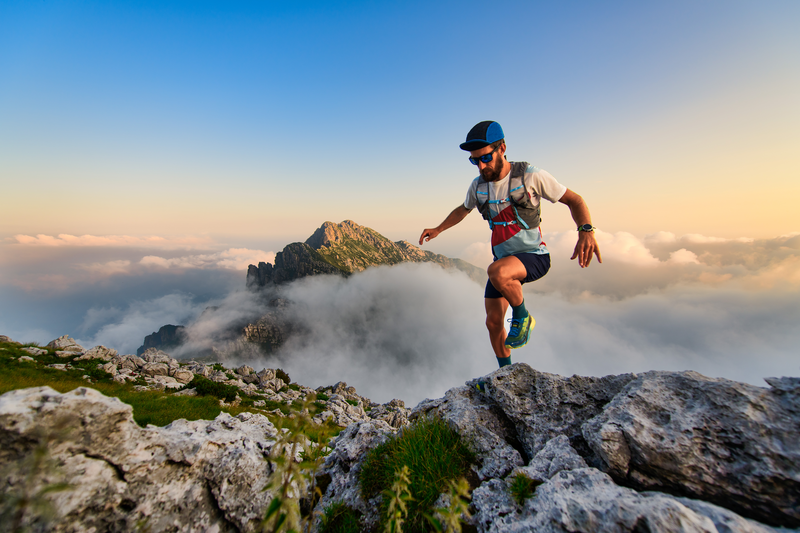
94% of researchers rate our articles as excellent or good
Learn more about the work of our research integrity team to safeguard the quality of each article we publish.
Find out more
ORIGINAL RESEARCH article
Front. Sustain. Food Syst.
Sec. Sustainable Food Processing
Volume 9 - 2025 | doi: 10.3389/fsufs.2025.1531601
This article is part of the Research Topic Sustainable Synergy: Balancing Food, Energy, and Water for Improving Sustainability View all articles
The final, formatted version of the article will be published soon.
You have multiple emails registered with Frontiers:
Please enter your email address:
If you already have an account, please login
You don't have a Frontiers account ? You can register here
The current study aims to develop and conduct a techno-environmental evaluation of a new sustainable forced convection solar dryer (SFCSD). Where the developed SFCSD was integrated with a unique electronic circuit that enables it to operate in two different modes: 1. forced air circulation (active mode) and 2. natural air circulation (passive mode), based on the air temperature (AT) inside the drying room and the ambient light intensity (Li). Furthermore, the SFCSD is equipped with an early warning system (SOS) that can send a warning message (SMS) to the operator in case of system failure. The Aswan region of Egypt uses the developed SFCSD to dry the most famous five date fruit varieties (Shamia, Bartamuda, Sakkoti, Malkabii, and Gondaila). The speed sensor of air suction fan, Li sensor, relative humidity (RH) sensor and AT sensor were calibrated against standard devices before used. The results showed a strong correlation between the measured and reference values. Despite the slight underestimation of the values, the sensors' response remains consistent and predictable. The R2 values for the speed sensor, the Li sensor, the AT sensor, and the RH sensor were, in that order, 0.9904, 0.987, and 0.9863. The average daily solar radiation, ambient AT, and RH during field tests were 494.78 W/m2, 29.46 °C, and 23.68 %, respectively. The initial moisture content (MC) of the different date fruit (DF) varieties used in the current study ranged between 10.32% and 12.56%, and the DF samples reached equilibrium MC at 9 days. The effective moisture diffusivity (EMD) ranged between 3.5569×10-7 m2/s and 3.9489×10-7 m2/s. The maximum efficiency of the photovoltaic (PV) system and the solar collector was 25.28% and 69.52%, respectively. The analysis of environmental impact revealed that the energy payback time (EPP) for the developed SFCSD is 7.15 years, which represented only 23.83% of the system’s lifetime. The developed SFCSD has a CO2 mitigation value of 93.2 tons and earned carbon credit (ECC) valued of 6757.02 USD throughout its estimated lifetime of 30 years. The environmental impact analysis demonstrates that the developed SFCSD is an appropriate alternative for preserving agricultural products while maintaining environmental sustainability.
Keywords: sustainability, earned carbon credit, moisture, diffusivity, sustainable agriculture
Received: 20 Nov 2024; Accepted: 28 Feb 2025.
Copyright: © 2025 Elwakeel, El-Mesery, Elbeltagi, Salem, Saleh, Moustapha, Abo-Taha and Elkot. This is an open-access article distributed under the terms of the Creative Commons Attribution License (CC BY). The use, distribution or reproduction in other forums is permitted, provided the original author(s) or licensor are credited and that the original publication in this journal is cited, in accordance with accepted academic practice. No use, distribution or reproduction is permitted which does not comply with these terms.
* Correspondence:
Ali Salem, Faculty of Engineering and Information Technology, University of Pécs, Pécs, Hungary
Disclaimer: All claims expressed in this article are solely those of the authors and do not necessarily represent those of their affiliated organizations, or those of the publisher, the editors and the reviewers. Any product that may be evaluated in this article or claim that may be made by its manufacturer is not guaranteed or endorsed by the publisher.
Research integrity at Frontiers
Learn more about the work of our research integrity team to safeguard the quality of each article we publish.