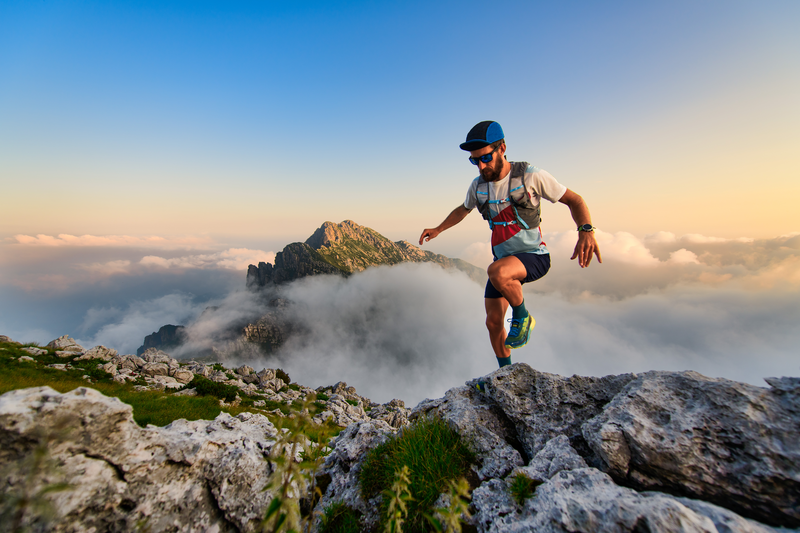
94% of researchers rate our articles as excellent or good
Learn more about the work of our research integrity team to safeguard the quality of each article we publish.
Find out more
REVIEW article
Front. Sustain. Food Syst.
Sec. Climate-Smart Food Systems
Volume 9 - 2025 | doi: 10.3389/fsufs.2025.1529837
This article is part of the Research Topic Transforming African Food Systems View all 15 articles
The final, formatted version of the article will be published soon.
You have multiple emails registered with Frontiers:
Please enter your email address:
If you already have an account, please login
You don't have a Frontiers account ? You can register here
In Low-Income Food Deficit Countries (LIFDCs), there is a growing demand for ruminant livestock products due to population growth, urbanization, and rising incomes. However, smallholder farmers in these regions face constant challenges in securing reliable year-round feed supply, which affects animal performance and the ability to meet increasing demand for animal products. This comprehensive review thus explores the potential of fruit by-products, specifically cashew apples, papayas, and mangoes, which are often discarded and contribute to environmental pollution but can be valuable resources for livestock farmers. The review examines the current state of small ruminant livestock production in LIFDCs, particularly in Sub-Saharan Africa and adopts a systems thinking approach to consider using cashew apple, papaya, and mango by-products as a potential feed source. Small ruminant livestock production is highlighted for efficiently converting nutrient-rich food waste from fruits like cashew apples, papayas, and mangoes into valuable milk and meat products. The review also addresses the environmental aspect, pointing out potential greenhouse gas emissions resulting from improper disposal of fruit wastes and the urgent need to convert them into animal feeds. It provides data on processing, preservation techniques, chemical composition, and the limited available information on the impact of these fruit by-products on feed intake, growth, carcass quality, methane emissions, and overall well-being of small ruminants. Challenges related to the storage and feeding of these by-products are also discussed. Despite limited data and conflicting evidence, the review strongly advocates using cashew apples, papaya, and mango by-products as vital feed resources for small ruminants. It emphasizes the need for further research to determine their nutritional value in local contexts, establish optimal inclusion levels, and devise strategies for prolonging shelf life. This effort holds promise for addressing food deficits and enhancing food security in LIFDCs where these challenges are most acute.
Keywords: Valorisation, Fruit by-products, Small ruminant, Circular economy, systems thinking, sustainability, Food security
Received: 17 Nov 2024; Accepted: 17 Mar 2025.
Copyright: © 2025 Anim-Jnr, Ishaq, Sasu, Gyimah, Greathead, Bosch, Mabiki and Emmambux. This is an open-access article distributed under the terms of the Creative Commons Attribution License (CC BY). The use, distribution or reproduction in other forums is permitted, provided the original author(s) or licensor are credited and that the original publication in this journal is cited, in accordance with accepted academic practice. No use, distribution or reproduction is permitted which does not comply with these terms.
* Correspondence:
Antoinette Simpah Anim-Jnr, Department of Animal Science, Faculty of Agriculture, Kwame Nkrumah University of Science and Technology, Kumasi, Ghana
Disclaimer: All claims expressed in this article are solely those of the authors and do not necessarily represent those of their affiliated organizations, or those of the publisher, the editors and the reviewers. Any product that may be evaluated in this article or claim that may be made by its manufacturer is not guaranteed or endorsed by the publisher.
Research integrity at Frontiers
Learn more about the work of our research integrity team to safeguard the quality of each article we publish.