis and meningitis (McNally et al., 2013), and is the leading cause of community and hospital infections. Many studies have highlighted the AMR threat in healthcare and agricultural settings worldwide, but particularly in low- and middle-income countries (Nhung et al., 2016; Yamasaki et al., 2017; Tran et al., 2019). Even if antibiotic resistance is a global issue, Asia remains the major source of resistance in the world. Almost 35% of the emerging infectious diseases identified in Asia between 1940 and 2004 correspond to the emergence of a new pattern of antimicrobial drug resistance (Jones et al., 2008; Horby et al., 2013). South East Asia including Vietnam is considered as a hot spot for AMR emergence because of the possible access to antimicrobials for humans without prescription despite the regulations and the high antimicrobial usage for livestock (Mao et al., 2015; Carrique-Mas et al., 2020). Despite the National Action Plan to combat AMR implemented since 2013, Vietnam is still heavily affected by the emergence and rapid spread of bacteria, resistant to many antibiotics. A recent large-scale study on 3,153 multidrug-resistant E. coli, Klebsiella pneumoniae and Acinetobacter baumannii isolates from two intensive care units (ICU) reported a high prevalence of AMR genes and evidence of extensive transmission between ICU patients in Vietnam (Roberts et al., 2022).
The spread of antibiotic resistance is mostly driven by horizontal gene transfer via conjugative plasmids (von Wintersdorff et al., 2016). Majority of carbapenem-resistant E. coli strains harbor blaNDM-1 in China (Zhang et al., 2017) and Vietnam (Tran et al., 2015), which emphasizes regionally dissemination of carbapenem-resistant Enterobacteriaceae depending on the horizontal transfer of their plasmid-mediated genes. Among the Enterobacteriaceae, E. coli can incorporate extracellular plasmids ‘naturally’ without special treatment (Tsen et al., 2002). According to the NCBI database (accessed on May 31, 2022), up to 17 different types of native plasmids can be found in the E. coli genome and they represent up to 14% of E. coli genetic material. Unlike chromosomes, plasmids can exist in multiple copies that increase the conjugation frequency and allow the rapid spread of AMR genes within microbial communities (Dimitriu et al., 2021). Plasmid-encoded genes are often carried in complex structures and exist in a variety of plasmid types (Hirabayashi et al., 2021). Given the vital role of plasmids in the acquisition and dissemination of AMR genes, it is important to thoroughly study their characteristics in multidrug-resistant clinical isolates. In the present study, we performed a large-scale analysis of putative plasmids using whole-genome sequencing data retrieved from several published studies on extended-spectrum beta-lactamase (ESBL)-producing and carbapenem-resistant (CR) E. coli isolates from Vietnamese patients. The presence of AMR genes on putative plasmids was investigated to assess the risk of transmission particularly of genes associated with resistance to carbapenems.
2. Materials and methods
2.1. Whole-genome sequencing data of multidrug-resistant Escherichia coli isolates in Vietnam
In this analysis, we searched all genomic studies investigating multidrug-resistant E. coli isolated from clinical samples in Vietnam. We eliminated studies that did not perform whole-genome sequencing using Illumina platforms or did not publish raw sequencing data, leaving 3 studies for inclusion. Raw sequencing data of 751 multidrug-resistant E. coli strains previously published by Roberts et al. (2022) (ENA Bioproject: PRJEB29424 and PRJEB28400), Hirabayashi et al. (2021) (provided by the authors), and Tuan-Anh et al. (2020) (ENA Bioproject PRJEB39354) were downloaded from the European Nucleotide Archive (ENA) database. Sixteen samples were from environmental surfaces (environmental isolates) and were collected at the National Hospital for Tropical Diseases, Hanoi, Vietnam. Among the other 735 clinical samples, 720 of the 721 isolates from the study by Roberts et al. (2022) were collected in two ICUs in Hanoi: 275 from the Bach Mai Hospital and 445 from the National Hospital for Tropical Diseases. One duplicate isolate (NHP1391) was excluded from the analysis. The two isolates (MH13 and MH17) from the study by Hirabayashi et al. (2021) were from the Military Hospital 103 in Hanoi, and the 27 isolates from the study by Tuan-Anh et al. (2020) were from the Children’s Hospital 1 in Ho Chi Minh city. Two E. coli isolates (TN1393 and XP817) were from our laboratory (NCBI SRA BioProject: PRJNA857185) and were from two patients hospitalized in the Saint Paul Hospital and the 108 Military Central Hospital, respectively. Most of the studied samples were collected between 2017 and 2019, except three samples (MH13, TN1393 and XP817) collected in 2012 and 2013. Each isolate is described in Supplementary Table S1.
2.2. Antimicrobial resistance profiling
The E. coli isolates from the study by Roberts et al. (2022) were classified into two phenotypes, ESBL-producing isolates and CR isolates, in function of their growth on selective media. The phenotype classification of the other isolates was based (i) on the antimicrobial susceptibility test results extracted from the studies by Hirabayashi et al. (2021) and Tuan-Anh et al. (2020), and (ii) for the two isolates from our laboratory (TN1393 and XP817), on antimicrobial susceptibility testing performed using ampicillin, amoxicillin-clavulanic acid, amikacin, piperacillin, ticarcillin, ticarcillin-clavulanic acid, cefalexin, cefoxitin, ceftazidime, colistin, fosfomycin, ofloxacin, imipenem, penicillin, and trimethoprim-sulfamethoxazole and the Kirby Bauer disk diffusion method, as previously described (EUCAST, 2020). In total, 4/751 strains were classified as non-ESBL-producing strains (see also Supplementary Table S1).
2.3. Genomic DNA extraction and Illumina sequencing
Genomic DNA (gDNA) of the two isolates from our laboratory was extracted with the Bacterial Genomic DNA Isolation Kit (Norgen Biotek Corp., Thorold, Ontario, Canada) following the manufacturer’s instructions. The gDNA samples were sheared randomly into small fragments with Covaris S/E210 or Bioruptor to prepare paired-end fragment libraries that were sequenced using an Illumina HiSeq 4,000 system at the Beijing Genomics Institute (Shenzhen, China). According to the information in the articles, gDNA was extracted with the QIACube and QIAamp 96 DNA QIACube HT kit (Qiagen, Hilden, Germany) and sequenced on a Illumina HiSeq X10 machine (Roberts et al., 2022); gDNA was extracted with the Wizard Kit (Promega) and sequenced on a Illumina MiSeq platform (Tuan-Anh et al., 2020); gDNA was extracted with the phenol-chloroform method and sequenced on a MiSeq (MH13 isolate) and on a HiSeq 4000 (MH17 isolate) apparatus (Hirabayashi et al., 2021).
2.4. Bioinformatics analysis
Raw whole-genome sequencing data were analyzed using the bioinformatics workflow described in Figure 1.
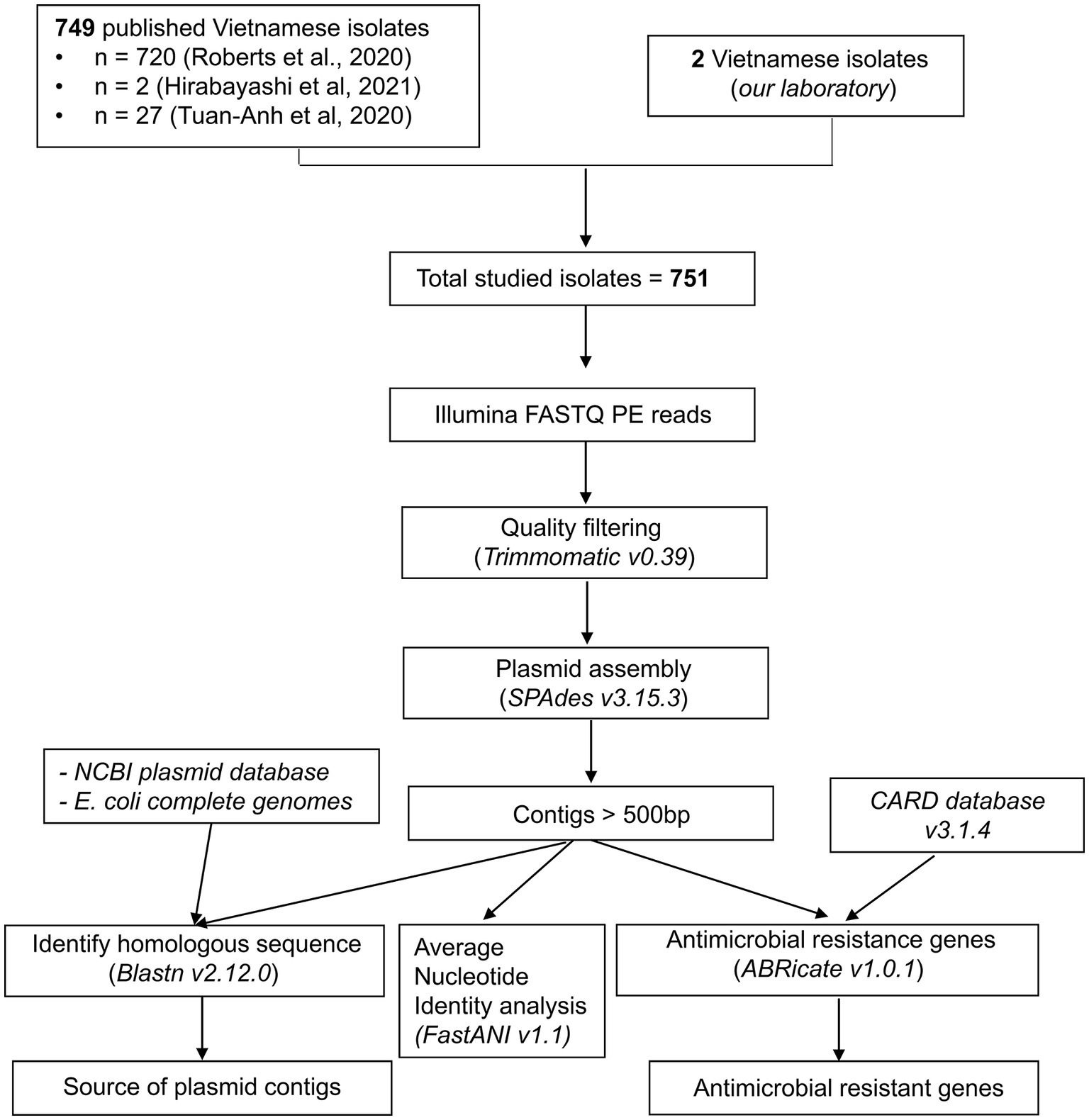
Figure 1. Bioinformatics workflow for plasmid analysis using raw sequencing data from multidrug-resistant E. coli isolates.
2.4.1. De novo assembly of whole-genome sequencing data
The paired-end raw reads were trimmed with Trimmomatic v0.39 (Bolger et al., 2014), using a Phred threshold score < 20, and the following parameters: SLIDINGWINDOW:4:20 LEADING:20 TRAILING:20 MINLEN:50. Trimmed reads were assembled into contigs using SPAdes de novo assembler v3.14.1 (Bankevich et al., 2012) with default parameters for genome assembly and with the ‘plasmid’ flag (plasmidSPAdes) for assembling plasmids (Antipov et al., 2016). Contigs <500 bp were discarded.
2.4.2. Identification of plasmid contigs and their origin
The contigs assembled with plasmidSPAdes were aligned to the NCBI RefSeq plasmid database (accessed on July 7, 2022) using BLASTn v2.12.0 (Altschul et al., 1990). A contig matched to the nearest neighbor (the highest BLAST bit score) in the plasmid database with a minimal identity of 95% and a minimal query coverage of 50% was defined as “putative plasmid contig.” Then, the remaining unassigned contigs were aligned against the 2,174 complete E. coli genomes available in the NCBI genome database (accessed on May 31, 2022) with BLASTn v2.12.0, and the previous thresholds were used to identify chromosomal contamination in the plasmidSPAdes assembly.
The taxonomic lineages of the plasmid homologs were obtained using the NCBI Taxonomy database (accessed on July 24, 2022). The plasmid homolog sequences were extracted from the plasmid databases using seqtk v1.3 (Li, 2013). The average nucleotide identity (ANI) between plasmid homologs was estimated using FastANI v1.1 (Jain et al., 2018). All pairs with ANI values <95% were excluded. The plasmid network was then built with Cytoscape v3.9.0 (Shannon et al., 2003).
2.4.3. Identification of chromosome contigs
For each isolate, contigs from the SPAdes assembly were aligned to their putative plasmid contigs from the plasmidSPAdes assembly using BLASTn v2.12.0 (Altschul et al., 1990). All contigs matched to plasmid contigs with a minimum identity of 99% and a minimum query coverage of 99% were filtered out, and the remaining contigs were identified as “chromosome contigs.”
2.4.4. Detection of antimicrobial resistance genes
AMR genes carried on contigs were identified using ABRicate v1.0.1 (Seemann, n.d.-a) and the CARD database v3.2.4 (Jia et al., 2017) and the following thresholds: 80% of minimum identity and 50% of minimum coverage. AMR genes were grouped into AMR gene families and drug classes using the CARD database v3.2.4. Contigs were functionally assessed with Rapid Annotations using Subsystem Technology (RAST) version 2.0 (Aziz et al., 2008).
2.4.5. Construction of the sequence similarity network
Pairwise alignments between putative plasmid contigs were carried out using BLASTn in the blastall (“BLAST all against all”) program with an e-value threshold of 1 × 10−10 (Camacho et al., 2009). The BLAST best hit for each pairwise alignment was determined. Alignments with identity <99% and minimum coverage <90% and also repetitive alignments were filtered out. The remaining best alignments were used to build the sequence similarity network with Cytoscape v3.9.0 (Shannon et al., 2003).
2.4.6. Multilocus sequence typing analysis
Multilocus sequence typing (MLST) data for the isolates were obtained from the published papers, or determined from the assembled genomes using mlst v2.22.0 (Seemann, n.d.-b) with the E. coli species scheme.
2.5. Statistical analyses
Statistical analyses and data visualization were done with R version 4.0.4 (R Core Team, 2016). The numbers of putative plasmid contigs and of AMR genes were compared in resistance phenotype groups with the Kruskal-Wallis test followed by the Dunn’s post-hoc tests for multiple pair comparisons using the kruskal.test function and the dunn.test function. The Fisher’s exact test was used to compare AMR genes between CR isolates and ESBL-producing isolates through fisher.test function. All p-values from multiple testing were corrected with the Benjamini-Hochberg method, and adjusted p-values <0.05 were considered significant using the p.adjust function. Correlation plots, box plots and bar plots were generated using the ggplot function from the ggplot2 package version 3.4.0. Binary heatmaps were constructed with the Heatmap function from the ComplexHeatmap package version 2.9.4 (Gu et al., 2016).
3. Results
3.1. Characteristics of putative plasmid contigs
The number of assembled plasmid contigs, using plasmidSPAdes, hugely varied among the 751 isolates, from 0 to 126 (median = 22, mean ± SD = 26 ± 19). In 8/751 isolates, no plasmid contig was identified. As expected, a moderate positive correlation was found between plasmid contig number and total assembly length (Pearson’s correlation coefficient, r = 0.55) (Figure 2A; Supplementary Table S1). Conversely, no correlation was found between plasmid contig number and sequencing coverage (r = 0.1) (Figure 2B; Supplementary Table S1), suggesting that the number of plasmid contigs in an isolate was linked to the presence of plasmids rather than to the sequencing coverage. Moreover, the number of plasmid contigs was higher in CR isolates (n = 197) than in ESBL-producing isolates (n = 550) (p = 0.008) and non-ESBL-producing isolates (n = 4) (p = 0.041) (Supplementary Figure S1). However, the highest number of plasmid contigs was found in an ESBL-producing strain (n = 126).
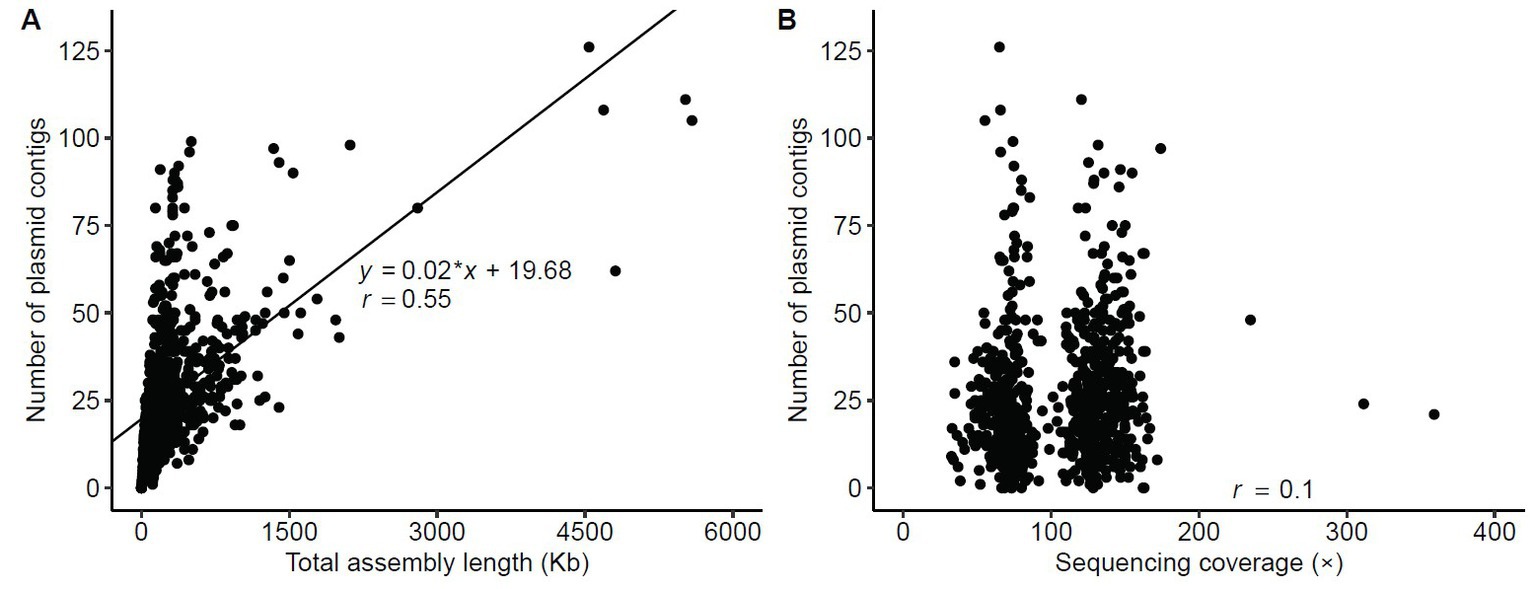
Figure 2. Statistics of contigs assembled using the plasmidSPAdes assembler. (A) Correlation of the number of plasmid contigs ≥500 bp with the total assembly length. (B) Relationship between number of contigs with length ≥ 500 bp and sequencing coverage. Correlations were assessed using a linear regression model; r, Pearson’s correlation coefficient.
Among the 19,894 plasmid contigs identified with plasmidSPAdes in the studied isolates, 18,098 (90.97%) were matched in the plasmid database (putative plasmid contigs) and 1,565 (7.87%) were matched to the E. coli chromosome with at least 95% sequence identity (Figure 3A). The other 231 contigs (1.16%) could not be matched (i.e., unassigned contigs). However, for 223/231 unassigned contigs, at least one homolog was found in the plasmid database or in the E. coli genome database, but with a low BLAST identity (between 70 and 93%). For the other unassigned contigs, a BLASTn homology search against the non-redundant (nr) nucleotide database found that four contigs shared high similarity with the A. baumannii chromosome (sequence identity >98%), one contig shared 96% of identity with an Escherichia phage, and three contigs could not be aligned to any sequence.
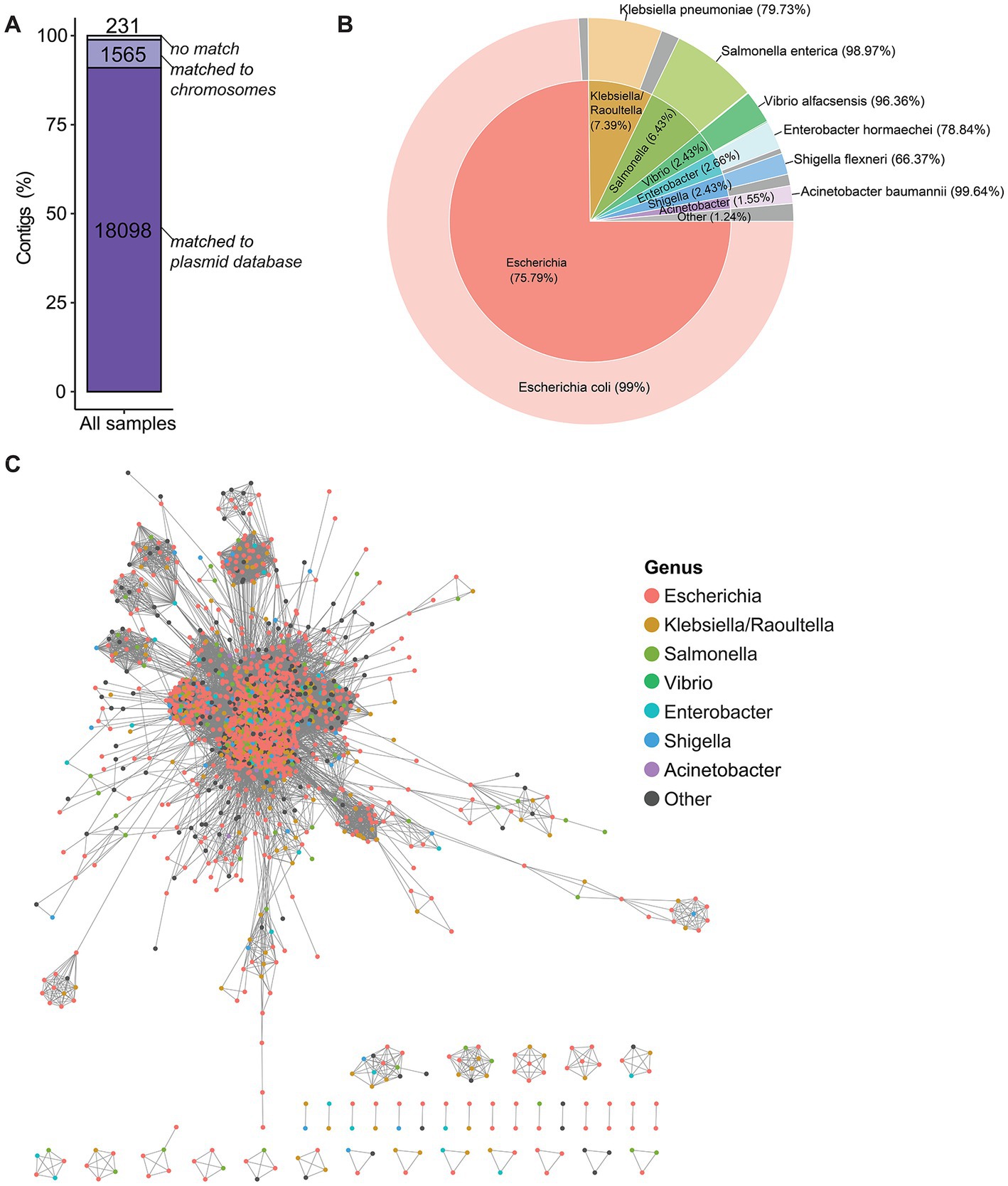
Figure 3. Origin of the putative plasmid contigs. (A) BLASTn homology search of contigs against the plasmid database and the complete E. coli genomes using the following thresholds: 95% of minimum identity and 90% of minimum query coverage. The numbers in the bars indicate the number of contigs. (B) Pie chart showing the percentages of plasmid homologs that matched to the putative plasmid contigs grouped by host genus and host species. “Other” (in gray): other species/genera with matching <1%. (C) Clustering of plasmid homologs by average nucleotide identity with a cut-off value of 95%. Each node represents a plasmid homolog and is color-coded in function of the host genus.
Next, the closest match of the putative plasmid contigs in the plasmid database, which was considered as the plasmid homolog, was investigated. Plasmid homologs were mostly from the Escherichia genus (75.79%), followed by the Klebsiella/Raoultella, Salmonella, Vibrio, Enterobacter, Shigella, and Acinetobacter genera. These genera mostly belong to the Enterobacteriaceae family, with the exception of Vibrio that belongs to the Vibrionaceae family and of Acinetobacter that belongs to the Moraxellaceae family. Each genus was dominated by a major species (between 66.37 and 99.64% of plasmid homologs for that genus) (Figure 3B). This indicates that plasmids could be shared between genera, but the transmissions of plasmids are more frequently observed within the same species than those between distinct species. A set of 2,331 plasmid types that represented all plasmid homologs was used for the ANI analysis based on whole plasmid alignments to identify the genetic relatedness among plasmid homologs. This analysis revealed that 1,955/2,331 plasmids from different genera were clustered together with an ANI value >95%, indicating that these plasmid homologs were very closely related. Conversely, 120/2,331 plasmids were in smaller clusters, and 256/2,331 plasmids did not share any similarity with the other plasmids (Figure 3C).
3.2. Antimicrobial resistance genes in the putative plasmid contigs
In total, 5,554 plasmid-carried AMR genes were found in 83.98% (624/743) of isolates with plasmids. Among these genes, 99.06% (5,502/5,554) were detected in contigs that were matched in the plasmid database (Supplementary Table S2) and the other 52 AMR genes were detected in contigs that matched to chromosomes and in unassigned contigs. More than 60% of AMR genes identified in putative plasmid contigs belonged to the major facilitator superfamily antibiotic efflux pump, sulfonamide resistance, trimethoprim resistant dihydrofolate reductase, aminoglycoside acetyltransferase ANT(3″), resistance-nodulation-cell division antibiotic efflux pump, macrolide phosphotransferase, CTX-M β-lactamase, and TEM β-lactamase families (Supplementary Table S2 and Supplementary Table S2). The mean number of AMR genes detected in plasmid contigs for each isolate was nine. Overall, the number of AMR genes in plasmid contigs was higher in CR isolates than ESBL-producing isolates (p < 0.001). As expected, non-ESBL-producing isolates, which had a lower resistance phenotype, had fewer AMR genes than CR and ESBL-producing isolates (Figure 4A). Both CR and ESBL-producing isolates harbored many different plasmid-carried AMR genes belonging to >40 different AMR gene families. Conversely, only five AMR gene families [i.e., major facilitator superfamily antibiotic efflux pump, sulfonamide resistance, TEM β-lactamase, aminoglycoside 6-phosphotransferase APH (6), aminoglycoside 3″-phosphotransferase were found in non-ESBL-producing isolates]. Although CR and ESBL-producing isolates showed similar frequencies of AMR gene families across the putative plasmid contigs, the frequency of genes encoding OXA, NDM and KPC β-lactamases, which are implicated in carbapenem resistance, varied between CR and ESBL-producing isolates (Figure 4B and Table 1). The presence/absence of AMR genes classed into AMR gene families was further investigated in each isolate. AMR genes belonging to the major facilitator superfamily, sulfonamide resistance, trimethoprim resistant dihydrofolate reductase, and aminoglycoside acetyltransferase ANT(3″) families were highly represented in the studied isolates. While there was no clear separation between groups on the binary heatmap of AMR gene family across the studied strains, the presence of AMR genes in isolates was not likely associated with their resistance phenotype, sequence type (MLST), location, and study. However, isolates belonging to the sequence type 617 (ST617) could be grouped in clusters on the basis of their AMR gene profile, and these clusters included mainly CR isolates (Figure 4C).
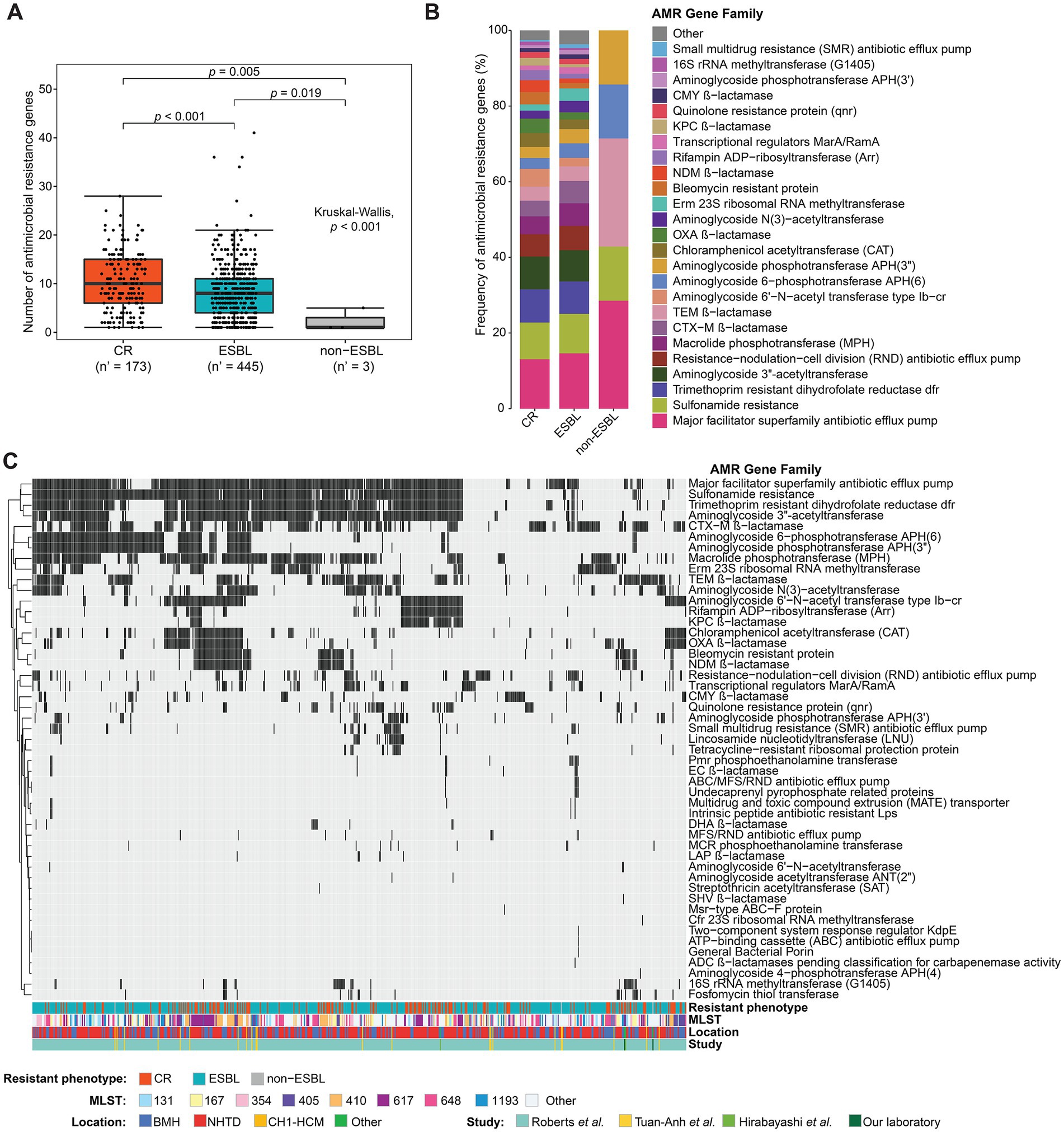
Figure 4. Antimicrobial resistance (AMR) genes in putative plasmid contigs. (A) Box plot showing the number of AMR genes detected in putative plasmid contigs of isolates grouped in function of their resistance phenotype; n’, number of isolates with the putative plasmid contigs carrying AMR genes. P-value calculated with the Kruskal-Wallis test followed by the Dunn’s post-hoc test and corrected for multiple testing with the Benjamini-Hochberg method. (B) Frequency of AMR genes, classified into AMR gene families, detected on putative plasmid contigs in carbapenem-resistant (CR) isolates, extended-spectrum beta-lactamase (ESBL)-producing isolates and non-ESBL-producing isolates. AMR genes with frequency < 1% were merged in the “Other” group. (C) Heatmap showing the presence (black) and absence (gray) of AMR gene families across isolates. Only isolates carrying at least one AMR gene were included. Hierarchical clustering was performed using Euclidean distances. The color bars at the bottom of the heatmap indicate the resistance phenotype, sequence type (MLST), location, and study from which the sequencing data of the isolates were taken. BMH, Bach Mai Hospital; NHTD, National Hospital for Tropical Diseases; CH1-HCM, Children’s Hospital 1-Ho Chi Minh city.
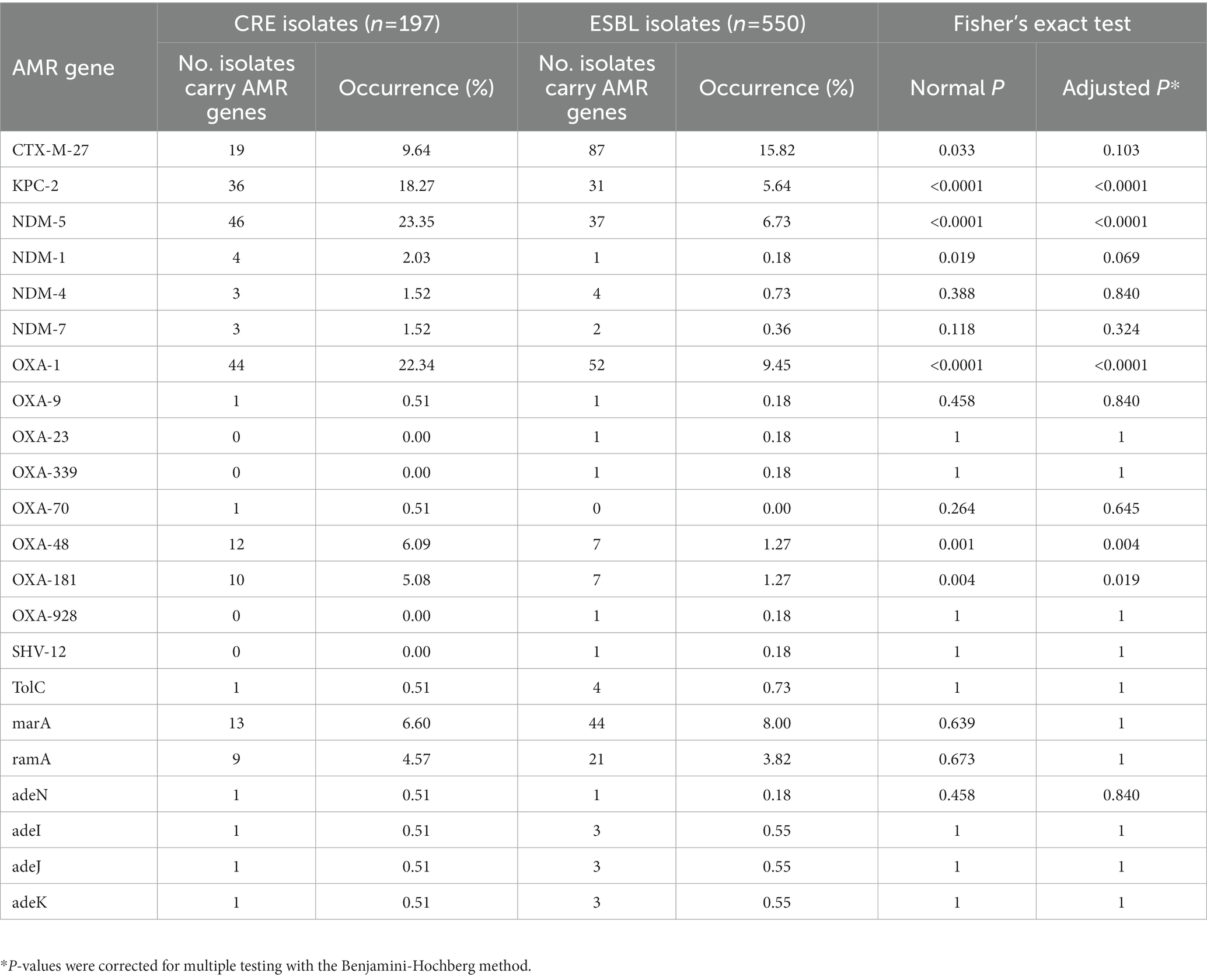
Table 1. Presence of AMR genes associated with resistance to carbapenems on putative plasmid contigs.
3.3. AMR genes and carbapenem resistance
Then, the analysis focused on 22 AMR genes that are associated with resistance to carbapenems. AMR genes belonging to the efflux pump and β-lactamase families were found in both CR and ESBL-producing isolates. Efflux pump genes (TolC, adeN, adeI, adeJ and adeK) were found in <1% of the studied strains. MarA and its homolog ramA, which encode bacterial transcriptional activators involved in regulating the AcrAB/TolC multidrug efflux pump, and porin genes were detected in ~11% of isolates. Carbapenemase-encoding genes (blaCTX-M, blaKPC, blaNDM and blaOXA) were detected in CR isolates and also in ESBL-producing isolates. Specifically, blaCTX-M-27, a β-lactamase-encoding gene that leads to resistance to carbapenems, cephalosporins, and penams, was the most frequently detected CR gene in putative plasmids (9.64% of CR and 15.82% of ESBL-producing isolates). Its presence in plasmids could be responsible for high resistance to antibiotics in CR and ESBL-producing isolates. Moreover, the frequency of blaKPC-2, blaNDM-5, blaOXA-1, blaOXA-48, and blaOXA-181 (β-lactamase-encoding genes) on putative plasmid contigs was higher in CR than ESBL-producing isolates (Fisher’s exact test: adjusted p-values <0.05). These genes are probably strongly associated with carbapenem resistance (Figure 5; Table 1). Additionally, >90% (n = 178/197) of the studied CR isolates carried at least one copy of the blaKPC, blaNDM, or blaOXA gene in plasmid or chromosome contigs. Specifically, 67 of these CR isolates carried these genes only on plasmid contigs, 60 only on chromosome contigs, and 51 on both plasmid and chromosome contigs. Together, these findings indicated that carbapenem hydrolysis by KPC, NDM, and OXA carbapenemases is the major mechanism underlying carbapenem resistance in E. coli isolates with a significant contribution from plasmids.
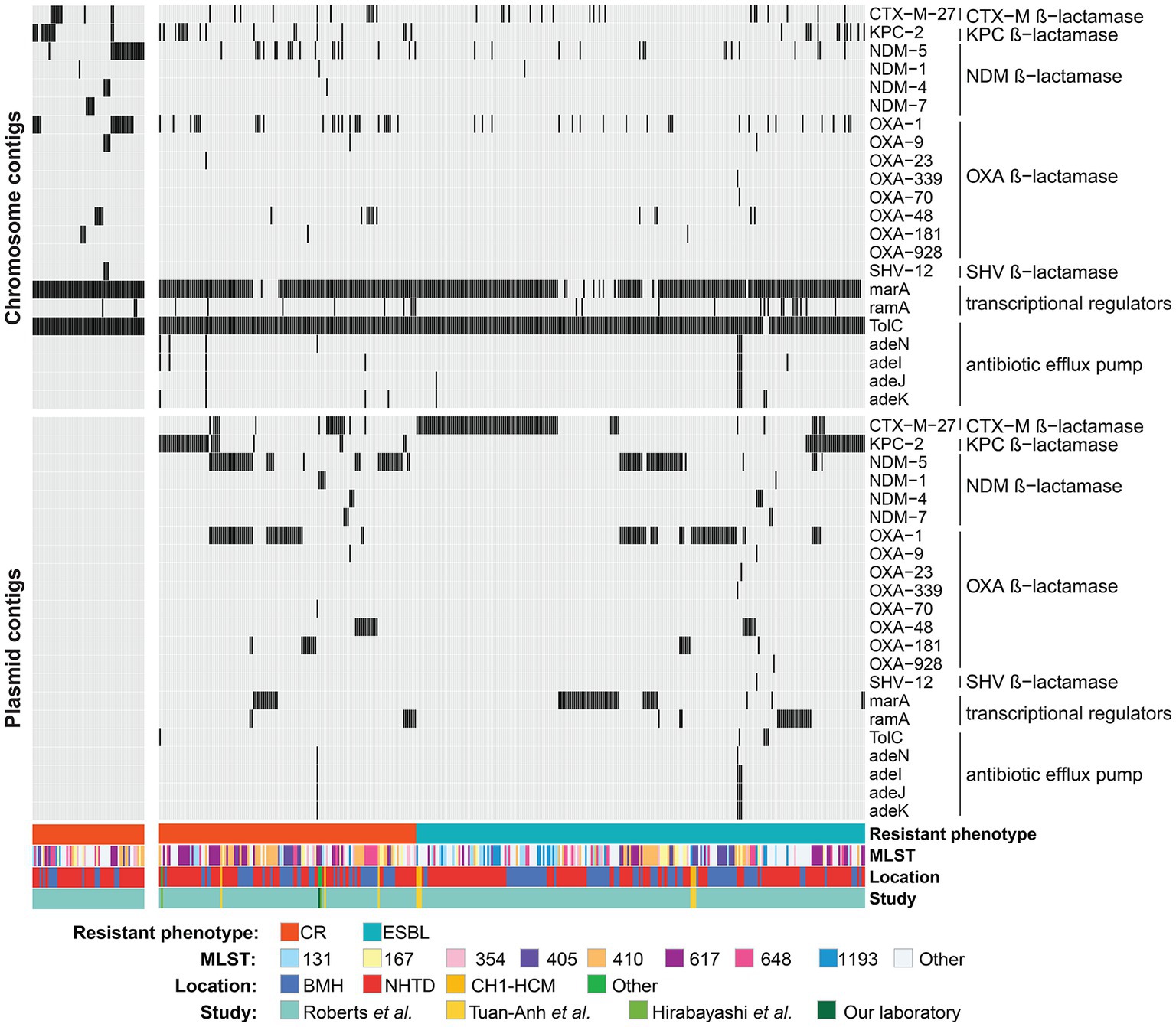
Figure 5. Carbapenem resistance (CR) genes on putative plasmid and chromosome contigs across isolates. All isolates carrying at least one carbapenem resistance gene on putative plasmid/chromosome contigs are shown on the right, whereas CR isolates without any carbapenem resistance genes on plasmid/chromosome contigs are on the left heatmap. Black and gray colors indicate presence and absence of AMR genes, respectively. The color bars at the bottom of the heatmaps indicate the resistance phenotype, sequence type (MLST), location and study from which the sequencing data of the isolates were taken. BMH, Bach Mai Hospital; NHTD, National Hospital for Tropical Diseases; CH1-HCM, Children’s Hospital 1-Ho Chi Minh city.
3.4. Highly conserved structural organization of plasmid-encoded β-lactamase genes
Next, a sequence similarity network was built using 304 putative plasmid contigs that harbored blaKPC, blaNDM and blaOXA genes to obtain a global view of the genetic organization of β-lactamase-encoding genes on plasmids. Plasmid contigs carrying the same β-lactamase-encoding gene shared a high degree of genetic similarity (sequence identity >99% and minimum query coverage of 90%) in pairwise alignments. All contigs carrying different blaNDM genes were grouped into one cluster. Contigs carrying different blaOXA genes showed a great diversity of genetic structures and were organized in four clusters. Moreover, plasmid contigs harboring blaOXA-48 were classified in two sub-clusters. Although the clusters of the plasmid contigs carrying blaOXA-1 and blaKPC-2 did not share any sequence similarity, they were connected through a contig that contained both the blaOXA-1 and blaKPC-2 genes (Figure 6A). The genetic environment surrounding β-lactamase-encoding genes was similar in CR and in ESBL-producing isolates. In agreement with the result of the sequence similarity network, the structural organization of β-lactamase-encoding genes within each cluster was highly conserved across plasmid contigs (Figure 6B). Transposable elements (TEs; including transposons and insertion sequences), which play important roles in the spread of AMR genes, particularly blaKPC, blaNDM and blaOXA, in healthcare settings (Partridge et al., 2018), were often located close to the β-lactamase-encoding genes.
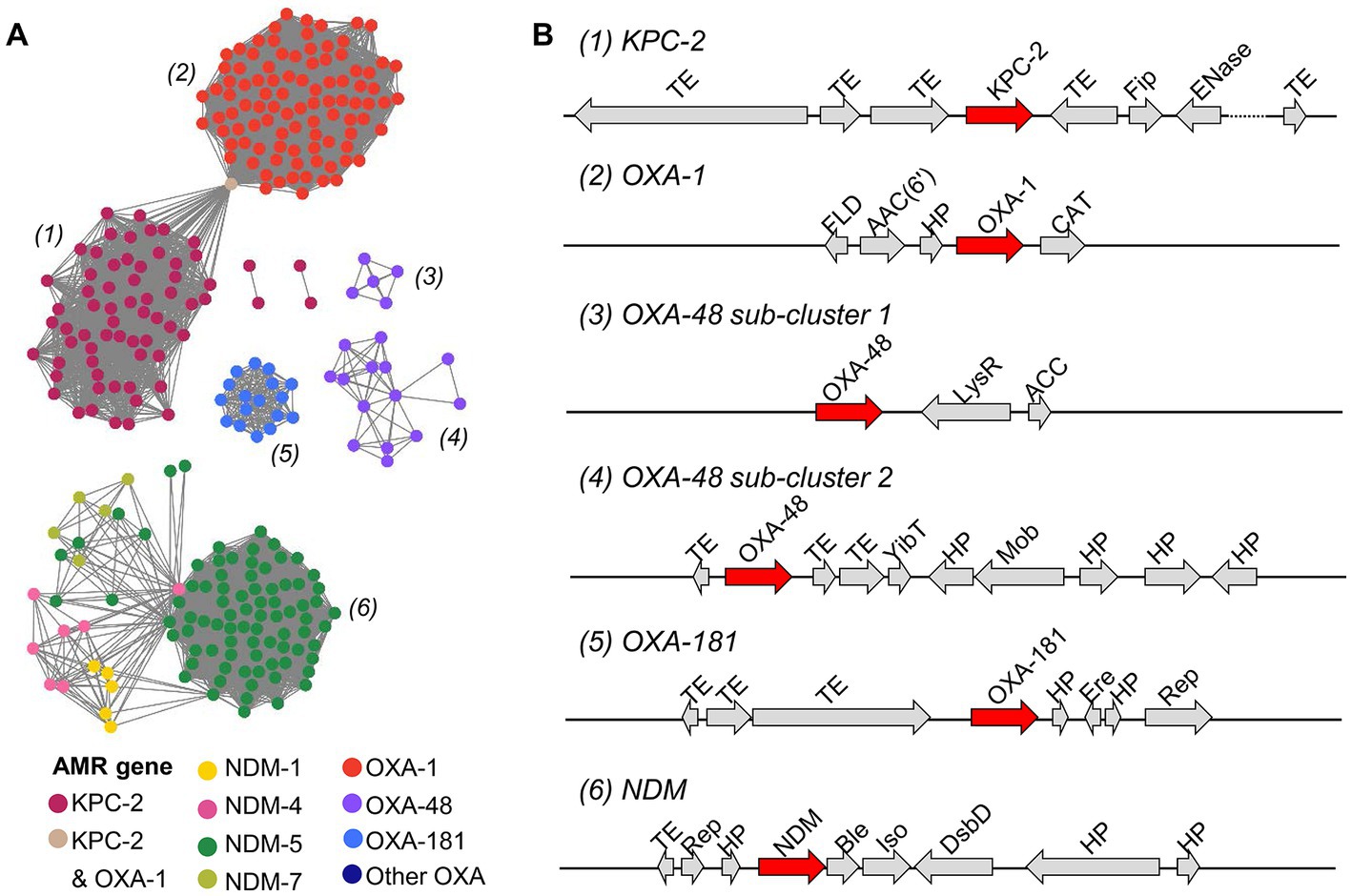
Figure 6. Characterization of putative plasmid contigs containing genes encoding the KPC, NDM and OXA β-lactamases in E. coli isolates. (A) Sequence similarity network of 304 plasmid contigs harboring blaKPC, blaNDM, and blaOXA genes. Pairwise alignments between contigs were done with BLASTn, reciprocal BLAST, and an E-value threshold of 1 × 10−10. Each node represents a plasmid contig and is colored in function of the carried gene. Each plasmid contig cluster is labeled with a number. (B) The most frequent genetic organization of β-lactamase-encoding genes in plasmid contig clusters. Gene products from the RAST annotation are shown. TE, transposable element; Fip, filamentous phage production; ENase, plasmid conjugative transfer endonuclease; FLD, S-(hydroxymethyl) glutathione dehydrogenase; AAC(6′), aminoglycoside 6′ − N − acetyl transferase type Ib − cr; HP, hypothetical protein; CAT, chloramphenicol O-acetyltransferase; LysR, LysR transcriptional regulator; ACC, acetyl-coenzyme A carboxyl transferase, YibT, uncharacterized protein YibT; Mob, mobilization protein; Ere, erythromycin esterase; Rep, DNA replication protein; Ble, bleomycin resistance gene; PRAI, phosphoribosylanthranilate isomerase; DsbD, cytochrome C-type biogenesis protein DsbD.
4. Discussion
The emergence of ESBL-producing and CR Enterobacteriaceae is a public health threat worldwide, including Vietnam, because the latest generation of β-lactam antibiotics would become ineffective against infectious diseases caused by these species. Importantly, AMR Enterobacteriaceae are spreading not only in hospital settings, but also in the communities and in agricultural settings (Carrique-Mas et al., 2020). Dissemination of AMR genes by mobile genetic elements, especially plasmids, is considered the main mechanism of the emergence of drug resistance in bacteria (Partridge et al., 2018). In this study, the plasmid assemblies of 751 multidrug-resistant E. coli from hospitals in Vietnam were generated using short-read sequencing data from four studies in Vietnam. Overall, the studied isolates contained many putative plasmids that harbored a great diversity of AMR genes, particularly carbapenemase-encoding genes.
4.1. Genetic similarity of plasmids
The BLASTn homology search of contigs (assembled with plasmidSPAdes) against the NCBI RefSeq plasmid database and E. coli complete genomes (to determine the closest relative putative plasmid contigs) showed that apart from E. coli origin, putative plasmid contigs were found to be originated from other Enterobacteriaceae species or from the Vibrionaceae and Moraxellaceae families. More importantly, despite their different species/genus origin, theplasmid homologs shared high genomic similarity, which is broadly in agreement with a previous study on the prokaryotic plasmidome (Redondo-Salvo et al., 2020). These findings suggest that genetic exchange events by plasmid conjugation occur frequently within the Enterobacteriaceae family, especially in E. coli species but also between species. The potential plasmidic horizontal transfers between bacterial species, e.g., A. baumanni and E. coli have been reported (Chatterjee et al., 2017; Cooper et al., 2017). This aspect is fundamental to explain the ecology of AMR and to understand the emergence of drug resistance in the different bacterial species of public health interest.
4.2. Molecular epidemiology of CTX-M β-lactamases
ESBL-producing and CR isolates harbored many different AMR genes on their plasmids. The most frequently detected genes encoded antibiotic efflux pumps that eliminate antimicrobial agents from host cells (Kumar et al., 2020). Genes encoding CTX-M β-lactamases also were frequently identified on plasmids of ESBL-producing and CR isolates, as already previously observed in clinical ESBL-producing E. coli isolates (Ma et al., 2005; Ghenea et al., 2022). Strikingly, each CTX-M variant is widespread over a particular geographic area, thus providing epidemiological evidence for the transmission of β-lactamase-encoding genes within and between communities (Canton and Coque, 2006; Rossolini et al., 2008). In the present study, the blaCTX-M-27, blaCTX-M-15 and blaCTX-M-55 genes were widely represented on plasmids of ESBL-producing and CR strains. Previous studies showed that the genes encoding these CTX-M variants are predominant in ESBL-producing E. coli isolates from humans and farm animals in Vietnam (Biedenbach et al., 2014; Nguyen et al., 2019). CTX-M-15 is the dominant CTX-M variant worldwide. Other CTX-M variants (e.g., CTX-M-55, CTX-M-27) that were initially specific to some world regions have now spread globally due to international travel (Bevan et al., 2017; Nakayama et al., 2020). Thus, monitoring the emergence of plasmid-carried blaCTX-M genes, particularly genes encoding new variants, could provide important information for developing strategies to reduce the transmission of AMR genes via plasmids in clinical settings.
4.3. Molecular epidemiology of carbapenemases
Plasmid-carried carbapenemase-encoding genes are considered the main cause of the resistance phenotype in CR E. coli. In our analysis, genes encoding the KPC-2, NDM-5, OXA-1, OXA-48, and OXA-181 β-lactamases were more frequently detected on plasmids in CR than ESBL-producing isolates. The blaKPC gene was first detected on a plasmid of a carbapenem-resistant K. pneumoniae strain in the southern United States in 2001 (Yigit et al., 2001). It has then rapidly spread to other bacterial pathogens, including E. coli, worldwide, including in Vietnam (Petrella et al., 2008; Woodford et al., 2008; Richter et al., 2011; Linh et al., 2021). Similarly, plasmid-carried blaNDM-5 was identified in patients in the United Kingdom and the United States (patient hospitalized in India) in 2016–2017 (Hornsey et al., 2011; Rojas et al., 2017), and in multidrug-resistant clinical E. coli strains in many countries (Pitart et al., 2015; Soliman et al., 2016; Zou et al., 2020). In Vietnam, plasmid-encoded blaNDM-1 was detected in clinical Enterobacteriaceae isolates from a surgical hospital in 2010 (Tran et al., 2015). To our knowledge, Roberts et al. (2022) were the first to describe the presence of blaNDM-5 in clinical isolates in Vietnam. In our study, plasmid-carried blaNDM-1, but not blaNDM-5, was detected in the XP817 and MH-13 isolates collected in 2012–2013, whereas plasmid-carried blaNDM-5 was detected in clinical samples isolated in 2017–2019. This suggests the emergence of plasmid-carried blaNDM-5 in Vietnam approximately in 2017, close to the year of its first report in other countries.
Plasmid-encoded OXA β-lactamases can hydrolyze β-lactam substrates, including carbapenems (Evans and Amyes, 2014). The KPC, NDM and OXA enzymes can mediate carbapenem resistance. Their hydrolytic activity depends on the expression level of the genes encoding these enzymes that is mostly influenced by their genetic environment and copy number (Roth et al., 2011; Evans and Amyes, 2014; Paul et al., 2017). This could explain why some ESBL-producing isolates that harbor these β-lactamase-encoding genes do not show any phenotypic resistance to carbapenems.
The sequence similarity network based on pairwise alignments of plasmid contigs harboring the blaKPC, blaNDM and blaOXA genes captured a high degree of similarity between contigs carrying the same AMR gene. This confirmed the results by Roberts et al. (2022) showing that AMR genes are transmitted within and between hospital settings in Vietnam. The genetic environment around the blaKPC-2 and blaNDM genes is largely consistent with what reported by previous studies (Dortet et al., 2012; Cuzon et al., 2013; Martins et al., 2020). Specifically, our analysis showed that blaKPC-2 is located between two TEs, whereas blaNDM genes are close to the bleomycin resistance gene located downstream of a TE and share the same promoter. Conversely, blaOXA genes were found in several clusters with different environmental structures, as previously reported by Evan & Amyes (Evans and Amyes, 2014). This could be explained by spontaneous DNA rearrangements following acquisition of the blaOXA by horizontal transfer.
4.4. Limitations
Our study, based on short-read whole-genome sequencing data, provided general and predictive information on plasmid profiles in 751 ESBL-producing and CR E. coli isolates. This work has certain limitations. We could only assemble partially plasmids from short-read data using the existing assembly tool and therefore part of plasmid information remains unknown. Another limitation of our study was sample isolation bias since most of the studied strains were isolated from patients of two hospitals in northern Vietnam. Nevertheless, the current study serves as a good foundation for further analysis on a larger scale to have a more detailed look into resistance plasmid epidemiology at the national and global level.
5. Conclusion
This study highlights the high potential of horizontal transfer and dissemination of AMR genes encoded by plasmids in multidrug-resistant E. coli isolates in Vietnam. These putative plasmids could be transferred among different Enterobacteriaceae species and genera. The number of plasmid-carried AMR genes was higher in CR than in ESBL-producing isolates, especially β-lactamase-encoding genes. The genetic environment surrounding carbapenemase-encoding genes in plasmid contigs was highly conserved with the presence of transposable elements that might facilitate their spread in microbial communities.
Data availability statement
The datasets presented in this study can be found in online repositories. The names of the repository/repositories and accession number(s) can be found in the article/Supplementary material.
Ethics statement
Ethical review and approval was not required for the study on human participants in accordance with the local legislation and institutional requirements. Written informed consent for participation was not required for this study in accordance with the national legislation and the institutional requirements.
Author contributions
QHN and TTTT: study concept and design. TTHL, STN, K-OTN, and DVQ: data acquisition. QHN, TTHL, STN, and TTTT: data analysis and interpretation. QHN, TTHL, STN, JH, A-LB, and TTTT: drafting of the manuscript. All authors contributed to the article and approved the submitted version.
Funding
This work was financially supported by funding from the USTH institutional S&T project (USTH.LS.01/21-22). QHN was funded by the Postdoctoral Scholarship Programme of Vingroup Innovation Foundation (VINIF), code VINIF.2022.STS.58.
Acknowledgments
We gratefully acknowledge Masato Suzuki and Aki Hirabayashi for kindly sharing their raw sequencing data. We thank the Laboratory of Antimicrobial Resistance, Department of Bacteriology, National Institute of Hygiene and Epidemiology, for sharing the bacterial strains, and the LMI DRISA, USTH-IRD for scientific support.
Conflict of interest
The authors declare that the research was conducted in the absence of any commercial or financial relationships that could be construed as a potential conflict of interest.
Publisher’s note
All claims expressed in this article are solely those of the authors and do not necessarily represent those of their affiliated organizations, or those of the publisher, the editors and the reviewers. Any product that may be evaluated in this article, or claim that may be made by its manufacturer, is not guaranteed or endorsed by the publisher.
Supplementary material
The Supplementary material for this article can be found online at: https://www.frontiersin.org/articles/10.3389/fmicb.2023.1094119/full#supplementary-material
Abbreviations
AMR, antimicrobial resistance; CR, carbapenem-resistant; MDR, multidrug-resistant; ESBL, extended spectrum beta-lactamase; TE, transposable element.
References
Altschul, S. F., Gish, W., Miller, W., Myers, E. W., and Lipman, D. J. (1990). Basic local alignment search tool. J. Mol. Biol. 215, 403–410. doi: 10.1016/S0022-2836(05)80360-2
Antimicrobial Resistance Collaborators (2022). Global burden of bacterial antimicrobial resistance in 2019: a systematic analysis. Lancet 399, 629–655. doi: 10.1016/S0140-6736(21)02724-0
Antipov, D., Hartwick, N., Shen, M., Raiko, M., Lapidus, A., and Pevzner, P. A. (2016). plasmidSPAdes: assembling plasmids from whole genome sequencing data. Bioinformatics 32, 3380–3387. doi: 10.1093/bioinformatics/btw493
Aziz, R. K., Bartels, D., Best, A. A., DeJongh, M., Disz, T., Edwards, R. A., et al. (2008). The RAST server: rapid annotations using subsystems technology. BMC Genom. 9:75. doi: 10.1186/1471-2164-9-75
Bankevich, A., Nurk, S., Antipov, D., Gurevich, A. A., Dvorkin, M., Kulikov, A. S., et al. (2012). SPAdes: a new genome assembly algorithm and its applications to single-cell sequencing. J. Comput. Biol. 19, 455–477. doi: 10.1089/cmb.2012.0021
Bevan, E. R., Jones, A. M., and Hawkey, P. M. (2017). Global epidemiology of CTX-M beta-lactamases: temporal and geographical shifts in genotype. J. Antimicrob. Chemother. 72, 2145–2155. doi: 10.1093/jac/dkx146
Biedenbach, D. J., Bouchillon, S. K., Hoban, D. J., Hackel, M., Phuong, D. M., Nga, T. T., et al. (2014). Antimicrobial susceptibility and extended-spectrum beta-lactamase rates in aerobic gram-negative bacteria causing intra-abdominal infections in Vietnam: report from the study for monitoring antimicrobial resistance trends (SMART 2009–2011). Diagn. Microbiol. Infect. Dis. 79, 463–467. doi: 10.1016/j.diagmicrobio.2014.05.009
Bolger, A. M., Lohse, M., and Usadel, B. (2014). Trimmomatic: a flexible trimmer for Illumina sequence data. Bioinformatics 30, 2114–2120. doi: 10.1093/bioinformatics/btu170
Camacho, C., Coulouris, G., Avagyan, V., Ma, N., Papadopoulos, J., Bealer, K., et al. (2009). BLAST+: architecture and applications. BMC Bioinform. 10:421. doi: 10.1186/1471-2105-10-421
Canton, R., and Coque, T. M. (2006). The CTX-M beta-lactamase pandemic. Curr. Opin. Microbiol. 9, 466–475. doi: 10.1016/j.mib.2006.08.011
Carrique-Mas, J. J., Choisy, M., Van Cuong, N., Thwaites, G., and Baker, S. (2020). An estimation of total antimicrobial usage in humans and animals in Vietnam. Antimicrob. Resist. Infect. Control 9:16. doi: 10.1186/s13756-019-0671-7
Chatterjee, S., Mondal, A., Mitra, S., and Basu, S. (2017). Acinetobacter baumannii transfers the blaNDM-1 gene via outer membrane vesicles. J. Antimicrob. Chemother. 72, 2201–2207. doi: 10.1093/jac/dkx131
Cooper, R. M., Tsimring, L., and Hasty, J. (2017). Inter-species population dynamics enhance microbial horizontal gene transfer and spread of antibiotic resistance. Elife 6:e25950. doi: 10.7554/eLife.25950
Cosgrove, S. E., and Carmeli, Y. (2003). The impact of antimicrobial resistance on health and economic outcomes. Clin. Infect. Dis. 36, 1433–1437. doi: 10.1086/375081
Costelloe, C., Metcalfe, C., Lovering, A., Mant, D., and Hay, A. D. (2010). Effect of antibiotic prescribing in primary care on antimicrobial resistance in individual patients: systematic review and meta-analysis. BMJ 340:c2096. doi: 10.1136/bmj.c2096
Cuzon, G., Bonnin, R. A., and Nordmann, P. (2013). First identification of novel NDM carbapenemase, NDM-7, in Escherichia coli in France. PLoS One 8:e61322. doi: 10.1371/journal.pone.0061322
Dimitriu, T., Matthews, A. C., and Buckling, A. (2021). Increased copy number couples the evolution of plasmid horizontal transmission and plasmid-encoded antibiotic resistance. Proc. Natl. Acad. Sci. U. S. A. 118:e2107818118. doi: 10.1073/pnas.2107818118
Dortet, L., Nordmann, P., and Poirel, L. (2012). Association of the emerging carbapenemase NDM-1 with a bleomycin resistance protein in Enterobacteriaceae and Acinetobacter baumannii. Antimicrob. Agents Chemother. 56, 1693–1697. doi: 10.1128/AAC.05583-11
EUCAST (2020). Antimicrobial susceptibility testing EUCAST disk diffusion method. Version 8.0. Available at: http://www.eucast.org/ast_of_bacteria/previous_versions_of_documents/
Evans, B. A., and Amyes, S. G. (2014). OXA beta-lactamases. Clin. Microbiol. Rev. 27, 241–263. doi: 10.1128/CMR.00117-13
Ghenea, A. E., Zlatian, O. M., Cristea, O. M., Ungureanu, A., Mititelu, R. R., Balasoiu, A. T., et al. (2022). TEM, CTX-M, SHV genes in ESBL-producing Escherichia coli and Klebsiella pneumoniae isolated from clinical samples in a county clinical emergency hospital Romania-predominance of CTX-M-15. Antibiotics 11:503. doi: 10.3390/antibiotics11040503
Gu, Z., Eils, R., and Schlesner, M. (2016). Complex heatmaps reveal patterns and correlations in multidimensional genomic data. Bioinformatics 32, 2847–2849. doi: 10.1093/bioinformatics/btw313
Hirabayashi, A., Yahara, K., Mitsuhashi, S., Nakagawa, S., Imanishi, T., Ha, V. T. T., et al. (2021). Plasmid analysis of NDM metallo-beta-lactamase-producing enterobacterales isolated in Vietnam. PLoS One 16:e0231119. doi: 10.1371/journal.pone.0231119
Horby, P. W., Pfeiffer, D., and Oshitani, H. (2013). Prospects for emerging infections in east and Southeast Asia 10 years after severe acute respiratory syndrome. Emerg. Infect. Dis. 19, 853–860. doi: 10.3201/eid1906.121783
Hornsey, M., Phee, L., and Wareham, D. W. (2011). A novel variant, NDM-5, of the New Delhi metallo-beta-lactamase in a multidrug-resistant Escherichia coli ST648 isolate recovered from a patient in the United Kingdom. Antimicrob. Agents Chemother. 55, 5952–5954. doi: 10.1128/AAC.05108-11
Jain, C., Rodriguez, R. L., Phillippy, A. M., Konstantinidis, K. T., and Aluru, S. (2018). High throughput ANI analysis of 90K prokaryotic genomes reveals clear species boundaries. Nat. Commun. 9:5114. doi: 10.1038/s41467-018-07641-9
Jia, B., Raphenya, A. R., Alcock, B., Waglechner, N., Guo, P., Tsang, K. K., et al. (2017). CARD 2017: expansion and model-centric curation of the comprehensive antibiotic resistance database. Nucleic Acids Res. 45, D566–D573. doi: 10.1093/nar/gkw1004
Jones, K. E., Patel, N. G., Levy, M. A., Storeygard, A., Balk, D., Gittleman, J. L., et al. (2008). Global trends in emerging infectious diseases. Nature 451, 990–993. doi: 10.1038/nature06536
Kahlmeter, G., Menday, P., and Cars, O. (2003). Non-hospital antimicrobial usage and resistance in community-acquired Escherichia coli urinary tract infection. J. Antimicrob. Chemother. 52, 1005–1010. doi: 10.1093/jac/dkg488
Kumar, S., Lekshmi, M., Parvathi, A., Ojha, M., Wenzel, N., and Varela, M. F. (2020). Functional and structural roles of the major facilitator superfamily bacterial multidrug efflux pumps. Microorganisms 8:266. doi: 10.3390/microorganisms8020266
Li, H. (2013). Seqtk: a fast and lightweight tool for processing FASTA or FASTQ sequences. Available at: https://github.com/lh3/seqtk/
Linh, T. D., Thu, N. H., Shibayama, K., Suzuki, M., Yoshida, L., Thai, P. D., et al. (2021). Expansion of KPC-producing Enterobacterales in four large hospitals in Hanoi, Vietnam. J. Glob. Antimicrob. Resist. 27, 200–211. doi: 10.1016/j.jgar.2021.09.007
Ma, L., Chang, F. Y., Fung, C. P., Chen, T. L., Lin, J. C., Lu, P. L., et al. (2005). Variety of TEM-, SHV-, and CTX-M-type beta-lactamases present in recent clinical isolates of Escherichia coli, Klebsiella pneumoniae, and Enterobacter cloacae from Taiwan. Microb. Drug Resist. 11, 31–39. doi: 10.1089/mdr.2005.11.31
Mao, W., Vu, H., Xie, Z., Chen, W., and Tang, S. (2015). Systematic review on irrational use of medicines in China and Vietnam. PLoS One 10:e0117710. doi: 10.1371/journal.pone.0117710
Martins, W., Nicolas, M. F., Yu, Y., Li, M., Dantas, P., Sands, K., et al. (2020). Clinical and molecular description of a high-copy IncQ1 KPC-2 plasmid harbored by the international ST15 Klebsiella pneumoniae clone. mSphere 5:e00756-20. doi: 10.1128/mSphere.00756-20
McNally, A., Cheng, L., Harris, S. R., and Corander, J. (2013). The evolutionary path to extraintestinal pathogenic, drug-resistant Escherichia coli is marked by drastic reduction in detectable recombination within the core genome. Genome Biol. Evol. 5, 699–710. doi: 10.1093/gbe/evt038
Nakayama, T., Kumeda, Y., Kawahara, R., and Yamamoto, Y. (2020). Quantification and long-term carriage study of human extended-spectrum/AmpC beta-lactamase-producing Escherichia coli after international travel to Vietnam. J. Glob. Antimicrob. Resist. 21, 229–234. doi: 10.1016/j.jgar.2019.11.001
Nguyen, V. T., Jamrozy, D., Matamoros, S., Carrique-Mas, J. J., Ho, H. M., Thai, Q. H., et al. (2019). Limited contribution of non-intensive chicken farming to ESBL-producing Escherichia coli colonization in humans in Vietnam: an epidemiological and genomic analysis. J. Antimicrob. Chemother. 74, 561–570. doi: 10.1093/jac/dky506
Nhung, N. T., Cuong, N. V., Thwaites, G., and Carrique-Mas, J. (2016). Antimicrobial usage and antimicrobial resistance in animal production in Southeast Asia: a review. Antibiotics 5:37. doi: 10.3390/antibiotics5040037
Partridge, S. R., Kwong, S. M., Firth, N., and Jensen, S. O. (2018). Mobile genetic elements associated with antimicrobial resistance. Clin. Microbiol. Rev. 31:e00088-17. doi: 10.1128/CMR.00088-17
Paul, D., Bhattacharjee, A., Bhattacharjee, D., Dhar, D., Maurya, A. P., and Chakravarty, A. (2017). Transcriptional analysis of blaNDM-1 and copy number alteration under carbapenem stress. Antimicrob. Resist. Infect. Control 6:26. doi: 10.1186/s13756-017-0183-2
Petrella, S., Ziental-Gelus, N., Mayer, C., Renard, M., Jarlier, V., and Sougakoff, W. (2008). Genetic and structural insights into the dissemination potential of the extremely broad-spectrum class a beta-lactamase KPC-2 identified in an Escherichia coli strain and an Enterobacter cloacae strain isolated from the same patient in France. Antimicrob. Agents Chemother. 52, 3725–3736. doi: 10.1128/AAC.00163-08
Pitart, C., Sole, M., Roca, I., Roman, A., Moreno, A., Vila, J., et al. (2015). Molecular characterization of blaNDM-5 carried on an IncFII plasmid in an Escherichia coli isolate from a nontraveler patient in Spain. Antimicrob. Agents Chemother. 59, 659–662. doi: 10.1128/AAC.04040-14
R Core Team (2016). R: A language and environment for statistical computing. (Vienna, Austria: R Foundation for Statistical Computing).
Redondo-Salvo, S., Fernandez-Lopez, R., Ruiz, R., Vielva, L., de Toro, M., Rocha, E. P. C., et al. (2020). Pathways for horizontal gene transfer in bacteria revealed by a global map of their plasmids. Nat. Commun. 11:3602. doi: 10.1038/s41467-020-17278-2
Richter, S. N., Frasson, I., Bergo, C., Parisi, S., Cavallaro, A., and Palu, G. (2011). Transfer of KPC-2 Carbapenemase from Klebsiella pneumoniae to Escherichia coli in a patient: first case in Europe. J. Clin. Microbiol. 49, 2040–2042. doi: 10.1128/JCM.00133-11
Roberts, L. W., Hoi, L. T., Khokhar, F. A., Hoa, N. T., Giang, T. V., Bui, C., et al. (2022). Genomic characterisation of multidrug-resistant Escherichia coli, Klebsiella pneumoniae, and Acinetobacter baumannii in two intensive care units in Hanoi, Vietnam: a prospective observational cohort study. Lancet Microbe 3, e857–e866. doi: 10.1016/S2666-5247(22)00181-1
Rojas, L. J., Hujer, A. M., Rudin, S. D., Wright, M. S., Domitrovic, T. N., Marshall, S. H., et al. (2017). NDM-5 and OXA-181 Beta-lactamases, a significant threat continues to spread in the Americas. Antimicrob. Agents Chemother. 61:e00454-17. doi: 10.1128/AAC.00454-17
Rossolini, G. M., D'Andrea, M. M., and Mugnaioli, C. (2008). The spread of CTX-M-type extended-spectrum beta-lactamases. Clin. Microbiol. Infect. 14, 33–41. doi: 10.1111/j.1469-0691.2007.01867.x
Roth, A. L., Kurpiel, P. M., Lister, P. D., and Hanson, N. D. (2011). Bla(KPC) RNA expression correlates with two transcriptional start sites but not always with gene copy number in four genera of gram-negative pathogens. Antimicrob. Agents Chemother. 55, 3936–3938. doi: 10.1128/AAC.01509-10
Seemann, T. (n.d.-a). Abricate Github. Available at: https://github.com/tseeman/abricate/ (Accessed May 12, 2020).
Seemann, T. (n.d.-b). mlst Github. Available at: https://github.com/tseemann/mlst (Accessed October 28, 2022).
Shannon, P., Markiel, A., Ozier, O., Baliga, N. S., Wang, J. T., Ramage, D., et al. (2003). Cytoscape: a software environment for integrated models of biomolecular interaction networks. Genome Res. 13, 2498–2504. doi: 10.1101/gr.1239303
Soliman, A. M., Khalifa, H. O., Ahmed, A. M., Shimamoto, T., and Shimamoto, T. (2016). Emergence of an NDM-5-producing clinical Escherichia coli isolate in Egypt. Int. J. Infect. Dis. 48, 46–48. doi: 10.1016/j.ijid.2016.05.003
Tran, H. H., Ehsani, S., Shibayama, K., Matsui, M., Suzuki, S., Nguyen, M. B., et al. (2015). Common isolation of New Delhi metallo-beta-lactamase 1-producing Enterobacteriaceae in a large surgical hospital in Vietnam. Eur. J. Clin. Microbiol. Infect. Dis. 34, 1247–1254. doi: 10.1007/s10096-015-2345-6
Tran, D. M., Larsson, M., Olson, L., Hoang, N. T. B., Le, N. K., Khu, D. T. K., et al. (2019). High prevalence of colonisation with carbapenem-resistant Enterobacteriaceae among patients admitted to Vietnamese hospitals: risk factors and burden of disease. J. Infect. 79, 115–122. doi: 10.1016/j.jinf.2019.05.013
Tsen, S. D., Fang, S. S., Chen, M. J., Chien, J. Y., Lee, C. C., and Tsen, D. H. (2002). Natural plasmid transformation in Escherichia coli. J. Biomed. Sci. 9, 246–252. doi: 10.1007/BF02256071
Tuan-Anh, T., Tuyen, H. T., Minh Chau, N. N., Toan, N. D., Triet, T. H., Triet, L. M., et al. (2020). Pathogenic Escherichia coli possess elevated growth rates under exposure to sub-inhibitory concentrations of azithromycin. Antibiotics 9:735. doi: 10.3390/antibiotics9110735
von Wintersdorff, C. J., Penders, J., van Niekerk, J. M., Mills, N. D., Majumder, S., van Alphen, L. B., et al. (2016). Dissemination of antimicrobial resistance in microbial ecosystems through horizontal gene transfer. Front. Microbiol. 7:173. doi: 10.3389/fmicb.2016.00173
Woodford, N., Zhang, J., Warner, M., Kaufmann, M. E., Matos, J., Macdonald, A., et al. (2008). Arrival of Klebsiella pneumoniae producing KPC carbapenemase in the United Kingdom. J. Antimicrob. Chemother. 62, 1261–1264. doi: 10.1093/jac/dkn396
Yamasaki, S., Le, T. D., Vien, M. Q., Van Dang, C., and Yamamoto, Y. (2017). Prevalence of extended-spectrum beta-lactamase-producing Escherichia coli and residual antimicrobials in the environment in Vietnam. Anim. Health Res. Rev. 18, 128–135. doi: 10.1017/S1466252317000160
Yigit, H., Queenan, A. M., Anderson, G. J., Domenech-Sanchez, A., Biddle, J. W., Steward, C. D., et al. (2001). Novel carbapenem-hydrolyzing beta-lactamase, KPC-1, from a carbapenem-resistant strain of Klebsiella pneumoniae. Antimicrob. Agents Chemother. 45, 1151–1161. doi: 10.1128/AAC.45.4.1151-1161.2001
Zhang, R., Liu, L., Zhou, H., Chan, E. W., Li, J., Fang, Y., et al. (2017). Nationwide surveillance of clinical Carbapenem-resistant Enterobacteriaceae (CRE) strains in China. EBioMedicine 19, 98–106. doi: 10.1016/j.ebiom.2017.04.032
Keywords: Escherichia coli, putative plasmid, carbapenem resistance, horizontal gene transfer, whole-genome sequencing
Citation: Nguyen QH, Le TTH, Nguyen ST, Nguyen K-OT, Quyen DV, Hayer J, Bañuls A-L and Tran TTT (2023) Large-scale analysis of putative plasmids in clinical multidrug-resistant Escherichia coli isolates from Vietnamese patients. Front. Microbiol. 14:1094119. doi: 10.3389/fmicb.2023.1094119
Edited by:
Qixia Luo, Zhejiang University, ChinaReviewed by:
Amjad Islam Aqib, Cholistan University of Veterinary and Animal Sciences, PakistanTasleem Kausar, Government Sadiq College Women University, Bahawalpur, Pakistan
Copyright © 2023 Nguyen, Le, Nguyen, Nguyen, Dong, Hayer, Bañuls and Tran. This is an open-access article distributed under the terms of the Creative Commons Attribution License (CC BY). The use, distribution or reproduction in other forums is permitted, provided the original author(s) and the copyright owner(s) are credited and that the original publication in this journal is cited, in accordance with accepted academic practice. No use, distribution or reproduction is permitted which does not comply with these terms.
*Correspondence: Tam Thi Thanh Tran, tran-thi-thanh.tam@usth.edu.vn