- 1Department of Fisheries, Faculty of Fisheries and Environmental Sciences, Gorgan University of Agricultural Sciences and Natural Resources, Gorgan, Iran
- 2Inland Waters Aquatics Resources Research Center, Iranian Fisheries Sciences Research Institute, Agricultural Research, Education and Extension Organization, Gorgan, Iran
- 3Department of Aquatic Animal Health, Faculty of Veterinary Medicine, University of Tehran, Tehran, Iran
- 4Infectious Diseases Research Center, Golestan University of Medical Sciences, Gorgan, Iran
- 5Department of Animal and Aquatic Sciences, Faculty of Agriculture, Chiang Mai University, Chiang Mai, Thailand
- 6Functional Feed Innovation Center (FuncFeed), Faculty of Agriculture, Chiang Mai University, Chiang Mai, Thailand
- 7Fish Nutrition Research Laboratory, Animal Production Department Faculty of Agriculture Cairo University, Cairo, Egypt
- 8Animal Biological Product Research Group, Academic Center for Education, Culture and Research (ACECR), Tehran Organization, Tehran, Iran
Probiotics are helpful bacteria that safeguard host animals from harmful pathogens. In fish farming, the primary aim of using probiotics is to preserve or reestablish a balance between pathogenic microorganisms and the native bacteria that constitute the intestinal and skin microbial communities of fish. This study investigates the effects of host-associated probiotic (HAP) vs commercial probiotic (CP) on the growth performance, antioxidant defense and immunity of Caspian whitefish fry. Three hundred whitefish fry (1.15 ± 0.03 g) were randomly divided into five treatments in triplicate. Treatments included feeding with the control group (zero), P. acidilactici as a commercial probiotic (CP) at 6 × 108 CFU g -1, and HA Pediococcus pentosaceus at 106, 107 and 108 CFU g-1 for eight weeks. Dietary HAP and CP did not have significant effects on growth indices compared to the control group (P > 0.05). However, HAP at 107 and 108 CFU g-1 and CP significantly increased protein in whitefish carcasses compared to the control group (P < 0.05). Different levels of HAP and CP had a significant effect on whole-body extract (WBE) lysozyme (LZM) activity (P < 0.05). HAP treatment significantly increased WBE ACH50 and bactericidal activity compared to the control and CP group (P < 0.05). Also, in the case of mucosal immune response, different levels of HAP could significantly increase LZM, total immunoglobulin (Ig), agglutination titer, protease and alkaline phosphatase activity compared to the control group (P < 0.05). Whitefish fed HAP showed a significant increase in the activity of WBE antioxidant parameters (SOD, CAT and GPx) compared to the control group (P < 0.05). Also, feeding with HAP could significantly increase autochthonous LAB levels compared control group (P < 0.05); while the total count of intestinal heterotrophic bacteria was not affected (P > 0.05). Overall, the present study showed HA Pediococcus pentosaceus can be considered as beneficial feed additive for whitefish.
1 Introduction
The growing demand for aquatic (fish and shrimp) as a food source for humans has led fish farmers to shift their production methods towards more intensive and super-intensive systems. This shift aims to boost production levels and offset the reduced availability of wild-caught fish and shrimp (Zhang et al., 2024). Such an intensive culture systems cause stress and drastically increases the risk of disease outbreak (Assefa and Abunna, 2018). On the other hand, antibiotics and chemotherapy have been banned or limited to avoid environmental issues regarding the emergence of muti-drug-resistant bacteria (Liu et al., 2020). In this context, dietary administration of probiotics has been suggested as a promising feed additive alternative to antibiotics.
Traditionally, commercial probiotics have been used in aquaculture (Dawood et al., 2020). However, considering the difference in physiological conditions, intestinal microbial ecology, and the culture environment, they were not always practical. Based on this fact, scientists stressed the isolation and administration of host-associated probiotics (Van Doan et al., 2019; Ringø et al., 2020), which allows them to be better adapted to the host’s specific environment and physiological conditions. It has been reported that dietary administration of HA probiotics offers significant advantages over traditional commercial probiotics (Tarkhani et al., 2019). Their ability to enhance growth, improve immune responses, and enhance antioxidant defense has been well-documented (Van Doan et al., 2019).
Caspian whitefish (Rutilus frisii kutum) is one of the native species of the Caspian Sea and a species of cyprinids (Hoseinifar et al., 2016a). Due to its suitable and excellent meat, this fish has a favorable market and has become one of the most popular fish for fishing (Rahbar et al., 2023). Given the decrease in natural stocks of the species as well as the importance of whitefish in the market, there has been an extensive attempt to breed and culture the species for restocking and cultivation purposes (Tarkhani et al., 2020). It has been reported that probiotics can help to improve larviculture and cultivation of early stages (Hoseinifar et al., 2018). On the other hand, considering the advantages of HA probiotics, the present study was designed to isolate an HA probiotic from adult whitefish to be used in the culture of whitefish fry. Also, to show the potential of the isolated probiotic, we used a common commercial probiotic (with approved beneficial effects on many species) in a separate treatment. Their possible effects on growth performance, systemic and mucosal immune response, antioxidant defense and gut microbiota have been examined.
2 Materials and methods
2.1 Isolation and screening of autochthonous LAB from intestine
Thirty adult Caspian whitefish were captured and immediately transported to the animal science laboratory of GUASNR. The abdominal surfaces were swabbed with 1% iodine solution (before dissection), dissected and the intestines were collected. In the next step, we emptied the intestinal contents and washed the inner surface of the intestine three times using 0.9% NaCl solution. After washing the intestines, the intestines were homogenized with the same solution. In the next step, one mL of homogenized solution was spread on tryptone soy agar (TSA) and De Man, Rogosa and Sharp (MRS) agar and then kept in an incubator at 37°C for 48 hours anaerobically.
2.2 Characteristics of isolated bacteria (phenotypic, biochemical and molecular)
Phenotypic traits such as gram staining, catalase and colony morphology were determined in all the selected isolates as described elsewhere (Tarkhani et al., 2020). Thereafter, bacterial colonies suspected of lactic acid were tested in terms of their ability to grow at different salinity levels and different temperatures (Wu et al., 2012). Also, their bactericidal activities were tested against the two common pathogens Yersinia ruckeri and Aeromonas hydrophila (Ramos et al., 2013) (Table 1). Finally, DNA was extracted using a gram-positive DNA extraction kit, SinaPure TM DNA, SinaClon company, Iran. PCR was performed using primers 27 F (AGAGTTTGATCMTGGCTCAG) and 1492 R (GGTTACCTTGTTACGACTT) according to the program published elsewhere (Lan et al., 2002; Frank et al., 2008), and sequencing was determined on the PCR product by the Sanger method and using a DNA analysis device (Bionair, Seoul, Korea).
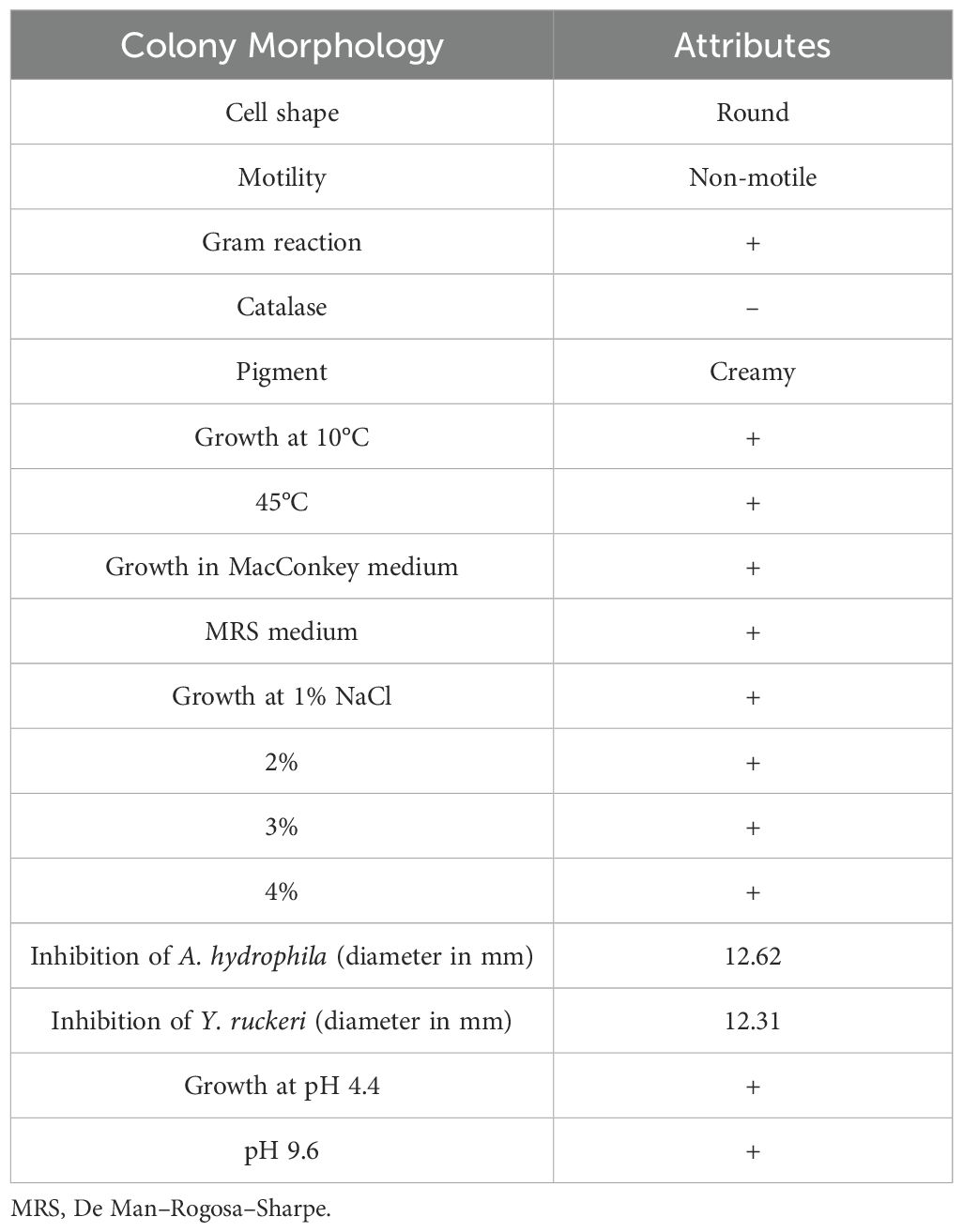
Table 1. Biochemical and phenotypic characteristics of P. pentosaceus isolated from Caspian whitefish.
2.3 In vivo experimental method
Whitefish fry were kindly supplied by the Caspian Sea Sejavel Teleost Fish Research Center (Golestan, Iran) and were quarantined in laboratory conditions for two weeks. After biometry (measurement of weight) and determination of biomass, they were stocked in 200-liter rectangular tanks that were washed and disinfected beforehand. Before starting the experiment, the fish were adapted to the conditions of the tanks and experimental diet (without probiotics) for two weeks. In order to perform in vivo experiments, three hundred R. frisii kutum fry (1.15 ± 0.03 g) were distributed randomly in 15 tanks assigned to five treatments in triplicates: Control group, P. acidilactici (Bactocel) as a commercial probiotic with a concentration of 6 × 108 CFU g-1 (CP), HA P. pentosaceus isolated from intestine of adult whitefish at 106, 107 and 108 CFU g-1 (in lyophilized form). Whitefish fry were fed with experimental diets for eight weeks. The basal diet of whitefish fry was prepared as described in our previous study of (Hoseinifar et al., 2013).
To measure CFU, a sample of the probiotic was diluted several times in a sterile PBS buffer solution. Then, one milliliter of the diluted sample was pipetted into a sterile petri dish and mixed with liquid MRS agar (probiotics growth medium). Petri dishes were incubated at 37°C for 2-3 days until visible colonies were formed. Then, the number of colonies on the plate was counted. This represents the CFU in the original diluted sample. To calculate the total CFU per serving, the number of colonies was multiplied by the dilution factor. Feeding was adjusted based on accurate biometry, 3% of fish body weight. During the eight weeks of rearing, the photoperiod of the fish was set to 12 hours of light and 12 hours of darkness; water temperature was 22.1 ± 0.2°C, dissolved oxygen was 6.89 ± 0.2 mg L -1, pH 7.6 - 7.9. Every two days, 50% of the water in the fish tanks was changed to maintain the proper water quality.
2.4 Growth parameters
At the end of the study, after 24 hours of stopping feed, the fish were weighed, and growth parameters such as final weight (FW), weight gain (WG), feed conversion ratio (FCR) and survival rate (SR) were evaluated (Khanzadeh et al., 2024a). Growth indices are determined based on the following formulas:
2.5 Evaluation of proximate body composition
At the end of the research, in order to investigate the effect of probiotics on the carcass quality indicators, after ensuring the complete emptying of the stomach contents of the fish, three fish were caught from each tank (9 fish from each treatment). Subsequently, the fish were immediately killed by a high dose of clove powder (200 mg/L). Then, the whole body was ground in a meat grinder, and the composition of the fish carcass was evaluated. The measured items included moisture, ash, crude protein and crude fat (AOAC, 1995).
2.6 Sampling (whole-body extract and mucus)
Due to the small size of the white fish and as blood collection was impossible, instead of assessing the immune and antioxidant defense in blood serum, they were measured in WBE, according to the protocol suggested by Holbech et al. (2001). Three fish from each tank (9 fish from each treatment) were taken for extraction. Briefly, the fish tissue was ground into a fine powder using a mortar while submerged in liquid nitrogen to preserve the integrity of the proteins and enzymes. Ice-cold homogenate buffer, twice the weight of the tissue, was added to the powdered tissue and thoroughly mixed until a homogeneous mixture was obtained. The homogenate was then centrifuged at a high speed of 50,000 × g for 60 minutes at a low temperature of 4°C. The supernatant was carefully removed and filtered through glass wool to remove any remaining particulates and stored at -80°C (Holbech et al., 2001).
To collect mucus, nine fish were randomly anesthetized from each treatment with clove powder (150 mg/L) after one day of food deprivation. To obtain mucus, fish were transferred to polyethylene bags (50 mM NaCl) and rubbed for 2 minutes to extract the mucus from the skin epidermis. After transferring the mucus samples to 2 mL tubes, they were centrifuged (1500 g for 10 min at 4°C), and the supernatants were stored at -80°C until immuno-assays were performed (Hoseinifar et al., 2015).
2.7 WBE immunological parameters
At the end of the period, to measure the lysozyme activity, the bacterial wall of Micrococcus luteus (9 mg) was dissolved in distilled water (30 mL). Then, ten μL of the sample and 90 μL of the bacterial wall (suspension) were added to the microplate wells and pipetted several times to mix well. The absorption number was set at a wavelength of 450 nm. Meanwhile, reading minutes were taken as zero and 10 minutes (Ross et al., 2000).
To measure the bactericidal activity, pathogenic Aeromonas hydrophila was used, and this bacterium was cultured in a blood-agar culture medium and then mixed with PBS. The resulting suspension was diluted to show an absorption number of 0.5 at a wavelength of 564 nm. Then, the suspension was serially diluted (1:10) five times, and the five dilutions obtained were used for bactericidal activity. In the following, 50 μL of the sample was mixed with 450 μL of bacterial suspension and incubated at 37°C for one hour. After one hour of incubation, 100 μL of the mentioned mixture was cultured on nutrient agar plates. In the end, the number of grown colonies was counted after 24 hours of incubation (Rao et al., 2006). Also, alternative complement pathway hemolytic activity (ACH50) was measured using the method of Tort et al. (2003).
2.8 Mucosal immunity parameters
According to Tarkhani et al. (2020). a commercial kit (Pars-Azmun Co., Tehran, Iran) was used to determine the activity of the mucus alkaline phosphatase (ALP) enzyme.
Lysozyme activity was measured based on WEB lysosome assay (Section 3.6) (Ross et al., 2000).
The method of Siwicki (1993) was used to measure the total immunoglobulin (Ig) of the mucus (Siwicki, 1993). First, the amount of mucus protein was determined. Then, 12% polyethylene glycol (PEG) was added to the mucus sample to incubate for 2 hours at room temperature (1 mL mucus × 0.1 mL of 12% PEG). After 2 hours, the samples were centrifuged, and the protein concentration in the upper part of the solution was measured again by the Bradford method (5000 × g, 4°C for 10 minutes). The amount of total Ig was calculated by subtracting the protein concentration in the initial sample and the protein concentration after adding PEG.
Mucus protease activity was measured by azocasein hydrolysis method by mixing 100 μL of mucus with 100 μL of 0.7% azocasein solution. This mixture was incubated for 20 hours at 30°C. To stop the reaction, 4.5% trichloroacetic acid (TCA) was added to the solution, and then the supernatant was separated by centrifugation (15,000 rpm × 5 minutes). In the end, the supernatant was mixed with 100 μL of sodium hydroxide (NaOH, 1 N), and the absorbance number was read at 450 nm (Hoseinifar et al., 2016).
With slight modifications, the mucus agglutination titer was measured by the Barnes method. To measure the mucus agglutination titer, the mucus was heated to inactivate the complement proteins (30 minutes at 45°C). Then, mucus (50 μL per well) was mixed with A. hydrophila dissolved in sterile PBS (50 μl) and incubated (22°C, 24 h). After one day of incubation, the bacteria deposited on the bottom of the wells were recorded (Barnes and Ellis, 2004).
2.9 Activity of WBE antioxidant enzymes
CAT activity was measured according to the manufacturer’s protocol (Commercial kit, ZellBio GmbH, Germany). Using ELISA, the absorbance was measured at a wavelength of 405 nm. A unit of CAT activity includes the amount of sample that catalyzes 1 μmol of H2O2 to H2O and O2 in 1 minute (Nedaei et al., 2019).
SOD activity was measured according to the manufacturer’s protocol (Commercial kit, ZellBio GmbH, Germany). Briefly, 10 μL of sample, 10 μL distilled water, 250 μL of reagent 1 and 10 μL of reagent 2 were poured into the microplate and pipetted well. In the following, 200 μL of chromogenic substance was added to the previous composition and absorbance was measured at 420 nm (Rufchaei et al., 2021).
Following Rufchaei et al. (2021), GPx activity was evaluated using a commercial kit (ZellBio GmbH, Germany). In the presence of H2O2, NADPH oxidation was measured at a wavelength of 340 nm, and GPx enzyme activity was reported as mg of protein per minute (Rufchaei et al., 2021).
2.10 Intestinal microbiota analysis
In order to analyze the intestinal microbiota, at the end of the experiment and 15 days after stopping the food containing different levels of probiotics, the number of LAB and the total number of heterotrophic aerobic bacteria in the intestine were evaluated. Three fish were randomly selected from each group (9 fish per treatment) and then killed with a high dose of clove powder. Then, intestinal samples from each treatment were collected and prepared for bacterial culture. After homogenizing the intestines, 100 μL of the homogenized intestines were spread onto plate count agar (PCA) (for total viable heterotrophic aerobic bacteria) and deMan, Rogosa and Sharpe (MRS) (for LAB) agar media (Hoseinifar et al., 2016b). The plates were incubated at a temperature of 25°C for five days, and after five days, the plates that statistically had 30-300 colonies were evaluated and calculated (Azimirad et al., 2016).
2.11 Statistical analysis
For statistical analysis, the Kolmogorov-Smirnove test was used for the standard normality of the data. Then, one-way analysis of variance (ANOVA) and Tukey’s test were used to show significance levels between groups. The data were set as the mean ± standard deviation (SD), and the level (P < 0.05) was set as the significance level.
3 Result
3.1 Evaluation of the characteristics of HAP candidates
At first, forty isolates were identified from the digestive tract of Caspian whitefish (Table 1). Out of 40 isolates, based on biochemical and phenotypic characteristics, only one isolate was selected. The selected isolate was round in appearance, gram-positive, catalase-negative, creamy in color and non-motile. In addition, this isolate was able to grow in MRS and MacConkey media, at 15 or 45°C, in acidic (pH 4.4) and alkaline (pH 9.4) conditions and in 1-4% salinity. One of the key properties of this isolate was to inhibit the growth of Y. ruckeri and A. hydrophila bacteria in-vivo conditions. After biochemical tests, sequencing (16S rRNA) and molecular studies showed that the isolated strain is P. pentosaceus.
3.2 Growth parameters
The results of the effect of HAP and CP on growth indices such as final weight (FW), weight gain (WG), feed conversion ratio (FCR) and survival rate (SR) after eight weeks of feeding are shown in Table 2. The results of this research showed that the HAP (106, 107 and 108 CFU g-1) and CP have no significant effect on the mentioned growth parameters compared to the control group in whitefish fry (P > 0.05) (Table 2). Also, no significant difference was observed between the treatments (P > 0.05).
3.3 Body composition
According to Table 3, after carcass analysis, a significant increase was observed in the amount of whitefish protein in the groups fed with HAP (106, 107 and 108 CFU g-1) and CP probiotics compared to the control group (P < 0.05). Also, there was a significant difference between the CP groups and 106 with 107 and 108 (P < 0.05). The highest amount of protein was observed in the HAP 3 (15.50) group, and the lowest amount was observed in the control (14.39) group. No significant difference was observed in the amount of fat, moisture and ash of whitefish fry carcasses after feeding with HAP and CP probiotics compared to the control group (P > 0.05). Also, no significant difference was observed in the amount of fat, moisture and ash of whitefish fry carcasses between the groups fed with HAP and CP probiotics (P > 0.05).
3.4 Nonspecific immunity parameters
3.4.1 Lysozyme
The results of the effect of HAP and CP probiotics on the WBE lysozyme activity of whitefish fry after eight weeks of feeding are shown in Figure 1A. The results of this research showed that HAP (106, 107 and 108 CFU g-1) and CP probiotics had a significant effect on lysozyme activity compared to the control group in whitefish fry (P < 0.05) (Figure 1A). However, no significant difference was observed between the treatments in different levels of HAP and CP (P > 0.05). It should be noted that the highest level of lysozyme activity was observed in the 107 group (46.96), and the lowest level was observed in the control group (34.1).
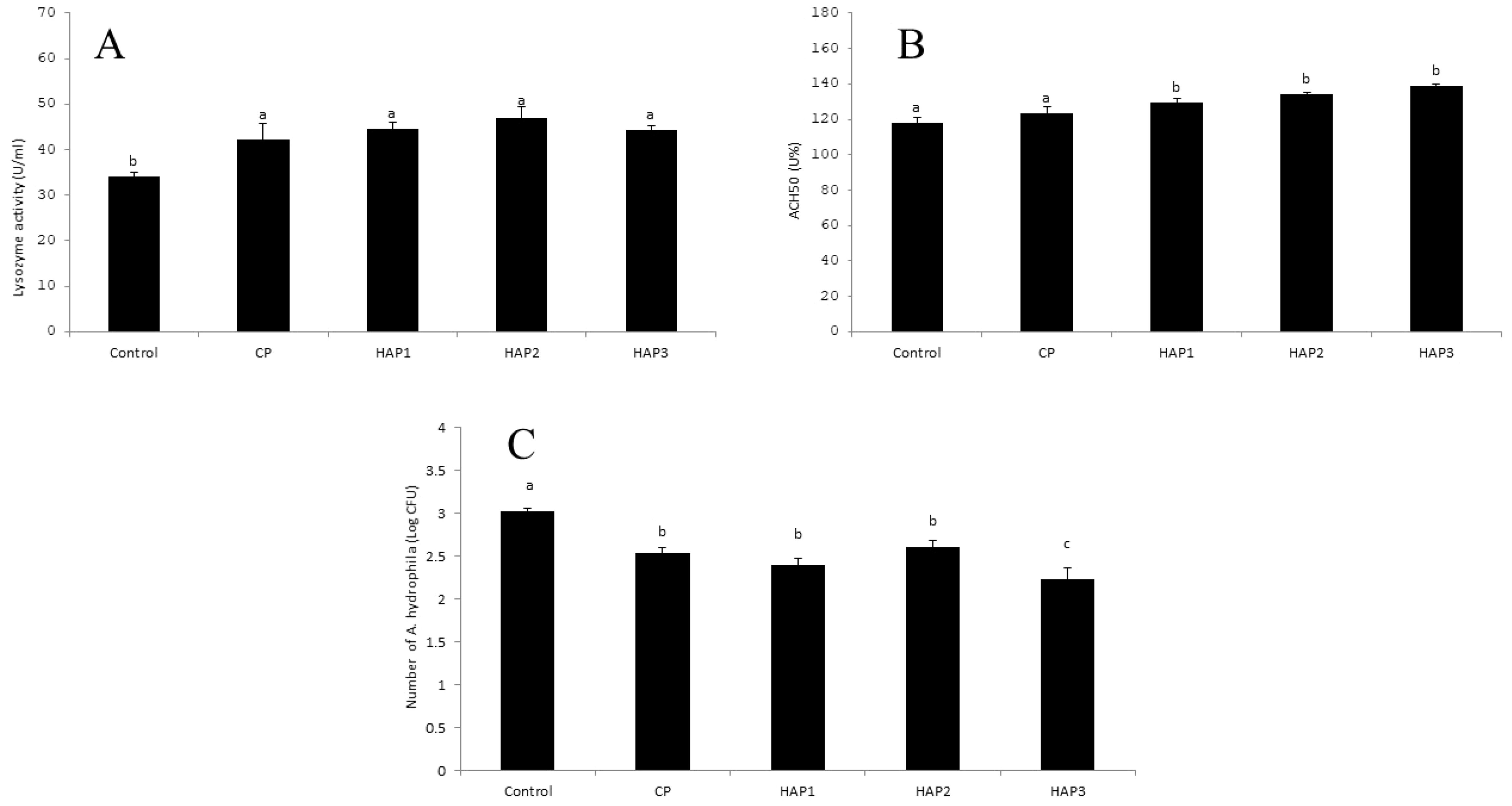
Figure 1. (A–C) The effect of different levels of host-associated vs. commercial probiotics on WBE immune parameters in whitefish fry (n = 9 fish per treatment). Bars assigned with different letters indicate significant differences (P < 0.05). HAP 1: 106, HAP 2: 107, HAP 3: 108 CFU g -1. (A) lysozyme activity, (B) ACH50 activity, (C) bactericidal activity.
3.4.2 ACH50
According to Figure 1B, the effect of different levels of HAP and CP probiotics in the whitefish fry diet on ACH50 showed a significant increase compared to the control and CP groups (P < 0.05). Also, a significant increase was observed between treatments containing HAP probiotics compared to CP (P < 0.05). The highest amount of ACH50 was observed in the HAP 3 treatment, and the lowest amount of ACH50 was observed in the control group.
3.4.3 Bactericidal activity against A. hydrophila
The results of the effect of HAP and CP probiotics on the bactericidal activity against A. hydrophila in whitefish after eight weeks of feeding are shown in Figure 1C. This research showed that HAP (106, 107 and 108 CFU-1) and CP have a significant decrease in the bactericidal activity compared to the control group in whitefish fry (P < 0.05) (Figure 1C). Also, a significant decrease was observed between the CP, HAP 1, and HAP 2 groups with the HAP 3 group (P < 0.05) (Figure 1C). Meanwhile, the highest bactericidal activity of WBE was observed in the control group (3.01), and the lowest bactericidal activity was observed in the HAP 3 group (2.23) (Figure 1C).
3.5 Nonspecific immunity parameters of mucus
3.5.1 Mucus lysozyme
The effect of different levels of HAP and CP in the whitefish fry diet on mucus lysozyme activity was significantly increased compared to the CP and control group (P < 0.05) (Figure 2A). Meanwhile, a significant increase was found among HAP groups compared to CP (P < 0.05). (Figure 2A). The highest amount of mucus lysozyme activity was observed in the HAP 3 group (36.55), and the lowest amount of mucus lysozyme activity was observed in the control group (27.1).
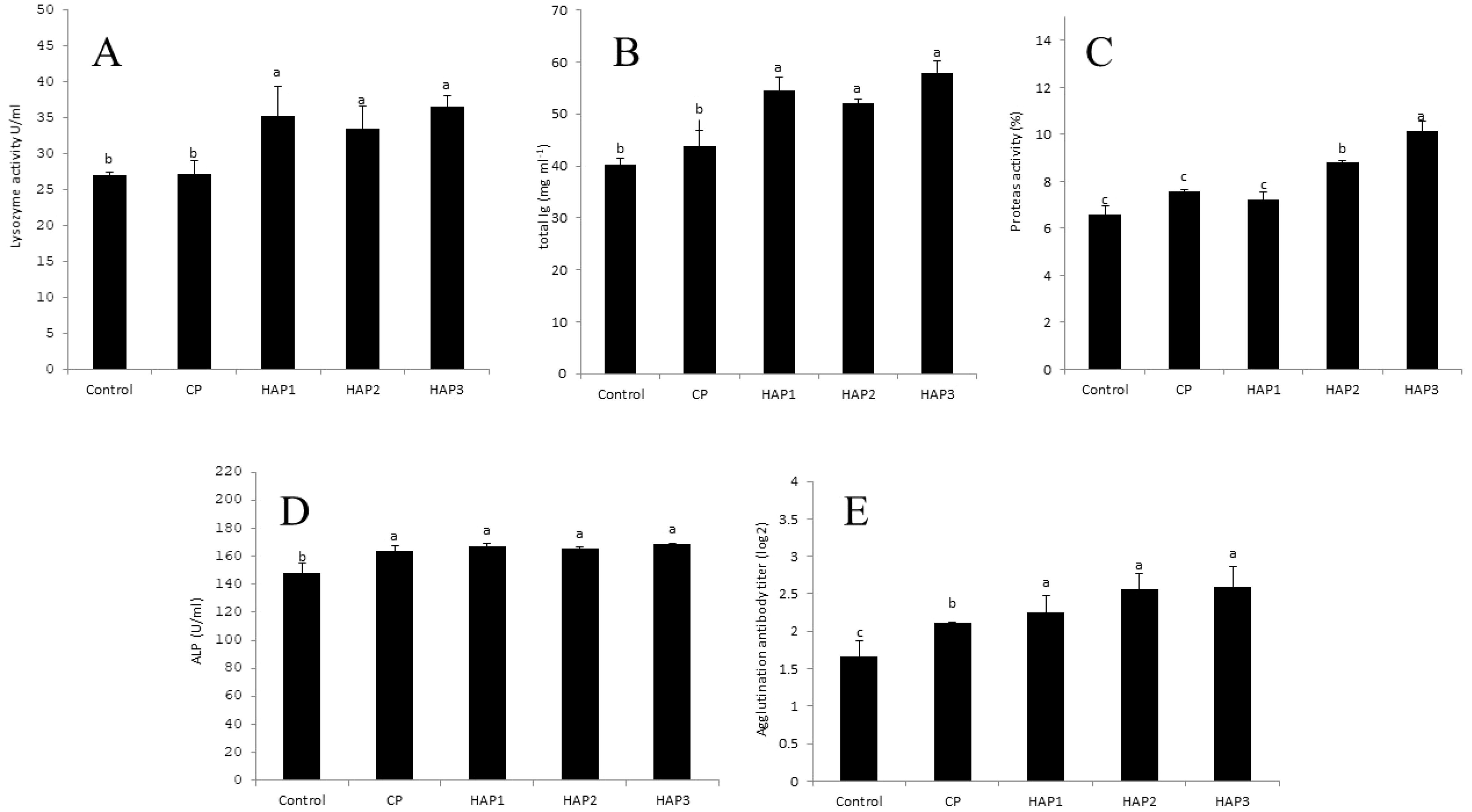
Figure 2. (A–E) The effect of different levels of host-associated vs. commercial probiotics on mucosal immune parameters in whitefish fry (n = 9 fish per treatment). Bars assigned with different letters indicate significant differences (P < 0.05). HAP 1: 106, HAP 2: 107, HAP 3: 108 CFU g -1. (A) mucus lysozyme activity, (B) mucus total Ig activity, (C) mucus protease activity, (D) mucus ALP activity, (E) mucus agglutination titer.
3.5.2 Mucus total Ig
The effect of different levels of HAP and CP in the diet of whitefish fry on the amount of mucus total Ig in the HAP 1, HAP 2 and HAP 3 groups had a significant increase compared to the CP and control group (P < 0.05) (Figure 2B). Meanwhile, a significant increase was found among HAP groups compared to the CP group (P < 0.05) (Figure 2B). The highest amount of mucus Ig was observed in the HAP 3 group (57.95), and the lowest amount of mucus Ig was observed in the control group (35.40).
3.5.3 Mucus protease enzyme activity
The results related to the mucus protease activity in groups fed with HAP and CP probiotics after eight weeks of feeding are presented in Figure 2C. The effect of different levels of HAP and CP probiotics in the diet of whitefish fry on the amount of mucus protease activity in HAP 2 and HAP 3 groups showed a significant increase compared to the control and CP groups (P < 0.05) (Figure 2C). Also, a significant increase was observed between CP and HAP 1 groups with HAP 2 and HAP 3 groups (P < 0.05) (Figure 2C). The highest amount of mucus protease activity was observed in the HAP 3 group (10.13), and the lowest amount of mucus protease activity was observed in the control group (6.57) (Figure 2C).
3.5.4 Mucus alkaline phosphatase activity
According to Figure 2D, the effect of different levels of HAP and CP in the diet of whitefish fry on the mucus ALP activity was significantly increased in HAP 1, HAP 2, HAP 3 and CP groups compared to the control group (P < 0.05), (Figure 2D). However, no significant difference was found among groups in different levels of probiotics (HAP and CP) in ALP activity (P > 0.05) (Figure 2D). The highest amount of mucus ALP was observed in the HAP 3 group (168.5), and the lowest amount of mucus ALP was observed in the control group (148.5) (Figure 2D).
3.5.5 Mucus agglutination titer
The results of mucus agglutination titer against A. hydrophila bacteria in treatments fed with different levels of HAP and CP after eight weeks of feeding are presented in Figure 2E. The results of this study showed a significant increase in the mucus agglutination titer against A. hydrophila in the groups fed with HAP and CP compared to the control group (P < 0.05) (Figure 2E). Also, there was a significant increase between the CP group and HAP 1, HAP 2, and HAP 3 groups (P < 0.05) (Figure 2E). Meanwhile, the highest mucus agglutination titer against A. hydrophila was observed in the HAP 3 group (2.599) and the lowest in the control group (1.655) (Figure 2E).
3.6 Antioxidant enzyme activity
The results related to the antioxidant enzyme activity in the groups fed with different levels of HAP and CP after eight weeks of feeding are presented in Figures 3A–C, respectively. The results of SOD enzyme activity in groups fed with different levels of HAP and CP after eight weeks of feeding are presented in Figure 3A. The effect of different levels of HAP and CP in the diet of whitefish on the SOD enzyme activity in all groups had a significant increase compared to the control group (P < 0.05) (Figure 3A). It should be noted that no significant difference was observed between the groups at different levels of probiotics (HAP and CP) in the amount of SOD enzyme (P > 0.05) (Figure 3A). The highest level of SOD enzyme activity was observed in HAP 3 group (118.5), and the lowest level of SOD enzyme was observed in the control group (95.5).
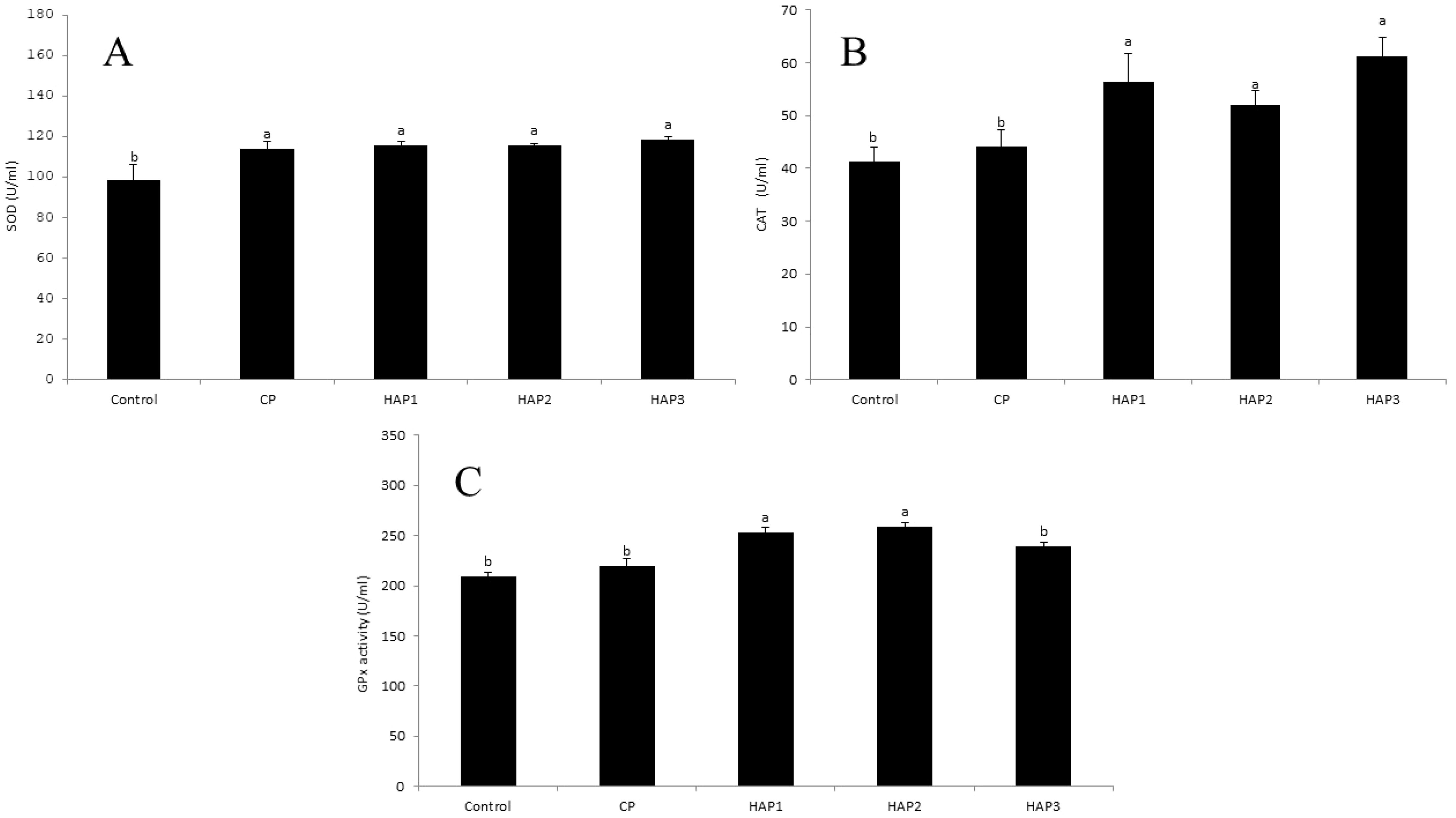
Figure 3. (A–C) The effect of different levels of host-associated vs. commercial probiotics on WBE antioxidant enzymes activity of whitefish fry (n = 9 fish per treatment). Bars assigned with different letters indicate significant differences (P < 0.05). HAP 1: 106, HAP 2: 107, HAP 3: 108 CFU g -1. (A) Superoxide dismutase (SOD), (B) Catalase (CAT), (C) Glutathione peroxidase (GPX).
Also, the effect of different levels of HAP and CP in the diet of whitefish fry on the level of CAT activity after eight weeks in HAP1, HAP2 and HAP3 groups had a significant increase compared to the control and CP groups (P < 0.05) (Figure 3B). In addition, a significant difference was observed between the HAP and CP groups (P < 0.05) (Figure 3B). The highest level of CAT enzyme activity was observed in the HAP 3 group (61.15), and the lowest level of WBE CAT enzyme activity was observed in the control group (41.35).
According to Figure 3C, the effect of different levels of HAP and CP probiotics in the diet of whitefish fry on the activity of GPx enzyme in HAP 1 and HAP 2 groups increased significantly compared to the control and CP groups (P < 0.05). Also, a significant difference was observed between HAP 1 and HAP 2 groups with HAP 3 and CP (P < 0.05) (Figure 3C). It should be noted that the highest amount of GPx enzyme was observed in the HAP 2 group (259), and the lowest amount of GPx enzyme was observed in the control group (209).
3.7 Intestinal microbiota studies
3.7.1 The total bacterial count at the of trial and 15 days after stopping probiotics
Figures 4 and 5 show the number of total gut bacteria counts at the end of the period (end of the 8th week) and 15 days after stopping probiotics using different levels of HAP and CP (Figures 4, 5). As can be seen in Figures 4 and 5, the effect of different levels of HAP and CP probiotics in the whitefish fry diet immediately after stopping food (at the end of the 8th week) and 15 days after stopping probiotics did not have a significant effect on the total number of gut bacteria compared to the control group (P > 0.05) (Figures 4, 5). Meanwhile, no significant difference was observed between the HAP and CP groups (P > 0.05) (Figures 4, 5). In addition, the highest number of bacteria (at the end of the 8th week) in the HAP 2 group (5.29) and the lowest in the control group (4.85) were observed. Also, the highest number of bacteria (15 days after stopping probiotics) in the HAP 3 group (5.12) and the lowest in the control group (4.65) were observed.
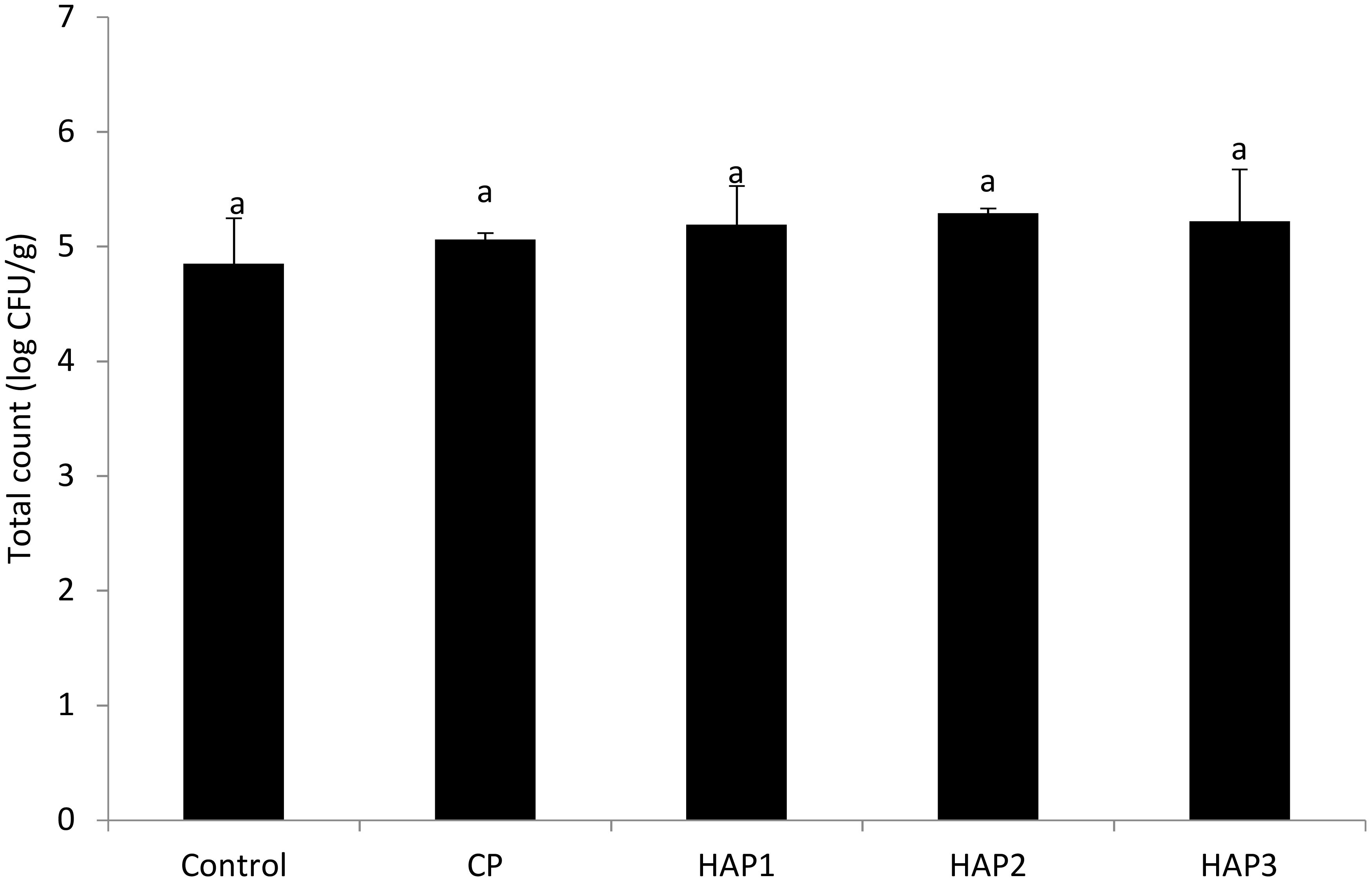
Figure 4. The effect of different levels of host-associated vs. commercial probiotics on the total count of bacteria immediately after stopping food in whitefish fry. HAP, host-associated probiotics; CP, commercial probiotic. (n = 9 fish per treatment). Homogeneous letters in each row indicate no significant differences (P > 0.05). Heterogeneous letters in each row indicate significant differences. HAP 1: 106, HAP 2: 107, HAP 3: 108 CFU g -1.
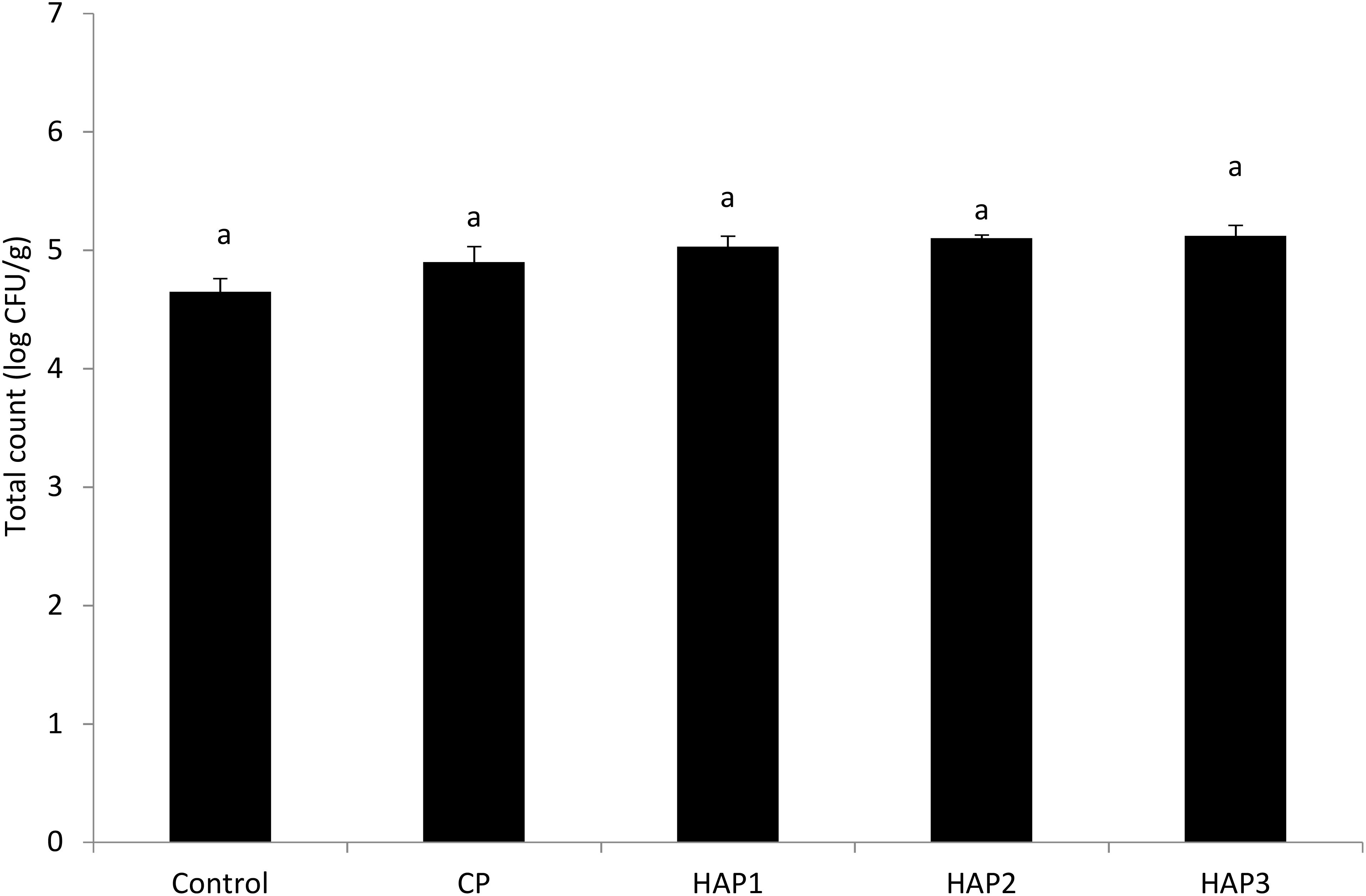
Figure 5. The effect of different levels of host-associated vs. commercial probiotics on the total count of bacteria 15 days after stopping probiotics in whitefish fry. HAP, host-associated probiotics; CP, commercial probiotic. (n = 9 fish per treatment). Bars assigned with the same letters indicate no significant differences (P > 0.05). HAP 1: 106, HAP 2: 107, HAP 3: 108 CFU g -1.
3.7.2 LAB count at the of trial and 15 days after stopping probiotics
Figures 6 and 7 show the number of LAB at the end of the period (end of the 8th week) and 15 days after stopping probiotics using different levels of HAP and CP (Figures 6, 7). As can be seen in Figures 6 and 7, the effect of different levels of HAP and CP probiotics in the whitefish fry diet immediately after stopping food (at the end of the 8th week) and 15 days after stopping probiotics did not have a significant effect on the LAB compared to the control group (P > 0.05) (Figures 6, 7). Also, a significant difference was observed between the HAP and CP groups (P < 0.05) (Figures 6, 7). Also, the highest number of LAB (at the end of the eighth week) was observed in the HAP 3 group (3.41) and the lowest in the control group (1.6). Also, the highest number of LAB (15 days after stopping the probiotic) was observed in the HAP 2 group (3.2) and the lowest in the control group (1.8).
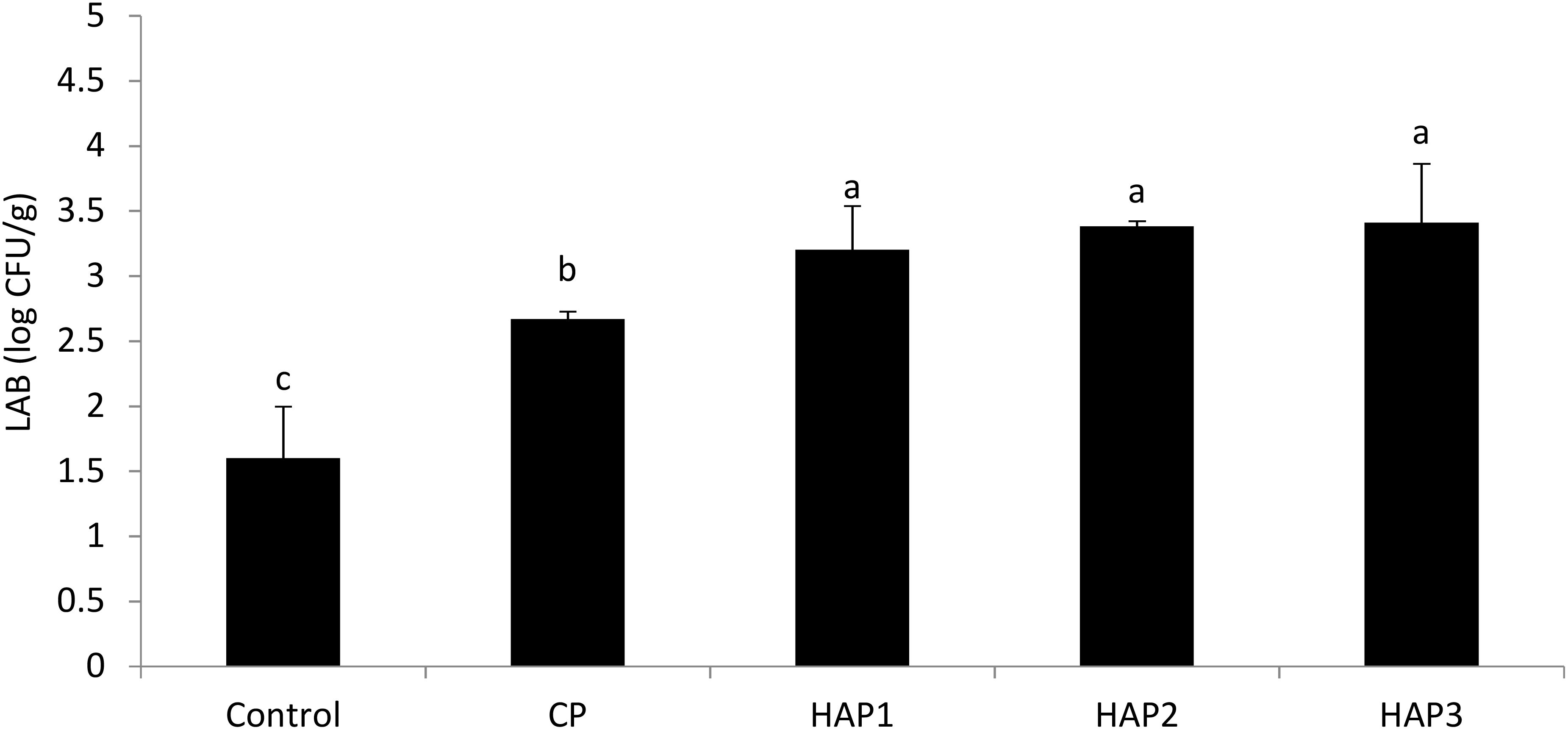
Figure 6. The effect of different levels of host-associated vs. commercial probiotics on the LAB immediately after stopping food in whitefish fry. LAB, lactic acid bacteria; HAP, host-associated probiotics; CP, commercial probiotic. (n = 9 fish per treatment). Bars assigned with the same indicate significant differences (P < 0.05). HAP 1: 106, HAP 2: 107, HAP 3: 108 CFU g -1.
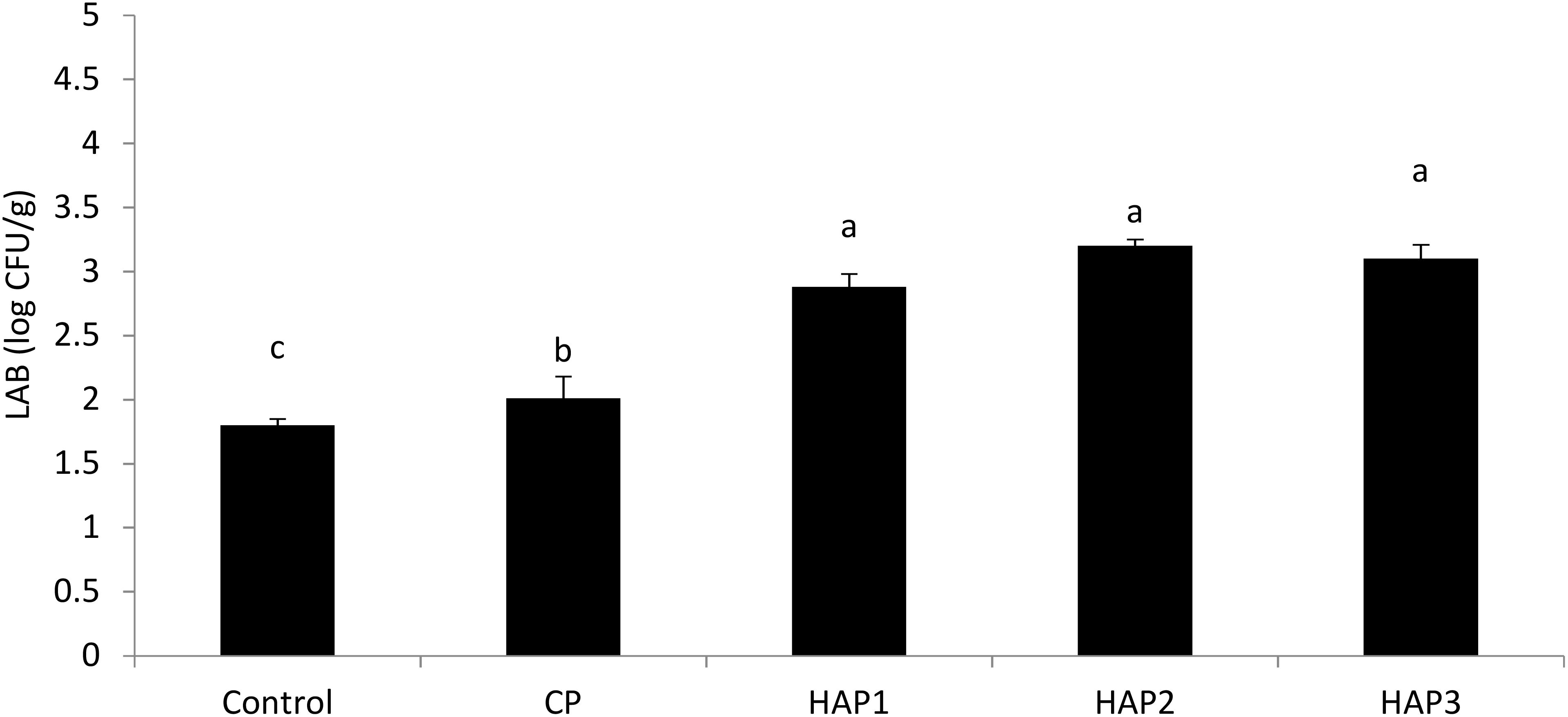
Figure 7. The effect of different levels of host-associated vs. commercial probiotics on the LAB 15 days after stopping probiotics in whitefish fry. LAB, lactic acid bacteria; HAP, host-associated probiotics; CP, commercial probiotic. (n = 9 fish per treatment). Bars assigned with the same indicate significant differences (P < 0.05). HAP 1: 106, HAP 2: 107, HAP 3: 108 CFU g -1.
4 Discussion
Introducing novel beneficial bacterial microorganisms sourced from aquatic animals holds significant promise for enhancing animal health management and performance in commercial aquaculture. Probiotics, besides improving growth performance and feed efficiency, exhibit the potential to fortify the host’s immune system, offering a compelling antibiotic-free solution (Chauhan and Singh, 2019). Within multicellular organisms, intricate microbial communities coexist, having evolved alongside the host over millions of years, each with distinct functions (Rosenberg and Zilber-Rosenberg, 2018). The gut microbiota, a complex and fascinating ecosystem, plays a pivotal role in modulating the host’s physiology, impacting behavior, regulating feeding patterns, influencing digestion and metabolism, and shaping immune responses (Li et al., 2019). The functionality of the gut microbiota is intricately linked to the microbial composition present, a factor influenced in part by environmental conditions and dietary inputs (Suriano et al., 2022). The administration of probiotics can influence gut composition and has demonstrated a multitude of advantageous effects on fish, including enhanced growth and feed efficiency, strengthened immune function, and heightened resistance to pathogens (El-Saadony et al., 2021). In the present study, based on several screening tests, we isolated Pediococcus pentosaceus (P. pentosaceus) from adult white fish. P. pentosaceus is one type of LAB that has been screened from aquatic products, raw plant and animal products, feces, and fermented foods, and there is much evidence that P. pentosaceus is a potential probiotic (Barros et al., 2001).
Growth serves as a critical metric for assessing the health of fish (Khanzadeh et al., 2024b). Providing fish with inadequate food quantities not only impacts their growth and feed utilization efficiency but also raises their susceptibility to diseases. Probiotics, classified as dietary supplements, provide advantages to the host by enhancing nutrient absorption and growth, optimizing enzymatic processes for food breakdown, suppressing opportunistic pathogens, exhibiting anti-mutagenic and anti-cancer properties, and enhancing immune function (Hoseinifar et al., 2018). The findings from this study indicated that the HAP and CP probiotics did not yield a notable impact on growth parameters like FW, percentage of WG, FCR and % SR. Consistent with the outcomes observed in this study, Enterococcus faecium demonstrated no significant influence on growth parameters like FCR and SGR in Nile tilapia (Tachibana et al., 2020). Merrifield et al. (2011) similarly documented that the P. acidilactici probiotic did not result in any notable improvements in growth parameters, including WG, % WG, FCR, and SGR in rainbow trout (Merrifield et al., 2011). In alignment with our findings, the collective impact of four probiotics (Bacillus amyloliquefaciens, Streptococcus faecalis, Lactococcus plantarum, and Bacillus mesentericus(on growth parameters in amberjack (Seriola dumerili) did not yield a significant distinction (Shadrack et al., 2023). In contrast to the outcomes derived from this research, a study isolating probiotics (B. amyloliquefaciens, B. subtilis, B. megaterium) from Labeo rohita demonstrated a notable impact on growth parameters (Saravanan et al., 2021). Variations in the researcher’s findings could stem from differences in the species being bred, their size, age, breeding duration, environmental and hygienic conditions, physiological traits, feeding habits, raw material composition and quality in feed preparation, formulations, probiotic purity, feed composition, dosage, method of supplement incorporation, and potentially unique microbial flora capable of utilizing it as a substrate.
It is widely acknowledged that body composition serves as a valuable indicator of the physiological condition of fish despite the fact that its measurement can be somewhat time-consuming (Einen and Thomassen, 1998). The water percentage derived from proximate analysis serves as a reliable indicator of the relative energy, protein, and lipid content. A lower water content is typically associated with higher lipid and protein levels, signifying a higher energy density in the fish (Ali et al., 2005; Ahmed et al., 2022). Based on the carcass analysis, the groups fed varying levels of HAP and CP probiotics exhibited a significant increase in carcass protein content compared to the control group. However, no significant differences were observed in other carcass components, such as fat, moisture, and ash between the HAP and CP groups compared to the control group. Consistent with our findings, the combined effect of four probiotics, (B. amyloliquefaciens, S. faecalis, L. plantarum, and B. mesentericus) on carcass components such as ash, moisture, and fat in amberjack (S. dumerili) did not make a significant difference (Shadrack et al., 2023). Similarly, the P. acidilactici probiotic did not exhibit a significant effect on carcass composition, including ash, moisture, and fat content, in rainbow trout (Merrifield et al., 2011). Mocanu et al. (2022) studied the impact of Lactobacillus acidophilus and Saccharomyces boulardii probiotics, both individually and in combination, on Siberian sturgeon (Acipenser baerii). Their findings revealed that these probiotics have no significant effect on the protein, fat, moisture, ash, and carbohydrate composition of the carcass (Mocanu et al., 2022). In a separate study, the individual and combined effects of B. cereus and Geotrichum candidum probiotics were examined in Rohu. The results indicated that these probiotics led to a significant increase in carcass compounds such as protein and fat. However, no significant impact was observed on carcass ash content (Ghori et al., 2022). The type of probiotic strain, method of application, rearing conditions, and fish species are crucial factors that can significantly influence the effectiveness of probiotics on carcass composition in aquaculture. Additionally, interactions between probiotics and the fish’s gut microbiota, as well as the overall health and physiological status of the fish, can also influence the outcomes on carcass composition. Further research is needed to better understand the mechanisms underlying the lack of significant effects of probiotics on fish carcass composition in certain studies.
The initial defense mechanism in fish is the nonspecific immune response, which is vital for supporting the secondary or specific immune system and maintaining body balance. Lysozyme is highly regarded and commonly used as a critical indicator of immune response because of its significant association with leukocytes. Lysozyme is generated by macrophages and triggered by diverse immune signals, such as pathogenic elements (Erfanmanesh et al., 2024). Feeding whitefish with varying concentrations of HAP probiotics (106, 107, and 108) and CP did not lead to a significant increase or decrease in lysozyme activity in WBE. Consistent with the findings of this study, Sepherfar et al. reported that the probiotic P. acidilactici does not have a significant impact on lysozyme activity in goldfish (Carassius auratus) (Sepehrfar et al., 2019). Similarly, in another study, the combination of probiotics (B. amyloliquefaciens 1 × 108 cfu/g, S. faecalis 2 × 108 cfu/g, L. plantarum 8 × 107 cfu/g, and B. mesentericus 2 × 104 cfu/g) with feed did not show a significant impact on plasma lysozyme levels in amberjack (S. dumerili) (Shadrack et al., 2023). As mentioned, Different factors like the gender and species of fish, the duration of the breeding period, the specific probiotic type, and its concentrations can influence the effectiveness of immune responses.
The complement system is a crucial component of the immune system in bony fish, comprising numerous proteins and activated through three pathways: classical, alternative, and lectin. These pathways converge to facilitate the direct destruction of microorganisms (Aoki et al., 2008). In the current study, administering HAP probiotics at concentrations of 106, 107, and 108, as well as CP probiotics, led to a significant increase in lysozyme activity in WBE in the groups treated with HAP compared to those receiving CP and the control group in whitefish. The enhancement of immune system efficiency, including the complement system, resulting from probiotic consumption, as observed in the current study, can be linked to alterations in intestinal microbiota. Countless studies have documented the beneficial and substantial impact of probiotics derived from the host on the complement system in aquatics. For instance, L. plantarum in L. rohita (Giri et al., 2013), B. amyloliquefaciens in striped catfish (Thy et al., 2017), B. licheniformis in Oreochromis mossambicus (Gobi et al., 2018), and Bacillus spp. in Nile tilapia (Gobi et al., 2018), they caused a notable enhancement of complement system activity. This change may lead to an increase in lactic acid bacteria and the Bacillus genus, known for their cell walls containing immune-stimulating components like lipopolysaccharides (Hoseinifar et al., 2015).
In the current study, the impact of varying levels of HAP and CP probiotics on the bactericidal activity of WBE against A. hydrophila was significantly decreased compared to the control group. This reduction underscores the potent bactericidal properties exhibited by the mentioned probiotics. Parallel with our findings, a recent study investigated the probiotic Bacillus licheniformis as a bactericide in rainbow trout. The results demonstrated that this probiotic robust bactericidal activity against Streptococcus agalactiae in rainbow trout (Taherpour et al., 2023). Additionally, Aspergillus oryzae was employed as a probiotic in Nile tilapia. The findings revealed that this probiotic led to a notable increase in bactericidal activity against A. hydrophila (Dawood et al., 2020). The bactericidal activity serves as a nonspecific immune response that hinders the growth of invading microorganisms, indicating the presence of proteins like complement, acute phase proteins, lysozyme, transferrins, and antiproteases in fish. Neutrophils and macrophages, functioning as phagocytic cells, play a crucial and pivotal role in antibacterial defense by engulfing and destroying bacteria through the production of active oxygen species such as superoxide anion, hydrogen peroxide, and hydroxyl radicals during the respiratory burst (Akhter et al., 2015).
The mucous surfaces of the skin serve as an initial physical barrier that pathogens encounter. Fish, being immersed in an aquatic environment, face continuous exposure to potential pathogens and harmful elements. Consequently, the skin plays a crucial role in the primary defense against pathogen invasion (Picchietti et al., 2021). In the present study, the administration of HAP probiotics at concentrations of 106, 107, and 108 CFU g-1 resulted in a significant enhancement of lysozyme activity in the skin mucus of whitefish compared to the group receiving CP and the control group. Parallel to our results, a study investigated the probiotic B. subtilis at a concentration of 1.4 ×108 CFU g-1 in Paralichthys olivaceus over 30 days. The results demonstrated a notable and positive impact of this probiotic on liver lysozyme activity (Gao et al., 2024).
Furthermore, probiotics derived from the mucus of Labeo calbasu, particularly B. cereus and B. albus, increased serum lysozyme activity (Bhatnagar and Rathi, 2023). Yousefi et al. (2023) reported that lactobacillus helveticus, when administered as a probiotic, leads to an increase in mucus lysozyme activity in common carp (Yousefi et al., 2023). Indeed, the activity levels of the lysozyme enzyme in the skin mucus can vary significantly among different fish species. This variability is influenced by the specific fish species under study and the environmental conditions they experience. For instance, a study conducted by Fast et al. (2002) showed that Atlantic salmon (Salmo salar) raised in freshwater had higher lysozyme activity in their skin mucus compared to conspecifics cultured in seawater (Fast et al., 2002). The administration of HAP probiotics at concentrations of 106, 107, and 108 CFU g-1 led to a significant enhancement of total Ig activity in the skin mucus of whitefish compared to the group receiving CP and the control group. Several reports indicate a substantial increase in the Ig levels of both total mucus and serum when probiotics are used, which will be discussed further. In a study, an increase in total mucus Ig levels was reported by L. helveticus as a probiotic in common carp (Yousefi et al., 2023). A combination of various commercial probiotics, such as B. subtilis, E. faecium, L. plantarum, and S. cerevisiae, resulted in a notable increase in total serum Ig levels in Pangasianodon hypophthalmus (Abdel-Latif et al., 2023). In another study, five bacterial strains (B. megaterium, B. subtilis, E. faecalis, B. velezensis, and B. siamensis) were isolated as probiotics from the Nibea albiflora and then administered to the same species. The results showed that B. megaterium and B. velezensis did not affect IgM levels, while B. subtilis and E. faecalis caused a significant decrease in mucus IgM. In contrast, B. siamensis led to a notable increase in mucus IgM levels (Ding et al., 2023). The findings of our study align with those of other research, demonstrating that the probiotic isolated from whitefish was able to stimulate mucosal immune factors, including total Ig.
Proteases are an integral component of the active factors present in fish mucus. These enzymes play a crucial role in the specific immune system of fish and have direct effects on pathogens during tissue damage and invasion by pathogenic agents. The secretion of proteases changes the composition of the fish skin mucus and ultimately increases its viscosity. The heightened viscosity leads to the entrapment of pathogenic agents, aiding in their removal from the surface of the fish’s body (Hussain and Sachan, 2023). The results of this study demonstrated that the activity of the mucus protease enzyme was significantly higher in the HAP 2 and HAP 3 groups compared to the control group and group receiving CP in whitefish. In accordance with the findings of this study, Bacillus sp. isolated from the intestine of Rhynchocypris lagowskii fish was found to enhance the activity of the mucin protease enzyme (Elsadek et al., 2023). In another study, Akbari et al. (2021) reported that the probiotic E. casseliflavus increased the mucus protease enzyme activity in common carp at varying levels (Akbari et al., 2021).
Additionally, in another study, Mohammadi et al. reported that the potential probiotic L. plantarum had a positive effect, leading to a significant increase in mucus protease enzyme activity in Nile tilapia (Mohammadi et al., 2020). The changes observed in mucus protease activity due to immune stimulation, infection, and stress highlight the crucial importance of proteases in mucosal immunity. Furthermore, it has been suggested that proteases play a more significant role compared to other enzymes involved in fish mucus immunity (Loganathan et al., 2013).
The ALP enzyme is recognized as an antibacterial agent because of its hydrolytic activity. Additionally, it plays a significant role in wound healing, stress responses, and combating parasitic infections in various organisms (Ross et al., 2000). This study found that different concentrations of HAP and CP probiotics significantly increased the activity of the ALP enzyme in whitefish mucus compared to the control group. Consistent with the findings of this study, the probiotic L. acidophilus was shown to enhance the activity of the alkaline phosphatase enzyme in the diet of tiger barb (Roosta and Hoseinifar, 2016). An increase in ALP activity has been documented following the administration of Saccharomyces cerevisiae probiotic in rainbow trout (Heidarieh et al., 2013) and L. acidophilus in swordtail (Hoseinifar et al., 2015).
The findings of this study demonstrate a notable increase in mucus agglutination titer against A. hydrophila in the groups that were fed with HAP and CP probiotics compared to the control group. In parallel with the findings of the current study, the increase in plasma agglutination titer using probiotic Bacillus spp. was reported in Nile tilapia post-challenge with S. agalactiae (Santos et al., 2023). Additionally, in another study, a significant increase in agglutination titer was observed in Nile tilapia after consuming probiotics S. cerevisiae and Bacillus sp, using inactive S. agalactiae (de Moraes et al., 2022).
Antioxidant enzymes serve as indicators of the body’s antioxidant system health, showcasing their ability to neutralize oxygen-free radicals and safeguard fish tissue from oxidative harm (Shan et al., 2019). In the present study, the use of varying concentrations of HAP and CP probiotics led to a significant increase in Superoxide Dismutase (SOD) enzyme activity compared to the control group. Additionally, different levels of HAP probiotics resulted in a notable rise in WBE GPx and CAT activity compared to the CP and control groups. Consistent with the findings of this study, the addition of L. acidophilus as a probiotic to the diet of Nile tilapia led to a significant increase in the activity of antioxidant enzymes such as SOD and CAT (Hassaan et al., 2021). In a separate study, a blend of powdered probiotics containing B. subtilis, E. faecium, L. plantarum, and S. cerevisiae at concentrations of 0.5, 1, and 1.5 g kg-1 demonstrated an enhancement in the activity of antioxidant markers, including SOD, CAT, and GPx in P. hypophthalmus fingerlings (Abdel-Latif et al., 2023). Kuebutornye et al. (2020) found that the presence of three Bacillus species (B. subtilis, B. amyloliquefaciens, B. velezensis) in Nile tilapia resulted in a notable elevation of antioxidant parameters like SOD and CAT levels in both the mucus and intestine of the fish (Kuebutornye et al., 2020). SOD, CAT, and GPx play crucial roles in the antioxidant defense mechanism. Elevated activity of these enzymes signifies the suppression of free radical actions and enhancement of the oxidative balance within the fish organism.
The fish intestine harbors a diverse array of bacterial species constituting the intestinal microbiota. While lactic acid bacteria represent a minor portion of this microbiota in fish, they are associated with various beneficial effects. Nowadays, there is a growing focus on enhancing the abundance of these bacteria in the intestinal microbiota through the administration of diverse probiotics. Prior research has demonstrated an augmentation in lactic acid bacteria populations in the intestine post oral probiotic supplementation (Azimirad et al., 2016). The current research revealed that HAP and CP probiotics did not have a significant effect on the total number of intestinal bacteria immediately post-stopping food and 15 days post-stopping food compared to the control group.
Nevertheless, these probiotics resulted in a significant increase in the population of LAB in the intestine compared to the control group, both immediately post-stopping food and 15 days post-stopping food. Consistent with the findings of this study, the isolation of five probiotics (E. xiangfangensis, Pseudomonas stutzeri, B. subtilis, Citrobacter freundii, and P. aeruginosa) from barb (Barbonymus gonionotus) resulted in an elevation of intestinal LAB numbers. However, these probiotics did not impact the total count of intestinal bacteria (Salam et al., 2021). Tarkhani et al. (2019) isolated the probiotic E. faecium from the Rutilus rutilus intestine and studied its impact on the intestinal bacterial population. Their findings revealed that E. faecium and P. acidilactici probiotics significantly increased both the total count of intestinal bacteria and the population of LAB in fish.
Additionally, they observed a significant increase in both total intestinal bacteria and LAB numbers 15 days after discontinuing the administration of these probiotics compared to the control group (Tarkhani et al., 2020). In another study, the impact of various probiotic species, including Lactobacillus spp. and Bacillus spp., was evaluated on the Indian carp (Cirrhinus mrigala). The results indicated that these probiotics led to a significant elevation in the total count of beneficial intestinal bacteria and the population of LAB in the fish (Hossain et al., 2022). Given the requirement for bacteria to compete for binding sites within the digestive system, coupled with the limited availability of these sites, the utilization of probiotic bacteria can lead to their dominance. Through effective competition and occupation of binding sites, probiotic bacteria can establish themselves in the digestive system, thereby impeding the colonization of other bacteria to some extent.
5 Conclusion
This study revealed that dietary supplementation with P. pentosaceus, isolated from adult Caspian whitefish, significantly enhanced beneficial intestinal bacteria (LAB), strengthened the mucosal immune system, and increased antioxidant enzyme activity in whitefish fry, demonstrating greater efficacy compared to the commercial strain P. acidilactici. Furthermore, the CP strain and HAP did not influence growth parameters, carcass proximate analysis (except for protein), and lysozyme WBE levels. Collectively, these findings indicate that utilizing HAP yields superior outcomes. Consequently, it is recommended for the aquaculture industry to prioritize the development of probiotics isolated from the autochthonous themselves, rather than employing commercial probiotics or nonspecific sources that may have vastly different environmental requirements and conditions.
Data availability statement
The raw data supporting the conclusions of this article will be made available by the authors, without undue reservation.
Ethics statement
All experiments were performed following the protocol approved by the ethics committee of the faculty of sciences of the University of Tehran (357; 8 November 2000). The study was conducted in accordance with the local legislation and institutional requirements.
Author contributions
SHH: Conceptualization, Data curation, Formal analysis, Funding acquisition, Project administration, Supervision, Validation, Visualization, Writing - original draft, Writing - review & editing. SMH: Investigation, Methodology, Writing - original draft. ATM: Conceptualization, Formal analysis, Writing - original draft. MG: Investigation, Methodology, Writing - original draft. HS-A: Methodology, Writing - original draft. HV: Conceptualization, Formal analysis, Writing - original draft. EE-H: Data curation, Writing - original draft. RS: Investigation, Methodology, Writing - original draft. MK: Resources, Software, Writing - original draft, Writing - review & editing.
Funding
The author(s) declare financial support was received for the research, authorship, and/or publication of this article. This research was funded by Center for International Scientific Studies and Collaboration.
Acknowledgments
This work has been supported by The Center For International Scientific Studies & Collaboration (CISSC), Ministry of Science Research and Technology.
Conflict of interest
The authors declare that the research was conducted in the absence of any commercial or financial relationships that could be construed as a potential conflict of interest.
The author(s) declared that they were an editorial board member of Frontiers, at the time of submission. This had no impact on the peer review process and the final decision.
Publisher’s note
All claims expressed in this article are solely those of the authors and do not necessarily represent those of their affiliated organizations, or those of the publisher, the editors and the reviewers. Any product that may be evaluated in this article, or claim that may be made by its manufacturer, is not guaranteed or endorsed by the publisher.
References
Abdel-Latif H. M. R., Chaklader M. R., Shukry M., Ahmed H. A., Khallaf M. A. (2023). A multispecies probiotic modulates growth, digestive enzymes, immunity, hepatic antioxidant activity, and disease resistance of Pangasianodon hypophthalmus fingerlings. Aquaculture 563, 738948. doi: 10.1016/j.aquaculture.2022.738948
Ahmed I., Jan K., Fatma S., Dawood M. A. O. (2022). Muscle proximate composition of various food fish species and their nutritional significance: A review. J. Anim. Physiol. Anim. Nutr. 106, 690–719. doi: 10.1111/jpn.13711
Akbari H., Shekrabi S. P. H., Soltani M., Mehrgan M. S. (2021). Effects of potential probiotic Enterococcus casseliflavus (EC-001) on growth performance, immunity, and resistance to Aeromonas hydrophila infection in common carp (Cyprinus carpio). Probiotics Antimicrobial Proteins 13, 1–10. doi: 10.1007/s12602-021-09771-x
Akhter N., Wu B., Memon A. M., Mohsin M. (2015). Probiotics and prebiotics associated with aquaculture: a review. Fish Shellfish Immunol. 45, 733–741. doi: 10.1016/j.fsi.2015.05.038
Ali M., Iqbal F., Salam A., Iram S., Athar M. (2005). Comparative study of body composition of different fish species from brackish water pond. Int. J. Environ. Sci. Technol. 2, 229–232. doi: 10.1007/BF03325880
AOAC (1995). Official methods of analysis. 16th edition (Washington, DC, USA: Association of Official Analytical Chemists).
Aoki T., Takano T., Santos M. D., Kondo H., Hirono I. (2008). Molecular innate immunity in teleost fish: review and future perspectives. Fisheries Global Welfare Environment Memorial Book 5th World Fisheries Congress, 263–276.
Assefa A., Abunna F. (2018). Maintenance of fish health in aquaculture: review of epidemiological approaches for prevention and control of infectious disease of fish. Veterinary Med. Int. 2018. doi: 10.1155/2018/5432497
Azimirad M., Meshkini S., Ahmadifard N., Hoseinifar S. H. (2016). The effects of feeding with synbiotic (Pediococcus acidilactici and fructooligosaccharide) enriched adult Artemia on skin mucus immune responses, stress resistance, intestinal microbiota and performance of angelfish (Pterophyllum scalare). Fish Shellfish Immunol. 54, 516–522. doi: 10.1016/j.fsi.2016.05.001
Barnes A. C., Ellis A. E. (2004). Role of capsule in serotypic differences and complement fixation by Lactococcus garvieae. Fish Shellfish Immunol. 16, 207–214. doi: 10.1016/S1050-4648(03)00079-2
Barros R. R., Carvalho M. D. G. S., Peralta J. M., Facklam R. R., Teixeira L. M. (2001). Phenotypic and genotypic characterization of Pediococcus strains isolated from human clinical sources. J. Clin. Microbiol. 39, 1241–1246. doi: 10.1128/JCM.39.4.1241-1246.2001
Bhatnagar A., Rathi P. (2023). Isolation and characterization of autochthonous probiotics from skin mucus and their in vivo validation with dietary probiotic bacteria on growth performance and immunity of Labeo calbasu (Hamilton 1822). Fish Physiol. Biochem. 49, 191–208. doi: 10.1007/s10695-022-01168-z
Chauhan A., Singh R. (2019). Probiotics in aquaculture: a promising emerging alternative approach. Symbiosis 77, 99–113. doi: 10.1007/s13199-018-0580-1
Dawood M. A. O., Eweedah N. M., Moustafa E. M., Farahat E. M. (2020). Probiotic effects of Aspergillus oryzae on the oxidative status, heat shock protein, and immune related gene expression of Nile tilapia (Oreochromis niloticus) under hypoxia challenge. Aquaculture 520, 734669. doi: 10.1016/j.aquaculture.2019.734669
de Moraes A. V., Owatari M. S., da Silva E., de Oliveira Pereira M., Piola M., Ramos C., et al. (2022). Effects of microencapsulated probiotics-supplemented diet on growth, nonspecific immunity, intestinal health and resistance of juvenile Nile tilapia challenged with Aeromonas hydrophila. Anim. Feed Sci. Technol. 287, 115286. doi: 10.1016/j.anifeedsci.2022.115286
Ding X.-Y., Wei C.-Y., Liu Z.-Y., Yang H.-L., Han F., Sun Y.-Z. (2023). Autochthonous Bacillus subtilis and Enterococcus faecalis improved liver health, immune response, mucosal microbiota and red-head disease resistance of yellow drum (Nibea albiflora). Fish Shellfish Immunol. 134, 108575. doi: 10.1016/j.fsi.2023.108575
Einen O., Thomassen M. S. (1998). Starvation prior to slaughter in Atlantic salmon (Salmo salar): II. White muscle composition and evaluation of freshness, texture and colour characteristics in raw and cooked fillets. Aquaculture 169, 37–53. doi: 10.1016/S0044-8486(98)00332-9
El-Saadony M. T., Alagawany M., Patra A. K., Kar I., Tiwari R., Dawood M. A. O., et al. (2021). The functionality of probiotics in aquaculture: An overview. Fish Shellfish Immunol. 117, 36–52. doi: 10.1016/j.fsi.2021.07.007
Elsadek M. M., Wang S., Wu Z., Wang J., Wang X., Zhang Y., et al. (2023). Characterization of Bacillus spp. isolated from the intestines of Rhynchocypris lagowskii as a potential probiotic and their effects on fish pathogens. Microbial Pathogenesis 180, 106163. doi: 10.1016/j.micpath.2023.106163
Erfanmanesh A., Beikzadeh B., Khanzadeh M., Alishahi M. (2024). Immuno-protective response of Asian seabass (Lates calcarifer) to inactivated vaccines against Streptococcus iniae and Vibrio harveyi. BMC Veterinary Res. 20, 89. doi: 10.1186/s12917-024-03935-x
Fast M. D., Sims D. E., Burka J. F., Mustafa A., Ross N. W. (2002). Skin morphology and humoral nonspecific defence parameters of mucus and plasma in rainbow trout, coho and Atlantic salmon. Comp. Biochem. Physiol. Part A: Mol. Integr. Physiol. 132, 645–657. doi: 10.1016/S1095-6433(02)00109-5
Frank J. A., Reich C. I., Sharma S., Weisbaum J. S., Wilson B. A., Olsen G. J. (2008). Critical evaluation of two primers commonly used for amplification of bacterial 16S rRNA genes. Appl. Environ. Microbiol. 74, 2461–2470. doi: 10.1128/AEM.02272-07
Gao Y., Tan R., Wang Z., Qiang L., Yao H. (2024). The effects of Bacillus subtilis on the immunity, mucosal tissue morphology, immune-related gene transcriptions, and intestinal microbiota in flounder (Paralichthys olivaceus) with two feeding methods: continuous versus discontinuous feeding. Veterinary Immunol. Immunopathol. 271, 110742. doi: 10.1016/j.vetimm.2024.110742
Ghori I., Tubassam M., Ahmad T., Zuberi A., Imran M. (2022). Gut microbiome modulation mediated by probiotics: Positive impact on growth and health status of Labeo rohita. Front. Physiol. 13, 949559. doi: 10.3389/fphys.2022.949559
Giri S. S., Sukumaran V., Oviya M. (2013). Potential probiotic Lactobacillus plantarum VSG3 improves the growth, immunity, and disease resistance of tropical freshwater fish, Labeo rohita. Fish Shellfish Immunol. 34, 660–666. doi: 10.1016/j.fsi.2012.12.008
Gobi N., Vaseeharan B., Chen J.-C., Rekha R., Vijayakumar S., Anjugam M., et al. (2018). Dietary supplementation of probiotic Bacillus licheniformis Dahb1 improves growth performance, mucus and serum immune parameters, antioxidant enzyme activity as well as resistance against Aeromonas hydrophila in tilapia Oreochromis mossambicus. Fish Shellfish Immunol. 74, 501–508. doi: 10.1016/j.fsi.2017.12.066
Hassaan M. S., El-Sayed A. M. I., Mohammady E. Y., Zaki M. A. A., Elkhyat M. M., Jarmołowicz S., et al. (2021). Eubiotic effect of a dietary potassium diformate (KDF) and probiotic (Lactobacillus acidophilus) on growth, hemato-biochemical indices, antioxidant status and intestinal functional topography of cultured Nile tilapia Oreochromis niloticus fed diet free fi. Aquaculture 533, 736147. doi: 10.1016/j.aquaculture.2020.736147
Heidarieh M., Mirvaghefi A. R., Akbari M., Sheikhzadeh N., Kamyabi-Moghaddam Z., Askari H., et al. (2013). Evaluations of H ilyses™, fermented S accharomyces cerevisiae, on rainbow trout (Oncorhynchus mykiss) growth performance, enzymatic activities and gastrointestinal structure. Aquacult. Nutr. 19, 343–348. doi: 10.1111/anu.2013.19.issue-3
Holbech H., Andersen L., Petersen G. I., Korsgaard B., Pedersen K. L., Bjerregaard P. (2001). Development of an ELISA for vitellogenin in whole body homogenate of zebrafish (Danio rerio). Comp. Biochem. Physiol. Part C: Toxicol. Pharmacol. 130, 119–131. doi: 10.1016/S1532-0456(01)00229-0
Hoseinifar S. H., Khalili M., Rostami H. K., Esteban M.Á. (2013). Dietary galactooligosaccharide affects intestinal microbiota, stress resistance, and performance of Caspian roach (Rutilus rutilus) fry. Fish Shellfish Immunol. 35, 1416–1420. doi: 10.1016/j.fsi.2013.08.007
Hoseinifar S. H., Khalili M., Sun Y. (2016a). Intestinal histomorphology, autochthonous microbiota and growth performance of the oscar (Astronotus ocellatus Agassiz 1831) following dietary administration of xylooligosaccharide. J. Appl. Ichthyol. 32, 1137–1141. doi: 10.1111/jai.13118
Hoseinifar S. H., Mirvaghefi A., Amoozegar M. A., Sharifian M., Esteban M.Á. (2015). Modulation of innate immune response, mucosal parameters and disease resistance in rainbow trout (Oncorhynchus mykiss) upon synbiotic feeding. Fish Shellfish Immunol. 45, 27–32. doi: 10.1016/j.fsi.2015.03.029
Hoseinifar S. H., Sun Y.-Z., Wang A., Zhou Z. (2018). Probiotics as means of diseases control in aquaculture, a review of current knowledge and future perspectives. Front. Microbiol. 9, 364982. doi: 10.3389/fmicb.2018.02429
Hoseinifar S. H., Zoheiri F., Lazado C. C. (2016b). Dietary phytoimmunostimulant Persian hogweed (Heracleum persicum) has more remarkable impacts on skin mucus than on serum in common carp (Cyprinus carpio). Fish Shellfish Immunol. 59, 77–82. doi: 10.1016/j.fsi.2016.10.025
Hossain M. K., Hossain M. M., Mim Z. T., Khatun H., Hossain M. T., Shahjahan M. (2022). Multi-species probiotics improve growth, intestinal microbiota and morphology of Indian major carp mrigal Cirrhinus cirrhosus. Saudi J. Biol. Sci. 29, 103399. doi: 10.1016/j.sjbs.2022.103399
Hussain A., Sachan S. G. (2023). Fish epidermal mucus as a source of diverse therapeutical compounds. Int. J. Pept. Res. Ther. 29, 36. doi: 10.1007/s10989-023-10505-6
Khanzadeh M., Hoseinifar S. H., Beikzadeh B. (2024a). Investigating the effect of hydroalcoholic extract of red algae (Laurencia caspica) on growth performance, mucosal immunity, digestive enzyme activity and resistance to Streptococcus iniae and Aeromonas hydrophila in Nile tilapia (Oreochromis niloticus). Aquacult. Rep. 35, 101984. doi: 10.1016/j.aqrep.2024.101984
Khanzadeh M., Hoseinifar S. H., Zargari A., Tabibi H., Van Doan H., Rabetimarghezar N. (2024b). Fucoidan derived from Sargassum ilicifolium affects growth and hemato-immunological parameters and antioxidant defense in Oscar (Astronotus ocellatus). Front. Mar. Sci. 11, 1370871. doi: 10.3389/fmars.2024.1370871
Kuebutornye F. K. A., Wang Z., Lu Y., Abarike E. D., Sakyi M. E., Li Y., et al. (2020). Effects of three host-associated Bacillus species on mucosal immunity and gut health of Nile tilapia, Oreochromis niloticus and its resistance against Aeromonas hydrophila infection. Fish Shellfish Immunol. 97, 83–95. doi: 10.1016/j.fsi.2019.12.046
Lan P. T. N., Hayashi H., Sakamoto M., Benno Y. (2002). Phylogenetic analysis of cecal microbiota in chicken by the use of 16S rDNA clone libraries. Microbiol. Immunol. 46, 371–382. doi: 10.1111/j.1348-0421.2002.tb02709.x
Li X., Ringø E., Hoseinifar S. H., Lauzon H. L., Birkbeck H., Yang D. (2019). The adherence and colonization of microorganisms in fish gastrointestinal tract. Rev. Aquacult. 11, 603–618. doi: 10.1111/raq.12248
Liu X., Sun W., Zhang Y., Zhou Y., Xu J., Gao X., et al. (2020). Impact of Aeromonas hydrophila and infectious spleen and kidney necrosis virus infections on susceptibility and host immune response in Chinese perch (Siniperca chuatsi). Fish Shellfish Immunol. 105, 117–125. doi: 10.1016/j.fsi.2020.07.012
Loganathan K., Arulprakash A., Prakash M., Senthilraja P. (2013). Lysozyme, protease, alkaline phosphatase and esterase activity of epidermal skin mucus of freshwater snake head fish Channa striatus. Int. J. Res. Pharm. Biosci. 3, 17–20.
Merrifield D. L., Bradley G., Harper G. M., Baker R. T. M., Munn C. B., Davies S. J. (2011). Assessment of the effects of vegetative and lyophilized Pediococcus acidilactici on growth, feed utilization, intestinal colonization and health parameters of rainbow trout (Oncorhynchus mykiss Walbaum). Aquacult. Nutr. 17, 73–79. doi: 10.1111/anu.2010.17.issue-1
Mocanu E. E., Savin V., Popa M. D., Dima F. M. (2022). The effect of probiotics on growth performance, haematological and biochemical profiles in Siberian sturgeon (Acipenser baerii Brandt 1869). Fishes 7, 239. doi: 10.3390/fishes7050239
Mohammadi G., Rafiee G., Abdelrahman H. A. (2020). Effects of dietary Lactobacillus plantarum (KC426951) in biofloc and stagnant-renewal culture systems on growth performance, mucosal parameters, and serum innate responses of Nile tilapia Oreochromis niloticus. Fish Physiol. Biochem. 46, 1167–1181. doi: 10.1007/s10695-020-00777-w
Nedaei S., Noori A., Valipour A., Khanipour A. A., Hoseinifar S. H. (2019). Effects of dietary galactooligosaccharide enriched commercial prebiotic on growth performance, innate immune response, stress resistance, intestinal microbiota and digestive enzyme activity in Narrow clawed crayfish (Astacus leptodactylus Eschscholtz, 182. Aquaculture 499, 80–89. doi: 10.1016/j.aquaculture.2018.08.076
Picchietti S., Miccoli A., Fausto A. M. (2021). Gut immunity in European sea bass (Dicentrarchus labrax): a review. Fish Shellfish Immunol. 108, 94–108. doi: 10.1016/j.fsi.2020.12.001
Rahbar M., Khoshkholgh M., Nazari S. (2023). Population structure of Caspian Kutum (Rutilus frisii, Nordmann 1840) in the southern coast of Caspian Sea using genome-wide single nucleotide polymorphism markers. Fisheries Res. 257, 106499. doi: 10.1016/j.fishres.2022.106499
Ramos C. L., Thorsen L., Schwan R. F., Jespersen L. (2013). Strain-specific probiotics properties of Lactobacillus fermentum, Lactobacillus plantarum and Lactobacillus brevis isolates from Brazilian food products. Food Microbiol. 36, 22–29. doi: 10.1016/j.fm.2013.03.010
Rao Y. V., Das B. K., Jyotyrmayee P., Chakrabarti R. (2006). Effect of Achyranthes aspera on the immunity and survival of Labeo rohita infected with Aeromonas hydrophila. Fish Shellfish Immunol. 20, 263–273. doi: 10.1016/j.fsi.2005.04.006
Ringø E., Van Doan H., Lee S. H., Soltani M., Hoseinifar S. H., Harikrishnan R., et al. (2020). Probiotics, lactic acid bacteria and bacilli: interesting supplementation for aquaculture. J. Appl. Microbiol. 129, 116–136. doi: 10.1111/jam.14628
Roosta Z., Hoseinifar S. H. (2016). The effects of crowding stress on some epidermal mucus immune parameters, growth performance and survival rate of tiger barb (Pentius tetrazona). Aquacult. Res. 47, 1682–1686. doi: 10.1111/are.12616
Rosenberg E., Zilber-Rosenberg I. (2018). The hologenome concept of evolution after 10 years. Microbiome 6, 1–14. doi: 10.1186/s40168-018-0457-9
Ross N. W., Firth K. J., Wang A., Burka J. F., Johnson S. C. (2000). Changes in hydrolytic enzyme activities of naive Atlantic salmon Salmo salar skin mucus due to infection with the salmon louse Lepeophtheirus salmonis and cortisol implantation. Dis. Aquat. Organisms 41, 43–51. doi: 10.3354/dao041043
Rufchaei R., Nedaei S., Hoseinifar S. H., Hassanpour S., Golshan M., Sayad Bourani M. (2021). Improved growth performance, serum and mucosal immunity, haematology and antioxidant capacity in pikeperch (Sander lucioperca) using dietary water hyacinth (Eichhornia crassipes) leaf powder. Aquacult. Res. 52, 2194–2204. doi: 10.1111/are.15072
Salam M. A., Islam M. A., Paul S. I., Rahman M. M., Rahman M. L., Islam F., et al. (2021). Gut probiotic bacteria of Barbonymus gonionotus improve growth, hematological parameters and reproductive performances of the host. Sci. Rep. 11, 10692. doi: 10.1038/s41598-021-90158-x
Santos G. G., Libanori M. C. M., Pereira S. A., Ferrarezi J. V. S., Ferreira M. B., Soligo T. A., et al. (2023). Probiotic mix of Bacillus spp. and benzoic organic acid as growth promoter against Streptococcus agalactiae in Nile tilapia. Aquaculture 566, 739212. doi: 10.1016/j.aquaculture.2022.739212
Saravanan K., Sivaramakrishnan T., Praveenraj J., Kiruba-Sankar R., Haridas H., Kumar S., et al. (2021). Effects of single and multi-strain probiotics on the growth, hemato-immunological, enzymatic activity, gut morphology and disease resistance in Rohu, Labeo rohita. Aquaculture 540, 736749. doi: 10.1016/j.aquaculture.2021.736749
Sepehrfar D., Hoseinifar S. H., Jafarnodeh A. (2019). The Effects of Singular or Combined Administration of Pediococcu sacidilactici and Raffinoson Mucosal Immune Parameters and Intestinal Histomorphology of Gold Fish (Carassiusa uratus). J. Plasma Biomark. 1, 25–34.
Shadrack R. S., Kotra K. K., Gereva S., Teiba I. I., El-Ratel I. T., El Basuini M. F. (2023). Utilizing dietary probiotics can boost amberjack (Seriola dumerili) lysozyme activity, antioxidant capacity, and gut microbiota. Sci. Afr. 22, e01905. doi: 10.1016/j.sciaf.2023.e01905
Shan H., Wang T., Dong Y., Ma S. (2019). Effects of dietary Ampithoe sp. supplementation on the growth, energy status, antioxidant capacity, and ammonia-N tolerance of the shrimp Litopenaeus vannamei: Continuous versus interval feeding. Aquaculture 509, 32–39. doi: 10.1016/j.aquaculture.2019.05.021
Siwicki A. K. (1993). “Nonspecific defense mechanisms assay in fish. II. Potential killing activity of neutrophils and monocytes, lysozyme activity in serum and organs and total immunoglobulin (Ig) level in serum,” in Fish Diseases Diagnosis and Prevention Methods. FAO-Project GCP/INT/526/JPN, 105-112.
Suriano F., Nyström E. E. L., Sergi D., Gustafsson J. K. (2022). Diet, microbiota, and the mucus layer: The guardians of our health. Front. Immunol. 13, 953196. doi: 10.3389/fimmu.2022.953196
Tachibana L., Telli G. S., de Carla Dias D., Gonçalves G. S., Ishikawa C. M., Cavalcante R. B., et al. (2020). Effect of feeding strategy of probiotic Enterococcus faecium on growth performance, hematologic, biochemical parameters and nonspecific immune response of Nile tilapia. Aquacult. Rep. 16, 100277. doi: 10.1016/j.aqrep.2020.100277
Taherpour M., Roomiani L., Islami H. R., Mehrgan M. S. (2023). Effect of dietary butyric acid, Bacillus licheniformis(probiotic), and their combination on hemato-biochemical indices, antioxidant enzymes, immunological parameters, and growth performance of Rainbow trout (Oncorhynchus mykiss). Aquacult. Rep. 30, 101534. doi: 10.1016/j.aqrep.2023.101534
Tarkhani R., Imani A., Hoseinifar S. H., Ashayerizadeh O., Moghanlou K. S., Manaffar R., et al. (2019). Comparative study of host-associated and commercial probiotic effects on serum and mucosal immune parameters, intestinal microbiota, digestive enzymes activity and growth performance of roach (Rutilus rutilus caspicus) fingerlings. Fish Shellfish Immunol. 98, 661–669. doi: 10.1016/j.fsi.2019.10.063
Tarkhani R., Imani A., Hoseinifar S. H., Moghanlou K. S., Manaffar R. (2020). The effects of host-associated Enterococcus faecium CGMCC1. 2136 on serum immune parameters, digestive enzymes activity and growth performance of the Caspian roach (Rutilus rutilus caspicus) fingerlings. Aquaculture 519, 734741. doi: 10.1016/j.aquaculture.2019.734741
Thy H. T. T., Tri N. N., Quy O. M., Fotedar R., Kannika K., Unajak S., et al. (2017). Effects of the dietary supplementation of mixed probiotic spores of Bacillus amyloliquefaciens 54A, and Bacillus pumilus 47B on growth, innate immunity and stress responses of striped catfish (Pangasianodon hypophthalmus). Fish Shellfish Immunol. 60, 391–399. doi: 10.1016/j.fsi.2016.11.016
Tort L., Balasch J. C., Mackenzie S. (2003). Fish immune system. A crossroads between innate and adaptive responses. Inmunología 22, 277–286.
Van Doan H., Hoseinifar S. H., Ringø E., Ángeles Esteban M., Dadar M., Dawood M. A. O., et al. (2020). Host-associated probiotics: A key factor in sustainable aquaculture. Rev. Fisheries Sci. Aquacult. 28, 16–42. doi: 10.1080/23308249.2019.1643288
Wu J. J., Ma Y. K., Zhang F. F., Chen F. S. (2012). Biodiversity of yeasts, lactic acid bacteria and acetic acid bacteria in the fermentation of “Shanxi aged vinegar”, a traditional Chinese vinegar. Food Microbiol. 30, 289–297. doi: 10.1016/j.fm.2011.08.010
Yousefi M., Farsani M. N., Ghafarifarsani H., Raeeszadeh M. (2023). Dietary Lactobacillus helveticus and Gum Arabic improves growth indices, digestive enzyme activities, intestinal microbiota, innate immunological parameters, antioxidant capacity, and disease resistance in common carp. Fish Shellfish Immunol. 135, 108652. doi: 10.1016/j.fsi.2023.108652
Keywords: whitefish, autochthonous probiotic, Pediococcus pentosaceus, growth indicators, nonspecific immunity
Citation: Hoseinifar SH, Hoseini SM, Taheri Mirghaed A, Ghelichpour M, Shirzad-Aski H, Van Doan H, El-Haroun E, Safari R and Khanzadeh M (2024) Comparison of the effects of host-associated (autochthonous) and commercial probiotics on immune responses, growth parameters and intestinal microbiota of Caspian whitefish (Rutilus frisii kutum) fry. Front. Mar. Sci. 11:1446927. doi: 10.3389/fmars.2024.1446927
Received: 14 June 2024; Accepted: 01 August 2024;
Published: 23 August 2024.
Edited by:
Helena Peres, University of Porto, PortugalReviewed by:
Won Je Jang, Pukyong National University, Republic of KoreaNeeraj Kumar, National Institute of Abiotic Stress Management (ICAR), India
Patricia Diaz-Rosales, Spanish National Research Council (CSIC), Spain
Copyright © 2024 Hoseinifar, Hoseini, Taheri Mirghaed, Ghelichpour, Shirzad-Aski, Van Doan, El-Haroun, Safari and Khanzadeh. This is an open-access article distributed under the terms of the Creative Commons Attribution License (CC BY). The use, distribution or reproduction in other forums is permitted, provided the original author(s) and the copyright owner(s) are credited and that the original publication in this journal is cited, in accordance with accepted academic practice. No use, distribution or reproduction is permitted which does not comply with these terms.
*Correspondence: Seyed Hossein Hoseinifar, hoseinifar@gau.ac.ir