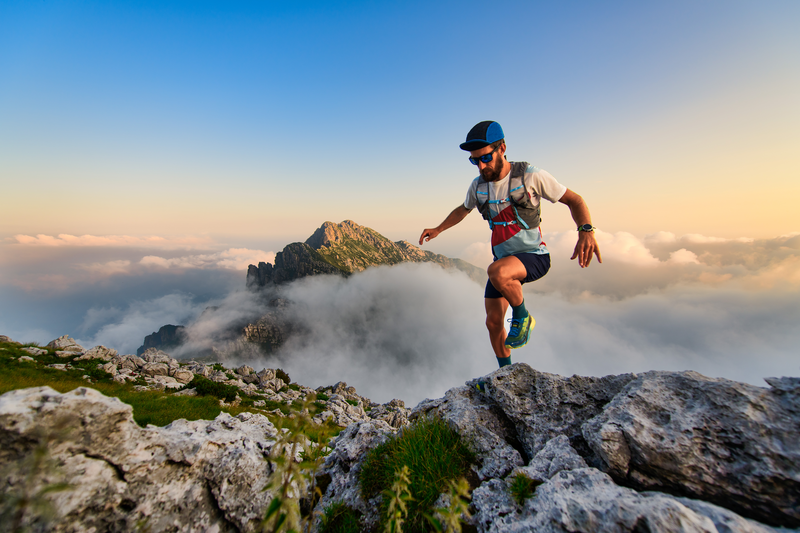
95% of researchers rate our articles as excellent or good
Learn more about the work of our research integrity team to safeguard the quality of each article we publish.
Find out more
SYSTEMATIC REVIEW article
Front. Sustain. Food Syst.
Sec. Nutrition and Sustainable Diets
Volume 9 - 2025 | doi: 10.3389/fsufs.2025.1497609
This article is part of the Research Topic Evidence Synthesis For Sustainable Agriculture And Food Systems View all 4 articles
The final, formatted version of the article will be published soon.
You have multiple emails registered with Frontiers:
Please enter your email address:
If you already have an account, please login
You don't have a Frontiers account ? You can register here
Objective: To establish the current body of evidence regarding the safety of cassava and cassava-based products for human consumption and to identify the most effective preparation methods to reduce toxicity. Introduction: Cassava is a good source of carbohydrates, calories, calcium, vitamins B and C, and other essential minerals. Cassava and Cassava based products are also a major source of income for rural women, small scale farmers, and small businesses in most of sub-Saharan Africa. Given it is consumed by many people in consuming areas globally and will also consider studies that report on ingested toxins related to the consumption of cassava and cassava-based products. Methods: The main databases searched were CABI, PubMed, CINAHL, EMBASE, the Cochrane Database of Systematic Reviews, and the JBI Evidence Synthesis, Science Direct, Agricola, AGRIS, Web of Science, Scopus and Proquest. We limited our search to studies published from 1980. Two reviewers independently reviewed identified titles and abstracts. We reviewed full papers of relevant abstracts. Data was extracted by two independent reviewers and pooled in a statistical meta-analysis for data synthesis.
Keywords: Cassava-based products, cassava, Hydrogen cyanide (HCN), gari, Food Safety, Cassava processing
Received: 17 Sep 2024; Accepted: 28 Mar 2025.
Copyright: © 2025 Forkum, Wung, Tata, Ndum, Alvin, Kamga, Nsaikila and Okwen. This is an open-access article distributed under the terms of the Creative Commons Attribution License (CC BY). The use, distribution or reproduction in other forums is permitted, provided the original author(s) or licensor are credited and that the original publication in this journal is cited, in accordance with accepted academic practice. No use, distribution or reproduction is permitted which does not comply with these terms.
* Correspondence:
Ambang Tatianne Forkum, Effective Basic Services (eBASE) Africa, Bamenda, Cameroon
Disclaimer: All claims expressed in this article are solely those of the authors and do not necessarily represent those of their affiliated organizations, or those of the publisher, the editors and the reviewers. Any product that may be evaluated in this article or claim that may be made by its manufacturer is not guaranteed or endorsed by the publisher.
Research integrity at Frontiers
Learn more about the work of our research integrity team to safeguard the quality of each article we publish.