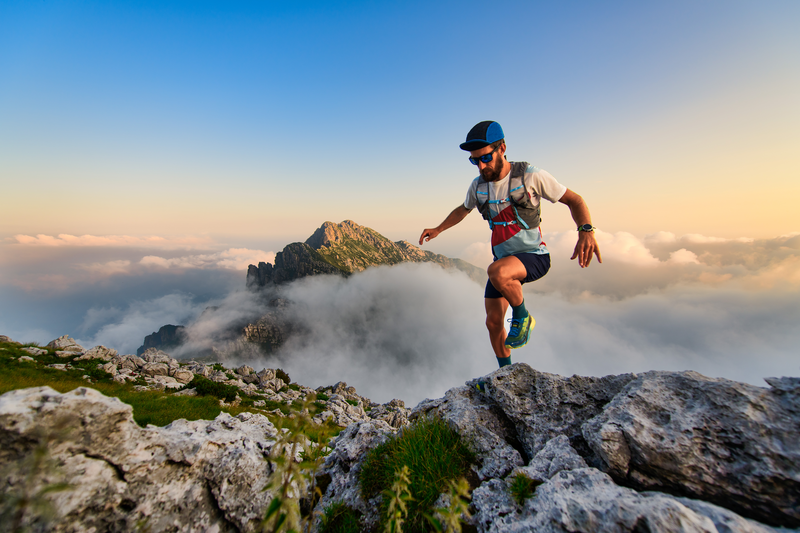
94% of researchers rate our articles as excellent or good
Learn more about the work of our research integrity team to safeguard the quality of each article we publish.
Find out more
ORIGINAL RESEARCH article
Front. Sustain. Food Syst.
Sec. Agroecology and Ecosystem Services
Volume 9 - 2025 | doi: 10.3389/fsufs.2025.1527898
This article is part of the Research Topic Innovative Approaches in Soil, Water, and Crop Management for Sustainable Agricultural Systems View all 5 articles
The final, formatted version of the article will be published soon.
You have multiple emails registered with Frontiers:
Please enter your email address:
If you already have an account, please login
You don't have a Frontiers account ? You can register here
This study assessed the impact of compost applications and multispecies cover crops on almond production and soil health with the working hypothesis that one or both practices would improve soil structure and fertility and therefore increase the overall productivity of the orchard. Treatments were applied to a 17-year-old organic almond orchard in a randomized complete block design for three consecutive years. Treatments included: 1) Fall-applied compost (COMP), 2) Fall-seeded multispecies cover crop (MSCC), 3) both compost and cover crop combined (COMP+MSCC), and 4) a non-treated control (CONT). Soil samples were collected in the fall of year one, before treatments were applied, and again in the fall of year three. Samples were analyzed for physical, chemical, and biological characteristics at the Center for Regenerative Agriculture & Resilient Systems’ (CRARS) Regenerative Agriculture Demonstration Lab and at a commercial soil lab. Almonds were harvested by row to determine yield within each plot and kernels were analyzed for nutrient content after the third growing season. Understory forage was collected in the spring of year three and analyzed for quality and dry matter production. After three years, soil in all treatment plots (COMP, MSCC, and COMP+MSCC) had higher quantities of soil organic matter (SOM), and therefore carbon, nitrogen, and other nutrients, as compared to CONT, with compost increasing SOM content more than the cover crop treatment (P<0.05). Microbial biomass was higher in COMP+MSCC plots compared to CONT plots (P<0.05) and microbial activity (soil respiration rate and % microbially active carbon) was higher in the COMP plots compared to CONT plots (P<0.05). Understory forage biomass and nutritional quality were significantly higher in the MSCC and COMP+MSCC treatment plots compared with plots without cover crops (P<0.05). This is an important benefit since orchard understory grazing with sheep is an important component of this farm's normal operation. Finally, almond yields and kernel nutrient densities were significantly higher in treatment plots compared to control plots (P<0.05). Collectively, these results demonstrate the multiple benefits of these regenerative/climate-smart practices in an organic orchard system.
Keywords: multispecies cover crop1, compost2, almond3, regenerative agriculture4, soil health5, climate-smart agriculture6, organic7, whole-system research8
Received: 14 Nov 2024; Accepted: 25 Feb 2025.
Copyright: © 2025 Krach, Daley and Liles. This is an open-access article distributed under the terms of the Creative Commons Attribution License (CC BY). The use, distribution or reproduction in other forums is permitted, provided the original author(s) or licensor are credited and that the original publication in this journal is cited, in accordance with accepted academic practice. No use, distribution or reproduction is permitted which does not comply with these terms.
* Correspondence:
Raquel Krach, CSU Chico Center for Regenerative Agriculture and Resilient Systems, Chico, United States
Disclaimer: All claims expressed in this article are solely those of the authors and do not necessarily represent those of their affiliated organizations, or those of the publisher, the editors and the reviewers. Any product that may be evaluated in this article or claim that may be made by its manufacturer is not guaranteed or endorsed by the publisher.
Research integrity at Frontiers
Learn more about the work of our research integrity team to safeguard the quality of each article we publish.