- 1Biology and Environmental Science Department, University of New Haven, West Haven, CT, United States
- 2Milford Laboratory, Northeast Fisheries Science Center, National Marine Fisheries Service, National Oceanic and Atmospheric Administration, Milford, CT, United States
- 3BioPath, Southern Connecticut State University, New Haven, CT, United States
- 4A.I.S. Inc., North Dartmouth, MA, United States
Observations of fish behavior can provide insights into habitat preferences and use. Black sea bass, Centropristis striata, are a territorial temperate reef finfish species known for their high commercial and recreational value and association with structured habitat. We used underwater action cameras to record video of black sea bass to assess territorial (agonistic, ambush, displacement) and occupancy (station-keeping) behaviors on shelf and bag style oyster aquaculture cages at a shellfish farm, and on boulders at a natural rock reef near Milford, Connecticut in Long Island Sound, Northwest Atlantic. Black sea bass at a variety of life stages were highly associated with cages, including young-of-the-year, and age 1+ fish. The high abundance of black sea bass observed on cages relative to boulders suggests this species has an affinity for the vertical structure created by aquaculture gear. When behaviors were normalized to the total fish sightings, black sea bass showed no significant difference in frequency of behaviors between habitats, indicating that per-fish rates of behavior were similar on cages and boulders. Demonstration of territorial and occupancy behaviors by black sea bass on, and around cages suggests that aquaculture gear provides structured habitat and ecosystem services for this species similar to natural reefs.These results suggest that essential fish habitat descriptions of manmade structures used by black sea bass could be broadened to include aquaculture gear. Our study provides novel information on behavioral interactions of black sea bass with oyster cages that may support aquaculture permitting and consultation processes.
Introduction
Commercial aquaculture that introduces complex structures into nearshore environments may provide ecosystem services to the local fish fauna. Shellfish farming practices in Long Island Sound (LIS), Connecticut, have expanded from traditional on-bottom culture methods, where oysters are grown on the seafloor, to include submerged aquaculture gear using multi-tiered cages for cultivation of oysters (Getchis, 2005). Oyster aquaculture cages, where shellfish are placed in mesh bags on tiered shelves, are increasing in popularity in New England waters as they can grow more animals on a smaller spatial footprint than traditional on-bottom methods (Archer et al., 2014; Scuderi and Chen, 2019). Multidimensional cages, which optimize surface area to enhance growth and survival of oysters within the structure of the gear itself (DeAlteris et al., 2004), may be utilized by structure-oriented fish species, as well.
Previous research has found a greater diversity and abundance of organisms in association with oyster cages, as compared to certain natural habitats (e.g., Erbland and Ozbay, 2008; Muething et al., 2020; Theuerkauf et al., 2021). Studies in the waters off the coasts of Connecticut (Mercaldo-Allen et al., 2021; 2023), New Jersey (Shinn et al., 2021), and Rhode Island (Tallman and Forrester, 2007) found that finfish communities used oyster grow-out cages similarly to nearby natural structured habitats, and it was evident these cages acted much like artificial reefs. Interstitial spaces in and amongst bags of oysters in cages may create areas of flow refuge, shelter from predators, focal sites for ambush, and proximate cover for benthic pick and scan foraging. Observations of fish behavior associated with aquaculture gear can provide insights into how these structures may provide habitat for temperate reef fish assemblages.
The behavior of highly territorial fish can influence the community composition and spatial distribution of fishes within a habitat. Territoriality is an important strategy in which an individual fish defends a localized resource, such as food, shelter, mates, or spawning sites, or guards eggs and/or offspring from competitors (Reebs, 2008; Scaia et al., 2018). Agonistic behaviors, where fish defend a habitat from other fish, and occupancy behavior, where fish hold position within a habitat (e.g., station keeping), may be interpreted as indicators of habitat quality. In tropical reef communities, the behaviors of aggressive fish, such as damselfish and parrotfish, influence biota distributions across the reef (Buckman and Ogden, 1973; Rasa, 1976; Barneche et al., 2009; Medeiros et al., 2010). For example, damselfish defend the algae gardens they cultivate as a vital food resource, and as nesting and spawning territory associated with the physical habitat structure (Barneche et al., 2009; Medeiros et al., 2010; Schrandt et al., 2012). In sub-tropical and temperate regions, territorial reef fish are known to aggressively defend structured habitats from conspecifics. Male cunner Tautogalabrus adspersus are known to maintain year-round territories by chasing juveniles and smaller adults (Martel and Green 1987). Gag Mycteroperca microlepis and scamp grouper M. phenax use lateral displays, chasing, and physical interactions (e.g., nudging and pushing) to ward off subordinate conspecifics from structured habitats (Gilmore and Jones 1992). While research efforts have focused on fish behavioral interactions in tropical or temperate reef environments, little is known about the territorial behavior of structure-oriented fish around aquaculture gear. Prior research has not focused directly on comparing territoriality in man-made and natural structures, resulting in uncertain findings (e.g., tautog Tautoga onitis use of wrecks in Chesapeake Bay; Arendt et al., 2001).
One such temperate reef species, the Black Sea Bass, Centropristis striata, is a demersal finfish (family Serranidae) found in the Northwestern Atlantic Ocean from the Gulf of Mexico to the Gulf of Maine (Wantanabe, 2011; Fabrizio et al., 2014; Miller et al., 2016; Cullen and Stevens, 2017a). This species is commercially fished using fish pots and hand-lines while recreational fishers use hook-and-line (Atlantic States Marine Fisheries Commission, 2021). Black sea bass are structure-oriented and have been observed using both natural and man-made structures as habitat (Drohan et al., 2007). Natural habitats include cobble and boulder, live bottom reefs and outcrops, vegetation, and shellfish, sponges, and other epifauna; man-made structures that black sea bass use as habitat include wharves, pilings, artificial reefs, shipwrecks, and fishing gear (Steimle and Zetlin, 2000; Scharf et al., 2006; Drohan et al., 2007; Fabrizio et al., 2014). Like those of many commercially fished and managed species, definitions of black sea bass Essential Fish Habitat (EFH) are largely based on historic survey data and available published literature (Levin and Stunz, 2005; Drohan et al., 2007). Existing black sea bass EFH definitions include both natural substrates and manmade structures (Drohan et al., 2007). Recent studies of fish abundance on shellfish farms have found a strong association of black sea bass with oyster cages, but information is needed to better understand habitat services provided to fish by aquaculture gear (Mercaldo-Allen et al., 2020a; 2021; 2023).
Black sea bass are territorial and congregate in structured environments, where interactions with conspecifics can induce aggressive behaviors. Spatially complex artificial substrates also elicit territorial responses from black sea bass, which suggests that man-made structures can serve as important habitats (Auster et al., 1996; Drohan et al., 2007; Campanella et al., 2019). Black sea bass are protogynous hermaphrodites, where juveniles develop first as females and those with dominant behavior transition to males between 2 and 5 years of age, and 23 to 33 cm in length (Wantanabe, 2011; Cullen and Stevens, 2017a). Dominant males establish territories they can successfully patrol and defend, which is evident through territorial behaviors such as chasing, fin flaring, and lateral displays (Fabrizio et al., 2014). Territoriality is not limited to adults, as juveniles actively defend favorable habitat from conspecifics within larger patches of structure (Drohan et al., 2007). While nearshore home ranges rely on the presence of structure, size of home ranges can vary depending on the size and complexity of structure (Fabrizio et al., 2014). Sex also influences habitat occupancy: males aggressively defend set territories featuring complex structure, while juveniles, typically female, move between structures based on resource availability, such as space and prey abundance (Able et al., 1995; Gwak, 2008). Interactions with physical structure in estuarine habitats may drive the spatial distributions of temperate fish, but to characterize relationships between complex structure and specific behaviors additional study is required (Levin and Stunz, 2005; Fabrizio et al., 2014).
We observed, characterized, and quantified territorial and occupancy behaviors in young-of-the year (YOY), and age 1+ black sea bass on cages and boulders to compare fish interactions on aquaculture gear with activity on natural structured reef habitat. We predicted that black sea bass occupancy and territorial behaviors would be more frequent on aquaculture cages than on natural boulder reefs. Greater occurrences of occupancy and territorial behavior on aquaculture gear could indicate that oyster cages function much like other natural and artificial habitats currently included in existing essential fish habitat descriptions for black sea bass (MAFMC, 1999; Drohan et al., 2007). Research on black sea bass behavior associated with oyster aquaculture cages and boulder seafloor may help to identify the ecosystem services provided to fish by aquaculture gear.
Materials and methods
Study sites, camera deployments and recording schedule
The two study sites included an eastern oyster (Crassostrea virginica) aquaculture cage farm (cage), and a natural cobble and boulder reef (boulder) located within the same embayment off Milford, Connecticut in Long Island Sound (Figure 1). The cage farm was located on a 0.11 km2 (28.4 acres) shellfish lease, permitted for up to 200 cages. An adjacent 0.21 km2 (52.7 acres) lease, permitted for up to 250 cages, was co-located at the cage farm site. Five shelf-and-bag style off-bottom oyster aquaculture study cages (Ketcham Supply, New Bedford MA, USA), stocked with ~2.5-5 cm seed oysters (150 per bag; 900 per cage per industry practices) were placed 47.5 m (156 ft) apart at the cage farm. The boulder reef was horseshoe-shaped, patchy and covered 0.25 km2 (61.8 acres) of seafloor and served as a structured reference site for natural hard bottom habitat. Cobble and boulders composed up to 70% of the bottom substrate (Mercaldo-Allen et al., 2011). Four 1-m boulders (10+ m apart and out of visual range of one another) were selected for study using stationary video cameras on the boulder reef. Water depths at high tide measured 4.6 m (15 ft) at the cage farm, and 6.1 m (20 ft) at the rock reef site. During the May to September study period, temperature and salinity in the Milford embayment ranged from 11.9 to 24.7°C and from 20.1 to 28.2 PSU, respectively.
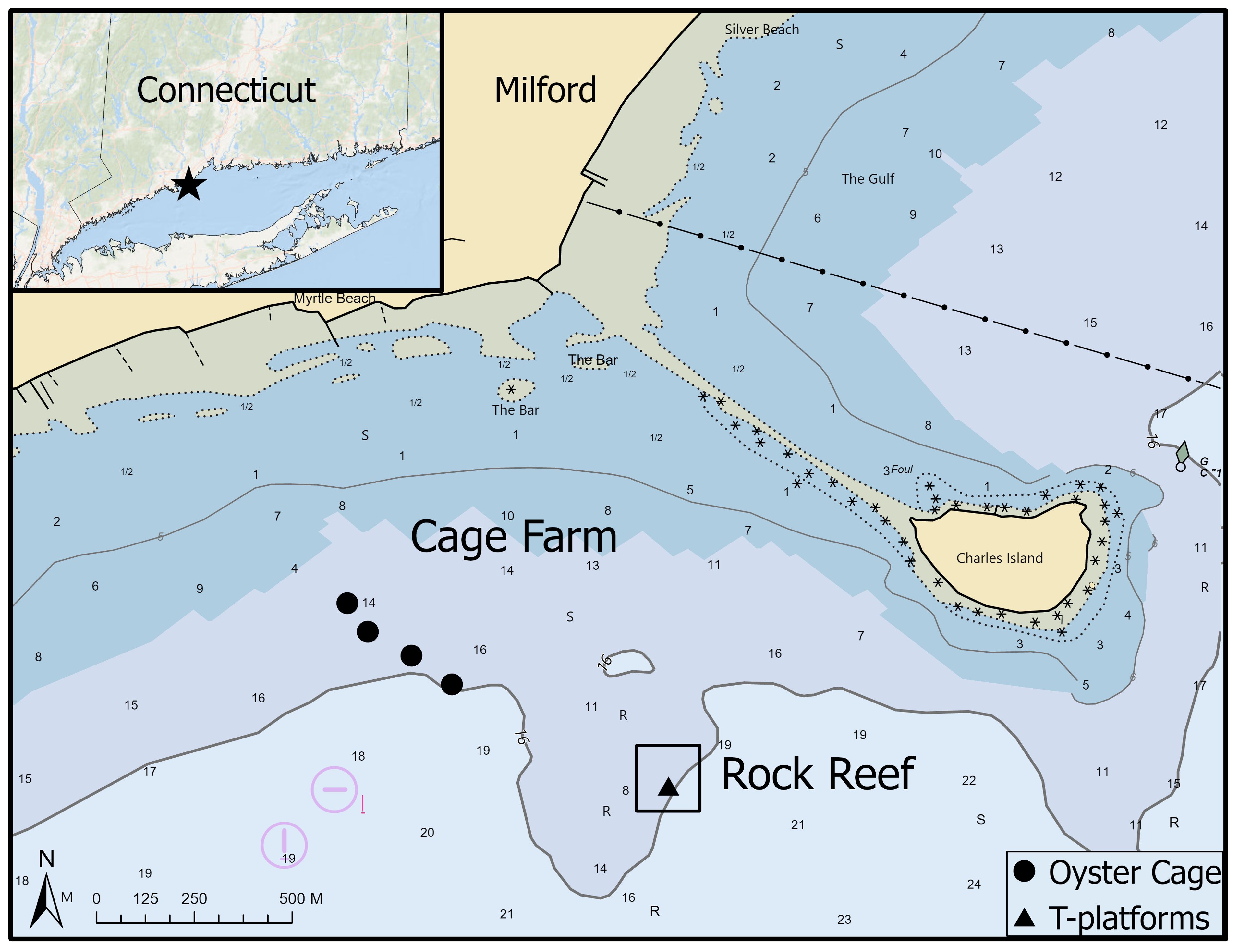
Figure 1. Map of study sites within an embayment near Milford, CT showing study cages placed on a commercial shellfish farm (cage farm) and T-platform camera-mounting stands on a natural cobble and boulder reef (rock reef). Inset map shows location of sites within Long Island Sound, USA. Symbols indicate the placement of study cages (circles) and the square box indicates the boulder area on the rock reef where we placed the four T-platform stands (inside triangle), which could not be delineated at this map scale. Numbers indicate water depth in meters.
Methods for deployment and retrieval of action cameras on oyster cages and adjacent to boulders are described in Mercaldo-Allen et al. (2021; 2023). Briefly, we attached two cameras to each study cage, one positioned to record across the cage top and the other with a view across two cage sides and the interface between the cage and the seafloor (Figure 2A). Similarly, divers attached two cameras to the T-platform mounting stands placed adjacent to each study boulder, with one camera positioned to record across the top boulder surface while the other camera captured the side of the boulder and the boulder-seafloor interface (Figure 2B). Video recording began approximately 24-hours after cameras were deployed, which was intended to reduce deployment-related disturbance effects on fish behavior. Cameras recorded for 8 minutes every hour from 7AM to 7PM, yielding 13 recordings per deployment, which enabled collection over a complete tidal cycle and most daylight hours. Water clarity varied between deployments and among hours within a single deployment, however visibility generally extended the full length of the cage/boulder. To minimize the effect of variable water clarity across videos, only those fish that could be definitively identified using morphological features and swimming behavior were included.
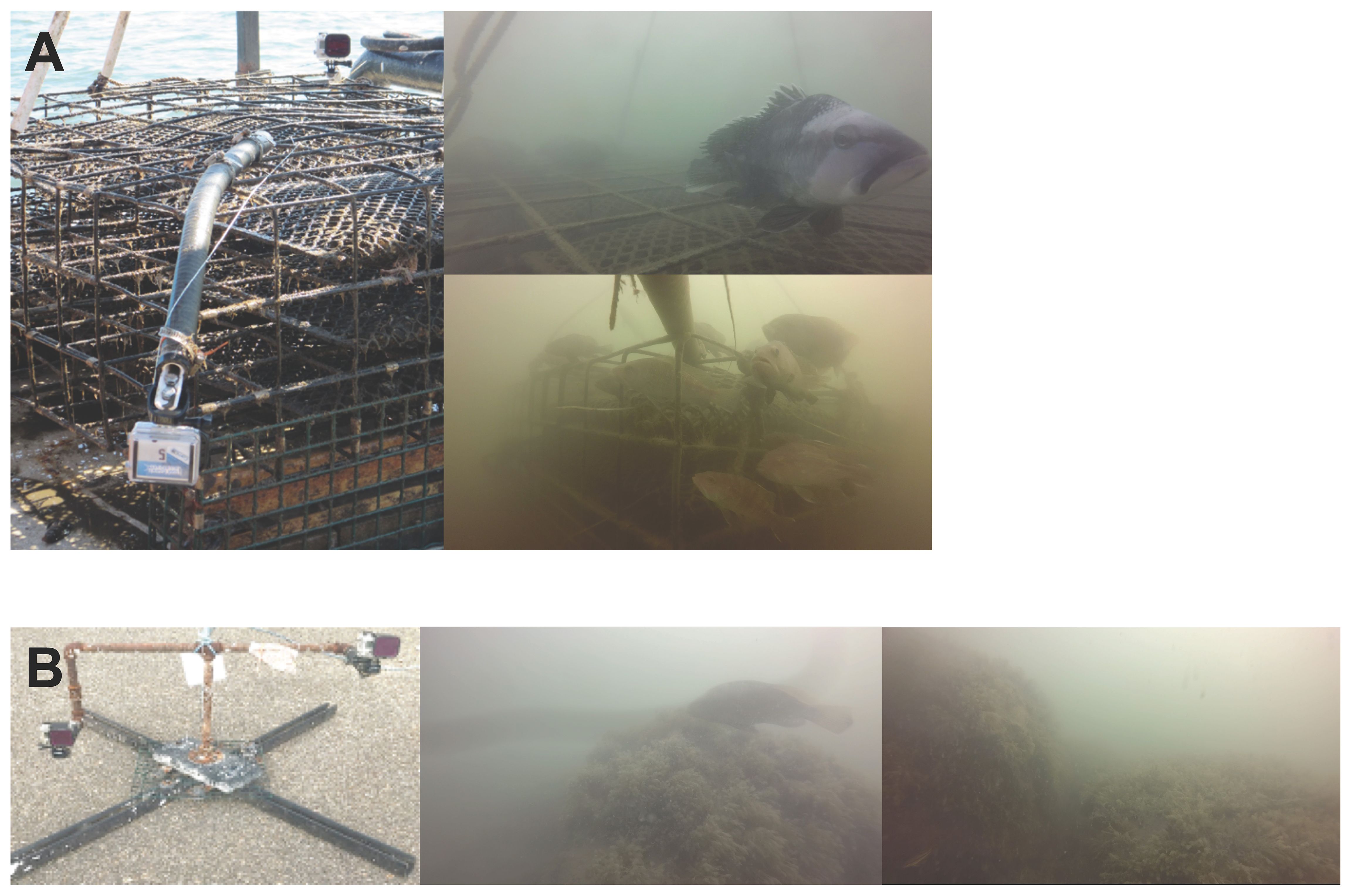
Figure 2. Photographs of camera placements on oyster aquaculture cages and T-platform stands, and the corresponding views provided by each camera. (A) Cameras were attached to cages that provided perspective across the top of the cage surface, down two sides of the cage, and the cage-seafloor interface. (B) Cameras were deployed adjacent to boulders using a minimal-structure T-platform stand that provided perspective across the boulder top, side, and boulder-seafloor interface (from Mercaldo-Allen et al., 2023).
Video was recorded concurrently on the cage farm and boulder reef sites during nine camera deployments conducted from May to September 2018. Videos from these deployments were previously analyzed to assess fish abundance and community composition on four study cages on the cage farm and four study boulders on the rock reef (Mercaldo-Allen et al., 2023). A monthly subsample of video on three cages and three T-platforms from four of the dates (June 6, July 11, August 15, and September 20; 125 hours of video) was subsequently reviewed and analyzed to quantify black sea bass territorial and occupancy behaviors throughout this period.
Behavioral analysis
The behavioral coding software The Observer XT (v. 14.2 and 15.0; Noldus Information Technology 2018) was used to identify and quantify observed behaviors. We developed a master ethogram for categorizing black sea bass behaviors associated with occupancy and territoriality that included station-keeping, ambush, displacement, and agonistic displays (Table 1). Behavioral definitions were based on observations of black sea bass activities and the proximity of those activities to conspecifics and the cage or boulder. Observed occupancy behaviors primarily consisted of station-keeping, defined as a fish holding position in and/or around the cage or boulder, generally in association with a preferred habitat (e.g., Egli and Babcock, 2004; Cullen and Stevens, 2017a). Observed territorial behaviors consisted of agonistic displays, ambushes, and aggressive displacement of conspecifics. Ambush was defined as a fish swimming directly at another fish that did not displace it from the cage or boulder while displacement occurred when a recipient fish was observed moving or being forced out of camera view, or its entire body shifted away from above or within the cage or boulder (Buckman and Ogden, 1973). Agonistic displays were any territorial behavior that was not a direct charge at another fish or resulted in the displacement of a fish. This included fin flaring, mouth to mouth where fish would approach each other with their mouths wide and have direct contact, and/or lateral display in association with the presence of a conspecific or heterospecific fish (Reebs, 2008; McCormick and Weaver, 2012; Black et al., 2014; Scaia et al., 2018). Fish sightings are defined as the number of fish observed entering the camera view during each sample day. A sample day consisted of 104 minutes of daily recording (8 minutes every hour over 13 hours) on each cage or boulder. Since camera perspectives did not allow observation of the entire cage/boulder at once, the same fish may have repeatedly moved in and out of view during an 8-minute recording interval. For this reason, fish sightings likely overestimate the total number of fish associated with a cage/boulder during any one recording period. To avoid inflating abundance estimates in determining the relative frequency of behaviors (i.e., the rate of behavior per individual), quantified behaviors were normalized using total fish sightings. Behaviors were recorded as instantaneous rather than continuous behaviors, in order to quantify behavior relative to frequency of occurrence over each recording period and compare frequency of behaviors to total number of fish sightings. Fish also demonstrated a number of other behaviors that were not quantified, such as foraging, grouping, courtship/reproductive activity, and escape from predators, that will be reported elsewhere (Mercaldo-Allen et al. in preparation).
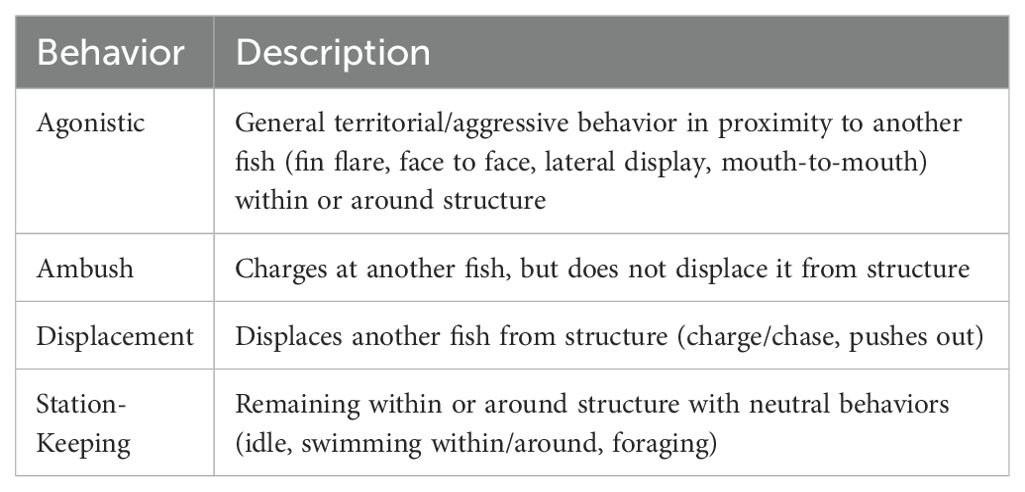
Table 1. Master ethogram for categorizing black sea bass (Centropristis striata) behaviors associated with occupancy and territoriality including station-keeping, ambush, displacement, and agonistic displays.
Life history stages were identified using a combination of fish body size and morphological characteristics (e.g., Able and Fahay, 1998; Wantanabe, 2011; Fabrizio et al., 2014; Cullen and Stevens, 2017a). Young-of-the-year (YOY) were identifiable by a black lateral stripe extending the length of their body from behind their eye to their caudal fin. Older age 1+ black sea bass were identified by the absence of the black lateral stripe, and a lighter/brighter body coloration but could not always be distinguished by sex as they appear physically identical at this stage. Adult males were distinguished by their large size, dark coloration and bright blue nuchal hump but were included in the age 1+ grouping since immature males could not be differentiated. Defining characteristics that were used to differentiate black sea bass from the other fish species present included a spiny dorsal fin with posterior fin ray, large oblique mouth, and double emarginate caudal tail with white trim (Collette and Klein-MacPhee, 2002). For the purposes of analysis and interpretation, juveniles and adult males were grouped together (age 1+) to increase sample size while YOY were assessed separately.
When variable light levels, wave action, currents and poor water clarity affected the portion of the cage or boulder that was clearly viewable and in focus within frame, discernible outlines or visible morphological features were used to identify fish species. During August and September, light availability diminished after 5pm hence some videos recorded between 6pm and 7pm were not usable due to light sensitivity of the imaging device in the camera. While cameras were positioned to maximize the amount of habitat observed within the field of view, cameras occasionally shifted slightly during deployment. Poor quality videos with low visibility were removed from analyses and no behavioral observations were recorded. Frequent camera deployments and the use of multiple replicate cages and boulders increased the overall sample size for analysis and increased the likelihood that observations could be recorded under favorable conditions in at least one location.
Statistical analysis
A Welch’s t-test was used to compare the number of black sea bass observed in both habitats throughout the study. Generalized linear mixed models (GLMM’s) with Poisson link functions were used to assess the influence of habitat type on the frequency of defined behaviors separately for YOY and age 1+, and also for all black sea bass combined. Fixed model effects were habitat type (cage or boulder). Since each deployment featured multiple replicate cages and boulders, and the specific location of replicates varied within the studied cages and boulders, replicate was included as a random effect. Counts of behaviors were normalized to black sea bass sightings per replicate in each model, thus models compare per capita rates of behaviors observed, unbiased by total number of fish sightings across the two habitats. Data visualization included overall frequency of behaviors observed on cages and boulders. Models were constructed to assess the effects of habitat on behavior for all black sea bass and age 1+ and YOY separately (i.e., three total models for each behavior). Sample size was sufficient to conduct significance tests. The importance of habitat to overall model fit was tested using χ2 likelihood ratio tests (LRT) of nested models (i.e., the improvement in model fit due to the inclusion of habitat was determined via comparison to a null model limited to the random effect replicate). Nested models were compared using Akaike’s Information Criterion (AIC). The explanatory power of each model was measured using coefficients of determination (R2). Both conditional (random and fixed effects combined) and marginal (only fixed effects) R2 values were calculated for each model (Nakagawa and Schielzth, 2013). All statistical analyses were performed in R (v1.4.1106; R Core Team 2020).
Results
Significantly more (t11.3 = 3.01, p = 0.01) black sea bass were observed on cages than boulders (Figure 3; Table 2). Often multiple black sea bass at different sizes and life stages were observed simultaneously around cages. On boulders, sightings were usually limited to a single fish for short periods of time. Age 1+ fish were the most frequently observed life stages on both cages and boulders, while YOY were less numerous and more evenly distributed between the 2 habitats. Black sea bass were more numerous in June and September versus July and August. Age 1+ fish drove this overall trend; their observed numbers in June and September were much greater than in July or August. Few YOY were observed during June and July, while abundance increased during August and reached the highest abundance in September.
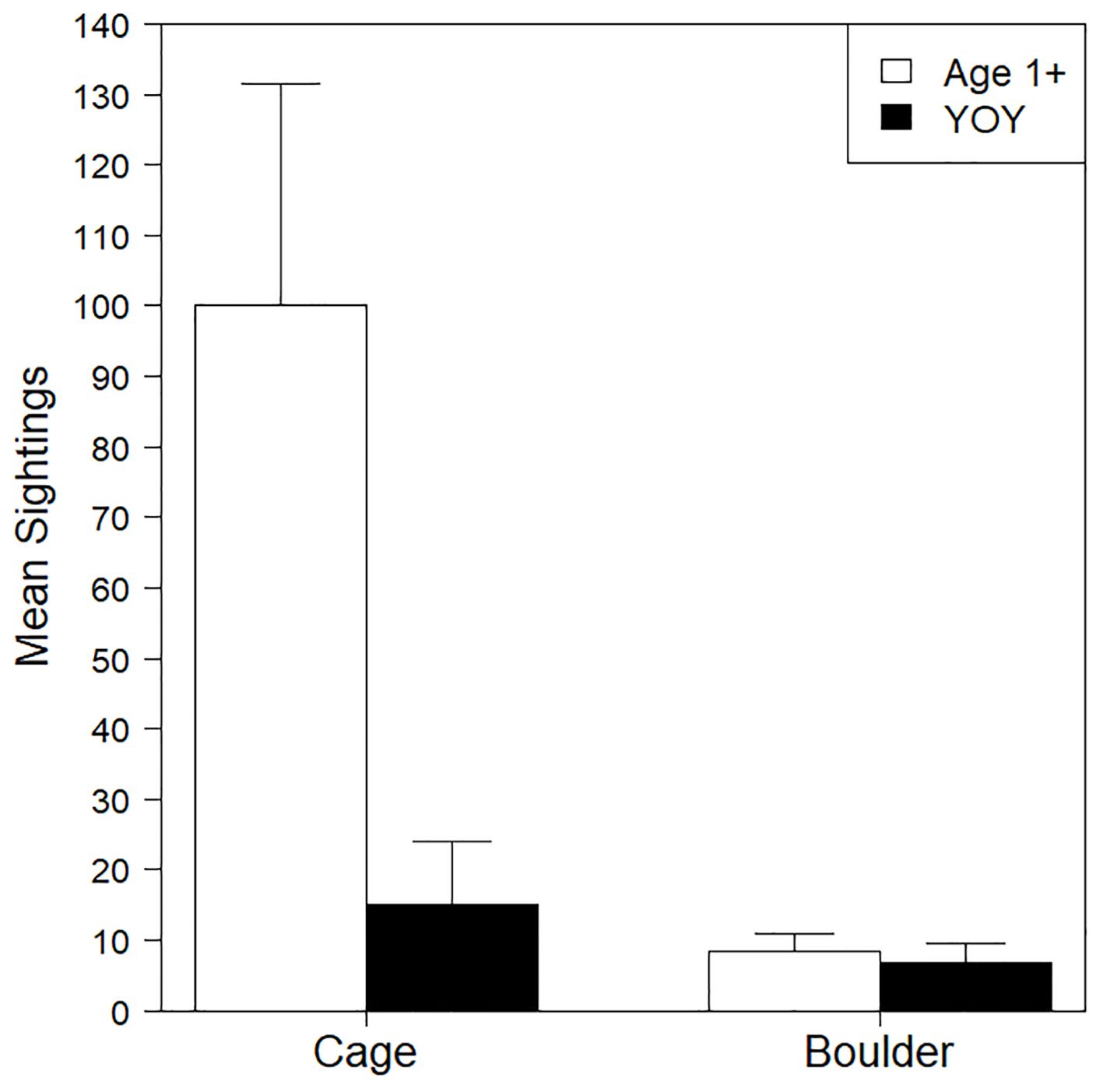
Figure 3. Barplot of mean number of age 1+ and YOY black sea bass sightings in cage or boulder habitat. Whiskers report standard error.
When data for both cage and boulder habitats were combined, station-keeping was the most frequent behavior observed for black sea bass of all life stages (mean = 6.49 ± 1.73SE occurrences per habitat per day), followed by agonistic displays (mean = 1.76 ± 0.71SE), instances of ambush (mean = 0.34 ± 0.17SE), and rarely, displacement behavior (mean = 0.24 ± 0.16SE). While station-keeping was frequently observed for age 1+ fish and YOY (age 1+ fish mean = 4.08 ± 1.23SE per habitat per day; YOY mean = 2.41 ± 0.89SE per habitat per day), YOY exhibited aggressive behaviors (i.e., agonistic displays, ambush, and displacement) less frequently (mean = 0.40 ± 0.25SE) than age 1+ (mean = 1.95 ± 1.33SE). Age 1+ fish demonstrated agonistic displays almost whenever they were present (mean = 1.53 ± 0.64SE), while both ambushes (mean = 0.22 ± 0.14SE) and successful instances of displacement (mean = 0.20 ± 0.16SE) occurred less often. Agonistic displays were far less frequent among YOY (mean = 0.23 ± 0.18SE), while ambush of a conspecific fish was limited to two observation periods (n = 1 instance on 8/15/18 and n = 11 instances on 9/20/18 on cage habitat), and successful displacement by a YOY was only observed four times (n = 1 instance on 8/15/2018 and n = 2 instances on 9/20/18 on cages; n = 1 instance on 8/15/18 on boulders).
Black sea bass were observed station-keeping on cages (mean = 11.83 ± 3.27SE) far more frequently than on boulders (mean = 1.15 ± 0.41SE; Figure 4A). Even with the stark contrast in abundance observed between the habitats included in the model of all stages (via the use of fish sightings as the offset), the occurrence of station-keeping differed marginally significantly between cage and boulder sites (LRT χ2 = 3.26, p = 0.07; Table 3). The relative rate of station keeping behavior was substantially higher on cages (0.10 observed instances of behavior per fish, as determined using fish sightings) than on boulders (0.06). Despite this, habitat alone had little explanatory power for the differences in the frequency of station-keeping observed on aquaculture gear and natural structure (marginal R2 = 0.01). Regardless of life stage, station keeping was more commonly observed on cages (age 1+ fish mean = 7.75 ± 2.36SE; YOY mean = 4.08 ± 1.72SE; Figures 4B, C) than on boulders (age 1+ mean = 0.42 ± 0.17SE; YOY mean = 0.73 ± 0.34SE). The models of station keeping behavior for age 1+ fish (LRT χ2 = 2.61, p = 0.11) and for YOY (LRT χ2 = 2.46, p = 0.12) did not reveal significant effects of habitat on the frequency of this behavior.
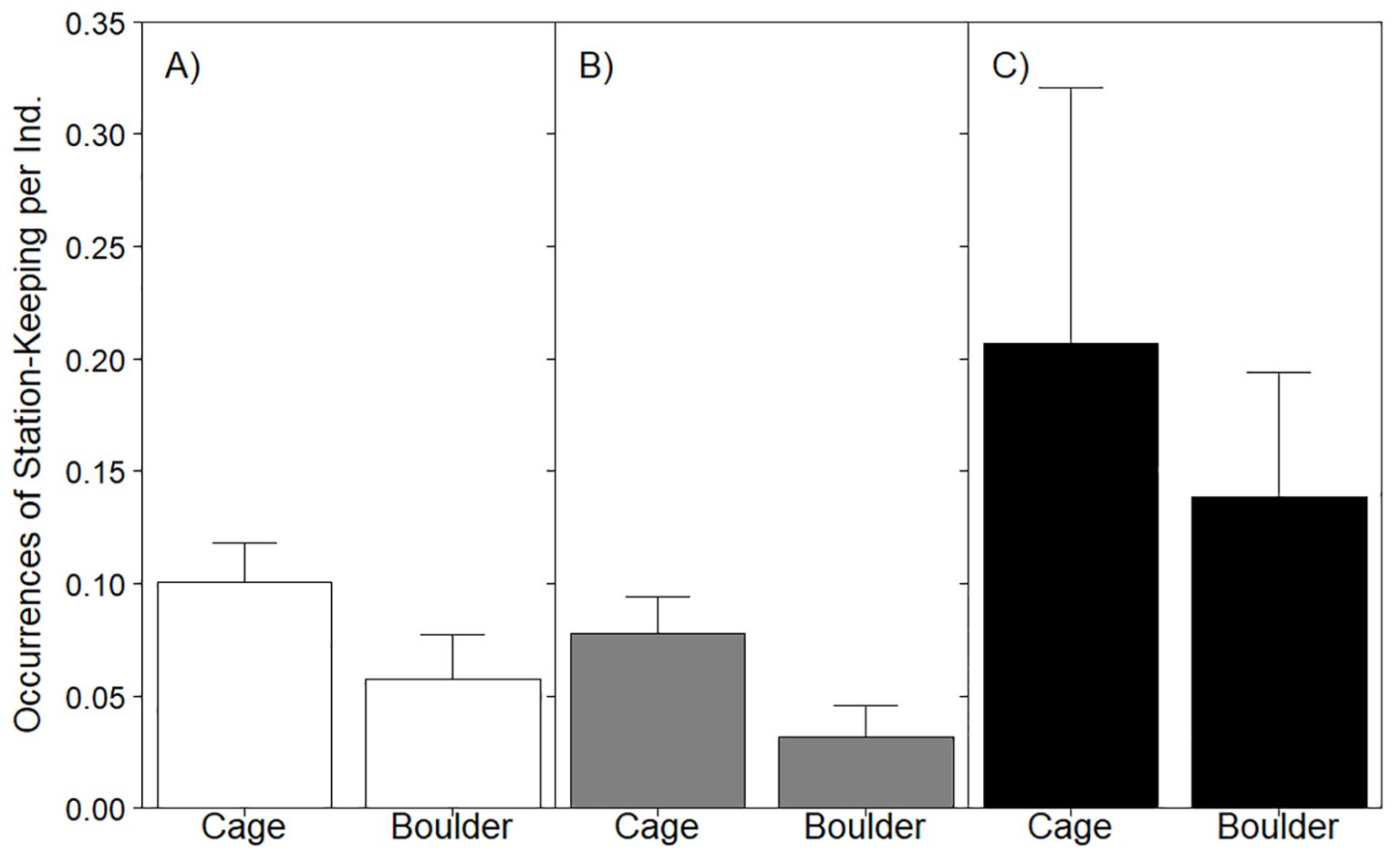
Figure 4. Barplot of mean station-keeping behavior occurrences per sighting displayed by all stages of black sea bass (A) as well as age 1+ (B) and YOY (C) in cage or boulder habitat. Whiskers report standard error. See Table 3 for stage-specific analyses assessing the effect of habitat on this behavior.
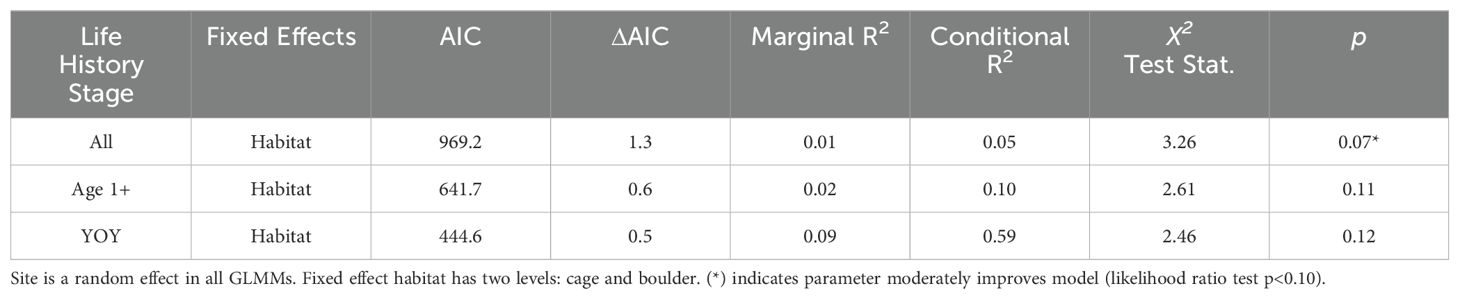
Table 3. Parameters of generalized linear mixed models (GLMM’s) used in analysis of station-keeping behaviors.
Agonistic behavior was the most common aggressive behavior observed (Figure 5). Black sea bass exhibited occurrences of agonistic behaviors more frequently around cages (mean = 3.36 ± 1.38SE) than boulders (mean = 0.18 ± 0.14SE). When behavior occurrence was normalized using abundance, the modeled effects of habitat on agonistic displays was marginally significant and explained nearly 6% of the observed variance (marginal R2 = 0.06; Table 4). The relative rate of agonistic behaviors (normalized using fish abundance) was 3 times greater on cages (0.03 observed instances of behavior per capita) as compared to boulders (0.01 observed instances of behavior per capita). Agonistic behavior in age 1+ fish was analyzed via mixed effects modeling, but there was insufficient data to analyze the habitat effects on this behavior in YOY (limited to 3 cage replicates). Despite the frequency of agonistic displays expressed by age 1+ fish on cages (mean = 2.90 ± 1.25SE); relative to boulders (mean = 0.18 ± 0.14SE), model results indicated that habitat did not affect the occurrence of this behavior (LRT χ2 = 0.68, p = 0.41); in fact, the inclusion of habitat as an explanatory variable did not improve model fit (null model AIC = 317.2; habitat model AIC = 318.5).
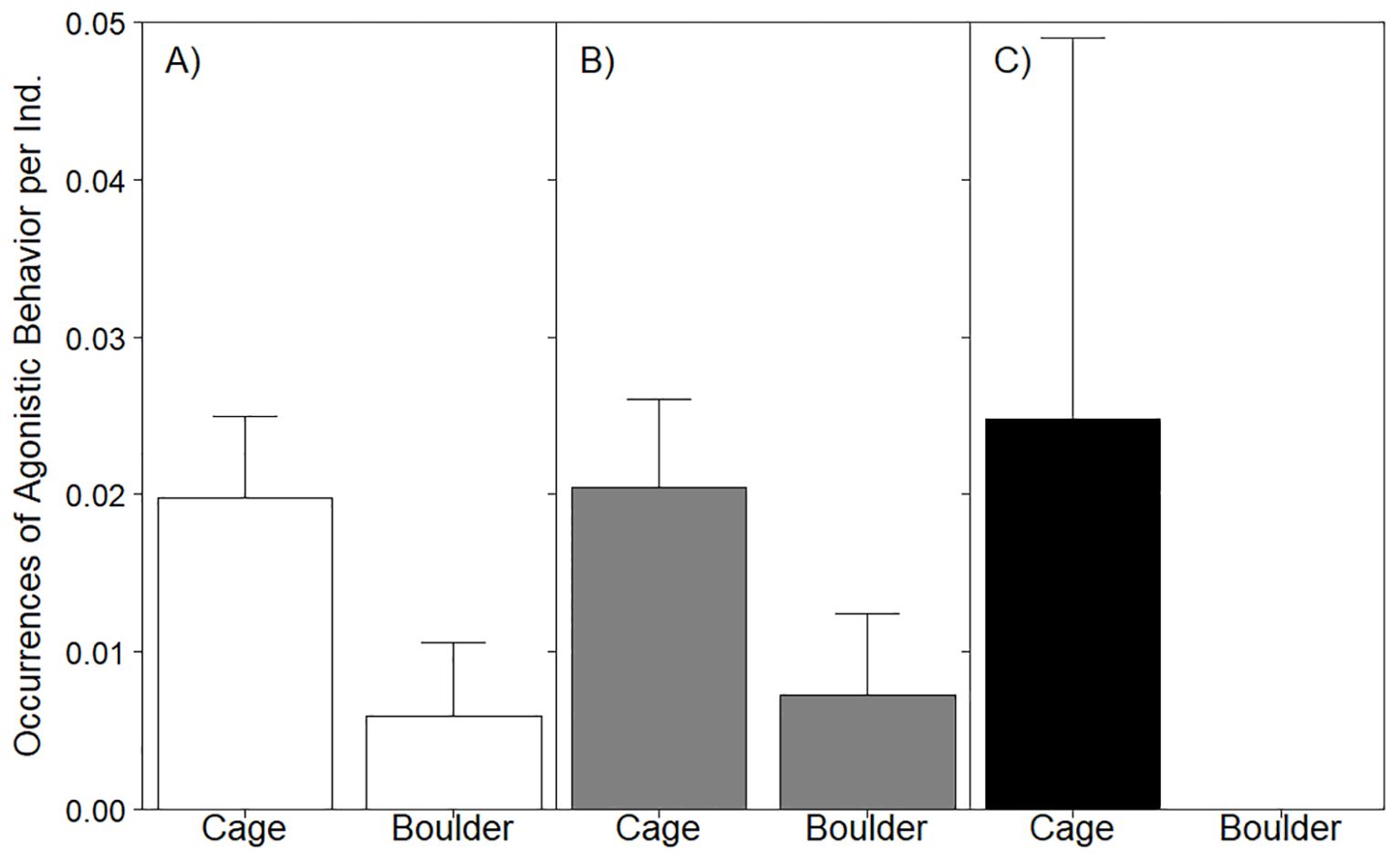
Figure 5. Barplot of mean agonistic behavior occurrences per sighting displayed by all stages of black sea bass (A) as well as age 1+ (B) and YOY (C) in cage or boulder habitat. YOY were not observed expressing agonistic behavior in boulder habitat. Whiskers report standard error. See Table 4 for stage-specific analyses assessing the effect of habitat on this behavior.
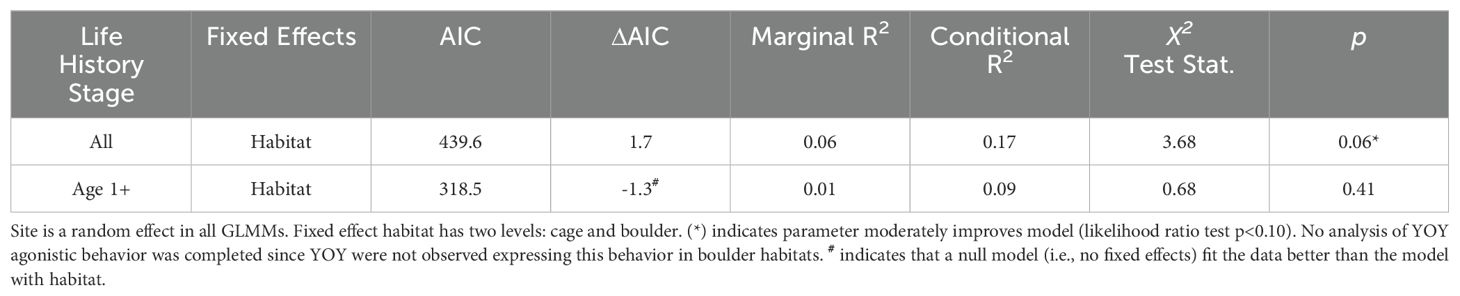
Table 4. Parameters of generalized linear mixed models (GLMM’s) used in analysis of agonistic behaviors.
Other territorial behaviors, such as ambush, were observed less frequently than agonistic displays (Figure 6). Ambushes were observed more often around cages (mean = 0.67 ± 0.34SE) than in boulder habitat (mean = 0.02 ± 0.2SE), where only a single ambush was observed. Models of ambushes by all black sea bass (LRT χ2 = 0.78, p = 0.38; Table 5) and age 1+ fish (LRT χ2 = 0.05, p = 0.82) revealed no significant influence of habitat on occurrence; best fit models in both analyses did not feature habitat (all stages null model AIC = 98.1, habitat model AIC = 99.3; age 1+ fish, null model AIC = 73.9, habitat model AIC = 75.9). No attempt to model YOY ambush behavior was attempted, since ambushes by YOY were limited to 3 occurrences on cages.
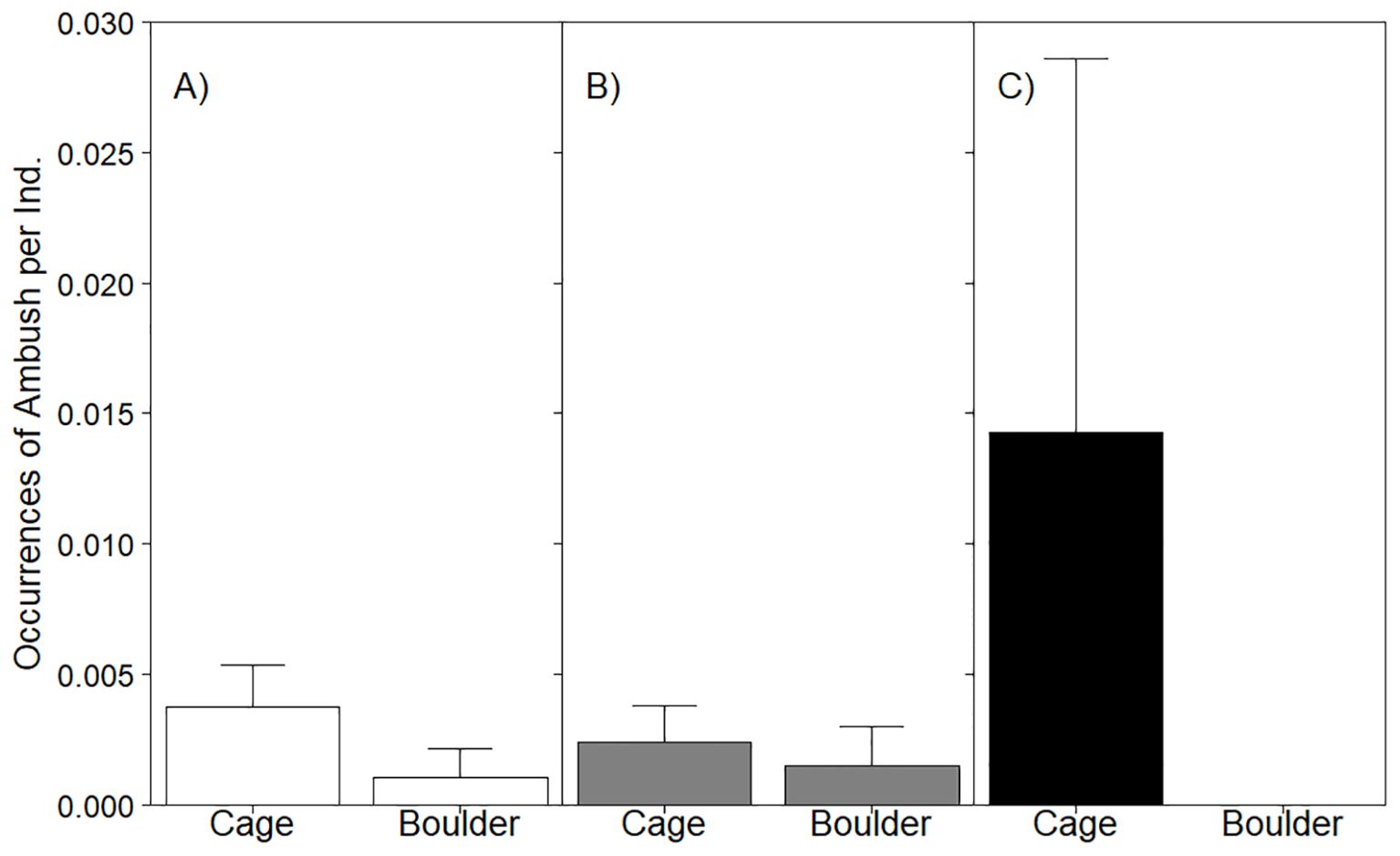
Figure 6. Barplot of mean ambush behavior occurrences per sighting displayed by all stages of black sea bass (A) as well as age 1+ (B) and YOY (C) in cage or boulder habitat. YOY were not observed expressing agonistic behavior in boulder habitat. Whiskers report standard error. See Table 5 for stage-specific analyses assessing the effect of habitat on this behavior.

Table 5. Parameters of generalized linear mixed models (GLMM’s) used in analysis of ambush behaviors.
Displacement behaviors were more frequently observed on cages (mean = 0.44 ± 0.32SE) than on boulders (mean = 0.04 ± 0.03SE) (Figure 7). While efforts to model the effects of habitat on age 1+ fish (null model AIC = 71.4; habitat model AIC = 73.3; Table 6), YOY (null model AIC = 34.8; habitat model AIC = 35.9), and all stages (null model AIC = 85.7; habitat model AIC = 87.7) combined were successful, the best fit model in each analysis did not feature habitat. None of the models featuring habitat detected significant differences in the occurrence of displacement between cage and boulder habitats (age 1+ fish LRT χ2 = 0.11, p = 0.74; YOY LRT χ2 = 0.94, p = 0.33; all stages and age 1+ fish LRT χ2 = 0.03, p = 0.87).
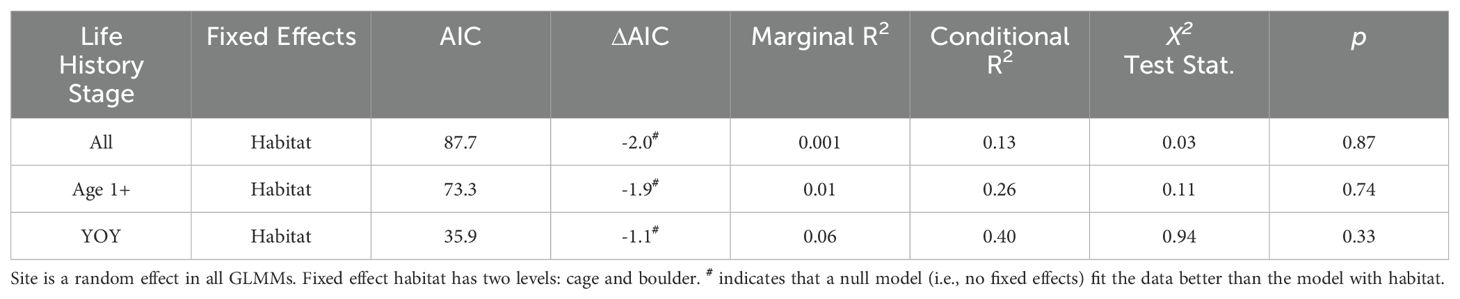
Table 6. Parameters of generalized linear mixed models (GLMM’s) used in analysis of displacement behaviors.
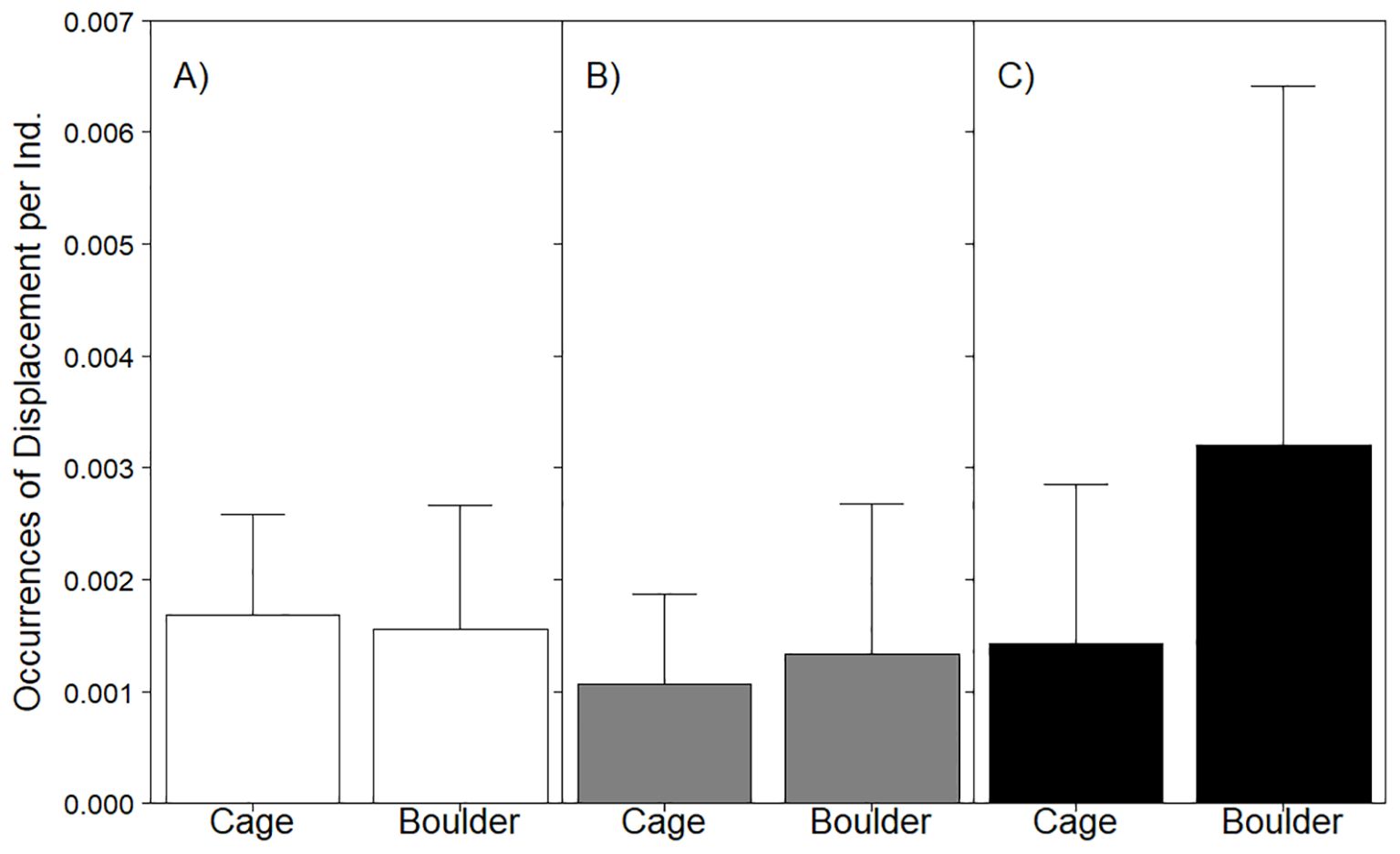
Figure 7. Barplot of mean displacement behavior occurrences per sighting displayed by all stages of black sea bass (A) as well as age 1+ (B) and YOY (C) in cage or boulder habitat. Whiskers report standard error. See Table 6 for stage-specific analyses assessing the effect of habitat on this behavior.
Discussion
Black sea bass were observed at higher abundance on oyster cages at the shellfish farm than around boulders on the rock reef. Sightings of age 1+ black sea bass were an order of magnitude greater on cages than boulders, despite the availability of both habitats well within the expected inshore home range established for this species (13.7 - 736.4 ha; Fabrizio et al., 2014). A previous analysis of this same video data set using the abundance metric MaxN similarly found significantly higher numbers of black sea bass on cages at the farm versus boulders on the reef (Mercaldo-Allen et al., 2023). Fish abundance estimates using MaxN, the maximum number of fish at a single time point, represent a conservative measure of abundance and avoids potential for double counting of fish that swim in and out of camera view (Wilson et al., 2015). While for assessing frequency of fish behaviors, the metric fish sightings accounts for instances where the same fish appears multiple times within a video segment and exhibits more than one behavior.
Placement of aquaculture gear on low relief seafloor may increase available habitat for shelter-oriented species like black sea bass. Introduction of oyster cages may extend the spatial coverage of structured habitats on generally featureless seafloor and may in part replace naturally occurring complex hard bottom environments that have been lost, such as natural oyster reefs (Steimle and Zetlin, 2000; Weigold and Pillsbury, 2014). In Long Island Sound where our study was conducted, natural boulder reef habitats represent a low proportion of the overall seafloor (Mercaldo-Allen et al., 2011). While the present study did not focus on how cages may affect overall black sea bass distribution, it seems unlikely that all the individuals associated with cages would have recruited to the nearby cobble and boulder reef in the absence of aquaculture gear (Tallman and Forrester, 2007). Research is needed to determine whether shellfish farms attract fish from other locations or provide settlement habitat that increases recruitment and enhances fish production.
Black sea bass demonstrated a variety of behaviors at both oyster cages on a shellfish farm and boulders on a rock reef including station-keeping, agonistic displays, ambush, and displacement. Fish demonstrated station-keeping more frequently on oyster cages than on boulders. Black sea bass also appeared to act aggressively towards conspecifics more frequently on cages than on boulders. The observed differences in behavior between these habitats may indicate substantially reduced shelter use requirements for black sea bass that occupy cages, which leads to questions about prey density and resource availability in these habitats. The use of tiered cages in oyster aquaculture may have implications for the distribution of this popular fishery target and the population density that the nearshore oyster farms may be able to support.
Station-keeping, the behavior observed most frequently, was exhibited by fish at multiple life stages, and occurred more often on cages than boulders. When standardized by number of fish sightings, the habitat-specific frequency of station-keeping was marginally significantly different. Black sea bass used small fin movements to maintain their position in the water column on the upper cage surface and on shelves inside cages. The top cage surface provided large flat areas where black sea bass were readily observed resting on their pectoral or pelvic fins, in some cases over an entire 8-minute video segment. Conversely, black sea bass were rarely observed resting on boulder surfaces for extended periods. Underwater video of black sea bass collected off the coast of Maryland showed fish performing similar resting behaviors under high current conditions (Cullen and Stevens, 2017a). These frequent observations of sea bass demonstrating “station-keeping” behavior on cages suggests that aquaculture gear may provide fish with physiological respite and/or a current refuge, perhaps reducing the energetic costs associated with more active swimming (Gerstner, 1998; Auster and Lindholm, 2005). Interestingly, station-keeping often persisted despite the presence of other fish concurrently demonstrating aggressive behaviors on the cage.
Territorial and aggressive behaviors occurred more frequently on cages than on boulders. However, when normalized by the number of fish sightings, the relative differences in the rate of agonistic behaviors between the two habitats were only marginally significant. Male black sea bass commonly use agonistic displays to defend their established territory, likely for courtship and/or spawning activity (Fabrizio et al., 2013; Cullen and Stevens, 2017a; 2017b). Territorial behavior related to reproduction has been observed in many reef fish species (Reebs, 2008; Barneche et al., 2009; LaManna and Eason, 2011), and in male black sea bass residing in complex reef habitats in Mid-Atlantic coastal waters (Cullen and Stevens, 2017a; 2017b). Although the relative frequency of agonistic displays in black sea bass was similar among the two habitats we studied, the types of aggressive behaviors differed. For example, agonistic behaviors displayed by males and to a lesser extent juveniles, including lateral displays, fin flaring, and ambush behaviors, were more common on cages versus boulders. Ambush and displacement behaviors were observed less frequently, perhaps because many subordinate fish responded to agonistic behaviors by withdrawing, and therefore aggressors did not need to chase subordinates out of their territory (Reebs, 2008). We did observe one instance of a juvenile attempting to ambush a small cunner (Tautogolabrus adspersus) from inside a cage. This observation suggests sea bass may be opportunistically hunting for smaller fish within cages. While piscivory is more common among juveniles south of Cape Hatteras (Bowman et al., 2000; Byron and Link, 2010), black sea bass are known to prey on fish above and adjacent to reefs in the southeast United States using reef features as cover to ambush prey (Auster et al., 2013; Campanella et al., 2019). Observations that sea bass behaved more aggressively on cages than boulders, suggests that aquaculture gear provides valuable habitat that fish were willing to actively defend.
Aggression among fish during early life history stages may relate to competition for refuge or access to resources. YOY and juvenile black sea bass are reliant on complex habitats and demonstrate high site fidelity (e.g., Feigenbaum et al., 1989; Drohan et al., 2007; Mercaldo-Allen et al., 2020a; 2020b). Multidimensional structures, such as cages and boulders, can protect small reef fish from predators, provide refuge from high current flow (Auster, 1987, 1989), and provide access to prey (attached organisms that colonize their surfaces and associated small crustaceans; Mercer, 1989; Auster et al., 1996; Drohan et al., 2007). Mortality rates of immature black sea bass are known to be lower on structured versus unstructured seafloor (Scharf et al., 2006), likely due to the shelter and protection from predation provided. In tank experiments, juvenile black sea bass were observed behaving aggressively and even attacking conspecifics in the vicinity of complex hard substrates, likely due to competition for resources (Gwak, 2003, 2008). Similarly, in other laboratory studies, YOY sea bass actively preferred oyster shells to bare sand seafloor, and were observed defending their shell from other fish or attempting to displace another fish from its shell (K. W. Able, unpubl. data in Able and Fahay, 1998). Interestingly, we observed just three instances of aggressive behavior in recently settled YOY black sea bass. This suggests that both cages and boulders provided ample habitat for young fish, possibly reducing aggressive competition for resources among conspecifics.
Beyond providing structurally complex habitat, it remains unclear how oyster cages affect black sea bass access to necessary resources. If the high densities and frequency of aggression observed on cages do correspond with smaller home ranges, then the availability of important resources, such as prey, may be sufficient within a smaller area than those associated with boulder reefs. Epifauna and flora, predominantly the colonial hydroid Campanularia spp. and lacy crust bryozoan Membranipora membranacea, were observed covering the surfaces of the cages in this study (Mercaldo-Allen et al., 2023). Although not quantified here, black sea bass in videos have been anecdotally observed foraging on cages (Mercaldo-Allen et al., 2021; 2023), perhaps consuming attached organisms and/or associated mobile animals. Juvenile black sea bass diets are primarily composed of small benthic and epi-benthic crustaceans (Drohan et al., 2007), which are known to inhabit and utilize introduced aquaculture gear (Marenghi et al., 2010). The spatial complexity and large surface area provided by tiered cages may potentially support greater prey densities than do natural boulder reefs. The role of cages in black sea bass foraging may also change throughout the course of development, as fish become larger and less shelter dependent. In this study, the placement of cages 47.5m apart on natural sediments may have provided black sea bass convenient access to nearby forage grounds. Black sea bass adjacent to cages and boulders were often observed arriving or leaving the habitat, possibly for foraging trips to neighboring fine sediment habitats where preferred prey can be found (Lindquist et al., 1994; Steimle and Figley, 1996; Mercaldo-Allen et al., 2020a). More study is needed to assess the ecosystem services provided by aquaculture cages to black sea bass and other fishes beyond their structural complexity.
Density is a commonly used proxy for habitat value under the assumption that the spatial distribution of individuals across a landscape can reflect the availability and abundance of resources (Fretwell and Lucas, 1970). However, using site specific density observed over a short period of time alone to infer habitat quality may be misleading, since density may be influenced by other factors including the size of established territories, overall population size, and recruitment. For instance, density may remain constant over time even as population abundance increases due to the ability of individual fish to defend territories aggressively (Fretwell and Lucas 1970). If this is the case, then density may in part reflect the ability of fish to maintain larger territories rather than resource density. Clarifying the relationship between fish density and realized habitat quality on oyster cages and boulders would require some measure of condition in the observed black sea bass, assessment of prey abundance, predation rates, or other resources in each habitat, or additional observations of density over a greater period of time. Aggression and territoriality in tropical reef fish can be strongly influenced by conspecific density (e.g., three-spot damselfish Segastes planifrons, Levin et al., 2000). During our study, black sea bass were observed behaving aggressively towards conspecifics around cages and boulders, approaching what appeared to be subordinate males in close proximity. Subordinate responses to territorial displays serve to limit aggressive interactions, maintain established territory and dominance without costly and dangerous direct physical interactions (Rasa, 1976; Schrandt et al., 2012). Many times, cages were occupied by multiple fish, and aggressors would limit subordinate intrusion into these areas by demonstrating warning behaviors included in agonistic displays.
Resource availability and habitat quality can also play an important role in the spatial distributions and behaviors of territorial species (Maher and Lott, 2000). Although measuring habitat quality in the absence of this information may not be possible, habitat specific rates of aggressive behavior can provide some insights. Spatial distribution (parrotfishes, Scarus spp. and Sparisoma spp.; Dubin, 1981), type (bluehead wrasse Thalassoma bifasciatum; Tecumseh et al., 1990), and quantity of food resources (whitefin damselfish Pomacentrus albicaudatus; Fricke, 1977) influence territoriality in fish, with aggression increasing in response to prey density, quality, and abundance. Availability of refuge also influences territorial behaviors in tropical reef fish, such as the green razorfish Xyrichtys splendens (Nemtzov, 1997), which more vigorously defends those areas having more refuge. Although increased territoriality is generally associated with higher quality habitat, more complex structures can also be associated with less agonistic behavior among certain tropical fish species (e.g., black triggerfish Melichthys niger; Kavanagh and Olney, 2006). The fitness benefits of aggressive activity may decline when resources are plentiful (reviewed by Grant, 1993). Research on fish territoriality has largely focused on obligate reef species in tropical systems whose ecology and life histories differ in important ways from the relatively large, temperate black sea bass that seasonally migrate off the northeast coast. The territorial reef fish in temperate waters that have been the subject of behavioral studies do vary in density with habitat complexity (e.g., gag grouper; Burge et al., 2012), but connections between density and habitat quality have not been clearly established. Despite this caveat, the greater number of black sea bass across life stages observed, more frequent instances of aggressive behavior among fish on and around cages, and similar relative rates of aggression when behavior is normalized to fish sightings between habitats, suggest that aquaculture gear provides this species with habitat of similar quality to that afforded by natural structured seafloors.
Conclusions
We observed more sightings of black sea bass on cages versus boulders, with a similar relative frequency of territorial and occupancy behaviors demonstrated by fish on both cages and boulders, suggesting that cages on shellfish farms may provide ecological benefits for this species similar to that afforded by natural boulder seafloor. Cages and boulders both provided habitat for black sea bass across life history stages. For this reason, essential fish habitat descriptions of manmade structures used by black sea bass could be broadened to include aquaculture gear. Our study provides novel information on behavioral interactions of black sea bass with oyster aquaculture cages that may support the essential fish habitat consultation and aquaculture permitting processes.
Data availability statement
The raw data supporting the conclusions of this article will be made available by the authors, without undue reservation.
Ethics statement
Ethical approval was not required for the study involving animals in accordance with the local legislation and institutional requirements because the research subjects were filmed remotely during regular oyster cage operations. No black sea bass were handled, collected, or disturbed during this study.
Author contributions
AA: Writing – review & editing, Writing – original draft, Visualization, Validation, Software, Methodology, Investigation, Formal analysis, Data curation, Conceptualization. RM-A: Writing – review & editing, Writing – original draft, Validation, Supervision, Software, Resources, Project administration, Methodology, Investigation, Funding acquisition, Formal analysis, Data curation, Conceptualization. JR: Writing – review & editing, Writing – original draft, Validation, Supervision, Software, Resources, Project administration, Methodology, Investigation, Funding acquisition, Formal analysis, Data curation, Conceptualization. KS: Writing – review & editing. PC: Writing – review & editing, Validation, Software, Methodology, Investigation, Formal analysis, Data curation, Conceptualization. GP: Writing – review & editing, Software, Methodology, Investigation, Data curation, Conceptualization. DR: Writing – review & editing, Software, Methodology, Investigation, Data curation, Conceptualization. CC: Writing – review & editing, Writing – original draft, Visualization, Validation, Supervision, Software, Methodology, Investigation, Formal analysis, Data curation, Conceptualization.
Funding
The author(s) declare financial support was received for the research, authorship, and/or publication of this article. We thank NOAA’s Northeast Fisheries Science Center and Office of Aquaculture for funding.
Acknowledgments
Field operations were conducted aboard the Milford Laboratory’s 15-m NOAA R/V Victor Loosanoff. We thank David Carey, Kristin DeRosia-Banick and Shannon Kelly of the State of Connecticut, Bureau of Aquaculture, Jim Bloom and Rachel Precious of Copps Island Oysters and James Markow of Noank Aquaculture Cooperative for the loan of seed oysters and deployment assistance, shellfish growers Gary Salce of G & B Shellfish and Charles Viens of Charles Island Oyster Farms for access to leased shellfish beds, NOAA divers Calandrea DeCastro, Mark Dixon, Keith Golden, Jerry Prezioso and Barry Smith for dive support, Erick Estela, Yuan Liu, Peter Hudson, Deaven Maull, and Max Mauro for support of field operations, Peter Auster for his review of the manuscript and NOAA’s Northeast Fisheries Science Center and Office of Aquaculture for funding.
Conflict of interest
Author GP was employed by the company A.I.S. Inc.
The remaining authors declare that the research was conducted in the absence of any commercial or financial relationships that could be construed as a potential conflict of interest.
Publisher’s note
All claims expressed in this article are solely those of the authors and do not necessarily represent those of their affiliated organizations, or those of the publisher, the editors and the reviewers. Any product that may be evaluated in this article, or claim that may be made by its manufacturer, is not guaranteed or endorsed by the publisher.
References
Able K. W., Fahay M. P. (1998). The first year in the life of estuarine fishes in the Middle Atlantic Bight (New Jersey: Rutgers University Press).
Able K. W., Fahay M. P., Shepherd G. R. (1995). Early life history of black sea bass, Centropristis striata, in the mid-Atlantic Bight and New Jersey estuary. Fish Bull. 93, 429–445. Available at: https://spo.nmfs.noaa.gov/content/early-life-history-black-sea-bass-centropristis-striata-mid-atlantic-bight-and-new-Jersey.
Archer A., Reitsma J., Murphy D. (2014). A comparison of bottom and floating gear for growing American oysters (Crassostrea virginica) in southeastern Massachusetts. Mar. Ext Bull, 4pp. Available at: https://www.capecodextension.org/wp-content/uploads/2013/02/Comparison-of-Bottom-and-Floating-Gear-for-Growing-American-Oysters-in-SE-Mass.pdf.
Arendt M. D., Lucy J., Munroe T. A. (2001). Seasonal occurrence and site-utilization patterns of adult tautog, Tautoga onitis (Labridae), at manmade and natural structures in lower chesapeake ba). Fishery Bull. 99 (4), 519–527. https://scholarworks.wm.edu/vimsarticles/582.
Atlantic States Marine Fisheries Commission. (2021). species - atlantic states marine fisheries commission. Available online at: http://www.asmfc.org/species/black-sea-bass (Accessed May 1, 2020). www.asmfc.org.
Auster P. J., Lindholm J. (2005). “The ecology of fishes on deep boulder reefs in the western gulf of maine. in: Diving for science,” in Proceedings of the american academy of underwater sciences (Groton, CT: Connecticut Sea Grant), p.89–p107.
Auster P. J. (1987). The effect of current speed of the small scale spatial distribution of fishes. NOAA Symp Ser. Undersea Res. 2, 7–16.
Auster P. J. (1989). “Species profiles: life histories and environmental requirements of coastal fishes and invertebrates (North Atlantic and Mid-Atlantic)—tautog and cunner,” in US Fish Wildlife Serv Biol Rep, 82(11.105), US Army Corps of Engineers, Vicksburg, MS, TR EL-82-4.
Auster P. J., Kracker L., Price V., Heupel E., McFall G., Grenda D. (2013). ‘Behavior webs of piscivores at subtropical live-bottom reefs’. Bull. Mar. Sci. 89, 377–396. doi: 10.5343/bms.2011.1123
Auster P. J., Malatesta R. J., LaRosa S. C. (1996). Patterns of microhabitat utilization by mobile megafauna on the southern New England (USA) continental shelf and slope. Mar. Ecol. Prog. Ser. 127, 77–85. doi: 10.3354/meps127077
Barneche D. R., Floeter S. R., Ceccarelli D. M., Frensel D. M. B., Dinslaken D. F., Mário H. F. S., et al. (2009). Feeding macroecology of territorial damselfishes (Perciformes: Pomacentridae). Mar. Biol. 156, 289–299. doi: 10.1007/s00227-008-1083-z
Black A., Imhoff V., Leese J., Weimann S., Gumm J., Richter M., et al. (2014). Attack intensity by two species of territorial damselfish (Pomacentridae) as estimates of competitive overlap with two species of wrasse (Labridae). J. Ethol 32, 623–668. doi: 10.1007/s10164-013-0393-x
Bowman R. E., Stillwell C. E., Michaels W. L., Grosslein M. D. (2000). Food of Northwest Atlantic fishes and two common species of squid. Available online at: https://repository.library.noaa.gov/view/noaa/3140.
Buckman N. S., Ogden J. C. (1973). Territorial behavior of the striped parrotfish scarus croicensis bloch (Scaridae). Ecol 54, 1377–1382. doi: 10.2307/1934202
Burge E. J., Atack J. D., Andrews C., Binder B. M., Hart Z. D., Wood A. C., et al. (2012). Underwater video monitoring of groupers and the associated hard-bottom reef fish assemblage of north carolina. Bull. Mar. Sci. 88 (1), 15–38. doi: 10.5343/bms.2010.1079
Byron C. J., Link J. S. (2010). Stability in the feeding ecology of four demersal fish predators in the US Northeast Shelf Large Marine Ecosystem. Mar. Ecol. Prog. Ser. 406, 239–250. doi: 10.3354/meps08570
Campanella F., Auster P. J., Taylor J. C., Muñoz R. C. (2019). ‘Dynamics of predator-prey habitat use and behavioral interactions over diel periods at sub-tropical reefs’. PloS One 14, e0211886. doi: 10.1371/journal.pone.0211886
Collette B. B., Klein-MacPhee G. (Eds.) (2002). Bigelow and schroeder’s fishes of the gulf of maine (Washington, DC: Smithsonian Institution Press), 748.
Cullen D., Stevens B. (2017a). Use of an underwater video system to record observations of black sea bass (Centropristis striata) in the waters off the coast of Maryland. Fish Bull. 115, 408–418. Available at: https://spo.nmfs.noaa.gov/content/fishery-bulletin/use-underwater-video-system-record-observations-black-sea-bass.
Cullen D. W., Stevens B. G. (2017b). Application of systematic adaptive cluster sampling for the assessment of black sea bass Centropristis striata abundance. Fish Sci. 83, 671–682. doi: 10.1007/s12562-017-1116-y
DeAlteris J. T., Kilpatrick B. D., Rheault R. B. (2004). A comparative evaluation of the habitat value of shellfish aquaculture gear, submerged aquatic vegetation and a non-vegetated seabed. J. Shellfish Res. 23, 867–874. Available at: https://digitalcommons.uri.edu/favs_facpubs/26/.
Drohan A. F., Manderson J. P., Packer D. B. (2007). Essential fish habitat source document: Black Sea Bass, Centropristis striata, life history and habitat characteristics second edition’, United States Department of Commerce, NOAA. Available online at: https://repository.library.noaa.gov/view/noaa/4038.
Dubin R. E. (1981). Social behaviour and ecology of some Caribbean parrotfish (Scaridae) (Edmonton: University of Alberta).
Egli D. P., Babcock R. C. (2004). Ultrasonic tracking reveals multiple behavioural modes of snapper (Pagrus auratus) in a temperate no-take marine reserve. ICES J. MarSci 61, 1137–1143. doi: 10.1016/j.icesjms.2004.07.004
Erbland P. J., Ozbay G. (2008). A comparison of the macrofaunal communities inhabiting a Crassostrea virginica oyster reef and oyster aquaculture gear in the Indian River Bay, Delaware. J. Shellfish Res. 17, 757–768. doi: 10.2983/0730-8000(2008)27[757:ACOTMC]2.0.CO;2
Fabrizio M. C., Manderson J. P., Pessutti J. P. (2013). Habitat associations and dispersal of black sea bass from a mid-Atlantic Bight reef. Mar. Ecol. Prog. Ser. 482, 241–253. doi: 10.3354/meps10302
Fabrizio M. C., Manderson J. P., Pessutti J. P. (2014). Home range and seasonal movements of the Black Sea Bass (Centropristis striata) during their inshore residency at a reef in the mid-Atlantic Bight. Fish Bull. 112, 82–97. Available at: https://spo.nmfs.noaa.gov/content/home-range-and-seasonal-movements-black-sea-bass-centropristis-striata-during-their-inshore.
Feigenbaum D., Bushing M., Woodward J., Friedlander A. (1989). Artificial reefs in Chesapeake Bay and nearby coastal waters. Bull. Mar. Sci. 44, 734–742. Available at: https://www.researchgate.net/publication/233640631_Artificial_Reefs_in_Chesapeake_Bay_and_Nearby_Coastal_Waters.
Fretwell S. D., Lucas H. L. (1970). On territorial behavior and other factors influencing habitat distribution in birds. I. Theor. Dev. Acta Biotheoretica 19, 16–36. doi: 10.1007/BF01601953
Fricke H. W. (1977). Community structure, social organization and ecological requirements of coral reef fish (Pomacentridae). Helgoländer Wiss Meeresunters 30, 412–426. doi: 10.1007/BF02207851
Gerstner C. L. (1998). Use of substratum ripples for flow refuge by Atlantic cod Gadus morhua. Environ. Biol. Fishes 51, 455–460. doi: 10.1023/A:1007449630601
Getchis T. S. (2005). An assessment of the needs of Connecticut’s shellfish aquaculture industry (Groton, CT: Connecticut Sea Grant Publication, CTSG-05-02), 1–12. Available at: https://seagrant.uconn.edu/2005/01/01/shellfish-aquaculture-industryassessment/.
Gilmore R. G., Jones R. S. (1992). Color variation and associated behavior in the epinepheline grouper Mycteroperca microlepis (Goode andBean) and M. phenax (Jordan and swain). Bull. Mar. Sci. 51, 83–103.
Grant J. W. A. (1993). Whether or not to defend? The influence of resource distribution. Mar. Behav. Physiol. 23, 137–153. doi: 10.1080/10236249309378862
Gwak W. S. (2003). Effects of shelter on growth and survival in age-0 black sea bass, Centropristis striata (L.). Aquac Res. 34, 1387–1390. doi: 10.1111/j.1365-2109.2003.00956.x
Gwak W. (2008). Behavior of juvenile black sea bass, Centropristis striata (Linnaeus) on oyster reefs. Korean J. Ichthyol 20, 173–178. Available at: https://www.semanticscholar.org/paper/Behavior-of-Juvenile-Black-Sea-Bass%2C-Centropristis-Gwak/ee00740c154dc0d45ee9fc9d1ea7efefe5eeb2fd.
Kavanagh K. D., Olney J. E. (2006). Ecological correlates of population density and behavior in the circumtropical black triggerfish Melichthys Niger (Balistidae). Environ. BiolFishes 76, 387–398. doi: 10.1007/s10641-006-9044-1
LaManna J. R., Eason P. K. (2011). Effects of fighting on pairing and reproductive success. Behaviour 148, 89–102. Available at: https://www.jstor.org/stable/25799799.
Levin P. S., Stunz G. W. (2005). Habitat triage for exploited fishes: Can we identify essential “Essential Fish Habitat. Estuar Coast. Shelf Sci. 64, 70–78. doi: 10.1016/j.ecss.2005.02.007
Levin P. S., Tolimieri N., Nicklin M., Sale P. F. (2000). Integrating individual behavior and population ecology: the potential for habitat-dependent population regulation in a reef fish. Behav. Ecol. 11, 565–571. doi: 10.1093/beheco/11.5.565
Lindquist D. G., Cahoon L. B., Clavijo I. E., Posey M. H., Bolden S. K., Pike L. A., et al. (1994). Reef fish stomach contents and prey abundance on reef and sand substrata associated with adjacent artificial and natural reefs in Onslow Bay. North Carolina. Bull. Mar. Sci. 55, 308–318. Available at: https://www.ingentaconnect.com/content/umrsmas/bullmar/1994/00000055/F0020002/art00005.
MAFMC. (1999). Amendment 12 to the summer flounder, scup, and black sea bass fishery management plan. Available online at: www.mafmc.org (Accessed July 17, 2024).
Maher C. R., Lott D. F. (2000). A review of ecological determinants of territoriality within vertebrate species. Am. Midl Natt 143, 1–29. Available at: https://www.jstor.org/stable/3082980.
Marenghi F., Ozbay G., Erbland P., Rossi-Snook K. (2010). A comparison of the habitat value of sub-tidal and floating oyster (Crassostrea virginica) aquaculture gear with a created reef in delaware's inland bays, USA. Aquaculture Int. 18, 69–81. doi: 10.1007/s10499-009-9273-3
Martel G., Green J. M. (1987). Differential spawning success among territorial male cunners, Tautogolabrus adspersus (Labridae). Copeia 3, 643–648. doi: 10.2307/1445656
McCormick M. I., Weaver C. J. (2012). ‘It pays to be pushy: intracohort interference competition between two reef fishes’. PloS One 7, e42590. doi: 10.1371/journal.pone.0042590
Medeiros P. R., Souza A. T., Ilarri M. I. (2010). Habitat use and behavioural ecology of the juveniles of two sympatric damselfishes (Actinopterygii: Pomacentridae) in the south-western Atlantic Ocean. J. Fish Biol. 77, 1599–1615. doi: 10.1111/j.1095-8649.2010.02795.x
Mercaldo-Allen R., Auster P. J., Clark P., Dixon M. S., Estela E., Liu Y., et al. (2023). ‘Oyster aquaculture cages provide fish habitat similar to natural structure with minimal differences based on farm location’. Front. Mar. Sci. 10. doi: 10.3389/fmars.2023.1058709
Mercaldo-Allen R., Clark P., Liu Y., Meseck S., Milke L., Redman D. (2020a). Macrofaunal assemblages on oyster aquaculture and rock reef habitat in Long Island Sound. North Amer J. Aquacult 82, 92–100. doi: 10.1002/naaq.10127
Mercaldo-Allen R., Clark P., Liu Y., Phillips G., Redman D., Auster P. J., et al. (2021). Exploring video and eDNA metabarcoding methods to assess oyster aquaculture cages as fish habitat. Aqua Environ. Interacts 13, 277–294. doi: 10.3354/aei00408
Mercaldo-Allen R., Clark P., Redman D., Liu Y., Meseck S., Milke L., et al. (2020b). Temperature-related changes in species composition of juvenile finfish on a rock reef in Long Island Sound. Fish Bull. 118, 275–283. doi: 10.7755/FB.118.3.6
Mercaldo-Allen R., Goldberg R., Clark P. E., Kuropat C. A. (2011). Observations of Juvenile Lobsters, Homarus americanus, on a Rock-Reef in Long Island Sound. Northeast Nat. 18, 45–60. Available at: https://www.jstor.org/stable/41315935.
Mercer L. P. (1989). Species profiles: life histories and environmental requirements of coastal fishes and invertebrates (South Atlantic)–black sea bass. U.S. Fish Wildl Serv. Biol. Rep. 82, 16. U.S. Army Corps of Engineers, TR EL-82-4.
Miller A. S., Shepherd G. R., Fratantoni P. S. (2016). Offshore habitat preference of overwintering juvenile and adult black sea bass, Centropristis striata, and the relationship to year-class success. PloS One 11, e0147627. doi: 10.1371/journal.pone.0156355
Muething K. A., Tomas F., Waldbusser G., Dumbauld B. R. (2020). On the edge: assessing fish habitat use across the boundary between Pacific oyster aquaculture and eelgrass in Willapa Bay Vol. 12 (Washington, USA: Aqua Environ Interact), 541–557. doi: 10.3354/aei00381
Nakagawa S., Schielzth H. (2013). A general and simple method for obtaining R2 from generalized linear mixed-effects models. Methods Ecol. Evol. 4, 133–142. doi: 10.1111/j.2041-210x.2012.00261.x
Nemtzov S. C. (1997). Intraspecific variation in home range exclusivity by female green razorfish, Xyrichtys splendens (family Labridae), in different habitats. Environ. Biol. Fishes 50, 371–381. doi: 10.1023/A:1007319622028
Rasa O. A. E. (1976). Aggression: appetite or aversion? – an ethologist’s viewpoint. Aggression Behav. 2, 213–222. doi: 10.1002/1098-2337(1976)2:3%3C213::AID-AB2480020306%3E3.0.CO;2-4
Reebs S. G. (2008). Aggression in fishes (Canada: Université de Moncton). Available at: www.howfishbeave.ca.
Scaia M. F., Morandini L., Anogeura C. A., Ramallo M. R., Somoza G. M., Pandolfi M. (2018). Fighting cichlids: Dynamic of intrasexual aggression in dyadic agonistic encounters. Behav. Processes 147, 61–69. doi: 10.1016/j.beproc.2017.12.015
Scharf F. S., Manderson J. P., Fabrizio M. C. (2006). The effects of seafloor habitat complexity on survival of juvenile fishes: Species-specific interaction with structural refuge. J. Exper Mar. Biol. Ecol. 335, 167–176. doi: 10.1016/j.jembe.2006.03.018
Schrandt M. N., Hardy K. M., Johnson K. M., Lema S. C. (2012). Physical habitat and social conditions across a coral reef shape spatial patterns of intraspecific behavioral variation in a demersal fish. Mar. Ecol. 33, 149–164. doi: 10.1111/j.1439-0485.2011.00475.x
Scuderi B., Chen X. (2019). Production efficiency in New England’s oyster aquaculture industry. Aquac Econ Manag 23, 45–64. doi: 10.1080/13657305.2018.1449272
Shinn J. P., Munroe D. M., Rose J. M. (2021). A fish’s-eye-view: accessible tools to document shellfish farms as marine habitat in New Jersey, USA. Aquacult. Environ. Interact. 13, 295–300. doi: 10.3354/aei00407
Steimle F. W., Figley W. (1996). The importance of artificial reef epifauna to Black Sea Bass diets in the Middle Atlantic Bight. N Am. J. Fish Manag 16, p433–p439. doi: 10.1577/1548-8675(1996)016%3C0433:TIOARE%3E2.3.CO;2
Steimle F. W., Zetlin C. (2000). Reef habitats in the Middle Atlantic Bight: Abundance, distribution, associated biological communities, and fishery resource use. Mar. Fish Rev. 62, 24–42. Available at: http://roa.midatlanticocean.org/project/steimle-and-zetlin-2000-reef-habitats-in-the-middle-atlantic-bight-abundance-distribution-associated-biological-communities-and-fishery-resource-use/.
Tallman J. C., Forrester G. E. (2007). Oyster grow-out cages function as artificial reefs for temperate fishes. Trans. Amer Fish Soci 136, p790–p799. doi: 10.1577/T06-119.1
Tecumseh W., Fitch W. T. S., Shapiro D. Y. (1990). Spatial dispersion and nonmigratory spawning in the bluehead wrasse (Thalassoma bifasciatum). Ethology 85, 199–211. doi: 10.1111/j.1439-0310.1990.tb00400.x
Theuerkauf S. J., Barrett L. T., Alleway H. K., Costa-Pierce B. A., St. Gelais A., Jones R. C. (2021). Habitat value of bivalve shellfish and seaweed aquaculture for fish and invertebrates: Pathways, synthesis and next steps. Rev. Aquac. 14, 54–72. doi: 10.1111/raq.12584
Wantanabe W. O. (2011). Species profile: black sea bass (Stoneville, MS: SRAC Publication No. 7207), p16.
Weigold M. E., Pillsbury E. (2014). “Long Island Sound: a socioeconomic perspective,” in Long island sound (Springer, New York, NY), 1–46.
Keywords: black sea bass, agonistic behavior, oyster aquaculture cages, rock reef, occupancy behavior, essential fish habitat
Citation: Armbruster AD, Mercaldo-Allen R, Rose JM, Seda K, Clark P, Phillips G, Redman D and Conroy CW (2024) Territorial and occupancy behavior of black sea bass on oyster aquaculture gear and boulder habitat. Front. Mar. Sci. 11:1380484. doi: 10.3389/fmars.2024.1380484
Received: 13 February 2024; Accepted: 31 July 2024;
Published: 30 August 2024.
Edited by:
James Morley, East Carolina University, United StatesReviewed by:
David Bryan, National Oceanic and Atmospheric Administration, United StatesJosé Lino Vieira De Oliveira Costa, University of Lisbon, Portugal
Copyright © 2024 Armbruster, Mercaldo-Allen, Rose, Seda, Clark, Phillips, Redman and Conroy. This is an open-access article distributed under the terms of the Creative Commons Attribution License (CC BY). The use, distribution or reproduction in other forums is permitted, provided the original author(s) and the copyright owner(s) are credited and that the original publication in this journal is cited, in accordance with accepted academic practice. No use, distribution or reproduction is permitted which does not comply with these terms.
*Correspondence: Adam D. Armbruster, aarmb15@gmail.com