- 1Chemistry of Natural Products Postgraduate Program, Institute of Research in Natural Products, Federal University of Rio de Janeiro, Rio de Janeiro, RJ, Brazil
- 2Seallg. Solutions, Innovation, and Products, Aquário de Ideias, Incubator for Science and Technology-Based Companies, São Paulo State University (UNESP), Registro, SP, Brazil
- 3Engineering of Biomaterials and Bioprocess Postgraduate Program, São Paulo State University (UNESP), Institute of Pharmacy, Araraquara, SP, Brazil
- 4Department of Fisheries Resources and Aquaculture (DERPA), Institute of Advanced Marine Studies (IEAMar), São Paulo State University (UNESP), Registro, SP, Brazil
- 5National Institute of Science and Technology (INCT) in Nanotechnology for Sustainable Agriculture (INCTNanoAgro), Coordenação de Aperfeiçoamento de Pessoal de Nível Superior, Brasília, Brazil
- 6Laboratory of Natural Products of Marine Algae (ALGAMAR), Institute of Biology, Fluminense Federal University, Niterói, RJ, Brazil
- 7Aquaculture Center (Caunesp), São Paulo State University (UNESP), Jaboticabal, SP, Brazil
The cosmetics industry is experiencing continuous growth and the search for dermoactive metabolites continues to increase, positioning natural marine products as an essential element in this market. The genus Sargassum, a cosmopolitan brown alga, stands out for its diversified arsenal of metabolites with biological properties of great interest for the cosmetic sector. This study presents an updated review of the dermocosmetic properties of 17 Sargassum species published between 2020 and 2024, emphasizing increasing interest in its antioxidant and photoprotective properties. Furthermore, the review highlights the crucial role of green extraction methodologies, such as ultrassom-assisted extraction (EAU), enzyme-assisted extraction (EAE) and microwave-assisted extraction (MAE). It is also provided a conceptual outline of the spectrometric analytical techniques used for characterization of extracts and identification of active composts, such as polysaccharides (alginate and fucoidane), phenolic composts (phlorotannins and phenylpropanóids) and terpenoids (diterpenoids, saponins and norisoprenóids). In addition to addressing bioprospecting and the potential of the biorefinery in the cosmetics sector, this review analyzes challenges related to quality control of raw materials, seasonal fluctuations of seaweed and regulations governing the collection and use of seaweed. To provide a detailed update on the dermocosmetic potential of these algae, the review aims to support future research and encourage bioprospection of this biomass as a sustainable and promising source for the development of new bioproducts.
1 Introduction
The cosmetics industry is a crucial sector in the global economy, generating hundreds of billions of dollars and ranking among the fastest-growing industrial sectors (Santos et al., 2023; Mondello et al., 2024). With the increasing global demand for products featuring cosmetic properties and a growing trend towards eco-consumerism, there is a heightened focus on ingredients and additives derived from natural and sustainable origins sources, driving research in natural product chemistry (Morais et al., 2021). Marine organisms emerge as biofactories producing metabolites with diverse, highly valuable structures, often attributed to the challenging conditions of marine ecosystems such as salinity, pH, and ultraviolet radiation (UV) exposure (Tziveleka et al., 2021; Karthikeyan et al., 2022; Obando et al., 2024).
The discovery of new candidates for skincare products derived from marine organisms is a crucial factor for the growth of the cosmetics industry. This innovation can not only address specific needs, such as anti-aging, hydration and UV protection, but also open new possibilities in product development. Specifically, marine algae, reveals a promising chemical arsenal, whose versatility and uniqueness allows the identification of new biological agents with innovative potential (Matias et al., 2023; Santos J. M. et al., 2024). Furthermore, seaweed can promote sustainable alternatives to guarantee that the industry can respond to the growing demands of the market as it advances in the direction of more ecological practices of this sector.
Within marine biodiversity, macroalgae are considered prolific sources of natural compounds with several biotechnological applications, stimulating the development of studies on their dermocosmetic potential (Holdt and Kraan, 2011; Obando et al., 2022; Flores-Contreras et al., 2023). These photosynthetic organisms are classified into three groups based on pigment production: Chlorophyta, or green algae (containing chlorophyll a and chlorophyll b); Rhodophyta, or red algae (containing chlorophyll a and phycobiliproteins); and Ochrophyta, or brown algae (containing chlorophyll a, chlorophyll c, and fucoxanthin) (Obando et al., 2022). Algal biomass is being explored for producing bioproducts such as food, fertilizers, and biofuels, thereby possessing significant potential to enhance the global economy (Pérez-Larrán et al., 2019; Cavallo et al., 2021; Santos et al., 2023).
The genus of macroalgae Sargassum (Family Sargassaceae), belonging to the group of brown algae, currently has 978 recorded species with a cosmopolitan distribution, occupying temperate, subtropical and tropical habitats throughout the world (Tanniou et al., 2014; Stiger-Pouvreau et al., 2023; Guiry and Guiry, 2024). These algae are known to form the “Great Atlantic Sargasso Belt” (GASB) that extends from West Africa, the Gulf of Mexico to the coast of Brazil (Arencibia-Carballo et al., 2020; Santos et al., 2023). Studies have reported that this belt has been increasingly transporting millions of tons of biomass to the coastal regions of the Caribbean, West Africa, and Brazil (Sissini et al., 2017; Lee et al., 2023; Stiger-Pouvreau et al., 2023).
Sargassum inundation events (SIEs) can cause several environmental, economic, and social problems, since their decomposition leads to the release of sulfide and ammonia, harming human health, tourism and fishing activities, in addition to compromising the local ecological balance (Arencibia-Carballo et al., 2020; Santos et al., 2023). This phenomenon can be intensified by climate change due to the increase in ocean temperatures, causing negative impacts in these regions. Therefore, it is necessary to think about strategies for the best use of this biomass, to reduce the impacts in the affected regions (Arencibia-Carballo et al., 2020). Furthermore, in the Ocean Decade, the importance of these algae goes beyond sustainability, intertwining with the Sustainable Development Goals (SDGs) established by the United Nations (UN), mainly SDG 14 (Life below water), which reinforces the conservation and sustainable use of marine ecosystems and the use of marine resources for sustainable development (Troell et al., 2023).
Sargassum species are renowned for their production of metabolites such as terpenoids, pigments, phenolic compounds, especially phlorotannins, sterols, and sulfated polysaccharides (fucoidans) (Santos et al., 2023). These compounds have demonstrated various biological activities of interest to humans, such as anti-inflammatory, antioxidant, neuroprotective, immunomodulatory, anticancer, hypolipidemic, and antimicrobial effects (Rushdi et al., 2020; Flores-Contreras et al., 2023; Santos T. C. et al., 2024). It is worth noting that a recent review study focused on the anti-aging properties of Sargassum, highlighting antioxidant, photoprotective, anti-inflammatory, anti-melanogenesis, and skin barrier repair activities reported in articles published since 2011 (Lee M. K. et al., 2022). Besides that, several species, including S. horneri, S. fusiforme, S. muticum, S. pallidum, S. siliquastrum, S. thunbergii, and S. polycystum, have been identified as potential sources of metabolites with cosmetic properties (Flores-Contreras et al., 2023).
Advances in research into natural Sargassum dermoactive products have been significant, and the synthesis of chemical and biological information is essential to assist researchers and highlight the market potential of these species (Lee M. K. et al., 2022; Catarino et al., 2023). While several reviews on cosmeceutical potential of Sargassum are focused on the collection of biological data, such as models, properties, and active concentrations. There is a noticeable gap in literature regarding reviews of methodological information related to extraction methodologies, the use of unconventional methods, pretreatment of biomass, and the chemical analysis conducted, all of which are fundamental for the bioprospecting of these products. Therefore, this review aims to fill this gap by providing a comprehensive and updated synthesis of the latest methodologies for extracting bioactive compounds from Sargassum species, offering new insights and practical guidance for the cosmetic industry’s bioprospecting efforts. Finally, the discussion will focus on the emerging opportunities and inherent challenges associated with industrial-scale bioprospecting of Sargassum as a source of dermocosmetic agents.
2 Methodology
A search was conducted using the Scopus and Web of Science databases, chosen for their high quality and quantity of publications. The research applied a temporal filter covering the January 2020 and July 2024 and utilized the descriptors “Sargassum AND cosmetic” and “Sargassum AND dermocosmetic”. The review focused on selecting original English-language articles that explored the cosmeceutical potential of macroalgae, specifically Sargassum species, while excluding studies that did not investigate dermocosmetic applications. Data on extraction, identification, and purification methods of natural products from Sargassum were organized into tables and graphs, alongside information on dermocosmetic potential and bioassays, providing clear and accessible information to researchers in biotechnology and natural product chemistry, as well as to startups and companies that can utilize technical and scientific information for their product development stages.
3 Advancing dermocosmetic applications of Sargassum: chemical approach, insights, and challenges
In this study, 30 articles published in the last years that addressed the cosmeoceutical potential of Sargassum were reviewed, which explored 17 species of Sargassum, the most explored species being S. horneri (30.0%), S. fusiforme (6.67%), S. fussum (6.67%) and S. muticum (6.67%). Regarding the source of biomass, most studies used algae obtained from natural banks (76.67%). However, algae from commercial sources (13.33%) and beach-cast biomass (6.67%) were also recorded. Despite the negative impacts caused by the phenomenon of uplift of the Sargassum belt in the Atlantic Ocean in countries on the American continent, the majority of registered publications come from Asian countries, such as South Korea (46.67%), China (10.00%) and Philippines (6.67%).
Revealing a significant gap in studies focused on exploring species from this genus for the cosmetic sector in Central America and Brazil. Data on dermocosmetic potential are presented in section 3.1, where the main effects observed, and bioassays applied are discussed. In section 3.2, the methodologies for extraction, purification, and identification of extracts, fractions and natural products with dermocosmetic potential are detailed. Finally, the section 3.3 discusses the perspectives on industrial bioprospecting of dermocosmetics derived from Sargassum, highlighting its social and environmental impacts.
3.1 Dermocosmetic properties of Sargassum genus macroalgae
The skin is the largest organ in the human body and the first line of defense against external physical, chemical, and biological aggressions (Cruz et al., 2023). Its aging occurs naturally over time, leading to the loss of fibrous tissue and a decline in cell regeneration (Hay et al., 2015). This process is accelerated by environmental factors such as exposure to ultraviolet (UV) radiation, reactive oxygen species (ROS), and pollution (Maranduca et al., 2020; Russell-Goldman and Murphy, 2020; Liang et al., 2023). These physiological and external factors result in a loss of elasticity, hyperpigmentation, appearance of spots, and tissue degeneration, among other effects (Cruz et al., 2023). Furthermore, the skin is vulnerable to various physiological changes, including inflammatory diseases, atopic dermatitis, acne, psoriasis and more serious pathologies such as skin cancer (Farage et al., 2013; Wong and Chew, 2021). Consequently, there is a growing demand in the cosmetic industry for compounds with dermocosmetic properties that improve photoprotection, hydration and delay skin aging (Jesumani et al., 2020).
In this study, was classified several effects, including photoprotection (20.0%), antioxidant activity (22.0%), skin lightening (16.0%), anti-inflammatory (14.0%), protection and repair of the skin barrier (10.0%), moisturizing (6.0%), anti-aging (6.0%), antimicrobial (4.0%), and preservative properties (2.0%), observed in various Sargassum species (Fernando et al., 2020a, Fernando et al., 2020b, Fernando et al., 2020c, Fernando et al., 2021a; Jesumani et al., 2020; Kim et al., 2020; Arguelles, 2021; Gam et al., 2021; Jang et al., 2021; Takashi, 2021; Lee et al., 2022; Kirindage et al., 2024) (Table 1). The majority of these studies utilized in vitro assays (82.4%), while in vivo bioassays were conducted in mouse and zebrafish models (5.9%), clinical trials (8.0%), and in silico experiments (2.9%). A schematic representation of the studied dermocosmetic properties and their respective bioassays is presented in Figure 1.
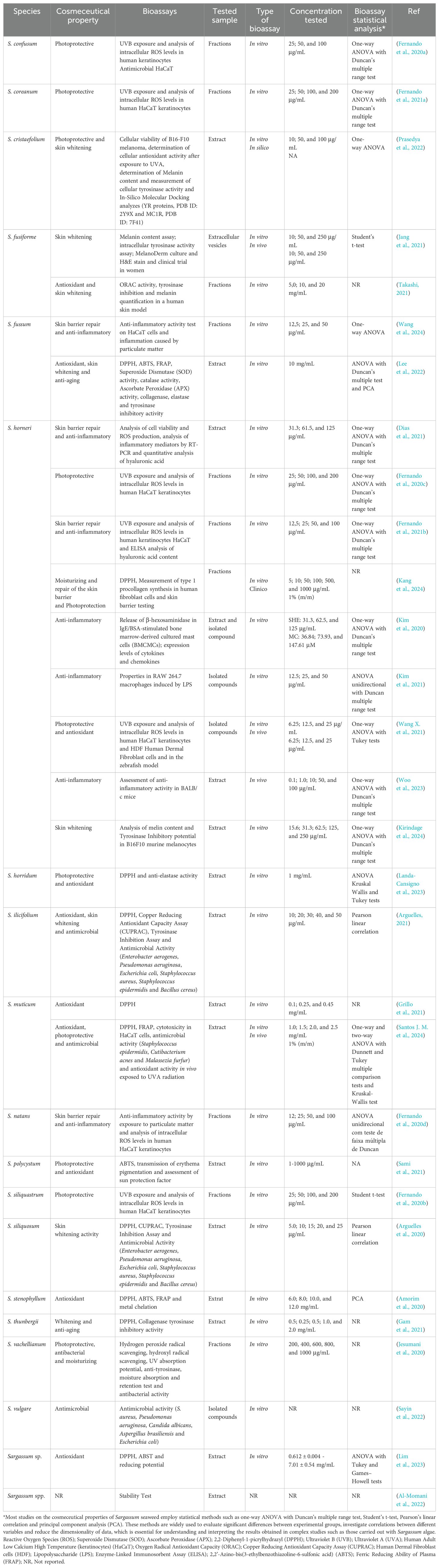
Table 1. Cosmeceutical properties and associated bioassays for Photoprotective, Skin Whitening, Antioxidant, Anti-inflammatory, and Skin Barrier Repair Activities in Sargassum.
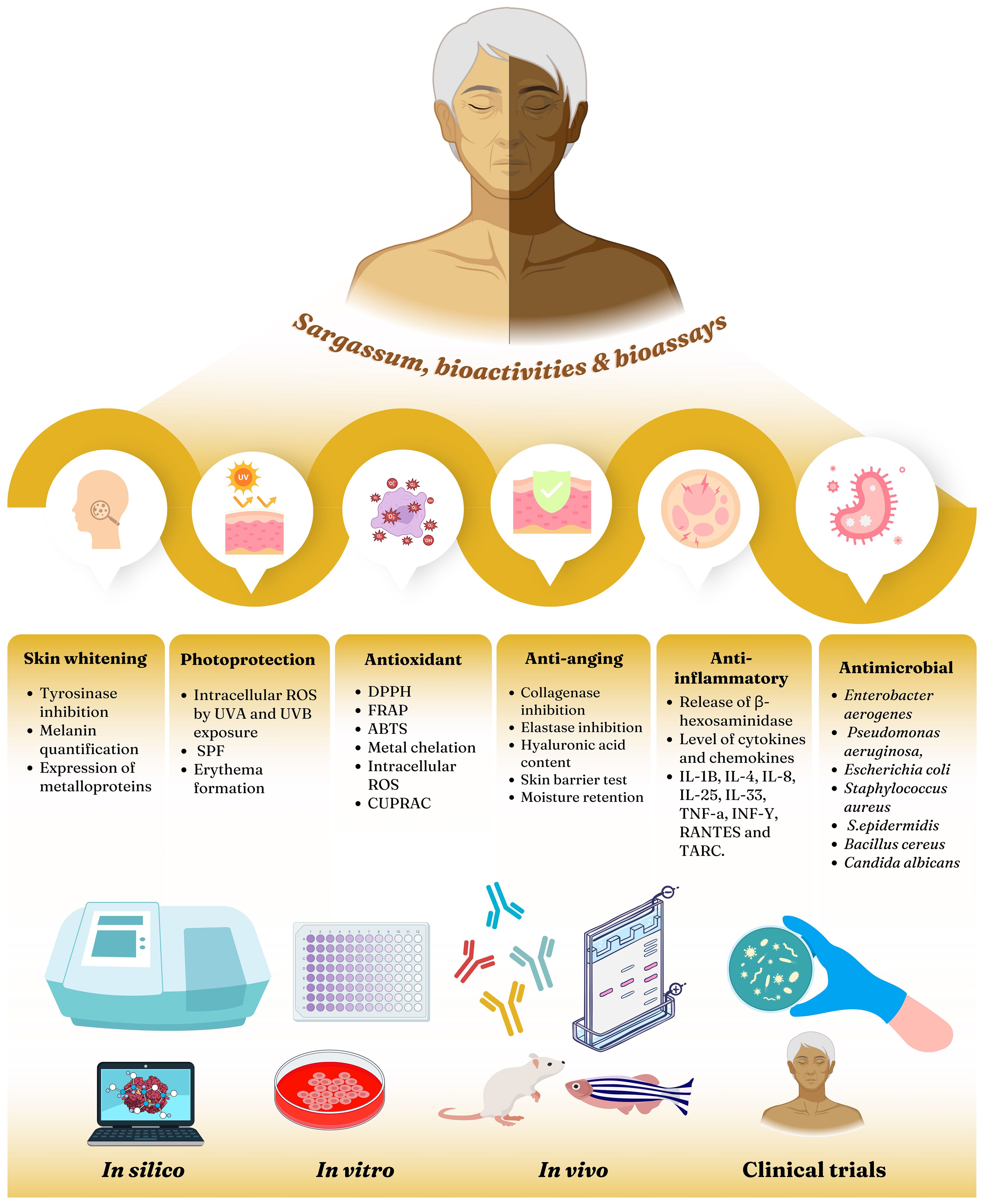
Figure 1. Schematic representation of the dermocosmetic properties of Sargassum genus and their main bioassays. Figures were created using Biorender® and Canva®.
3.1.1 Photoprotective and antioxidant effect
Photoprotection and antioxidant activities are closely linked to the prevention of photoaging, helping to combat the harmful effects of UV radiation on the skin (Sami et al., 2021). Exposure to UV radiation (UVA and UVB) can lead to the development of degenerative skin diseases, induce inflammatory responses, cause wrinkles formation, skin thickening, sunburn and even melanomas due to increased formation of ROS (Arguelles, 2021; Fernando et al., 2021a). These free radicals intensify collagenase activity, suppress collagen synthesis, leading to damage to the extracellular matrix, and consequently resulting in loss of skin elasticity (Kang et al., 2024). Strategies to mitigate these effects through studying the antioxidant properties and photoprotective agents of Sargassum are being explored to combat the effects of photoaging (Fernando et al., 2020a, Fernando et al., 2020b, Fernando et al., 2020c, Fernando et al., 2021a; Jesumani et al., 2020; Sami et al., 2021; Wang L. et al., 2021; Santos J. M. et al., 2024).
The ethanolic extract of S. cristaefolium led to a decrease in the production of intracellular free radicals in B16-F10 melanoma cells exposed to UVA radiation in a concentration-dependent manner, suggesting its potential as a UV protection agent for the skin (Prasedya et al., 2022). The ethanolic extract of S. polycystum has shown the capacity to absorb UV rays, thereby reducing exposure that could cause erythema and pigmentation in the skin. The antioxidant action, evaluated through the assay with 2,2′-azino-bis(3-ethylbenzothiazoline-6-sulfonic acid) (ABTS) reagent, indicated that the extract presented an IC50 of 79.29 μg/mL. Regarding the sun protection factor (SPF), the species provided a minimum protection of 2.41 ± 0.22% (Sami et al., 2021).
Additionally, extracts prepared with eutectic solvents from S. muticum exhibited antioxidant effects in both the 1,1-diphenyl-2-picrylhydrazyl (DPPH) and ferric reducing antioxidant power (FRAP) assays, as well as in the HaCaT model (Santos J. M. et al., 2024). In the DPPH assay, extracts prepared with L-lactic acid (7:1) showed a significant reduction in radicals, exceeding 60% efficiency. This activity was attributed to the presence of phenolic compounds (1314.3 - 129.8 mg gallic acid equivalent (GAE)/L), including phlorotannins (Prasedya et al., 2022). In a clinical trial involving topical application of a cosmetic formulation containing 1% (m/m) of this extract, greater efficacy was observed in preventing β-carotene discoloration after UVA exposure compared to negative controls. It’s worth noting that carotene has chromophores that lose their capacity when oxidized, and this discoloration can be measured through colorimetry (Wang et al., 2022).
Fractions rich in polysaccharides and polyphenols obtained from S. vachellianum have been investigated for their free radical scavenging action and UV absorption potential (Jesumani et al., 2020). The hydroxyl radical scavenging assay (-OH) demonstrated that the polyphenol-rich fraction showed slightly more effective activity than the polysaccharide fraction, with IC50 values of 1.31 and 0.98 mg/mL, respectively. This trend was also observed in the hydrogen peroxide elimination assay (IC50 1.12 and 0.8 mg/mL). The fraction rich in phenolic compounds effectively absorbed UVB and UVA rays, indicating photoprotective potential for dermocosmetic application.
The literature describes the antioxidant and photoprotective mechanisms of phenolic compounds from brown algae (Figure 2). UVB radiation typically causes oxidative stress, including ROS production, lipid peroxidation, and DNA damage. However, phenolic compounds can boost antioxidant enzymes like catalase (CAT), heme-oxygenase 1 (HO-1) and superoxide dismutase (SOD) under photo-oxidative stress. These phenolic compounds stabilize free radicals by donating electrons, forming intermediate phenoxyl radicals (PhO•), which are then stabilized through resonance or hydrogen bonding, or they dimerize to form new C-O or C-C linked compounds (Phang et al., 2023).
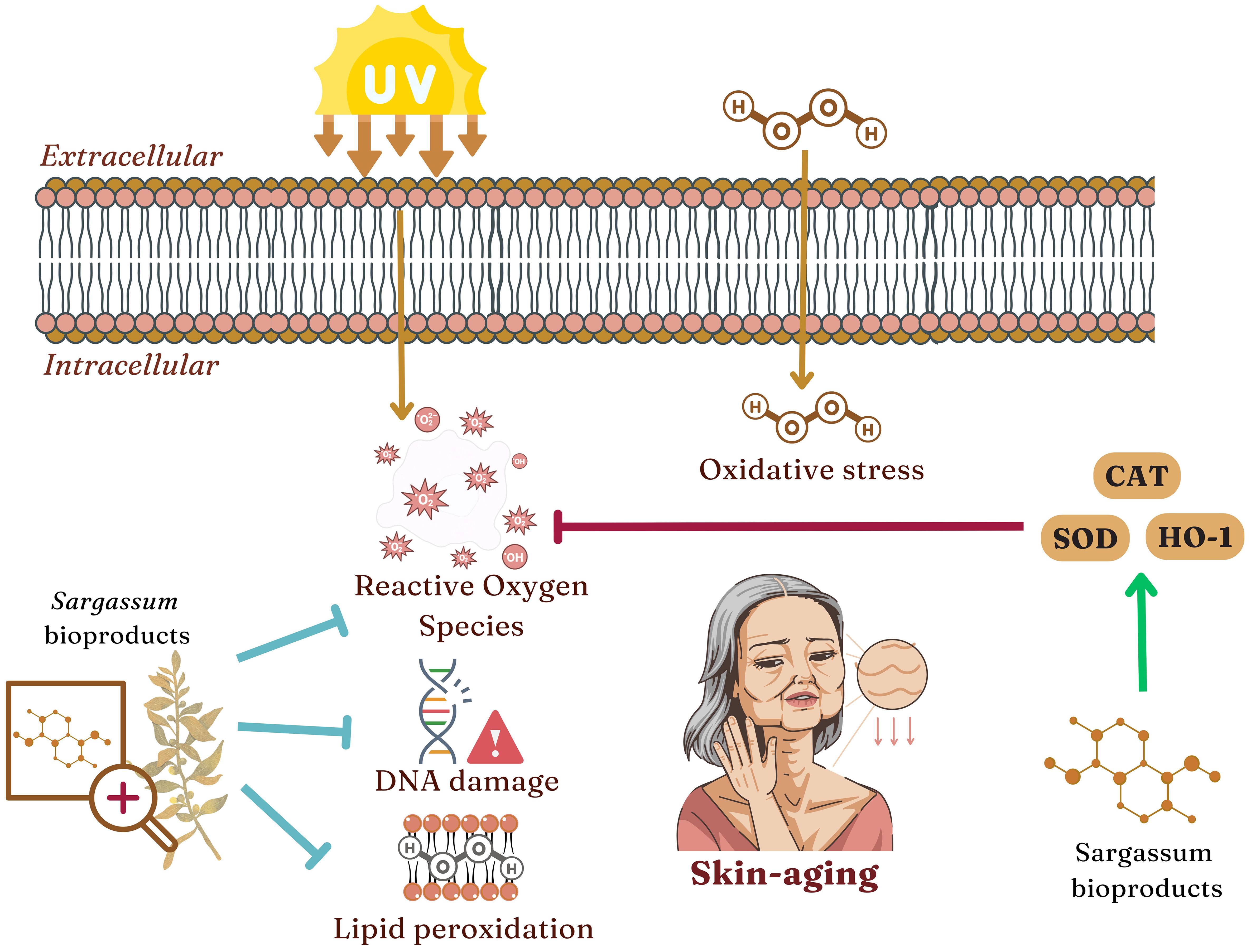
Figure 2. Proposed mechanisms for antioxidant and anti-aging activities of compounds from Sargassum species. Ultraviolet (UV) radiation generates reactive oxygen species (ROS) upon interaction with water in irradiated skin tissues, leading to lipid peroxidation and protein and DNA modification. On the one hand, pretreatment of Sargassum extracts activates the expression of antioxidant enzymes such as catalase (CAT), heme-oxygenase 1 (HO-1) and superoxide dismutase (SOD) (green arrow), which protect cells by neutralizing free radicals and preventing oxidative damage (pink arrow). Meanwhile, compounds from Sargassum, such as phlorotannins, can directly stabilize these effects through electron delocalization via resonance, thereby mitigating oxidative damage (blue arrows).
The photoprotective and antioxidant effect of polysaccharides obtained from S. horneri, S. confusum, S. coreanum and S. siliquastrum, on human HaCaT keratinocytes were evaluated (Fernando et al., 2020a, Fernando et al., 2020b, Fernando et al., 2020c, Fernando et al., 2021a). The low molecular weight fucoidan (1) (~20 kDa) from S. confusum led to a reduction in intracellular ROS levels (60.83% at a concentration of 100 μg/mL) and suppressed the formation of apoptotic bodies induced by UVB (Fernando et al., 2020a). The polysaccharide with a molecular weight in the range of ~50 kDa from the macroalgae S. coreanum also reduced ROS levels, showing greater recovery at a concentration of 100 μg/mL (Fernando et al., 2021a). For the algae S. horneri and S. siliquastrum the best photoprotective effects were obtained for concentrations of 25-100 μg/mL (Fernando et al., 2020b, Fernando et al., 2020c).
The antioxidant and photoprotective effects of S. horneri were also investigated by Kang et al. (2023). Fucoidan extract (SHFE) exhibited antioxidant properties using DPPH assay (IC50, 4.89 μg/mL). Furthermore, treatment of UVB-induced fibroblasts with the polysaccharide significantly increased procollagen synthesis compared to the positive control and inhibited the expression of metalloproteinase-type collagenases (MMP-1 and MMP-3). A clinical study of SHFE lotion showed that it improved skin barrier effects on forearms and decreased transepidermal water loss (TEWL) values after three weeks of use, compared to a placebo (Kang et al., 2024). Moreover, the compound (-)-loliode (3), isolated from hydromethanolic extract of S. horneri, prevented oxidative damage to HaCaT models and Human Dermal Fibroblast (HDF) cells, with the greatest effect observed at a concentration of 25 μg/mL (Wang L. et al., 2021). This compound also reduced nitric oxide (NO) levels and suppressed lipid peroxidation in in vivo zebrafish model.
3.1.2 Skin whitening effects and anti-blemish action on the skin
The skin lightening effect refers to the process of reducing or eliminating hyperpigmentation, dark spots, freckles, melasma, or other skin discolorations using cosmetic products (Guerrero, 2012; Zhao et al., 2022). Several natural products have been incorporated into cosmetics to inhibit the production of melanin, pigment responsible for skin color and UV protection. Pointing out that this inhibition targets tyrosinase a crucial protein in the synthesis of melanin through the hydroxylation of L-tyrosine (Mohiuddin, 2019; Zhao et al., 2022; Kirindage et al., 2024). The evaluation of the skin whitening properties of extracts and fractions obtained from the genus Sargassum has been conducted using proteins such as tyrosine to inhibit the formation of melanin.
Prasedya et al. (2022) showed the melanin inhibition activity of S. cristaefolium ethanolic extract, suggesting its potential for skin whitening. The study revealed that the extract can reduce melanin production and tyrosinase activity, as well as decrease ROS levels in cells irradiated with UVA. The diterpene compound kaurenoic acid (9) present in the extract is pointed out as a possible contributor to melanin inhibition activity. The potential of extracts of S. fusiforme as skin lightening agents by tyrosinase inhibition assays was also studied (Lee et al., 2022). The main results indicated that the fresh aqueous extract showed the highest inhibition of tyrosinase (28.2%), followed by methanol (23.3%) and ethanol (14.9%) extracts. For S. fusiforme seaweed treated with hot water before extraction, the methanol extract showed the highest inhibitory activity (30.5%), followed by ethanol (25.1%) and water (22.9%) extracts. Notably, the pre-treated methanol and ethanol extracts of S. fusiforme exhibited inhibitory activities of tyrosinase 1.3 times and 1.7 times higher, respectively, compared to fresh extracts.
Jang et al. (2021) developed a method to isolate extracellular vesicles from S. fusiforme. These vesicles showed potential inhibiting melanin production in human melanoma cells (MNT-1), at a concentration of 250 mg/mL downregulated the expression of tyrosinase-1 (TRP-1) and microphthalmia-associated transcription factor (MITF). In the artificial skin model, Sargassum was able to reduce pigmented cells at a concentration of 50 μg/mL. In the artificial skin model, Sargassum was able to reduce pigmented cells at a concentration of 50 μg/mL.
The methanolic fraction obtained from the aqueous residue of the edible algae of S. fusiforme was tested for its anti-tyrosinase action and melanin reduction in a three-dimensional model of human skin (Takashi, 2021). The results suggest that the fraction prevented melanin pigmentation at all concentrations tested, with maximum protection of 67 ± 4% at a concentration of 20 mg/mL. Furthermore, the fraction presented an IC50 of 3.1 μg/mL of tyrosinase inhibition, demonstrating higher activity than the aqueous extract (IC50 51 μg/mL).
Kirindage et al. (2024) investigated the effect of S. horneri ethanolic extract on melanogenesis in B16F10 murine melanocytes stimulated with α-melanocyte-stimulating hormone (α-MSH). The results demonstrated that the extract significantly reduced melanin content and cellular tyrosinase activity in these melanocytes. Additionally, the sample treatment resulted in a significant decrease in the expression levels of MITF, tyrosinase, TRP-related protein-1 and tyrosinase-related protein-2 (TRP-2) proteins in B16F10 melanocytes stimulated with α-MSH. The hydromethanolic extract of S. ilicifolium also inhibited tyrosinase production, with an inhibition of 89.78% at a concentration of 125 μg/mL (Arguelles, 2021).
Efforts have been dedicated to establishing optimal extraction conditions aimed at producing bioactive compounds with antioxidant activity, as well as skin lightening and anti-wrinkle effects, using green extraction methodologies in Sargassum thunbergii (Gam et al., 2021). The S. thunbergii extract demonstrated significant inhibitory effects on the mRNA expression of proteins related to TRP-1, MMP-1 and MMP-9, the main genes involved in melanin synthesis and collagen hydrolysis. The tyrosinase inhibitory activity of S. thunbergii extracts was evaluated under 17 ultrasound-assisted extraction (EAU) conditions. The maximum activity value was 92.6%, occurring in 12.0 minutes, 79.6°C and 50.0% ethanol concentration, while the minimum, 55.3%, was observed in 12.0 minutes, 51.0°C and 0.0% ethanol concentration.
3.1.3 Repair of the skin barrier and anti-inflammatory action
The skin is susceptible to pathophysiological changes induced by intrinsic and extrinsic factors that can compromise cell regeneration and trigger pro-inflammatory processes (Maranduca et al., 2020; Russell-Goldman and Murphy, 2020). Exposure to irritating agents such as UV radiation, free radicals and pollution, as well as endogenous factors such as aging, hormonal imbalances and stress, can decrease epidermal function, inhibiting cell regeneration (Delavary et al., 2011). Bioproducts that promote the repair of the skin barrier and that have anti-inflammatory effects can aid in healing and managing dermopathies such as atopic dermatitis. This is of great interest to the cosmetic industry, sparking interest in research in this area (Dias et al., 2021; Fernando et al., 2021b; Ho et al., 2023; Woo et al., 2023; Wang et al., 2024).
Wang et al. (2024a) extracted a fucoidan from S. fusiforme and evaluated its effects on skin damage in HaCaT cells and HDF cells stimulated with particulate matter. The polysaccharide led to a decrease in pro-inflammatory cytokines (Tumor Necrosis Factor Alpha - TNF-α, Interleukin-1 - IL-1 and Interleukin-6 - IL-6) in addition to reducing the production of intracellular free radicals, with the most active concentration being 50 μg/mL (Wang et al., 2024). It is noteworthy that excessive secretion of IL-6 can stimulate the expression of MMPs, leading to collagen degradation. Similar effects were observed for the ethanolic extract of S. horneri (Dias et al., 2021). The extract upregulated anti-inflammatory cytokines (Interleukin-4 - IL-4) and suppressed pro-inflammatory immune regulators (IL-1β, IL-6, Interleukin-8 - IL-8, TNF-α, Thymic Stromal Lymphopoietin - TSLP, Thymus and activation-regulated chemokine - TARC and Regulated upon Activation, Normal T Cell Expressed - RANTES), in addition to suppressing inflammatory cytokines that act directly on the skin such as Interleukin-25 (IL-25) and Interleukin-33 (IL-33).
The skin barrier repair effect of the fucoidan-rich extract of S. horneri was evaluated in a clinical study in healthy women (Kang et al., 2024). After three weeks of using a formulation containing 1% (m/m) of macroalgae extract, there was a reduction in transepidermal water loss compared to placebo. Other studies focused on evaluating the UVB protective effects of fucoidans derived from S. confusum on human keratinocytes (Fernando et al., 2020a). The SCFC4 fraction, with a lower molecular weight, demonstrated the best protective effects, reducing intracellular ROS levels and improving cell viability after exposure to UVB (97.24% at a concentration of 100 μg/mL). This fraction also reduced the formation of apoptotic bodies and DNA damage induced by UVB, while repressing upstream mediators of UVB-induced inflammatory responses, which could impair the hydration of the stratum corneum.
On the other hand, Fernando et al. (2021b), evaluated the effectiveness of fucoidan fractions from an enzymatic extract of S. horneri in ameliorating inflammatory responses induced by fine dust in HaCaT keratinocytes and in recovering skin barrier dysfunction. Treatment with polysaccharide fractions dose-dependently reduced intracellular ROS levels, while increasing cell viability. The fucoidan-rich fraction (SHC4-6) reduced inflammatory cytokines, including TNF-α, IL-1β, Interleukin-5 (IL-5), IL-6, IL-8, Interleukin-13 (IL-13), interferon-γ (INF-γ). The findings suggest that the SHC4-6 fraction may be a promising candidate for the development of cosmetic products aimed at combating particulate matter-induced skin inflammation.
Additionally, the ethanolic extract of S. horneri and isolated compound mojabancromannol (2) were evaluated for their anti-inflammatory and anti-allergic properties through the β-hexosaminidase release assay and levels of cytokines and chemokines (Kim et al., 2020). The isolated compound showed a higher inhibitory effect on β-hexosaminidase release than the extract, with IC50 38.54 ± 0.34 μM and 210.12 ± 0.11 mg/mL, respectively. Furthermore, majochromanol suppressed the expression of cytokines related to allergic processes IL-4, IL-6, IL-13, IFN-γ. Norisoprenoids isolated from this same species ((-)-loliolide (3), 3-hydroxy-5,6-epoxy-β-ionone (4) and apo-9′-fucoxanthinone (5)) showed potential anti-inflammatory properties in RAW 264.7 macrophages induced by lipopolysaccharide (LPS), in a dose-dependent response, with the most active concentration of 50 μg/mL for both metabolites (Kim et al., 2021).
Aqueous extracts of S. horneri called SHHWE were tested for their action on Atopic Dermatitis (AD) induced by the application of 2,4-dinitrochlorobenzene in BALB/c mice (Woo et al., 2023). The extract showed a significant reduction in splenocyte proliferation in BALB/c mice, decreasing by approximately 83%. Furthermore, there was a decrease in IL-4 and IL-5 levels by about 35% and 42%, respectively, and concentrations of 50 and 100 μg/mL of SHHWE increased the survival rate of splenocytes from normal mice by up to 123.5% and 129%, respectively, compared to the control. These results suggest that SHHWE may be effective in the treatment of AD, by regulating the inflammatory response and cytokine levels.
3.1.4 Antimicrobial effect
Jesumani et al. (2020) investigated several activities for two extracts from the seaweed S. vachellianum: one rich in fucoidan polysaccharides (SPS) and another rich in polyphenols (SPP), including moisture preservation activities and antibacterial activity. Moisture absorption and retention efficiency test results demonstrated that SPS had a moisture absorption rate of 50.5% after 72 hours at 80% relative humidity, while SPP recorded an absorption rate of 40%. After 72 hours. Regarding antibacterial activity, only SPP showed maximum inhibition against Staphylococcus aureus and Escherichia coli with 12.3 and 7.2 mm inhibition zone, respectively. These results are useful for the formulation of cosmetic products with antibacterial potential and indicate that the mixture of SPP and SPS may be promising for protecting the skin.
The alginate-type polysaccharide extracted from the species S. vulgare was tested for its preservative and antimicrobial action against the microorganisms Pseudomonas aeruginosa, S. aureus, Candida albicans, Escherichia coli and Aspergillus brasiliensis (Sayin et al., 2022). The limit value for the number of microorganisms obtained for the extracted alginate was less than 10 CFU/g, ten times below the limit established for cosmetic products. The results showed that the compound from S. vulgare is more effective against microorganisms in less time than a commercial herbal preservative (herbal 705), achieving the desired reduction in microorganisms by the 7th day, suggesting its applicability in cosmetics.
Furthermore, the antimicrobial potential of the hydromethanolic extract of S. ilicifolium against skin pathogenic bacteria, such as methicillin-resistant S. aureus, S. aureus and S.epidermidis, was investigated to explore its application as a cosmetic additive (Arguelles, 2021). The minimum inhibitory concentration results were 125 μg/mL, 125 μg/mL, and 250 μg/mL, respectively. Santos et al. (2024), obtained extracts enriched with antioxidants from seaweeds found on the Portuguese coast, including S. muticum, using natural eutectic solvents and evaluated the antimicrobial activity of the extracts on three microorganisms from the skin microbiota, the bacteria S. epidermidis and Cutibacterium acnes and the fungus Malassezia furfur (Santos J. M. et al., 2024). Some extracts led to significant inhibitions of up to 66% in the growth of S. epidermidis. However, no samples had an effect on the fungus M. furfur, a fungus naturally found on the skin, showing the ability of these extracts to maintain the balance of the skin’s microbiota.
3.2 Chemical methods and processes for obtaining compounds from Sargassum
Data regarding drying procedures, pre-treatment, type of extraction, and metabolite identification methods from the 30 articles reviewed were collected to highlight the most current approaches for extracting dermoactive bioproducts (Table 2). The Figure 3 illustrates the main approaches used to obtain extracts, fractions, and isolated compounds with dermocosmetic properties from Sargassum.
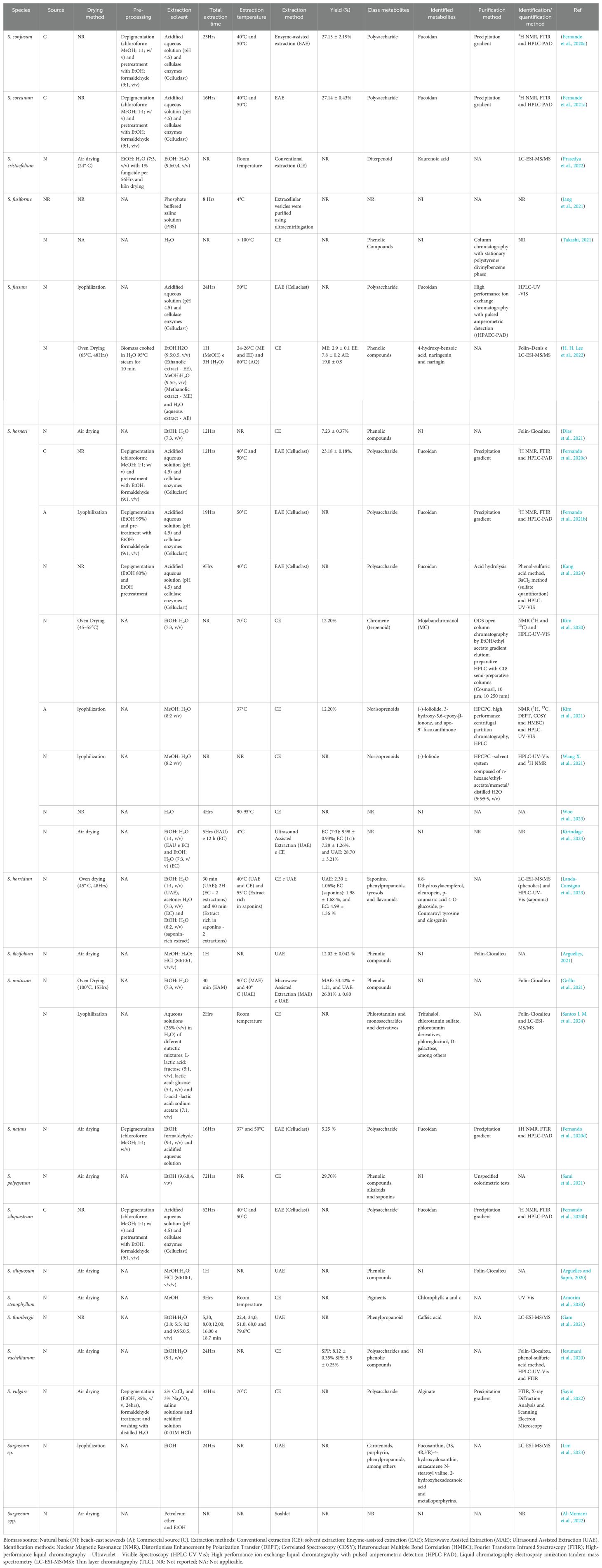
Table 2. Methods for extraction, identification and purification of natural products with dermocosmetic properties from the Sargassum genus.
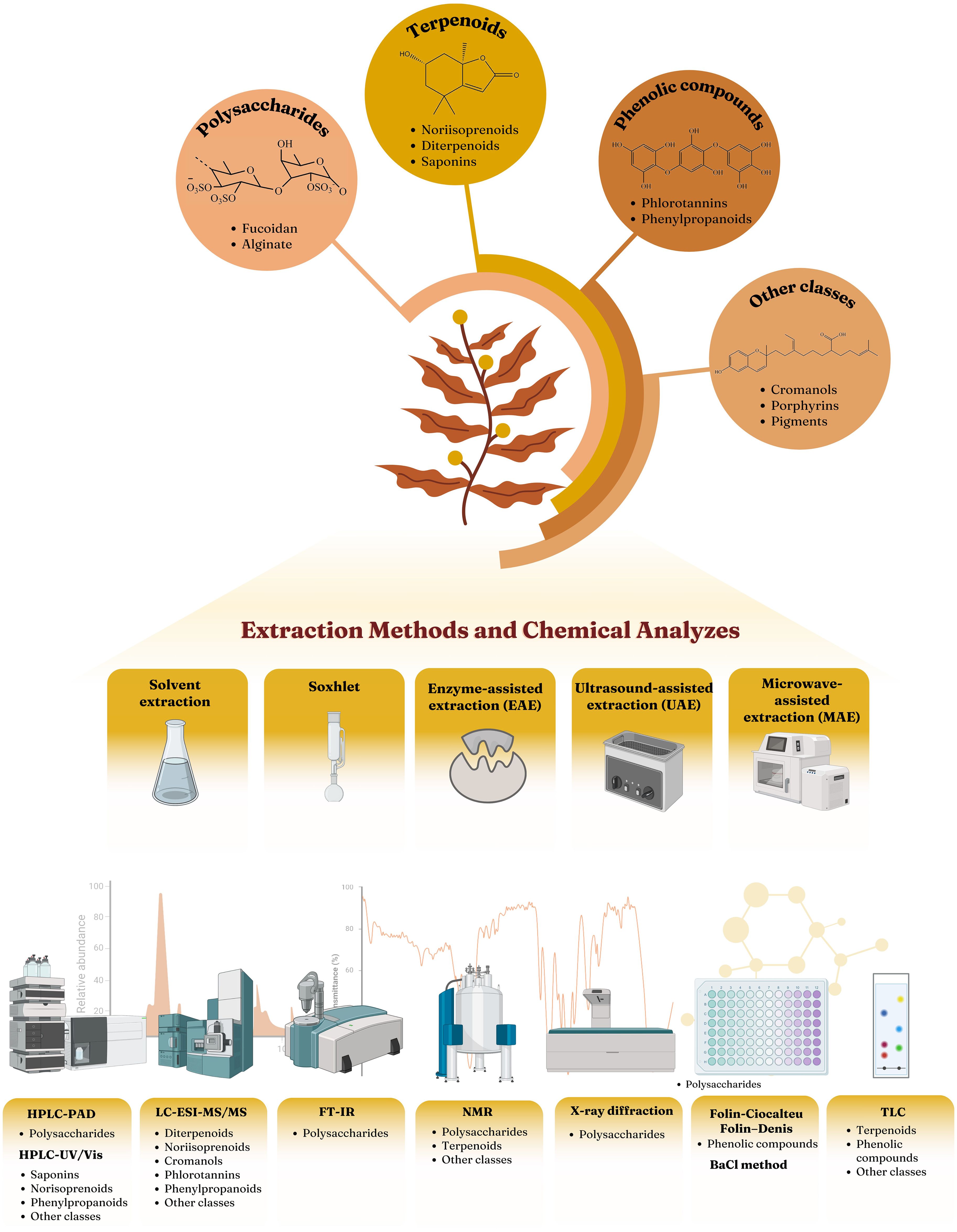
Figure 3. Green and conventional methods for extracting Sargassum biocompounds, chemical analysis techniques and representative classes of metabolites with dermocosmetic properties. Figures were created using Biorender® and Canva®.
3.2.1 Drying methods
In this review, the most commonly identified drying method was air drying at room temperature or with airflow (36.7%) (Jesumani et al., 2020; Arguelles, 2021; Dias et al., 2021; Sami et al., 2021; Al-Momani et al., 2022; Prasedya et al., 2022; Sayin et al., 2022; Kirindage et al., 2024), followed by freeze-drying (20.0%) (Kim et al., 2021; Wang X. et al., 2021; Lim et al., 2023; Santos J. M. et al., 2024; Wang et al., 2024) and oven drying (13.3%) (Lee et al., 2022; Kim et al., 2020; Grillo et al., 2021; Landa-Cansigno et al., 2023). Additionally, several studies (30%) did not specify the drying method used for biomass treatment. Some studies also carried out pre-treatment procedures for algal biomass to remove pigments, aiming for a targeted extraction of polysaccharides (Fernando et al., 2020a, Fernando et al., 2020b, Fernando et al., 2020c, Fernando et al., 2021a). Given their high moisture content (90–95%), seaweeds are perishable (Djaeni and Sari, 2015). Drying them not only extends their shelf life but also enhances supply chain efficiency, enabling distribution across various regions while simplifying storage and transportation - an essential process in bioprospecting and biorefinery (Santhoshkumar et al., 2023).
It’s worth noting that air drying, typically conducted in laboratory settings, offers a high cost-benefit ratio due to the absence of energy consumption; however, the extended drying time can promote microorganism growth, potentially compromising biomass quality (Mingu et al., 2024). In contrast, the literature indicates that freeze-drying retains the highest total antioxidant activity among all seaweed drying methods, as the low-temperature and oxygen-free environment significantly reduces the degradation of antioxidant compounds (Zhu et al., 2022; Santhoshkumar et al., 2023). While oven drying accelerates the process, it has been reported that exposure to temperatures above 50°C for extended periods can lead to substantial losses of pigments and antioxidant compounds in algae (Uribe et al., 2018). Overall, while air drying is prevalent, freeze-drying emerges as superior for maintaining bioactive properties, underscoring the importance of method selection in biomass processing (Amorim et al., 2020; Santhoshkumar et al., 2023).
3.2.2 Conventional extraction methods
Regarding extraction methods, most articles performed solvent extraction (conventional extraction) (45.2%) (Amorim et al., 2020; Dias et al., 2021; Lee et al., 2022; Landa-Cansigno et al., 2023; Kirindage et al., 2024). Solvent extraction is the most used method for obtaining seaweed compounds, using different solvents and mixtures such as water, ethanol, methanol, ethyl acetate, hexane, among others (Flores-Contreras et al., 2023). The choice of solvent is important for targeting specific types of compounds. In this review, a wide variety of solvent systems used to prepare extracts were observed, with a predominance of polar systems such as ethanol:water (96:4; 95:5; 80:20; 70:30 and 50:50, v/v) and methanol water: (80:20, v/v) or 100% ethanol and water.
The extraction of S. horneri algae, known for its anti-inflammatory and moisturizing properties, was carried out with 70% ethanol for 12 hours (Dias et al., 2021). Composition analysis revealed that the extract yield was 7.23 ± 0.37%, with a higher polyphenol content (13.74 ± 0.78%) compared to carbohydrates and proteins. Thin layer chromatography (TLC) analysis revealed the presence of polyphenolic compounds associated with antioxidant activity. These compounds were visualized using fluorescence under UV light and various staining methods specific to different functional groups, including: 10% sulfuric acid in ethanol, p-anisaldehyde, sulfuric vanillin, potassium permanganate (KMnO4), iodine and ferric chloride (FeCl3).
Prasedya et al. (2022) employed an extraction method by maceration of the dry biomass of S. cristaefolium in 96% ethanol, in a ratio of 1:10 (w/v), with constant stirring at 100 rpm on a magnetic stirrer (Prasedya et al., 2022). The identification of bioactive compounds in the extract was carried out using the ultra-performance liquid chromatography technique associated with tandem mass spectrometry (UPLC-MS/MS). Through analysis, pheophorbide A (6) was identified as the most abundant compound, followed by 2-monoolein (7), eicosapentaenoic acid (8) and the diterpenoid kaurenoic acid (9).
On the other hand, Lee et al. (2022), used two forms of S. fusiforme (SF): one traditional and the other vaporized (SSF), subjecting them to extraction with hot water, 95% ethanol and methanol. Water extractions yielded the highest yields, 25% for SF and 19% for SSF (Lee et al., 2022). The ethanolic and methanolic extracts showed higher total polyphenol contents than those of SF, suggesting that heat treatment may lead to the loss of phenolic compounds. These results highlight the influence of solvents and heat treatment on the phenolic compound content of these seaweeds.
In addition, another traditional extraction method was recorded, such as soxhlet extraction (3.2%). In some research more focused on cosmetic formulations, as exemplified by the study conducted by Al-Momani et al. (2022), it is common to use the Soxhlet extractor. In this study, samples of Sargassum spp. were dried in the shade, pulverized, and extracted using a Soxhlet extractor with petroleum ether and ethanol solvents. It is important to highlight that detailed chemical analysis of these extracts were not addressed by the authors, as the focus is on evaluating the stability of the formulations. It is crucial to emphasize that several studies are conducted with a more applied focus. In view of this, it is essential to dedicate efforts to the chemical characterization of the extracts obtained in order to explore their real composition and, consequently, raise the quality standard of the products, ensuring the effectiveness and safety of products intended for consumers.
3.2.3 Green extraction methods
Green extraction techniques such as enzyme-assisted extraction (EAE) (25.8%), ultrasound-assisted extraction (UAE) (19.4%), and microwave-assisted extraction (MAE) (3.2%) were applied to obtain Sargassum extracts (Yuan and Macquarrie, 2015; Chemat et al., 2017; Fernando et al., 2020a, Fernando et al., 2020b, Fernando et al., 2021a, Fernando et al., 2021b; Dias et al., 2021). The MAE uses microwave energy as a volumetrically distributed heat source, generated by ionic conduction of dissolved ions and dipole rotation of polar solvents (Yuan and Macquarrie, 2015; Pekkoh et al., 2023). In contrast, the UAE uses physical forces generated by acoustic cavitation, such as shear, shock waves, microjets and acoustic flow, to extract molecules. Acoustic cavitation results in the rapid formation and collapse of cavitation bubbles within an irradiated liquid medium, causing intense stress and irreversible rupture of the chains (Yan et al., 2016). The Figure 4 summarizes the most notable characteristics of these green extraction techniques, including cost, yield, extraction time, selectivity, and environmental impact, compared to conventional extraction.
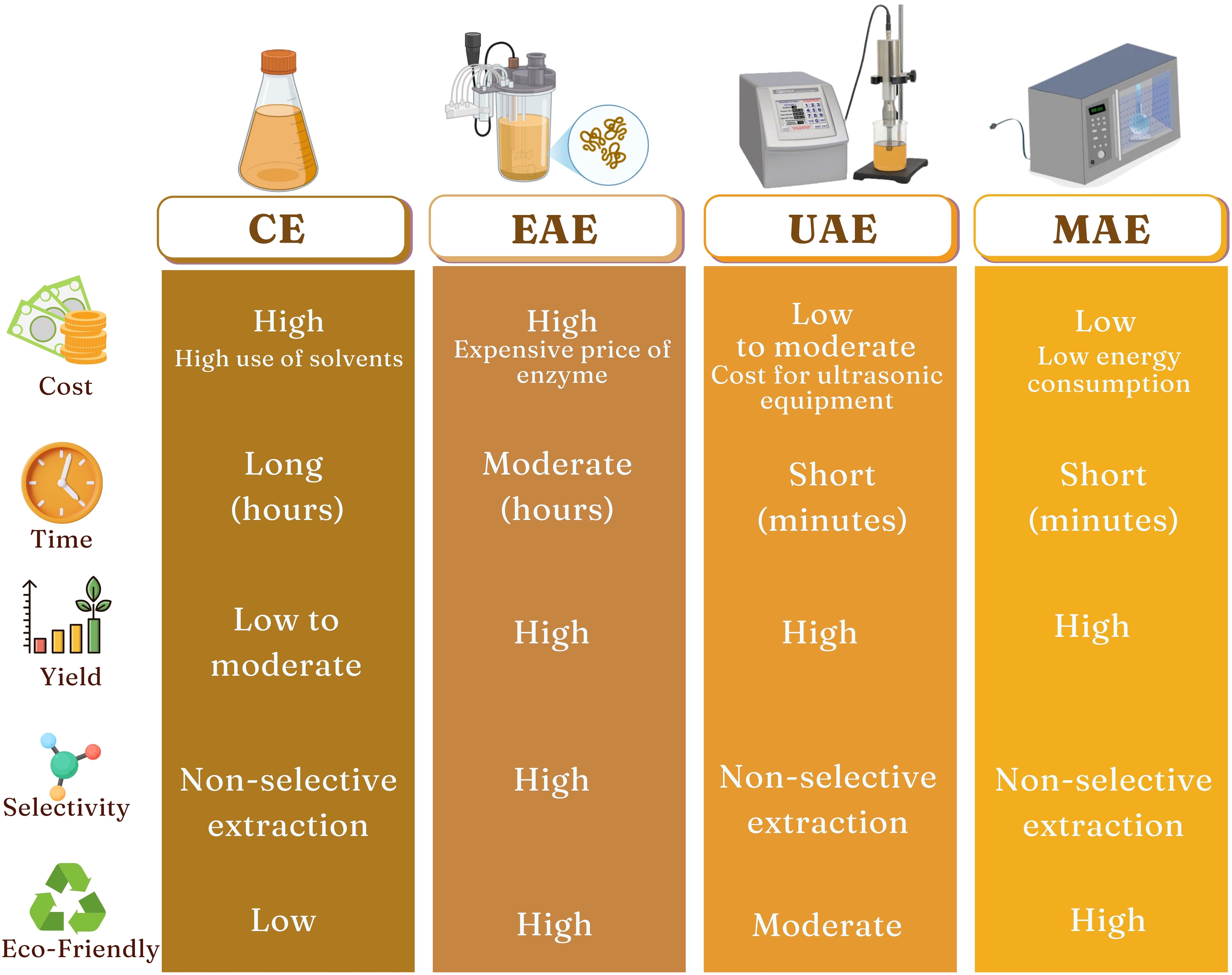
Figure 4. Compares four extraction methods: Ultrasound-Assisted Extraction (UAE), Microwave-Assisted Extraction (MAE), Enzymatic Extraction (EE), and Conventional Extraction (CE). This highlights the trade-offs in efficiency, cost, environmental impact, and duration among the methods relevant for scientific and industrial applications. indicating that the figures were created using Biorender® and Canva®.
Among the studies reviewed, the MAE technique was used to treat S. muticum (Grillo et al., 2021). In the study, algal biomass was mixed with a hydroalcoholic solution and subjected to a microwave reactor with temperature and pressure control, followed by filtration and freeze-drying. The MAE extraction efficiency was 22.14%, and total polyphenol analysis carried out using the Folin-Ciocalteau method revealed a content of 19.77 mg GAE/g in dry matrix and 25.88 mg GAE/g in the organic fraction. Furthermore, UAE has been shown to be effective in extracting other compounds such as polysaccharides and salts. The analysis of the results demonstrates the influence of the algal matrix on the extraction effectiveness, highlighting the importance of evaluating not only the quantity of extracted compounds but also the selectivity and efficiency of the process.
The UAE was employed in other studies included in this review (Arguelles et al., 2020; Gam et al., 2021; Grillo et al., 2021; Landa-Cansigno et al., 2023; Lim et al., 2023). Landa-Cansigno et al. (2023) prepared extracts rich in phenolic compounds, using two methods: (i) ultrasound-assisted extraction (UAE -SH), in which the algae were subjected to an extraction process in an ultrasound bath using an ethanol:water solution (50:50 v/v) for 30 minutes, at a temperature of 40°C; and (ii) conventional extraction (CONV-SH), in which the algal biomass was macerated in an acetone: water solution (70%, v/v) for 2 hours, maintaining a temperature of 40°C and continuous stirring at 200 rpm in a dark environment. The yield percentage was 2.30 ± 1.06% for UAE-SH and 4.99 ± 1.36% for CONV-SH, with no statistically significant differences between them. The EAU-SH extract presented a phenolic compound content of 26.17 ± 5.95 μg GAE/g dry weight.
Optimization of maximum extraction conditions using UAE of bioactive compounds from the alga S. thunbergii, demonstrating efficacy in extracting bioactives with potential for skin lightening and anti-wrinkle effects, was evaluated (Gam et al., 2021). In the UAE process using an ultrasound device with an electrical power of 200 W and a frequency of 40 kHz, the sample powder was placed in a pressure vessel with solvent and mixed. Seventeen different extraction conditions were tested, varying the time, temperature and ethanol concentration. For chemical characterization, the technique of liquid chromatography with electrospray ionization associated with tandem mass spectrometry (LC-ESI-MS/MS) was used. Among the compounds identified, caffeic acid (10) was highlighted as one of the main peaks, suggesting its significant presence in the S. thunbergii extract.
The EAE technique has been explored to obtain dermatofunctional polysaccharides from Sargassum (Fernando et al., 2020a, Fernando et al., 2020b, Fernando et al., 2020c, Fernando et al., 2020d, Fernando et al., 2021a, Fernando et al., 2021b; Kang et al., 2024; Wang et al., 2024). In summary, the methodology uses enzymatic hydrolysis, where the rigid and heterogeneous structures of the cell wall are weakened or ruptured, releasing the biocompounds of interest into the extraction medium (Teixeira-Guedes et al., 2023). In some studies where the focus was on obtaining polysaccharides, algal biomass was initially prepared by spraying and then subjected to a series of treatment steps. Initially, the biomass was suspended in a formaldehyde/ethanol solution (9:1, v/v) at 40°C - 50°C for 5 hours for depigmentation, followed by washing with 80% ethanol to remove formaldehyde residues and subsequently dehydrated at 50°C. After pretreatment, the powder was suspended in deionized water and adjusted to pH 5.0 for the addition of cellulases (Celluclast) and incubation for 8 hours. After enzymatic digestion, the suspension underwent a filtration process and gradient precipitation process with ethanol (Fernando et al., 2020a, Fernando et al., 2021a). The chemical characterization steps of polysaccharides involve the use of FTIR, 1H NMR, and HPLC-PAD techniques.
Celluclast extraction of S. coreanum showed a yield of 27.14 ± 0.43%, with the highest yield (12.25%) in the first fraction obtained from the precipitation gradient (SCOC1). This fraction had a content of 57.92%, 32.76% and 32.76% for fucose, mannose and sulfate, respectively. Furthermore, infrared and 1H NMR spectral patterns corresponded to fucoidans (Fernando et al., 2021a). Using this extraction method for the alga S. confusum, a yield of 27.13 ± 2.19% of fucoidans was obtained, with the active fraction (SCFC4) presenting levels of 23.62 ± 0.53% for sulfate and 36. 06 ± 0.94% for fucose (Fernando et al., 2020a). Finally, the gross yield of photoprotective fucoidans of different molecular weights (40 - 160, 50 - 95, 25 - 75 and 8 - 25 kDa) obtained from S. siliquastrum was 23.18 ± 0.18% (Fernando et al., 2020b). The fraction tested in this study (SSQC4; 8–25 kDa) had fucose and sulfate content of 40.94 ± 1.25% and 21.92 ± 0.46, respectively. The review also recorded a method for extracting extracellular vesicles (3.2%) from the alga S. fusiforme using phosphate-buffered saline (PBS) (Jang et al., 2021). This study carried out the extraction and subsequent centrifugation and filtration of the vesicles, using them in tests to regulate melanin synthesis.
3.2.4 Bioactive compounds
Polysaccharides, phenolic compounds, norisoprenoids, phenylpropanoids, diterpenes, pigments and saponins were the classes of metabolites identified in this review, demonstrating efficiency in several bioactivities and cosmeceutical applications. It is important to highlight that polysaccharides are widely recognized for their moisturizing and film-forming properties, which are essential in the formulation of products intended for moisture retention and skin protection. They may contribute to cell regeneration and promote smoother, more flexible skin (Kalasariya et al., 2024). Phenolic compounds have also garnered significant interest due to their strong antioxidant and anti-inflammatory activities, which directly protect the skin against oxidative stress and premature aging (Kalasariya and Pereira, 2022). Norisoprenoids, diterpenes, and phenylpropanoids are known for their anti-inflammatory, antimicrobial and antioxidant properties, making them effective in the treatment of inflammatory skin conditions,preventing infections, and stabilizing free radicals (Peng et al., 2018; Morais et al., 2021).
Pigments, in addition to their antioxidant functions, may offer protection from solar radiation (Sami et al., 2021). Finally, saponins are known for their emulsifying and cleansing properties, making them ideal for formulations intended for deep cleansing of skin and hair. In addition, they have anti-inflammatory activities and can help improve overall skin and scalp health (Mietlińska, 2023). The Figure 5 illustrates some compounds identified in Sargassum species, the results of which were discussed throughout sections 3.1 and 3.2.
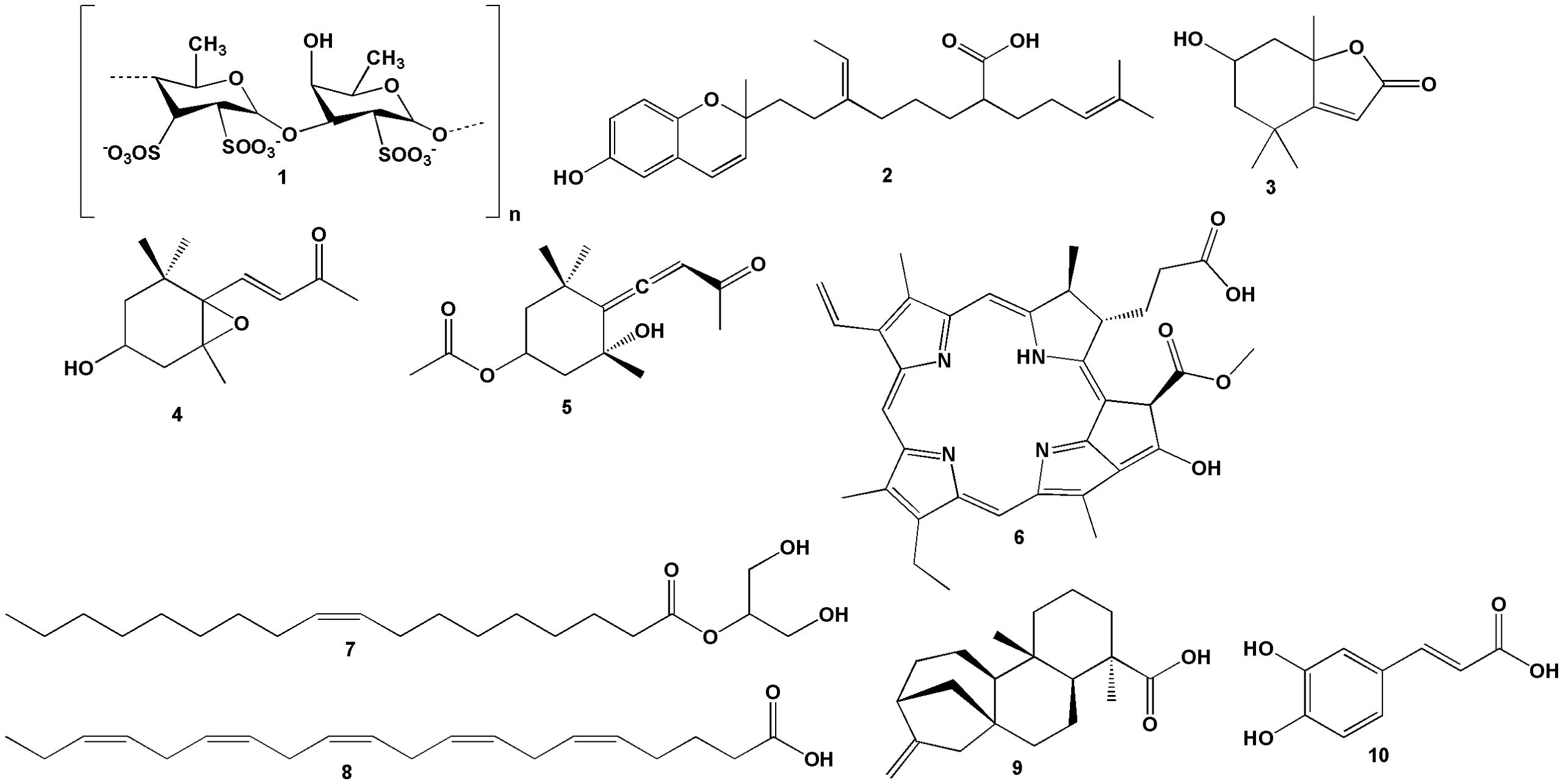
Figure 5. Compounds identified in Sargassum species with dermocosmetic properties. (1) Fucoidan (Fernando et al., 2020a, Fernando et al., 2020b, Fernando et al., 2021a, Fernando et al., 2021b); (2) mojabanchromanol (Kim et al., 2020); (3) (-)-loliolide (Kim et al., 2021); (4) 3-hydroxy-5,6-epoxy-β-ionone (Kim et al., 2021); (5) apo-9′-fucoxanthinone (Kim et al., 2021); (6) pheophorbide A (Prasedya et al., 2022); (7) 2-monoolein (Prasedya et al., 2022); (8) eicosapentaenoic acid (Prasedya et al., 2022); (9) kaurenoic acid (Prasedya et al., 2022) and (10) caffeic acid (Gam et al., 2021).
3.3 Industrial bioprospection of dermocosmetics based on Sargassum and its social and environmental impact
As discussed throughout this work, extracts, and compounds derived from Sargassum have several dermocosmetic properties, demonstrating significant potential for innovation and development (I&D) of new cosmetic products. The growing demand for components and additives from natural and sustainable sources has driven the cosmetic industry to seek eco-friendly alternatives (Bom et al., 2019; Cavallo et al., 2021; Matos et al., 2021; Troell et al., 2023). In this context, studies that investigate green methodologies for obtaining bioproducts from Sargassum can offer valuable contributions to various industrial sectors, especially cosmetics, thus aligning with the requirements of sustainability and efficiency (Grillo et al., 2021; Morais et al., 2021; Gager et al., 2024).
It is worth noting that Sargassum species are still underexploited, considering the tons of biomass deposited along the coasts of the Atlantic Ocean (Sissini et al., 2017; Arencibia-Carballo et al., 2020; Lee et al., 2023). Bioprospecting studies of Beach-Cast biomass have raised possibilities for exploration in numerous sectors, such as bioenergy, for the generation of biofuel; food, to obtain alginate and fucoidan; pharmaceutical; agricultural, through the production of animal feed and biostimulants; in addition to other applications, such as carbon sequestration (Gouvêa et al., 2020; Minicante et al., 2022; Santos et al., 2023). Despite these opportunities, it is essential to highlight some limitations involved in the bioprospecting of Sargassum in the cosmetics sector, among which the following stand out: (i) the quality control of the raw material and respective concerns with microbiological contamination and pollutants; (ii) seasonal fluctuations in Beach-Cast seaweed influenced by climate change; and (iii) regulations for the harvest and use of a beach-cast seaweeds.
Authors have reported concerns about the quality control of algal biomass collected from sites with many anthropogenic activities (Saldarriaga-Hernandez et al., 2020). The risk of microbiological contamination and heavy metals is an eminent concern, especially considering its cosmetic application. A recent study evaluated the heavy metal content in Sargassum samples obtained from four locations in the Caribbean, revealing values below 0.5 parts per million (ppm) for cadmium, mercury and lead, values below the levels allowed for most cosmetics (Lee et al., 2023). Despite these results, it is crucial to continue research monitoring these indices to ensure the safety of using Sargassum extracts as cosmetic additives.
The development of protocols to evaluate the quality of collected material is important not only for risk assessment but also to guarantee its cosmeceutical effects, mainly taking into account variations in the chemical profile related to biotic and abiotic factors at the collection sites (Obando et al., 2022). A possible strategy to ensure quality control and mitigate the effects of biomass fluctuations is investment in research on cultivation, aimed at the domestication of Sargassum in different cultivation systems, a strategy directly related to blue biotechnology. Furthermore, the rapid degradation of Sargassum on the beaches and the difficulty in processing the large amount of beach-cast seaweeds biomass are considered a limiting factor in the bioprospecting of these algae. Establishing a production chain that integrates different sectors to appropriate this marine resource is essential. In addition to what has been mentioned, the absence of a clear legislative framework regarding the use of beach-cast seaweeds biomass is also a significant issue that impedes the sector from developing effectively (Sousa et al., 2012; Andrade et al., 2020).
In pursuit of this goal, integrated efforts from multiple sectors (e.g., regulatory, Business to Consumer (B2C) companies, Business to business (B2B) companies, scientific and technological startups, and universities) are necessary. The scope of this interaction should be aligned with technological development at different maturity levels (Technology Readiness Levels – TRL), from basic research (TRL 1-3) to prototyping and validation (TRL 4-7), and scalability to commercialization (TRL 8-9). In this context, an integrative approach is essential for establishing and standardizing methods that provide transversal investigation of bioactive potentials and biosafety validation (Barthe et al., 2021).
To fully utilize Sargassum biomass as a raw material in the cosmeceutical industry with biosafety and economic, social, and environmental viability, it is essential to advance beyond extensive biotechnological knowledge and improve aspects of yield and process costs. These improvements are foundational for operational evaluations and scenarios needed to define concepts for scaling up biorefineries (Caxiano et al., 2022). This challenge also includes the considerations of scalability, safety, and replicability of bioproducts derived from Sargassum. In this approach, the concepts of green technologies (e.g., supercritical fluid extraction, pressurized liquids, UAE, and MAE) applied to industrial biorefineries are fundamental to being employed in investigative research as a mechanism to accelerate technological development (Hempel et al., 2023) (Figure 6).
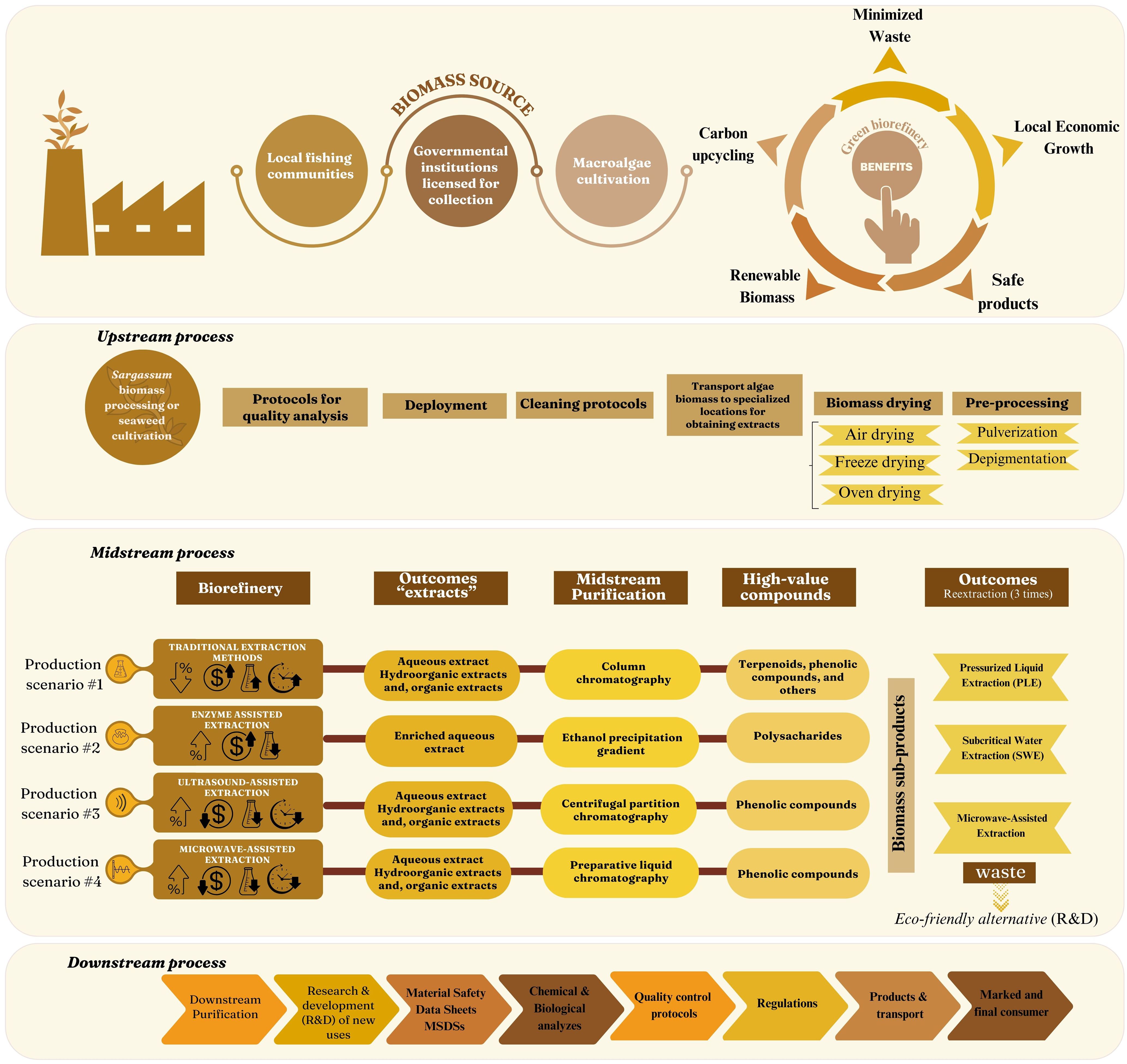
Figure 6. Illustrative scenarios of integrated biorefineries for the cosmetic industry involving the processing of Sargassum macroalgae biomass using green technologies. Encompassing the entire value chain, from sourcing biomass (upstream) and intermediate processing (midstream), to refining final products (downstream).
Overcoming these challenges and the implementation of sustainable Sargassum biorefineries will help establish an economic chain based on the use of Sargassum to obtain high value-added products. This will contribute to the consolidation and achievement of several SDGs, including the promotion of sustainable economic growth (SDG 8), the establishment of sustainable production and consumption patterns (SDG 12), and life below water (SDG 14).
4 Conclusions
This study explored the properties of 17 species of Sargassum, with S. horneri being the most widely researched globally, making it an interesting target for bioprospecting due to its cosmopolitan distribution and years of progress in related studies. Aspects such as photoprotection, antioxidant activity, and anti-inflammatory effects are receiving increasing attention, reflecting a broader interest in health promotion within the cosmetic field. On the other hand, the experimental models predominantly involve in vitro tests; however, in vivo bioassays in mouse and zebrafish models, clinical trials, and in silico experiments are also documented, indicating recent interest in the in vivo validation of bioactivities for cosmetic purposes using this algae. There is a growing interest in green extraction methodologies, particularly enzymatic extraction focused on obtaining polysaccharides. These methodologies are often complemented by advanced identification and characterization techniques, including LC-MS and HPLC-PAD. These innovative approaches not only emphasize sustainability but also enhance the efficiency and yield of extracting valuable compounds from natural sources. This trend highlights the importance of utilizing, investigating, and developing these techniques, opening up opportunities for sustainable cosmetic development within the goals of the blue economy.
Research on Sargassum faces several significant challenges that must be addressed to unlock its potential. Key requirements include ensuring quality control of raw materials, particularly regarding microbiological contamination and pollutants. Additionally, fluctuations in the availability of seaweeds, driven by climate change, present obstacles to consistent biomass supply. Moreover, the regulatory frameworks governing the collection and use of beach-cast seaweeds are still quite limited. These challenges have increasingly attracted the attention of researchers, especially in regions with abundant biomass and Beach-Cast effects. Addressing these concerns will be vital for advancing Sargassum as a sustainable resource in various applications of the cosmetic sector.
Author contributions
TC: Conceptualization, Data curation, Formal analysis, Investigation, Methodology, Supervision, Writing – original draft, Writing – review & editing, Visualization. LP: Writing – review & editing, Resources. ALVPS: Data curation, Writing – original draft. RC: Writing – review & editing. DC: Writing – review & editing. GW: Funding acquisition, Project administration, Resources, Supervision, Writing – review & editing. AM: Writing – review & editing. JC: Conceptualization, Data curation, Formal analysis, Investigation, Methodology, Supervision, Writing – original draft, Writing – review & editing.
Funding
The author(s) declare financial support was received for the research, authorship, and/or publication of this article. This workwas supported by the Research Center on Biodiversity Dynamics and Climate Change – CbioClima FAPESP Grants No. 21/10639-5 and Grants No. 22/02756-4 and National Institute of Science and Technology in Nanotechnology for Sustainable Agriculture – MCTI/CNPq/INCT Nano Agro #405924/2022-4 and Grants DT #303653/2022-1.
Acknowledgments
We would like to thank the Coordenação de Aperfeiçoamento de Pessoal de Nível Superior (CAPES) for PhD (TC) and we thank the INCT Nanotechnology for Sustainable Agriculture, the Coordination for the Improvement of Higher Education Personnel – Brazil (MEC-CAPES INCTNanoAgro #888887.986628/2024-00), and the São Paulo Research Foundation (FAPESP) for the postdoctoral fellowship.
Conflict of interest
The authors declare that the research was conducted in the absence of any commercial or financial relationships that could be construed as a potential conflict of interest.
Publisher’s note
All claims expressed in this article are solely those of the authors and do not necessarily represent those of their affiliated organizations, or those of the publisher, the editors and the reviewers. Any product that may be evaluated in this article, or claim that may be made by its manufacturer, is not guaranteed or endorsed by the publisher.
References
Al-Momani R. M., Arabeyyat Z. H., Malkawi E. E., Al-Zibdah M. K. (2022). Formulation and evaluation of herbal antioxidant face cream using extract of the marine seaweed Sargassum spp. (Phaeophyceae). J. Pharm. Res. 26, 828–833. doi: 10.29228/jrp.180
Amorim A. M., Nardelli A. E., Chow F. (2020). Effects of drying processes on antioxidant properties and chemical constituents of four tropical macroalgae suitable as functional bioproducts. J. Appl. Phycol. 32, 1495–1509. doi: 10.1007/s10811-020-02059-7
Andrade H. M. M., de Q., Rosa L. P., de Souza F. E. S., da Silva N. F., Cabral M. C., et al. (2020). Seaweed production potential in the Brazilian Northeast: A study on the eastern coast of the State of Rio Grande do Norte, RN, Brazil. Sustainability (Switzerland). 12, 780. doi: 10.3390/su12030780
Arencibia-Carballo G., Irañeta Batallán J. M., Morell J., González A. R. M. (2020). Arribazones de Sargassum en la costa norte occidental de Cuba. JAINA Costas y Mares ante el Cambio Climático 2, 19–30. doi: 10.26359/52462.0220
Arguelles E., de L. R., Sapin A. B. (2020). Bioactive properties of Sargassum siliquosum J. Agardh (Fucales, Ochrophyta) and its potential as source of skin-lightening active ingredient for cosmetic application. J. Appl. Pharm. Sci. 10, 51–58. doi: 10.7324/JAPS.2020.10707
Arguelles E. D. L. R. (2021). Evaluation of antioxidant capacity, tyrosinase inhibition, and antibacterial activities of brown seaweed, sargassum ilicifolium Available online at: https://li01.tci-thaijo.org/index.php/JFE/article/view/242157 (Turner) C. Agardh 1820 for cosmeceutical application. J. Fish. Environ. 45, 64–77.
Barthe M., Bavoux C., Finot F., Mouche I., Cuceu-Petrenci C., Forreryd A., et al. (2021). Safety testing of cosmetic products: Overview of established methods and new approach methodologies (nams). Cosmetics. 8, 50. doi: 10.3390/cosmetics8020050
Bom S., Jorge J., Ribeiro H. M., Marto J. (2019). A step forward on sustainability in the cosmetics industry: A review. J. Clean. Prod. 225, 270–290. doi: 10.1016/j.jclepro.2019.03.255
Catarino M. D., Silva-Reis R., Chouh A., Silva S., Braga S. S., Silva A. M., et al. (2023). Applications of antioxidant secondary metabolites of Sargassum spp. Mar. Drugs 21, 172. doi: 10.3390/md21030172
Cavallo G., Lorini C., Garamella G., Bonaccorsi G. (2021). Seaweeds as a “palatable” challenge between innovation and sustainability: A systematic review of food safety. Sustainability (Switzerland). 13, 7652. doi: 10.3390/su13147652
Caxiano I. N., Mello P. A., Alijó P. H. R., Teixeira L. V., Cano R. F., Maia J. G. S. S., et al. (2022). Continuous design and economic analysis of a Sargassum muticum biorefinery process. Bioresour. Technol. 343, 126152. doi: 10.1016/j.biortech.2021.126152
Chemat F., Rombaut N., Sicaire A. G., Meullemiestre A., Fabiano-Tixier A. S., Abert-Vian M. (2017). Ultrasound assisted extraction of food and natural products. Mechanisms, techniques, combinations, protocols and applications. A review. Ultrason. Sonochem. 34, 540–560. doi: 10.1016/j.ultsonch.2016.06.035
Cruz A. M., Gonçalves M. C., Marques M. S., Veiga F., Paiva-Santos A. C., Pires P. C. (2023). In vitro models for anti-aging efficacy assessment: A critical update in dermocosmetic research. Cosmetics. 10, 66. doi: 10.3390/cosmetics10020066
Delavary B. M., van der Veer W. M., van Egmond M., Niessen F. B., Beelen R. H. J. (2011). Macrophages in skin injury and repair. Immunobiology. 216, 753–762. doi: 10.1016/j.imbio.2011.01.001
Dias M. K. H. M., Madusanka D. M. D., Han E. J., Kim H. S., Jeon Y. J., Jee Y., et al. (2021). Sargassum horneri (Turner) C. Agardh ethanol extract attenuates fine dust-induced inflammatory responses and impaired skin barrier functions in HaCaT keratinocytes. J. Ethnopharmacol. 273, 114003. doi: 10.1016/j.jep.2021.114003
Djaeni M., Sari D. A. (2015). Low temperature seaweed drying using dehumidified air. Proc. Environ. Sci. 23, 2–10. doi: 10.1016/j.proenv.2015.01.002
Farage M. A., Miller K. W., Elsner P., Maibach H. I. (2013). Characteristics of the aging skin. Adv. Wound. Care (New Rochelle) 2, 5–10. doi: 10.1089/wound.2011.0356
Fernando I. P. S., Dias M. K. H. M., Madusanka D. M. D., Han E. J., Kim M. J., Heo S. J., et al. (2021a). Fucoidan fractionated from Sargassum coreanum via step-gradient ethanol precipitation indicate promising uvb-protective effects in human keratinocytes. Antioxidants. 10, 1–17. doi: 10.3390/antiox10030347
Fernando I. P. S., Dias M. K. H. M., Madusanka D. M. D., Han E. J., Kim M. J., Heo S. J., et al. (2021b). Low molecular weight fucoidan fraction ameliorates inflammation and deterioration of skin barrier in fine-dust stimulated keratinocytes. Int. J. Biol. Macromol. 168, 620–630. doi: 10.1016/j.ijbiomac.2020.11.115
Fernando I. P. S., Dias M. K. H. M., Madusanka D. M. D., Han E. J., Kim M. J., Jeon Y. J., et al. (2020a). Fucoidan refined by Sargassum confusum indicate protective effects suppressing photo-oxidative stress and skin barrier perturbation in UVB-induced human keratinocytes. Int. J. Biol. Macromol. 164, 149–161. doi: 10.1016/j.ijbiomac.2020.07.136
Fernando I. P. S., Dias M. K. H. M., Madusanka D. M. D., Han E. J., Kim M. J., Jeon Y. J., et al. (2020b). Step gradient alcohol precipitation for the purification of low molecular weight fucoidan from Sargassum siliquastrum and its UVB protective effects. Int. J. Biol. Macromol. 163, 26–35. doi: 10.1016/j.ijbiomac.2020.06.232
Fernando I. P. S., Dias M. K. H. M., Madusanka D. M. D., Han E. J., Kim M. J., Jeon Y. J., et al. (2020c). Human keratinocyte uvb-protective effects of a low molecular weight fucoidan from Sargassum horneri purified by step gradient ethanol precipitation. Antioxidants. 9, 340. doi: 10.3390/antiox9040340
Fernando I. P. S., Sanjeewa K. K. A., Lee H. G., Kim H. S., Vaas A. P. J. P., De Silva H. I. C., et al. (2020d). Characterization and cytoprotective properties of Sargassum natans fucoidan against urban aerosol-induced keratinocyte damage. Int. J. Biol. Macromol. 159, 773–781. doi: 10.1016/j.ijbiomac.2020.05.132
Flores-Contreras E. A., Araújo R. G., Rodríguez-Aguayo A. A., Guzmán-Román M., García-Venegas J. C., Nájera-Martínez E. F., et al. (2023). Polysaccharides from the sargassum and brown algae genus: extraction, purification, and their potential therapeutic applications. Plants. 12, 2445. doi: 10.3390/plants12132445
Gager L., Connan S., Cérantola S., Petek S., Couteau C., Coiffard L., et al. (2024). An eco-friendly extraction and purification approach for obtaining active ingredients for cosmetics from two marine brown seaweeds. Mar. Drugs 22, 112. doi: 10.3390/md22030112
Gam D. H., Park J. H., Hong J. W., Jeon S. J., Kim J. H., Kim J. W. (2021). Effects of Sargassum thunbergii extract on skin whitening and anti-wrinkling through inhibition of TRP-1 and MMPs. Molecules. 26, 7381. doi: 10.3390/molecules26237381
Gouvêa L. P., Assis J., Gurgel C. F. D., Serrão E. A., Silveira T. C. L., Santos R., et al. (2020). Golden carbon of Sargassum forests revealed as an opportunity for climate change mitigation. Sci. Total Environ. 729, 138745. doi: 10.1016/j.scitotenv.2020.138745
Grillo G., Tabasso S., Solarino R., Cravotto G., Toson C., Ghedini E., et al. (2021). From seaweeds to cosmeceutics: A multidisciplinar approach. Sustainability (Switzerland) 13, 13443. doi: 10.3390/su132313443
Guerrero D. (2012). Dermocosmetic management of hyperpigmentations. Ann. Dermatol. Venereol. 139, 166–169. doi: 10.1016/S0151-9638(12)70121-7
Guiry M. D., Guiry G. M. (2024). AlgaeBase (World-wide electronic publication, University of Galway). Available online at: https://www.algaebase.org (Accessed 20 May 2024).
Hay R. J., Augustin M., Griffiths C. E. M., Sterry W. (2015). The global challenge for skin health. Br. J. Dermatol. 172, 1469–1472. doi: 10.1111/bjd.13854
Hempel M., de S. S., Colepicolo P., Zambotti-Villela L. (2023). Macroalgae biorefinery for the cosmetic industry: basic concept, green technology, and safety guidelines. Phycology. 3, 211–241. doi: 10.3390/phycology3010014
Ho T. L., Lee J., Ahn S. Y., Lee D. H., Song W. J., Kang I., et al. (2023). Immunostimulatory effects of marine algae extracts on in vitro antigen-presenting cell activation and in vivo immune cell recruitment. Food. Sci. Nutr. 11, 6560–6570. doi: 10.1002/fsn3.3605
Holdt S. L., Kraan S. (2011). Bioactive compounds in seaweed: Functional food applications and legislation. J. Appl. Phycol. 23, 543–597. doi: 10.1007/s10811-010-9632-5
Jang B., Chung H., Jung H., Song H. K., Park E., Choi H. S., et al. (2021). Extracellular vesicles from korean Codium fragile and Sargassum fusiforme negatively regulate melanin synthesis. Mol. Cells 44, 736–745. doi: 10.14348/MOLCELLS.2021.2167
Jesumani V., Du H., Pei P., Aslam M., Huang N. (2020). ). Comparative study on skin protection activity of polyphenol-rich extract and polysaccharide-rich extract from Sargassum vachellianum. PloS One 15, e0227308. doi: 10.1371/journal.pone.0227308
Kalasariya H. S., Maya-Ramírez C. E., Cotas J., Pereira L. (2024). Cosmeceutical significance of seaweed: A focus on carbohydrates and peptides in skin applications. Phycology. 4, 276–313. doi: 10.3390/phycology4020015
Kalasariya H. S., Pereira L. (2022). Dermo-cosmetic benefits of marine macroalgae-derived phenolic compounds. Appl. Sci. 12, 11954. doi: 10.3390/app122311954
Kang J. W., Hyun S. H., Kim H. M., Park S. Y., Lee J. A., Lee I. C., et al. (2024). The effects of fucoidan-rich polysaccharides extracted from Sargassum horneri on enhancing collagen-related skin barrier function as a potential cosmetic product. J. Cosmet. Dermatol. 23, 1365–1373. doi: 10.1111/jocd.16108
Karthikeyan A., Joseph A., Nair B. G. (2022). Promising bioactive compounds from the marine environment and their potential effects on various diseases J. Genet. Eng. Biotechnol. 20, 14. doi: 10.1186/s43141-021-00290-4
Kim H. S., Fernando I. P. S., Lee S. H., Ko S. C., Kang M. C., Ahn G., et al. (2021). Isolation and characterization of anti-inflammatory compounds from Sargassum horneri via high-performance centrifugal partition chromatography and high-performance liquid chromatography. Algal. Res. 54, 102209. doi: 10.1016/j.algal.2021.102209
Kim H. S., Han E. J., Fernando I. P. S., Sanjeewa K. K. A., Jayawardena T. U., Kim H. J., et al. (2020). Anti-allergy effect of mojabanchromanol isolated from Sargassum horneri in bone marrow-derived cultured mast cells. Algal Res. 48, 101898. doi: 10.1016/j.algal.2020.101898
Kirindage K. G. I. S., Jayasinghe A. M. K., Ko C.-I., Ahn Y.-S., Heo S.-J., Oh J.-Y., et al. (2024). Unveiling the potential of ultrasonic-assisted ethanol extract from sargassum horneri in inhibiting tyrosinase activity and melanin production in B16F10 murine melanocytes. Front. Biosci. 29, 194. doi: 10.31083/j.fbl2905194
Landa-Cansigno C., Serviere-Zaragoza E., Morales-Martínez T. K., Ascacio-Valdes J. A., Morreeuw Z. P., Gauyat C., et al. (2023). The antioxidant and anti-elastase activity of the brown seaweed Sargassum horridum (Fucales, Phaeophyceae) and their early phenolics and saponins profiling for green cosmetic applications. Algal. Res. 75, 103271. doi: 10.1016/j.algal.2023.103271
Lee H. H., Kim J. S., Jeong J. H., Lee S. Y., Kim C. S. (2022). Comparative analysis of biological activities and phenolic content between fresh and steamed sargassum fusiforme in different extraction solvents. Appl. Sci. 12, 12161. doi: 10.3390/app122312161
Lee M. C., Yeh H. Y., Chang C. M., Liou Y. F., Nan F. H., Wungen-Sani J. (2023). Tracking and utilizing sargassum, an abundant resource from the caribbean sea. Water (Switzerland) 15, 2694. doi: 10.3390/w15152694
Lee M. K., Ryu H., Lee J. Y., Jeong H. H., Baek J., Van J. Y., et al. (2022). Potential beneficial effects of sargassum spp. in skin aging. Mar. Drugs 20, 540. doi: 10.3390/md20080540
Liang Y., Su W., Wang F. (2023). Skin ageing: A progressive, multi-factorial condition demanding an integrated, multilayer-targeted remedy. Clin. Cosmet. Investig. Dermatol. 16, 1215–1229. doi: 10.2147/CCID.S408765
Lim M. W., Yow Y. Y., Gew L. T. (2023). LC–MS profiling-based non-targeted secondary metabolite screening for deciphering cosmeceutical potential of Malaysian algae. J. Cosmet. Dermatol. 22, 2810–2815. doi: 10.1111/jocd.15794
Maranduca M., Hurjui L., Branisteanu D., Serban D., et al. (2020). Skin - a vast organ with immunological function (Review). Exp. Ther. Med. 20, 18–23. doi: 10.3892/etm.2020.8619
Matias M., Martins A., Alves C., Silva J., Pinteus S., Fitas M., et al. (2023). New insights into the dermocosmetic potential of the red seaweed Gelidium corneum. Antioxidants. 12, 1684. doi: 10.3390/antiox12091684
Matos G. S., Pereira S. G., Genisheva Z. A., Gomes A. M., Teixeira J. A., Rocha C. M. R., et al. (2021). foods advances in extraction methods to recover added-value compounds from seaweeds: sustainability and functionality. Foods. 10, 516. doi: 10.3390/foods10030516
Mietlińska K. (2023). Biotechnology and Food Science Polyphenols and saponins-properties and application in cosmetics. Biotechnol. Food. Sci. 85, 16–33. doi: 10.34658/bfs.2023.85.1.16-33
Mingu N., Saad K. M., Mamat H., Siddique M. S., Majid M. D., Sarjadi M. S. (2024). Comparative Study of Drying Methods on Seaweeds (Kappaphycus sp. and Padina sp.) Based on Their Phytochemical and Polysaccharaide Content Located in Sabah: Comparative study of drying methods on Seaweeds. Borneo J. Resour. Sci. Technol. 14, 112–122. doi: 10.33736/bjrst.6089.2024
Minicante S. A., Bongiorni L., De Lazzari A. (2022). Bio-based products from mediterranean seaweeds: italian opportunities and challenges for a sustainable blue economy. Sustainability. (Switzerland). 14, 5634. doi: 10.3390/su14095634
Mohiuddin A. K. (2019). Skin lightening & Management of hyperpigmentation. Pharm. Sci. Anal. Res. 2, 180020–180068. doi: 10.53555/bp.v5i6.964
Mondello A., Salomone R., Mondello G. (2024). Exploring circular economy in the cosmetic industry: Insights from a literature review. Environ. Impact. Assess. Rev. 105, 107443. doi: 10.1016/j.eiar.2024.107443
Morais T., Cotas J., Pacheco D., Pereira L. (2021). Seaweeds compounds: An ecosustainable source of cosmetic ingredients? Cosmetics. 8, 1–28. doi: 10.3390/COSMETICS8010008
Obando J. M. C., Santos T. C., dos Bernardes M., Nascimento N., Villaça R. C., Barbarino E., et al. (2024). Diterpene biosynthesis of seaweed Canistrocarpus cervicornis a potential method by diterpene obtention in brown algae using aqueous extract as an enzyme source. HSOA J. Aquac. Fish. 87, 1–7. doi: 10.24966/AAF-5523/100087
Obando J. M. C., Santos T. C., Martins R. C. C., Teixeira V. L., Barbarino E., Cavalcanti D. N. (2022). Current and promising applications of seaweed culture in laboratory conditions. Aquaculture. 560, 738596. doi: 10.1016/j.aquaculture.2022.738596
Pekkoh J., Ruangrit K., Kaewkod T., Tragoolpua Y., Hoijang S., Srisombat L., et al. (2023). Innovative eco-friendly microwave-assisted rapid biosynthesis of ag/agCl-NPs coated with algae bloom extract as multi-functional biomaterials with non-toxic effects on normal human cells. Nanomaterials. 13, 2141. doi: 10.3390/nano13142141
Peng Y., Huang R. M., Lin X. P., Liu Y. H. (2018). Norisoprenoids from the Brown alga Sargassum naozhouense Tseng et Lu. Molecules 23, 348. doi: 10.3390/molecules23020348
Pérez-Larrán P., Torres M. D., Flórez-Fernández N., Balboa E. M., Moure A., Domínguez H. (2019). Green technologies for cascade extraction of Sargassum muticum bioactives. J. Appl. Phycol. 31, 2481–2495. doi: 10.1007/s10811-018-1725-6
Phang S. J., Teh H. X., Looi M. L., Arumugam B., Fauzi M. B., Kuppusamy U. R. (2023). Phlorotannins from brown algae: A review on their antioxidant mechanisms and applications in oxidative stress-mediated diseases. J. Appl. Psychol. 35, 867–892. doi: 10.1007/s10811-023-02913-4
Prasedya E. S., Padmi H., Ilhami B. T. K., Martyasari N. W. R., Sunarwidhi A. L., Widyastuti S., et al. (2022). Brown macroalgae sargassum cristaefolium extract inhibits melanin production and cellular oxygen stress in B16F10 melanoma cells. Molecules. 27, 8585. doi: 10.3390/molecules27238585
Rushdi M. I., Abdel-Rahman I. A. M., Saber H., Attia E. Z., Abdelraheem W. M., Madkour H. A., et al. (2020). Pharmacological and natural products diversity of the brown algae genus: Sargassum. RSC Adv. 10, 24951–24972. doi: 10.1039/d0ra03576a
Russell-Goldman E., Murphy G. F. (2020). The pathobiology of skin aging: new insights into an old dilemma. Am. J. Pathol. 190, 1356–1369. doi: 10.1016/j.ajpath.2020.03.007
Saldarriaga-Hernandez S., Hernandez-Vargas G., Iqbal H. M. N., Barceló D., Parra-Saldívar R. (2020). Bioremediation potential of Sargassum sp. biomass to tackle pollution in coastal ecosystems: Circular economy approach. Sci. Total. Environ. 715, 136978. doi: 10.1016/j.scitotenv.2020.136978
Sami F. J., Soekamto N. H., Firdaus Latip J. (2021). Bioactivity profile of three types of seaweed as an antioxidant, uv-protection as sunscreen and their correlation activity. Food. Res. 5, 441–447. doi: 10.26656/fr.2017.5(1).389
Santhoshkumar P., Yoha K. S., Moses J. A. (2023). Drying of seaweed: Approaches, challenges and research needs. Trends Food Sci. Technol. 138, 153–163. doi: 10.1016/j.tifs.2023.06.008
Santos T. C., dos, Obando J. M. C., Leite P. E. C., Pereira M. R., Leitão M., de F., et al. (2024). Approaches of marine compounds and relevant immune mediators in Autism Spectrum Disorder: Opportunities and challenges. Eur. J. Med. Chem. 266, 116153. doi: 10.1016/j.ejmech.2024.116153
Santos J. M., Jesus B. C., Ribeiro H., Martins A., Marto J., Fitas M., et al. (2024). Extraction of macroalgae phenolic compounds for cosmetic application using eutectic solvents. Algal. Res. 79, 103438. doi: 10.1016/j.algal.2024.103438
Santos T. C., Vale T. M., Cavalcanti D. N., MaChado L. P., Barbarino E., Martins R. C. C., et al. (2023). Bioactive metabolites and biotechnological applications of macroalgae of the genus sargassum: A review. Rev. Virt. Quím. 22, 1–18. doi: 10.21577/1984-6835.20220125
Sayin S., Depci T., Naz M., Sezer S., Karaaslan M. G., Aras A., et al. (2022). Characterization and evaluation of the antimicrobial properties of algal alginate; a potential natural protective for cosmetics. J. Pharm. Res. 26, 198–209. doi: 10.29228/jrp.117
Sissini M. N., De Barros Barreto M. B. B., Szećhy M. T. M., De Lucena M. B., Oliveira M. C., Gower J., et al. (2017). The floating Sargassum (Phaeophyceae) of the South Atlantic Ocean - Likely scenarios. Phycologia. 56, 321–328. doi: 10.2216/16-92.1
Sousa F. E. S., Moura E. A., Marinho-Soriano E. (2012). Use of geographic information systems (GIS) to identify adequate sites for cultivation of the seaweed Gracilaria birdiae in Rio Grande do Norte, Northeastern Brazil. Rev. Bras. farmacogn. 22, 868–873. doi: 10.1590/S0102
Stiger-Pouvreau V., Mattio L., De Ramon N’Yeurt A., Uwai S., Dominguez H., Flórez-Fernández N., et al. (2023). A concise review of the highly diverse genus Sargassum C. Agardh with wide industrial potential. J. Appl. Phycol. 35, 1453–1483. doi: 10.1007/s10811-023-02959-4
Takashi H. (2021). Cosmetic potential of boiled water of Hijiki (Sargassum fusiforme) grown in the ocean in Okinawa, Japan. Res. Square. doi: 10.21203/rs.3.rs-278127/v1
Tanniou A., Vandanjon L., Incera M., Serrano Leon E., Husa V., Le Grand J., et al. (2014). Assessment of the spatial variability of phenolic contents and associated bioactivities in the invasive alga Sargassum muticum sampled along its European range from Norway to Portugal. J. Appl. Phycol. 26, 1215–1230. doi: 10.1007/s10811-013-0198-x
Teixeira-Guedes C., Gomes-Dias J. S., Cunha S. A., Pintado M. E., Pereira R. N., Teixeira J. A., et al. (2023). Enzymatic approach for the extraction of bioactive fractions from red, green and brown seaweeds. Food Bioprod. Process. 138, 25–39. doi: 10.1016/j.fbp.2022.12.005
Troell M., Costa-Pierce B., Stead S., Cottrell R. S., Brugere C., Farmery A. K., et al. (2023). Perspectives on aquaculture’s contribution to the Sustainable Development Goals for improved human and planetary health. J. World Aquac. Soc 54, 251–342. doi: 10.1111/jwas.12946
Tziveleka L. A., Tammam M. A., Tzakou O., Roussis V., Ioannou E. (2021). Metabolites with antioxidant activity from marine macroalgae. Antioxidants. 10, 1431. doi: 10.3390/antiox10091431
Uribe E., Vega-Gálvez A., Vargas N., Pasten A., Rodríguez K., Ah-Hen K. S. (2018). Phytochemical components and amino acid profile of brown seaweed Durvillaea Antarctica as affected by air drying temperature. J. Food Sci. Technol. 55, 4792–4801. doi: 10.1007/s13197-018-3412-7
Wang L., Cui Y. R., Wang K., Fu X., Xu J., Gao X., et al. (2022). Anti-inflammatory effect of fucoidan isolated from fermented Sargassum fusiforme in in vitro and in vivo models. Int. J. Biol. Macromol. 222, 2065–2071. doi: 10.1016/j.ijbiomac.2022.10.005
Wang L., Kim H. S., Je J. G., Fu X., Huang C., Ahn G., et al. (2021). In vitro and in vivo photoprotective effects of (-)-loliode isolated from the brown seaweed Sargassum horneri. Molecules. 26, 6898. doi: 10.3390/molecules26226898
Wang X., Huang C., Yang F., Wang K., Cha S. H., Mao X., et al. (2024). Fucoidan isolated from the edible seaweed Sargassum fusiforme suppresses skin damage stimulated by airborne particulate matter. Algal. Res. 77, 103339. doi: 10.1016/j.algal.2023.103339
Wang X., Zhang Z., Zhang S., Yang F., Yang M., Zhou J., et al. (2021). Antiaging compounds from marine organisms. Food Res. Int. 143, 110313. doi: 10.1016/j.foodres.2021.110313
Wong Q. Y. A., Chew F. T. (2021). Defining skin aging and its risk factors: a systematic review and meta-analysis. Sci. Rep. 11, 22075. doi: 10.1038/s41598-021-01573-z
Woo G. E., Hwang H. J., Park A. Y., Sim J. Y., Woo S. Y., Kim M. J., et al. (2023). Anti-atopic activities of sargassum horneri hot water extracts in 2,4-dinitrochlorobezene- induced mouse models. J. Microbiol. Biotechnol. 33, 363–370. doi: 10.4014/jmb.2211.11007
Yan J. K., Wang Y. Y., Ma H., Wang Le, Bin. Z. (2016). Ultrasonic effects on the degradation kinetics, preliminary characterization and antioxidant activities of polysaccharides from Phellinus linteus mycelia. Ultrason. Sonochem. 29, 251–257. doi: 10.1016/j.ultsonch.2015.10.005
Yuan Y., Macquarrie D. (2015). Microwave assisted extraction of sulfated polysaccharides (fucoidan) from Ascophyllum nodosum and its antioxidant activity. Carbohydr. Polym. 129, 101–107. doi: 10.1016/j.carbpol.2015.04.057
Zhao W., Yang A., Wang J., Huang D., Deng Y., Zhang X., et al. (2022). Potential application of natural bioactive compounds as skin-whitening agents: A review. J. Cosmet. Dermatol. 21, 6669–6687. doi: 10.1111/jocd.15437
Keywords: brown seaweed, marine natural products, cosmeceutical, bioeconomy, bioprospecting, dermoactive
Citation: Cunha dos Santos T, Pompermayer Machado L, Pimentel Santos ALV, Campos Martins RC, Cavalcanti DN, Wolff Bueno G, Madeira Sanches AL and Concha Obando JM (2024) Dermocosmetic properties of bioproducts from Sargassum macroalgae: chemical aspects, challenges, and opportunities. Front. Mar. Sci. 11:1500778. doi: 10.3389/fmars.2024.1500778
Received: 23 September 2024; Accepted: 16 October 2024;
Published: 28 November 2024.
Edited by:
Saravana Periaswamy Sivagnanam, Cork Institute of Technology, IrelandReviewed by:
Byung-Soo Chun, Pukyong National University, Republic of KoreaMonjurul Haq, Jashore University of Science and Technology, Bangladesh
Copyright © 2024 Cunha dos Santos, Pompermayer Machado, Pimentel Santos, Campos Martins, Cavalcanti, Wolff Bueno, Madeira Sanches and Concha Obando. This is an open-access article distributed under the terms of the Creative Commons Attribution License (CC BY). The use, distribution or reproduction in other forums is permitted, provided the original author(s) and the copyright owner(s) are credited and that the original publication in this journal is cited, in accordance with accepted academic practice. No use, distribution or reproduction is permitted which does not comply with these terms.
*Correspondence: Johana Marcela Concha Obando, am9oYW5hbWFyY2VsYUBzZWFsbGcuY29t; Thalisia Cunha dos Santos, dGhhbGlzaWFAc2VhbGxnLmNvbQ==