- Institute of Breast Health Medicine, State Key Laboratory of Biotherapy, West China Hospital, Sichuan University and Collaborative Innovation Center, Chengdu, Sichuan, China
Breast cancer is a major threat to women’s health worldwide. Although the 5-year survival rate is relatively high, treating recurrent and metastatic breast cancer remains challenging. Existing anticancer drugs are often accompanied by adverse reactions; thus, there is an urgent need to explore safer and more effective treatment options. Marine natural compounds, especially alkaloids, are considered to be a potential treasure trove of new anticancer drugs due to their unique chemical structure and wide range of biological activities. A variety of marine alkaloids against breast cancer, including ecteinascidins, halichondrins, manzamines, and trabectedins, have opened new avenues for breast cancer treatment by employing multiple mechanisms, such as inducing cell apoptosis and autophagy, blocking cell cycle, inhibiting angiogenesis, targeting oncogene pathways, and inhibiting metastasis and invasion. Currently, Yondelis (trabectedin) has completed phase II clinical trials in patients with breast cancer and has shown certain efficacy. However, the clinical application of marine alkaloids still needs further research and development. This article deeply explores the mechanism of action of marine alkaloids against breast cancer and anticipates their clinical application prospects. With the deepening of research and the advancement of development, marine alkaloids are expected to bring new breakthroughs in breast cancer treatment.
Introduction
More than 2.3 million cases of breast cancer occur annually, and breast cancer has surpassed lung cancer as the leading cause of cancer worldwide, accounting for 11.7% of all cancer cases (Sung et al., 2021). It is estimated that more than 680,000 people died from breast cancer in 2020, making it the primary cause of cancer deaths among women (Sung et al., 2021). Breast cancer mortality is markedly different due to differences in access to diagnosis, treatment, and palliative care. To eliminate inequalities in breast cancer outcomes, the World Health Organization launched a Global Breast Cancer Initiative (GBCI) in 2021, aiming to reduce breast cancer by 2.5% per year through health promotion and early detection, timely diagnosis, and comprehensive breast cancer management (Trapani et al., 2022). Currently, the mainstay of treatment for breast cancer is surgical resection and chemotherapy. For patients with advanced metastatic breast cancer, radiotherapy and/or chemotherapy alone is usually used, with a 5-year survival rate of less than 30% (Burguin et al., 2021; Miller et al., 2022). However, chemotherapy drugs are usually highly toxic. At the same time, traditional drug preparations lack tumor-targeting activity, which results in only a few drugs being able to effectively target tumor tissues, leading to low drug utilization (Mayer, 2013). Therefore, there is an urgent need to discover and develop new safe and effective anticancer drugs.
Natural compounds have emerged as essential sources of novel pharmaceuticals. These compounds, derived from diverse natural resources, exhibit a remarkable chemical variety and thus demonstrate an astonishing diversity of pharmacological processes (Hussain et al., 2023). Oceans, which cover more than 70% of the Earth’s surface and account for 95% of the entire biosphere, stand as a vast and invaluable natural treasure trove of compounds (Hussain et al., 2023). Over 30,000 marine natural products have been isolated from the aquatic world (Lyu et al., 2021). These organisms, along with their secondary metabolites, present attractive structures and a wide range of biological activities that may be useful for finding drugs with greater efficacy and specificity for treating many human diseases (Proksch et al., 2002). According to the US Food and Drug Administration (FDA) (https://www.fda.gov) and the Australian Therapeutic Goods Administration (TGA) (https://www.tga.gov.au), as of March 2024, the FDA or TGA has approved 15 marine-derived drugs, 10 of which are used in cancer treatments. Ara-C (cytarabine) isolated from Caribbean sponges Cryptotheca crypta, which is the first marine drug, received FDA approval in 1969 to treat leukemia (Glaser and Mayer, 2009). Eribulin mesylate is a synthetic analog of the marine natural product halichondrin B, which was approved by the FDA in 2010 for the treatment of metastatic breast cancer (Huyck et al., 2011). Trabectedin, a marine-derived alkylating agent from tunicates, underwent a phase II clinical trial in patients with progressive breast cancer and was approved for use in soft tissue sarcoma and ovarian cancer (Zelek et al., 2006; Barone et al., 2017; Gadducci and Cosio, 2022).
There are still many marine secondary metabolites undergoing preclinical studies, of which alkaloids are among the most diverse and studied compounds. Marine alkaloids are alkaline natural products with important biological activities, including amine nitrogen functional groups and complex carbon skeleton ring structures (Zhou et al., 2021). In the past decade of research, it has shown extraordinary pharmacological potential due to its anti-tumor ability, not only showing significant effects in the treatment of breast cancer, but also showing potential therapeutic value in other types of malignant tumors, such as pancreatic cancer and mesothelioma (Hoda et al., 2016; Li Petri et al., 2020; Fernandes et al., 2022; Carbone et al., 2023; Hussain et al., 2023). These alkaloids mainly act on different tumor cells through various mechanistic pathways, such as cytotoxicity, antiproliferation, apoptosis, cell cycle arrest, and autophagy, to inhibit tumor progression (Figure 1). Rehman et al. compiled a review of the anticancer bioactivity of marine-derived compounds and their potential mechanisms (Wali et al., 2019) and Bhubalan et al. reviewed the potential applications of natural products synthesized by sponge-associated microorganisms in medicine and other industries (Amelia et al., 2022). Both reviews describe the anti-breast cancer activity exhibited by marine derivatives. In 2011, Gali-Muhtasib et al. published a review on anticancer marine alkaloids, discussing extensively studied marine alkaloids and their mechanisms of action (Tohme et al., 2011). However, it did not specifically focus on treating breast cancer. Hussain et al. recently summarized the cytotoxic effects of various marine derivatives on breast cancer cells in their review (Hussain et al., 2023), but this review only included 24 marine alkaloids and did not provide a comprehensive description of marine alkaloids. In this review, we retrospectively summarize the marine alkaloids with cytotoxic effects on breast cancer cell lines, along with the clinical breast cancer therapeutic efficacy of some compounds, and discuss their potential targets and mechanisms of action.
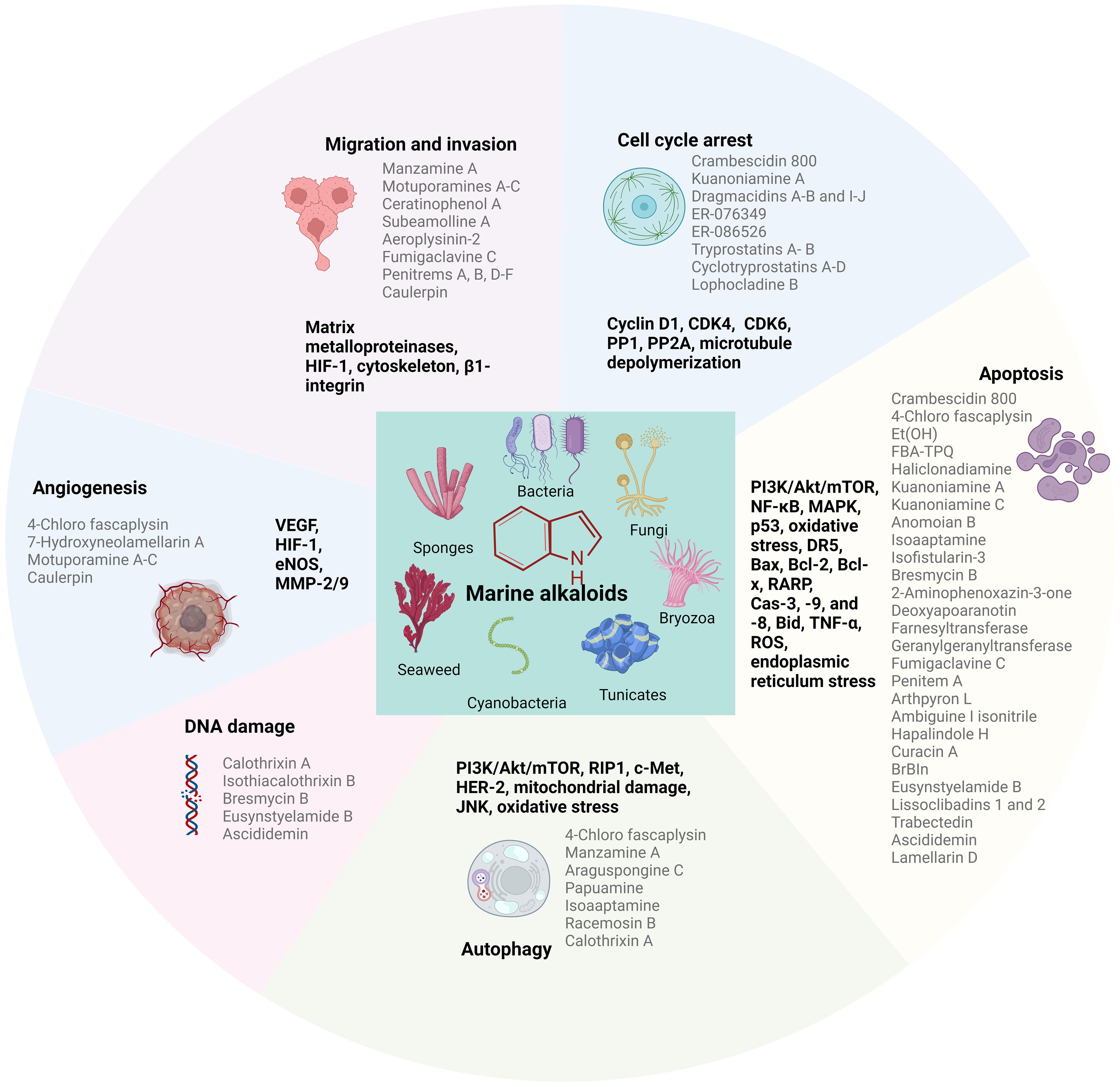
Figure 1 Major sources of marine alkaloids and their primary mechanisms of action as anticancer agents. The graphic is completed by using BioRender.com.
Marine alkaloids: an overview
Marine alkaloids, a class of secondary metabolites of marine organisms with several nitrogenated structures, are among the most important natural products mainly derived from sponges, bacteria, fungi, cyanobacteria, algae, and tunicates. Nearly 40% of the 800 compounds extracted from cyanobacteria were alkaloids, which may be due to the remarkable nitrogen-fixing ability of cyanobacteria, which facilitates the production of alkaloids (Han et al., 2022). The presence of nitrogen electron-donor atoms in the structure of marine alkaloids significantly enhances their interaction with target proteins, enzymes, and receptors by making several types of interactions, such as hydrogen bonds, dipole–dipole interactions, hydrophobic interactions, van der Waals forces, and stacking interactions (Vitaku et al., 2014). This structural feature is crucial for the biological activity of these compounds and their potential as lead compounds in drug development. Marine alkaloids usually have complex and specific chemical structures and can be divided into approximately 20 different chemical classes, including acridine, β-carboline, bromotyrosine, brominated, dimeric aaptamine, guanidine, imidazole, indole, peptide, piperidine, pyrimidine, pyridine, pyrrole, pyrroloiminoquinone, quinoline and quinolizidine, tetrahydroisoquinoline, steroidal, terpenoidal, manzamine, and sesquiterpene quinone/hydroquinone alkaloids (Elissawy et al., 2021). Among marine alkaloids, approximately 25% are indole alkaloids, which are the most common and complex alkaloids (Kobayashi et al., 1990). Moreover, marine alkaloids exhibit a strong diversity of biological activities and are considered lead compounds for the development of potent antibacterial, antifungal, antiviral, antiprotozoal, antimalarial, antituberculosis, anti-inflammatory, antidiabetic, immunomodulatory, or neurological diseases control agents (Gul and Hamann, 2005; Arai et al., 2008; Souza et al., 2020; Willems et al., 2020; Ajebli et al., 2021; Tempone et al., 2021; Izumida et al., 2022; Montuori et al., 2022). At the same time, numerous studies have shown their significant cytotoxic effects on different types of cancer cells (Tohme et al., 2011; Imperatore et al., 2014).
Marine anti-breast cancer alkaloids
Marine sponge
Sponges are the most primitive invertebrates in the marine ecosystem, accounting for 30% of all marine natural products (Bian et al., 2020). The particularly porous structure of sponges provides raw materials, sites, and storage space and releases opportunities for the synthesis of secondary metabolites with special structures and functions (Table 1). Sponges generally obtain sufficient nutrients by filtering large amounts of water, and this filter-feeding behavior introduces bacteria and other microorganisms into the sponge’s waterways, thus forming a sponge–microbe holobiont (Amelia et al., 2022). Studies have gradually shown that some sponge-derived metabolites may not originate from sponges but from sponge-associated bacteria (Amelia et al., 2022). It is worth noting that not all sponge-derived metabolites can be confirmed to originate from marine biological hosts or bacterial symbionts. Therefore, the sponge-derived alkaloids involved here may originate from sponge-associated microorganisms. The structures of some alkaloids from marine sponges are shown in Figure 2.
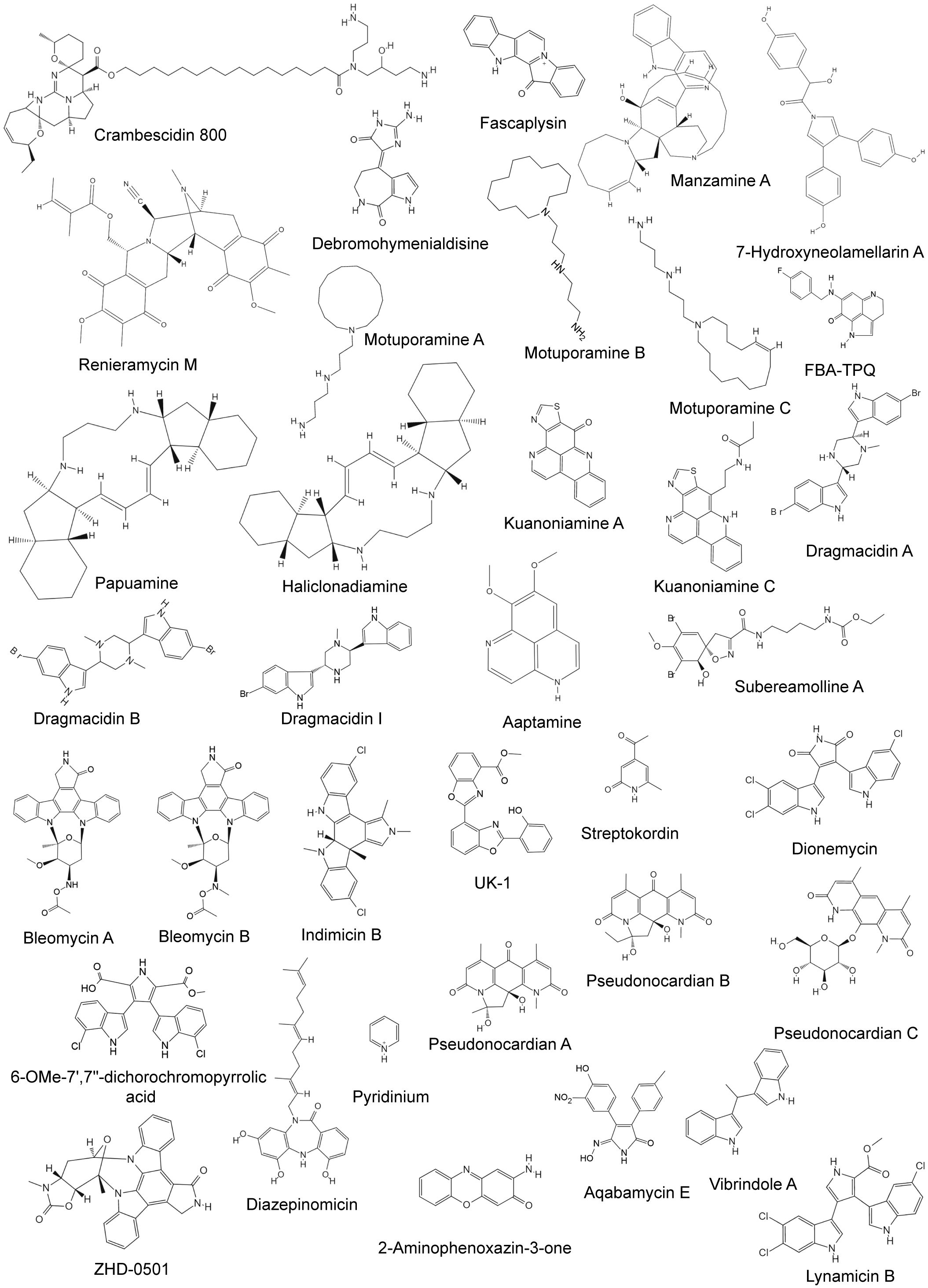
Figure 2 Chemical structures of anti-breast cancer alkaloids derived from marine sponge and bacteria.
Crambescidin 800, a guanidine alkaloid isolated from the sponge Monanchora viridis, was found to exhibit cytotoxicity against various breast cancer cell lines, especially the triple-negative breast cancer cell lines T11 and SUM159PT, with IC50 values of 0.07 ± 0.01 and 0.59 ± 0.08 μM, respectively (Shrestha et al., 2018). The cytotoxic effect of crambescidin 800 occurs mainly through inhibiting the protein expression of cyclin D1, CDK4, and CDK6, which causes tumor cells to undergo cell cycle arrest in the G2/M phase. Furthermore, this inhibition is associated with decreased phosphorylation of the Akt/mTOR, NF-κB, and MAPK pathways, which, in turn, mediates tumor cell apoptosis (Shrestha et al., 2018). Alkaloids sourced from different species within the Monanchora genus are recognized for their diverse chemical structures and biological activities. Compounds such as monanchoradin A, crambescidin 814, monalidine A, monanchomycalin C, and ptilomycalin A exhibit antiproliferative effects on different cancer cells. Among them, crambescidin 814 is more cytotoxic, with an IC50 of approximately 0.05 μM for the MDA-MB-435 cell line (Tabakmakher et al., 2013; El-Demerdash et al., 2016; Gogineni et al., 2020).
Angiogenesis is a prominent characteristic of various types of cancer and is a critical process in tumor growth and progression. Fascaplysin is a beta-carboline alkaloid isolated from Thorectandra sp. sponge that exhibits significant cytotoxicity against MCF-7 and T-47D cells, with IC50 values of 0.03–0.38 mg/mL and 5 ± 0.2 μM, respectively (Charan et al., 2004; Zhidkov et al., 2019). 4-Chloro fascaplysin, an analog of fascaplysin, has been shown to inhibit VEGF-dependent angiogenesis by affecting key proangiogenic factors such as HIF-1α, eNOS, and MMP-2/9 (Sharma et al., 2017). It also inhibited survival in the MDA-MB-231 cell line by modulating the PI3K/Akt/mTOR signaling cascade, simultaneously inducing autophagy and apoptosis (IC50 = 0.3 μM). Notably, 4-chloro fascaplysin does not show significant toxicity in experimental tumor mice at therapeutic doses, indicating good safety (Sharma et al., 2017). In addition, other analogs of fascaplysin, 1-deoxysecofascaplysin A, 3-bromofascaplysin, and 3,10-dibromofascaplysin can also inhibit the proliferation of breast cancer cells (Charan et al., 2004; Zhidkov et al., 2019). 3-Bromofascaplysin and 3,10-dibromofascaplysin can be used to synthesize the alkaloids 14-bromoreticulatate and 14-bromoreticulatine, the latter of which is cytotoxic to a variety of cell lines (Zhidkov et al., 2019).
Et(OH), an extract obtained from the sponge Stylissa carteri, exhibited potential dose-dependent cytotoxic effects on different breast cancer cell lines, such as HCC-1954, MDA-MB-231, MDA-MB-468, and SKBR3 (with IC50 < 15 μg/mL) (Bashari et al., 2019). In the HCC-1954 cell line, it exerts cytotoxic effects by inhibiting growth and inducing apoptosis (Bashari et al., 2019). In the triple-negative breast cancer cell line MDA-MB-231, Et(OH) not only has anti-metastatic effects but also synergistically enhances cell death when combined with adriamycin or paclitaxel (Bashari et al., 2019). Debromohymenialdisine, also isolated from Stylissa species, is a pyrrole seven-membered ring lactam alkaloid (Yang et al., 2015). When combined with radiotherapy, it can increase the MCF-7 inhibition rate and exert a strong radiotherapy effect by downregulating the expression of pCHK1/2 while reducing the survival rate of breast cancer CD44+/CD24− stem cells (Yang et al., 2015).
Manzamine A exhibited significant cytotoxicity in inhibiting the proliferation, migration, and invasion of both MDA-MB-231 and MCF-7 breast cancer cells, with IC50 values of 7.87 ± 0.30 and 2.86 ± 0.19 μM, respectively (Wang et al., 2023). Mechanistic studies have shown that manzamine A treatment reduces the expression level of RIP1, a key upstream regulator of autophagy, thereby mediating the autophagy process of tumor cells through the inhibition of the Akt/mTOR pathway (Wang et al., 2023). Acanthostrongylophora ingens can produce a variety of manzamine alkaloids. In addition to manzamine A, (+)-8-hydroxymanzamine A and (+)-manzamine A were also isolated, and both showed significant anti-breast cancer activity (Samoylenko et al., 2009). In addition to manzamine alkaloids, A. ingens is a rich source of pyrimidine β-carboline alkaloids. Among them, annomontine, acanthomine A, 1,2,3,4-tetrahydronorharman-1-one, and ingenine E can significantly inhibit the activity of various tumors, including breast cancer, colorectal cancer, and lung cancer (Ibrahim and Mohamed, 2017).
7-Hydroxyneolamellarin A, a laminin-like alkaloid derived from the sponge Dendrilla nigra, serves as an inhibitor of HIF-1 and VEGF. It exhibits cytotoxic effects by suppressing hypoxia-induced activation of HIF-1 (with an IC50 of 1.9 μM), subsequently reducing the activation of its downstream target gene, VEGF (Liu et al., 2007).
A variety of bis-1-oxaquinolizidine alkaloids can be isolated from the sponge Xestospongia, including araguspongines A, C, E, L, and N–P and meso-araguspongine C, all of which are capable of killing breast cancer cells (Dung et al., 2019). Among them, meso-araguspongine C has the most potent cytotoxicity (IC50 = 0.44 μM), and araguspongine C is cytotoxic to different breast cancer cell lines (Akl et al., 2015; Dung et al., 2019). In BT-474, which overexpress HER-2, araguspongine C significantly induces autophagy cell death, which is mainly achieved by inhibiting the activation of c-Met and the receptor tyrosine kinase HER-2 (Akl et al., 2015). Renieramycin M is also isolated from Xestospongia sp. and can effectively inhibit the growth of cancer cells. The IC50 values for breast cancer MDA-MB-231 and MDA-MB-435 cells reached nanomolar levels, which were 3.8 and 6.3 ± 0.1 nM, respectively (Charupant et al., 2009; Hussain et al., 2023). For the MDA-MB-435 cell line, renieramycin M treatment significantly downregulated the expression of PTPRK (Charupant et al., 2009). Motuporamines A–C are anti-angiogenic and anti-invasive alkaloids extracted from the marine sponge Xestospongia exigua that can inhibit the invasion of breast and prostate cancers into the basement membrane in vitro (Tohme et al., 2011). Among them, motuporamine C is the most effective. It hinders the migration and angiogenesis of tumor cells by changing the cytoskeleton and inhibiting the activation of β1-integrin, but it does not affect cell proliferation (Tohme et al., 2011).
Makaluvamines are marine alkaloids from a wide range of sources, including Zyzzya cf. marsalis, Histodermella species, Zyzzya fuliginosa, and Smenospongia aurea (Shinkre et al., 2007). Its multiple analogs exhibit in vitro cytotoxicity against breast cancer, providing a solid foundation for the development of new anticancer drugs (Shinkre et al., 2007; Wang et al., 2009). Among them, FBA-TPQ exhibited significant anticancer effects on the MDA-MB-231 and MCF-7 cell lines through the induction of apoptosis, with IC50 values of 0.125 μmol/L and 0.097 μmol/L, respectively (Wang et al., 2009). These effects are primarily mediated through the activation of p53, which then regulates the cell cycle, apoptosis, and DNA damage-related proteins (Wang et al., 2009). Encouragingly, these anticancer mechanisms were independent of the intracellular p53 status, suggesting that FBA-TPQ may have potential roles in different contexts (Wang et al., 2009).
Papuamine derived from the marine sponge Haliclona sp. has a significant cytotoxic effect on the MCF-7 cells, with an inhibition rate of 98.7% at 20 μM (Kanno et al., 2013). Papuamine initiates MCF-7 autophagy by inducing mitochondrial damage and JNK pathway activation, thereby reducing the cell survival rate (Kanno et al., 2013; Yamazaki et al., 2013). Haliclonadiamine, 3-dodecyl pyridine containing a terminal cyano group, and haliclonacyclamine A, also from Haliclona species, also have antiproliferative effects on MCF-7 cell lines (Mani et al., 2011; Yamazaki et al., 2013; Zhang et al., 2016). Haliclonadiamine can induce cancer cell apoptosis, but the mechanisms of action of the remaining two compounds are still unknown and await further research (Yamazaki et al., 2013).
Kuanoniamines A and C are two pyridine acridine alkaloids isolated from Oceanapia sagittaria. Kuanoniamine A has a significant growth inhibitory effect on both ER+ (MCF-7) and ER− (MDA-MB-231) breast cancer cells (Kijjoa et al., 2007). However, kuanoniamine C can only decrease the viability of ER+ breast cancer cell lines, and its antiproliferative effect may be related to estrogen receptors (Kijjoa et al., 2007). Both can cause an increase in the number of apoptotic MCF-7 cells, but kuanoniamine A treatment can cause G1 phase cell cycle arrest, while kuanoniamine C does not (Kijjoa et al., 2007).
Dragmacidins A–B and I–J were obtained from Hexadella sp. and Dragmacidon sp., respectively. This family of compounds can cause cell cycle arrest by inhibiting the phosphorylation activity of PP1 and/or PP2A, thereby affecting cell proliferation. Dragmacidins A, I, and J showed low micromolar cytotoxicity toward MDA-MB-231 cells (Cruz et al., 2018). Anomoian B also comes from Hexadella species, and it can mediate cytotoxicity against MDA-MB-231 cells by inducing apoptosis (Tarazona et al., 2017).
Aaptamine is a marine alkaloid isolated from Aaptos sp. Its various derivatives including demethyl(oxy)aaptamine, isoaaptamine, and 2,3-dihydro-2,3-dioxoaaptamine, all have specific anticancer effects (Dyshlovoy et al., 2014; Wu et al., 2018). Among them, isoaaptamine mainly exerts cytotoxic effects through apoptosis and autophagy induced by oxidative stress (Wu et al., 2018).
Isofistularin-3 is a DNA methyltransferase inhibitor derived from Aplysina aerophoba (Florean et al., 2016). It can not only induce G0/G1 phase cycle arrest but also induce the expression of TRAIL receptor death receptor 5 by triggering endoplasmic reticulum stress, thereby causing cell apoptosis and showing cytotoxicity to a variety of tumor cells (Florean et al., 2016).
Halichondrin B is a tubulin depolymer with significant in vitro and in vivo anticancer activity, and ER-076349 and ER-086526 (eribulin mesylate) are its two analogs (Towle et al., 2001). In the MDA-MB-435 cell line, ER-076349 and ER-086526 can exhibit nanomolar cytotoxicity by inducing G2/M phase cell cycle arrest and disrupting the mitotic spindle (Towle et al., 2001).
Ceratinophenol A, subeamolline A, and aeroplysinin-2 have all been shown to inhibit the migration of breast cancer cells, among which subeamolline A can also prevent invasion (Shaala et al., 2012, 2015a, b). In addition to the above-mentioned alkaloids, a large number of alkaloids of various structural types with anti-breast cancer activity have also been found in other sponges, but their specific anticancer mechanisms need to be further studied (Table 1).
Marine bacteria
Marine bacteria are an important source of bioactive secondary metabolites. The Streptomyces genus is known for its ability to produce novel structures and bioactive metabolites (Table 2), many of which are unique alkaloids (Toumatia et al., 2015). The structures of some alkaloids from marine bacteria are shown in Figure 2. Two indolocarbazole alkaloids, bresmycins A and B, are produced by Streptomyces sp. NBU3142 (Ding et al., 2023). Both have potential cytotoxicity, with bresmycin B exhibiting greater inhibitory effects on MCF-7 cells (IC50 = 2.01 ± 0.35 μM) than bresmycin A (IC50 = 5.01 ± 0.82 μM) (Ding et al., 2023). Furthermore, bresmycin B also had significant inhibitory effects on MCF/ADR cells (a doxorubicin-resistant MCF-7 cell line), with an inhibition rate exceeding 50% (IC50 = 4.47 μM). DNA damage and cell apoptosis may be the possible mechanisms underlying these effects (Ding et al., 2023). Indimicins A–E and spiroindimicins A–D, bisindole alkaloids, were isolated from Streptomyces sp. SCSIO 03032, a deep-sea actinomycete. However, only indimicin B exhibited moderate cytotoxicity (IC50 = 10.0 ± 0.3 μM) against the MCF-7 cell line (Zhang et al., 2012, 2014). Several other alkaloids from different Streptomyces species have been shown to have inhibitory effects on MCF-7 cells (Table 2). Notably, UK-1 (GI50 = 0.65 µM) exhibited even greater potency (Hohmann et al., 2009). Streptokordin, originating from Streptomyces sp. KORDI-3238, possesses potent cytotoxic activity against MDA-MB-231 cells with an IC50 value of 7.5 μg/mL (Jeong et al., 2006). Dionemycin and 6-OMe-7′,7″-dichorochromopyrrolic acid, both isolated from Streptomyces sp. SCSIO 11791, have significant cytotoxic effects on the MDA-MB-435 and MDA-MB-231 cell lines, exhibiting IC50 values ranging from 3.9 to 25 μM (Song et al., 2020).
HD-0501 is a dimeric indole alkaloid isolated from Actinomadura sp. 007 that can inhibit the proliferation of the thermosensitive mouse breast cancer cell line tsFT210 (Han et al., 2005). Iodinin, 1,6-phenazinediol, questiomycin A, chandrananimycins A–C, and phenoxazin-3-one are also from Actinomadura species, among which iodinin and 1,6-phenazinediol showed obvious cytotoxicity to the breast cancer cell line MAXF 401NL (IC50 < 4.0 μg/mL), while other compounds showed antitumor activity against MCF-7 (IC70 < 1.4 μg/mL) (Maskey et al., 2003).
Diazepinomicin and pyridinium were obtained from the actinomycetes Micromonospora DPJ12 and Amycolatopsis alba var. nov. DVR D4, respectively. The former has a cytotoxic effect on MDA-MB-231 cells (IC50 = 2.1 µM), while the latter can significantly inhibit the proliferation of MCF-7 cells (Gourdeau et al., 2008; Dasari et al., 2012). Pseudonocardians A–C are produced by Pseudonocardia sp. SCSIO 01299, which is also a member of marine actinomycetes. All three compounds showed effective cytotoxicity to MCF-7 cells, among which pseudonocardians A and B were more active, with IC50 values of 0.027 and 0.021 μM, respectively (Li et al., 2011).
2-Aminophenoxazin-3-one, 2-amino-6-hydroxyphenoxazin-3-one, and 2-amino-8-benzoyl-6-hydroxyphenoxazin-3-one were all derived from Halomonas sp. strain GWS-BW-H8hM. All of these compounds showed obvious cytotoxic effects on the MCF-7 cell line, with GI50 values of 0.13, 1.6, and 2.0 μg/mL, respectively (Bitzer et al., 2006). Among them, 2-aminophenoxazin-3-one can reduce the ratio of G0/G1 cells and induce the apoptosis of tumor cells (Bitzer et al., 2006). Aqabamycin E and vibrindole A are produced by certain Vibrio species and have certain cytotoxic effects on both the MDA-MB-231 and MCF-7 cell lines, with IC50 values between 20 and 50 μg/mL (Al-Zereini et al., 2010). Lynamicin B isolated from Marinispora sp. NPS12745 showed antitumor effects against MDA-MB-435 and MDA-MB-231, with IC50 values of 6.9 and 28.6 μM, respectively (Song et al., 2020).
Marine fungi
Because of the extreme ecological environments faced by marine fungi (e.g., high salinity, UV exposure, low temperature, restricted growth substrates, and extreme hydrostatic pressure), they produce secondary metabolites with unique structures that exhibit diverse biological activities, including antimicrobial, antidiabetic, and anticancer properties (Debbab et al., 2010; Gonçalves et al., 2022). These metabolites have significant therapeutic potential (Table 3), making marine fungi an important fresh source of secondary metabolites for drug discovery purposes (Ding et al., 2018). The structures of some alkaloids from marine fungi are shown in Figure 3.
Deoxyapoaranotin, a diketopiperazine disulfide, is produced by Aspergillus sp. KMD 901 (Choi et al., 2011). Deoxyapoaranotin induces mitochondria-mediated tumor cell apoptosis by regulating Bax, Bcl-2, and Bcl-xl in a dose-dependent manner, and upregulates the cleavage of PARP, and Cas-3, -9, and -8 (Choi et al., 2011). Gliotoxin is also from a marine-derived Aspergillus species, is a dual inhibitor of farnesyltransferase and geranylgeranyltransferase I, has significant antitumor activity, and can mediate growth inhibition of various breast cancer cells by inhibiting prenyltransferase enzymes (IC50 range, 38–985 nM) (Vigushin et al., 2004). Farnesyltransferase and geranylgeranyltransferase play crucial roles in the posttranslational modification of Ras proteins that regulate cell proliferation, differentiation, and apoptosis (Klochkov et al., 2019).
Fumigaclavine C, tryprostatins A and B, and cyclotryprostatins A–D were all isolated from Aspergillus fumigatus (Cui et al., 1995, 1997; Li et al., 2013). Fumigaclavine C not only significantly inhibits the growth of MCF-7 cells (inhibition rate of 93% at 20 μM) but also significantly inhibits the migration and invasion of tumor cells by downregulating the expression of relevant matrix metalloproteinases (Li et al., 2013). It mainly achieves a cytotoxic effect by arresting the cell cycle at the G1 phase, inducing the expression of the proapoptotic proteins Bax and Bid, and promoting the protein hydrolysis activity of caspase-3, -8, and -9, thereby causing cell apoptosis (Li et al., 2013). Importantly, fumigaclavine C is also an effective and specific chemosensitizer that can completely reverse BCRP-mediated drug resistance in vitro (Rabindran et al., 2000), but it has severe neurotoxic effects (Allen et al., 2002). Its novel tetracyclic analogs Ko132, Ko134, and Ko143 all exhibited significant cytotoxicity against MCF-7 cells (Allen et al., 2002). Ko143 not only significantly increased the oral availability of topotecan in mice but also showed no signs of toxicity in vitro and in vivo (Allen et al., 2002). Thus, Ko143 is the first potent and specific BCRP inhibitor applicable in vivo (Allen et al., 2002). Tryprostatins A and B are indole alkaloids that cause G2/M cell cycle arrest in mammalian cells (Cui et al., 1995). Tryprostatin A not only significantly inhibited the growth of the thermosensitive tsFT210 mouse cell line but also exhibited an obvious cytotoxic effect on MCF-7 cells as a BCRP inhibitor (IC50 = 0.013 ± 0.006 μM) (Cui et al., 1995). Cyclotryprostatins A–D also inhibit the growth of tsFT210 cells by arresting cell cycle progression in the G2/M phase with IC50 values of 5.6 μM, 19.5 μM, 23.4 μM, and 25.3 μM, respectively (Cui et al., 1997). Preussin, isolated from the marine sponge-associated fungus Aspergillus candidus, has been shown to reduce cell viability, impair cell proliferation, and induce cell death in both 2D and 3D cell cultures in a dose-dependent manner (Seabra et al., 2023). It exhibits antiproliferative effects against the MDA-MB-231, MCF-7, and SKBR3 cell lines (Malhão et al., 2019).
The Penicillium genus is an important source of biologically active secondary metabolites. Indole diterpene alkaloids can be directly isolated from Penicillium species, including penitrems A, B, D, E, and F, and paspaline, emnidole SB, semisynthetic 6-bromopenitrems B and E, and 25-o-methylpenitrem A (Table 3) exhibited good antiproliferation, anti-migration, and anti-invasion properties against MDA-MB-231 and MCF-7 (Sallam et al., 2013a, 2013; Goda et al., 2018). Among them, penitrem A can reduce the expression of high-conductance calcium-activated potassium channels and increase the expression of TNF-α while inducing G1 cell cycle arrest in breast cancer cells and upregulating the expression of the arrest protein p27 (Goda et al., 2018). In addition, penitrem A can produce synergistic antiproliferative effects with anti-HER drugs by reducing the active forms of AKT and STAT3 (Goda et al., 2018). Brevicompanine G; penicillivinacine; penochalasins K, I, and J; chaetoglobosins C, F, and G; and cytoglobosin C are also from the Penicillium species, while penochalasins K and I showed significant cytotoxicity to MDA-MB-435 cells (Table 3, IC50 < 10 μM) (Asiri et al., 2015; Huang et al., 2016; Zhu et al., 2017; Yang et al., 2018).
Arthpyrone L, a novel pyridone alkaloid isolated from Arthrinium species, exhibits significant antiproliferative effects on both MCF-7 and MDA-MB-231 cells. Arthpyron L induces G0/G1 cell cycle arrest and simultaneously inhibits tumor cell growth by activating the caspase-regulated apoptosis pathway and downregulating the PI3K/Akt pathway (Zhang et al., 2021). In addition, terretrione A, loonamycin A, and isochromophilone X were isolated from Aspergillus terreus, Nocardiopsis flavescens, and Diaporthe sp., respectively (Asiri et al., 2015; Yang et al., 2020), and also had certain cytotoxic effects on the MDA-MB-231 (IC50 = 17.7 μM), SUM1315 (IC50 = 121.3 nM), and MCF-7 (IC50 = 14.9 µM) cell lines. Luteoalbusins A and B, T988A, and gliocladines C and D were all isolated from Acrostalagmus luteoalbus in deep-sea sediments. These five compounds all showed significant cytotoxicity to the MCF-7 cell line, and the cytotoxicities of luteoalbusins A and B were significantly greater than those of the other compounds (Wang et al., 2012). Avrainvilamide, which is isolated from Avrainvillea species, binds to the proposed oncogenic nuclear chaperone nucleophosmin, which is overexpressed in many different human tumors. In vitro experiments have shown that avrainvillamide has a dose-dependent antiproliferative effect on the BT-549 and T-47D cell lines, with IC50 values of 34 and 72 nM, respectively (Fenical et al., 2000).
Marine seaweed
Seaweed is found in all oceans and is widely consumed as a food and medicinal herb, especially in some historical Asian countries such as China and Japan (El Gamal, 2010). In traditional Chinese medicine, seaweed is mainly used to treat diseases such as goiter, scrofula, testicular swelling, and edema, and is also used as a source of vitamin supplements, cholesterol-lowering agents, and blood sugar-lowering drugs (El Gamal, 2010). Recent studies have shown that the secondary metabolism of seaweed has various biological effects, such as antioxidant, anti-inflammatory, antidiabetic, antibacterial, and anticancer effects (Lee et al., 2013; Sharifuddin et al., 2015). The structures of some alkaloids from marine seaweed are shown in Figure 4.
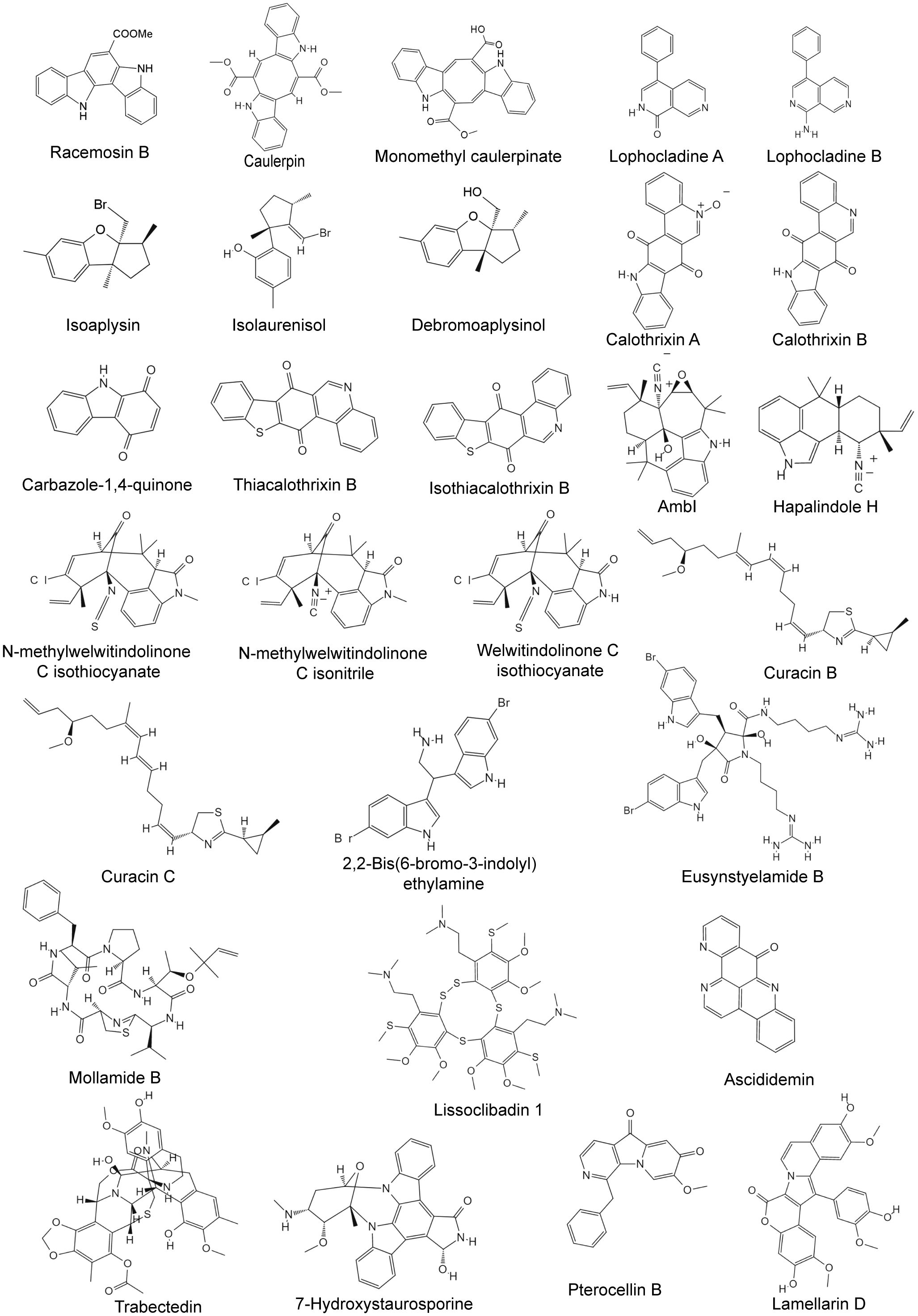
Figure 4 Chemical structures of anti-breast cancer alkaloids derived from marine seaweed, cyanobacteria, tunicate, and bryozoa.
Racemosin B is a marine alkaloid with an unusual indolo[3,2-a]carbazole skeleton that is isolated from the green alga Caulerpa racemose. MTT assays of racemosin B and several derivatives revealed that many of its derivatives could significantly inhibit the growth of MDA-MB-231 and MCF-7 cells (Table 4). Further studies revealed that compound 25, which was designed and synthesized based on racemosin B, showed stronger and more effective activities against MDA-MB-231 (IC50 = 1.06 μM), MCF-7 (IC50 = 2.00 μM), and 4T1 (IC50 = 2.77 μM) cells (Xiao et al., 2018). Compound 25 can induce G2/M cell cycle arrest and promote apoptosis by blocking autophagic flux in breast cancer cells, and the inhibition of autophagy is achieved by regulating the phosphorylation level of mTOR (Xiao et al., 2018).
Caulerpin was first isolated from the green algae genus Caulerpa and was also recently discovered in n-hexane extracts of the macroalgae Halimeda cylindracea. In vitro evaluation revealed that caulerpin can exhibit strong cytotoxic activity against SK-BR-3 cells, with an IC50 value of 3.71 μM (Dini et al., 2021). Moreover, caulerpin exhibited dose-dependent cytostatic effects on the T47D, MCF-7, and MDA-MB-231 cell lines (Table 4). Under hypoxic conditions, caulerpin inhibits hypoxia-induced HIF-1 gene activation, VEGF protein secretion, and tumor angiogenesis in T47D cells (Liu et al., 2009). In addition to inducing angiogenesis, HIF-1 is involved in regulating the expression of genes related to complex metastasis processes. In addition, caulerpin has been shown to have anti-metastatic activity in MDA-MB-231-based wound healing models. Monomethyl caulerpinate, also isolated from Caulerpa cylindracea, has strong cytotoxic effects on MCF-7 and MDA-MB-231 cells, with IC50 values of 16.82 ± 1.12 and 13.96 ± 0.52 μM, respectively (Erol et al., 2022).
Lophocladines A and B are two 2,7-naphthyridine alkaloids isolated from the marine red alga Lophocladia, among which lophocladine B can induce microtubule depolymerization and G2/M cell cycle arrest in MDA-MB-435 cells, resulting in significant cytotoxicity (IC50 = 3.1 μM). While lophocladine A lacks cytotoxicity to tumor cells, it was found to have an affinity for NMDA receptors and appears to be a delta-opioid receptor antagonist (Gross et al., 2006).
Isoaplysin, isolaurenisol, and debromoaplysinol are all isolated from the sesquiterpenes of Laurencia pacifica, where isoaplysin and debromoaplysinol (Table 4) showed promising cytostatic effects on MCF-7 cells and were two times more potent in MCF-7 cells than in nontumor-derived normal breast MCF10A cells (Zaleta-Pinet et al., 2014).
Marine cyanobacteria
Cyanobacteria, also known as blue-green algae, are ancient photosynthetic prokaryotes. They have extremely strong adaptability and are widely distributed in various environments around the world, from freshwater to oceans, soil, and extreme environments (such as glaciers and hot springs) (Demay et al., 2019). Cyanobacteria can produce toxins, which can lead to algae blooms, disrupt the ecological balance of water bodies, and have a negative impact on human and animal health (Demay et al., 2019). However, they also produce a variety of secondary metabolites with different biological activities, and these complexes exhibit enormous potential beneficial properties in various fields, including antibacterial, antifungal, anticancer, immunosuppressive, anti-inflammatory, and antituberculosis activities (Demay et al., 2019). The structures of some alkaloids from marine cyanobacteria are shown in Figure 4.
Calothrixins A and B are carbazole-1,4-quinone alkaloids isolated from Calothrix sp. These compounds, along with analogs such as 3-fluoro-calothrixin 15h and 4-fluoroquinocarbazole 21b, have been found to exhibit potent cytotoxicity against the MCF-7 and MDA-MB-231 cell lines (Table 5), with GI50 values in the range of 0.02–0.95 μM (Muthu Ramalingam et al., 2018). Calothrixins A and B both stabilize the cleavable complex of topo I-DNA, but calothrixin A induces extensive DNA damage. At low concentrations (0.1 μM), calothrixin A can induce G1 cell cycle arrest in tumor cells, and at high concentrations, calothrixin A can cause reversible G2/M arrest, thereby generating reactive oxygen species and inducing cell death through autophagy (Muthu Ramalingam et al., 2018). In another study, the biological activity of analogs of calothrixin B in which the carbazole nitrogen (NH) was replaced with a sulfur atom was explored. Thiacalothrixin B was less cytotoxic than calothrixin B (Dhatchana Moorthy et al., 2018). However, isothiacalothrixin B and its fluorinated analogs (3-fluoro-isothiacalothrixin B and 11-fluoro-isothiacalothrixin B) produced after isomerization have excellent anticancer effects, and the GI50 values of MCF-7 and MDA-MB-231 cells range from 0.22 to 1.9 μM (Dhatchana Moorthy et al., 2018). The cytotoxicity of the sulfur analogs of calothrixin B is mediated in part by the induction of cellular DNA strand breaks and the arrest of cells. Among them, isothiacalothrixin B induces apoptosis by irreparable DNA damage (Dhatchana Moorthy et al., 2018).
The indole alkaloids ambiguine I isonitrile (AmbI) and hapalindole H (Hap H) are both obtained from Fischerella ambigua (Acuña et al., 2015, 2018). AmbI and Hap H are potent inhibitors of NF-κB, which prevents cancer cells from entering an apoptotic state and has been shown to contribute to the progression of certain tumors (Acuña et al., 2015, 2018). AmbI significantly inhibited MCF-7 cell proliferation (EC50 = 1.7 μM), and AmbI treatment caused mitochondrial dysfunction and increased ROS levels in MCF-7 cells, subsequently leading to caspase-independent cell death (Acuña et al., 2015). The antiproliferative effect of Hap H on MCF-7 is weaker than that of AmbI (EC50 = 5.96 µM), but its mechanism of inducing cell death is similar to that of AmbI. Hap H treatment causes damage to the mitochondrial outer membrane, which, in turn, mediates caspase-3-independent apoptosis (Acuña et al., 2018). In addition to Hap H, indole alkaloids such as hapalindoles A, C, I, J, and U; anhydrohapaloxindole A and fischerindole L; and two hapalindole-type alkaloids, hapalindole X and 13-hydroxy dechlorofontonamide, can also be isolated from Westiellopsis sp. and Fischerella muscicola (Table 5). Among them, fischerindole L and hapalindole A showed relatively more effective cytotoxic effects on MCF-7 cells (Kim et al., 2012).
N-Methylwelwitindolinone C isothiocyanate, N-methylwelwitindolinone C isonitrile, and welwitindolinone C isothiocyanate, which are members of the Welwitindolinones family, are isolated from the blue-green algae Hapalosiphon welwitschii, and they strongly inhibit the proliferation of the MCF-7 cells (Table 5), with IC50 values of 3.03, 0.12, and 0.13 μM, respectively (Smith et al., 1995). Moreover, the presence of N-methylwelwitindolinone C isothiocyanate has been shown to diminish the resistance of MCF-7/ADR cells to anticancer drugs such as vincristine, paclitaxel, doxorubicin, daunorubicin, and colchicine (Smith et al., 1995).
Curacins A, B, and C are all extracted from Lyngbya majuscule and have been proven to have anti-mitotic properties (Verdier-Pinard et al., 1998). Among them, curacin A is the most obvious and can significantly inhibit MCF-7 cell proliferation, with an IC50 of 0.038 μM (Verdier-Pinard et al., 1998). Further studies revealed that curacin A can inhibit microtubule assembly and induce cell cycle arrest and apoptosis (Wipf et al., 2004).
Marine tunicate
Because of their powerful immune defense systems and associated bioactive symbiotic microorganisms, tunicates have become a high-priority pharmaceutical resource in the ocean and are considered one of the most intensively studied organisms of the 21st century (Palanisamy et al., 2017; Ramesh et al., 2021). Statistics on the structures of secondary metabolites of 572 kinds of tunicates reported from 1994 to 2014 revealed that approximately 74% of the secondary metabolites were alkaloids (Palanisamy et al., 2017). The secondary metabolites of tunicates have a variety of biological activities, but anticancer drugs are the main type of marine natural product derived from tunicates (64%), followed by antimalarial drugs (6%). In addition, they can also be used for antibacterial, antidiabetic, anti-HIV, antiviral, anti-inflammatory, antifungal, and other applications (Palanisamy et al., 2017). The structures of some alkaloids from marine tunicate are shown in Figure 4.
2,2-Bis(6-bromo-3-indolyl)ethylamine (BrBIn) can be derived from the tunicate Didemnum candidum and can also be obtained from the sponge Orina. BrBIn has effective cytotoxicity against a variety of tumor cells (Supplementary Table 1), e.g., MCF-7 cells (IC50 = 10.0 μM). BrBIn can inhibit the expression of antiapoptotic factors (Bcl-2/Bcl-x) while enhancing the expression of proapoptotic factors (Bax), thereby inducing the release of cytochrome c from mitochondria, leading to the cleavage of caspase-9 and promoting the activation of caspase-3, which ultimately mediates tumor cell death through apoptosis (Salucci et al., 2018). Eusynstyelamide B, a bisindole alkaloid also identified from Didemnum candidum, can induce G2 cell cycle arrest in tumor cells, trigger apoptosis, and exhibit significant cytotoxicity in MDA-MB-231 cells (IC50 = 5 μM). In addition, eusynstyelamide B has also been shown to be a nonintercalative topoisomerase II toxic agent, leading to DNA double-strand breaks (Liberio et al., 2015). Granulatimide and isogranulatimide, which are extracted from Didemnum granulatum, are G2-specific cell cycle checkpoint inhibitors. Both exhibit strong cytotoxicity against p53-deficient MCF-7 cells, with IC50 values in the range of 1–1.8 μM (Berlinck et al., 1998; Roberge et al., 1998). Mollamide B and fascaplysin A are also isolated from Didemnum species, both of which have obvious cytotoxic effects on breast cancer, and the fascaplysin A derivative 3-bromofascaplysin can inhibit the growth of a variety of breast cancer cells (Supplementary Table 1), including HS 578T, BT-549, and T-47D cells (Segraves et al., 2004; Donia et al., 2008).
Lissoclibadins 1, 2, 3, 8, and 14, as well as lissoclinotoxins E and F, are derived from Lissoclinum cf. badium, and two monomeric compounds of lissoclibadins [3,4-dimethoxy-6-(2′-N,N-dimethylaminoethyl)-5-(methylthio)benzotrithiane, N,N-dimethyl-5-(methylthio)varacin] have significant cytotoxic effects on a variety of breast cancer cell lines (Supplementary Table 1), including T-47D, MDA-MB-231, MDA-MB-468, and MCF-7 (Davis et al., 2003; Oda et al., 2007; Tatsuta et al., 2017). The antiproliferative effects of lissoclibadins 1 and 2 are more prominent, and lissoclibadin 1 inhibits tumor cell growth mainly by inducing intrinsic caspase-dependent apoptosis (Tatsuta et al., 2017).
Trabectedin, which is isolated from Ecteinascidia turbinata, has been approved in the European Union since 2009 for the treatment of platinum-sensitive recurrent ovarian cancer in combination with pegylated liposomal doxorubicin. Subsequently, in 2015, trabectedin was approved by the U.S. FDA for the treatment of unresectable or metastatic liposarcoma or leiomyosarcoma in patients who had previously received anthracycline-based chemotherapy (Gadducci and Cosio, 2022). Trabectedin also shows obvious cytotoxicity to breast cancer cells. For breast cancer cells of different genotypes, trabectedin can activate apoptosis mediated by exogenous and/or endogenous pathways, thereby causing the death of tumor cells (Atmaca et al., 2013).
Aplicayanins A–F are brominated derivatives isolated from Aplidium cyaneum (Supplementary Table 1), among which aplicayanins B and D–F have strong cytotoxic and antimitotic effects on MCF-7 cells (Reyes et al., 2008b). Botryllamides K and L and caelestines A–D were extracted from Aplidium altarium and Aplidium caelestis (Supplementary Table 1), respectively, but these six compounds showed poor cytotoxicity to MCF-7 cells, with IC50 values ranging from 39 to 91 μM (Yin et al., 2010a, b).
7-Hydroxystaurosporine (UCN-01) was isolated from Eudistoma sp. and was originally used as a protein kinase C inhibitor (Mizuno et al., 1995). It was later discovered that UCN-01 can eliminate S and G2 phase cell cycle arrest and enhance cisplatin cytotoxicity and apoptosis in breast cancer cells without itself being cytotoxic (Lee et al., 1999).
Ascididemin, which is isolated from Cystodytes dellechiajei, is also a topoisomerase II inhibitor. Ascididemin can induce DNA damage and the release of reactive oxygen species, followed by the activation of caspase-2, leading to oxidative stress-dependent apoptosis, which has potent antiproliferative effects on the MCF-7 cell line (Morvan, 2013). Two indolocarbazole alkaloids, 7-oxo-3,8,9-trihydroxystaurosporine and 7-oxo-8,9-dihydroxy-4′-N-demethylstaurosporine, were also extracted from Cystodytes species and showed strong cytotoxic effects on MDA-MB-231, with GI50 values of 28.4 and 32.1 nM, respectively (Reyes et al., 2008a). Arnoamines A and B are also extracted from the same genus and are pentacyclic pyridoacridine alkaloids. Both natural products have cytotoxic effects on MCF-7 cells, with IC50 values of 0.3 and 2–3 μg/mL, respectively (Plubrukarn and Davidson, 1998).
Botryllamides E–G are extracted from Botryllus tyreus, and they have been shown to have cytotoxic effects on breast cancer carcinoma, exhibiting IC50 values of 27, 41, and 6.9 μM, respectively (Palanisamy et al., 2017). 3,6-Dibromoindole, 6-bromo-3-chloroindole, and 6-bromo-2-oxindole are produced by Distaplia skoogi, but these three compounds do not have strong cytotoxic effects on MDA-MB-231 cells, with IC50 values of 117.72, 72.53, and 74.41 μM, respectively (Palanisamy et al., 2017). Tanjungides A and B are two dibrominated indoleamide alkaloids extracted from Diazona cf. formosa, and both have strong antitumor effects on MDA-MB-231 cells, with GI50 values of 0.23 and 1.63 μM, respectively (Murcia et al., 2014).
Marine bryozoa
Bryozoa are a type of aquatic suspension-feeding invertebrate that is abundant, diverse, and widely distributed, with more than 6,000 species (Figuerola and Avila, 2019). Bryozoa are extremely vulnerable to biological pollution, predator predation, and pathogen attack. To adapt to the harsh living environments, bryozoans have evolved a chemical defense strategy by producing potent secondary metabolites (Hanssen et al., 2021). The metabolites produced by bryozoans have a variety of biological activities, such as anticancer, antiviral, antiparasitic, anti-Alzheimer’s disease, and anti-Parkinson’s disease activities (Ciavatta et al., 2020). The structures of some alkaloids from marine bryozoa are shown in Figure 4.
Pterocellins A and B are two alkaloids with novel heterocyclic skeletons isolated from Pterocella vesiculosa. Both of these compounds showed significant cytotoxicity to a variety of breast cancer cell lines (Supplementary Table 2), among which the toxicity to MDA-MB-435 (GI50: 0.2, 0.2 μM) and MDA-N (GI50: 0.2, 0.2 μM) cell lines was the most significant (Yao et al., 2003; Pejin et al., 2014). Securidine A is a novel brominated alkaloid isolated from Securiflustra securifrons (Michael et al., 2017). In vitro MTS experiments revealed that securidine A did not have any significant anticancer activity against the A2058, HT29, and MCF7 cell lines at concentrations of 50 µM or 100 µM (Supplementary Table 2). Securamines C, E and H–J are also extracted from S. securifrons. Studies have shown that only Securamines H, I, C, and E can affect tumor cell proliferation. Among them, H, I, and E have stronger potent cytotoxic effects on MCF-7 cells, with IC50 values of 2.1, 2.4, and 8.3 μM, respectively (Hansen et al., 2017). Tambjamine K is a 2,20-bipyrrolic class of cytotoxic alkaloids obtained from Azorean nudibranch Tambja ceutae. This compound can inhibit the proliferation of MB-231 cells, with an IC50 value of 15.3 μM (Aldrich et al., 2010). In addition, analysis of the crude extract of the bryozoan Cryptosula zavjalovensis Kubanin revealed that the ethyl acetate fraction of C. zavjalovensis had excellent cytotoxic activity against the MCF-7 cell line (Supplementary Table 2).
Other marine sources of anti-breast cancer alkaloids
Lamellarin D is a hexacyclic pyrrole alkaloid extracted from the marine mollusk Lamellaria sp (Pla et al., 2006). It was originally identified as a topoisomerase I inhibitor that induces apoptosis by mediating the DNA damage response. Recently, studies have shown that lamellarin D can also mediate cell death by activating the intrinsic, mitochondria-dependent apoptotic pathway in cancer cells (Ballot et al., 2009). Lamellarin D is highly cytotoxic to the MDA-MB-231 cell line with a GI50 of 0.25 μM (Pla et al., 2006).
Marine alkaloids combat breast cancer: in vivo study
In vitro models are very important for evaluating the mechanism of action and complex assessment of candidate compounds. Owing to the large differences between in vitro and in vivo environments, in vivo models are often required to further evaluate the safety, efficacy, and delivery of candidate drugs (Table 6).
4-Chloro fascapysin exhibits obvious pharmacological activity through oral or intraperitoneal administration and can significantly hinder HIF-1α/VEGF-mediated microvessel sprouting and angiogenesis in C57/BL6J mice infected with MDAMB-231 cells and inhibit Ehrlich ascites carcinoma growth (Sharma et al., 2017). At the doses tested, 4-chloro fascapysin treatment did not have any toxic effects, such as weight loss or mortality (Sharma et al., 2017).
Halichondrin B is a highly potent antimitotic drug, and ER-076349 and ER-086526 are two macrocyclic ketone analogs that retain the significant efficacy of the parent compound. In the MDA-MB-435 xenograft model, both ER-076349 and ER-086526 can significantly inhibit tumor growth after an intravenous injection of 0.25–1.0 mg/kg (Towle et al., 2001). However, the efficacy of ER-086526 is even more powerful, achieving 95% tumor regression after 14 days of treatment and showing a fourfold therapeutic window (Towle et al., 2001).Two phase I clinical studies have shown that eribulin mesylate has therapeutic activity in patients with advanced breast cancer (Synold et al., 2005; Goel et al., 2009). Three phase II studies showed that the objective response rate and clinical benefit rate of ER-086526 treatment in patients with metastatic breast cancer who were previously treated with anthracyclines and taxanes were 9.3%–21.3% and 17.1%–27.5 (Table 7), respectively (Vahdat et al., 2009; Cortes et al., 2010; Aogi et al., 2012). Encouraged by these results, two open-label, randomized, controlled, parallel-group phase III studies were subsequently conducted, and eribulin showed a significant and clinically meaningful improvement in overall survival compared with treatment of physician’s choice in women with heavily pretreated metastatic breast cancer, but was not shown to be superior to capecitabine concerning OS or PFS (Cortes et al., 2011; Kaufman et al., 2015). Eribulin was approved in the USA (2010) and Europe (2011) for the treatment of patients with metastatic breast cancer who have previously received at least two chemotherapeutic regimens for the treatment of metastatic disease (Huyck et al., 2011; Orditura et al., 2017). Since then, many phase I/II clinical studies have been conducted to evaluate the efficacy and safety of eribulin in patients with breast cancer with different molecular subtypes (Table 7) as first- or second-line chemotherapy, and in combination with cisplatin S-1, trastuzumab, pembrolizumab, and gemcitabine (Aogi et al., 2012; Koczywas et al., 2014; Wilks et al., 2014; Inoue et al., 2016; Takashima et al., 2016; Orditura et al., 2017; Hayashida et al., 2018; Sakaguchi et al., 2018; Iwasa et al., 2019; Tolaney et al., 2019; Pellegrino et al., 2021; Hasler-Strub et al., 2023).
FBA-TPQ is a synthetic derivative of makaluvamine. The results of in vivo experiments by Wang et al. showed that this compound could significantly inhibit breast cancer MCF-7 xenograft tumor growth at three tested concentrations (5, 10, and 20 mg/kg), with inhibition rates ranging from 36.2% to 71.6% (Wang et al., 2009). However, experiments have also shown that high doses (10 and 20 mg/kg) can cause weight loss in mice (Wang et al., 2009).
In the N-methyl-N-nitrosourea rat mammary carcinoma model, gliotoxin showed significant antitumor activity, and all rats that completed gliotoxin treatment experienced a certain degree of tumor regression (Vigushin et al., 2004). However, gliotoxin treatment can cause local toxicity at the injection site such as itching, alopecia, induration, and occasional ulceration. Fortunately, these reactions are transient, and systemic toxicity does not occur (Vigushin et al., 2004).
Trabectedin was approved by the US FDA in 2015 for the treatment of unresectable or metastatic liposarcoma and leiomyosarcoma and is approved in multiple countries for the treatment of patients with recurrent platinum-sensitive ovarian cancer (Barone et al., 2017). Previous preclinical studies have shown that sequential treatment with paclitaxel and trabectedin improves the antitumor effects in nude mice with MX-1 xenografts (Takahashi et al., 2002). Moreover, a phase II study (Table 7) showed that trabectedin can induce response and tumor control in previously treated patients with advanced breast cancer with controllable toxicity (Zelek et al., 2006).
In vitro, both UCN-01 and tamoxifen can individually inhibit the proliferation of MCF-7 cells in a concentration-dependent manner, and their combined use exhibits superior synergistic antitumor effects at different concentrations (Koh et al., 2003). However, for Br-10 and MCF-7 xenograft tumors, UCN-01 and tamoxifen can only exert marginal antitumor effects when used alone and can have positive antitumor effects when combined (Koh et al., 2003). A phase I clinical trial by Fracasso et al. showed that the combination of UCN-01 and irinotecan induced responses in patients with advanced breast cancer (Fracasso et al., 2011). However, the results from further phase II clinical trials showed that the overall response rate of patients with metastatic triple-negative breast cancer to irinotecan combined with UCN-01 was only 4% (Ma et al., 2013).
Conclusion
Breast cancer is the most common cancer worldwide, affecting an estimated 12.9% of women in their lifetime (Delgado et al., 2021). For early-stage breast cancer, complete or breast-conserving surgical resection is the cornerstone of oncologic treatment. Usually, local treatment (radiotherapy) or systemic treatment (chemotherapy and targeted therapy) is supplemented before surgery (neoadjuvant) or after surgery (adjuvant) to prevent tumor recurrence (Harbeck et al., 2019). Unfortunately, approximately 30% of early-stage breast cancers will experience recurrence and metastasis (Pisani et al., 2002). Currently, effective treatments for advanced and recurrent breast cancer are lacking. Chemotherapy and systemic treatment can only be used to control tumor spread, improve quality of life, and extend life expectancy (Harbeck et al., 2019). Therefore, there is an urgent need to develop new effective drugs to treat cancer through the application of new technologies, new ideas, and new methods.
Marine resources account for 80% of the Earth’s biological resources, and the special living environments (such as high pressure, low temperature, high salt, and lack of light and oxygen) of marine organisms have resulted in the metabolism and accumulation of numerous natural compounds with unique chemical structures and various biological activities, providing new tools for tumor treatment. Marine alkaloids, which are mainly derived from sponges, fungi with symbiotic relationships, bacteria, cyanobacteria with nitrogen-fixing capabilities, and tunicates, are important natural compounds in marine organisms. In recent years, with the advancement of modern technology, significant progress has been made in the field of marine chemistry, especially important breakthroughs in the separation and purification of natural marine compounds. The improvement of separation and purification technology has made it increasingly possible to extract different alkaloids from marine organisms. However, there are still some challenges. For example, most marine alkaloids still face problems such as small extraction volumes and low efficiency (Zhou et al., 2021). In addition, protecting and preserving natural marine habitats has become urgently important. To overcome these challenges, methods such as rational drug design, structural modification of lead compounds, and chemical synthesis can be used (Ballot et al., 2010).
Encouragingly, numerous studies have shown that marine alkaloids can exhibit significant antitumor activity by inducing apoptosis and autophagy, inhibiting mitophagy, migration, invasion, angiogenesis, and oncogene pathways (such as the PI3K/Akt/mTOR signaling cascade), and causing DNA damage. These findings not only provide a valuable resource for the development of new anticancer drugs, but also are closely linked to the goal of the GBCI, which is to reduce breast cancer mortality through innovation and global collaboration. Although most studies on marine alkaloids have been conducted in vitro, and only a small proportion of compounds have undergone in vivo studies and clinical trials, these preliminary results have pointed the way for the development of marine drugs for the treatment of breast cancer.
Therefore, it is crucial to further explore the anticancer mechanism of marine alkaloids, which will not only help to fully understand their potential as anticancer drugs but also contribute to the realization of the goals of GBCI. Some of the substances summarized in this review may provide researchers with a useful blueprint for selecting candidate anticancer drugs and inspire medicinal chemists in this field to actively explore and develop new marine drugs for breast cancer. As these studies progress, we can expect to see more marine alkaloids developed into effective anticancer drugs, thus playing a key role in the global fight against breast cancer, bringing new hope to patients with breast cancer and directly contributing to reducing breast cancer mortality, which is in line with GBCI’s ambitious goals.
Author contributions
HS: Funding acquisition, Project administration, Writing – original draft, Writing – review & editing, Supervision. JY: Writing – original draft, Writing – review & editing. XW: Writing – original draft, Writing – review & editing. PD: Writing – original draft, Writing – review & editing, Funding acquisition.
Funding
The author(s) declare financial support was received for the research, authorship, and/or publication of this article. This work was supported by (1) the National Key Research and Development Program of China [2022YFA1207300 (2022YFA1207303)]; (2) the National Natural Science Foundation of China (No. 82172634); (3) the Key Program of the Science and Technology Bureau of Sichuan (No. 2021YFSY0007); (4) the 1.3.5 project for disciplines of excellence, West China Hospital, Sichuan University (No. ZYGD23028); and (5) the National Natural Science Foundation of China (No. 82104212).
Conflict of interest
The authors declare that the research was conducted in the absence of any commercial or financial relationships that could be construed as a potential conflict of interest.
Publisher’s note
All claims expressed in this article are solely those of the authors and do not necessarily represent those of their affiliated organizations, or those of the publisher, the editors and the reviewers. Any product that may be evaluated in this article, or claim that may be made by its manufacturer, is not guaranteed or endorsed by the publisher.
Supplementary material
The Supplementary Material for this article can be found online at: https://www.frontiersin.org/articles/10.3389/fmars.2024.1440928/full#supplementary-material
References
Acuña U. M., Mo S., Zi J., Orjala J., EJC D. E. B. (2018). Hapalindole H induces apoptosis as an inhibitor of NF-ĸB and affects the intrinsic mitochondrial pathway in PC-3 androgen-insensitive prostate cancer cells. Anticancer Res. 38, 3299–3307. doi: 10.21873/anticanres.12595
Acuña U. M., Zi J., Orjala J., Carcache de Blanco E. J. (2015). Ambiguine I isonitrile from fischerella ambigua induces caspase-independent cell death in MCF-7 hormone dependent breast cancer cells. Int. J. Cancer Res. (Tortola) 49, 1655–1662.
Ajebli M., Khan H., Eddouks M. (2021). Natural alkaloids and diabetes mellitus: A review. Endocr. Metab. Immune Disord. Drug Targets 21, 111–130. doi: 10.2174/1871530320666200821124817
Akl M. R., Ayoub N. M., Ebrahim H. Y., Mohyeldin M. M., Orabi K. Y., Foudah A. I., et al. (2015). Araguspongine C induces autophagic death in breast cancer cells through suppression of c-Met and HER2 receptor tyrosine kinase signaling. Mar. Drugs 13, 288–311. doi: 10.3390/md13010288
Allen J. D., van Loevezijn A., Lakhai J. M., van der Valk M., van Tellingen O., Reid G., et al. (2002). Potent and specific inhibition of the breast cancer resistance protein multidrug transporter in vitro and in mouse intestine by a novel analogue of fumitremorgin C. Mol. Cancer Ther. 1, 417–425.
Aldrich L. N., Stoops S. L., Crews B. C., Marnett L. J., Lindsley C. W. (2010). Total synthesis and biological evaluation of tambjamine K and a library of unnatural analogs. Bioorganic Medicinal Chem. Lett. 20, 5207–5211. doi: 10.1016/j.bmcl.2010.06.154
Al-Zereini W., Fotso Fondja Yao C. B., Laatsch H., Anke H. (2010). Aqabamycins A-G: novel nitro maleimides from a marine Vibrio species. I. Taxonomy, fermentation, isolation and biological activities. J. Antibiot. (Tokyo) 63, 297–301. doi: 10.1038/ja.2010.34
Amelia T. S. M., Suaberon F. A. C., Vad J., Fahmi A. D. M., Saludes J. P., Bhubalan K. (2022). Recent advances of marine sponge-associated microorganisms as a source of commercially viable natural products. Mar. Biotechnol. 24, 492–512. doi: 10.1007/s10126-022-10130-2
Aogi K., Iwata H., Masuda N., Mukai H., Yoshida M., Rai Y., et al. (2012). A phase II study of eribulin in Japanese patients with heavily pretreated metastatic breast cancer. Ann. Oncol. 23, 1441–1448. doi: 10.1093/annonc/mdr444
Arai M., Sobou M., Vilchéze C., Baughn A., Hashizume H., Pruksakorn P., et al. (2008). Halicyclamine A, a marine spongean alkaloid as a lead for anti-tuberculosis agent. Bioorganic Medicinal Chem. 16, 6732–6736. doi: 10.1016/j.bmc.2008.05.061
Asiri I. A. M., Badr J. M., Youssef D. T. A. (2015). Penicillivinacine, antimigratory diketopiperazine alkaloid from the marine-derived fungus Penicillium vinaceum. Phytochem. Lett. 13, 53–58. doi: 10.1016/j.phytol.2015.05.014
Atmaca H., Bozkurt E., Uzunoglu S., Uslu R., Karaca B. (2013). A diverse induction of apoptosis by trabectedin in MCF-7 (HER2-/ER+) and MDA-MB-453 (HER2+/ER-) breast cancer cells. Toxicol. Lett. 221, 128–136. doi: 10.1016/j.toxlet.2013.06.213
Ballot C., Kluza J., Lancel S., Martoriati A., Hassoun S. M., Mortier L., et al. (2010). Inhibition of mitochondrial respiration mediates apoptosis induced by the anti-tumoral alkaloid lamellarin D. Apoptosis 15, 769–781. doi: 10.1007/s10495-010-0471-2
Ballot C., Kluza J., Martoriati A., Nyman U., Formstecher P., Joseph B., et al. (2009). Essential role of mitochondria in apoptosis of cancer cells induced by the marine alkaloid Lamellarin D. Mol. Cancer Ther. 8, 3307–3317. doi: 10.1158/1535-7163.MCT-09-0639
Barone A., Chi D. C., Theoret M. R., Chen H., He K., Kufrin D., et al. (2017). FDA approval summary: trabectedin for unresectable or metastatic liposarcoma or leiomyosarcoma following an anthracycline-containing regimen. Clin. Cancer Res. 23, 7448–7453. doi: 10.1158/1078-0432.CCR-17-0898
Bashari M. H., Huda F., Tartila T. S., Shabrina S., Putri T., Qomarilla N., et al. (2019). Bioactive compounds in the ethanol extract of marine sponge stylissa carteri demonstrates potential anti-cancer activity in breast cancer cells. Asian Pac J. Cancer Prev. 20, 1199–1206. doi: 10.31557/APJCP.2019.20.4.1199
Berlinck R. G. S., Britton R., Piers E., Lim L., Roberge M., Moreira da Rocha R., et al. (1998). Granulatimide and isogranulatimide, aromatic alkaloids with G2 checkpoint inhibition activity isolated from the Brazilian ascidian didemnum granulatum: Structure elucidation and synthesis. J. Organic Chem. 63, 9850–9856. doi: 10.1021/jo981607p
Bian C., Wang J., Zhou X., Wu W., Guo R. (2020). Recent advances on marine alkaloids from sponges. Chem. Biodiv. 17, 10. doi: 10.1002/cbdv.202000186
Bitzer J., Große T., Wang L., Lang S., Beil W., Zeeck A. (2006). New aminophenoxazinones from a marine halomonas sp.: fermentation, structure elucidation, and biological activity. J. Antibiotics 59, 86–92. doi: 10.1038/ja.2006.12
Burguin A., Diorio C., Durocher F. (2021). Breast cancer treatments: updates and new challenges. J. Pers. Med. 11 (8), 808. doi: 10.3390/jpm11080808
Capon R. J., Peng C., Dooms C. (2008). Trachycladindoles A-G: cytotoxic heterocycles from an Australian marine sponge, Trachycladus laevispirulifer. Org. Biomol. Chem. 6, 2765–2771. doi: 10.1039/b803455a
Carbone D., De Franco M., Pecoraro C., Bassani D., Pavan M., Cascioferro S., et al. (2023). Structural manipulations of marine natural products inspire a new library of 3-amino-1,2,4-triazine PDK inhibitors endowed with antitumor activity in pancreatic ductal adenocarcinoma. Mar. Drugs 21, 288. doi: 10.3390/md21050288
Carney J. R., Scheuer P. J., Kelly-Borges M. (1993). Makaluvamine G, a cytotoxic pigment from an an Indonesian Sponge Histodermella sp. Tetrahedron 49, 38, 8483–8486. doi: 10.1016/S0040-4020(01)96256-8
Chantana C., Sirion U., Iawsipo P., Jaratjaroonphong J. (2021). Short total synthesis of (±)-gelliusine E and 2,3′-bis(indolyl)ethylamines via PTSA-catalyzed transindolylation. J. Organic Chem. 86, 13360–13370. doi: 10.1021/acs.joc.1c01461
Charan R. D., McKee T. C., Boyd M. R. (2004). Cytotoxic alkaloids from the marine sponge Thorectandra sp. Nat. Prod Res. 18, 225–229. doi: 10.1080/14786410310001622077
Charupant K., Suwanborirux K., Daikuhara N., Yokoya M., Ushijima-Sugano R., Kawai T., et al. (2009). Microarray-based transcriptional profiling of renieramycin M and jorunnamycin C, isolated from Thai marine organisms. Mar. Drugs 7, 483–494. doi: 10.3390/md7040483
Choi E. J., Park J. S., Kim Y. J., Jung J. H., Lee J. K., Kwon H. C., et al. (2011). Apoptosis-inducing effect of diketopiperazine disulfides produced by Aspergillus sp. KMD 901 isolated from marine sediment on HCT116 colon cancer cell lines. J. Appl. Microbiol. 110, 304–313. doi: 10.1111/jam.2010.110.issue-1
Ciavatta M. L., Lefranc F., Vieira L. M., Kiss R., Carbone M., van Otterlo W. A. L., et al. (2020). The phylum bryozoa: from biology to biomedical potential. Mar. Drugs 18 (4), 200. doi: 10.3390/md18040200
Coello L., Martín M. J., Reyes F. (2009). 1,5-diazacyclohenicosane, a new cytotoxic metabolite from the marine sponge Mycale sp. Mar. Drugs 7, 445–450. doi: 10.3390/md7030445
Cortes J., O'Shaughnessy J., Loesch D., Blum J. L., Vahdat L. T., Petrakova K., et al. (2011). Eribulin monotherapy versus treatment of physician's choice in patients with metastatic breast cancer (EMBRACE): a phase 3 open-label randomised study. Lancet 377, 914–923. doi: 10.1016/S0140-6736(11)60070-6
Cortes J., Vahdat L., Blum J. L., Twelves C., Campone M., Roché H., et al. (2010). Phase II study of the halichondrin B analog eribulin mesylate in patients with locally advanced or metastatic breast cancer previously treated with an anthracycline, a taxane, and capecitabine. J. Clin. Oncol. 28, 3922–3928. doi: 10.1200/JCO.2009.25.8467
Cruz P. G., Martínez Leal J. F., Daranas A. H., Pérez M., Cuevas C. (2018). On the mechanism of action of dragmacidins I and J, two new representatives of a new class of protein phosphatase 1 and 2A inhibitors. ACS Omega 3, 3760–3767. doi: 10.1021/acsomega.7b01786
Cui C. B., Kakeya H., Okada G., Onose R., Ubukata M., Takahashi I., et al. (1995). Tryprostatins A and B, novel mammalian cell cycle inhibitors produced by Aspergillus fumigatus. J. Antibiot. (Tokyo) 48, 1382–1384. doi: 10.7164/antibiotics.48.1382
Cui C.-B., Kakeya H., Osada H. (1997). Novel mammalian cell cycle inhibitors, cyclotroprostatins A–D, produced by Aspergillus fumigatus, which inhibit mammalian cell cycle at G2/M phase. Tetrahedron 53, 59–72. doi: 10.1016/S0040-4020(96)00978-7
Dasari V. R. R. K., Muthyala M. K. K., Nikku M. Y., Donthireddy S. R. R. (2012). Novel Pyridinium compound from marine actinomycete, Amycolatopsis alba var. nov. DVR D4 showing antimicrobial and cytotoxic activities in vitro. Microbiological Res. 167, 346–351. doi: 10.1016/j.micres.2011.12.003
Davis R. A., Sandoval I. T., Concepcion G. P., Moreira da Rocha R., Ireland C. M. (2003). Lissoclinotoxins E and F, novel cytotoxic alkaloids from a Philippine didemnid ascidian. Tetrahedron 59, 2855–2859. doi: 10.1016/S0040-4020(03)00335-1
Debbab A., Aly A. H., Lin W. H., Proksch P. (2010). Bioactive compounds from marine bacteria and fungi. Microb. Biotechnol. 3, 544–563. doi: 10.1111/j.1751-7915.2010.00179.x
Delgado E., Taylor K. A., Tran P. (2021). Treatment updates for metastatic breast cancer. US Pharm. 46, 42–46. Available at: https://www.uspharmacist.com/article/treatment-updates-for-metastatic-breast-cancer.
Demay J., Bernard C., Reinhardt A., Marie B. (2019). Natural products from cyanobacteria: focus on beneficial activities. Mar. Drugs 17 (6), 320. doi: 10.3390/md17060320
de Oliveira J. H., Grube A., Köck M., Berlinck R. G., Macedo M. L., Ferreira A. G., et al. (2004). Ingenamine G and cyclostellettamines G-I, K, and L from the new Brazilian species of marine sponge Pachychalina sp. J. Nat. Prod 67, 1685–1689. doi: 10.1021/np0498713
de Oliveira J. H., Nascimento A. M., Kossuga M. H., Cavalcanti B. C., Pessoa C. O., Moraes M. O., et al. (2007). Cytotoxic alkylpiperidine alkaloids from the Brazilian marine sponge Pachychalina alcaloidifera. J. Nat. Prod 70, 538–543. doi: 10.1021/np060450q
Dhatchana Moorthy N., Muthu Ramalingam B., Iqbal S., Mohanakrishnan A. K., Gunasekaran K., Vellaichamy E. (2018). Novel isothiacalothrixin B analogues exhibit cytotoxic activity on human colon cancer cells in vitro by inducing irreversible DNA damage. PloS One 13, e0202903. doi: 10.1371/journal.pone.0202903
Diana P., Carbone A., Barraja P., Martorana A., Gia O., DallaVia L., et al. (2007). 3,5-Bis(3′-indolyl)pyrazoles, analogues of marine alkaloid nortopsentin: Synthesis and antitumor properties. Bioorganic Medicinal Chem. Lett. 17, 6134–6137. doi: 10.1016/j.bmcl.2007.09.042
Ding Y.-S., Kim W.-S., Park S. J., Kim S.-K. (2018). Apoptotic effect of physcion isolated from marine fungus Microsporum sp. in PC3 human prostate cancer cells. Fisheries Aquat. Sci. 21, 22. doi: 10.1186/s41240-018-0099-7
Ding L., Li W., Zhong X., Feng F., Xin Y., Yan X., et al. (2023). Bresmycins A and B, potent anti-breast cancer indolocarbazole alkaloids from the sponge-associated Streptomyces sp. NBU3142. J. Mol. Structure 1290, 135809. doi: 10.1016/j.molstruc.2023.135809
Dini I., Soekamto N., Firdaus F., Supratman U., Latip J. (2021). Alkaloid Caulerpin and Cytotoxic Activity against NCL-H460 Lung Cancer Cells Isolated along with β-sitosterol from the Halimeda cylindracea Decaisne. Sains Malaysiana 50, 2663–2674. doi: 10.17576/jsm-2021-5009-14
Donia M. S., Wang B., Dunbar D. C., Desai P. V., Patny A., Avery M., et al. (2008). Mollamides B and C, Cyclic hexapeptides from the Indonesian tunicate Didemnum molle. J. Nat. Prod 71, 941–945. doi: 10.1021/np700718p
Dung D. T., Hang D. T. T., Yen P. H., Quang T. H., Nhiem N. X., Tai B. H., et al. (2019). Macrocyclic bis-quinolizidine alkaloids from Xestospongia muta. Nat. Prod Res. 33, 400–406. doi: 10.1080/14786419.2018.1455043
Dyshlovoy S. A., Fedorov S. N., Shubina L. K., Kuzmich A. S., Bokemeyer C., Keller-von Amsberg G., et al. (2014). Aaptamines from the marine sponge Aaptos sp. display anticancer activities in human cancer cell lines and modulate AP-1-, NF-κB-, and p53-dependent transcriptional activity in mouse JB6 Cl41 cells. BioMed. Res. Int. 2014, 469309. doi: 10.1155/2014/469309
Dyson L., Wright A. D., Young K. A., Sakoff J. A., McCluskey A. (2014). Synthesis and anticancer activity of focused compound libraries from the natural product lead, oroidin. Bioorg. Med. Chem. 22, 1690–1699. doi: 10.1016/j.bmc.2014.01.021
El-Demerdash A., Moriou C., Martin M. T., Rodrigues-Stien Ade S., Petek S., Demoy-Schneider M., et al. (2016). Cytotoxic Guanidine Alkaloids from a French Polynesian Monanchora n. sp. Sponge. J. Nat. Prod 79, 1929–1937. doi: 10.1021/acs.jnatprod.6b00168
El Gamal A. A. (2010). Biological importance of marine algae. Saudi Pharm. J. 18, 1–25. doi: 10.1016/j.jsps.2009.12.001
Elissawy A. M., Soleiman Dehkordi E., Mehdinezhad N., Ashour M. L., Mohammadi Pour P. (2021). Cytotoxic alkaloids derived from marine sponges: A comprehensive review. Biomolecules 11 (2), 258. doi: 10.3390/biom11020258
Erol E., Orhan M. D., Avsar T., Akdemir A., Okudan E. S., Alim Toraman G. O., et al. (2022). Anti-SARS-CoV-2 and cytotoxic activity of two marine alkaloids from green alga Caulerpa cylindracea Sonder in the Dardanelles. RSC Adv. 12, 29983–29990. doi: 10.1039/D2RA03358E
Fenical W., Jensen P. R., Cheng X. C. (2000). Avrainvillamide, a cytotoxic marine natural product, and derivatives thereof. U.S. Patent 6, 066,635. Available at: https://patents.google.com/patent/US6066635A/en
Fernandes A. S., Oliveira C., Reis R. L., Martins A., Silva T. H. (2022). Marine-inspired drugs and biomaterials in the perspective of pancreatic cancer therapies. Mar. Drugs 20, 689. doi: 10.3390/md20110689
Figuerola B., Avila C. (2019). The phylum bryozoa as a promising source of anticancer drugs. Mar. Drugs 17 (8), 477. doi: 10.3390/md17080477
Florean C., Schnekenburger M., Lee J. Y., Kim K. R., Mazumder A., Song S., et al. (2016). Discovery and characterization of Isofistularin-3, a marine brominated alkaloid, as a new DNA demethylating agent inducing cell cycle arrest and sensitization to TRAIL in cancer cells. Oncotarget 7, 24027–24049. doi: 10.18632/oncotarget.v7i17
Fracasso P. M., Williams K. J., Chen R. C., Picus J., Ma C. X., Ellis M. J., et al. (2011). A Phase 1 study of UCN-01 in combination with irinotecan in patients with resistant solid tumor Malignancies. Cancer Chemother. Pharmacol. 67, 1225–1237. doi: 10.1007/s00280-010-1410-1
Gadducci A., Cosio S. (2022). Trabectedin and lurbinectedin: Mechanisms of action, clinical impact, and future perspectives in uterine and soft tissue sarcoma, ovarian carcinoma, and endometrial carcinoma. Front. Oncol. 12. doi: 10.3389/fonc.2022.914342
Glaser K. B., Mayer A. M. (2009). A renaissance in marine pharmacology: from preclinical curiosity to clinical reality. Biochem. Pharmacol. 78, 440–448. doi: 10.1016/j.bcp.2009.04.015
Goda A. A., Siddique A. B., Mohyeldin M., Ayoub N. M., El Sayed K. A. (2018). The maxi-K (BK) channel antagonist penitrem A as a novel breast cancer-targeted therapeutic. Mar. Drugs 16 (5), 157. doi: 10.3390/md16050157
Goel S., Mita A. C., Mita M., Rowinsky E. K., Chu Q. S., Wong N., et al. (2009). A phase I study of eribulin mesylate (E7389), a mechanistically novel inhibitor of microtubule dynamics, in patients with advanced solid Malignancies. Clin. Cancer Res. 15, 4207–4212. doi: 10.1158/1078-0432.CCR-08-2429
Gogineni V., Oh J., Waters A. L., Kelly M., Stone R., Hamann M. T. (2020). Monanchocidin A from subarctic sponges of the genus monanchora and their promising selectivity against melanoma. vitro 7, 58. doi: 10.3389/fmars.2020.00058
Gonçalves M. F. M., Esteves A. C., Alves A. (2022). Marine fungi: opportunities and challenges. Encyclopedia 2 (1), 559–577. doi: 10.3390/encyclopedia2010037
Gourdeau H., McAlpine J. B., Ranger M., Simard B., Berger F., Beaudry F., et al. (2008). Identification, characterization and potent antitumor activity of ECO-4601, a novel peripheral benzodiazepine receptor ligand. Cancer Chemother. Pharmacol. 61, 911–921. doi: 10.1007/s00280-007-0544-2
Gross H., Goeger D. E., Hills P., Mooberry S. L., Ballantine D. L., Murray T. F., et al. (2006). Lophocladines, bioactive alkaloids from the red alga Lophocladia sp. J. Nat. Prod 69, 640–644. doi: 10.1021/np050519e
Gul W., Hamann M. T. (2005). Indole alkaloid marine natural products: an established source of cancer drug leads with considerable promise for the control of parasitic, neurological and other diseases. Life Sci. 78, 442–453. doi: 10.1016/j.lfs.2005.09.007
Han X.-X., Cui C.-B., Gu Q.-Q., Zhu W.-M., Liu H.-B., Gu J.-Y., et al. (2005). ZHD-0501, a novel naturally occurring staurosporine analog from Actinomadura sp. 007. Tetrahedron Lett. 46, 6137–6140. doi: 10.1016/j.tetlet.2005.06.154
Han N., Li J., Li X. (2022). Natural marine products: anti-colorectal cancer in vitro and in vivo. Mar. Drugs 20 (6), 349. doi: 10.3390/md20060349
Hansen K., Isaksson J., Bayer A., Johansen J. A., Andersen J. H., Hansen E. (2017). Securamine derivatives from the arctic bryozoan securiflustra securifrons. J. Nat. Prod 80, 3276–3283. doi: 10.1021/acs.jnatprod.7b00703
Hanssen K., Hansen I., Richard C., Andersen J. (2021). Antimicrobial activity of securamines from the bryozoan securiflustra securifrons. Natural Product Commun. 16 (2), 1934578X21996180. doi: 10.1177/1934578X21996180
Harbeck N., Penault-Llorca F., Cortes J., Gnant M., Houssami N., Poortmans P., et al. (2019). Breast cancer. Nat. Rev. Dis. Primers 5, 66. doi: 10.1038/s41572-019-0111-2
Hasler-Strub U., Mueller A., Li Q., Thuerlimann B., Ribi K., Gerber S., et al. (2023). Eribulin as first-line treatment in older patients with advanced breast cancer: A multicenter phase II trial [SAKK 25/14]. J. Geriatric Oncol. 14 (1), 101372. doi: 10.1016/j.jgo.2022.09.001
Hayashida T., Jinno H., Mori K., Sato H., Matsui A., Sakurai T., et al. (2018). Phase II trial of eribulin mesylate as a first- or second-line treatment for locally advanced or metastatic breast cancer: a multicenter, single-arm trial. BMC Cancer 18, 701. doi: 10.1186/s12885-018-4628-7
Hoda M. A., Pirker C., Dong Y., Schelch K., Heffeter P., Kryeziu K., et al. (2016). Trabectedin is active against Malignant pleural mesothelioma cell and xenograft models and synergizes with chemotherapy and bcl-2 inhibition in vitro. Mol. Cancer Ther. 15, 2357–2369. doi: 10.1158/1535-7163.MCT-15-0846
Hohmann C., Schneider K., Bruntner C., Irran E., Nicholson G., Bull A. T., et al. (2009). Caboxamycin, a new antibiotic of the benzoxazole family produced by the deep-sea strain Streptomyces sp. NTK 937. J. Antibiotics 62, 99–104. doi: 10.1038/ja.2008.24
Huang S., Chen H., Li W., Zhu X., Ding W., Li C. (2016). Bioactive chaetoglobosins from the mangrove endophytic fungus penicillium chrysogenum. Mar. Drugs 14 (10), 172. doi: 10.3390/md14100172
Hussain A., Bourguet-Kondracki M. L., Majeed M., Ibrahim M., Imran M., Yang X. W., et al. (2023). Marine life as a source for breast cancer treatment: A comprehensive review. BioMed. Pharmacother. 159, 114165. doi: 10.1016/j.biopha.2022.114165
Huyck T. K., Gradishar W., Manuguid F., Kirkpatrick P. (2011). Eribulin mesylate. Nat. Rev. Drug Discovery 10, 173–174. doi: 10.1038/nrd3389
Ibrahim S. R., Mohamed G. A. (2017). Ingenine E, a new cytotoxic β-carboline alkaloid from the Indonesian sponge Acanthostrongylophora ingens. J. Asian Nat. Prod Res. 19, 504–509. doi: 10.1080/10286020.2016.1213723
Imperatore C., Aiello A., D'Aniello F., Senese M., Menna M. (2014). Alkaloids from marine invertebrates as important leads for anticancer drugs discovery and development. Molecules 19, 20391–20423. doi: 10.3390/molecules191220391
Inoue K., Saito T., Okubo K., Kimizuka K., Yamada H., Sakurai T., et al. (2016). Phase II clinical study of eribulin monotherapy in Japanese patients with metastatic breast cancer who had well-defined taxane resistance. Breast Cancer Res. Treat 157, 295–305. doi: 10.1007/s10549-016-3808-x
Iwasa T., Tsurutani J., Watanabe S., Kato R., Mizuno Y., Kojima Y., et al. (2019). Multicentre, phase II study of eribulin in combination with S-1 in patients with advanced breast cancer. BMC Cancer 19, 962. doi: 10.1186/s12885-019-6200-5
Izumida M., Kotani O., Hayashi H., Smith C., Fukuda T., Suga K., et al. (2022). Unique mode of antiviral action of a marine alkaloid against ebola virus and SARS-coV-2. Viruses 14 (4), 816. doi: 10.3390/v14040816
Jeong S.-Y., Shin H. J., Kim T. S., Lee H.-S., Park S.-K., Kim H. M. (2006). Streptokordin, a new cytotoxic compound of the methylpyridine class from a marine-derived streptomyces sp. KORDI-3238. J. Antibiotics 59, 234–240. doi: 10.1038/ja.2006.33
Kadiri S. K., Nagendra, Yarla A., Vidavalur S. (2013). Isolation and identification of A novel aporphine alkaloid SSV, an antitumor antibiotic from fermented broth of marine associated streptomyces sp. KS1908. J. Mar. Sci.: Res. Dev. 3, 1–5. doi: 10.4172/2155-9910
Kanno S., Yomogida S., Tomizawa A., Yamazaki H., Ukai K., Mangindaan R. E., et al. (2013). Papuamine causes autophagy following the reduction of cell survival through mitochondrial damage and JNK activation in MCF-7 human breast cancer cells. Int. J. Oncol. 43, 1413–1419. doi: 10.3892/ijo.2013.2093
Kaufman P. A., Awada A., Twelves C., Yelle L., Perez E. A., Velikova G., et al. (2015). Phase III open-label randomized study of eribulin mesylate versus capecitabine in patients with locally advanced or metastatic breast cancer previously treated with an anthracycline and a taxane. J. Clin. Oncol. 33, 594–601. doi: 10.1200/JCO.2013.52.4892
Kijjoa A., Wattanadilok R., Campos N., Nascimento M. S., Pinto M., Herz W. (2007). Anticancer activity evaluation of kuanoniamines A and C isolated from the marine sponge Oceanapia sagittaria, collected from the Gulf of Thailand. Mar. Drugs 5, 6–22. doi: 10.3390/md502006
Kim H., Lantvit D., Hwang C. H., Kroll D. J., Swanson S. M., Franzblau S. G., et al. (2012). Indole alkaloids from two cultured cyanobacteria, Westiellopsis sp. and Fischerella muscicola. Bioorg. Med. Chem. 20, 5290–5295. doi: 10.1016/j.bmc.2012.06.030
Klochkov S. G., Neganova M. E., Yarla N. S., Parvathaneni M., Sharma B., Tarasov V. V., et al. (2019). Implications of farnesyltransferase and its inhibitors as a promising strategy for cancer therapy. Semin. Cancer Biol. 56, 128–134. doi: 10.1016/j.semcancer.2017.10.010
Kobayashi J.i., Murayama T., Ishibashi M., Kosuge S., Takamatsu M., Ohizumi Y., et al. (1990). Hyrtiosins A and B, new indole alkaloids from the Okinawan marine sponge Hyrtios erecta. Tetrahedron 46, 7699–7702. doi: 10.1016/S0040-4020(01)90065-1
Koczywas M., Frankel P. H., Synold T. W., Lenz H. J., Mortimer J. E., El-Khoueiry A. B., et al. (2014). Phase I study of the halichondrin B analogue eribulin mesylate in combination with cisplatin in advanced solid tumors. Br. J. Cancer 111, 2268–2274. doi: 10.1038/bjc.2014.554
Koh J., Kubota T., Koyama T., Migita T., Hashimoto M., Hosoda Y., et al. (2003). Combined antitumor activity of 7-hydroxystaurosporine (UCN-01) and tamoxifen against human breast carcinoma in vitro and in vivo. Breast Cancer 10, 260–267. doi: 10.1007/BF02966727
Lee S. I., Brown M. K., Eastman A. (1999). Comparison of the efficacy of 7-hydroxystaurosporine (UCN-01) and other staurosporine analogs to abrogate cisplatin-induced cell cycle arrest in human breast cancer cell lines. Biochem. Pharmacol. 58, 1713–1721. doi: 10.1016/S0006-2952(99)00258-0
Lee J. C., Hou M. F., Huang H. W., Chang F. R., Yeh C. C., Tang J. Y., et al. (2013). Marine algal natural products with anti-oxidative, anti-inflammatory, and anti-cancer properties. Cancer Cell Int. 13, 55. doi: 10.1186/1475-2867-13-55
Li Y. X., Himaya S. W., Dewapriya P., Zhang C., Kim S. K. (2013). Fumigaclavine C from a marine-derived fungus Aspergillus fumigatus induces apoptosis in MCF-7 breast cancer cells. Mar. Drugs 11, 5063–5086. doi: 10.3390/md11125063
Li S., Tian X., Niu S., Zhang W., Chen Y., Zhang H., et al. (2011). Pseudonocardians A-C, new diazaanthraquinone derivatives from a deap-sea actinomycete Pseudonocardia sp. SCSIO 01299. Mar. Drugs 9, 1428–1439. doi: 10.3390/md9081428
Liberio M. S., Sadowski M. C., Davis R. A., Rockstroh A., Vasireddy R., Lehman M. L., et al. (2015). The ascidian natural product eusynstyelamide B is a novel topoisomerase II poison that induces DNA damage and growth arrest in prostate and breast cancer cells. Oncotarget 6, 43944–43963. doi: 10.18632/oncotarget.v6i41
Li Petri G., Pecoraro C., Randazzo O., Zoppi S., Cascioferro S. M., Parrino B., et al. (2020). New imidazo[2,1-b][1,3,4]Thiadiazole derivatives inhibit FAK phosphorylation and potentiate the antiproliferative effects of gemcitabine through modulation of the human equilibrative nucleoside transporter-1 in peritoneal mesothelioma. Anticancer Res. 40, 4913–4919. doi: 10.21873/anticanres.14494
Liu R., Liu Y., Zhou Y. D., Nagle D. G. (2007). Molecular-targeted antitumor agents. 15. Neolamellarins from the marine sponge Dendrilla nigra inhibit hypoxia-inducible factor-1 activation and secreted vascular endothelial growth factor production in breast tumor cells. J. Nat. Prod 70, 1741–1745. doi: 10.1021/np070206e
Liu Y., Morgan J. B., Coothankandaswamy V., Liu R., Jekabsons M. B., Mahdi F., et al. (2009). The Caulerpa pigment caulerpin inhibits HIF-1 activation and mitochondrial respiration. J. Nat. Prod 72, 2104–2109. doi: 10.1021/np9005794
Lyu C., Chen T., Qiang B., Liu N., Wang H., Zhang L., et al. (2021). CMNPD: a comprehensive marine natural products database towards facilitating drug discovery from the ocean. Nucleic Acids Res. 49, D509–d515. doi: 10.1093/nar/gkaa763
Ma C. X., Ellis M. J., Petroni G. R., Guo Z., Cai S. R., Ryan C. E., et al. (2013). A phase II study of UCN-01 in combination with irinotecan in patients with metastatic triple negative breast cancer. Breast Cancer Res. Treat 137, 483–492. doi: 10.1007/s10549-012-2378-9
Malhão F., Ramos A. A., Buttachon S., Dethoup T., Kijjoa A., Rocha E. (2019). Cytotoxic and antiproliferative effects of preussin, a hydroxypyrrolidine derivative from the marine sponge-associated fungus aspergillus candidus KUFA 0062, in a panel of breast cancer cell lines and using 2D and 3D cultures. Mar. Drugs 17 (8), 448. doi: 10.3390/md17080448
Mani L., Petek S., Valentin A., Chevalley S., Folcher E., Aalbersberg W., et al. (2011). The in vivo anti-plasmodial activity of haliclonacyclamine A, an alkaloid from the marine sponge, Haliclona sp. Nat. Prod Res. 25, 1923–1930. doi: 10.1080/14786419.2010.547858
Manzo E., van Soest R., Matainaho L., Roberge M., Andersen R. J. (2003). Ceratamines A and B, antimitotic heterocyclic alkaloids isolated from the marine sponge Pseudoceratina sp. collected in Papua New Guinea. Org. Lett. 5, 4591–4594. doi: 10.1021/ol035721s
Martin G. D., Tan L. T., Jensen P. R., Dimayuga R. E., Fairchild C. R., Raventos-Suarez C., et al. (2007). Marmycins A and B, cytotoxic pentacyclic C-glycosides from a marine sediment-derived actinomycete related to the genus Streptomyces. J. Nat. Prod 70, 1406–1409. doi: 10.1021/np060621r
Maskey R. P., Li F., Qin S., Fiebig H. H., Laatsch H. (2003). Chandrananimycins A approximately C: production of novel anticancer antibiotics from a marine Actinomadura sp. isolate M048 by variation of medium composition and growth conditions. J. Antibiot. (Tokyo) 56, 622–629. doi: 10.7164/antibiotics.56.622
Mayer E. L. (2013). Early and late long-term effects of adjuvant chemotherapy. Am. Soc. Clin. Oncol. Educ. Book, 9–14. doi: 10.14694/EdBook_AM.2013.33.9
Michael P., Hansen K., Isaksson J., Andersen J. H., Hansen E. (2017). A novel brominated alkaloid securidine A, isolated from the marine bryozoan securiflustra securifrons. Molecules 22 (7), 1236. doi: 10.3390/molecules22071236
Miller K. D., Nogueira L., Devasia T., Mariotto A. B., Yabroff K. R., Jemal A., et al. (2022). Cancer treatment and survivorship statistic. CA Cancer J. Clin. 72, 409–436. doi: 10.3322/caac.21731
Mizuno K., Noda K., Ueda Y., Hanaki H., Saido T. C., Ikuta T., et al. (1995). UCN-01, an anti-tumor drug, is a selective inhibitor of the conventional PKC subfamily. FEBS Lett. 359, 259–261. doi: 10.1016/0014-5793(95)00042-8
Montuori E., de Pascale D., Lauritano C. (2022). Recent discoveries on marine organism immunomodulatory activities. Mar. Drugs 20 (7), 422. doi: 10.3390/md20070422
Mooberry S. L., Tien G., Hernandez A. H., Plubrukarn A., Davidson B. S. (1999). Laulimalide and isolaulimalide, new paclitaxel-like microtubule-stabilizing agents. Cancer Res. 59, 653–660.
Morvan D. (2013). Functional metabolomics uncovers metabolic alterations associated to severe oxidative stress in MCF7 breast cancer cells exposed to ascididemin. Mar. Drugs 11, 3846–3860. doi: 10.3390/md11103846
Murcia C., Coello L., Fernández R., Martín M. J., Reyes F., Francesch A., et al. (2014). Tanjungides A and B: new antitumoral bromoindole derived compounds from Diazona cf formosa. isolation and total synthesis. Mar. Drugs 12, 1116–1130. doi: 10.3390/md12021116
Muthu Ramalingam B., Dhatchana Moorthy N., Chowdhury S. R., Mageshwaran T., Vellaichamy E., Saha S., et al. (2018). Synthesis and biological evaluation of calothrixins B and their deoxygenated analogues. J. Medicinal Chem. 61, 1285–1315. doi: 10.1021/acs.jmedchem.7b01797
Oda T., Kamoshita K., Maruyama S., Masuda K., Nishimoto M., Xu J., et al. (2007). Cytotoxicity of Lissoclibadins and Lissoclinotoxins, Isolated from a Tropical Ascidian Lissoclinum cf. badium, against Human Solid-Tumor-Derived Cell Lines. Biol. Pharm. Bull. 30, 385–387. doi: 10.1248/bpb.30.385
Orditura M., Gravina A., Riccardi F., Diana A., Mocerino C., Leopaldi L., et al. (2017). Eribulin for metastatic breast cancer (MBC) treatment: a retrospective, multicenter study based in Campania, south Italy (Eri-001 trial). ESMO Open 2, e000176. doi: 10.1136/esmoopen-2017-000176
Palanisamy S. K., Rajendran N. M., Marino A. (2017). Natural products diversity of marine ascidians (Tunicates; ascidiacea) and successful drugs in clinical development. Nat. Prod Bioprospect 7, 1–111. doi: 10.1007/s13659-016-0115-5
Pejin B., Mojović M. D., Savić A. (2014). Novel antitumour natural products from the phylum Bryozoa. (Serbia: Biologia Serbica).
Pellegrino B., Cavanna L., Boggiani D., Zamagni C., Frassoldati A., Schirone A., et al. (2021). Phase II study of eribulin in combination with gemcitabine for the treatment of patients with locally advanced or metastatic triple negative breast cancer (ERIGE trial). Clinical and pharmacogenetic results on behalf of the Gruppo Oncologico Italiano di Ricerca Clinica (GOIRC). ESMO Open 6 (1), 100019. doi: 10.1016/j.esmoop.2020.100019
Pisani P., Bray F., Parkin D. M. (2002). Estimates of the world-wide prevalence of cancer for 25 sites in the adult population. Int. J. Cancer 97, 72–81. doi: 10.1002/ijc.1571
Pla D., Marchal A., Olsen C. A., Francesch A., Cuevas C., Albericio F., et al. (2006). Synthesis and structure-activity relationship study of potent cytotoxic analogues of the marine alkaloid Lamellarin D. J. Med. Chem. 49, 3257–3268. doi: 10.1021/jm0602458
Plubrukarn A., Davidson B. S. (1998). Arnoamines A and B, new cytotoxic pentacyclic pyridoacridine alkaloids from the ascidian cystodytes sp. J. Organic Chem. 63, 1657–1659. doi: 10.1021/jo9719721
Proksch P., Edrada R. A., Ebel R. (2002). Drugs from the seas - current status and microbiological implications. Appl. Microbiol. Biotechnol. 59, 125–134. doi: 10.1007/s00253-002-1006-8
Rabindran S. K., Ross D. D., Doyle L. A., Yang W., Greenberger L. M. (2000). Fumitremorgin C reverses multidrug resistance in cells transfected with the breast cancer resistance protein. Cancer Res. 60, 47–50.
Ramesh C., Tulasi B. R., Raju M., Thakur N., Dufossé L. (2021). Marine natural products from tunicates and their associated microbes. Mar. Drugs 19 (6), 308. doi: 10.3390/md19060308
Reyes F., Fernández R., Rodríguez A., Bueno S., de Eguilior C., Francesch A., et al. (2008a). Cytotoxic staurosporines from the marine ascidian Cystodytes solitus. J. Nat. Prod 71, 1046–1048. doi: 10.1021/np700748h
Reyes F., Fernández R., Rodríguez A., Francesch A., Taboada S., Ávila C., et al. (2008b). Aplicyanins A–F, new cytotoxic bromoindole derivatives from the marine tunicate Aplidium cyaneum. Tetrahedron 64, 5119–5123. doi: 10.1016/j.tet.2008.03.060
Roberge M., Berlinck R. G., Xu L., Anderson H. J., Lim L. Y., Curman D., et al. (1998). High-throughput assay for G2 checkpoint inhibitors and identification of the structurally novel compound isogranulatimide. Cancer Res. 58, 5701–5706.
Saha S., Reddy Ch V., Xu S., Sankar S., Neamati N., Patro B. (2013). Synthesis and SAR studies of marine natural products ma'edamines A, B and their analogues. Bioorg. Med. Chem. Lett. 23, 5135–5139. doi: 10.1016/j.bmcl.2013.07.017
Sakaguchi K., Nakatsukasa K., Koyama H., Kato M., Sakuyama A., Matsuda T., et al. (2018). Phase II clinical trial of first-line eribulin plus trastuzumab for advanced or recurrent HER2-positive breast cancer. Anticancer Res. 38, 4073–4081. doi: 10.21873/anticanres.12697
Sallam A. A., Ayoub N. M., Foudah A. I., Gissendanner C. R., Meyer S. A., El Sayed K. A. (2013a). Indole diterpene alkaloids as novel inhibitors of the Wnt/β-catenin pathway in breast cancer cells. Eur. J. Med. Chem. 70, 594–606. doi: 10.1016/j.ejmech.2013.09.045
Sallam A. A., Houssen W. E., Gissendanner C. R., Orabi K. Y., Foudah A. I., El Sayed K. A. (2013b). Bioguided discovery and pharmacophore modeling of the mycotoxic indole diterpene alkaloids penitrems as breast cancer proliferation, migration, and invasion inhibitors. Medchemcomm 4 (10), 10. doi: 10.1039/c3md00198a
Salucci S., Burattini S., Buontempo F., Orsini E., Furiassi L., Mari M., et al. (2018). Marine bisindole alkaloid: A potential apoptotic inducer in human cancer cells. Eur. J. Histochem 62, 2881. doi: 10.4081/ejh.2018.2881
Samoylenko V., Khan S. I., Jacoba M. R., Tekwani B. L., Walker L. A., Hufford C. D., et al. (2009). Bioactive (+)-manzamine A and (+)-8-hydroxymanzamine A tertiary bases and salts from Acanthostrongylophora ingens and their preparations. Nat. Prod Commun. 4, 185–192. doi: 10.1177/1934578X0900400204
Seabra R., Malhão F., Correia A., Costa C., Kijjoa A., Rocha E. (2023). Effects and mechanisms of action of preussin, a marine fungal metabolite, against the triple-negative breast cancer cell line, MDA-MB-231, in 2D and 3D cultures. Mar. Drugs 21 (3), 166. doi: 10.3390/md21030166
Segraves N. L., Robinson S. J., Garcia D., Said S. A., Fu X., Schmitz F. J., et al. (2004). Comparison of fascaplysin and related alkaloids: a study of structures, cytotoxicities, and sources. J. Nat. Prod 67, 783–792. doi: 10.1021/np049935+
Shaala L. A., Youssef D. T. A. (2019). Cytotoxic psammaplysin analogues from the verongid red sea sponge aplysinella species. Biomolecules 9 (12), 841. doi: 10.3390/biom9120841
Shaala L. A., Youssef D. T. A. (2021). Hemimycalins C-E; cytotoxic and antimicrobial alkaloids with hydantoin and 2-iminoimidazolidin-4-one backbones from the red sea marine sponge hemimycale sp. Mar. Drugs 19 (12), 691. doi: 10.3390/md19120691
Shaala L. A., Youssef D. T., Badr J. M., Harakeh S. M. (2016). Bioactive 2(1H)-pyrazinones and diketopiperazine alkaloids from a tunicate-derived actinomycete streptomyces sp. Molecules 21 (9), 1116. doi: 10.3390/molecules21091116
Shaala L. A., Youssef D. T. A., Badr J. M., Harakeh S. M., Genta-Jouve G. (2019). Bioactive diketopiperazines and nucleoside derivatives from a sponge-derived streptomyces species. Mar. Drugs 17 (10), 584. doi: 10.3390/md17100584
Shaala L. A., Youssef D. T., Badr J. M., Sulaiman M., Khedr A. (2015a). Bioactive secondary metabolites from the Red Sea marine Verongid sponge Suberea species. Mar. Drugs 13, 1621–1631. doi: 10.3390/md13041621
Shaala L. A., Youssef D. T. A., Badr J. M., Sulaiman M., Khedr A., El Sayed K. A. (2015b). Bioactive alkaloids from the Red Sea marine Verongid sponge Pseudoceratina arabica. Tetrahedron 71, 7837–7841. doi: 10.1016/j.tet.2015.08.024
Shaala L. A., Youssef D. T., Sulaiman M., Behery F. A., Foudah A. I., Sayed K. A. (2012). Subereamolline A as a potent breast cancer migration, invasion and proliferation inhibitor and bioactive dibrominated alkaloids from the Red Sea sponge Pseudoceratina arabica. Mar. Drugs 10, 2492–2508. doi: 10.3390/md10112492
Sharifuddin Y., Chin Y. X., Lim P. E., Phang S. M. (2015). Potential bioactive compounds from seaweed for diabetes management. Mar. Drugs 13, 5447–5491. doi: 10.3390/md13085447
Sharma S., Guru S. K., Manda S., Kumar A., Mintoo M. J., Prasad V. D., et al. (2017). A marine sponge alkaloid derivative 4-chloro fascaplysin inhibits tumor growth and VEGF mediated angiogenesis by disrupting PI3K/Akt/mTOR signaling cascade. Chem. Biol. Interact. 275, 47–60. doi: 10.1016/j.cbi.2017.07.017
Shinkre B. A., Raisch K. P., Fan L., Velu S. E. (2007). Analogs of the marine alkaloid makaluvamines: synthesis, topoisomerase II inhibition, and anticancer activity. Bioorg. Med. Chem. Lett. 17, 2890–2893. doi: 10.1016/j.bmcl.2007.02.065
Shrestha S., Sorolla A., Fromont J., Blancafort P., Flematti G. R. (2018). Crambescidin 800, isolated from the marine sponge monanchora viridis, induces cell cycle arrest and apoptosis in triple-negative breast cancer cells. Mar. Drugs 16 (2), 53. doi: 10.3390/md16020053
Smith C. D., Zilfou J. T., Stratmann K., Patterson G. M., Moore R. E. (1995). Welwitindolinone analogues that reverse P-glycoprotein-mediated multiple drug resistance. Mol. Pharmacol. 47, 241–247.
Song Y., Yang J., Yu J., Li J., Yuan J., Wong N. K., et al. (2020). Chlorinated bis-indole alkaloids from deep-sea derived Streptomyces sp. SCSIO 11791 with antibacterial and cytotoxic activities. J. Antibiot. (Tokyo) 73, 542–547. doi: 10.1038/s41429-020-0307-4
Sorek H., Rudi A., Gueta S., Reyes F., Martin M. J., Aknin M., et al. (2006). Netamines A–G: seven new tricyclic guanidine alkaloids from the marine sponge Biemna laboutei. Tetrahedron 62, 8838–8843. doi: 10.1016/j.tet.2006.06.063
Souza C. R. M., Bezerra W. P., Souto J. T. (2020). Marine alkaloids with anti-inflammatory activity: current knowledge and future perspectives. Mar. Drugs 18 (3), 147. doi: 10.3390/md18030147
Sung H., Ferlay J., Siegel R. L., Laversanne M., Soerjomataram I., Jemal A., et al. (2021). Global cancer statistics 2020: GLOBOCAN estimates of incidence and mortality worldwide for 36 cancers in 185 countries. CA Cancer J. Clin. 71, 209–249. doi: 10.3322/caac.21660
Synold T. W., Morgan R. J., Newman E. M., Lenz H. J., Gandara D. R., Colevas A. D., et al. (2005). A phase I pharmacokinetic and target validation study of the novel anti-tubulin agent E7389: A California Cancer Consortium trial. J. Clin. Oncol. 23, 3036–3036. doi: 10.1200/jco.2005.23.16_suppl.3036
Taamma A., Misset J. L., Riofrio M., Guzman C., Brain E., Lopez Lazaro L., et al. (2001). Phase I and pharmacokinetic study of ecteinascidin-743, a new marine compound, administered as a 24-hour continuous infusion in patients with solid tumors. J. Clin. Oncol. 19, 1256–1265. doi: 10.1200/JCO.2001.19.5.1256
Tabakmakher K. M., Denisenko V. A., Guzii A. G., Dmitrenok P. S., Dyshlovoy S. A., Lee H. S., et al. (2013). Monanchomycalin C, a new pentacyclic guanidine alkaloid from the far-eastern marine sponge Monanchora pulchra. Nat. Prod Commun. 8, 1399–1402. doi: 10.1177/1934578X1300801014
Takahashi N., Li W., Banerjee D., Guan Y., Wada-Takahashi Y., Brennan M. F., et al. (2002). Sequence-dependent synergistic cytotoxicity of ecteinascidin-743 and paclitaxel in human breast cancer cell lines in vitro and in vivo. Cancer Res. 62, 6909–6915.
Takashima T., Tokunaga S., Tei S., Nishimura S., Kawajiri H., Kashiwagi S., et al. (2016). A phase II, multicenter, single-arm trial of eribulin as first-line chemotherapy for HER2-negative locally advanced or metastatic breast cancer. SpringerPlus 5, 164. doi: 10.1186/s40064-016-1833-1
Tang W. Z., Yang Z. Z., Sun F., Wang S. P., Yang F., Jiao W. H., et al. (2019). (-)-Calcaridine B, a new chiral aminoimidazole-containing alkaloid from the marine sponge Leucetta chagosensis. J. Asian Nat. Prod Res. 21, 1123–1128. doi: 10.1080/10286020.2018.1499729
Tang W. Z., Yang Z. Z., Sun F., Wang S. P., Yang F., Lin H. W. (2017). Leucanone A and naamine J, glycerol ether lipid and imidazole alkaloid from the marine sponge Leucandra sp. J. Asian Nat. Prod Res. 19, 691–696. doi: 10.1080/10286020.2016.1240171
Tarazona G., Santamaría G., Cruz P. G., Fernández R., Pérez M., Martínez-Leal J. F., et al. (2017). Cytotoxic anomoian B and aplyzanzine B, new bromotyrosine alkaloids from Indonesian sponges. ACS Omega 2, 3494–3501. doi: 10.1021/acsomega.7b00417
Tatsuta T., Hosono M., Rotinsulu H., Wewengkang D. S., Sumilat D. A., Namikoshi M., et al. (2017). Lissoclibadin 1, a Polysulfur Aromatic Alkaloid from the Indonesian Ascidian Lissoclinum cf. badium, Induces Caspase-Dependent Apoptosis in Human Colon Cancer Cells and Suppresses Tumor Growth in Nude Mice. J. Nat. Prod 80, 499–502. doi: 10.1021/acs.jnatprod.6b01051
Tempone A. G., Pieper P., Borborema S. E. T., Thevenard F., Lago J. H. G., Croft S. L., et al. (2021). Marine alkaloids as bioactive agents against protozoal neglected tropical diseases and malaria. Nat. Prod Rep. 38, 2214–2235. doi: 10.1039/D0NP00078G
Tohme R., Darwiche N., Gali-Muhtasib H. (2011). A journey under the sea: the quest for marine anti-cancer alkaloids. Molecules 16, 9665–9696. doi: 10.3390/molecules16119665
Tolaney S. M., Barroso-Sousa R., Keenan T., Trippa L., Hu J., Luis I. M. V. D., et al. (2019). Randomized phase II study of eribulin mesylate (E) with or without pembrolizumab (P) for hormone receptor-positive (HR+) metastatic breast cancer (MBC). J. Clin. Oncol. 37, 1004–1004. doi: 10.1200/JCO.2019.37.15_suppl.1004
Toumatia O., Yekkour A., Goudjal Y., Riba A., Coppel Y., Mathieu F., et al. (2015). Antifungal properties of an actinomycin D-producing strain, Streptomyces sp. IA1, isolated from a Saharan soil. J. Basic Microbiol. 55, 221–228. doi: 10.1002/jobm.201400202
Towle M. J., Salvato K. A., Budrow J., Wels B. F., Kuznetsov G., Aalfs K. K., et al. (2001). In vitro and in vivo anticancer activities of synthetic macrocyclic ketone analogues of halichondrin B. Cancer Res. 61, 1013–1021.
Tran T. D., Cartner L. K., Bokesch H. R., Henrich C. J., Wang X. W., Mahidol C., et al. (2021). NMR characterization of rearranged staurosporine aglycone analogues from the marine sponge Damiria sp. Magn. Reson. Chem. 59, 534–539. doi: 10.1002/mrc.4932
Trang D. T., Huu Tai B., Hang D. T., Yen P. H., Huong P. T. T., Nhiem N. X., et al. (2021). Chemical constituents of the marine sponge aaptos aaptos (Schmidt 1864) and their cytotoxic activity. Natural Product Commun. 16, 1934578X21993345. doi: 10.1177/1934578X21993345
Trapani D., Ginsburg O., Fadelu T., Lin N. U., Hassett M., Ilbawi A. M., et al. (2022). Global challenges and policy solutions in breast cancer control. Cancer Treat Rev. 104, 102339. doi: 10.1016/j.ctrv.2022.102339
Vahdat L. T., Pruitt B., Fabian C. J., Rivera R. R., Smith D. A., Tan-Chiu E., et al. (2009). Phase II study of eribulin mesylate, a halichondrin B analog, in patients with metastatic breast cancer previously treated with an anthracycline and a taxane. J. Clin. Oncol. 27, 2954–2961. doi: 10.1200/JCO.2008.17.7618
Verdier-Pinard P., Lai J. Y., Yoo H. D., Yu J., Marquez B., Nagle D. G., et al. (1998). Structure-activity analysis of the interaction of curacin A, the potent colchicine site antimitotic agent, with tubulin and effects of analogs on the growth of MCF-7 breast cancer cells. Mol. Pharmacol. 53, 62–76. doi: 10.1124/mol.53.1.62
Vigushin D. M., Mirsaidi N., Brooke G., Sun C., Pace P., Inman L., et al. (2004). Gliotoxin is a dual inhibitor of farnesyltransferase and geranylgeranyltransferase I with antitumor activity against breast cancer in vivo. Med. Oncol. 21, 21–30. doi: 10.1385/MO:21:1
Vitaku E., Smith D. T., Njardarson J. T. (2014). Analysis of the structural diversity, substitution patterns, and frequency of nitrogen heterocycles among U.S. FDA approved pharmaceuticals. J. Med. Chem. 57, 10257–10274. doi: 10.1021/jm501100b
Wali A. F., Majid S., Rasool S., Shehada S. B., Abdulkareem S. K., Firdous A., et al. (2019). Natural products against cancer: Review on phytochemicals from marine sources in preventing cancer. Saudi Pharm. J. 27, 767–777. doi: 10.1016/j.jsps.2019.04.013
Wang F. Z., Huang Z., Shi X. F., Chen Y. C., Zhang W. M., Tian X. P., et al. (2012). Cytotoxic indole diketopiperazines from the deep sea-derived fungus Acrostalagmus luteoalbus SCSIO F457. Bioorg. Med. Chem. Lett. 22, 7265–7267. doi: 10.1016/j.bmcl.2012.08.115
Wang X., Liu Y., Qin H., Qi G., Chen X., Lyu Y., et al. (2023). RIP1 mediates manzamine-A-induced secretory autophagy in breast cancer. Mar. Drugs 21 (3), 151. doi: 10.3390/md21030151
Wang W., Rayburn E. R., Velu S. E., Nadkarni D. H., Murugesan S., Zhang R. (2009). In vitro and in vivo anticancer activity of novel synthetic makaluvamine analogues. Clin. Cancer Res. 15, 3511–3518. doi: 10.1158/1078-0432.CCR-08-2689
Wei X., Nieves K., Rodríguez A. D. (2010). Neopetrosiamine A, biologically active bis-piperidine alkaloid from the Caribbean sea sponge Neopetrosia proxima. Bioorg. Med. Chem. Lett. 20, 5905–5908. doi: 10.1016/j.bmcl.2010.07.084
Wilks S., Puhalla S., O'Shaughnessy J., Schwartzberg L., Berrak E., Song J., et al. (2014). Phase 2, multicenter, single-arm study of eribulin mesylate with trastuzumab as first-line therapy for locally recurrent or metastatic HER2-positive breast cancer. Clin. Breast Cancer 14, 405–412. doi: 10.1016/j.clbc.2014.04.004
Willems T., De Mol M. L., De Bruycker A., De Maeseneire S. L., Soetaert W. K. (2020). Alkaloids from marine fungi: promising antimicrobials. Antibiotics (Basel) 9 (6), 340. doi: 10.3390/antibiotics9060340
Wipf P., Reeves J. T., Day B. W. (2004). Chemistry and biology of curacin A. Curr. Pharm. Des. 10, 1417–1437. doi: 10.2174/1381612043384853
Woehlecke H., Osada H., Herrmann A., Lage H. (2003). Reversal of breast cancer resistance protein-mediated drug resistance by tryprostatin A. Int. J. Cancer 107, 721–728. doi: 10.1002/ijc.11444
Wu C. F., Lee M. G., El-Shazly M., Lai K. H., Ke S. C., Su C. W., et al. (2018). Isoaaptamine induces T-47D cells apoptosis and autophagy via oxidative stress. Mar. Drugs 16 (1), 18. doi: 10.3390/md16010018
Xiao X., Xu M., Yang C., Yao Y., Liang L. N., Ed Chung P., et al. (2018). Novel racemosin B derivatives as new therapeutic agents for aggressive breast cancer. Bioorg. Med. Chem. 26, 6096–6104. doi: 10.1016/j.bmc.2018.11.014
Yamazaki H., Wewengkang D. S., Kanno S., Ishikawa M., Rotinsulu H., Mangindaan R. E., et al. (2013). Papuamine and haliclonadiamine, obtained from an Indonesian sponge Haliclona sp., inhibited cell proliferation of human cancer cell lines. Nat. Prod Res. 27, 1012–1015. doi: 10.1080/14786419.2012.688050
Yang Z. X., Sun Y. H., He J. G., Cao H., Jiang G. Q. (2015). Increased activity of CHK enhances the radioresistance of MCF-7 breast cancer stem cells. Oncol. Lett. 10, 3443–3449. doi: 10.3892/ol.2015.3777
Yang B., Sun W., Wang J., Lin S., Li X. N., Zhu H., et al. (2018). A new breviane spiroditerpenoid from the marine-derived fungus penicillium sp. TJ403-1. Mar. Drugs 16 (4), 110. doi: 10.3390/md16040110
Yang C. L., Zhang B., Xue W. W., Li W., Xu Z. F., Shi J., et al. (2020). Discovery, biosynthesis, and heterologous production of loonamycin, a potent anticancer indolocarbazole alkaloid. Organic Lett. 22, 4665–4669. doi: 10.1021/acs.orglett.0c01456
Yao G., Kondratyuk T. P., Tan G. T., Pezzuto J. M., Chang L. C. (2009). Bioactive sulfated sesterterpene alkaloids and sesterterpene sulfates from the marine sponge Fasciospongia sp. J. Nat. Prod 72, 319–323. doi: 10.1021/np8005343
Yao B., Prinsep M. R., Nicholson B. K., Gordon D. P. (2003). The pterocellins, novel bioactive alkaloids from the marine bryozoan Pterocella vesiculosa. J. Nat. Prod 66, 1074–1077. doi: 10.1021/np030104y
Yin S., Boyle G. M., Carroll A. R., Kotiw M., Dearnaley J., Quinn R. J., et al. (2010a). Caelestines A-D, brominated quinolinecarboxylic acids from the Australian ascidian Aplidium caelestis. J. Nat. Prod 73, 1586–1589. doi: 10.1021/np100329w
Yin S., Cullinane C., Carroll A. R., Quinn R. J., Davis R. A. (2010b). Botryllamides K and L, new tyrosine derivatives from the Australian ascidian Aplidium altarium. Tetrahedron Lett. 51, 3403–3405. doi: 10.1016/j.tetlet.2010.04.104
Zaleta-Pinet D. A., Holland I. P., Muñoz-Ochoa M., Murillo-Alvarez J. I., Sakoff J. A., van Altena I. A., et al. (2014). Cytotoxic compounds from Laurencia pacifica. Org. Med. Chem. Lett. 4, 8. doi: 10.1186/s13588-014-0008-8
Zang L.-Y., Wei W., Wang T., Guo Y., Tan R.-X., Ge H.-M. (2012). Isochromophilones from an endophytic fungus Diaporthe sp. Natural Products Bioprospecting 2, 117–120. doi: 10.1007/s13659-012-0023-2
Zelek L., Yovine A., Brain E., Turpin F., Taamma A., Riofrio M., et al. (2006). A phase II study of Yondelis (trabectedin, ET-743) as a 24-h continuous intravenous infusion in pretreated advanced breast cancer. Br. J. Cancer 94, 1610–1614. doi: 10.1038/sj.bjc.6603142
Zhang Y., Li M., Zhang Q., Wang Z., Li X., Bao J., et al. (2021). Arthpyrone L, a new pyridone alkaloid from a deep-sea arthrinium sp., inhibits proliferation of MG63 osteosarcoma cells by inducing G0/G1 arrest and apoptosis. Chem. Biodivers 18, e2000639. doi: 10.1002/cbdv.202000639
Zhang W., Liu Z., Li S., Yang T., Zhang Q., Ma L., et al. (2012). Spiroindimicins A-D: new bisindole alkaloids from a deep-sea-derived actinomycete. Org. Lett. 14, 3364–3367. doi: 10.1021/ol301343n
Zhang Y. M., Liu B. L., Zheng X. H., Huang X. J., Li H. Y., Zhang Y., et al. (2017). Anandins A and B, two rare steroidal alkaloids from a marine streptomyces anandii H41-59. Mar. Drugs 15 (11), 355. doi: 10.3390/md15110355
Zhang H., Loveridge S. T., Tenney K., Crews P. (2016). A new 3-alkylpyridine alkaloid from the marine sponge Haliclona sp. and its cytotoxic activity. Nat. Prod Res. 30, 1262–1265. doi: 10.1080/14786419.2015.1054826
Zhang W., Ma L., Li S., Liu Z., Chen Y., Zhang H., et al. (2014). Indimicins A–E, bisindole alkaloids from the deep-sea-derived streptomyces sp. SCSIO 03032. J. Natural Products 77, 1887–1892. doi: 10.1021/np500362p
Zhidkov M. E., Smirnova P. A., Tryapkin O. A., Kantemirov A. V., Khudyakova Y. V., Malyarenko O. S., et al. (2019). Total syntheses and preliminary biological evaluation of brominated fascaplysin and reticulatine alkaloids and their analogues. Mar. Drugs 17 (9), 496. doi: 10.3390/md17090496
Zhou S., Huang G., Chen G. (2021). Synthesis and anti-tumor activity of marine alkaloids. Bioorg. Med. Chem. Lett. 41, 128009. doi: 10.1016/j.bmcl.2021.128009
Keywords: marine organisms, marine alkaloids, breast cancer, cancer therapy, antitumor mechanism
Citation: Yu J, Wang X, Du P and Shi H (2024) The therapeutic potential and application of marine alkaloids in treating breast cancer. Front. Mar. Sci. 11:1440928. doi: 10.3389/fmars.2024.1440928
Received: 30 May 2024; Accepted: 25 June 2024;
Published: 15 July 2024.
Edited by:
Daniel Loic Pouliquen, Inserm UMR 1307 (CRCI2NA), ICO., FranceReviewed by:
Patrizia Diana, University of Palermo, ItalyTan Suet May Amelia, University of Malaysia Terengganu, Malaysia
Copyright © 2024 Yu, Wang, Du and Shi. This is an open-access article distributed under the terms of the Creative Commons Attribution License (CC BY). The use, distribution or reproduction in other forums is permitted, provided the original author(s) and the copyright owner(s) are credited and that the original publication in this journal is cited, in accordance with accepted academic practice. No use, distribution or reproduction is permitted which does not comply with these terms.
*Correspondence: Hubing Shi, c2hpaGJAc2N1LmVkdS5jbg==
†These authors have contributed equally to this work