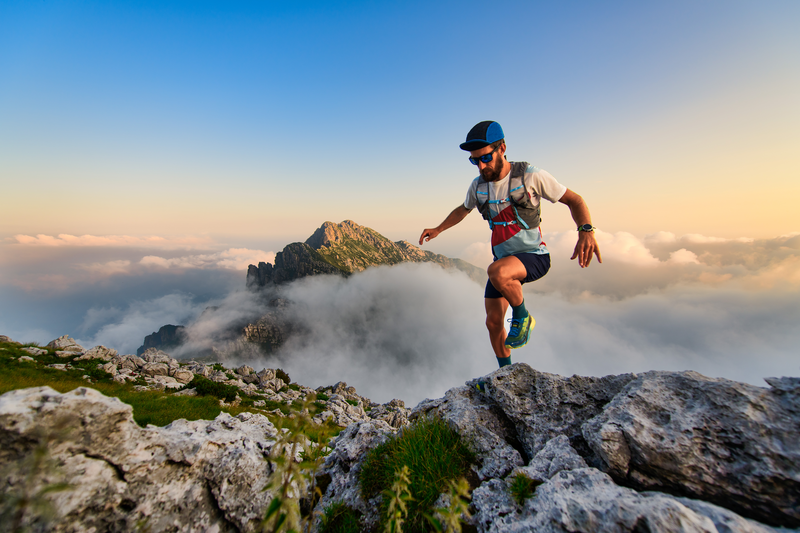
95% of researchers rate our articles as excellent or good
Learn more about the work of our research integrity team to safeguard the quality of each article we publish.
Find out more
ORIGINAL RESEARCH article
Front. Sustain. Food Syst. , 19 March 2025
Sec. Waste Management in Agroecosystems
Volume 9 - 2025 | https://doi.org/10.3389/fsufs.2025.1556396
This article is part of the Research Topic Agri-Food Waste Utilization for Sustainable Future: Challenges and Opportunities View all 10 articles
Introduction: Rice straw burning is a major environmental concern in India, contributing to severe air pollution and climate change. Sustainable alternatives for its utilization are essential to mitigate these issues. One promising approach is repurposing rice straw as a growing medium for microgreens, addressing both agricultural waste management and the need for sustainable substrates. This study evaluates the feasibility of using pretreated rice straw as a substrate for microgreen cultivation and compares its performance with conventional substrates like cocopeat and vermiculite.
Methods: Rice straw was pretreated with sodium hydroxide (NaOH) at varying concentrations (1%–7%) to improve its physicochemical properties. Changes in ash content, water holding capacity (WHC), and suitability as a growing medium were assessed. Microgreens of amaranth, cabbage, and cauliflower were cultivated on pretreated rice straw, cocopeat, and vermiculite. Growth parameters, including plant length and fresh weight, were recorded. Additionally, the micronutrient content (Fe, Zn, Mn) of plants grown in different substrates was analyzed. Principal Component Analysis (PCA) was conducted to identify key factors influencing plant growth and nutrient uptake.
Results: NaOH pretreatment significantly improved the quality of rice straw, reducing ash content from 20.05% (untreated) to 7.10%–11.10% and increasing WHC to 673%, which was comparable to cocopeat (891%) and superior to vermiculite (389%). Among the microgreens tested, cabbage achieved the highest plant length (6.2 cm) and fresh weight (59.83 mg plant−1) when grown on rice straw. While vermiculite supported higher levels of essential micronutrients, including Fe, Zn, and Mn, rice straw demonstrated remarkable performance in plant growth and biomass production. PCA revealed that rice straw was positively associated with plant weight and length, whereas vermiculite correlated with micronutrient accumulation.
Discussion: The findings highlight the potential of pretreated rice straw as an effective alternative to conventional growing media. Despite vermiculite providing higher micronutrient availability, rice straw supported robust plant growth, making it a viable substrate for microgreen cultivation. Further fortification of rice straw could enhance its nutrient-holding capacity, making it even more comparable to existing commercial substrates. This study demonstrates that utilizing rice straw as a growing medium offers an eco-friendly, cost-effective solution for sustainable agriculture while reducing environmental pollution caused by straw burning.
Rice (oryza sativa) straw burning is a significant environmental concern in India, particularly in agriculturally intensive states like Punjab, Haryana, and Uttar Pradesh (Parihar et al., 2023). This practice is widely adopted to quickly clear fields for the subsequent wheat crop, due to the short time interval between rice harvest and wheat planting. However, while efficient for field clearance, it has severe environmental and health repercussions. Burning rice straw releases large quantities of greenhouse gases, such as carbon dioxide (CO2), methane (CH4), and nitrous oxide (N2O). One ton of rice straw burning emits 1,460 kg of CO2, 60 kg CO, 5.7 kg of CH4, and 0.07 kg of N2O (Singh et al., 2017), contributing significantly to air pollution and climate change. In Punjab alone, 23 million tons of rice straw are burned annually, exacerbating atmospheric pollution (Asfar et al., 2021).
Addressing this problem requires sustainable alternatives to rice straw burning, and one promising solution is repurposing rice straw for microgreen production. Microgreens, the young and tender seedlings of various vegetables and herbs, have gained substantial popularity in recent years due to their intense flavours, vibrant colours, and high nutritional value. These miniature greens are typically harvested when the first true leaves have emerged, usually within 7–21 days after germination (Ghoora et al., 2020). Studies have shown that microgreens contain higher concentrations of vitamins, minerals and antioxidants compared to their mature counterparts, making them a valuable addition to the human diet (Bhaswant et al., 2023). With the growing demand for these nutrient-dense plants, there is an increasing interest in developing sustainable and efficient methods for their cultivation. One significant challenge in microgreen production is finding a suitable growing medium. Traditional substrates such as soil, peat moss, coco peat, perlite and vermiculite are commonly used, but they come with various drawbacks. Soil, cocopeat and peat moss can harbour pathogens and pests, requiring sterilization or treatment, which adds to the production cost and labour (Hoang and Vu, 2022). Vermiculite, although sterile and lightweight, is a mined product, raising concerns about its sustainability and environmental impact.
In this context, agricultural byproducts such as rice straw present a promising alternative. Rice straw is the dry stalk left after the harvesting of rice grains. It is an abundant and renewable resource, especially in rice-producing countries like India, China, and Japan. However, rice straw is often considered waste and is either burned or left to decompose in fields, leading to environmental pollution and waste management issues in India. Utilizing rice straw as a growing medium for microgreens could offer a sustainable solution, reducing agricultural waste while providing a cost-effective and eco-friendly substrate (Bhattacharyya et al., 2021).
Previous studies have explored the use of various agricultural residues as growing media for plants. For example, coconut coir, a byproduct of coconut processing, has been successfully used as a substrate for horticultural crops due to its excellent water retention and aeration properties (Fussy and Papenbrock, 2022). Similarly, research has shown that rice hulls, another rice byproduct, can be used effectively as a growing medium for various plants (Asfar et al., 2021). However, the use of rice straw specifically for microgreen production has not been extensively studied. The development of a seed growing substrate from rice straw involves few key steps: pretreatment of the straw to modify its physical properties, and the formation of the substrate. Pretreatment is a critical phase that can influence the softness and texture of the straw. Sodium hydroxide (NaOH) pretreatment is a widely used method to enhance the properties of lignocellulosic biomass, making it more suitable for various applications. NaOH treatment breaks down the lignin and hemicellulose components, increasing the accessibility of cellulose fibers and improving the softness and pliability of the material (Shah et al., 2023).
In this study, we aim to standardize a method for preparing a growing media from rice straw for microgreen production. The process includes pretreatment of dried rice straw with different concentrations of NaOH solution for varying durations, followed by neutralization and drying. The effectiveness of the rice straw as growing media was evaluated by cultivating microgreens such as amaranth, cauliflower and cabbage. The performance of the rice straw was then compared to traditional control media (cocopeat and vermiculite) in terms of microgreen growth and mineral content. The successful development of rice straw as growing media could revolutionize the microgreen industry by providing a sustainable and cost-effective alternative to traditional substrates. Moreover, this approach could help mitigate the environmental impact of rice straw disposal and contribute to a more circular economy in agriculture. By converting agricultural waste into valuable resources, we can promote sustainable agricultural practices and enhance food security.
Rice straw was sourced from the ICAR-RCER farm located at 25°35′30″N and 85°5′3″E, air-dried, and chopped into 2–4 cm pieces using a mechanical chopper. Chopped rice straw was pretreated with NaOH solutions at concentrations of 1, 2, 5, and 7% (w/v) for varying durations of 10, 20, and 30 min at boiling temperature to determine optimal conditions for softening (Sharma et al., 2019; Han et al., 2019; Böhm et al., 2024). The sample treated with 1% NaOH for 30 min produced the softest pulp signifying the removal of lignin and hence produces less ash content. Which is crucial for ensuring the purity and quality of the seed growing media and also producers can minimize costs associated with excessive chemical use. The NaOH-treated pulp was dipped in dilute acid solution for some time and then thoroughly washed with tap water to neutralize its pH, ensuring it was safe for plant growth. The neutralized pulp was then dried in a hot air oven at 65°C for 2 days to prepare it for use as a growing medium (Figure 1).
The surface morphology of rice straw was investigated by scanning electron microscopy (SEM) (Zeiss EVO MA10). A measured amount of prepared rice straw media, vermiculite, and cocopeat was submerged in distilled water at room temperature until reaching swelling equilibrium. The swollen samples were then separated from any unabsorbed water by filtering them through filter paper. Water absorbency (W) of the superabsorbent composite was determined by weighing the swollen samples, and the absorbency (W) was calculated using Equation 1 (Kirti, 2016).
where, m1 and m2 are the weights of the dry sample and the water-swollen sample in grams.
In order to evaluate the softening effect, the residual amount of ash was measured for without treated and pretreated straws with different NaOH solution as described by Mukherjee et al. (2018).
where A represents the ash content in percentage.
Microgreens, including amaranth (Amaranthus tricolor), cauliflower (Brassica oleracea var. botrytis) and cabbage (Brassica oleracea var. capitata) were cultivated on the vermiculite, cocopeat and rice straw substrate. The seeds were uniformly broadcasted onto petri plates containing a moist substrate, ensuring optimal germination conditions. The plates were maintained indoors at room temperature. All treatments were established with three replications. Plant samples were harvested on 21 days after sowing, following established protocols for seedling growth and development (Braunstein, 2013).
At harvest, 21 days after sowing, the following morphological parameters were recorded: fresh and dry weight of seedlings (mg, measured using a laboratory scale) and seedling length (cm, including leaves, measured using a ruler). These measurements were taken from 10 randomly selected seedlings per tray. Dry weight was determined by drying the samples at 105°C for 24 h until a constant weight was achieved (Frąszczak and Kula-Maximenko, 2022). For mineral analysis the microgreens were oven-dried at 65°C to determine dry weight. The dried samples were ground using a 1-mm sieve and analyzed for their mineral profile (Poudel et al., 2023).
Mineral content was determined using atomic absorption spectrophotometry (AAS). Oven-dried microgreen samples (0.5 g) were predigested with nitric acid then heated with mixture of nitric acid and perchloric acid (3:1 v/v) at 150°C until the solution became clear. The digested solution was diluted with deionized water and filtered. The concentrations of iron (Fe), zinc (Zn) and manganese (Mn) were determined using AAS (Saurabh et al., 2024).
The effects of microgreen species (amaranth, cauliflower, and cabbage) and substrate types (rice straw, cocopeat, and vermiculite) on plant height, weight, and nutrient content were analyzed using a two-way ANOVA. The analysis was conducted under the assumptions of normality and homogeneity of variances. Tukey’s HSD test was applied for post-hoc comparisons at a significance level of p < 0.05 to identify significant differences among means. All statistical analyses were performed using R software. Principal component analysis (PCA) was conducted in R using the “prcomp” function to capture and summarize the variation present in the microgreen dataset.
Ash content in rice straw provides an opportunity on the effectiveness of a sample in terms of its disposal (Mukherjee et al., 2018). Ash content is an indicator of the inorganic residue present in the biomass after combustion (Vassilev et al., 2017). By studying the ash content of rice straw after alkali treatment, we can evaluate the effectiveness of the treatment in removing unwanted inorganic materials, which is crucial for ensuring the purity and quality of the seed growing media. Knowing the ash content helps in optimizing the alkali treatment process, which can be cost-intensive. By ensuring that the treatment is effective in reducing ash content, producers can minimize costs associated with excessive chemical use and processing time. The analysis of ash content in rice straw after alkali treatment reveals significant differences among the untreated and treated samples, as shown in Table 1. The untreated rice straw had the highest ash content (20.05%), while the treated samples exhibited a range of ash percentages from 7.10 to 11.10%, depending on the NaOH concentration used. The Tukey’s test revealed that the 1% NaOH-treated sample had the lowest ash content (7.1%), significantly different from the untreated sample (20.05%), and the samples treated with 2, 5, and 7% NaOH showed similar ash contents (9.4–11.1%). This indicates that lower NaOH concentrations (1%) were more effective in reducing ash content. Similar result was reported by Han et al. (2019).
High ash content can lead to faster degradation of the seed growing rice straw-based media. By studying and controlling the ash content, the longevity and durability of the media can be improved, ensuring it can be reused for microgreen production. In the present study, the ash content was found to be 7.1% when treated with 1% NaOH and heated for 30 min, which falls within the typical range of 5–12% reported in other studies on rice straw (Mukherjee et al., 2018). The rice straw pretreated with 1% w/v NaOH for 30 min yielded a soft pulp suitable for media formation. This concentration and duration effectively broke down the lignocellulosic structure of the straw, facilitating its water absorption ability. NaOH treatment breaks down the lignin and hemicellulose components, increasing the accessibility of cellulose fibers and improving the softness and pliability of the material (Kaur and Phutela, 2016). After pretreatment of rice straw, they are able to hold water, which is beneficial for plants as rice straw is natural fibre. After neutralization and washing, the rice straw media showed no microbial contamination, a critical factor for healthy microgreen growth (Deng et al., 2020).
Scanning electron microscopy (SEM) is a versatile technique for examining the surface morphology and structural details of materials with high magnification and resolution. The SEM analysis reveals substantial morphological differences between raw rice straw and rice straw treated with NaOH (Figure 2). Raw rice straw exhibits a smooth, compact, and rigid surface with visible epidermal cells, resulting from the dense lignin, hemicellulose, and cellulose structure acting as a protective barrier. NaOH treatment significantly alters this morphology, disrupting the lignin and hemicellulose matrix and creating a rougher, more porous surface. This process causes the fibers to swell and separate, increasing the surface area and water holding capacity and making it perfect for seed growing media formation (Han et al., 2019).
Figure 2. Scanning electron microscopy images of rice straw (a) untreated and (b) treated with NaOH solution.
The SEM micrographs corroborate these findings, showing ruptures in the silicon waxy structure, surface degradation, and morphological changes due to heating and NaOH treatment (Shah et al., 2023). Enhanced surface roughness after alkali pretreatment effectively increases the rice straw’s surface area (Kumari and Singh, 2022). In untreated rice straw, rigid and highly ordered fibrils are visible, while NaOH-treated rice straw exhibits significant structural changes, including loss of rigidity and order, exposure of microfibrils, and the development of cracks and increased porosity. These changes result in enhanced exposure of cellulosic material, facilitating more effective bioconversion (Shetty et al., 2017).
A good growing medium should have a high water-holding capacity, while also possessing sufficient macropores to ensure proper drainage and prevent waterlogging (Gupta et al., 2023). For the water holding capacity (WHC) of growing media, studies have shown that cocopeat and vermiculite have differing properties. The WHC of cocopeat and vermiculite was 891 and 389% whereas, that of pretreated rice straw was 673% of their weight (Figure 3). Cocopeat generally has a very high WHC, due to its fibrous structure and ability to retain water efficiently, making it suitable for moisture-dependent crops. Vermiculite, on the other hand, holds significantly less water. Pretreated rice straw has been explored as an alternative, offering intermediate WHC values, which balances moisture retention with aeration. The WHC of a growing medium is crucial for plant growth, as it affects moisture availability for seeds and seedlings (Kalaivani and Jawaharlal, 2019; Yan et al., 2024).
Microgreens of amaranth, cauliflower and cabbage were grown on the rice straw media and cocopeat, demonstrated better growth rates to those grown on vermiculite (Figures 4a–c). The plant length of amaranth, cauliflower, and cabbage microgreens showed variation based on the substrate used (Figures 5a,b). For amaranth, all three substrates (rice straw, vermiculite, and cocopeat) showed similar performance, with no significant differences in height. The average height ranged around 4–5 cm. Cabbage microgreens exhibited the greatest plant length in rice straw (6.2 cm), followed by cocopeat (6 cm) and vermiculite (5.8 cm). In cauliflower microgreens, rice straw and cocopeat produced significantly longest plants (5.4 and 5 cm), followed by vermiculite (4 cm). The ability of rice straw and cocopeat to retain moisture and support root growth likely explains the greater growth in plant length in these substrates. The higher plants length in rice straw and cocopeat could be attributed to its organic composition, better aeration, water absorption and drainage. Good oxygen availability to the roots supported healthy growth, resulting in taller plants (Poudel et al., 2023).
Figure 5. (a) Boxplot of plant length (cm) across substrates for different microgreens. (b) Error bar plot of plant length (cm) for microgreens across substrates.
The fresh weight of microgreens per plant varied across substrates, with rice straw generally producing the highest biomass (Figure 6). amaranth microgreens grown on rice straw had the highest fresh weight (18.21 mg plant−1), closely followed by cocopeat (18.15 mg plant−1) and vermiculite (17.43 mg plant−1). Cabbage microgreens also displayed the highest fresh weight when grown on rice straw (59.83 mg plant−1), followed by cocopeat (58.67 mg plant−1) and vermiculite (56.32 mg plant−1). Cauliflower microgreens exhibited a similar trend, with the highest fresh weight observed in plants grown on rice straw (54.80 mg plant−1), and followed by cocopeat (53.92 mg plant−1) and vermiculite (50.31 mg plant−1).
Figure 6. (a) Boxplot of fresh weight (mg/plant) across substrates for different microgreens. (b) Error bar plot of fresh weight (mg/plant) for microgreens across substrates.
Using Tukey’s significance test to compare means, Table 2 displays the effects of various substrates—rice straw, vermiculite, and cocopeat—on the weight and length of microgreens. Amaranth showed no discernible variation in weight or length between substrates. On the other hand, there were notable distinctions between cauliflower and cabbage. Vermiculite generated the shortest (5.80 cm) and lightest (56.32 g) microgreens for cabbage, whereas rice straw produced the longest (6.20 cm) and heaviest (59.83 g) microgreens. In a similar way, rice straw produced cauliflower that was noticeably longer (5.4 cm) and heavier (54.8 g) than vermiculite, which produced the lowest values. Rice straw is the most efficient growth medium for these microgreens, according to Tukey’s test.
This study assessed different growing media for indoor microgreen cultivation, including vermiculite (an inorganic material), cocopeat (a natural organic fiber), and rice straw (an organic residue). While peat-based substrates are widely used for microgreen production, they are expensive and non-renewable. Coconut coir, an organic and renewable alternative, has potential but comes with drawbacks like high salt content and elevated fungal and bacterial levels. Low-cost, renewable options could include fibrous materials like polyester, cotton, or jute. Other inorganic media, such as perlite, vermiculite, and rockwool, are available but expensive, energy-intensive to produce, and difficult to dispose of after use. This experiment seeks to explore more eco-friendly and cost-effective alternatives, particularly utilizing rice residues, which are often burned by farmers. The results highlight the significant impact of substrate choice on the growth and biomass production of microgreens. The choice of growing medium is crucial in determining the yield and quality of microgreens. Rice straw consistently resulted in the highest plant length and fresh weight across all species, indicating its potential as a superior substrate for microgreen cultivation. Its organic composition and ability to retain moisture likely contributed to enhanced nutrient uptake and growth. Bulgari et al. (2021) obtained highest yield of rocket on jute substrate, an organic by-product and discarded material from several industrial processes as compared to cocopeat and vermiculite. Paglialunga et al. (2023) reported lower fresh yields of radish and savoy cabbage microgreens grown on cellulose sponge and coconut fiber, attributing it to the lower air capacity of these substrates compared to peat. The higher micropore content in cellulose sponge and coconut fiber, typical of cohesive substrates, likely impeded root growth. Bayineni and Herur (2022) evaluated growth parameters like germination rate, plant height, fresh and dried weight, and the quality (chlorophyll a and b, carotenoids, microbial safety) of beet microgreens grown on substrates containing Synedrella nodiflora residues, vermicompost, red soil, and coco peat in various combinations. The findings revealed that incorporating Synedrella residues in the growing substrate improved yields, quality, germination rate, and ensured microbial safety. Beet microgreens grown with Synedrella had low microbiological contamination (total coliforms), making them a healthy and safe option for fresh vegetable salads.
In another study rock wool growing media with AB mix nutrition showed the best results for seedling height, leaf count, fresh weight, and chlorophyll content compared to cocopeat and soil (Khairunnisa et al., 2023). These findings are in line with previous research observations that emphasizes the importance of substrate selection in microgreen production. Substrates that provide adequate moisture retention, aeration, and nutrient availability are critical for optimizing plant growth and biomass accumulation (Poudel et al., 2023; Gunjal et al., 2024). Thepsilvisut et al. (2023) found that lower bulk density and higher total pore space in growing media (coconut coir dust, leaf compost, and their 1:1 combination) significantly increased the yield of sunflower and water-spinach microgreens.
The rice straw media were structurally stable and remained intact throughout the growth period, highlighting their potential for practical application. Additionally, using agricultural byproducts like rice straw reduces waste and supports sustainable farming practices (Singh et al., 2023). It can be used for production of seedlings/microgreens of high value vegetable, flower, spice and even plantation crops. It aids in quick germination of seeds and production of disease-free, organically-raised microgreens. The microgreens raised in this medium develop robust root system (Figure 7).
Abad et al. (2001) found that the ideal growing substrate for seed germination should have a water holding capacity of 55–70% v/v and aeration of 20–30% v/v to support protoplasm hydration and enzymatic activity. Coco coir mixed with soil and sand or rice husk provided low bulk density, aiding air and water movement essential for germination. Muchjajib et al. (2014) suggested that using local organic materials like coco coir dust, sugarcane filter cake, and vermicompost in microgreen production boosted fresh yield and reduced production costs.
Table 3 evaluates the impact of substrates—rice straw, vermiculite, and cocopeat—on manganese (Mn), zinc (Zn), and iron (Fe) concentrations in amaranth, cabbage, and cauliflower microgreens. Vermiculite consistently yielded the highest Zn and Fe levels across all microgreens, with notable values for amaranth (Zn: 5.43 mg/kg, Fe: 304.05 mg/kg), cabbage (Zn: 7.4 mg/kg, Fe: 286.87 mg/kg), and cauliflower (Zn: 7.02 mg/kg, Fe: 155.95 mg/kg). Mn levels varied, with vermiculite and rice straw performing better for cabbage, while vermiculite alone excelled for cauliflower. Substrate type significantly affected micronutrient concentrations (p ≤ 0.05), with vermiculite generally being the most effective, and the interaction between microgreens and substrate also proved significant. In amaranth, Mn concentrations across substrates (1.38, 2.13, and 1.59 mg/kg) share no significant differences. In contrast, for cabbage, vermiculite and rice straw for Zn, showing significant variation. The systematic annotation helps identify which substrate treatments yield significantly different micronutrient levels within each microgreen summarizes in Figures 8b, 9b, 10b. The box plot also shows that vermiculite consistently produces the highest Mn concentrations across all microgreens, followed by rice straw and cocopeat. Amaranth has the highest Fe levels, while cauliflower exhibits the lowest concentrations across all substrates summarizes in Figures 8a, 9a, 10a.
Figure 8. (a) Boxplot of Mn across different microgreens for different substrates. (b) Error bar plot of Mn across substrates for different microgreens.
Figure 9. (a) Boxplot of Zn across different microgreens for different substrates. (b) Error bar plot of Zn across substrates for different microgreens.
Figure 10. (a) Boxplot of Fe across different microgreens for different substrates. (b) Error bar plot of Fe across substrates for different microgreens.
Limited data exist on the chemical composition of microgreens grown on various substrates, and the specific mineral requirements of these plants remain largely unknown. Understanding the nutrient uptake by microgreens could help optimize fertilization strategies based on the growing substrate used (Kyriacou et al., 2016). Since mineral elements play a vital role in human nutrition, analyzing the mineral composition of microgreens provides valuable insights into their potential contribution to the human diet (Bulgari et al., 2017). Effective nutrient management could enhance both the yield and nutritional quality of microgreens.
Amaranth showed the highest Fe concentration when grown in vermiculite (304.05 mg/kg), followed by rice straw (78.31 mg/kg) and cocopeat (23.72 mg/kg). For Mn and Zn, vermiculite consistently provided the highest concentration (Mn: 2.13 mg/kg; Zn: 5.43 mg/kg), suggesting that vermiculite might have higher metal ion exchange or adsorption capacity for these nutrients. Cocopeat also supported moderate Mn and Zn levels, while rice straw had the lowest Zn content. Cabbage followed a similar trend, with the highest Fe and Zn concentrations observed in vermiculite (Fe: 286.87 mg/kg; Zn: 7.4 mg/kg). Mn levels in cabbage were lower in cocopeat and rice straw (0.93 mg/kg and 1.17 mg/kg, respectively) but higher in vermiculite (1.64 mg/kg), implying that vermiculite might have provided a better source of these essential micronutrients for cabbage growth. Cauliflower grown in vermiculite also showed a higher Fe (155.95 mg/kg) and Zn (7.02 mg/kg) concentration compared to rice straw and cocopeat. The Mn levels were relatively low in cocopeat and rice straw (0.38 mg/kg and 0.35 mg/kg, respectively), while vermiculite significantly enhanced Mn concentration (1.87 mg/kg).
Vermiculite, across all crops, had the highest Fe and Zn concentrations, suggesting it enhanced the availability of these micronutrients. This could be attributed to vermiculite’s cation exchange capacity (CEC) and its ability to retain and release metal ions efficiently. Studies have shown that vermiculite improves nutrient retention due to its layered structure, which increases soil aeration and provides better nutrient retention compared to other substrates (Valášková and Martynkova, 2012; Bulgari et al., 2021). Cocopeat, though organic, had lower metal retention compared to vermiculite, which can be seen in its relatively lower Fe and Zn concentrations. However, cocopeat still offered a moderate level of nutrient availability, particularly Mn, which was likely due to its good water retention properties that supported micronutrient solubility. Rice straw, with lower values for Zn and Mn in most crops, might not be as efficient in micronutrient retention, potentially due to its higher decomposition rate and lower CEC compared to vermiculite. Its performance was better in Fe retention, especially for amaranth (78.31 mg/kg), but generally performed lower than vermiculite across the board.
Amaranth had the highest overall Fe absorption, particularly from vermiculite, suggesting it has a high demand for Fe, which plays a crucial role in chlorophyll synthesis and electron transport during photosynthesis. Cabbage also absorbed high levels of Fe and Zn when grown in vermiculite, indicating that it benefits from a nutrient-dense growing medium. Cauliflower, while having lower Mn and Zn uptake in cocopeat and rice straw, absorbs a significant amount of Fe and Zn in vermiculite, highlighting its preference for a nutrient-rich environment.
Vermiculite proved to be the most effective medium for enhancing the availability of Fe, Zn, and Mn across all crops studied, particularly for amaranth and cabbage. Its superior performance in micronutrient retention makes it a valuable substrate in organic and inorganic farming systems, especially where nutrient availability is a limiting factor. Cocopeat and rice straw, though beneficial in terms of organic content, may require supplementation to match the micronutrient availability seen in vermiculite-based systems.
Similar results were reported by Nyenhuis and Drelich (2015) where they investigated whether crops can be biofortified with micronutrients by growing alfalfa sprouts on mineral-enriched cellulose fiber mats. Sprouts grown on mats containing copper ions or nanoparticles showed significant increases in copper content. The findings suggested engineered fiber mats as a potential method to enhance the nutritional value of microgreens. Bulgari et al. (2021) investigated the impact of three growing media (vermiculite, coconut fiber, and jute fabric) on the yield and quality of two basil varieties (Ocimum basilicum L.-green basil and O. basilicum var. purpurecsens-red basil) and rocket (Eruca sativa Mill.) microgreens. They have observed higher values of anthocyanins and phenolic index in red basil microgreens grown on vermiculite and jute compared to coconut fibre.
Principal component analysis (PCA) was applied to all measured variables in the microgreens dataset to summarize the findings detailed in ANOVA Table 2, 3 and Tukey’s ANOVA (Figures 5b, 6b, 8b, 9b, 10b). Separate PCA analyses were performed for each species to better evaluate the impact of growing media on microgreens’ yield and nutritional quality, as interactions between species and growing media were observed for most variables. Figures 11a–c illustrates the PCA biplots, showcasing the yield, mineral profile, and nutritional attributes for amaranth, cabbage and cauliflower, respectively. In case of amaranth, PC1 and PC2 explains 91.3 and 8.7% variation of the data. The cocopeat medium was associated with length, rice straw is associated with weight. Additionally, for cabbage, PCA1 explains 88% and PCA2 explains 12% variance in the data. Here, rice straw is associated with weight and length, vermiculite is associated with Fe, Mn and Zn. For cauliflower, PCA1 explains 98.5% and PCA2 explains 1.5% variation in the dataset. Similar to the cabbage, rice straw is associated with weight and length, vermiculite is associated with Fe, Mn and Zn.
Figure 11. (a) PCA biplot (PC1 vs. PC2) showing the distribution of the several parameters of amaranth grown in different growing media. (b) PCA biplot (PC1 vs. PC2) showing the distribution of the several parameters of cabbage grown in different growing media. (c) PCA biplot (PC1 vs. PC2) showing the distribution of the several parameters of cauliflower grown in different growing media.
This study successfully developed an efficient and sustainable growing medium from rice straw for microgreen cultivation. The optimal pretreatment involved using a 1% w/v NaOH solution boiling for 30 min, which effectively softened the rice straw, transforming it into a suitable medium. The resulting rice straw substrate supported the healthy growth of microgreens like cauliflower, amaranth, and cabbage, offering better water retention and root development compared to traditional media such as vermiculite.
Nutritional analysis revealed that vermiculite provided the highest Zn and Fe levels, while rice straw performed well in Mn accumulation and plant biomass. PCA validated these findings, linking rice straw to improved biomass and plant length, while vermiculite was associated with higher micronutrient accumulation. Additionally, the nutrient content of microgreens grown on rice straw can be further improved by supplementing or fortifying the rice straw-based media with essential nutrients. This approach promotes sustainable agriculture by converting waste into valuable resources, contributing to a circular economy. The findings highlight the significant potential of rice straw as a practical growing medium for microgreen production, supporting efforts to enhance food security and environmental sustainability. Further research is recommended for a deeper understanding and optimization of this method.
The original contributions presented in the study are included in the article/supplementary material, further inquiries can be directed to the corresponding author.
KSa: Conceptualization, Data curation, Formal analysis, Funding acquisition, Investigation, Methodology, Validation, Visualization, Writing – original draft. HR: Data curation, Formal analysis, Software, Validation, Visualization, Writing – review & editing. KSh: Investigation, Methodology, Resources, Writing – review & editing. PS: Investigation, Methodology, Resources, Writing – review & editing. VP: Software, Validation, Writing – review & editing. TK: Writing – review & editing. AM: Writing – review & editing. BS: Writing – review & editing. AS: Writing – review & editing. AD: Writing – review & editing. AU: Writing – review & editing. PJ: Writing – review & editing. SK: Writing – review & editing. RS: Writing – review & editing.
The author(s) declare that no financial support was received for the research and/or publication of this article.
The authors declare that the research was conducted in the absence of any commercial or financial relationships that could be construed as a potential conflict of interest.
The reviewer DA declared a shared institution with the author HR to the handling editor at the time of review.
The authors declare that no Gen AI was used in the creation of this manuscript.
All claims expressed in this article are solely those of the authors and do not necessarily represent those of their affiliated organizations, or those of the publisher, the editors and the reviewers. Any product that may be evaluated in this article, or claim that may be made by its manufacturer, is not guaranteed or endorsed by the publisher.
Abad, M., Noguera, P., and Bures, S. (2001). National inventory of organic wastes for use as growing media for ornamental potted plant production: a case study in Spain. Bioresour. Technol. 77, 197–200. doi: 10.1016/S0960-8524(00)00152-8
Asfar, A. M. I. A., Asfar, A. M. I. T., Thaha, S., Kurnia, A., and Syaifullah, A. (2021). The potential processing of rice husk waste as an alternative media for ornamental plants. Riau J. Empower. 4, 129–138. doi: 10.31258/raje.4.3.129-138
Bayineni, V. K., and Herur, K. (2022). Natural Synedrella residues as a growing substrate ingredient: an eco-friendly way to improve yield and quality of beet (Beta vulgaris) microgreens. Eur. J. Agric. Food Sci. 4, 1–5. doi: 10.24018/ejfood.2022.4.6.593
Bhaswant, M., Shanmugam, D. K., Miyazawa, T., Abe, C., and Miyazawa, T. (2023). Microgreens—a comprehensive review of bioactive molecules and health benefits. Molecules 28:867. doi: 10.3390/molecules28020867
Bhattacharyya, P., Bisen, J., Bhaduri, D., Priyadarsini, S., Munda, S., Chakraborti, M., et al. (2021). Turn the wheel from waste to wealth: economic and environmental gain of sustainable rice straw management practices over field burning in reference to India. Sci. Total Environ. 775:145896. doi: 10.1016/j.scitotenv.2021.145896
Böhm, M., Jerman, M., Keppert, M., Kobetičová, K., Koňáková, D., and Pavlíková, M. (2024). Alkaline treatment of straw for composite material production and its impact on water vapor adsorption characteristics. J. Renew. Mater. 13, 363–383. doi: 10.32604/jrm.2024.056984
Braunstein, M. M. (2013). Microgreen garden: indoor grower’s guide to gourmet greens. Summertown, TN: Book Publishing Company.
Bulgari, R., Baldi, A., Ferrante, A., and Lenzi, A. (2017). Yield and quality of basil, Swiss chard, and rocket microgreens grown in a hydroponic system. N. Z. J. Crop. Hortic. Sci. 45, 119–129. doi: 10.1080/01140671.2016.1259642
Bulgari, R., Negri, M., Santoro, P., and Ferrante, A. (2021). Quality evaluation of indoor-grown microgreens cultivated on three different substrates. Horticulturae 7:96. doi: 10.3390/horticulturae7050096
Deng, Y., Qiu, Y., Yao, Y., Ayiania, M., and Davaritouchaee, M. (2020). Weak-base pretreatment to increase biomethane production from wheat straw. Environ. Sci. Pollut. Res. 27, 37989–38003. doi: 10.1007/s11356-020-09914-7
Frąszczak, B., and Kula-Maximenko, M. (2022). The biometric parameters of microgreen crops grown under various light conditions. Agriculture 12:576. doi: 10.3390/agriculture12050576
Fussy, A., and Papenbrock, J. (2022). An overview of soil and soilless cultivation techniques—chances, challenges and the neglected question of sustainability. Plan. Theory 11:1153. doi: 10.3390/plants11091153
Ghoora, M. D., Babu, D. R., and Srividya, N. (2020). Nutrient composition, oxalate content and nutritional ranking of ten culinary microgreens. J. Food Compos. Anal. 91:103495. doi: 10.1016/j.jfca.2020.103495
Gunjal, M., Singh, J., Kaur, J., Kaur, S., Nanda, V., Mehta, C. M., et al. (2024). Comparative analysis of morphological, nutritional, and bioactive properties of selected microgreens in alternative growing medium. S. Afr. J. Bot. 165, 188–201. doi: 10.1016/j.sajb.2023.12.038
Gupta, A., Qureshi, N., Dangwal, A., and Kumar, C. (2023). Nutrient analysis of different media used for nursery production. Int. J. Plant Soil Sci. 35, 728–734. doi: 10.9734/ijpss/2023/v35i214034
Han, X., Luo, Q., Bao, A., and Luo, S. (2019). Research on the mechanism of improving rice straw self-bonding via NaOH solution pretreatment. Bioresources 14, 9352–9363. doi: 10.15376/biores.14.4.9352-9363
Hoang, G. M., and Vu, T. T. (2022). Selection of suitable growing substrates and quality assessment of Brassica microgreens cultivated in greenhouse. Acad. J. Biol. 44, 133–142. doi: 10.15625/2615-9023/16833
Kalaivani, K., and Jawaharlal, M. (2019). Study on physical characterization of coco peat with different proportions of organic amendments for soilless cultivation. J. Pharmacogn. Phytochem. 8, 2283–2286.
Kaur, K., and Phutela, U. G. (2016). Enhancement of paddy straw digestibility and biogas production by sodium hydroxide-microwave pretreatment. Renew. Energy 92, 178–184. doi: 10.1016/j.renene.2016.01.083
Khairunnisa, N., Warnita, W., and Hervani, D. (2023). Impact of growing media and nutrition on growth and yield of broccoli microgreens (Brassica oleracea). Int. J. Environ. Agric. Biotechnol. 8, 30–34. doi: 10.22161/ijeab
Kirti, S. (2016). “Nanoclay polymer composites (NCPCs) with biodegradable polymers for controlled release of nitrogen in rice and wheat crops” in Doctoral dissertation (New Delhi: Division of Soil Science & Agricultural Chemistry, Indian Agricultural Research Institute).
Kumari, D., and Singh, R. (2022). Rice straw structure changes following green pretreatment with petha wastewater for economically viable bioethanol production. Sci. Rep. 12:10443. doi: 10.1038/s41598-022-14627-7
Kyriacou, M. C., Rouphael, Y., Di Gioia, F., Kyratzis, A., Serio, F., Renna, M., et al. (2016). Micro-scale vegetable production and the rise of microgreens. Trends Food Sci. Technol. 57, 103–115. doi: 10.1016/j.tifs.2016.09.005
Muchjajib, U., Muchjajib, S., Suknikom, S., and Butsai, J. (2014). Evaluation of organic media alternatives for the production of microgreens in Thailand. XXIX International Horticultural Congress on Horticulture: Sustaining lives, Livelihoods and Landscapes (IHC2014). 367–372
Mukherjee, A., Banerjee, S., and Halder, G. (2018). Parametric optimization of delignification of rice straw through central composite design approach towards application in grafting. J. Adv. Res. 14, 11–23. doi: 10.1016/j.jare.2018.05.004
Nyenhuis, J., and Drelich, J. W. (2015). Essential micronutrient biofortification of sprouts grown on mineral-fortified fiber mats. Int. Scholarly Sci. Res. Innov. 9, 981–984. doi: 10.5281/zenodo.1107016
Paglialunga, G., El Nakhel, C., Proietti, S., Moscatello, S., Battistelli, A., Formisano, L., et al. (2023). Substrate and fertigation management modulate microgreens production, quality and resource efficiency. Front. Sustain. Food Syst. 7:1222914. doi: 10.3389/fsufs.2023.1222914
Parihar, D. S., Narang, M. K., Dogra, B., Prakash, A., and Mahadik, A. (2023). Rice residue burning in northern India: an assessment of environmental concerns and potential solutions–a review. Environ. Res. Commun. 5:062001. doi: 10.1088/2515-7620/acb6d4
Poudel, P., Duenas, A. E., and Di Gioia, F. (2023). Organic waste compost and spent mushroom compost as potential growing media components for the sustainable production of microgreens. Front. Plant Sci. 14:1229157. doi: 10.3389/fpls.2023.1229157
Saurabh, K., Kumar, S., Kumar, R., Mishra, J. S., Das, A., Srivastava, A. K., et al. (2024). Biofortifying drought-tolerant rice: enhancing growth and nutritional quality with foliar iron and zinc applications in Eastern India. J. Plant Nutr. 48, 164–176. doi: 10.1080/01904167.2023.2262257
Shah, T. A., Khalid, S., Nafidi, H. A., Salamatullah, A. M., and Bourhia, M. (2023). Sodium hydroxide hydrothermal extraction of lignin from rice straw residue and fermentation to biomethane. Sustainability 15:8755. doi: 10.3390/su15118755
Sharma, S., Nandal, P., and Arora, A. (2019). Ethanol production from NaOH pretreated rice straw: a cost effective option to manage rice crop residue. Waste Biomass Valorization 10, 3427–3434. doi: 10.1007/s12649-018-0360-4
Shetty, D. J., Kshirsagar, P., Tapadia-Maheshwari, S., Lanjekar, V., Singh, S. K., and Dhakephalkar, P. K. (2017). Alkali pretreatment at ambient temperature: a promising method to enhance biomethanation of rice straw. Bioresour. Technol. 226, 80–88. doi: 10.1016/j.biortech.2016.12.003
Singh, M., Biswas, A., Verma, A., Singh, T., and Choudhary, O. P. (2023). Way forward to adopt agricultural practices for paddy straw management based on carbon sequestration and GHG emissions. Paddy Water Environ. 21, 295–305. doi: 10.1007/s10333-023-00931-z
Singh, Y., Jat, M., Sidhu, H. S., Singh, P., and Varma, A. (2017). Innovative viable solution to rice residue burning in rice-wheat cropping system through concurrent use of super straw management system-fitted combines and turbo happy seeder. Policy Brief 2:16.
Thepsilvisut, O., Sukree, N., Chutimanukul, P., Athinuwat, D., Chuaboon, W., Poomipan, P., et al. (2023). Efficacy of agricultural and food wastes as the growing media for sunflower and water spinach microgreens production. Horticulturae 9:876. doi: 10.3390/horticulturae9080876
Valášková, M., and Martynkova, G. S. (2012). “Vermiculite: structural properties and examples of the use” in Clay minerals in nature—their characterization, modification, and application (Rijeka: IntechOpen), 209–238.
Vassilev, S. V., Vassileva, C. G., Song, Y. C., Li, W. Y., and Feng, J. (2017). Ash contents and ash-forming elements of biomass and their significance for solid biofuel combustion. Fuel 208, 377–409. doi: 10.1016/j.fuel.2017.07.036
Keywords: rice straw, eco-friendly growing media, agri-waste management, microgreens, micronutrients
Citation: Saurabh K, Roy HS, Shubha K, Sundaram PK, Prakash V, Koley TK, Mukherjee A, Sarkar B, Singh AK, Das A, Upadhyaya A, Jeet P, Kumar S and Singh RR (2025) Transforming rice straw into eco-friendly growing medium for microgreens: a solution for agricultural waste management. Front. Sustain. Food Syst. 9:1556396. doi: 10.3389/fsufs.2025.1556396
Received: 06 January 2025; Accepted: 04 March 2025;
Published: 19 March 2025.
Edited by:
Muzaffar Hasan, Central Institute of Agricultural Engineering (ICAR), IndiaReviewed by:
Vaibhav Kumar, ICAR-Indian Institute of Pulses Research, IndiaCopyright © 2025 Saurabh, Roy, Shubha, Sundaram, Prakash, Koley, Mukherjee, Sarkar, Singh, Das, Upadhyaya, Jeet, Kumar and Singh. This is an open-access article distributed under the terms of the Creative Commons Attribution License (CC BY). The use, distribution or reproduction in other forums is permitted, provided the original author(s) and the copyright owner(s) are credited and that the original publication in this journal is cited, in accordance with accepted academic practice. No use, distribution or reproduction is permitted which does not comply with these terms.
*Correspondence: Kirti Saurabh, a2lydGkuc2F1cmFiaEBpY2FyLmdvdi5pbg==
Disclaimer: All claims expressed in this article are solely those of the authors and do not necessarily represent those of their affiliated organizations, or those of the publisher, the editors and the reviewers. Any product that may be evaluated in this article or claim that may be made by its manufacturer is not guaranteed or endorsed by the publisher.
Research integrity at Frontiers
Learn more about the work of our research integrity team to safeguard the quality of each article we publish.