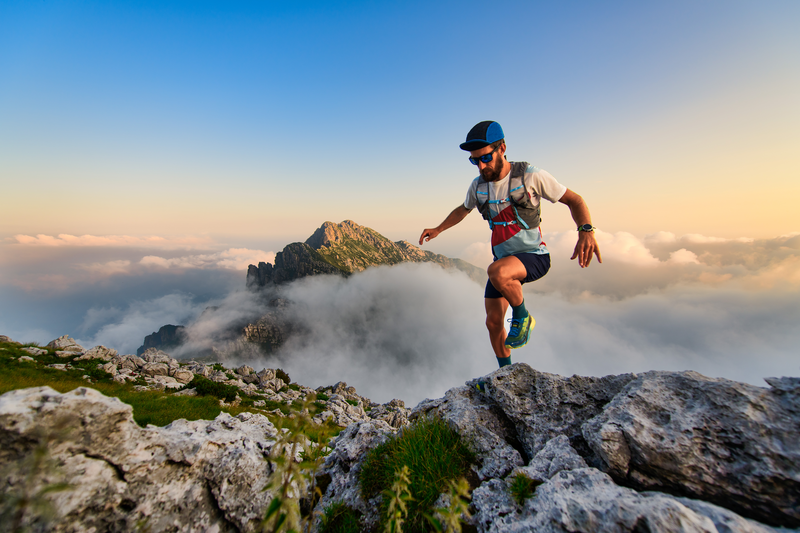
95% of researchers rate our articles as excellent or good
Learn more about the work of our research integrity team to safeguard the quality of each article we publish.
Find out more
REVIEW article
Front. Sustain. Food Syst.
Sec. Climate-Smart Food Systems
Volume 9 - 2025 | doi: 10.3389/fsufs.2025.1499982
The final, formatted version of the article will be published soon.
You have multiple emails registered with Frontiers:
Please enter your email address:
If you already have an account, please login
You don't have a Frontiers account ? You can register here
Climate-smart agriculture (CSA) plays a crucial role in addressing the global impacts of climate change by integrating adaptation and mitigation strategies into agricultural practices. Despite its growing importance, a comprehensive analysis of the CSA research landscape is essential to understanding its development and key trends, informing future research and policy formulation. This study investigates the evolution and current state of CSA research from 2010 to 2023, with a focus on its conceptual structure and thematic development. Data sourced from the Scopus database were analysed using bibliometric techniques. The findings reveal an increasing volume of academic publications, indicating heightened global interest in CSA. The study uncovers strong interconnections among CSA practices, economic implications, sustainability, policy development, practical implementation, and regional adaptation. Notably, there has been a shift from foundational concepts to practical applications, reflecting the field's maturation in response to challenges in agricultural sustainability and climate change mitigation. However, significant gaps persist in exploring CSA's socio-economic dimensions and integrating diverse knowledge systems. Future research should prioritise the socioeconomic impacts of CSA practices on household income and food security, ensuring equitable implementation across regions. Additionally, targeted studies on technology adoption and greenhouse gas emissions mitigation are necessary. Emphasis should be placed on developing context-specific and culturally relevant strategies, analysing the global political economy's influence on CSA, and incorporating nuanced critiques of CSA's effectiveness. This study provides a valuable framework for future research, aiming to enhance the effectiveness and sustainability of CSA in addressing global climate challenges.
Keywords: climate-smart agriculture (CSA), bibliometric analysis, Research trends, Thematic analysis, Climate Change
Received: 22 Sep 2024; Accepted: 31 Mar 2025.
Copyright: © 2025 Manyike, Ningi, Mokhaukhau and Maka. This is an open-access article distributed under the terms of the Creative Commons Attribution License (CC BY). The use, distribution or reproduction in other forums is permitted, provided the original author(s) or licensor are credited and that the original publication in this journal is cited, in accordance with accepted academic practice. No use, distribution or reproduction is permitted which does not comply with these terms.
* Correspondence:
Jabulile Zamokuhle Manyike, University of Fort Hare, Alice, 5700, South Africa
Thulani Ningi, National Agricultural Marketing Council (NAMC), Pretoria, South Africa
Disclaimer: All claims expressed in this article are solely those of the authors and do not necessarily represent those of their affiliated organizations, or those of the publisher, the editors and the reviewers. Any product that may be evaluated in this article or claim that may be made by its manufacturer is not guaranteed or endorsed by the publisher.
Research integrity at Frontiers
Learn more about the work of our research integrity team to safeguard the quality of each article we publish.