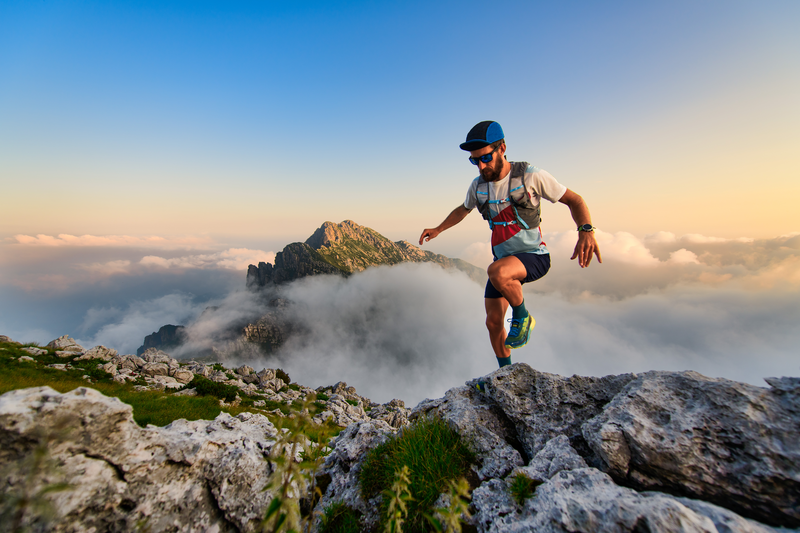
94% of researchers rate our articles as excellent or good
Learn more about the work of our research integrity team to safeguard the quality of each article we publish.
Find out more
ORIGINAL RESEARCH article
Front. Sustain. Food Syst.
Sec. Crop Biology and Sustainability
Volume 9 - 2025 | doi: 10.3389/fsufs.2025.1502362
This article is part of the Research Topic Integration of Legume Intercropping into Sustainable Farming Systems for Nitrogen Fixation, Soil Health, and Climate Resilience View all 5 articles
The final, formatted version of the article will be published soon.
You have multiple emails registered with Frontiers:
Please enter your email address:
If you already have an account, please login
You don't have a Frontiers account ? You can register here
Maize and peanut intercropping can optimize allocation of rainfall through crop canopies, enhancing crop resilience to drought. However, the mechanisms underlying this process remain unclear. This study investigates the impact of strip width on rainfall redistribution to the soil in maize (MS) and peanut (PS) monoculture systems, as well as in intercropping systems with strip configurations of 2:2 (M2P2), 4:4 (M4P4), and 8:8 (M8P8). Results showed that maize/peanut intercropping consistently improved system water use efficiency (WUE) over the three-year experiment, with the M4P4 treatment maintaining the highest WUE throughout. Strip width significantly influenced stemflow and throughfall in maize rows, as well as throughfall in peanut rows, with maize plant height and leaf area playing key roles. Among the 17 rainfall events studied, maize rows in the M2P2, M4P4, and M8P8 treatments obtained 17.4%, 10.8%, and 5.4% more rainfall, respectively, compared to the MS. However, compared to PS, water captured by intercropped peanut rows decreased by 20.6%, 13.2%, and 7.1%, respectively. An edge effect was observed in the intercropping treatments, with stemflow in maize rows increasing by 23.7%, 17.8%, and 14.6%, and throughfall by 12.2% (M2P2), 10.6% (M4P4), and 8.6% (M8P8) compared to MS. Conversely, the M2P2, M4P4, and M8P8 treatments decreased throughfall in peanut by 20.6%, 18.0%, and 16.0%, respectively, compared with PS. Overall, our findings suggest that optimizing strip width in intercropping systems can improve both crop productivity and water management, offering insights for sustainable agricultural practices in regions with limited water resources.
Keywords: intercropping, Canopy, Strip width, stemflow, throughfall, Maize, peanut 9-Sep
Received: 26 Sep 2024; Accepted: 11 Mar 2025.
Copyright: © 2025 Sun, Zhang, Zhang, Yang, Xia and Feng. This is an open-access article distributed under the terms of the Creative Commons Attribution License (CC BY). The use, distribution or reproduction in other forums is permitted, provided the original author(s) or licensor are credited and that the original publication in this journal is cited, in accordance with accepted academic practice. No use, distribution or reproduction is permitted which does not comply with these terms.
* Correspondence:
Guimin Xia, College of Water Conservancy, Shenyang Agricultural University, Shenyang, 110161, Liaoning, China
Liangshan Feng, Liaoning Academy of Agricultural Sciences, Shenyang, China
Disclaimer: All claims expressed in this article are solely those of the authors and do not necessarily represent those of their affiliated organizations, or those of the publisher, the editors and the reviewers. Any product that may be evaluated in this article or claim that may be made by its manufacturer is not guaranteed or endorsed by the publisher.
Research integrity at Frontiers
Learn more about the work of our research integrity team to safeguard the quality of each article we publish.