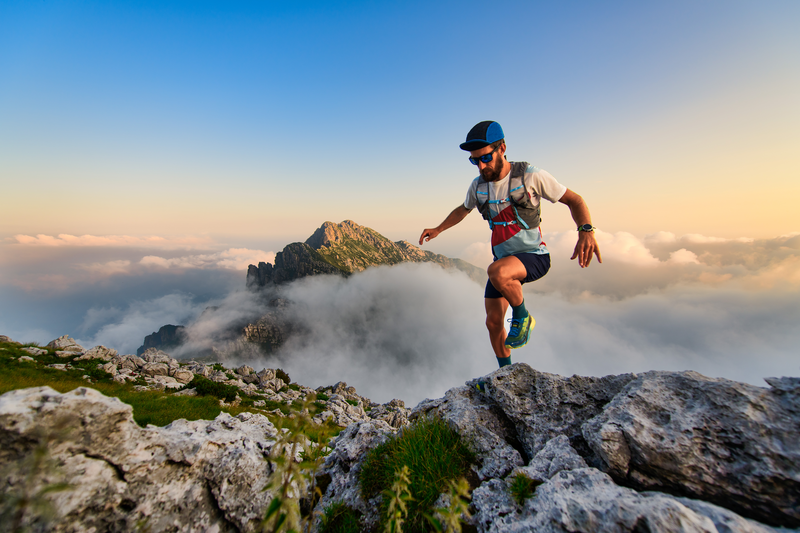
95% of researchers rate our articles as excellent or good
Learn more about the work of our research integrity team to safeguard the quality of each article we publish.
Find out more
REVIEW article
Front. Sustain. Food Syst. , 06 December 2024
Sec. Agro-Food Safety
Volume 8 - 2024 | https://doi.org/10.3389/fsufs.2024.1509384
In sub-Saharan Africa (SSA), many crops are contaminated by Aspergillus section Flavi fungi with highly toxic, carcinogenic aflatoxins. This contamination has severe negative impacts on health, trade, income, and development sectors, hindering progress toward various objectives of most Sustainable Development Goals (SDGs), including SDG 2 – Zero Hunger and SDG 3 – Good Health and Wellbeing. Farmers, industries, and governments need sound aflatoxin management strategies to effectively limit aflatoxin contamination throughout the crop value chain. One effective technology is biocontrol using native atoxigenic isolates of A. flavus coated on a carrier that is applied on growing crops. Atoxigenic A. flavus competitively displaces aflatoxin producers in the field, and this form of bioprotection results in reduced aflatoxin in crops. Over 15 years ago, field tests in Nigeria using a manually manufactured biocontrol product showed promising results. However, it became evident that scaling up the manufacturing process was essential to make this bioprotectant widely accessible to millions of farmers and achieve tangible impact in the context of sustainable food systems. The objective of this paper is to document the evolution of biocontrol manufacturing from small-scale, lab-based production to industrial manufacturing at different scales. The improvements in product formulation, and manufacturing processes and design are highlighted to better address “fit-to-scale” product demand in different countries. Industrializing the manufacturing process coupled with incentivization, commercialization strategies, and effective partnerships has allowed the manufacturing of thousands of tons of biocontrol products. This has enabled production of over a million tons of aflatoxin-safe maize, groundnut, and sorghum, contributing to enhanced food safety and security in several countries in SSA. The key lesson learnt is that for any input-based technology to mitigate aflatoxin (or any food safety issue), their large-scale manufacturing and commercialization is crucial for achieving tangible results. Only widespread adoption of any technology can address the great challenge posed by aflatoxins, a critical step toward meeting several SDGs. The urgency to combat aflatoxin contamination is increasing as its impacts are intensifying in several regions across the globe.
Several input-based innovations with the potential to control aflatoxin contamination exist. However, the utility of an input alone is not sufficient. Its benefits can only be realized if it is manufactured, made available, and adopted at scale. Unfortunately, the need for process manufacturing is often overlooked or inadequately addressed by the developers of most input-based aflatoxin control technologies (Ortega-Beltran and Bandyopadhyay, 2023). Keeping technologies in the category of promising or potential without advancing them for large-scale use will maintain aflatoxin contamination as a challenge for decades. In some cases, university researchers may transfer patents and know-how to private companies or spin-offs from a university. However, certain information and processes in patents may not always be made publicly available, which can limit large dissemination of innovations (Alderucci and Baumol, 2013). In the context of aflatoxin control, slow dissemination of innovations can increase the time for making substantial progress for reducing aflatoxin contamination and exposure. In this review article, we trace our 15-year journey in multiple progressive stages to connect a technological innovation—an aflatoxin biocontrol product—with the market to effectively address the aflatoxin challenge. Achieving the connection required industrial-scale manufacturing of the product.
One of the greatest challenges in sustainably scaling input-based mycotoxin control innovations developed by public institutions is the low private sector investment. These innovations aim primarily to improve public health and the private sector tends to prioritize investments in technologies that demonstrate clear potential for financial returns. This is one of the reasons why the International Institute of Tropical Agriculture (IITA) and its partners not only developed and registered the aflatoxin biocontrol products in multiple countries (i.e., Nigeria, Kenya, Senegal, The Gambia, Burkina Faso, Ghana, Tanzania, Mozambique, Malawi, Zambia, Mali, Rwanda, and Democratic Republic of Congo) but also took additional steps to enable large-scale adoption. Manufacturing an input-based innovation is a key step in scaling (Box 1). The lessons from these efforts described in subsequent sections can guide the scaling of other input-based innovations for overcoming the serious challenges posed by aflatoxins in tropical and subtropical regions, as well as in temperate regions now frequently experiencing contamination events due to climate change (Logrieco et al., 2021; Camardo Leggieri et al., 2021; Miller, 2023). By sharing this narrative in the public domain, we hope to contribute to further advances in aflatoxin biocontrol/mitigation efforts, and other critical areas of food safety as well. Our ultimate goal is to benefit people globally who depend on crops prone to food safety hazards, including aflatoxin. To maintain the thematic coherence in this article, we have sometimes presented actions out of chronological order. It is important to recognize that despite several success stories, many structural challenges—some still unresolved—continue to hinder greater adoption of the aflatoxin biocontrol technology in sub-Saharan Africa (SSA) countries.
BOX 1 Aflatoxin biocontrol in practice requires a multi-disciplinary, multi-year effort.
Making products available for widespread use in each country where the Aflasafe Initiative operates has required the following:
• Develop baseline data on aflatoxin prevalence and causal agents of contamination.
• Detect native candidate atoxigenic genetic groups of Aspergillus flavus and select the most superior ones as active ingredients of biocontrol products.
• Conduct farmer field efficacy trials and enhance local research capacity.
• Satisfy regulatory requirements to obtain registration and, if necessary, strengthen capacity of local regulator.
• Conduct market analysis, explore commercialization options.
• Mobilize the private sector by selection of manufacturer and/or distributer partners, and awareness raising.
• Test the market with investor, conduct large-scale demonstration and awareness raising.
• Collaborate with investors to manufacture and distribute the biocontrol product, build awareness, improve the product.
Describing in detail all elements of the process is not possible due to the large amount of information that has been generated. We touch certain aspects of several of those topics, but we primarily focus on the manufacturing processes necessary for scaling up product availability. The convergence with other practical and effective approaches is essential for mitigating aflatoxins. Other components of the process have been detailed in several publications, most of which are referenced in the text.
In tropical and subtropical regions, aflatoxin contamination of staple crops caused by fungi from Aspergillus section Flavi, especially A. flavus, is a common problem. The contamination negatively impacts health, productivity, income, trade, and development, directly hindering progress toward various objectives of most Sustainable Development Goals (SDGs), including SDG 2 – Zero Hunger and SDG 3 – Good Health and Wellbeing (Wu, 2015; Ortega-Beltran and Bandyopadhyay, 2021). Crops in many regions across SSA are prone to aflatoxin contamination due to the co-existence of favorable environmental conditions and the presence of highly toxigenic species (Williams et al., 2004; Probst et al., 2014; Logrieco et al., 2021). Most farmers in SSA lack access to organized, commercial agriculture, and when opportunities arise, they struggle to meet stringent aflatoxin standards for domestic or international premium markets (Udomkun et al., 2017a). While it is possible to produce crops that meet stringent aflatoxin standards in SSA using integrated management systems (Ortega-Beltran and Bandyopadhyay, 2021), institutional, infrastructural, and logistical barriers prevent farmers from accessing the available aflatoxin management technologies (Udomkun et al., 2017b; Wu et al., 2008).
An effective strategy to limit aflatoxin contamination is to use biocontrol products containing atoxigenic (i.e., unable to produce aflatoxins) isolates of A. flavus as active ingredients (Mehl et al., 2012). Atoxigenic isolates are relatively common in nature. Aflatoxin biocontrol was pioneered in the US by the United States Department of Agriculture – Agricultural Research Service (USDA-ARS) (Cotty et al., 2007). In the US, two biocontrol products are registered with the US Environmental Protection Agency (US-EPA): Aspergillus flavus AF36 and Afla-Guard® (Cotty et al., 2008; Dorner, 2009). The Arizona Cotton Research and Protection Council (ACRPC), a farmer-driven organization, produces AF36. Syngenta®, a transnational, produces Afla-Guard®. Each product contains as active ingredient a different isolate of A. flavus belonging to an atoxigenic genotype common across the US. The products are used in cotton, maize, groundnut, pistachio, almond, and fig grown in aflatoxin-prone areas of the US. Their use results in a significant reduction in aflatoxin content—80 to 100% less than crops from untreated neighboring fields. These bioprotectants are effective if applied at the optimal time (2 to 3 weeks before flowering) using the correct dose (10 kg/ha). Aflatoxin reductions are even more pronounced when biocontrol is combined with other pre- and post-harvest interventions that discourage aflatoxin formation, such as planting resistant varieties, timely harvest, sorting, drying, and using hermetic storage.
AF36 was initially produced using an industrial process that enabled the manufacture of large quantities (dozens of tons per day) (Cotty et al., 2007). The original formulation of AF36 was manufactured by inoculating autoclaved wheat with a suspension of the active ingredient fungus, incubating the inoculated wheat for 18 h to allow for relatively low colonization, and then drying the product to halt further fungal growth, before packaging. The manufacturing process has since evolved. Instead of wheat, sorghum is used as substrate and carrier for the biocontrol isolate and a dry, less laborious, and less expensive process is used. This formulation is named AF36 Prevail®. A similar transition in formulation and manufacturing is explained later in the context of the African biocontrol products, collectively known as Aflasafe®.
The aflatoxin biocontrol technology has been adapted and improved for use in SSA through a team effort among IITA, USDA-ARS, and several national and international institutions (Bandyopadhyay et al., 2016). This partnership, known as the Aflasafe Initiative, began in 2003. The first product developed through this initiative, Aflasafe™, was designed for use in Nigeria and contains as active ingredient fungi four atoxigenic isolates of A. flavus native to the country’s major maize- and groundnut-producing regions (Atehnkeng et al., 2014, 2016). These isolates possess natural genetic defects in the aflatoxin biosynthesis gene cluster, which prevent them from producing aflatoxins (Adhikari et al., 2016). As in the US, when applied in the field at the right crop stage and dosage, Aflasafe consistently and effectively reduces aflatoxin content (Bandyopadhyay et al., 2019). In Nigeria, over 400,000 ha have been treated with Aflasafe, with over 95% of the treated crops showing safe aflatoxin levels.
Substantial progress has been made in Nigeria since 2003, including transferring the technology to the private sector (Schreurs et al., 2019; Ola et al., 2022). The technology has also been developed, registered, and transferred to private sector partners in Senegal, Tanzania, and Mozambique as well as to the public/private sector in Kenya (Ortega-Beltran and Bandyopadhyay, 2023). Product development, testing, registration, and transference are currently at different stages in 23 SSA countries (Box 2). This long journey had modest beginnings in the laboratory to identify candidate atoxigenic A. flavus isolates and to produce a few kilos of biocontrol product for the initial efficacy and demonstration trials followed by extensive interactions with regulators to achieve registration, multidisciplinary efforts to design the industrial manufacturing process, and a well defined approach to technology transfer.
BOX 2 Pathway followed by the Aflasafe Initiative for scaling aflatoxin biocontrol products and progress across 23 countries in Africa.
Effectively addressing each stage (X-axis)—through research, delivery, and partnership actions—is necessary for successfully scaling the atoxigenic-based biocontrol technology. In the final stage, there is ongoing collaboration among partners, making this dynamic process continually categorized as ‘Being Implemented’.
In this descriptive article on our long journey from product development to commercialization, we focus on multidisciplinary efforts to design the industrial manufacturing process and a meticulously structured approach to technology transfer. First, we summarize the modest beginnings that started in the laboratory to identify active ingredient fungi for biocontrol products and to produce a few kilos of biocontrol product for the initial efficacy and demonstration trials. Extensive interactions with regulators to gain registration and outcomes of demonstration trials pointed toward the need for commercial manufacturing of the products in Africa. We then highlight research on elements necessary for adapting and improving an industrial process as a precursor to designing a manufacturing plant for aflatoxin biocontrol in Africa. Next, we describe the design and operationalization of the first industrial-scale aflatoxin biocontrol manufacturing plant in Nigeria followed by a modular manufacturing system in Kenya. Additionally, we highlight how these experiences were leveraged to establish biocontrol manufacturing in other countries. After demonstrating that commercial-scale manufacturing is possible in Africa, we discuss the commercialization process to attract manufacturing and distribution partners for the scaling-up process that enabled thousands of farmers to adopt aflatoxin biocontrol in Africa. Finally, we describe actions taken and offer suggestions to reduce the cost of biocontrol. Manufacturing of aflatoxin biocontrol at scale in African countries is contributing to safer crops for better health, and increased income, trade, and food security. We believe that such efforts are key to addressing the serious challenge posed by aflatoxins.
In 2003, the German Government funded a project to sample maize and soil from major maize-producing regions of Nigeria. The project provided an opportunity to train students from Cameroon and Germany in characterizing Aspergillus communities. It also importantly strengthened Nigeria’s technical capacity to conduct chemical and microbiological analyses. Through those efforts, >4,200 Aspergillus isolates were collected, and those with defects in the aflatoxin biosynthesis gene cluster were identified. Twenty vegetative compatibility groups (VCGs) composed exclusively of atoxigenic members to potentially constitute a biocontrol product were shortlisted (Atehnkeng et al., 2008a; Donner et al., 2009, 2010). After on-station field testing during 2007–2008 and analyzing the environmental distribution of the candidate fungi, four superior atoxigenic VCGs were selected to constitute the bioprotectant Aflasafe (Atehnkeng et al., 2008b, 2014, 2016). In 2009, Nigeria’s National Agency for Food and Drug Administration and Control (NAFDAC) provisionally registered the product, allowing large-scale testing of the laboratory-manufactured product in many farmers’ fields from 2009 to 2012 (Bandyopadhyay et al., 2019).
Before manufacturing in the laboratory, extensive research was conducted to identify the most suitable and practical grain as a nutritive source and carrier for the biocontrol fungi. Wheat or barley grains were typically used for the products manufactured in the US. Grains of either crop are too expensive in SSA. Sorghum, which is widely available and cheaper than wheat and barley, is a more viable option in SSA, especially since A. flavus thrives on this substrate, particularly white sorghum (unpublished). Additionally, sorghum’s smaller grain size allows for better distribution across treated fields compared to wheat and barley (Bandyopadhyay et al., 2022). While other substrates, such as pellets made from cassava peels (Okike et al., 2015) were explored, none outperformed sorghum. Consequently, sorghum grain was chosen for practical and economic reasons.
In between 2007 to 2013, over 50 tons of biocontrol product were manufactured in Pathology/Mycotoxin Laboratory and Food and Nutrition Science Laboratory at IITA headquarters in Ibadan, Nigeria for conducting on-station and farmers’ field trials. The manufacturing process followed in Ibadan was a simplified version (Figure 1) of the original industrial manufacturing process for AF36, which relied on several machinery and instruments. This process was labor- and electricity-intensive, taking several days to complete. About 300 kg of biocontrol product were manufactured in the laboratory through a preparatory period of at least 2 weeks. The process began with growing the active ingredient fungi for 7 days in dozens of Petri dishes, followed by preparation of several liters of a spore suspension (106 spores/mL) of each isolate. White sorghum grain was manually cleaned, soaked in water for 2 h, drained, and autoclaved at 15 psi for 45 min in polyethylene bags (45 × 20 cm). After cooling, 1-kg batches of sterile grain were seeded with 100 mL spore suspension of a single atoxigenic isolate, mixed to ensure even distribution, and incubated for 18 h at 31°C to promote fungal colonization of grain surface but without visible growth (Atehnkeng et al., 2014). Then, grains were dried in cotton cloth bags (4 days, 50°C) to halt fungal growth. Several batches were prepared for each active ingredient isolate. Finally, the product was formulated by combining 2.5-kg batches of each of the four isolates in a polyethylene bag and shaking it by hand to ensure uniform distribution of the four active ingredients. The final product was placed in 5-kg or 10-kg plastic containers, sealed, and stored at room temperature until needed (Atehnkeng et al., 2014).
Figure 1. Diagram showing the eight-step laboratory-scale process used to manufacture the aflatoxin biocontrol product Aflasafe during the initial years (2007–2012) of effectiveness trials in Nigeria, Senegal, Kenya, among other countries.
The lab manufacturing process effectively produced sufficient material for initial efficacy trials and market linkage studies. However, it proved inefficient, time-consuming, expensive, and slow for larger-scale production. To address the increased demand during the factory construction period from 2012 to 2014 (detailed later), the intermediate manufacturing processes (Figure 2) described in a subsequent section were introduced allowing for the production of up to 600 kg/day.
Figure 2. Intermediate processes used in 2013 and 2014 before the industrial manufacturing of aflatoxin biocontrol products in Nigeria began in 2014. Initially, sorghum grain was manually cleaned, roasted in a kitchen oven, and then manually-coated with spores of the active ingredient fungi by several people in the laboratory. The product was then weighed and packaged manually [Process (A)]. The process later evolved, with up to 600 kg of manually cleaned sorghum grain being roasted per day in a cabinet drier. The heat-killed grain was then coated with spores using a manually operated drum mixer, followed by weighing and packaging manually [Process (B)].
In 2009 and 2010, the trials conducted in Nigeria evaluated the efficacy of a biocontrol product to reduce aflatoxin contamination in 130 maize and 48 groundnut fields. While the testing expanded in 2011 and 2012 on a modest scale, its focus shifted toward connecting farmers with buyers who were conscious of aflatoxin safety, assessing both the farmers’ willingness to adopt the technology and whether food/feed processors would offer premium prices for aflatoxin-safe crops (Bandyopadhyay et al., 2019). While there was interest, scaling the manufacturing process of the biocontrol product posed a major challenge. The existing lab-based production methods were insufficient to treat large areas, meaning only a limited number of hectares could be treated in any given country under the current system. To achieve widespread impact, a more efficient production method was required.
In 2010, the Bill and Melinda Gates Foundation (BMGF) engaged Doreo Partners1 to assess the private sector’s demand for the biocontrol product and conduct a market survey of potential manufacturers. In 2011, Doreo Partners reported that several farmer organizations expressed demand for the product, and investors showed interest to manufacture it. The possibility of using biocontrol to mitigate aflatoxin in Nigeria and other SSA countries was becoming evident. However, it was clear that meaningful impact was possible only with an industrial-scale manufacturing process and the establishment of a manufacturing plant.
The availability of a functional, certified manufacturing facility is also a prerequisite for NAFDAC to approve unrestricted registration of a biocontrol product. However, the complex and costly design of the world’s first aflatoxin biocontrol manufacturing facility in Phoenix, Arizona, was difficult to replicate in Nigeria and other SSA countries. As noted earlier, manufacturing the product involved an extensive process of autoclaving sorghum grain, inoculating it with the biocontrol fungus, incubating it, and drying the formulation before packaging. The introduction of water for sterilization and incubation for fungal colonization necessitated the removal of water in subsequent steps to ensure long storability and high quality. The wet process relied on pressure vessels for steam sterilization, heavy machinery and instruments, several days of processing, and intensive electricity use. Therefore, it was necessary to develop a more efficient, dry manufacturing process, and build a manufacturing facility practical for SSA, which NAFDAC could certify.
In 2011, USAID funded a CGIAR-US University Linkage Grant to develop cost-effective options for biocontrol manufacturing to enable its large-scale adoption in SSA. Before the approval of the Linkage Grant, USDA-ARS in Tucson had been collaborating with ACRPC in Phoenix to develop a new dry process aiming to circumvent the heavy equipment-dependent, and resource-intensive wet process available at that time. Through that Linkage Grant, the Aflasafe Initiative used lessons from the USDA-ARS/ACRPC collaboration to develop elements of a dry manufacturing process adaptable for use in SSA. To adapt the game-changing dry process, a series of experiments were conducted in Tucson and Ibadan that:
• Evaluated different sorghum types for their performance as carrier and nutrient source for active ingredients in the formulations.
• Demonstrated that sorghum sterilization is possible by dry heat delivered by a grain roaster.
• Standardized the temperature and duration of dry heat needed to sterilize and kill the grains, which serve as a carrier and food sources of the active ingredients during field deployment.
• Determined that hot roasted grains must be cooled before coating with the active ingredients.
• Showed that mixing the active ingredients with the sterile sorghum grain using a seed coater is an efficient and rapid method of delivering the inoculum on the carrier.
• Identified a polymer sticker that aided in the coating of the active ingredients on the carrier, and a food dye that was best able to differentiate treated grain from regular sorghum grain.
• Developed methods to scale up the production of spores of the active ingredients through simple sorghum fermentation processes.
• Established faster, less laborious quality control methods to measure the quantity of active ingredients spores before coating on the sterile sorghum grains and in the final product.
• Demonstrated that batches of sorghum grain coated with four strains simultaneously performed similarly to those in which each strain was coated individually as done in the laboratory-scale process.
• Assessed the shelf-life stability of product manufactured using grain-coating technology under varying storage conditions showing stability for over two years under ambient conditions. Later research showed that the product remained active for at least four years in storage (Ortega-Beltran et al., 2024).
Some elements described previously are crucial for developing effective microbial-based bioprotectants. For example, Rocha et al. (2019) argued that seed coating using seed treaters is an efficient alternative to deliver microbial biocontrol agents. Gressel (2023) considered inexpensive production of inoculum and an extended product shelf-life as two of the four pillars for successful fungal-based products. Teixidó et al. (2022) elaborated on the need for stable, easy-to-apply products and that different formulation and packaging processes must be tested and validated.
The information generated through the Linkage Grant and prior work done by USDA-ARS and ACRPC on grain sterilization and inoculation provided the foundational knowledge for designing a low-cost, rapid, dry process that could be optimized for biocontrol manufacturing at different scales. The most effective process eliminated the need for autoclaving, inoculation, incubation, and drying steps previously required in the formulation process. The new dry process simplified the wet process by the following major steps:
• Roasting: Dry heat sterilization and killing of the carrier using a grain roaster replacing the traditional moist heat sterilization (autoclaving).
• Cooling: Rapid cooling of the hot sterile carrier to an appropriate temperature with forced sterile air to prevent water condensation.
• Seed coater: Spores of the atoxigenic active ingredients are uniformly distributed on the cooled carrier surface using a commercial seed treater.
Laboratory tests concluded that the laboratory-scale product and the new formulation yielded the same number of spores when incubated in moist chambers. In addition, both formulations were equally effective in limiting aflatoxin contamination in farmer field trials (Bandyopadhyay et al., 2019). Thus, the more efficient dry process was adopted for implementing commercial manufacturing of Aflasafe in Nigeria and later in other SSA countries. Meanwhile, ACRPC and USDA-ARS collected data on several aspects of the new dry process over several years in the US. Due to the simplicity, efficiency, effectiveness, and speed, ACRPC registered the improved dry process with US-EPA to produce AF36 using the simplified dry process in Phoenix. This new AF36 formulation is known as AF36 Prevail®.
In September 2011, a delegation of BMGF leadership, led by its co-founder, visited farmers’ fields in Kano, Northern Nigeria, to observe various technologies implemented by IITA, including Aflasafe, and to hear both farmers’ and scientists’ perspectives on utilities of those technologies. Details of this visit have been described (Bandyopadhyay et al., 2022), but for context in this document, relevant background information is provided. The delegation recognized the potential of Aflasafe to reduce aflatoxin in Nigeria and elsewhere, but emphasized that the achievement of considerable impact would depend on several factors. Some of the key factors were (i) the capabilities to manufacture the product at scale, (ii) innovative mechanisms to make the product available to farmers, (iii) creation of market opportunities for aflatoxin standard-compliant crops, and (iv) building awareness about aflatoxins and methods to decrease contamination (Bandyopadhyay et al., 2022).
Following that visit and given the negative impact of aflatoxin on health and trade in SSA, BMGF consulted with various development partners and stakeholders to develop a comprehensive program for aflatoxin control in Africa. These consultations culminated in BMGF’s decision to establish a multi-institutional and multi-dimensional program aimed at understanding aflatoxin issues as developing policies and promoting technologies with high potential to reduce the aflatoxin burden in African food systems. In November 2011, BMGF approved the program, called the Partnership for Aflatoxin Control in Africa (PACA), led by the African Union Commission at its headquarters in Addis Ababa, Ethiopia. Three interconnected components of Aflasafe, co-led by IITA and USDA-ARS, were included in the PACA program: (1) development of new biocontrol products for key countries such as Ghana, Mali, and Tanzania; (2) establishment of a manufacturing plant in Ibadan to produce Aflasafe at scale; and (3) further simplification of the manufacturing process, which led to the concept of modular manufacturing.
At that time, the biocontrol product had been tested and provisionally registered in Nigeria. Donors and Governments were supporting the Aflasafe Initiative’s work to replicate and adapt the technology in several SSA countries. However, as mentioned previously, the missing piece was an industrial-scale manufacturing facility located in Africa. BMGF provided funds to build a prototype demonstration-scale manufacturing plant at IITA-Ibadan. IITA and USDA-ARS also developed and optimized a design for turnkey manufacturing facilities for biocontrol products, initially at a small scale, but with the ability to scale up quickly and constructed at lower cost across SSA as demand for biocontrol increased in various countries (Bandyopadhyay et al., 2022). Emphasis was placed on procuring and installing easily accessible manufacturing equipment while using an improved dry manufacturing process.
Another objective of building the facility was to enable further research on cheaper, more effective formulations and processes (see dry spore section in a subsequent paragraph). The facility also allowed for the large-scale production of Aflasafe, which helped lay the groundwork for building awareness, demand, and aggregation channels for low-aflatoxin crops.
The demonstration plant served as a model that could be adapted in other SSA countries where biocontrol research was either at different stages of development or about to begin. It was critical to develop easily transferable systems for commercial manufacturers to produce large amounts of biocontrol products in various countries to meet market demands (Bandyopadhyay et al., 2022). Additionally, once biocontrol products were available at scale, development investors and governments were able to earmark funds for the purchase and distribution of these product to smallholder farmers in addition to commercial demand from maize and groundnut value chains.
It took about 18 months to build a functional facility in Nigeria because it had to be constructed, and equipment procured, installed, and fine-tuned. Finally, regulatory approval had to be obtained. As a result, the efficient manufacturing process of the under construction facility had to first be prototyped at a small scale—an intermediate step between the laboratory-scale and industrial-scale processes. The growing demand for biocontrol, which the lab-scale process could not meet, prompted the development of the process of the intermediate step. This process allowed us to fulfill the rising demand for the product.
There were two intermediate processes, both using makeshift arrangements, based on the findings of the Linkage grant mentioned previously. The first involved sterilizing the sorghum grain by roasting it in a kitchen oven (~60 kg/day), cooling the grain, followed by coating the grains with a spore suspension. Several people manually shook grains with spore suspension in plastic containers to coat the grains (~20 kg coated/10 min) and packaging them (Figure 2A). The second process had a higher capacity: sorghum grains were sterilized in a large cabinet drier belonging to IITA’s Cassava Breeding Unit (~600 kg/day), coated with the spores using a manually operated 25-liter drum (~20 kg coated/10 min), and manually packaged (Figure 2B). These intermediate processes enabled us to meet the increasing demand in several countries (e.g., Nigeria, Senegal, and Burkina Faso). Appropriate precautions were taken during critical steps (e.g., coating of grains with spores and packaging) in the manufacturing process to ensure product integrity. Purity of the active ingredients was further confirmed through VCG analysis. Necessary approvals were taken from plant quarantine authorities of the exporting (Nigeria) and importing countries.
Most of the equipment selected to produce the biocontrol product are commonly used by seed and food processing industries in Nigeria and elsewhere (Bandyopadhyay et al., 2016). Emphasis was placed on the types of manufacturing equipment that could be easily procured and installed to operationalize the dry manufacturing process. This process relied on the previous foundational work done in the US, the results of the USAID Linkage Grant, plus the experiences of scaling the intermediate processes described previously. The manufacturing plant in Nigeria was designed to have the following in sequence:
• A grain intake facility for unloading white sorghum grain meeting quality standards (i.e., size and purity) and conveyed into a grain debearder for threshing any unthreshed grains to remove sorghum ‘caps’, and then conveyed to a combined pre- and fine-cleaning machine.
• A grain cleaner containing a scalperator for scalping, re-scalping, and aspiration (pre-cleaning) to remove roughage, light fines, and chaff (fine cleaning) in a single step.
• A weighing machine to determine the quantity of clean sorghum grain, which is used to calculate payments to grain suppliers based on the weight of clean grain delivered.
• A 100-ton silo to temporarily store the clean grain prior to sterilization.
• An industrial grain roaster that uses gas flame to sterilize and kill the grain. Grains are sterilized by passing through the gas flame (~2,500°C) for a fraction of a second, raising the temperature of the carrier to approximately ~140°C. This gas-powered roaster was chosen for Nigeria due to the low cost and easy availability of natural gas.
• A cooling system to lower the temperature of the hot grain with sterile air, preventing condensation and contamination. A high-efficiency (99.99%) particulate air (HEPA) filter provides sterile air that circulates through the silo for cooling.
• Silos of 50-ton capacity to store the sterile grain. A grain conveying system transfers the cooled sterile grain to the storage silos.
• A conveyor system to move sterile grain from the silos to the seed treater, or transfer directly from the cooler to the treater when small quantities of product are required.
• A commercial seed treater for coating the grain carrier with the active ingredient fungi and the other ingredients (polymer and dye).
• A conveyor to move the formulated product to a bagging unit.
• A bagging unit containing electronic scales to fill bags with desired product content (e.g., 2.5 kg, 5 kg, 10 kg).
• A sealer to hermetically seal the bags.
• A bag printer to imprint the products’ batch code, manufacturing, and expiring date.
Considering the large area in Nigeria where aflatoxin-prone crops are grown, the factory was designed with equipment capable of producing 5 tons of product per hour. Several companies in Africa and in the US were consulted to obtain the most appropriate equipment, at competitive prices, and shorter delivery times. Eventually, this archetype was modified (see subsequent section).
In early 2013, the Aflasafe Initiative secured a site within IITA-Ibadan premises to start constructing the first aflatoxin biocontrol manufacturing facility in Africa, and the third globally. One part of that area (a covered shed attached to, but outside the main building) was being used as a dump yard, while the main building had two sections, one for storing fertilizers and the other for pesticides, separated by a corridor (Figure 3). Draft plans were prepared based on the available space and manufacturing requirements. The plant was designed with four distinct sections:
• Section 1. Grain intake, cleaning, and sterilization.
• Section 2. Grain cooling and storing.
• Section 3. Laboratories to produce spores of the active ingredients, and quality control.
• Section 4. Production hall for coating the sterile sorghum grain with the active ingredient spores, polymer, and dye, and packaging.
Figure 3. Premises in IITA-Ibadan, where the first aflatoxin biocontrol manufacturing facility in Africa—and third globally—was constructed. Part of the site was previously used as a dump yard (A). The main building had one section for storing fertilizers and another for pesticides, separated by a corridor (B). Construction began in June 2013 and completed in June 2014 (C), and operated until December 2020. In 2021, the facility was dismantled (D) after the private sector company HarvestField Industries Ltd. (HIL) was licensed by IITA to commercially produce Aflasafe for Nigeria. HIL purchased most of the equipment from IITA to install in their new facility (E,F) in Mowe, Ogun state, Nigeria.
Section 1 was positioned outside the main building, replacing the covered shed, to isolate the main building from: (i) the physical and microbial contaminants present in the grain and generated during grain cleaning, and (ii) the heat and fumes generated during sterilization and cooling. Section 2 was placed close to sections 1 and 4 with the cooling unit adjacent to the sterilizer and the storage silos outside the building. The former chemical store was repurposed for the aseptic microbiology laboratories; section 3. The former fertilizer store became the manufacturing and storage hall, section 4, which included a dedicated room for mixing inoculum, polymer, and dye before coating. Additionally, the facility featured an underground sewage tank ensuring proper disposal of factory wastes. Offices were provided for the plant manager, supervisor, and laboratory manager, along with a control room to monitor the manufacturing process. The facility also included restrooms and shower rooms for the factory and laboratory workers.
Based on the accepted draft plan, several detailed layouts were created for the laboratory and factory, specifying the exact equipment positions (Figure 4). The manufacturing process was designed to accommodate both batch and continuous processing, with the latter having demonstrated several advantages in other industries (Lee et al., 2015). This versatility allowed for adjustments in time, labor, cost, and flexibility depending on production volume or production process. These plans were shared with partners and equipment manufacturers for feedback before creating working drawings. Competitive tenders were thereafter floated for the civil works and procurement of both laboratory and factory equipment. The construction began in June 2013 and ended in June 2014 (Figure 3).
Figure 4. Blueprint of the demonstration-scale manufacturing plant with four sections: Blue area (220 m2) – grain intake, cleaning, and sterilization. Green area (145 m2) – laboratories for producing active ingredient spores and quality control. Pink area (196 m2) – silos to store clean grain (stand-alone silo) and roasted, sterile grain (group of three silos). Red area (440 m2) – production hall for coating sterile sorghum grain with the active ingredient spores, polymer, and dye, followed by packaging.
Once the facility was completed, large-scale adoption efforts for the product began under the Nigeria AgResults Aflasafe Pilot Project.2 The project converged several elements of aflatoxin management and relied on a pull mechanism where public or private enterprises (implementers) received incentives only if they successfully worked with farmers to produce aflatoxin-safe maize using Aflasafe (Bandyopadhyay et al., 2022). The project began in 2013 and ended in September 2019. During this period, >750 tons of the product was produced in the demonstration plant and > 75,000 smallholder farmers used it to produce >315,000 tons of aflatoxin standard-compliant maize.
The factory supported initial commercialization activities in Nigeria, and produced country-specific products for use in other West African countries such as Senegal, The Gambia (Senghor et al., 2021), Ghana (Agbetiameh et al., 2020), and Burkina Faso (Bonkoungou et al., 2024), which were transported by road or by sea freight. In addition, in 2015, the Government of Kenya ordered 270 tons of Aflasafe KE01, a Kenya-specific product developed through a collaboration among USDA-ARS, IITA, and Kenya Agricultural and Livestock Research Organization (KALRO), to treat maize in aflatoxin-prone areas. Eight tons were airlifted because of the urgent need for application in one area, while the remaining 262 tons were transported via sea freight (Bandyopadhyay et al., 2016). The use of Aflasafe KE01, manufactured in Ibadan, had significant positive impacts on the supply of safe food to vulnerable people (Bandyopadhyay et al., 2022). Nevertheless, the transport of large quantities of bulky products proved costly, highlighting the necessity of local production a must. The Ibadan facility sparked interest among private sector entities to invest in the technology and for various stakeholders (researchers, government officers, donors, ministers of agriculture, among others) to consider developing biocontrol products for their respective countries.
All results from farmer field efficacy and market linkage trials conducted between 2009 and 2012 were summarized, packaged into a registration dossier, which also included toxicological and eco-toxicological data from biocontrol products registered in the US. The dossier was then submitted to NAFDAC for full registration (Bandyopadhyay et al., 2016). NAFDAC also requested toxicological data demonstrating the safety of the product for birds fed with biocontrol-treated maize (Aikore et al., 2019).
In 2014, NAFDAC granted registration for both the Aflasafe product and the manufacturing process including the facility. NAFDAC staff periodically inspected the construction offering valuable inputs to ensure that the facility met the required specifications. The manufacturing section of the dossier detailed the nine quality assurance tests conducted to ensure the manufacture of high-quality biocontrol products (Supplementary Figure S1).
A manufacturing facility in Kenya became necessary due to the increasing demand for aflatoxin biocontrol products. It was economically unviable to manufacture the product in Ibadan and transport it to Kenya. However, establishing a manufacturing facility in Kenya with the same production capacity as the one in Nigeria would not be sustainable given the initial demands. The demonstration plant in Ibadan had a capacity too large for the initial demands in any country. The Ibadan plant could manufacture 40 tons of product in an 8-h shift, which can treat 4,000 ha. For initial demands, say 500 tons/year or less, production could be completed in just a few weeks, leaving the equipment idle for most of the year. Idle equipment deteriorates quickly and is uneconomical to maintain. It was necessary to adopt a scale-to-fit approach for Kenya, starting with a smaller production capacity and increasing it gradually as demand for the product grew.
The business principle of starting small and gradually expanding as the demand exceeds supply led to the introduction of modular factories, which allow manufacturers to build plants that can increase capacity as demand grows. These plants consist of four modules (Figure 5), briefly described as follows:
• Module I&C – Intake and Cleaning. White sorghum grain meeting quality standards is unloaded and cleaned to remove sorghum ‘caps’, roughage, light fines, and chaff.
• Module R – Roasting. High temperature roasting rapidly sterilizes and kills sorghum grains and prepares it for coating. Both gas-fired (e.g., those used in feed industry) and electric roasters (e.g., peanut or soybean roaster) heat grains to temperatures that kill grains and microbial contaminants. The hot grains are transferred to a holding bin where they are incubated for 10 min to prolong the hot period of the roasted grain. The roasted grains are then cooled with sterile air in a mechanically vented silo to avoid water condensation.
• Module C – Coating. Sterile sorghum grains are coated with active ingredients, a blue food dye, and a polymer using a seed treater, producing the formulated Aflasafe product.
• Module P – Packaging. Aflasafe is packaged in plastic bags of various distribution sizes (e.g., 2 kg, 2.5 kg, 5 kg).
Figure 5. Construction of the first modular aflatoxin biocontrol manufacturing facility in Katumani, Kenya is depicted. The plant consists of four modules (from left to right): grain intake and cleaning (in black), roasting and cooling system (red), seed treater (blue), and packaging (brown). The number or throughput of any of the low-capacity modules (e.g., roaster) can be increased to augment production as demand for the product grows (A). Intake and cleaning equipment, and roasting unit (B). HEPA filter and bulk cooling silo (C). Seed treater and packing unit are visible (D). An exterior view of the completed plant (E).
As in the demonstration plant, off-the-shelf equipment from the seed, food, and feed processing industries are used. To expand production as demand increases, one or more of the four rate-limiting out-of-sync modules can be upgraded or added to. The first modular plant was built in Katumani, Machakos County, Kenya through a collaboration between IITA, USDA-ARS, and KALRO (Figures 5, 6). Bandyopadhyay et al. (2022) described this modular plant as having three modules with the grain intake and cleaning section considered part of Module R. However, it is more appropriate to consider the grain intake and cleaning part as a separate Module, I&C, because it is structurally and functionally distinct from Module R.
Figure 6. Manufacturing facilities constructed by HarvestField Industries Limited in Nigeria (A,B), BAMTAARE SA in Senegal (C), A to Z Textiles Limited in Tanzania (D), and Kenya Agricultural and Livestock Research Organization (KALRO) in Kenya (E).
The demonstration manufacturing plant in Nigeria and the modular plant in Kenya proved that commercial production is feasible at relatively low-cost using materials and equipment available all over SSA. Simultaneously, monitoring and evaluation studies showed that Aflasafe use offers substantial benefits by ensuring safer crops, healthier families, and substantial increase in farmers’ income through sustainable biocontrol adoption models (Narayan et al., 2019; Narayan and Geyer, 2022). The research, development, and delivery actions conducted thus far highlight that manufacturing and distribution of aflatoxin biocontrol at scale is possible in several SSA countries but required private sector investment for meaningful impact (Bandyopadhyay et al., 2022).
The Aflasafe Initiative, guided by the CGIAR’s Intellectual Assets Principles, issued manufacturing and/or distribution licenses to both public and private sector organizations through a 5-phase commercialization approach, explained earlier (Konlambigue et al., 2020; Ortega-Beltran and Bandyopadhyay, 2023). Importantly, an engineer from the Aflasafe Initiative provided technical support to manufacturers by assisting with facility design, supervising construction, recommending suitable equipment, overseeing installation and adjustments, and commissioning the plant.
To date, rights for both manufacturing and distribution have been licensed to local private companies Harvestfield Industries Ltd. (HIL) for Nigeria, BAMTAARE, SA for Senegal and The Gambia, A to Z Textiles Ltd. (A to Z) for Tanzania, and AflaLivre for Mozambique. In Kenya, manufacturing rights for the modular plant in Katumani were licensed to KALRO, which in turn granted distribution rights to Koppert Biological Systems. Modular plants were built in Kahone, Senegal by BAMTAARE, SA, in Arusha, Tanzania by A to Z, at IITA-Bukavu, DR Congo by the Government of DR Congo. In addition, modular plants are nearing completion in Bujumbura, Burundi and Nampula, Mozambique. A small production plant was established in 2021 in Khartoum in collaboration with private sector partner Samil Industries Ltd. with the support of Agence Française de Dévelopement (AFD) for manufacturing the Sudan-specific product for field-testing. Unfortunately, a modular manufacturing plant that was under construction in Khartoum has stalled due to the conflict that started in April 2023.
In countries where manufacturing capacity is currently lacking, distribution rights for products have been granted to companies in Ghana (CalliGhana), Burkina Faso (SAPHYTO), and Mali (Mali Protection Cultures, MPC). These companies import products from the closest licensed factories, with oversight from the Aflasafe Initiative. The distribution partners were selected through a competitive process described earlier (Konlambigue et al., 2020).
Those obtaining manufacturing licenses built their facilities (Figure 6) in consultation with, and with technical backstopping of the Aflasafe Initiative, following the guidance provided by the commercialization strategy developed for each country. Investments for these facilities varied based on several factors, ranging from US$ 435,000 to 1.35 million. Key costs for establishing the facilities fall in three main groups: (i) Capital expenditures to acquire the fixed assets (e.g., land, buildings, equipment); (ii) Business operating expenditures (includes fixed and variable costs); and (iii) Financial expenditures to acquire the necessary capital to maintain operations and/or expand services (includes fundraising costs). Building costs varied significantly—from ~US$ 15,000 for partners who had existing building/warehouse with utilities (e.g., power, water, roadworks) to around US$ 650,000 for those starting on an open site with no building and utilities.
In Nigeria, after initially testing the Aflasafe market through product distribution, HIL expressed interest in establishing its own manufacturing facility. Since IITA had already demonstrated the feasibility of commercial-scale biocontrol production, and with a company expressing interest to commercially manufacture the product, it was no longer necessary for IITA to operate the commercial-scale facility at IITA-Ibadan. HIL purchased most of the equipment of the plant in Ibadan, and in 2021, the facility at IITA-Ibadan was dismantled (Figure 3D). The equipment was subsequently installed at HIL’s manufacturing facility (Figures 3E,F), with manufacturing now licensed to the company.
The materials required to produce one ton of finished product are: (1) 1 ton of sterile, roasted sorghum grain; (2) a master suspension containing 10 L spore suspension (4 × 107 spores/mL) of the active ingredients, 1.5 L polymer, 2 L blue dye, and 10.5 L sterile water; and (3) packing bags. This costs about US$ 600, broken down as follows: 80% for sorghum, 13% for active ingredient, 2.5% for polymer, 4.4% for blue dye, and 0.1% for the packing bag. Then, costs of CAPEX, production and distribution costs, and margin must be factored in. About eight years ago, it was calculated that distributors would need to charge a maximum of US$ 18.75 for 10 kg of product to ensure profitability while keeping the product affordable for smallholder farmers (Bandyopadhyay et al., 2016). Willingness to pay studies in Nigeria and Kenya revealed that farmers are more likely to adopt biocontrol if awareness about aflatoxin is raised, product value is demonstrated, and the cost of treatment is reduced (Ayedun et al., 2017; Migwi et al., 2020). Therefore, it is necessary to explore cost-reduction strategies. In subsequent paragraphs are some actions taken to lower production costs without compromising product effectiveness. However, conducting appropriate cost reduction analyses are needed to identify and quantify the financial benefits of these measures.
A critical step to manufacture an atoxigenic-based biocontrol product is the mass production of active ingredient spores. For the demonstration plant in Nigeria and the modular plant in Kenya, a mass spore production method was developed based on solid fermentation using sorghum grain (Callicott et al., 2018; Bandyopadhyay et al., 2019). Industrial manufacturing was scheduled in a manner to ensure that fresh spores were available for coating grain carriers at the time of production. This required considerable planning for mass producing the spores including backend steps such as regrowing mother cultures, conducting quality checks, preparing for fermentation, managing fermentation time, spore harvest and dilution, and performing final quality checks. To further simplify and economize the mass spore production process, a fortuitous situation in Senegal presented an opportunity. BAMTAARE planned to start Aflasafe SN01 production and distribution in 2019 for Senegal and The Gambia but faced challenges in setting up their spore production laboratory due to delayed equipment delivery. In response, the Aflasafe Initiative quickly developed a process to produce spores in Ibadan, dry them to a small volume, and send via courier to Kahone. There, BAMTAARE staff combined the spores with polymer and dye to manufacture Aflasafe SN01 (Ortega-Beltran et al., 2021).
Products manufactured either with freshly produced or dry spores are equally effective in limiting aflatoxin (Ortega-Beltran et al., 2021). This finding was shared with other manufacturers (HIL, A to Z, AflaLivre), who chose to procure dry spores produced by IITA at cost, through a public-private partnership. With 1 kg of dry spores (250 g spores of each of the four constituent atoxigenic fungi), 50 tons of product—enough to treat 5,000 ha —can be produced. The use of dry spores offers several advantages:
• Reduced construction, capital investment, and running costs.
• Availability of dry spores, with a shelf life of at least 2 years (unpublished), shortens the lead time to manufacture a product once orders are received.
• Small volume of spores procured can be stored in a refrigerator for future use.
• High spore quality and reduced risk of product contamination.
Dry Products manufactured using dry spores are being used to treat commercial crops in Senegal, The Gambia, Nigeria, Ghana, Tanzania, Burkina Faso, Malawi, Mozambique, and Mali. Experimental products have also been manufactured with dry spores in licensed plants in Senegal and Tanzania, and a research plant in Sudan to conduct large-scale effectiveness trials in Niger, Togo, Burundi, DRC, Uganda, Rwanda, and Sudan. These trials have generated critical information required to register products with national or regional regulators3. A new spore production facility at IITA-Ibadan now caters to the needs of the manufacturers across various countries.
Several grain carriers have been evaluated for formulating atoxigenic-based products in the US. These include wheat, sorghum, black-eyed peas, black beans, soybeans, barley, rye, oats, maize, cottonseed, finger millet, and rice (Bock and Cotty, 1999). Synthetic solid carriers such as alginate pellets containing wheat gluten and atoxigenic fungal mycelia (Daigle and Cotty, 1995) and conidia-impregnated bioplastics (Abbas et al., 2017) supported excellent sporulation. However, these synthetic carriers are not commercially viable for use in SSA due to their high cost and limited availability. All commercially available atoxigenic-based biocontrol products use grains as carriers. In the US, two products use wheat grain (initially for AF36) and dehulled barley (Afla-Guard) as carriers. All Aflasafe products in Africa, as well as AF-X1® in Italy (Mauro et al., 2018) and AF36 Prevail in the US, use white sorghum as carrier grain.
Over the years, the use of sorghum grain in SSA for biocontrol formulation has raised concern since it is a staple (Bandyopadhyay et al., 2016). Pellets made from several organic sources readily available in Nigeria and other SSA countries, such as cassava peels, cocoa pod shafts, rice bran, and palm kernel shaft, have been tested. Although promising results were obtained in laboratory trials with cassava peels (Okike et al., 2015), none of those pellets were solid alternatives to sorghum (unpublished). The main challenges were in producing pellets of the size and structural integrity similar to sorghum grain. The pellets were too fragile leading to pulverization, which would make them difficult to broadcast in the field. Also, the biocontrol fungi did not grow as effectively in these pellets as on sorghum grain. Recently, dried distillers’ grains with solubles (DDGS) has been explored as an alternative with Purdue University and preliminary results suggest that DDGS pellets could replace sorghum grain (K. Ileleji, personal communication). Meanwhile, procuring white sorghum at harvest when prices are lowest and negotiating contracts with growers for direct supply remain viable options to keep costs of Aflasafe as low as possible.
Manufacturers can lower costs by sourcing equipment from companies in countries like Turkey, Italy, India, Mexico, and Brazil, which offer high-quality cleaners, treaters, silos, and other machinery at competitive prices. This enables quicker cost recovery and, ultimately, lower product prices, without compromising quality.
As mentioned previously, Aflasafe manufacturing equipment are similar to that used in seed, food, and feed industries. To reduce costs, factory idle time, and operational costs, we worked with food processors Samil in Sudan and AflaLivre in Mozambique to create multiple use facilities, where equipment and buildings are used for manufacture of Aflasafe and food products. The design of Mozambique factory allows shared use of common building and same equipment for intake, cleaning, and roasting (Modules IC and R) for biocontrol manufacture and food processing (peanut roasting). In Sudan, intake, cleaning, roasting, and cooling was performed in an existing food processing facility.
Some stakeholders require formulations for use in organic agriculture. Therefore, products have been manufactured using either milk powder or gum Arabic instead of the industrial polymer used as a sticker, and without blue food dye. Use of either organic or conventional formulations in Togo in 2022 resulted in low aflatoxin levels in groundnut treated in aflatoxin-prone areas (Bonkoungou et al., 2024). In Senegal, delays in procuring the polymer and dye for the 2023 production season led to the manufacturing of Aflasafe SN01 using milk powder as the binder, and no blue food dye was added. Quality control tests revealed that the organic formulation supported excellent sporulation of the biocontrol fungi.
Not adding colored dye in the formulated product can have drawbacks. In 2023, in Nigeria’s Niger State, where the regular blue-dyed formulation was sold as food in a market after washing the dye4. Investigations revealed that a sudden surge in sorghum price made the biocontrol product cheaper, leading to its consumption. The Niger State Government quickly recalled the product to prevent further misuse. A follow-up recommended better awareness campaigns to ensure that Aflasafe is used correctly for its intended usage5. Appropriate measures must be taken to ensure that the organic non-dyed organic product is used for its intended purpose.
The development and commercial availability of 14 out of the 17 aflatoxin biocontrol products globally (Moral et al., 2020) align with the Sustainable Development Goals (Ortega-Beltran and Bandyopadhyay, 2021), notably contributing to Goal 2 (Zero Hunger) and Goal 3 (Good Health and Well-being). The Aflasafe Initiative, through a 20+ year effort, led the development, testing, and registration of these products, marking a significant milestone as the first generation of non-seed technologies of CGIAR to transition successfully from laboratory prototypes to industry application benefiting farmers and consumers at scale. Despite relatively low profitability potential, innovative approaches attracted over >US$ 10 million in private investment for manufacturing and distribution. Four factories are operational, with more in the offing. As a result, farmers across various SSA countries have applied Aflasafe products on over 600,000 ha, producing over 1 million tons of safe maize, groundnut, and sorghum. This has contributed considerably to food security, public health, and well-being of consumers of protected crops (Ola et al., 2022; Ortega-Beltran and Bandyopadhyay, 2023).
What is on the horizon for aflatoxin contamination? In the tropics and subtropics, aflatoxin contamination events have been frequent and serious for decades and this trend is expected to worsen due to factors such as shifts in the fungal population, unforeseen consequences of crop breeding and climate change (Miller, 2023) unless corrective actions are taken. Areas traditionally considered safe are now experiencing frequent contamination and the temperate zones have been at risk of aflatoxin contamination for 15+ years because of climate change (Cotty and Jaime-Garcia, 2007; Magan et al., 2011; Battilani et al., 2016). Nowadays, reports of contamination are common in various crops/foods in temperate regions in European and North America. Addressing the growing problem requires converging technical, institutional, and policy actions if the challenge is to be tackled in a meaningful manner (Ndiame and Dube, 2022). While research and delivery efforts have made important progress, much more needs to be done.
Achieving effective aflatoxin control is complex and requires a multifaceted approach. Here we focused on one of the key elements of biocontrol-centered approaches: its manufacturing. For manufacturing to be appropriately planned and executed, market studies must be conducted before building a factory. For countries with small demand, regional factories serving multiple countries are more suitable than country-specific ones. Developers of aflatoxin control technologies must address all stages development and deployment to ensure sustainable, large-scale use (Box 1, 2) to solve this great challenge. Due to the multi-sectoral nature of aflatoxin contamination, it can only be effectively implemented on the ground by building multidisciplinary, multi-institutional, and multi-sectoral partnerships (Ortega-Beltran and Bandyopadhyay, 2023).
LK: Formal analysis, Writing – review & editing, Visualization, Validation, Supervision, Resources, Project administration, Methodology, Investigation, Data curation, Conceptualization. AO-B: Writing – original draft, Funding acquisition, Writing – review & editing, Visualization, Validation, Supervision, Resources, Project administration, Methodology, Investigation, Formal analysis, Data curation, Conceptualization. JA: Writing – review & editing, Visualization, Supervision, Resources, Project administration, Methodology, Investigation, Data curation. MK: Writing – review & editing, Visualization, Supervision, Resources, Project administration, Methodology, Investigation, Conceptualization. JK: Writing – review & editing, Visualization, Validation, Methodology, Investigation, Formal analysis, Data curation. RB: Writing – review & editing, Visualization, Validation, Supervision, Resources, Project administration, Methodology, Investigation, Funding acquisition, Data curation, Conceptualization.
The author(s) declare that financial support was received for the research, authorship, and/or publication of this article. Several institutions and governments have funded the aflatoxin control program of IITA: The Bill & Melinda Gates Foundation (BMGF; OPP1007117 and OPP1133356); United States Agency for International Development (USAID); Meridian Institute on behalf of the Partnership for Aflatoxin Control in Africa (PACA); Agricultural Research Service (ARS) and Foreign Agricultural Service (FAS) of USDA; Bundesministerium für wirtschaftliche Zusammenarbeit und Entwicklung (BMZ, German Federal Ministry for Economic Cooperation and Development); AgResults [an initiative between BMGF, Australia’s Department of Foreign Affairs and Trade (DFAT), Department for International Development of the United Kingdom (DFID), Global Affairs Canada, and USAID]; MycoGlobe, MycoRed, and MycoKey funded by the European Union Commission; Austrian Development Cooperation; Commercial Agriculture Development Program of the Government of Nigeria; Government of DRC; French Development Agency; Royal Government of Norway through the Climate Smart Agricultural Technologies (CSAT; MLI-17/0008, NER-17/0005) for Mali and Niger; AGRA; CGIAR A4NH and MAIZE Research Programs, and CGIAR’s Plant Health and Rapid Response to Protect Food Security and Livelihoods Initiative (Plant Health Initiative) through contributions of various donors to the CGIAR Trust Fund: www.cgiar.org/funders/.
We are grateful to all farmers, government ministries and agencies, donors, researchers, public institutions, and private investors who have strongly supported the aflatoxin biocontrol technology and have made possible its development, registration, commercialization, and technology transfer for sustainable use of aflatoxin biocontrol products in multiple countries in Africa. We are grateful to several staff in USDA-ARS, ACRPC (particularly Larry Antilla), and agricultural industries in Arizona, California, and Texas for facilitating the free transfer of the aflatoxin biocontrol technology for use in Africa by sharing experiences and methodologies with IITA and partners. A special mention goes to Peter Cotty, formerly with USDA-ARS, who was instrumental in generating and freely sharing several background technologies and supported the Aflasafe Initiative with time, ideas, funding acquisition, training, and equipment.
The authors receive no direct financial benefit from the manufacturing and marketing of any of the aflatoxin biocontrol products mentioned in this article. The Aflasafe name is a Trademark of the International Institute of Tropical Agriculture (IITA). IITA charges a small licensing fee to manufacturers for the use of the Aflasafe trademark and costs associated with technology transfer and technical backstopping. This fee is reinvested for providing technical support to the manufacturers and distributors when needed, to develop new products/practices for more farmers to use the technology, and for further research to improve the technology. A portion of the fee is also set aside for fulfilling reasonable Access and Benefit Sharing obligations related to the Convention on Biodiversity. LK, AO-B, JA, MK, and JW are employed by IITA.. RB is an Emeritus Scientist with IITA.
The author(s) declare that Generative AI was used in the creation of this manuscript. ChatGPT was used solely for spelling/grammar checks and editing the final version of the manuscript before submission.
All claims expressed in this article are solely those of the authors and do not necessarily represent those of their affiliated organizations, or those of the publisher, the editors and the reviewers. Any product that may be evaluated in this article, or claim that may be made by its manufacturer, is not guaranteed or endorsed by the publisher.
The Supplementary material for this article can be found online at: https://www.frontiersin.org/articles/10.3389/fsufs.2024.1509384/full#supplementary-material
1. ^Doreo Partners is a for-profit Nigerian impact investing firm which invests in early-stage businesses that improve the livelihoods of Nigerian smallholder farmers.
2. ^agresults.org/projects/nigeria
3. ^https://cgspace.cgiar.org/handle/10568/121531
4. ^https://dailypost.ng/2023/07/22/desist-from-consuming-highly-poisonous-aflasafe-niger-govt-cautions-residents/
5. ^https://von.gov.ng/niger-state-warns-residents-against-use-of-aflasafe-products/
Abbas, H. K., Accinelli, C., and Shier, W. T. (2017). Biological control of aflatoxin contamination in US crops and the use of bioplastic formulations of Aspergillus flavus biocontrol strains to optimize application strategies. J. Agric. Food Chem. 65, 7081–7087. doi: 10.1021/acs.jafc.7b01452
Adhikari, B. N., Bandyopadhyay, R., and Cotty, P. J. (2016). Degeneration of aflatoxin gene clusters in Aspergillus flavus from Africa and North America. AMB Express 6:62. doi: 10.1186/s13568-016-0228-6
Agbetiameh, D., Ortega-Beltran, A., Awuah, R. T., Atehnkeng, J., Elzein, A., Cotty, P. J., et al. (2020). Field efficacy of two atoxigenic biocontrol products for mitigation of aflatoxin contamination in maize and groundnut in Ghana. Biol. Control 150:104351. doi: 10.1016/j.biocontrol.2020.104351
Aikore, M. O. S., Ortega-Beltran, A., Eruvbetine, D., Atehnkeng, J., Falade, T., Cotty, P. J., et al. (2019). Performance of broilers fed with maize colonized by either toxigenic or atoxigenic strains of aspergillus flavus with and without an aflatoxin-sequestering agent. Toxins 11:565. doi: 10.3390/toxins11100565
Alderucci, D., and Baumol, W. J. (2013). Patents and the dissemination of inventions. J. Econ. Perspect. 27, 223–226.
Atehnkeng, J., Donner, M., Ojiambo, P. S., Ikotun, B., Augusto, J., Cotty, P. J., et al. (2016). Environmental distribution and genetic diversity of vegetative compatibility groups determine biocontrol strategies to mitigate aflatoxin contamination of maize by Aspergillus flavus. Microb. Biotechnol. 9, 75–88. doi: 10.1111/1751-7915.12324
Atehnkeng, J., Ojiambo, P. S., Cotty, P. J., and Bandyopadhyay, R. (2014). Field efficacy of a mixture of atoxigenic Aspergillus flavus link: FR vegetative compatibility groups in preventing aflatoxin contamination in maize (Zea mays L.). Biol. Control 72, 62–70. doi: 10.1016/j.biocontrol.2014.02.009
Atehnkeng, J., Ojiambo, P. S., Donner, M., Ikotun, T., Sikora, R. A., Cotty, P. J., et al. (2008a). Distribution and toxigenicity of Aspergillus species isolated from maize kernels from three agro-ecological zones in Nigeria. Int. J. Food Microbiol. 122, 74–84. doi: 10.1016/j.ijfoodmicro.2007.11.062
Atehnkeng, J., Ojiambo, P. S., Ikotun, T., Sikora, R. A., Cotty, P. J., and Bandyopadhyay, R. (2008b). Evaluation of atoxigenic isolates of Aspergillus flavus as potential biocontrol agents for aflatoxin in maize. Food Addit. Contam. Part A Chem. Anal. Control Expo. Risk Assess. 25, 1264–1271. doi: 10.1080/02652030802112635
Ayedun, B., Okpachu, G., Manyong, V., Atehnkeng, J., Akinola, A., Abu, G. A., et al. (2017). An assessment of willingness to pay by maize and groundnut farmers for aflatoxin biocontrol product in northern Nigeria. J. Food Prot. 80, 1451–1460. doi: 10.4315/0362-028X.JFP-16-281
Bandyopadhyay, R., Atehnkeng, J., Ortega-Beltran, A., Akande, A., Falade, T. D. O., and Cotty, P. J. (2019). “Ground-truthing” efficacy of biological control for aflatoxin mitigation in farmers’ fields in Nigeria: from field trials to commercial usage, a 10-year study. Front. Microbiol. 10:2528. doi: 10.3389/fmicb.2019.02528
Bandyopadhyay, R., Ortega-Beltran, A., Akande, A., Mutegi, C., Atehnkeng, J., Kaptoge, L., et al. (2016). Biological control of aflatoxins in Africa: current status and potential challenges in the face of climate change. World Mycotoxin J. 9, 771–789. doi: 10.3920/WMJ2016.2130
Bandyopadhyay, R., Ortega-Beltran, A., Konlambigue, M., Kaptoge, L., Falade, T. D. O., and Cotty, P. J. (2022). “Development and scale-up of bioprotectants to keep staple foods safe from aflatoxin contamination in Africa” in Microbial bioprotectants for plant disease management. eds. J. Köhl and W. Ravensberg (Cambridge: Burleigh Dodds Science Publishing), 1–41.
Battilani, P., Toscano, P., Van Der Fels-Klerx, H. J., Moretti, A., Camardo Leggieri, M., Brera, C., et al. (2016). Aflatoxin B1 contamination in maize in Europe increases due to climate change. Sci. Rep. 6, 1–7. doi: 10.1038/srep24328
Bock, C. H., and Cotty, P. J. (1999). Wheat seed colonized with atoxigenic Aspergillus flavus: characterization and production of a biopesticide for aflatoxin control. Biocontrol Sci. Tech. 9, 529–543. doi: 10.1080/09583159929497
Bonkoungou, S., Dagno, K., Basso, A., Ekanao, T., Atehnkeng, J., Agbetiameh, D., et al. (2024). Mitigation of aflatoxin contamination of maize, groundnut, and sorghum by commercial biocontrol products in farmers’ fields across Burkina Faso, Mali, Niger, and Togo. CABI Agric. Biosci. 5:106. doi: 10.1186/s43170-024-00313-3
Callicott, K. A., Kachapulula, P., Edmunds, D., Singh, P., Islam, M. S., Jaime, R., et al. (2018). Brief protocols for research on management of aflatoxin-producing fungi. 2nd Edn. Tucson: Agricultural Research Service, United States Department of Agriculture, 302.
Camardo Leggieri, M., Toscano, P., and Battilani, P. (2021). Predicted aflatoxin B1 increase in Europe due to climate change: actions and reactions at global level. Toxins 13, 1–21. doi: 10.3390/toxins13040292
Cotty, P. J., Antilla, L., and Wakelyn, P. J. (2007). “Competitive exclusion of aflatoxin producers: farmer-driven research and development” in Biological control: A global perspective. eds. C. Vincent, M. S. Goettel, and G. Lazarovits (Oxfordshire: CABI), 241–253.
Cotty, P. J., and Jaime-Garcia, R. (2007). Influences of climate on aflatoxin producing fungi and aflatoxin contamination. Int. J. Food Microbiol. 119, 109–115. doi: 10.1016/j.ijfoodmicro.2007.07.060
Cotty, P. J., Probst, C., and Jaime-Garcia, R. (2008). “Etiology and management of aflatoxin contamination” in Mycotoxins: Detection methods, management, public health and agricultural trade. eds. J. F. Leslie, R. Bandyopadhyay, and A. Visconti (Oxfordshire: CABI), 287–299.
Daigle, D. J., and Cotty, P. J. (1995). Formulating atoxigenic Aspergillus flavus for field release. Biocontrol Sci. Tech. 5, 175–184. doi: 10.1080/09583159550039891
Donner, M., Atehnkeng, J., Sikora, R. A., Bandyopadhyay, R., and Cotty, P. J. (2009). Distribution of Aspergillus section Flavi in soils of maize fields in three agroecological zones of Nigeria. Soil Biol. Biochem. 41, 37–44. doi: 10.1016/j.soilbio.2008.09.013
Donner, M., Atehnkeng, J., Sikora, R. A., Bandyopadhyay, R., and Cotty, P. J. (2010). Molecular characterization of atoxigenic strains for biological control of aflatoxins in Nigeria. Food Addit. Contam. Part A Chem. Anal. Control Expo. Risk Assess. 27, 576–590. doi: 10.1080/19440040903551954
Dorner, J. W. (2009). Development of biocontrol technology to manage aflatoxin contamination in peanuts. Peanut Sci. 36, 60–67. doi: 10.3146/AT07-002.1
Gressel, J. (2023). Four pillars are required to support a successful biocontrol fungus. Pest Manag. Sci. 80, 35–39. doi: 10.1002/ps.7417
Konlambigue, M., Ortega-Beltran, A., Bandyopadhyay, R., Shanks, T., Landreth, E., and Jacob, O. (2020). Lessons learned on scaling Aflasafe® through commercialization in sub-Saharan Africa. A4NH strategic brief 2020. Available online at: https://ebrary.ifpri.org/digital/collection/p15738coll2/id/133956
Lee, S. L., O’Connor, T. F., Yang, X., Cruz, C. N., Chatterjee, S., Madurawe, R. D., et al. (2015). Modernizing pharmaceutical manufacturing: from batch to continuous production. J. Pharm. Innov. 10, 191–199. doi: 10.1007/s12247-015-9215-8
Logrieco, A., Battilani, P., Leggieri, M. C., Jiang, Y., Haesaert, G., Lanubile, A., et al. (2021). Perspectives on global mycotoxin issues and management from the Mycokey maize working group. Plant Dis. 105, 525–537. doi: 10.1094/PDIS-06-20-1322-FE
Magan, N., Medina, A., and Aldred, D. (2011). Possible climate-change effects on mycotoxin contamination of food crops pre- and postharvest. Plant Pathol. 60, 150–163. doi: 10.1111/j.1365-3059.2010.02412.x
Mauro, A., Garcia-Cela, E., Pietri, A., Cotty, P. J., and Battilani, P. (2018). Biological control products for aflatoxin prevention in Italy: commercial field evaluation of atoxigenic aspergillus flavus active ingredients. Toxins 10:30. doi: 10.3390/toxins10010030
Mehl, H. L., Jaime, R., Callicott, K. A., Probst, C., Garber, N. P., Ortega-Beltran, A., et al. (2012). Aspergillus flavus diversity on crops and in the environment can be exploited to reduce aflatoxin exposure and improve health. Ann. N. Y. Acad. Sci. 1273, 7–17. doi: 10.1111/j.1749-6632.2012.06800.x
Migwi, B., Mutegi, C., Mburu, J., Wagacha, J., Cotty, P., Bandyopadhyay, R., et al. (2020). Assessment of willingness-to-pay for Aflasafe KE01, a native biological control product for aflatoxin management in Kenya. Food Addit. Contam. Part A Chem. Anal. Control Expo. Risk Assess. 37, 1951–1962. doi: 10.1080/19440049.2020.1817571
Miller, J. D. (2023). “Mycotoxins: still with us after all these years” in Present knowledge in food safety. eds. M. E. Knowles, L. E. Anelich, A. R. Boobis, and B. Popping (Cambridge: Academic Press), 62–78.
Moral, J., Garcia-Lopez, M. T., Camiletti, B. X., Jaime, R., Michailides, T. J., Bandyopadhyay, R., et al. (2020). Present status and perspective on the future use of aflatoxin biocontrol products. Agronomy 10, 1–19. doi: 10.3390/agronomy10040491
Narayan, T. A., and Geyer, J. (2022). Can results-based prizes to private sector incentivize technology adoption by farmers? Evidence from the AgResults Nigeria project that uses prizes to incentivize adoption of Aflasafe™. Agric. Food Secur. 11, 1–11. doi: 10.1186/s40066-022-00377-2
Narayan, T., Mainville, D., Geyer, J., Hausdorff, K., and Cooley, D. (2019). AgResults impact evaluation report: Nigeria Aflasafe™ challenge project. Rockville, MD: Abt Associates.
Ndiame, F., and Dube, L. (2022). Food convergence innovation: promises of building back more sustainable food systems in Africa. Devel. Finan. Agenda (DEFA) 7, 12–13.
Okike, I., Samireddypalle, A., Kaptoge, L., Fauquet, C., Atehnkeng, J., Bandyopadhyay, R., et al. (2015). Technical innovations for small-scale producers and households to process wet cassava peels into high quality animal feed ingredients and aflasafe™ substrate. Food Chain 5, 71–90. doi: 10.3362/2046-1887.2015.005
Ola, O. T., Ogedengbe, O. O., Raji, T. M., Eze, B., Chama, M., Ilori, O. N., et al. (2022). Aflatoxin biocontrol effectiveness in the real world — private sector-led efforts to manage aflatoxins in Nigeria through biocontrol-centered strategies. Front. Microbiol. 13:977789. doi: 10.3389/fmicb.2022.977789
Ortega-Beltran, A., Aikore, M. O., Kaptoge, L., Agbetiameh, D., Moral, J., and Bandyopadhyay, R. (2024). Impact of storage conditions on the shelf life of aflatoxin biocontrol products containing atoxigenic isolates of aspergillus flavus as active ingredient applied in various countries in Africa. CABI Agric. Biosci. 5, 1–9. doi: 10.1186/s43170-024-00283-6
Ortega-Beltran, A., and Bandyopadhyay, R. (2021). Contributions of integrated aflatoxin management strategies to achieve the sustainable development goals in various African countries. Glob. Food Sec. 30:100559. doi: 10.1016/j.gfs.2021.100559
Ortega-Beltran, A., and Bandyopadhyay, R. (2023). Aflatoxin biocontrol in practice requires a multidisciplinary, long-term approach. Front. Sustain. Food Syst. 7:1110964. doi: 10.3389/fsufs.2023.1110964
Ortega-Beltran, A., Kaptoge, L., Senghor, A. L., Aikore, M. O. S., Jarju, P., Momanyi, H., et al. (2021). Can it be all more simple? Manufacturing aflatoxin biocontrol products using dry spores of atoxigenic isolates of aspergillus flavus as active ingredients. Microb. Biotechnol. 15, 901–914. doi: 10.1111/1751-7915.13802
Probst, C., Bandyopadhyay, R., and Cotty, P. J. (2014). Diversity of aflatoxin-producing fungi and their impact on food safety in sub-Saharan Africa. Int. J. Food Microbiol. 174, 113–122. doi: 10.1016/j.ijfoodmicro.2013.12.010
Rocha, I., Ma, Y., Souza-Alonso, P., Vosatka, M., Freitas, H., and Oliveira, R. S. (2019). Seed coating: a tool for delivering beneficial microbes to agricultural crops. Front. Plant Sci. 10:1357. doi: 10.3389/fpls.2019.01357
Schreurs, F., Bandyopadhyay, R., Kooyman, C., Ortega-Beltran, A., Akande, A., Konlambigue, M., et al. (2019). “Commercial products promoting plant health in African agriculture” in Critical issues in plant health: 50 years of research in African agriculture. eds. P. Neuenschwander and M. Tamò (Cambridge: Burleigh Dodds Science Publishing), 345–363.
Senghor, A. L., Ortega-Beltran, A., Atehnkeng, J., Jarju, P., Cotty, P. J., and Bandyopadhyay, R. (2021). Aflasafe SN01 is the first biocontrol product approved for aflatoxin mitigation in two nations, Senegal and The Gambia. Plant Dis. 105, 1461–1473. doi: 10.1094/PDIS-09-20-1899-RE
Teixidó, N., Usall, J., and Torres, R. (2022). Insight into a successful development of biocontrol agents: production, formulation, packaging, and shelf life as key aspects. Horticulturae 8:305. doi: 10.3390/horticulturae8040305
Udomkun, P., Wiredu, A. N., Nagle, M., Bandyopadhyay, R., Müller, J., and Vanlauwe, B. (2017a). Mycotoxins in sub-Saharan Africa: present situation, socio-economic impact, awareness, and outlook. Food Control 72, 110–122. doi: 10.1016/j.foodcont.2016.07.039
Udomkun, P., Wiredu, A. N., Nagle, M., Müller, J., Vanlauwe, B., and Bandyopadhyay, R. (2017b). Innovative technologies to manage aflatoxins in foods and feeds and the profitability of application – a review. Food Control 76, 127–138. doi: 10.1016/j.foodcont.2017.01.008
Williams, J. H., Phillips, T. D., Jolly, P. E., Stiles, J. K., Jolly, C. M., and Aggarwal, D. (2004). Human aflatoxicosis in developing countries: a review of toxicology, exposure, potential health consequences, and interventions. Am. J. Clin. Nutr. 80, 1106–1122. doi: 10.1093/ajcn/80.5.1106
Wu, F. (2015). Global impacts of aflatoxin in maize: trade and human health. World Mycotoxin J. 8, 137–142.
Keywords: aflatoxin biocontrol, industrial manufacturing, lab to field, food safety, food security, bioprotectant, Aflasafe, scaling up
Citation: Kaptoge L, Ortega-Beltran A, Atehnkeng J, Konlambigue M, Kamau JW and Bandyopadhyay R (2024) The challenge of industrialization of a nature-based solution that allows farmers to produce aflatoxin-safe crops in various African countries. Front. Sustain. Food Syst. 8:1509384. doi: 10.3389/fsufs.2024.1509384
Received: 10 October 2024; Accepted: 19 November 2024;
Published: 06 December 2024.
Edited by:
Chibundu Ngozi Ezekiel, Babcock University, NigeriaReviewed by:
Martin Epafras Kimanya, Nelson Mandela African Institution of Science and Technology, Tanzania†PRESENT ADDRESSES:
Lawrence Kaptoge, IITA, Kinshasa, Democratic Republic of CongoJoseph Atehnkeng,IITA, Lumumbashi, Democratic Republic of CongoMatieyedou Konlambigue,IITA, Kigali, RwandaCopyright © 2024 Kaptoge, Ortega-Beltran, Atehnkeng, Konlambigue, Kamau and Bandyopadhyay. This is an open-access article distributed under the terms of the Creative Commons Attribution License (CC BY). The use, distribution or reproduction in other forums is permitted, provided the original author(s) and the copyright owner(s) are credited and that the original publication in this journal is cited, in accordance with accepted academic practice. No use, distribution or reproduction is permitted which does not comply with these terms.
*Correspondence: Ranajit Bandyopadhyay, ci5iYW5keW9wYWRoeWF5QGNnaWFyLm9yZw==; Lawrence Kaptoge, bC5rYXB0b2dlQGNnaWFyLm9yZw==
Disclaimer: All claims expressed in this article are solely those of the authors and do not necessarily represent those of their affiliated organizations, or those of the publisher, the editors and the reviewers. Any product that may be evaluated in this article or claim that may be made by its manufacturer is not guaranteed or endorsed by the publisher.
Research integrity at Frontiers
Learn more about the work of our research integrity team to safeguard the quality of each article we publish.