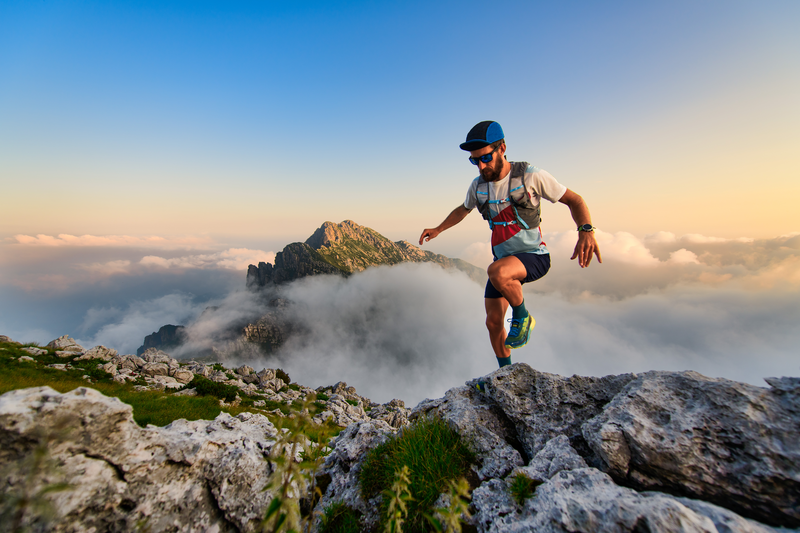
95% of researchers rate our articles as excellent or good
Learn more about the work of our research integrity team to safeguard the quality of each article we publish.
Find out more
ORIGINAL RESEARCH article
Front. Mar. Sci. , 27 January 2023
Sec. Marine Biology
Volume 10 - 2023 | https://doi.org/10.3389/fmars.2023.1116470
This article is part of the Research Topic The Biology and Conservation of Elasmobranchs and Chimaeras View all 12 articles
Introduction: The current Isogomphodon oxyrhynchus (Daggernose Shark) population status Q7points to 99% losses in the last decade due to certain biological traits, site fidelity, and historical high representativeness as bycatch in artisanal fisheries. This species is listed as Critically Endangered (CR), both in the IUCN and the Brazilian Red Lists. Its vulnerability is so high that its recovery potential requires protection from ongoing fishing pressure.
Objective: In this context, this study aimed to evaluate the health status of Daggernose sharks and their ability to cope with allostatic overload in a comparative analysis with other Carcharhinid and Sphyrnid sharks.
Methods: Sharks incidentally caught by the artisanal fleet in the state of Maranhão, on the Brazilian Amazon Coast, were sampled for blood, and serum was used to assess biochemical markers.
Results: The findings indicate significant differences in Daggernose Shark homeostatic capacity for ALP, ALT/GTP, creatinine, lactate, urea, total cholesterol, and triglycerides, pointing to lower health scores and recovery capacity when compared to other Carcharhiniformes inhabiting the same region.
Discussion and conclusions: It is possible that such vulnerability is a result of fisheries-induced evolution, leading to remaining populations with very low chances of fully recovering. Conservation planning is thus urgent, as current legislation based on fishing bans does very little for the species. International collaboration and longterm recovery measures are necessary, including the creation of MPAs specially designed for the species and captive maintenance aiming to monitor health status and carry out breeding attempts.
The Daggernose Shark, Isogomphodon oxyrhynchus is the Carcharhiniformes representative exhibiting one of the narrowest geographic distributions, found only in coastal areas from Trinidad and Tobago and eastern Venezuela to the state of Maranhão, in northern Brazil (Lessa et al., 2016). It is one of the 24 carcharhinid sharks listed as Critically Endangered, displaying severe population declines of up to 99% in the past three generations, placing it as one of the sharks presenting the highest risk for extinction (Pollom et al., 2020). Threats to this species include intensive fishing pressure, mainly as bycatch of artisanal fleets targeting commercial teleost fish (Pollom et al., 2020), and habitat loss (Magris and Barreto, 2010). According to demographic analyses, the species’ resilience to fishing is extremely low, with low genetic variability across extremely fragmented remaining populations (Lessa et al., 2016). Data on physiological vulnerability is non-existent. This is of particular concern, as nothing is known regarding capture survival rates and the potential success of release measures, if implemented.
An increasing interest in the potential effects of fishing-induced evolution on predatory fish has been noted (Enberg et al., 2011), mostly focused on sexual maturation size impacts and the reproductive outcomes of affected populations. Yet, the effects of reduced genetic diversity caused by overfishing on shark physiology remain poorly explored. In a recent review, Hollins et al. (2018) presented a physiological perspective on this topic, focusing on the effects of fisheries-induced evolution on the energy balance, swimming capacity, stress response, and sensory physiology of fishes. Possible outcomes, however, were treated only theoretically, and empirical studies are required to prove these hypotheses. As decreased genetic diversity causes a concomitant decrease in expressed phenotypes, it is plausible to infer that a population strongly affected by fishing will display less plasticity in the face of stressors, whether environmental or anthropogenic. Thus, low genetic diversity adverse effects can, for example, decrease capture resistance and the chances of post-release survival, leading to a cascade of mortality events that may not be reversed unless long-term conservation programs are established.
In this context, the present study aimed to carry out a novel assessment on the physiological profile of Daggernose Sharks incidentally caught off the Maranhão coast, in northern Brazil, focusing on evaluating the systemic health status of one of the remaining Daggernose Shark populations and its responses to capture stress. Furthermore, we also aimed to assess the status of energy stores mobilized in fight-or-flight situations, if the health of Daggernose sharks is compromised in relation to other Carcharhiniformes commonly caught under the same conditions and, finally, if this species is more sensitive to capture stress when compared to other representatives of the same order. Specific serological markers were chosen for this end, based on their shark roles and validation. More specifically, alkaline phosphatase (ALP) and alanine transaminase (ALT) activities were evaluated to test liver integrity and functionality, bilirubin was assessed to evaluate gallbladder function, serum creatinine was determined to evaluate kidney integrity and functionality, and the stress markers lactate, phosphorus, and urea were evaluated to assess potential allostatic overload caused by capture. Lastly, triglycerides and total cholesterol were assessed to test nutritional status.
Five I. oxyrhynchus individuals incidentally captured by the artisanal fleet of the state of Maranhão, in northeastern Brazil, were sampled to assess physiological vulnerability. For comparative purposes, other Carcharhiniform sharks captured by artisanal fleets in the same region were also evaluated (Table 1). Animals were caught with surface longlines in fishing operations lasting about 10 h. Only recently deceased sharks were considered (score 1 for all categories), using a freshness index, considering the following variables: overall gill color (1 for reddish and 0 for pinkish or whitish coloration), ocular retraction level (1 for non-retracted and bright and 0 for retracted and opaque), blood clotting (1 for unclotted and 0 for partially or fully clotted), and rigor-mortis (1 for complete absence and 0 for partial or complete presence). Prior to necropsies, the individuals were measured and sexed and species identification was performed according to Compagno (2001).
Table 1 Data on the shark specimens captured by artisanal fleets in the state of Maranhão, Brazil, evaluated in the present study.
All sharks were caught between August 2018 and May 2019. Blood samples (10 mL) were obtained by caudal venipuncture using an 18G needle attached to a 20 mL disposable syringe and immediately transferred to ultra-pure polypropylene microcentrifuge tubes (2 mL) (Tubes® 3810X, Eppendorf - Hamburg Germany). Samples were centrifuged for 7 min at room temperature (20°C) at 2,000 x g. The sera from all sharks were separated and frozen at - 20°C until analysis. Sampling was approved by the Brazilian Ministry of Environment (IBAMA/ICMBio-SISBIO #60306-1).
Shark sera were used to determine physiological markers indicative of systemic health and stress response upon capture. Dilutions (1:50) with ultrapure water were performed only for urea, according to a previously established protocol (Wosnick et al., 2017). ALP (Labtest – Brazil; catalog n. 40 wave-length 590 nm), ALT (catalog n. 108; wave-length 340 nm), bilirubin (catalog n. 31; wave-length 525 nm), creatinine (catalog n. 35; wave-length 510 nm), lactate (catalog n. 138-1/50; wave-length 550 nm), phosphorus (catalog n. 42; wave-length 650 nm), urea (catalog n. 27; wave-length 600 nm), triglycerides (catalog n. 87; wave-length 505 nm), and total cholesterol (catalog n. 76; wave-length 500 nm) were quantified colorimetrically (Visible UV Spectrophotometer Q898U2M5 Quimis, Brazil). All analyses were carried out following the manufacturer’s instructions and employing previously sterilized material.
A Kruskal-Wallis test with a post hoc Dunn’s test was used to assess serum markers differences among Carcharhinus spp., Sphyrna spp., and I. oxyrhynchus, considered adequate for assessments where different numbers of specimens are compared (López-Vásquez et al., 2009; Subotić et al., 2013; Páez-Rosas et al., 2018; Kehrig et al., 2022). A statistical significance of 0.05 was established for all tests. Analyses were conducted and data were plotted using the SigmaPlot 12 software (Systat Software, San Jose, CA).
Considering systemic health indicators, significant differences were observed for ALP activity, which was higher in I. oxyrhynchus compared to Carcharhinus spp. and Sphyrna spp. (p = 0.002) (Figure 1A). No difference was observed between Carcharhinus spp. and Sphyrna spp. Concerning ALT, significant differences were observed between all groups, higher in I. oxyrhynchus, followed by Carcharhinus spp. and Sphyrna spp. (p = 0.001) (Figure 1B). Regarding bilirubin concentrations, significant differences were observed only between Carcharhinus spp. and Sphyrna spp. (p = 0.004) (Figure 1C). Furthermore, significant differences were observed for creatinine concentrations, which were higher in I. oxyrhynchus compared to Carcharhinus spp. and Sphyrna spp. (p = 0.001) (Figure 1D). No difference was observed between Carcharhinus spp. and Sphyrna spp.
Figure 1 Serum markers indicating organ integrity and functionality. (A) Alkaline Phosphatase (ALP) activities; (B) Alanine transaminase (ALT) activities; (C) Bilirubin concentrations; (D) Creatinine concentrations. The shark groups are indicated in the graph by 1) Carcharhinus spp., 2) Sphyrna spp., and 3) I oxyrhynchus. Statistical differences are represented by lowercase letters.
Concerning stress markers, significant differences were observed for lactate concentrations among all groups (p = 0.001), higher in I. oxyrhynchus, followed by Sphyrna spp. and Carcharhinus spp. (Figure 2A). With regard to phosphorus concentrations, significant differences were observed between I. oxyrhynchus and Carcharhinus spp. (p = 0.001) and between Carcharhinus spp. and Sphyrna spp. (p = 0.002) (Figure 2B). No difference, however, was observed between I. oxyrhynchus and Sphyrna spp., with the highest concentrations observed for both groups. Furthermore, significant differences were observed in urea concentrations for all groups (p = 0.001), lower in I. oxyrhynchus, followed by Carcharhinus spp. The highest concentrations were observed in Sphyrna spp. (Figure 2C).
Figure 2 Secondary stress markers in shark serum. (A) Lactate concentrations; (B) Phosphorus concentrations; (C) Urea concentrations. The shark groups are indicated in the graph by 1) Carcharhinus spp., 2) Sphyrna spp., and 3) I oxyrhynchus. Statistical differences are represented by lowercase letters.
Considering the determined energetic markers, significant differences were observed for total cholesterol, higher in I. oxyrhynchus when compared to Carcharhinus spp. and Sphyrna spp. (p = 0.002) (Figure 3A). No difference, however, was observed between Carcharhinus spp. and Sphyrna spp. Regarding serum triglyceride concentrations, significant differences were observed between all groups (p = 0.001), higher in I. oxyrhynchus, followed by Carcharhinus spp. The lowest concentrations were observed in Sphyrna spp. (Figure 3B).
Figure 3 Energy metabolism indicators. (A) Total cholesterol concentrations; (B) Triglyceride concentrations. The shark groups are indicated in the graph by 1) Carcharhinus spp., 2) Sphyrna spp., and 3) I oxyrhynchus. Statistical differences are represented by lowercase letters.
This is the first investigation of the physiological status of the Critically Endangered I. oxyrhynchus, focused on Maranhão’s remaining population. Considering our first research question, results indicate that the studied Daggernose Shark population exhibits lower health scores when compared to other Carcharhiniformes, evidenced mostly by the higher activities of both ALP and ALT and higher circulating creatinine levels, all indicative of systemic health impairment in vertebrates (Gowda et al., 2010). An increase in enzyme flow to serum may result from increased cellular leakage due to structural damage or increased enzyme synthesis due to pathologies (Brancaccio et al., 2010). Flow rates are generally very expressive when enzyme leakage originates from cellular damage and is closely associated to the severity of tissue damage (Melesse et al., 2011), and are widely deployed as a diagnosis tool. It is important to note that enzyme activity in sharks is very species-specific (Manire et al., 2001), preventing its use as a diagnostic tool for most species, as reference intervals are still lacking. Furthermore, as some enzymes can be expressed by several organs (e.g., ALP), it may be difficult to adequately track their origin and therefore, the causes related to increased activities.
In the case of ALP, in some vertebrates its increase in activity is closely related to hepatic damage (Boyd, 1983; Boone et al., 2005), while in others its origin may be cardiac or from skeletal musculature. Therefore, increased leakage to the circulation may be due not only to hepatic pathologies, but also cardiac impairment (Dasgupta et al., 2001). In the case of elasmobranchs, ALP is also a precursor of tesserae mineralization (Omelon et al., 2014), and for some sharks, its activity is related to kidney integrity (Johnson and Aubin, 2015). Thus, holistic approaches (i.e., the assessment of several enzymes) become increasingly necessary to better understand enzyme dynamics in sharks. Our findings indicate that ALP activities were significantly higher in Daggernose sharks compared to other Carcharhiniformes, potentially indicating lower liver integrity in the studied population. However, as ALP may also increase due to capture stress in sharks (Manire et al., 2001), it is also possible that higher activity in I. oxyrhynchus indicates a lower allostatic overload resilience due to strenuous exercise (i.e., skeletal musculature leakage).
Alanine Aminotransferase (ALT) activity/leakage was also higher in I. oxyrhynchus when compared to other Carcharhiniformes. As ALT expression is higher in vertebrates when liver damage is observed (Center, 2007; Yang et al., 2009) and detected in cases of hepatobiliary impairment in Tiger sharks (Galeocerdo cuvier) (Wosnick et al., 2020), it seems likely that such a pattern may be another indication of poor liver integrity in the studied Daggernose sharks. No data on the effects of capture stress on ALT activity are currently available, leading us to believe that ALT is a promising marker to assess liver health, along with ALP (and other enzymes, whenever possible). However, as ALT exhibited no increase in a liver-damaged Sand Tiger Shark (Carcharias taurus), its sole use should be cautionary (Otway, 2015).
Higher circulating creatinine levels were also observed in I. oxyrhynchus. This marker is often used to assess kidney integrity in vertebrates, and an increase in serum levels may indicate structural damage to this organ due to the inability to properly excrete this compound (Hanedan et al., 2018). However, as creatinine is a byproduct of creatine phosphate activity in muscle (Volfinger et al., 1994), it is also possible that high circulating levels are a result of strenuous exercise during flight or fight behavior upon capture, although previous studies on capture stress have indicated no increase in creatinine levels in sharks (Manire et al., 2001). That being said, it is plausible to infer that kidney function on Daggernose sharks sampled in the present study was also impaired, at least when compared to other Carcharhiniformes caught in the same region.
Taken together, these results point out a higher systemic vulnerability of I. oxyrhynchus, potentially caused by several factors, such as lower genetic diversity, chronic exposure to environmental pollution (Brown et al., 2009), poor diet, and even higher evolutionary specialization (i.e., hammerhead sharks, Gallagher et al., 2014a). Environmental pollution, in particular, may directly decrease organism resilience to other stressors, as it results in significantly decreased immune system responses in many vertebrates, including fish (Watts et al., 2001). In fact, in a study previously performed with sharks in the same region, including I. oxyrhynchus, results pointed to a negative metal bioaccumulation effect on their systemic health (Wosnick et al., 2021a) which, combined with fishing pressure, may explain the poor health status of the studied population. Thus, future studies on genetic structure, ecotoxicology, and molecular biology are necessary to better understand the underlying factors that are affecting the health and potentially the fitness of Daggernose sharks not only in the state of Maranhão, but among other fragmented populations. Such data is, in fact, imperative, as conservation planning is based on population viability, and health assessments are crucial to determine the chances a population has to thrive.
Based on our second research question, results also indicate that I. oxyrhynchus is more vulnerable to mortality due to capture stress than other Carcharhiniformes. To date, hammerhead sharks are considered the most sensitive to the negative effects of capture, exhibiting consistently high stress marker levels coupled with the highest mortality rates among studied sharks (Gallagher et al., 2014b; Butcher et al., 2015; Gulak et al., 2015; Jerome et al., 2018). It is believed that their evolutionary history is in part responsible for such vulnerability, as their extreme morphological alterations (i.e., cephalofoil) make them less resistant to stressors (Gallagher et al., 2014a). For this reason, Sphyrinid sharks were used in the present study, aiming to investigate if I. oxyrhynchus is as sensitive as hammerhead sharks. In fact, the results indicate similar, if not higher, vulnerability considering the employed stress markers.
In this regard, circulating lactate levels were higher in Daggernose sharks when compared to both Carcharhinus spp. and Sphyrna spp., although concentrations were also very elevated in the latter, consistent with previous studies (Gallagher et al., 2014b; Jerome et al., 2018). Lactate is the most reliable stress marker for elasmobranchs (Skomal and Mandelman, 2012), being consistently high in stressed animals as a byproduct of anaerobic metabolism due to strenuous exercise (Skomal and Bernal, 2010; Wosnick et al., 2017; Jerome et al., 2018). Lactate concentrations are very species-specific, and post-mortem data offers a more reliable picture than traditional reference intervals, as it is crucial to establish which values are lethal/non-recoverable rather than which values represent a “non-stressed animal” (Wosnick et al., 2017). Lactate levels in I. oxyrhynchus were up to 30 mmol L-1, indicating that putative control for Daggernose sharks should not exceed 70% of established lethal concentrations in order to ensure adequate recovery. However, lactic acidosis can be reverted by activating other compensatory mechanisms, such as ethanol conversion and carbonic anhydrase compensation (Shoubridge and Hochachka, 1980; Aspatwar et al., 2022). Thus, it is possible that individuals exhibiting higher lactate levels are still able to recover, indicating the need for further studies on other physiological parameters, including serum pH, pCO2, and carbonic anhydrase activity, to better understand how Daggernose sharks respond to systemic lactic acidosis and if the concentrations established in the present study are, in fact, always lethal.
Interestingly, phosphorus concentrations were not significantly different between I. oxyrhynchus and Sphyrna spp., in both cases very elevated when compared to Carcharhinid sharks. As phosphorus is a predominantly intracellular ion, increased extracellular concentrations indicate cell disruption, leading to excessive leakage (D’Arcy, 2019). In this context, as elasmobranch mortality due to fishing is mainly caused by flight or fight responses, biomarkers indicative of cell rupture/damage have been proven reliable (Wosnick et al., 2017; Wosnick et al., 2021a), always increasing circulating levels upon allostatic overload, as in the case of lactate. That being said, our results indicate that I. oxyrhynchus exhibits similar vulnerability to capture stress when compared to the well-known vulnerable hammerhead sharks, configuring another shark species heavily affected by fishing with little chances of post-release survival. Such a pattern is problematic, as captures are mostly incidental when targeting other fishes (Lessa et al., 2016), so compensatory release might not be the best strategy to reduce bycatch mortality as proposed for several elasmobranchs in both IPOA-sharks and the Brazilian NPOA (Pan-Tubarões).
In the present study, urea concentrations were significantly lower in I. oxyrhynchus, which may be related to their ability to make incursions in more dilute waters or even freshwater systems (Ballantyne and Robinson, 2010; Feitosa et al., 2019). As euryhalinity seems to be more common than traditionally proposed (Wosnick and Freire, 2013), records of individuals caught in different salinities alongside the lower urea concentrations observed in the present study are strong evidence that this is the case for Daggernose sharks. Therefore, the use of urea as an allostatic overload indicator should be cautionary. It is also important to consider the potential effects of such plasticity on stress responses, as euryhaline elasmobranchs may respond differently depending on environmental salinity upon capture. It seems that environmental conditions may influence capture mortality, as in the case of reports of artisanal fishers capturing live Daggernose sharks and releasing them very responsive in higher freshwater input regions (personal communication, N. Wosnick), indicating a better ability to deal with allostatic overload under these conditions. However, studies are required to further investigate this influence, aiming to generate data that might benefit conservation measures based on the higher efficiency of compensatory release, for instance.
As for our third research question, results indicate higher metabolite mobilization in I. oxyrhynchus when compared to the other evaluated Carcharhiniforms. While triglycerides are mobilized during exercise, increasing survival chances (Ballantyne, 1997; Wang et al., 2018), total cholesterol levels may increase in serum as a result of energy mobilization or cellular rupture/membrane denaturation (Durstine et al., 1983; Brett et al., 2000; Fines et al., 2001). Thus, it is necessary to evaluate both metabolites when accessing the effects of capture stress on energy mobilization. For example, upon stress, when triglycerides levels are low and cholesterol levels are high, it is plausible to infer that dynamics are being dictated by cellular rupture/membrane denaturation (Leite et al., in prep.). When both are observed at high circulating levels, it is more likely that concentrations are being dictated by adaptive mechanisms, as observed in vertebrates that can cope with stressful situations (Pickering and Pottinger, 1995). As stated by Romero and Beattie (2022), higher circulating metabolite levels indicate a higher capacity/adaptative response to cope with stressors. Based on such a premise, our results indicate that Daggernose sharks are energetically capable of dealing with allostatic overload, although such a response is not enough to ensure their survival, as some physiological impairments (e.g., systemic acidosis) cannot be reserved through energy mobilization itself. Furthermore, we cannot rule out the potential effects that lower health scores might have on metabolite mobilization, as hepatic impairments have the potential to alter metabolites dynamics, leading to higher circulating levels of both analyzed markers (Boyd, 1983; Wosnick et al., 2020). Hepatic damage may also result in decreased environmental contaminant metabolization and subsequent excretion, as the liver is the main detoxifying organ in vertebrates (Yao et al., 2019). This, in turn, leads to higher circulating pollutant levels and significant deleterious physiological effects, including oxidative stress, altered hepatocellular lipid metabolism, citotoxicity and, potentially, genotoxicity which have been reported for many taxonomic groups (Hui et al., 2017; Gabriel et al., 2020; de Farias Araujo et al., 2022), including sharks (Hauser-Davis et al., 2021; Wosnick et al., 2021a), potentially affecting animal health conditions due to altered physiological, systemic and, ultimately, behavioral aspects (Wosnick et al., 2021b; Willmer et al., 2022; Wosnick et al., 2022).
Taken together, our results indicate that the systemic health of the Daggernose shark studied population is impaired when compared to other Carcharhiniformes that inhabit the same region. In general, poor health conditions are related to low-quality prey, environmental pollution, or lower plasticity due to genetic limitations, including very fragmented populations and low gene flow (Neff et al., 2011; Murray et al., 2015; Sueiro et al., 2020). Exposure to constant stressors can also compromise population health (Stott, 1981), which becomes a significant concern under the current climate change scenario, as this shifting condition is increasingly affecting aquatic ecosystems, resulting in altered water mass flows and the transport patterns of and consequent exposure to a myriad of environmental contaminants, such as metals and persistent organic pollutants (Teran et al., 2012; Hauser-Davis and Wosnick, 2022). Furthermore, certain climate change effects, such as higher temperatures in all environmental compartments (i.e., water, soil, atmosphere) and ocean acidification have been indicated as significantly increasing pollutant bioavailability and toxicity (Ficke et al., 2007; Hauser-Davis and Wosnick, 2022) for many vertebrates, including fish. In this regard, environmental contaminant level effect assessments in elasmobranchs, such as different biochemical response assessments (i.e., protein determinations, immune response assessments and gene expression evaluations) in particular, have been recommended in a multifaceted approach to better understand whole organism/population fitness and responses to stressors in this group (Skomal and Mandelman, 2012). In addition, the physiology of several species is bound to be affected by climate change effects, with several authors indicating that the most probable response will comprise altered migration shifts, both in timing and established routes, and geographic distributions (Field et al., 2009), in turn making organisms more vulnerable, significantly aggravating ecological risks in a continuous cause-and-effect cycle.
In the case of the sharks evaluated herein, prey availability seems not the case, nor differentiated pollution gradients, as all assessed species share the same habit and are part of the same trophic chain. Although species-specific differences cannot be disregarded, the phylogenetic proximity among the sharks evaluated in the present study leads us to believe that the lower health condition of I. oxyrhynchus might be a result of low genetic diversity, leading to poorer physiological plasticity as a negative outcome of fisheries-induced evolution.
The same was observed for the Daggernose Shark capacity to overcome allostatic overload, indicating that their ability to cope with stress is now severely compromised. In fact, stress markers were as elevated as those observed in hammerheads, considered the most physiologically vulnerable sharks. Once again, low genetic diversity leading to limited physiological plasticity as a result of fisheries-induced evolution might explain the observed vulnerability. Of course, intrinsic lower species-specific capacity to cope with stressors cannot be ruled out, and further studies employing other markers along with population genetics investigations are necessary to shed light on how much fisheries-induced evolution may be affecting the remaining Daggernose sharks. Furthering our knowledge on the adverse effects of pollution in this species is paramount, to better understand how its survival/ability to cope with other stressors are being affected. Pathology investigations are also urgent, aiming to evaluate Daggernose shark health status as a whole.
Some conservation and fisheries management aspects must also be considered. First, no conservation planning has been developed for the Daggernose Shark to date. Although this species is highlighted in the Brazilian NPOA and specific fishing regulations prohibit their capture, transport, and commercialization, there are no management plans that consider the negative consequences related to mortality due to commercial capture, or the overall health of the remaining populations. In fact, fishing bans do very little for Daggernose sharks, as most current captures are incidental, and even if release is adopted as a mitigating measure, the chances of survival appear to be low, resulting in little or no efficiency. Unfortunately, it is likely that the chances of reversing the current situation of this species are low, or practically nil, especially considering the low genetic diversity of the remaining populations coupled with their physiological vulnerability described herein. Thus, international efforts and long-term recovery programs are urgent. More specifically, it is possible that the only chances of the species rely on captive maintenance, which is a challenge for most shark species. However, this should not hinder joint efforts, not only to more effectively protect free-ranging individuals (e.g., Marine Protected Areas and Sanctuaries), but also to direct individuals to institutions that can maintain them under adequate human care and perform long term health assessments, aiming to monitor the real status of remaining individuals, as well as to advance assisted reproduction attempts.
The raw data supporting the conclusions of this article will be made available by the authors, without undue reservation.
Ethical review and approval was not required for the animal study because Only dead animals from commercial fishing were used, requiring only permission from the Brazilian government to collect samples (IBAMA/ICMBio-SISBIO #60306-1).
Conceptualization – NW. Data curation – NW, AC, HD, AN. Formal analysis – NW and AC. Funding acquisition – JN. Investigation – NW. Methodology – NW, AC, RH-D. Writing - original draft – NW and RH-D Writing - review & editing – NW, RH-D, AC, HD, AN, JN. All authors contributed to the article and approved the submitted version.
Financial support to NW and JN through the Fundação de Amparo à Pesquisa do Maranhão (FAPEMA - BEPP-02106/18; BPD-04215/17; AQUIPESCA-06605/16) and the Coordenação de Aperfeiçoamento de Pessoal de Nível Superior (CAPES) through a Post-doctoral Fellowship. NW also thanks Pedro Calixto for help with statistics.
The authors declare that the research was conducted in the absence of any commercial or financial relationships that could be construed as a potential conflict of interest.
All claims expressed in this article are solely those of the authors and do not necessarily represent those of their affiliated organizations, or those of the publisher, the editors and the reviewers. Any product that may be evaluated in this article, or claim that may be made by its manufacturer, is not guaranteed or endorsed by the publisher.
Aspatwar A., Tolvanen M. E., Barker H., Syrjänen L., Valanne S., Purmonen S., et al. (2022). Carbonic anhydrases in metazoan model organisms: molecules, mechanisms, and physiology. Physiol. Rev. 102 (3), 1327–1383. doi: 10.1152/physrev.00018.2021
Ballantyne J. S. (1997). Jaws: the inside story. The metabolism of elasmobranch fishes. Comp. Biochem. Physiol. B, Biochem. Mol. Biol. 118(4), 703–742. doi: 10.1016/S0305-0491(97)00272-1
Ballantyne J. S., Robinson J. W. (2010). Freshwater elasmobranchs: a review of their physiology and biochemistry. J. Comp. Physiol. B. 180 (4), 475–493. doi: 10.1007/s00360-010-0447-0
Boone L., Meyer D., Cusick P., Ennulat D., Bolliger A. P., Everds N., et al. (2005). Selection and interpretation of clinical pathology indicators of hepatic injury in preclinical studies. Vet. Clin. Pathol. 34 (3), 182–188. doi: 10.1111/j.1939-165x.2005.tb00041.x
Boyd J. W. (1983). The mechanisms relating to increases in plasma enzymes and isoenzymes in diseases of animals. Vet. Clin. Pathol. 12 (2), 9–24. doi: 10.1111/j.1939-165x.1983.tb00609.x
Brancaccio P., Lippi G., Maffulli N. (2010). Biochemical markers of muscular damage. Clin. Chem. Lab. Med. 48 (6), 757–767. doi: 10.1515/CCLM.2010.179
Brett S. E., Ritter J. M., Chowienczyk P. J. (2000). Diastolic blood pressure changes during exercise positively correlate with serum cholesterol and insulin resistance. Circulation 101 (6), 611–615. doi: 10.1161/01.cir.101.6.611
Brown A. R., Hosken D. J., Balloux F., Bickley L. K., LePage G., Owen S. F., et al. (2009). Genetic variation, inbreeding and chemical exposure–combined effects in wildlife and critical considerations for ecotoxicology. Philos. Trans. R. Soc. Lond. B. Biol. Sci. 364 (1534), 3377–3390. doi: 10.1098/rstb.2009.0126
Butcher P. A., Peddemors V. M., Mandelman J. W., McGrath S. P., Cullis B. R. (2015). At-Vessel mortality and blood biochemical status of elasmobranchs caught in an Australian commercial longline fishery. Glob. Ecol. Conserv. 3, 878–889. doi: 10.1016/J.GECCO.2015.04.012
Center S. A. (2007). Interpretation of liver enzymes. Vet. Clin. North. Am. Small. Anim. Prac. 37 (2), 297–333. doi: 10.1016/j.cvsm.2006.11.009
Compagno L. J. (2001). Sharks of the world: an annotated and illustrated catalogue of shark species known to date. Food & Agriculture Org. (Vol. 2).
D’Arcy M. S. (2019). Cell death: a review of the major forms of apoptosis, necrosis and autophagy. Cell. Biol. Int. 43 (6), 582–592. doi: 10.1002/cbin.11137
Dasgupta A., Chow L., Wells A., Datta P. (2001). Effect of elevated concentration of alkaline phosphatase on cardiac troponin I assays. J. Clin. Lab. Anal. 15 (4), 175–177. doi: 10.1002/jcla.1023
de Farias Araujo G., Soares L. O. S., Junior S. F. S., de Carvalho L. V. B., Rocha R. C. C., Saint’Pierre T., et al. (2022). Oxidative stress and metal homeostasis alterations in Danio rerio (zebrafish) under single and combined carbamazepine, acetamiprid and cadmium exposures. Aquat. Toxicol. 245, 106122. doi: 10.1016/j.aquatox.2022.106122
Durstine J. L., Miller W., Farrell S., Sherman W. M., Ivy J. L. (1983). Increases in HDL-cholesterol and the HDLLDL cholesterol ratio during prolonged endurance exercise. Metabolism 32 (10), 993–997. doi: 10.1016/0026-0495(83)90141-5
Enberg K., Jørgensen C., Dunlop E. S., Varpe Ø., Boukal D. S., Baulier L., et al. (2011). Fishing-induced evolution of growth: concepts, mechanisms and the empirical evidence. Mar. Ecol. 33 (1), 1–25. doi: 10.1111/j.1439-0485.2011.00460.x
Feitosa L. M., Martins A. P. B., Lessa R. P. T., Barbieri R., Nunes J. L. S. (2019). Daggernose shark: An elusive species from northern south America. Fisheries 44 (3), 144–147. doi: 10.1002/fsh.10205
Ficke A. D., Myrick C. A., Hansen L. J. (2007). Potential impacts of global climate change on freshwater fisheries. Rev. Fish. Biol. Fish. 17, 581–613. doi: 10.1007/s11160-007-9059-5
Field I. C., Meekan M. G., Buckworth R. C., Bradshaw C. J. (2009). Susceptibility of sharks, rays and chimaeras to global extinction. Adv. Mar. Biol. 56, 275–363. doi: 10.1016/S0065-2881(09)56004-X
Fines G. A., Ballantyne J. S., Wright P. A. (2001). Active urea transport and an unusual basolateral membrane composition in the gills of a marine elasmobranch. Am. J. Physiol. Regul. Integr. Comp. Physiol. 280 (1), R16–R24. doi: 10.1152/ajpregu.2001.280.1.R16
Gabriel F.Â., Hauser-Davis R. A., Soares L., Mazzuco A. C. A., Rocha R. C. C., Saint Pierre T. D., et al. (2020). Contamination and oxidative stress biomarkers in estuarine fish following a mine tailing disaster. PeerJ. 8, e10266. doi: 10.7717/peerj.10266
Gallagher A. J., Hammerschlag N., Shiffman D. S., Giery S. T. (2014a). Evolved for extinction: the cost and conservation implications of specialization in hammerhead sharks. BioScience 64 (7), 619–624. doi: 10.1093/biosci/biu071
Gallagher A. J., Serafy J. E., Cooke S. J., Hammerschlag N. (2014b). Physiological stress response, reflex impairment, and survival of five sympatric shark species following experimental capture and release. Mar. Ecol. Prog. Ser. 496, 207–218. doi: 10.3354/meps10490
Gowda S., Desai P. B., Kulkarni S. S., Hull V. V., Math A. A., Vernekar S. N. (2010). Markers of renal function tests. N. Am. J. Med. Sci. 2 (4), 170.
Gulak S. J. B., de Ron Santiago A. J., Carlson J. K. (2015). Hooking mortality of scalloped hammerhead Sphyrna lewini and great hammerhead Sphyrna mokarran sharks caught on bottom longlines. Afr. J. Mar. Sci. 37 (2), 267–273. doi: 10.2989/1814232X.2015.1026842
Hanedan B., Ozkaraca M., Kirbas A., Kandemir F. M., Aktas M. S., Kilic K., et al. (2018). Investigation of the effects of hesperidin and chrysin on renal injury induced by colistin in rats. Biomed. Pharmacother. 108, 1607–1616. doi: 10.1016/j.biopha.2018.10.001
Hauser-Davis R. A., Rocha R. C. C., Saint’Pierre T. D., Adams D. H. (2021). Metal concentrations and metallothionein metal detoxification in blue sharks, Prionace glauca l. West. North Atl. Ocean. J. Trace Elem. Med. Biol. 68, 126813. doi: 10.1016/j.jtemb.2021.126813
Hauser-Davis R. A., Wosnick N. (2022). Climate change implications for metal and metalloid dynamics in aquatic ecosystems and its context within the decade of ocean sciences. Water 14 (15), 2415. doi: 10.3390/w14152415
Hollins J., Thambithurai D., Koeck B., Crespel A., Bailey D. M., Cooke S. J., et al. (2018). A physiological perspective on fisheries-induced evolution. Evol. Appl. 11 (5), 561–576. doi: 10.1111/eva.12597
Hui Z., Li R., Chen L. (2017). The impact of exposure to environmental contaminant on hepatocellular lipid metabolism. Gene 622, 67–71. doi: 10.1016/j.gene.2017.04.024
Jerome J. M., Gallagher A. J., Cooke S. J., Hammerschlag N. (2018). Integrating reflexes with physiological measures to evaluate coastal shark stress response to capture. ICES J. Mar. Sci. 75 (2), 796–804. doi: 10.1093/icesjms/fsx191
Johnson M., Aubin D. (2015)Tissue distribution and serum levels of selected enzymes in five species of sharks: Sphyrna lewini, prionace glauca, carcharhinus taurus, c. plumbeus and isurus oxyrinchus. In: IAAAM proceedings. Available at: https://www.vin.com/doc/?id=6696240 (Accessed August 5, 2022).
Kehrig H. A., Hauser-Davis R. A., Muelbert M. M., Almeida M. G., Di Beneditto A. P. M., Rezende C. E. (2022). Mercury and stable carbon and nitrogen isotopes in the natal fur of two Antarctic pinniped species. Chemosphere 288, 132500. doi: 10.1016/j.chemosphere.2021.132500
Lessa R., Batista V. S., Santana F. M. (2016). Close to extinction? the collapse of the endemic daggernose shark (Isogomphodon oxyrhynchus) off Brazil. Glob. Ecol. Conserv. 7, 70–81. doi: 10.1016/j.gecco.2016.04.003
López-Vásquez K., Castro-Pérez C. A., Val A. L. (2009). Digestive enzymes of eight Amazonian teleosts with different feeding habits. J. Fish Biol. 74 (7), 1620–1628. doi: 10.1111/j.1095-8649.2009.02196.x
Magris R. A., Barreto R. (2010). Mapping and assessment of protection of mangrove habitats in Brazil. Pan-Am. J. Aquat. 5 (4), 546–556.
Manire C., Hueter R., Hull E., Spieler R. (2001). Serological changes associated with gill-net capture and restraint in three species of sharks. Trans. Am. Fish. Soc 130 (6), 1038–1048. doi: 10.1577/1548-8659(2001)130<1038:SCAWGN>2.0.CO;2
Melesse A., Maak S., Schmidt R., Von Lengerken G. (2011). Effect of long-term heat stress on key enzyme activities and T3 levels in commercial layer hens. Int. J. Livest. Prod 2 (7), 107–116. doi: 10.1002/jcla.1023
Murray M., Edwards M. A., Abercrombie B., St. Clair C. C. (2015). Poor health is associated with use of anthropogenic resources in an urban carnivore. Proc. R. Soc B. 282 (1806), 20150009. doi: 10.1098/rspb.2015.0009
Neff B. D., Garner S. R., Pitcher T. E. (2011). Conservation and enhancement of wild fish populations: preserving genetic quality versus genetic diversity. Can. J. Fish. Aquat. Sci. 68 (6), 1139–1154. doi: 10.1139/f2011-029
Omelon S., Georgiou J., Variola F., Dean M. N. (2014). Colocation and role of polyphosphates and alkaline phosphatase in apatite biomineralization of elasmobranch tesserae. Acta Biomater. 10 (9), 3899–3910. doi: 10.1016/j.actbio.2014.06.008
Otway N. M. (2015). Serum biochemical reference intervals for free-living sand tiger sharks (Carcharias taurus) from east Australian waters. Vet. Clin. Pathol. 44 (2), 262–274. doi: 10.1111/vcp.12254
Páez-Rosas D., Insuasti-Zarate P., Riofrío-Lazo M., Galván-Magaña F. (2018). Feeding behavior and trophic interaction of three shark species in the Galapagos marine reserve. PeerJ. 6, e4818. doi: 10.7717/peerj.4818
Pickering A. D., Pottinger T. G. (1995). “Biochemical effects of stress,” in Biochemistry and molecular biology of fishes. Eds. Hochachka P. W., Mommsen T. P. (Amsterdam: Elsevier), 349–379.
Pollom R., Charvet P., Faria V., Herman K., Lasso-Alcalá O., Marcante F., et al. (2020). Isogomphodon oxyrhynchus. IUCN Red List Threatened Species, 2020 T60218A3094144. doi: 10.2305/IUCN.UK.2020-3.RLTS.T60218A3094144.en
Romero L. M., Beattie U. K. (2022). Common myths of glucocorticoid function in ecology and conservation. J. Exp. Zool. A: Ecol. Integr. Physiol. 337 (1), 7–14. doi: 10.1002/jez.2459
Shoubridge E. A., Hochachka P. W. (1980). Ethanol: Novel end product of vertebrate anaerobic metabolism. Science 209, 308–309. doi: 10.1126/science.7384807
Skomal G., Bernal D. (2010). “Physiological responses to stress in sharks,” in Sharks and their relatives II: Biodiversity, adaptive physiology, and conservation. Eds. Carrier. J. C., Musick. J. A., Heithaus M. R. (Boca Raton: CRC Press), 457–488.
Skomal G. B., Mandelman J. W. (2012). The physiological response to anthropogenic stressors in marine elasmobranch fishes: a review with a focus on the secondary response. Comp. Biochem. Physiol. Part A Mol. Integr. 162 (2), 146–155. doi: 10.1016/j.cbpa.2011.10.002
Stott G. H. (1981). What is animal stress and how is it measured? J. Anim. Sci. 52 (1), 150–153. doi: 10.2527/jas1981.521150x
Subotić S., Spasić S., Višnjić-Jeftić Ž., Hegediš A., Krpo-Ćetković J., Mićković B., et al. (2013). Heavy metal and trace element bioaccumulation in target tissues of four edible fish species from the Danube river (Serbia). Ecotoxicol. Environ. Saf. 98, 196–202. doi: 10.1016/j.ecoenv.2013.08.020
Sueiro M. C., Awruch C., Gilardoni C., Demetrio M., Palacios M. G. (2020). Immunity and health of two wild marine fishes naturally exposed to anthropogenic pollution. Sci. Total Environ. 726, 138303. doi: 10.1016/j.scitotenv.2020.138303
Teran T., Lamon L., Marcomini A. (2012). Climate change effects on POPs’ environmental behaviour: a scientific perspective for future regulatory actions. Atmos. pollut. Res. 3 (4), 466–476. doi: 10.5094/APR.2012.054
Volfinger L., Lassourd V., Michaux J. M., Braun J. P., Toutain P. L. (1994). Kinetic evaluation of muscle damage during exercise by calculation of amount of creatine kinase released. Am. J. Physiol. Regul. Integr. Comp. Physiol. 266 (2), R434–R441. doi: 10.1152/ajpregu.1994.266.2.R434
Wang Y., Liu Z., Han Y., Xu J., Huang W., Li Z. (2018). Medium chain triglycerides enhances exercise endurance through the increased mitochondrial biogenesis and metabolism. PloS One 13 (2), e0191182. doi: 10.1371/journal.pone.0191182
Watts M., Munday B. L., Burke C. M. (2001). Immune responses of teleost fish. Aust. Vet. J. 79 (8), 570–574. doi: 10.1111/j.1751-0813.2001.tb10753.x
Willmer I. Q., Wosnick N., Rocha R. C. C., Saint’Pierre T. D., Vianna M., Hauser-Davis R. A. (2022). First report on metal and metalloid contamination of ampullae of lorenzini in sharks: A case study employing the Brazilian sharpnose shark Rhizoprionodon lalandii from southeastern Brazil as an ecotoxicological model. Mar. Pol. Bull. 179, 113671. doi: 10.1016/j.marpolbul.2022.113671
Wosnick N., Bornatowski H., Ferraz C., Afonso A., Sousa Rangel B., Hazin F. H. V., et al. (2017). Talking to the dead: using post-mortem data in the assessment of stress in tiger sharks (Galeocerdo cuvier)(Péron and lesueur 1822). Fish Physiol. Biochem. 43 (1), 165–178. doi: 10.1007/s10695-016-0276-5
Wosnick N., Chaves A. P., Leite R. D., Nunes J. L. S., Saint’Pierre T. D., Willmer I. Q., et al. (2021b). Nurse sharks, space rockets and cargo ships: Metals and oxidative stress in a benthic, resident and large-sized mesopredator, Ginglymostoma cirratum. Env. Pol. 288, 117784. doi: 10.1016/j.envpol.2021.117784
Wosnick N., Chaves A. P., Niella Y. V., Takatsuka V., Hazin F. H. V., Nunes J. L. S., et al. (2020). Physiological impairment as a result of bile accumulation in an apex predator, the tiger shark (Galeocerdo cuvier péron & lesueur 1822). Animals 10 (11), 2030. doi: 10.3390/ani10112030
Wosnick N., Freire C. A. (2013). Some euryhalinity may be more common than expected in marine elasmobranchs: The example of the south American skate Zapteryx brevirostris (Elasmobranchii, rajiformes, rhinobatidae). Comp. Biochem. Physiol. Part A Mol. Integr. 166 (1), 36–43. doi: 10.1016/j.cbpa.2013.05.002
Wosnick N., Leite R. D., Giareta E. P., Morick D., Hauser-Davis R. A. (2022). Unraveling metabolite provisioning to offspring through parental fluids: A case study of the Brazilian guitarfish, Pseudobatos horkelii. Front. Physiol. 13, 1123. doi: 10.2305/IUCN.UK.2020-3.RLTS.T60218A3094144.en
Wosnick N., Niella Y., Hammerschlag N., Chaves A. P., Hauser-Davis R. A., da Rocha R. C. C., et al. (2021a). Negative metal bioaccumulation impacts on systemic shark health and homeostatic balance. Mar. Pol. Bull. 168, 112398. doi: 10.1016/j.marpolbul.2021.112398
Yang R. Z., Park S., Reagan W. J., Goldstein R., Zhong S., Lawton M., et al. (2009). Alanine aminotransferase isoenzymes: molecular cloning and quantitative analysis of tissue expression in rats and serum elevation in liver toxicity. Hepatology 49 (2), 598–607. doi: 10.1002/hep.22657
Keywords: conservation physiology, Carcharhinidae, Sphyrnidae, capture stress, fisheries management
Citation: Wosnick N, Chaves AP, Dias HN, Onodera Palmeira Nunes AR, Nunes JLS and Hauser-Davis RA (2023) Assessment of the physiological vulnerability of the endemic and critically endangered Daggernose Shark: A comparative approach to other Carcharhiniformes. Front. Mar. Sci. 10:1116470. doi: 10.3389/fmars.2023.1116470
Received: 05 December 2022; Accepted: 18 January 2023;
Published: 27 January 2023.
Edited by:
Elizabeth Grace Tunka Bengil, University of Kyrenia, CyprusReviewed by:
Annalisa Zaccaroni, University of Bologna, ItalyCopyright © 2023 Wosnick, Chaves, Dias, Onodera Palmeira Nunes, Nunes and Hauser-Davis. This is an open-access article distributed under the terms of the Creative Commons Attribution License (CC BY). The use, distribution or reproduction in other forums is permitted, provided the original author(s) and the copyright owner(s) are credited and that the original publication in this journal is cited, in accordance with accepted academic practice. No use, distribution or reproduction is permitted which does not comply with these terms.
*Correspondence: Natascha Wosnick, bi53b3NuaWNrQGdtYWlsLmNvbQ==; Rachel Ann Hauser-Davis, cmFjaGVsLmhhdXNlci5kYXZpc0BnbWFpbC5jb20=
Disclaimer: All claims expressed in this article are solely those of the authors and do not necessarily represent those of their affiliated organizations, or those of the publisher, the editors and the reviewers. Any product that may be evaluated in this article or claim that may be made by its manufacturer is not guaranteed or endorsed by the publisher.
Research integrity at Frontiers
Learn more about the work of our research integrity team to safeguard the quality of each article we publish.