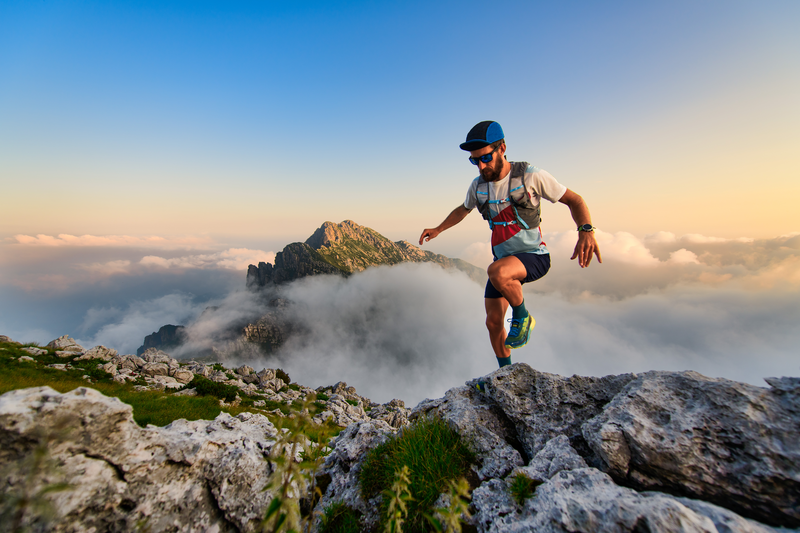
94% of researchers rate our articles as excellent or good
Learn more about the work of our research integrity team to safeguard the quality of each article we publish.
Find out more
ORIGINAL RESEARCH article
Front. Sustain. Food Syst.
Sec. Agro-Food Safety
Volume 9 - 2025 | doi: 10.3389/fsufs.2025.1502841
The final, formatted version of the article will be published soon.
You have multiple emails registered with Frontiers:
Please enter your email address:
If you already have an account, please login
You don't have a Frontiers account ? You can register here
Introduction: Poultry litter (PL) compost and heat-treated PL-pellets (PP) are common organic fertilizer/soil amendments in specialty crop production and soil health management. However, such products may enhance survival and persistence of foodborne pathogens and their transfer to fresh produce. Methods: A replicated (n=4) 2-year, randomized complete block field-plot inoculated-challenge study, with PL treatments (composted APL and PP) and cover crop/soil placement, was conducted to assess soil persistence/transfer of generic gE.coli rif-R to cantaloupe, cucumber, spinach, and table radish, in cover-cropped USDA-National Organic Program (NOP) 8-years managed as certified plots. Amended soils were analyzed monthly for E. coli up through 90-120day NOP required wait periods between PL application and produce harvest. Cantaloupe, cucumber, spinach, and table radish, grown compliant with NOP standards, were assayed at harvest for pathogens (Salmonella, Listeria monocytogenes, Staphylococcus aureus) and gE. coli. Results and Discussion: All amended, inoculated soils were positive for the inoculated E. coli (6-log MPN/g) immediately after inoculation. At 90 dpi, all plot soils were positive for the inoculated gEc rif-R ; by 120 dpi, gEc rif-R concentrations in soils declined significantly (p<0.05) both in 2018 and 2019. No other pathogens (Salmonella, Listeria monocytogenes, Staphylococcus aureus) were detected at 0 or 90 dpi in plot soils. Generic Ec rif-R was detected on four of 16 cantaloupes, four of 16 cucumbers from APL plots; four of 20 radish bulbs from till incorporated and four from subsurface-applied APL tested positive for gEc rif-R at 90 dpi. By 120 dpi, only two spinach plants (n=20) were positive from subsurface APL plots; no other pathogens or gEc rif-R were recovered for any crops in 2018-2019. Significant, consistent declines and decreasing survival throughout the growing seasons in populations of inoculated E. coli in soil, resulted in very low detection on any unwashed produce by 90 and 100 dpi in accord with NOP manure amendment-to-harvest wait times. Exposure to a combination of environmental conditions, including soil moisture, splash transfer of soil to produce, and temperatures (soil and air), impact E. coli populations in tilled and subsurface PL product-amended soils and on produce managed in compliance with organic production requirements.
Keywords: Food Safety, E. coli, Salmonella, Listeria monocytogenes, Staphylococcus aureus, poultry litter biological soil amendments (BSAAO), Organic Agriculture, cover crops Food safety
Received: 27 Sep 2024; Accepted: 18 Feb 2025.
Copyright: © 2025 Millner, McKenzie-Reynolds, Hashem, Marsh, Smith, Kenney, Allen, Parveen and Collick. This is an open-access article distributed under the terms of the Creative Commons Attribution License (CC BY). The use, distribution or reproduction in other forums is permitted, provided the original author(s) or licensor are credited and that the original publication in this journal is cited, in accordance with accepted academic practice. No use, distribution or reproduction is permitted which does not comply with these terms.
* Correspondence:
Fawzy Hashem, University of Maryland Eastern Shore, Princess Anne, 21853, Maryland, United States
Disclaimer: All claims expressed in this article are solely those of the authors and do not necessarily represent those of their affiliated organizations, or those of the publisher, the editors and the reviewers. Any product that may be evaluated in this article or claim that may be made by its manufacturer is not guaranteed or endorsed by the publisher.
Research integrity at Frontiers
Learn more about the work of our research integrity team to safeguard the quality of each article we publish.