- 1Department of Microbiology, Landmark University, Omu-Aran, Nigeria
- 2Research and Development Unit, Ludipe Biocrest, Omu-Aran, Nigeria
- 3Department of Microbiology, Federal University Oye Ekiti, Oye, Nigeria
- 4Bamidele Olumilua University of Education, Science and Technology, Ikere, Nigeria
- 5Industrial Chemistry Programme, Department of Physical Sciences, Landmark University, Omu-Aran, Nigeria
- 6Department of Biochemistry, Landmark University, Omu-Aran, Nigeria
- 7Environmental Biology Unit, Department of Cell Biology and Genetics, University of Lagos, Lagos, Nigeria
Introduction: The valorization of agricultural waste from indigenous sub-Saharan African food processes remains underexplored. By-products from the processing of Parkia biglobosa seeds into condiments are often regarded as pollutants. This research assessed their potential for development in various industrial applications.
Materials and methods: This study employed a standardized protocol adopted in the processing of P. biglobosa seed into condiments, enabling the quantification of food and by-products generated as a percentage. A comparative analysis of the proximate and mineral constituents of the dried food condiment and seed coat (testa) was conducted. Furthermore, the phytochemical constituent of effluents from the two stages of processing was characterized using qualitative and quantitative methods, including Fourier-transform infrared spectroscopy (FTIR) and gas chromatography–mass spectrometry (GC–MS).
Results and discussion: The results showed that 66.27% of each 100 g processed P. biglobosa seed used could be considered waste, with 23.19% in seed coat and 29.47% in effluent(s). The seed coat has moisture absorption potential and is fibrous in nature, as confirmed by proximate fiber analysis—15.03 ± 0.13% compared to 9.07 ± 0.10% in the dried condiment. Both the condiments and seed coat contained considerable amounts of sustenance minerals. Effluents from the boiling process exhibited a characteristic starchy effect on textiles. The concentrated effluent from the first stage of boiling had a chocolate-like aroma, sticky texture, and dark-brown color compared to the effluent from the second boiling stage. The FTIR analysis indicated the presence of alcohols, alkenes, aromatic rings, carboxylic acids, and amines in the effluent samples. GCMS characterization reported the presence of specific fatty acids with known health benefits. When premised on the waste-to-wealth initiative, the quantified and characterized by-products of P. biglobosa seed processing, as reported in this study, have potential applications across various industrial processes, including food, cosmetics, pharmaceutical, and agriculture, among others.
1 Introduction
Agriculture is a significant contributor to the gross domestic product (GDP) of many countries. The sub-Saharan Africa region is one of the most prominent examples where agriculture not only plays a crucial role in economic growth but also serves as the primary source of livelihood for a large portion of the rural population (Modi, 2019; Sassi, 2023). Despite this, a significant challenge in the sector is the prevalence of crude, unsustainable practices and the inefficient utilization of bioresources (Gérard, 2020). Notably, several processes during plant or crop harvesting and postharvesting, whether from domestic or commercial food production, often generate tremendous amounts of organic waste (Tamasiga et al., 2022). This problem is exacerbated by the lack of appropriate policies, infrastructures, and waste management practices in many rural and even urban areas across sub-Saharan Africa, thus contributing to environmental pollution in the regions. On the contrary, these organic by-products (i.e., food and/or agricultural waste) have potential as a valuable biomass resource; however, in the subcontinental Africa region, they still remain vastly underutilized. Nevertheless, the significant advances in the conversion of agricultural and food waste for valorization open up mediums for possible innovation in managing, upcycling, and utilizing waste for crop productivity while reducing negative environmental impact (Bhat, 2021; Mohd et al., 2022; Ribeiro et al., 2022).
Over the years, the concept of upcycling and valorization of food waste, especially from plant parts such as seeds, fruits, and vegetative parts, has gained traction (Bos-Brouwers et al., 2012; Sagar et al., 2018). These extends beyond food production to industrial processes such as pharmaceuticals, cosmetics, manufacturing, construction, and energy, among others. For instance, constituents such as cellulose, phytochemicals, and macromolecules are recovered from grains/seeds processing by-products using different physical, chemical, and biological methods (Burange et al., 2016). These constituents have found use for applications related to the development of drugs and/or drug discovery, personal care products, packaging materials, textiles, biofuels, organic fertilizers, biocomposite, hydrocolloids, and additives in food, among others. Representative reviews of these have been accounted for by numerous reports (Lemes et al., 2022; Nguyen et al., 2023; Rao and Rathod, 2019; Rațu et al., 2023). Most of the recorded progress in this field of food waste valorization across the world is notably driven by advancements in regional research and industrial collaboration. In contrast, aside from already known traditional practices of upcycling food by-products, there is a general marginalization of interest in conventional food crops indigenous to sub-Saharan Africa. This is evident in the limited investigation and reporting on their development (Akinola et al., 2020; Ngwasiri et al., 2022), with even less focus on the valorization of food waste from these sources. This lack of research hinders the realization of the potential of such waste, which could significantly contribute to sustainable and healthy food systems in the region.
Parkia biglobosa is a socioeconomically and culturally significant tree legume in West Africa, particularly valued for its seeds, which are used to produce various popular regional condiments (Parkouda et al., 2009). Aside from its culinary use, the seed condiments are attributed to have therapeutic properties suited for consumers as a healthier alternative to commercial food additives and flavor enhancers such as monosodium glutamate (MSG) (Musara et al., 2020; Shahidah et al., 2019). These factors have created a growing demand for P. biglobosa condiments and a source of income for local communities. However, an increase in the usage of P. biglobosa condiments is projected to result in an increase in food waste output, and this presents a significant challenge to waste management. Meanwhile, despite the advances in mechanized production processes of P. biglobosa condiments, crude and tedious methods of processing are still rampant (Coulibaly Diakite et al., 2020; Okunola et al., 2019; Olaniran et al., 2020). Even at present, the burden of waste from processing the seeds of P. biglobosa has, in no small measure, contributed to land, soil, and water pollution (Amusat et al., 2020). General preconceptions about the waste from processing the seed of P. biglobosa have been trailed by some negative presumptions as a pollutant—characterized by the pungent smell from processing locations and potential harm to the environment. The challenge related to P. biglobosa seed waste management also extends to the preprocessing of seed, resulting in the accumulation of biomass in the form of empty pods and pulp (Ibraheem et al., 2022; Ojewumi et al., 2017). Similar waste generation challenges have been described in Pentaclethra macrophylla, which is another indigenous legume plant in the region (Nsude et al., 2022). With respect to this, cultural perceptions of waste management play a crucial role, as most rural communities across West Africa have traditional practices (i.e., indiscriminate waste disposal) that largely do not align with modern waste management techniques (Roberts and Okereke, 2017). Conventional agricultural waste disposal methods have historically favored simplicity and low initial costs but often result in environmental issues such as land degradation and methane emissions. Nevertheless, emerging studies are focusing on improving waste management practices through biomass conversion technologies and nutrient recycling, emphasizing the upcycling and valorization of food waste to mitigate losses associated with traditional methods.
The potential of valorizing waste from the processing of P. biglobosa seeds is indicative of the use of its effluent in traditional/local settings as pest control. Research studies have similarly reported on potential antibacterial activity, conversion into nanoparticles for clothing, and adsorptive properties of the dehulled seed coat, among others (Aguda and Lateef, 2021; Amusat et al., 2022; Babalola et al., 2016; Olukunle et al., 2019). More attention should be diverted to studies on effective means to repurpose such food waste and by-products in maximizing the use of their bioactive natural product for economic benefit. Such measures are to be taken in the context of “Quantitative Bioeconomy” as outlined by the USADEL LAB. The goal is to establish or optimize the use of plants and agricultural or horticultural plant residuals for the extraction of plant compounds to support the bioeconomy. This is, similarly, in line with the United Nations SDG Goal 12 (Responsible Consumption and Production), among others. Thus, this study aimed to quantify the amount of waste generated from the processing of P. biglobosa seed in condiment production and subsequently characterize its constituent for potential as a biomass resource for valorization.
2 Materials and methods
2.1 Plant collection and identification
Specimens of P. biglobosa L. plant pods and leaves were collected from the field environment within Landmark University, Omu-Aran. The samples were assigned voucher number LUH: 9937 upon botanical identification and authentication by a botanist at the Herbarium of the Department of Botany, University of Lagos. De-pulped seeds extracted from P. biglobosa pods were dried and subsequently stored at room temperature (25°C).
2.2 Seed processing and extraction
The steam-pressure boiling method, as described by Olaniran et al. (2020) and Omodara and Aderibigbe (2018) was modified for the processing of P. biglobosa seed into condiments. In a 1:10 dried weight-to-volume ratio, 100 g of dried P. biglobosa seeds was boiled in 1000 mL of distilled water using a Steam Autoclave (LS-B100L) at 121°C. This first stage of boiling was for a period of three (3) h to soften the seed testa and was mechanically dehulled (seed coats/testa separated from the cotyledon). The effluent from the first boiling (EF1) was collected along with an additional 500 mL of distilled water used for washing the cotyledon. The seed coat was also collected separately, and cotyledon was boiled under steam pressure for a second time in a 1:1 ratio of wet weight to volume of distilled water for 30 min. The effluent was collected from the second boiling (second effluent stage [EF2]), and the cotyledon was fermented in a laboratory incubator at 37°C for 48 h. The seed coat and fermented cotyledon (condiment) were dried at 40°C. Immediately after each effluent collection, the solution was centrifuged (SearchTech, Model 800D) at 4,000 rpm for 10 min. Supernatants from centrifugation were filtered through a Whatman™ 1 qualitative filter paper, and the residue was dried with a seed coat. The effluent filtrate was concentrated in a rotary evaporator at 60°C and subsequently oven-dried at 50°C. The final dried weight of each component part from each 100 g of processed P. biglobosa seeds was recorded and stored at 4°C until further use. The seed processing protocol described (Figure 1) was carried out 10 times to determine the average weight of each component part of the processed seeds.
2.3 Proximate and mineral analysis of seed coat and condiment
The analysis of the proximate and mineral content of the resulting condiment and seed coat from P. biglobosa seed processing was performed on a dry weight basis according to the Association of Official Analytical Chemists (AOAC) standard protocol. Using a GallenKamp oven (Model OV 880, England), the moisture content was determined at 105°C for a duration of 6 h (Sanni et al., 2024). Protein content was determined using the macro Kjeldahl method as described by Asante et al. (2024) and Owusu (2012). Crude fat was extracted using petroleum ether in a Soxhlet setup, then dried and weighed to calculate the yield relative to the initial weight of the sample (Abraham et al., 2022; Asante et al., 2024). The defatted sample was further processed for crude fiber determination (Abraham et al., 2022; Gurusmatika and Amira, 2021). Ash content was determined with samples ignited in porcelain crucibles at 550–600°C for 2 h. The samples were then cooled, weighed, and the remaining inorganic residue was calculated by weight deduction (Gurusmatika and Amira, 2021; Mardiah et al., 2020). The nitrogen-free extract (NFE) or digestible carbohydrate was calculated as a percent subtraction of other analyzed constituents (i.e., moisture, protein, crude fat, crude fiber, and ash) (Maliki et al., 2023). The energy content of the samples was calculated based on the Atwater equation, with the factors 9, 4, and 4 for fat, protein, and NFE, respectively (Bikila et al., 2020; Okwunodulu et al., 2021; Shifarew et al., 2024). Mineral content analysis of condiment and seed coat from P. biglobosa seed processing was performed using the atomic absorption spectrometry (AAS) technique (Abraham et al., 2022; Asante et al., 2024; Thomas et al., 2023). The samples were digested in nitric and perchloric acids on a hot plate at 200°C, diluted, and filtered before analyzing concentrations of each element (Ca, Mg, Mn, Fe, Cu, Zn, Cr, Cd, Pb, and Ni) at their respective wavelengths. Calibration curves were prepared, and mineral contents were expressed as mg/g of the sample.
2.4 Screening for organic macromolecule in effluents
Concentrated effluents collected from each stage of P. biglobosa seed processing were screened for the presence of organic molecules using various methods. The Molisch test was used for the detection of the presence of carbohydrates, while the Fehling test was used for reducing sugar, and Barfoed’s test was conducted to identify the presence of specific monosaccharides (Agrawal and Methwani, 2019; Khan et al., 2024). The presence of protein was screened for using the Biuret test, Millions test, and Ninhydrin test (Jain et al., 2020; Porwal et al., 2023). The Sudan test was used to detect the presence of oils and fats (Godlewska et al., 2022).
2.5 Fourier-transform infrared analysis of effluents
Using the FTIR, a comparative analysis of functional groups in concentrated effluents from the P. biglobosa seed processing was conducted with a Thermo Scientific FTIR spectrometer (NICOLET IS5). After prepping and pelletizing with spectroscopy-grade potassium bromide (KBr), the samples were scanned for transmittance over the frequency range of 4,000–400 cm−1. The resulting infrared spectroscopy was interpreted using a correlation table (Guha et al., 2024; Hemalatha et al., 2016).
2.6 Gas chromatography–mass spectrometry analysis of effluents
GCMS analysis was used to identify and quantify some phytochemicals in the effluents from the P. biglobosa seed processing (Orabueze et al., 2020; Ukwubile et al., 2019). The Agilent Technologies system, consisting of a 7890 GC coupled with a 5975 MS detector, was used to analyze the reconstituted effluents. The instrument temperature was programmed to increase from 80°C, held for 2 min, then raised at a rate of 10°C/min to 240°C, and held for 6 min. At an ionization energy of 70 eV, the sample volume of 1 μL was injected and carrier gas—helium was set at 2 mL/min. The volatile components of the test fractions were identified based on retention time, and a post-analysis was performed using the system solution software. For identification, the generated mass spectra were compared to those of known compounds in the National Institute of Standards and Technology (NIST) library.
2.7 Statistical analysis
All collated experimental data were analyzed using Statistical Package for the Social Sciences (SPSS) version 26.0 and reported as mean and standard deviation. Origin 8 was used for the graphical presentation of FTIR results.
3 Results and discussion
The conception and design of this research on the processing of P. biglobosa seeds into condiments and the valorization of its waste aimed to address various aspects of the United Nations Sustainable Developmental Goal, particularly in promoting responsible consumption and production (Goal 12). The standardization of the P. biglobosa seed processing, as shown in Figure 1, was based on progressive research conducted on its potential for mechanized production and energy conservation. The traditional process of converting P. biglobosa seeds into condiments typically takes more than 12 h (Ugwuanyi and Okpara, 2019), requires high temperatures exceeding 100°C, and consumes large amounts of water due to evaporation and other factors. This study used the steam pressure-cooking method, which resulted in a total time of less than 4 h of boiling. By employing the steam pressure-cooking method, the process was able to significantly reduce water consumption and energy wastage during the processing stages. Previous research by Olaniran et al. (2020) for mechanically dehulling at 398 rpm recommended 2 h as the optimum duration using a pressure cooker in the first boiling stage. Similarly, Ajayi et al. (2015), in an effort to develop shelf-stable “Iru”—a Yoruba variety of the P. biglobosa condiment into bouillon cubes, reported a modified procedure of first cooking for 2 h and then de-hulling and boiling again for 30 min. However, the preliminary investigation in this study on processing time for optimal manual dehulling in this study was 3 h after the first boiling, which was comparability similar to the protocol by Omodara and Aderibigbe (2018). The need for optimum energy optimization coupled with other factors, such as extraction of phytochemicals, was taken into consideration in the determination of the final boiling time and extraction protocol in the processing of the P. biglobosa seeds.
In this study, notable characteristics of the waste by-products were observed from P. biglobosa seeds processing, which is relevant for its valorization. Figure 2 presents the results of the condiments obtained, showing the relative weight and quantification of the amount of waste generated. The final dried seed condiment comprised only 33.73% of the processed whole P. biglobosa seeds while the remaining 66.27% is considered as waste. Of these, the dried seed coat/testa constituent was 23.19%, and the effluents from the first and second stages of boiling were 27.39 and 2.08%, respectively. Relative to the weight of the processed seeds, 13.62% could be considered unrecovered matter, attributed to losses during sample handling, fermentation of the condiment, and moisture differences from the dried seed materials initially used in the processing. The high percentage of seed waste generated highlights the need to develop value-added applications for these by-products, ultimately improving the overall efficiency and sustainability of the food processing system. The first effluent [first effluent stage (EF1)] from the seed processing had a sticky texture, chocolate-like aroma, and dark brown color after concentration and drying. In contrast, the concentrated effluent from the EF2 displayed a crispier texture, lacked a distinct smell, and had a lighter color. These physicochemical differences indicate variation in phytoconstituents based on the boiling stage, as the first stage of boiling likely extracted more soluble compounds from the seeds, resulting in a more flavorful effluent after concentration. The chocolate-like aroma in EF1 could be attributed to Maillard reaction products typically found in some browned foods and cocoa bean processing. These compounds form as a result of interactions between amino acids and reducing sugars at high temperatures, resulting in a complex mixture of aromatic compounds (Shakoor et al., 2022). A report had previously indicated a similar characteristic smell in raw, roasted seeds of P. biglobosa known as either “Sudan coffee” or “café nègre” (Musara et al., 2020). This aromatic smell is similarly observed in the roasting of cocoa beans and the processing of other tree legumes (seeds and pod products), such as Ceratonia siliqua L (i.e., locust bean gum or carob powder), which have been recommended as a potential cocoa substitute (Loullis and Pinakoulaki, 2018; Quelal et al., 2023). These findings on the shared aroma characteristics between P. biglobosa seeds and these other tree legume seeds open up possibilities for further exploration and utilization of its by-products in various applications, similar to cocoa beans and locust bean gum; which have been well-developed for use in a range of industrial purposes related to flavor enhancement and flavor substitute for chocolate in food, cosmetics, shoe polish, insecticides among other application. Similarly, the starchy nature of the effluent when in contact with any piece of clothing/textile indicates the presence of starch-like compounds, which could potentially be utilized in textile sizing or as a stiffening agent. Locally, at the grassroots farm settings, this property might be harnessed as a binder in briquettes, contributing to the durability, density, and calorific value of biomass briquettes for domestic applications (Demeke et al., 2023; Ezéchiel et al., 2022). Indigenous communities in northern Nigeria have traditionally used locust bean pod waste and extracts as a sustainable construction material for building protection, as a binder, and as a soil enhancer (Bala, 2022). Notable examples of such sites include the ancient Gidan Makama and the city fencing of Kofar Na’isa, among others.
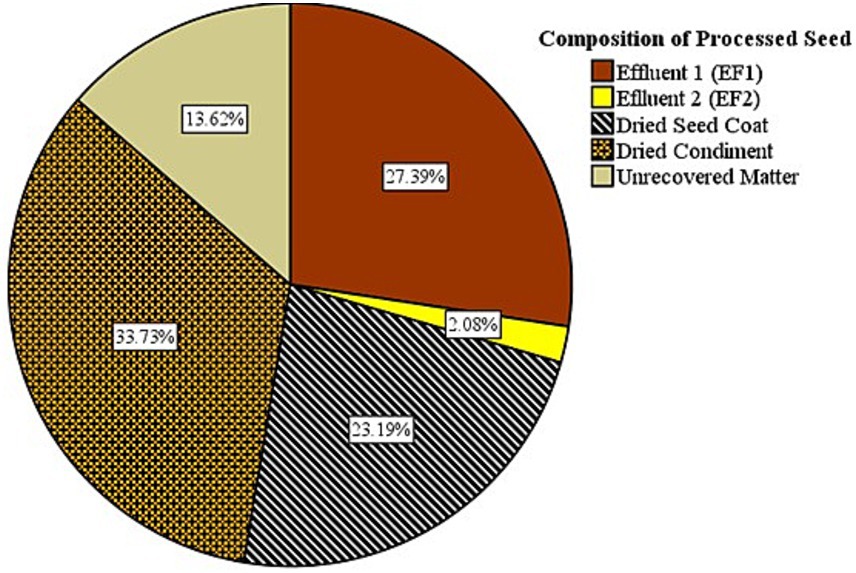
Figure 2. Percentage composition of condiments and by-products from processing seeds of Parkia biglobosa.
Further observations included the short and fibrous texture of the seed coats, along with their moisture absorbance capacity, which exceeded double their final dried weight at room temperature. In comparison, the processed wet cotyledon product also exhibited a significantly high moisture absorbance, approximately 3 times that of the final dried condiment. The moisture content analysis revealed that the dried condiment had a moisture content of 6.36 ± 0.09, while the seed coat samples showed a moisture content of 8.20 ± 0.08%. Similar to seed coats from another legume bean, the P. biglobosa seed coats have potential applications in moisture absorption, feed inclusion (as a source of dietary fiber), paper production, or as a filler in composite materials and other related sustainable agriculture practices. For example, a recent report by Babalola et al. (2016) suggested that dried seed coat waste and its cellulosic extract have potential applications in removing harmful metals and dyes from an aqueous environment. An increase in seed coat from similar legumes, such as soybean, in texturized vegetable protein has also been reported to lead to higher water absorption (Park et al., 2023). Another proximate result from P. biglobosa seed processing, as shown in Table 1, indicates that the dried seed coat has a higher fiber content (15.03 ± 0.13%) than the condiments (9.07 ± 0.10%). This is consistent with the fact that seed coats generally contain greater amounts of structural carbohydrates. Attuquaye et al. (2023) indicated the presence of some phytochemicals, mainly phenolic compounds, in P. biglobosa seed coat. Seed coats from lupin beans have been reported as a potentially valuable food ingredient due to their high dietary fiber content (79.84–86.59% total dietary fiber) among other properties (Zhong et al., 2020), and faba bean (Vicia faba) husk has been recommended for use in animal feed (Krenz et al., 2023), whereas fiber and cellulose from other legumes bean seed coat have been reported to have potential application for use as eco-paper for food packaging (Tassoni et al., 2020). While the seed coat shows promise as a possible ingredient in food ingredient and animal feed, caution and additional research would be appropriate in determining safety levels as legumes are known to have high amounts of antinutritive factors (Akinbisoye et al., 2024; Zhong et al., 2018). The higher crude fat content in the seed coat (8.37 ± 0.11%) than in the condiment (5.27 ± 0.11%), might have been influenced by the process of boiling, fermentation, and postprocessing, especially of the condiment. Conversely, the results of other higher proximate content, such as ash—2.83 ± 0.08% in the condiment and 2.47 ± 0.09% in the seed coat; protein—22.05 ± 0.12% in the condiment and 19.65 ± 0.10% in the seed coat; carbohydrate content—54.43 ± 0.17% in the condiment and 46.28 ± 0.24% in the seed coat; and total calculated energy—353.35 kcal/g for the condiment and 339.05 kcal/g for the seed coat, are consistent in support the use of the condiments as food. The proximate results are consistent with reports on similar legume plants, particularly regarding fiber content observed in the seed coat and starch as the main component in the dry matter of legumes, although protein content in legumes is generally significantly higher compared to reported in grain (Ozolina et al., 2023). Furthermore, the results of the mineral composition reveal that both the condiment and seed coat are good sources of calcium (9.96 mg/g in condiment and 8.893 mg/g in seed coat), magnesium (3.67 mg/g in condiment and 4.08 mg/g in seed coat), iron (2.01 mg/g in condiment and 2.23 mg/g in seed coat), and zinc (0.08 mg/g in condiment and 0.05 mg/g in seed coat), all which are beneficial for bone health, other physiological functions and metabolism, thus useful for biofortification (Kamboj and Nanda, 2018; Kumar and Pandey, 2020). The manganese (Mn) content was 0.08 mg/g in the condiment, while the seed coat contained 0.07 mg/g. Copper (Cu) content in the condiment was 0.03 and 0.03 mg/g in the seed coat. The level of chromium (Cr) content in the condiment was observed to be 0.01 and 0.01 mg/g in the seed coat. The presence of these minerals is significant, as the bioavailability of most minerals in peas is low due to their high phytate content (Kumar and Pandey, 2020). Both the condiment and the seed coat had negligible amounts of heavy metals such as cadmium, lead, and nickel, indicating that they are safe for consumption and can be considered for various food and non-food applications (Pastor et al., 2024).
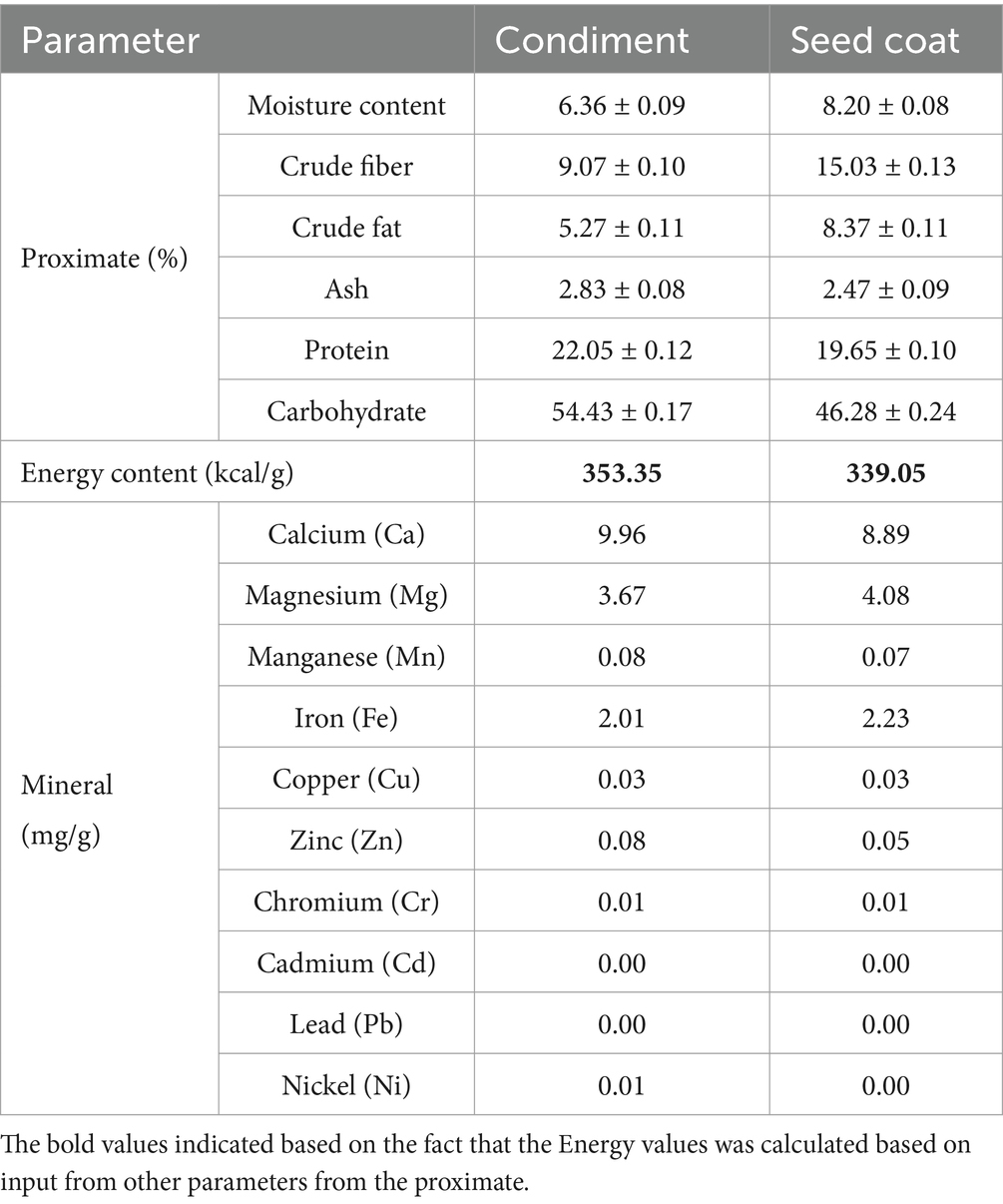
Table 1. Proximate and mineral analysis of condiment cotyledon and seed coat shaft from Parkia biglobosa seed processing.
A qualitative screening test on the effluents generated during the processing of P. biglobosa seeds identified the presence of carbohydrates and fatty acids (i.e., oil), shown in Table 2. The Molisch test—a general test for the presence of carbohydrates—was positive for both effluent samples EF1 and EF2. However, the negative results for reducing sugars such as monosaccharides and/or simple reducing disaccharides using the Fehling and Barfoed’s tests imply that the carbohydrates present are likely complex or non-reducing (Pandey et al., 2020). A qualitative test for protein was negative in the three protocols used for detection (Millon’s, Ninhydrin, and Biuret) in both effluents. This is somewhat surprising given that P. biglobosa seeds are reported to contain a significant protein content. It could be suggested that high temperatures during the boiling process could have potentially caused denaturation and precipitation of leached protein, thus they would not be detected in the effluent; cooking along with another processing step in legume beans such as soybean is known to be important in enhancing protein hydrolysis and reducing antinutritional compounds (Kohli and Singha, 2024; Zahir et al., 2020). Conversely, it is also possible that the protein content is primarily retained within the seed itself and not significantly released into the effluent (liquid phase) during the boiling process. Proteins in seeds are often embedded in a matrix that requires specific conditions to be broken down, such as enzymatic activity during germination or, in this case, specific processing methods such as fermentation. An additional notable finding is the presence of oils and fats as detected in both samples using the Sudan test in both EF1 and EF2 samples. Oils and fats play crucial roles as biomolecules with a wide range of biological functions, they have diverse applications in food and other industries. Their varied applications extend to the pharmaceuticals and cosmetics sectors, where they are typically employed as excipients, co-adjuvants, transdermal carriers, and skin emollients of importance in various formulations (Godlewska et al., 2022). Thus, the identification of oils and fats in the P. biglobosa seed effluent suggests that it could be a potential source of valuable lipid-based compounds and could be extracted for utilization in various related applications (Table 2).
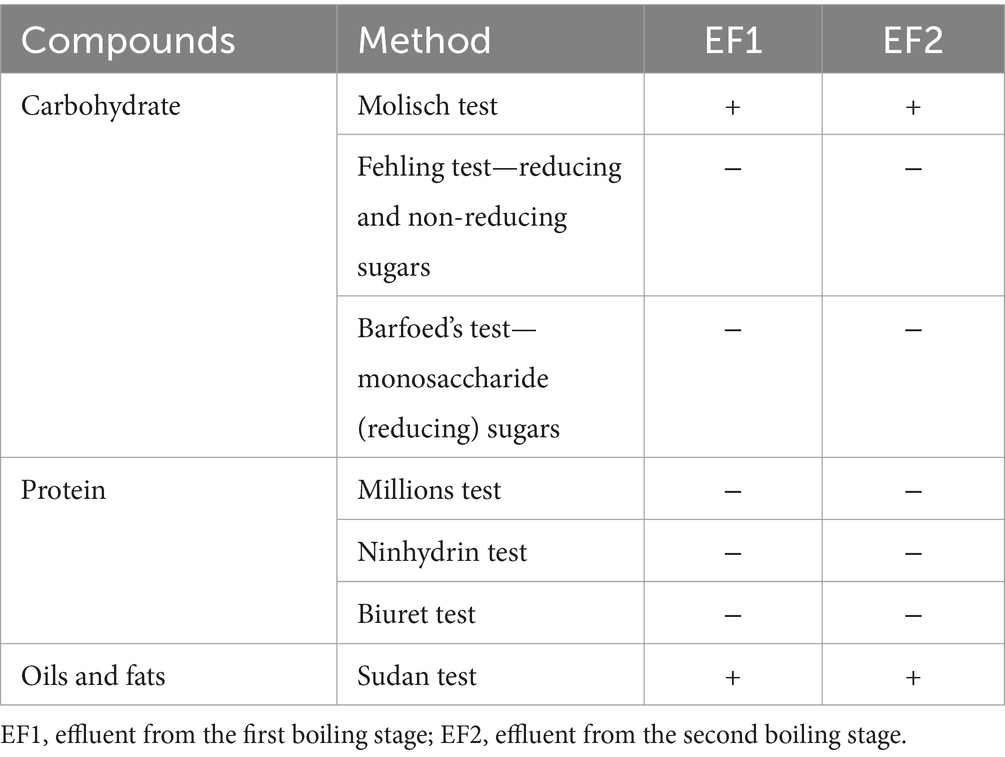
Table 2. Qualitative screening test for macromolecules in effluent from Parkia biglobosa seed processing.
The FTIR screening provides a qualitative assessment of the functional groups present in the concentrated effluents. The results suggest the presence of alcohols, alkenes, aromatic rings, carboxylic acids, and amines in the samples. A previous study by Aguda and Lateef (2021), also reported that various functional groups in the effluents from P. biglobosa seed were involved in the biosynthesis of silver nanoparticles. For this study, the FTIR spectra from the screening of the wavelength of functional groups in concentrated effluents are presented in Figure 3. In the analytical region, strong and broad peaks at 3385.37 cm−1 and 3404.48 cm−1 were observed for EF1 and EF2, respectively. These peaks correspond to the O–H stretching functional group, which suggests the presence of alcohols, and the broadness of these peaks reflects hydrogen bonding effects in both samples. Phenolic compounds are known phytochemicals that contain hydroxyl groups and possess a range of antimicrobial, antioxidant, and other biological activities (Sukhikh et al., 2022). At a wavelength of 2935.17 cm−1, a medium peak is representative of the C–H stretching functional group. The presence of C–H groups indicates the molecule’s overall hydrophobic character, which may influence the solubility, absorption, bioavailability, and membrane interaction in antimicrobial development (Hornemann et al., 2022; Monroe et al., 2020). Furthermore, peaks at 1606.98 and 1643.99 cm−1 for EF1 and EF2, respectively, are representative of the C=C stretching functional group for alkene and aromatic rings. Aromatic compounds are a class of cyclic, planar, and highly stable organic molecules that are widely found in natural products. The aromatic rings can serve as building blocks for the synthesis of more complex molecules and contribute to the biological activity and pharmacological properties of plant secondary metabolites (Suástegui and Shao, 2016). On the contrary, at the fingerprint region, medium peaks of 1401.88 cm−1 for EF1 and 1402.21 cm−1 for EF2 represent functional groups such as carboxylic acid and alcohol. At wavelengths of 1101.18 and 1078.16 cm−1 for EF1 and EF2, respectively, the medium peak is representative of C–N stretching (amine). Although the fingerprint region is highly informative and particularly significant, its interpretation can be somewhat probabilistic. Carboxylic acids, for example, are versatile and can participate in various organic reactions, such as esterification, amination, and reduction, making them valuable intermediates in organic synthesis. Regarding bioactivity, the presence of carboxylic acid in citric acid powder was suggested to be responsible for its antimicrobial activity against Staphylococcus aureus and Escherichia coli, as reported by Hilmi et al. (2019). The mechanism behind this activity involves the reduction of the local pH in bacteria, which leads to changes in bacterial membrane permeability. This change in permeability affects substrate transportation, ultimately leading to bacterial death. Similarly, the amine functional screened in the effluent FTIR, as opposed to the absence of protein detection in qualitative phytochemical tests, is indicative of compounds with nitrogen-containing functional groups, which could have different biological activities compared to proteins. The bioactivity of amines includes various enzymatic reactions, receptor interactions, and other biological macromolecules, reflecting their therapeutic profiles. Amines from plants, such as those found in Aloe species, exhibit therapeutic potential in pharmaceutical, nutraceutical, and cosmeceutical applications (Yadeta, 2024). However, in application, excessive consumption of certain biogenic amines can lead to toxicological effects, necessitating careful monitoring and analysis (Kabir et al., 2020).
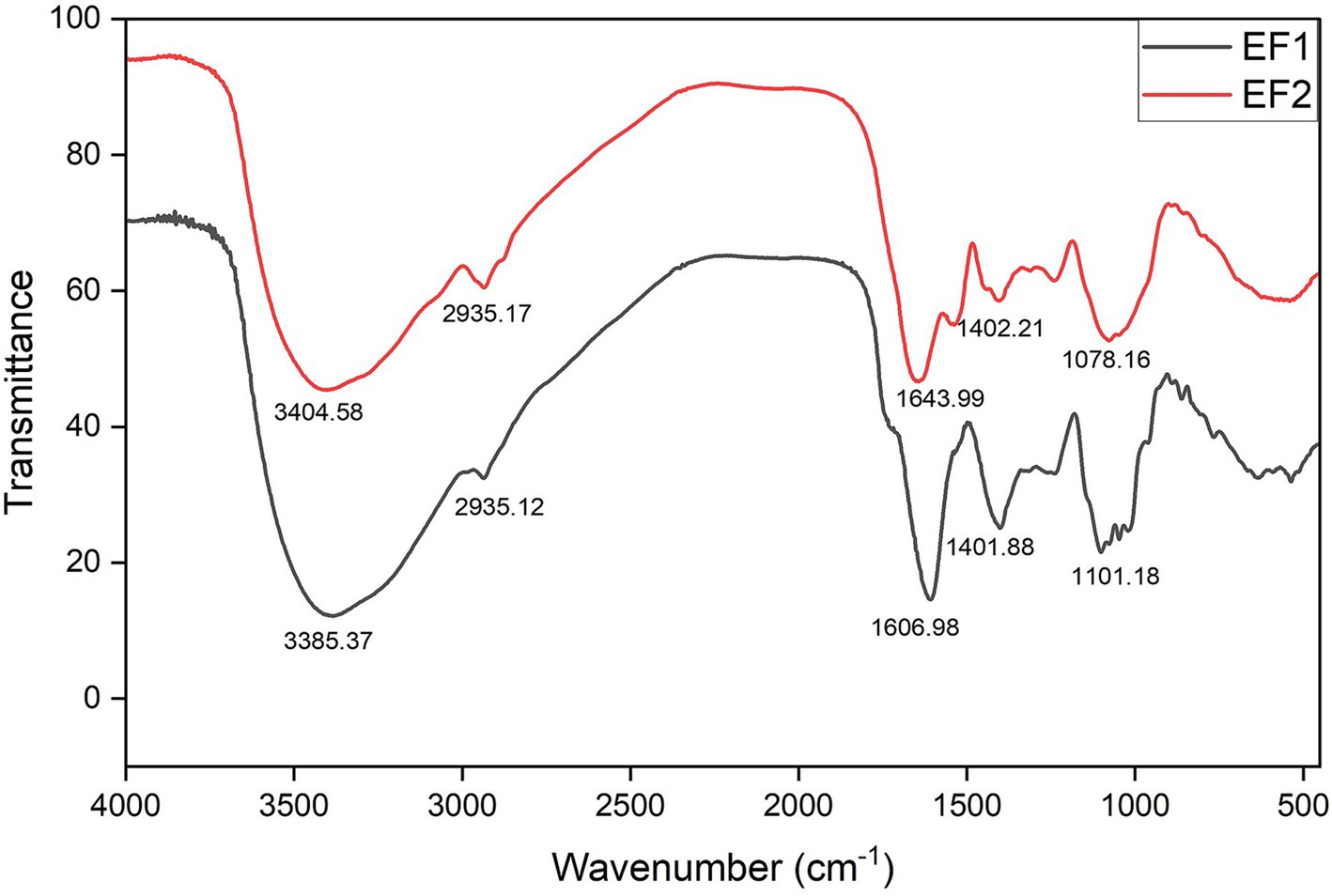
Figure 3. Fourier-transform infrared (FTIR) spectrograph of effluent 1 (EF1) and effluent 2 (EF2) from Parkia biglobosa seed processing.
The GC–MS analysis of the P. biglobosa seed effluent identified some noteworthy chemical compounds in the two extracts, especially fatty acids, most of which have known applications in the pharmaceutical and cosmetic industry. In the first effluent (EF1), shown in Table 3 Card-20(22)-enolide (C41H64O13) was identified at a proportion of 10.93%, followed by methyl 4-O-methyl-D-arabinopyranoside (C7H14O5) at 10.11%, and methyl 4-O-methyl-D-mannoside (C7H14O6) at 3.87%. Important fatty acids such as pentadecanoic acid, 14-methyl-, methyl ester (C17H34O2) accounted for 5.85%, while n-hexadecanoic acid (C16H32O2) was the most abundant at 19.30%. N-Hexadecanoic acid (i.e., palmitic acid) is a saturated fatty acid prevalent in natural fats and oils with nutritional significance. Its derivative, sodium palmitate, is widely used in cosmetics for its emollient properties. Recent studies have challenged historical negative health associations of palmitic acid, revealing anti-inflammatory, antioxidant, immune-boosting, and potential antitumor effects (Wang et al., 2023). Additionally, non-esterified palmitic acid exhibits bioactivity, mediating several biochemical and antimicrobial pathways to improve human health (Johnson and Abugri, 2014). Similarly, the fatty acid pentadecanoic acid, demonstrates safer and more extensive clinically relevant activities, particularly in reducing inflammation (Venn-Watson and Schork, 2023). Other identified compounds in the first effluent included 9,12-octadecadienoic acid, methyl ester, (E,E)- (C19H34O2, 4.57%), 11-octadecenoic acid, methyl ester (C19H36O2, 5.88%), and 9,12-octadecadienoic acid (Z,Z)- (C18H32O2, 11.82%), which indicates the diversity of fatty acids in the seed effluents. For example, 9,12-octadecadienoic acid (Z,Z)- commonly referred to as α-Linoleic acid, a polyunsaturated fatty acid essential for human health is found in plant seeds and proposed to serve as a functional food ingredient and dietary supplements in disease management (Yuan et al., 2014). Furthermore, GC–MS characterization of the first effluent indicated cannabidiol (C21H30O2) in two peaks, with relative areas of 12.63 and 15.04%. Cannabidiol is a known non-psychoactive, which makes it a compound of interest for potential therapeutic applications without the psychoactive effects (Campos et al., 2016; Martinez Naya et al., 2023). Thus, cannabidiol in the effluent from P. biglobosa seeds suggests potential health benefits and applications in nutraceuticals.
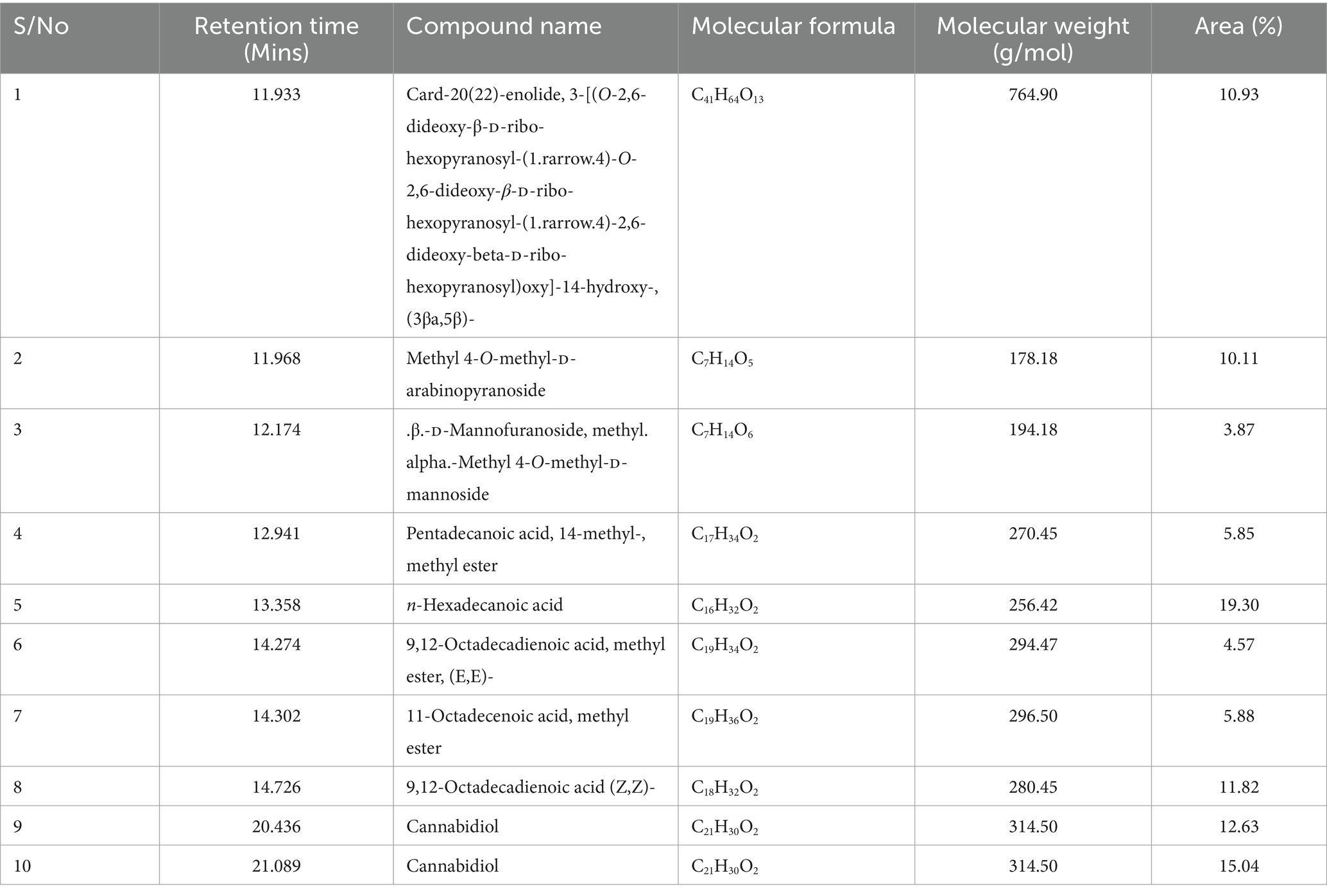
Table 3. Compounds identified in the first effluent stage (EF1) during Parkia biglobosa seed processing by gas chromatography–mass spectrometry (GC–MS).
In the EF2 from P. biglobosa seed processing, GC–MS characterization indicated the abundance of squalene, shown in Table 4 which comprised 44.09% of the total area. Squalene is a triterpene compound and a precursor in sterol biosynthesis and has gained attention for its diverse applications in skin protection in cosmetics, as well as for pharmaceutical and nutraceutical purposes. It is found in various natural sources, including deep-sea shark liver oil and several plant oils (Popa et al., 2015; Sumi et al., 2018). Similarly, Friedelan-3-one, another triterpenoid compound, was detected in various peaks, accounting for 31.06, 3.56, 1.64, and 2.08% of the total area. Friedelan-3-one exhibits anti-inflammatory, antioxidant, anticancer, and antimicrobial activities, with potential applications in agriculture due to its anti-insect effects (Singh et al., 2023). An in vitro report by Odeh et al. (2016) on friedelan-3-one from Pterocarpus santalinoides, demonstrated significant antimicrobial activity against various pathogens, including methicillin-resistant Staphylococcus aureus (MRSA) and Candida tropicalis, among others. Other compounds identified in the second effluent from P. biglobosa seed processing included hexadecane (2.30%), hexadecanoic acid methyl ester (2.98%), methyl stearate (3.61%), nonacos-1-ene (5.67%), 2,4,7,14-tetramethyl-4-vinyl-tricyclo[5.4.3.0(1,8)]tetradecan-6-ol (1.68%), and ethene, 1-(anthracen-9-yl)-2-(5-nitro-1-naphthyl)-, (E)- (1.34%). Of these compounds, hexadecanoic acid methyl ester (methyl palmitate), is the methyl ester of palmitic acid, which has been identified as a component in various biological sources and studied for its potential applications in cosmetic and pharmaceutical formulations. It demonstrates antibacterial activity against multidrug-resistant bacteria as characterized in clove alcoholic extract (Shaaban et al., 2021). A study by Cruz et al. (2020) on waste products from citrus essential oil purification has recommended fatty acid methyl esters as a sustainable source for biodiesel production. Methyl stearate, a fatty acid ester, specifically derived from stearic acid, has been reported to effectively inhibit Meloidogyne incognita in nematode management, reducing egg hatching and juvenile nematode survival while promoting banana plant growth (Lu et al., 2020). As a biodiesel component, it demonstrates favorable chemical kinetics (Naik et al., 2011). Its fatty acid characteristics also point to its potential use in cosmetic products, particularly for its emulsion properties and ability to improve product consistency.
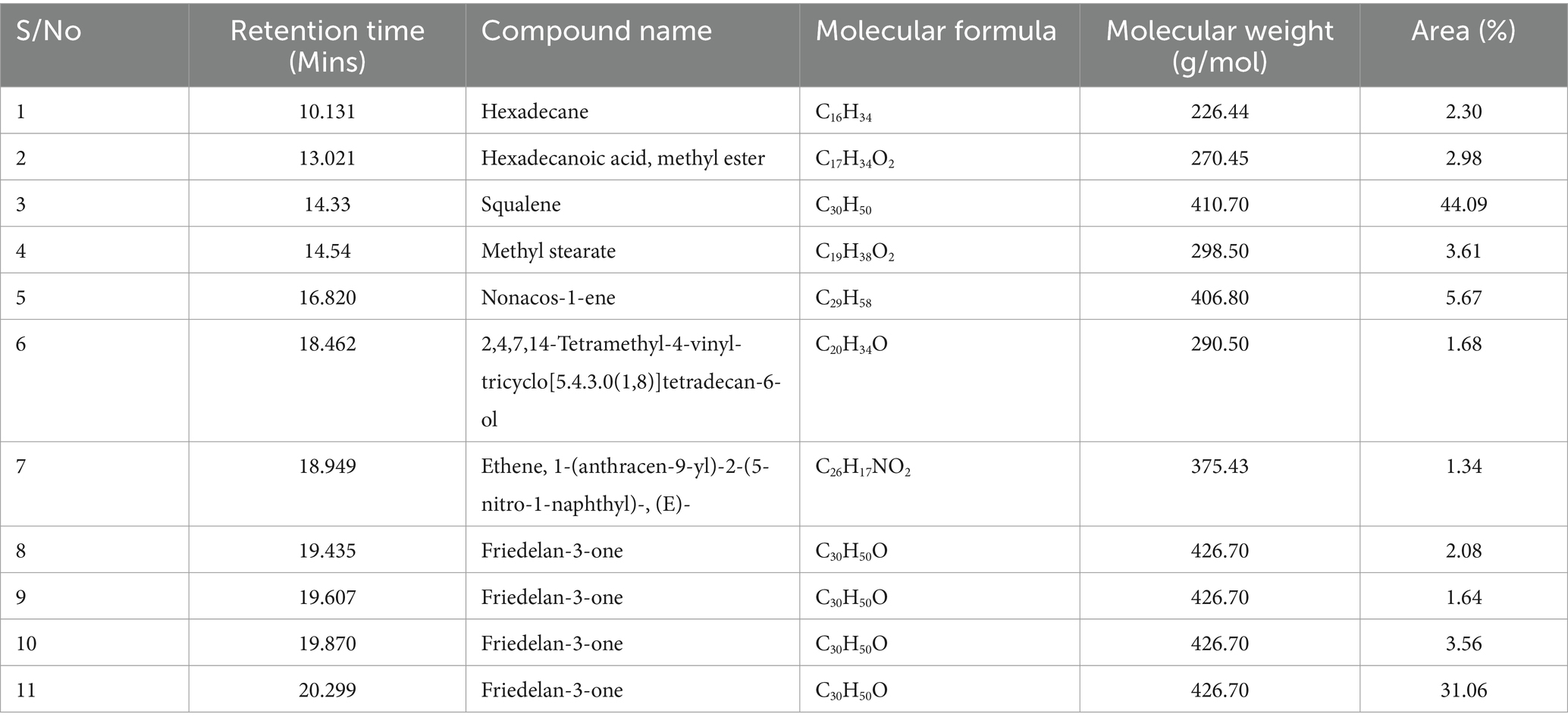
Table 4. Compounds identified in the second effluent stage (EF2) during Parkia biglobosa seed processing by gas chromatography–mass spectrometry (GC–MS).
The compounds identified from this study also help in re-examining previous discussions on the bioactivity of P. biglobosa (jacq) benth seed effluent and extracts, such as termiticidal activity, toxicity, and antibacterial activity. Studies by Olugbemi (2012) and Ojewumi et al. (2017) reported varied levels of termiticidal activity, although they employed different extraction methods and solvents on pulverized seeds of P. biglobosa. Although, the reported methodology by the authors are in contract to the by-product effluent from the aqueous boiling of whole seeds used in the traditional setting for termite control, as modeled in the present study. The study by Olugbemi (2012) specifically suggested that the presence of some heavy metals, as well as the interacting polar organic compounds in the seed extracts, was responsible for the termiticidal activity. However, observation from this study suggests that in addition to the possible presence of identified heavy metals, the GC–MS compounds identified could also have a significant effect on the termiticidal activity, especially as they relate directly to the traditional use of the effluent. Other studies indicating the bioactivity of effluents from P. biglobosa seed processing include research on biotoxicity in fish (Clarias gariepinus) (Amusat et al., 2020; Ojutiku et al., 2012) and potential in vitro antibacterial activity (Olukunle et al., 2019). Some results from these studies might be presumptive, especially as effluents were collected from local processing centers, and various factors, including the quality of water used, could have influenced results. The significant progress in the application of effluent from P. biglobosa seed processing is the synthesis of nanoparticles possessing antimicrobial activities for use in textiles. Thus, based on these reports and observed characterization results, the identified compounds could be explored for their potential use as natural additives in cosmetics, sources of pharmacological compounds, antimicrobial, eco-friendly pesticides, and preservatives, providing a safer alternative to synthetic chemicals. The potential economic benefit of valorization of the seed waste generated from P. biglobosa seed processing includes substantial reductions in environmental waste, the creation of new products of value, and additional job opportunities/diversified income streams for local communities. Furthermore, there is a consistent demand for the traditional P. biglobosa condiments across West Africa. This prompts for an easily scalable model, which can facilitate the integration of valorized products into existing supply chains.
4 Conclusion
This study on the potential valorization of food waste from P. biglobosa seed processing is justifiable based on the significant waste (66.27%) of the processed seeds not consumed, highlighting an opportunity for waste recovery and utilization. Various parts of the seed components can be repurposed; for example, the fibrous seed coat can be used as a biocomposite, a biodegradable material, or incorporated into animal feed. The effluent’s unique aromatic chocolate smell and the presence of characterized compounds can be used in food flavoring, cosmetic, and pharmaceutical industries. This could serve as a model across sub-Saharan Africa in converting food waste into valuable products as the region aspires to embrace sustainable practices and align with the global waste-to-wealth initiative. Future research should focus on specific optimization techniques and bioconversion for these by-products to maximize their industrial potential.
Data availability statement
The original contributions presented in the study are included in the article/supplementary material, further inquiries can be directed to the corresponding authors.
Author contributions
EO: Conceptualization, Data curation, Formal analysis, Funding acquisition, Investigation, Methodology, Software, Visualization, Writing – original draft, Writing – review & editing, Resources. AA: Methodology, Project administration, Supervision, Validation, Visualization, Writing – original draft, Writing – review & editing. AOw: Formal analysis, Investigation, Methodology, Project administration, Resources, Software, Visualization, Writing – original draft, Writing – review & editing. AOl: Data curation, Formal analysis, Methodology, Project administration, Validation, Writing – original draft, Writing – review & editing. YA: Formal analysis, Methodology, Validation, Writing – original draft, Writing – review & editing. CN: Investigation, Methodology, Software, Validation, Writing – original draft, Writing – review & editing. BA: Investigation, Methodology, Project administration, Resources, Writing – original draft, Writing – review & editing. ED: Resources, Supervision, Validation, Writing – original draft, Writing – review & editing. SO: Conceptualization, Methodology, Project administration, Resources, Supervision, Validation, Writing – original draft, Writing – review & editing.
Funding
The author(s) declare that financial support was received for the research, authorship, and/or publication of this article. The research work was stated as part of the PhD dissertation of the first author at Landmark University.
Acknowledgments
The authors express their gratitude to Nodza George of the Department of Botany at the University of Lagos for his assistance in facilitating the identification and authentication of plant samples at the University’s Herbarium.
Conflict of interest
A section of the work as part of a PhD dissertation is considered for patent by the first author.
The remaining authors declare that the research was conducted in the absence of any commercial or financial relationships that could be construed as a potential conflict of interest.
Publisher’s note
All claims expressed in this article are solely those of the authors and do not necessarily represent those of their affiliated organizations, or those of the publisher, the editors and the reviewers. Any product that may be evaluated in this article, or claim that may be made by its manufacturer, is not guaranteed or endorsed by the publisher.
References
Abraham, J. D., Sekyere, E. K., and Gyamerah, I. (2022). Effect of boiling on the nutrient composition of Solanum torvum. Int. J. Food Sci. 2022:7539151. doi: 10.1155/2022/7539151
Agrawal, K. M., and Methwani, N. S. (2019). Qualitative and quantitative phytochemical investigation of Trapa bispinpsa Roxb., leaves extract. World J. Pharm. Res. 7, 2144–2155. doi: 10.20959/wjpr20197-15194
Aguda, O., and Lateef, A. (2021). Novel biosynthesis of silver nanoparticles through valorization of Parkia biglobosa fermented-seed wastewater: antimicrobial properties and nanotextile application. Environ. Technol. Innov. 24:102077. doi: 10.1016/j.eti.2021.102077
Ajayi, O. A., Akinrinde, I. M., and Akinwunmi, O. O. (2015). Towards the development of shelf stable ‘iru’ (Parkia biglobosa) condiment bouillon cubes using corn, cassava and potato starch extracts as binders. Nigerian Food J. 33, 67–72. doi: 10.1016/j.nifoj.2015.04.006
Akinbisoye, A., Babarinde, G., Otutu, O., and Ade-Omowaye, B. (2024). Characterisation of pretreated African yam bean and Bambara groundnut seed coats for possible use in food formulations. Asian Food Sci. J. 23, 43–59. doi: 10.9734/afsj/2024/v23i8734
Akinola, R., Pereira, L. M., Mabhaudhi, T., de Bruin, F.-M., and Rusch, L. (2020). A review of indigenous food crops in Africa and the implications for more sustainable and healthy food systems. Sustain. For. 12:3493. doi: 10.3390/su12083493
Amusat, M., Ayeloja, A., Dada-Joel, O., and Eneh, K. (2020). Toxicity assessment of African locust bean effluents on agricultural soil and aquatic organism at Jimba-oja and Temidire locust bean (Parkia biglobosa) processing centers. IFE J. Sci. 22, 55–64. doi: 10.4314/ijs.v22i1.6
Amusat, M., Bolarin, F., Onyemize, C., Popoola, O., Dada-Joel, O., Ibrahim, A., et al. (2022). Effectiveness of African locust bean wastewater as a resource for preserving agricultural produce (grain as a case study). FUDMA J. Agric. Agric. Technol. 8, 223–226. doi: 10.33003/jaat.2022.0801.086
Asante, J. O., Oduro, I., Wireko-Manu, F., and Larbie, C. (2024). Assessment of the antioxidant and nutritive profile of Solanum nigrum and Solanum torvum swart leaves and berries. Appl. Food Res. 4:100438. doi: 10.1016/j.afres.2024.100438
Attuquaye, G. A. K., Somuah, D., Frimpong, T., and Badu, M. (2023). African locust bean (Parkia biglobosa) seed coat: a source of phenolic compounds. Food Hum. 1, 634–641. doi: 10.1016/j.foohum.2023.07.012
Babalola, J. O., Bamidele, T. M., Adeniji, E. A., Odozi, N. W., Olatunde, A. M., and Omorogie, M. O. (2016). Adsorptive modelling of toxic cations and ionic dyes onto cellulosic extract. Model. Earth Syst. Environ. 2, 1–15. doi: 10.1007/s40808-016-0246-z
Bala, I. (2022). Locust bean pod extract, a binder for landscape construction in Nigeria. Afr. J. Landsc. Architec. 4:9.
Bhat, R. (2021). “Sustainability challenges in the valorization of agri-food wastes and by-products” in Valorization of agri-food wastes and by-products. ed. R. Bhat (Academic Press), 1–27. Available at: https://www.sciencedirect.com/science/article/pii/B9780128240441000222
Bikila, A. M., Tola, Y., Esho, T. B., and Forsido, S. F. (2020). Effect of predrying treatment and drying temperature on proximate composition, mineral contents, and thermophysical properties of anchote (Coccinia abyssinica (lam.) Cogn.) flour. Food Sci. Nutr. 8, 5532–5544. doi: 10.1002/fsn3.1860
Bos-Brouwers, H., Langelaan, H., Sanders, J., van Dijk, M., and van Vuuren, A. (2012). Chances for biomass: integrated valorisation of biomass resources : Staff Publications - Wageningen University & Research, Issue. Available at: https://research.wur.nl/en/publications/chances-for-biomass-integrated-valorisation-of-biomass-resources
Burange, A., Clark, J. H., and Luque, R. (2016). Trends in food and agricultural waste valorization. In D. A. Atwood (Ed.), Sustainable inorganic chemistry (pp. 43–52). John Wiley & Sons, Ltd.
Campos, A. C., Fogaça, M. V., Sonego, A. B., and Guimarães, F. S. (2016). Cannabidiol, neuroprotection and neuropsychiatric disorders. Pharmacol. Res. 112, 119–127. doi: 10.1016/j.phrs.2016.01.033
Coulibaly Diakite, M., Parkouda, C., Compaore, S. C., and Savadogo, A. (2020). Traditional technologies for processing African locust bean seeds (Parkia biglobosa Jacq. R. Br.) in West Africa: review on the main derivatives and production constraints. J. Appl. Biosci. 152, 15698–15708. doi: 10.35759/JABs.152.8
Cruz, A. G., Mtz-Enríquez, A. I., Díaz-Jiménez, L., Ramos-González, R., Valdés, J. A. A., Flores, M. E. C., et al. (2020). Production of fatty acid methyl esters and bioactive compounds from citrus wax. Waste Manag. 102, 48–55. doi: 10.1016/j.wasman.2019.10.021
Demeke, E. D., Desta, M. A., and Mekonnen, Y. S. (2023). The potential of industrial sludge and textile solid wastes for biomass briquettes with avocado peels as a binder. Environ. Sci. Pollut. Res. 30, 86155–86164. doi: 10.1007/s11356-023-28493-x
Ezéchiel, K., Joel, T. K., Abdon, A., and Roger, D. D. (2022). Accessibility and effects of binder types on the physical and energetic properties of ecological coal. Heliyon 8:e11410. doi: 10.1016/j.heliyon.2022.e11410
Gérard, B. (2020). Sustainable intensification of African agriculture: a necessity, but not yet a reality. Front. Agr. Sci. Eng. 7, 383–389. doi: 10.15302/j-fase-2020361
Godlewska, K., Pacyga, P., Szumny, A., Szymczycha-Madeja, A., Wełna, M., and Michalak, I. (2022). Methods for rapid screening of biologically active compounds present in plant-based extracts. Molecules 27:7094. doi: 10.3390/molecules27207094
Guha, S., Acharya, S., and Chinnasamy, T. (2024). Phyto-assisted eco-friendly fabrication of haemocompatible vanadium nanoparticles using Azadirachta indica leaf extract inherent with antioxidant and anti-bacterial activity. Part. Sci. Technol. 42, 715–727. doi: 10.1080/02726351.2023.2281454
Gurusmatika, S., and Amira, W. (2021). The effect of food additive on physicochemical characteristics of seaweed stick snack and consumer acceptance. Canrea J. 4, 102–113. doi: 10.20956/canrea.v4i2.424
Hemalatha, R., Nivetha, P., Mohanapriya, C., Sharmila, G., Muthukumaran, C., and Gopinath, M. (2016). Phytochemical composition, GC-MS analysis, in vitro antioxidant and antibacterial potential of clove flower bud (Eugenia caryophyllus) methanolic extract. J. Food Sci. Technol. 53, 1189–1198. doi: 10.1007/s13197-015-2108-5
Hilmi, B., Bustami, Y., Trongsatitkul, T., and Hamid, Z. A. A. (2019). The effect of natural antimicrobial agents on Staphylococcus aureus and Escherichia coli growth. J. Phys. Sci. 30, 55–63. doi: 10.21315/jps2019.30.s2.5
Hornemann, A., Eichert, D. M., Hoehl, A., Tiersch, B., Ulm, G., Ryadnov, M. G., et al. (2022). Investigating membrane-mediated antimicrobial peptide interactions with synchrotron radiation far-infrared spectroscopy. ChemPhysChem 23:e202100815. doi: 10.1002/cphc.202100815
Ibraheem, S. A., Abdulameed, H. T., Jaafar, M., Tanimu, F. B., Anchau, H. G., Micah, M. M., et al. (2022). Functional characterization and biological properties of pectin from Parkia biglobosa pulp. Bioact. Carbohydr. Diet. Fibre 27:100300. doi: 10.1016/j.bcdf.2021.100300
Jain, R., Jayswal, S., and Maitreya, B. (2020). Phytochemical screening of Prosopis cineraria L. druce pods. Int. J. Res. Appl. Sci. Eng. Technol. 8, 2006–2010. doi: 10.22214/ijraset.2020.6328
Johnson, M., and Abugri, D. A. (2014). “Occurrence, biochemical, antimicrobial and health effects of palmitic acid” in Palmitic Acid. ed. L. F. Porto (Nova Science), 17–43. Available at: https://novapublishers.com/shop/palmitic-acid-occurrence-biochemistry-and-health-effects/
Kabir, A., Mocan, A., Piccolantonio, S., Sperandio, E., Ulusoy, H. I., and Locatelli, M. (2020). “Analysis of amines” in Recent advances in natural products analysis. eds. A. Sanches Silva, S. F. Nabavi, M. Saeedi, and S. M. Nabavi (Elsevier), 569–591. Available at: https://www.academia.edu/43272611/RECENT_ADVANCES_IN_NATURAL_PRODUCTS_ANALYSIS
Kamboj, R., and Nanda, V. (2018). Proximate composition, nutritional profile and health benefits of legumes-a review. Legume Res. Int. J. 41, 325–332. doi: 10.18805/LR-3748
Khan, I. A., Khan, S., and Bilal, H. (2024). Qualitative phytochemical screening of Buddleja crispa (roots and stem). Phytopharmacol. Res. J. 3, 1–7.
Kohli, V., and Singha, S. (2024). Protein digestibility of soybean: how processing affects seed structure, protein and non-protein components. Discov. Food 4:7. doi: 10.1007/s44187-024-00076-w
Krenz, L. M. M., Grebenteuch, S., Zocher, K., Rohn, S., and Pleissner, D. (2023). Valorization of faba bean (Vicia faba) by-products. Biomass Convers. Biorefinery, 1–18. doi: 10.1007/s13399-023-03779-9
Kumar, S., and Pandey, G. (2020). Biofortification of pulses and legumes to enhance nutrition. Heliyon 6:e03682. doi: 10.1016/j.heliyon.2020.e03682
Lemes, A. C., Coelho, M. A. Z., Gautério, G. V., de Paula, L. C., Filho, J. G. D. O., and Egea, M. B. (2022). “Industrial wastes and by-products: a source of functional foods, nutraceuticals, and biopolymers” in Biopolymers in nutraceuticals and functional foods. eds. S. Gopi, P. Balakrishnan, and M. Bračič (The Royal Society of Chemistry).
Loullis, A., and Pinakoulaki, E. (2018). Carob as cocoa substitute: a review on composition, health benefits and food applications. Eur. Food Res. Technol. 244, 959–977. doi: 10.1007/s00217-017-3018-8
Lu, Q., Liu, T., Wang, N., Dou, Z., Wang, K., and Zuo, Y. (2020). Nematicidal effect of methyl palmitate and methyl stearate against Meloidogyne incognita in bananas. J. Agric. Food Chem. 68, 6502–6510. doi: 10.1021/acs.jafc.0c00218
Maliki, M., Ikhuoria, E. U., and Aluyor, P. (2023). Proximate analysis of selected agricultural waste for their nutritional potential. North Afr. J. Food Nutr. Res. 7, 117–125. doi: 10.51745/najfnr.7.15.117-125
Mardiah, M., Andini, F., Hafiani, N., Fitrilia, T., and Widowati, S. (2020). Effect of drying method on physicochemical properties of pumpkin flour. Int. J. Adv. Sci. Technol. 29, 3174–3189.
Martinez Naya, N., Kelly, J., Corna, G., Golino, M., Abbate, A., and Toldo, S. (2023). Molecular and cellular mechanisms of action of Cannabidiol. Molecules 28:5980. doi: 10.3390/molecules28165980
Modi, R. (2019). “The role of agriculture for food security and poverty reduction in sub-Saharan Africa” in The Palgrave handbook of contemporary international political economy. eds. T. M. Shaw, L. C. Mahrenbach, R. Modi, and X. Yi-chong (Palgrave Macmillan UK), 391–410. Available at: https://www.springerprofessional.de/en/the-role-of-agriculture-for-food-security-and-poverty-reduction-/16354876
Mohd, M., Sehreen, R., and Ummyiah, H. M. (2022). “In situ and ex situ agricultural waste management system” in Agricultural waste, Ch. 3. eds. A. Fiaz and S. Muhammad (IntechOpen).
Monroe, J., Barry, M., DeStefano, A., Aydogan Gokturk, P., Jiao, S., Robinson-Brown, D., et al. (2020, 2020). Water structure and properties at hydrophilic and hydrophobic surfaces. Annu. Rev. Chem. Biomol. Eng. 11, 523–557. doi: 10.1146/annurev-chembioeng-120919-114657
Musara, C., Aladejana, E. B., Mudyiwa, S. M., and Karavina, C. (2020). Parkia biglobosa (Mimosaceae): botany, uses, phytochemical properties and pharmacological potential. J. Pharm. Nutr. Sci 10, 101–115. doi: 10.29169/1927-5951.2020.10.03.4
Naik, C. V., Westbrook, C. K., Herbinet, O., Pitz, W. J., and Mehl, M. (2011). Detailed chemical kinetic reaction mechanism for biodiesel components methyl stearate and methyl oleate. Proc. Combust. Inst. 33, 383–389. doi: 10.1016/j.proci.2010.05.007
Nguyen, T. L., Ora, A., Häkkinen, S. T., Ritala, A., Räisänen, R., Kallioinen-Mänttäri, M., et al. (2023). Innovative extraction technologies of bioactive compounds from plant by-products for textile colorants and antimicrobial agents. Biomass Convers. Biorefinery 14, 24973–25002. doi: 10.1007/s13399-023-04726-4
Ngwasiri, P. N., Ambindei, W. A., Adanmengwi, V. A., Ngwi, P., Mah, A. T., Ngangmou, N. T., et al. (2022). A review paper on agro-food waste and food by-product valorization into value added products for application in the food industry: opportunities and challenges for Cameroon bioeconomy. Asian J. Biotechnol. Bioresour. Technol. 8, 32–61. doi: 10.9734/ajb2t/2022/v8i330128
Nsude, O. P., Orie, K. J., Udeozo, P. I., Ogbobe, O., and Chime, C. C. (2022). Isolation, physicochemical and BET analysis of cellulose from Pentaclethra macrophylla Benth (oil bean) pod biomass wastes. Int. Res. J. Pure Appl. Chem. 23, 9–22. doi: 10.9734/irjpac/2022/v23i530474
Odeh, I. C., Tor-Anyiin, T. A., Igoli, J. O., and Anyam, J. V. (2016). In vitro antimicrobial properties of friedelan-3-one from Pterocarpus santalinoides L’Herit, ex dc. Afr. J. Biotechnol. 15, 531–538. doi: 10.5897/AJB2015.15091
Ojewumi, M. E., Ayomide, A. A., Obanla, O. M., Awolu, O. O., and Ojewumi, E. O. (2017). Pozzolanic properties of waste agricultural biomass-African locust bean pod waste. World J. Environ. Biosci. 6, 1–7.
Ojewumi, M. E., Eluagwule, B., Ayoola, A. A., Ogunbiyi, A. T., Adeoye, J., Emetere, M. E., et al. (2017). Termiticidal effects of African locust bean (Parkia biglobosa) seed oil extracts. Int. J. Curr. Res. 9, 53929–53934.
Ojutiku, R., Avbarefe, E., Kolo, R., and Asuwaju, F. (2012). Toxicity of Parkia biglobosa pod extract on Clarias gariepinus juveniles. Int. J. Fish. Aquacult. 4, 133–138. doi: 10.5897/IJFA11.118
Okunola, A. A., Adekanye, T., Ayooluwa, A., Okonkwo, C. E., Alake, S., Olayanju, T., et al. (2019). Development of a locust bean seed Dehulling cum washing machine. Int. J. Mech. Eng. Technol. 10, 1321–1330.
Okwunodulu, N., Osuagwu, C., Onwuzuruike, U., and Uzochukwu, U. (2021). Physicochemical, Atwater factor and acceptability changes of Moi-Moi from cowpea (Vigna unguiculate (L) Walp) as a function of white maize substitution. Sustain. Food Prod. 10, 1–16. doi: 10.18052/www.scipress.com/SFP.10.1
Olaniran, A. F., Okonkwo, C. E., Erinle, O. C., Owolabi, A. O., Ojediran, J. O., and Olayanju, T. A. (2020). Optimum boiling duration and its effect on nutritional quality and acceptability of mechanically Dehulled unfermented locust bean seeds. Prevent. Nutr. Food Sci. 25, 219–224. doi: 10.3746/pnf.2020.25.2.219
Olugbemi, B. (2012). Termiticidal activity of Parkia biglobosa (Jacq) Benth seed extracts on the termite Coptotermes intermedius Silvestri (Isoptera: Rhinotermitidae). Psyche 2012:869415.
Olukunle, O. F., Umar, O. K., and Oriola, O. B. (2019). Antimicrobial properties of cooked African locust beans (Parkia biglobosa) effluent with and without its chaff. Microbiol. Res. J. Int. 27, 1–9. doi: 10.9734/mrji/2019/v27i430105
Omodara, T., and Aderibigbe, E. (2018). Microbiological, physicochemical and enzymatic changes in fermented African locust bean (Parkia biglobosa) seeds using Bacillus subtilis and additives. Sustain. Food Product. 1, 22–29. doi: 10.18052/www.scipress.com/SFP.1.22
Orabueze, C. I., Ota, D. A., and Coker, H. A. (2020). Antimalarial potentials of Stemonocoleus micranthus harms (leguminoseae) stem bark in plasmodium berghei infected mice. J. Tradit. Complement. Med. 10, 70–78. doi: 10.1016/j.jtcme.2019.03.001
Owusu, A. A. (2012). Formulation and shelf life evaluation of avocado (Persea americana) fruit spread Kwame Nkrumah : University of Science and Technology. Available at: https://ir.knust.edu.gh/bitstream/123456789/4727/1/AKUA.pdf
Ozolina, K., Sarenkova, I., and Muizniece-Brasava, S. (2023). The anti-nutritional factors of legumes and their treatment possibilities: a review. Res. Rural Dev. 38, 68–76. doi: 10.22616/RRD.29.2023.010
Pandey, S., Goswami, S. K., and Jain, B. P. (2020). Protocols in biochemistry and clinical biochemistry : Academic Press. Available at: https://shop.elsevier.com/books/protocols-in-biochemistry-and-clinical-biochemistry/pandey/978-0-12-822007-8#full-description
Park, C. S., Seo, M. S., Jung, S. Y., Lee, S., Park, B., Park, S. Y., et al. (2023). Quality properties of texturized vegetable protein made from defatted soybean flour with different soybean seed coat contents. Korean J. Food Preserv. 30, 896–904. doi: 10.11002/kjfp.2023.30.5.896
Parkouda, C., Nielsen, D. S., Azokpota, P., Ivette Irène Ouoba, L., Amoa-Awua, W. K., Thorsen, L., et al. (2009). The microbiology of alkaline-fermentation of indigenous seeds used as food condiments in Africa and Asia. Crit. Rev. Microbiol. 35, 139–156. doi: 10.1080/10408410902793056
Pastor, K., Nastić, N., Ilić, M., Skendi, A., Stefanou, S., Ačanski, M., et al. (2024). A screening study of elemental composition in legume (Fabaceae sp.) cultivar from Serbia: nutrient accumulation and risk assessment. J. Food Compos. Anal. 130:106127. doi: 10.1016/j.jfca.2024.106127
Popa, O., Băbeanu, N. E., Popa, I., Niță, S., and Dinu-Pârvu, C. E. (2015). Methods for obtaining and determination of squalene from natural sources. Biomed. Res. Int. 2015:367202, 1–16. doi: 10.1155/2015/367202
Porwal, N., Gupta, B., Gakkhar, A. K., Tiwari, R. C., and Mittal, B. (2023). An evaluation of physicochemical parameters and quantitative phytochemical analysis of Datura metel-a research article. J. Ayurv. Herbal Integr. Med. 3, 1–13. doi: 10.29121/jahim.v3.i2.2023.30
Quelal, O. M., Hurtado, D. P., Benavides, A. A., Alanes, P. V., and Alanes, N. V. (2023). Key aromatic volatile compounds from roasted cocoa beans, cocoa liquor, and chocolate. Fermentation 9:166. doi: 10.3390/fermentation9020166
Rao, P., and Rathod, V. (2019). Valorization of food and agricultural waste: a step towards greener future. Chem. Rec. 19, 1858–1871. doi: 10.1002/tcr.201800094
Rațu, R. N., Veleșcu, I. D., Stoica, F., Usturoi, A., Arsenoaia, V. N., Crivei, I. C., et al. (2023). Application of agri-food by-products in the food industry. Agriculture 13:1559. doi: 10.3390/agriculture13081559
Ribeiro, T. B., Voss, G. B., Coelho, M. C., and Pintado, M. E. (2022). “Food waste and by-product valorization as an integrated approach with zero waste: future challenges” in Future foods. ed. R. Bhat (Elsevier), 569–596.
Roberts, O. I., and Okereke, C. I. (2017). Cultural beliefs on waste and the need for integration into present domestic waste management: evidence from selected communities in rivers state, Nigeria. Int. J. Soc. Sci. Manag. Res. 3, 1–12.
Sagar, N. A., Pareek, S., Sharma, S., Yahia, E. M., and Lobo, M. G. (2018). Fruit and vegetable waste: bioactive compounds, their extraction, and possible utilization. Compr. Rev. Food Sci. Food Saf. 17, 512–531. doi: 10.1111/1541-4337.12330
Sanni, J. A., Sanni, G. O., Awoniyi, R. R., Osanyinlusi, R., Richards, Y. E., Adesina, G. I., et al. (2024). Effects of processing on the proximate composition, mineral content and the phytochemical analysis of groundnut seeds (Arachis hypogeae). Biol. Med. Nat. Prod. Chem. 13, 63–71. doi: 10.14421/biomedich.2024.131.63-71
Sassi, M. (2023). Economic Connectiveness and pro-poor growth in sub-Saharan Africa: the role of agriculture. Sustain. For. 15:2026. doi: 10.3390/su15032026
Shaaban, M. T., Ghaly, M. F., and Fahmi, S. M. (2021). Antibacterial activities of hexadecanoic acid methyl ester and green-synthesized silver nanoparticles against multidrug-resistant bacteria. J. Basic Microbiol. 61, 557–568. doi: 10.1002/jobm.202100061
Shahidah, A. A., Farouq, A., Magashi, M., and Ibrahim, A. (2019). Taste profile and consumer preference of “dawadawa” produced from the seeds of Parkia biglobosa, Glycine max and Hibiscus sabdariffa. Int. J. Biol. Chem. Sci. 13, 178–185. doi: 10.4314/ijbcs.v13i1.15
Shakoor, A., Zhang, C., Xie, J., and Yang, X. (2022). Maillard reaction chemistry in formation of critical intermediates and flavour compounds and their antioxidant properties. Food Chem. 393:133416. doi: 10.1016/j.foodchem.2022.133416
Shifarew, T., Asere, T. G., Yitibarek, M., and Diro, A. (2024). Proximate analysis and mineral contents of atella from traditional tella brewers in Jimma City, Ethiopia. Bull. Chem. Soc. Ethiop. 38, 825–837. doi: 10.4314/bcse.v38i4.1
Singh, S. K., Shrivastava, S., Mishra, A. K., Kumar, D., Pandey, V. K., Srivastava, P., et al. (2023). Friedelin: structure, biosynthesis, extraction, and its potential health impact. Molecules 28:7760. doi: 10.3390/molecules28237760
Suástegui, M., and Shao, Z. (2016). Yeast factories for the production of aromatic compounds: from building blocks to plant secondary metabolites. J. Ind. Microbiol. Biotechnol. 43, 1611–1624. doi: 10.1007/s10295-016-1824-9
Sukhikh, S., Prosekov, A., Ivanova, S., Maslennikov, P., Andreeva, A., Budenkova, E., et al. (2022). Identification of metabolites with antibacterial activities by analyzing the FTIR spectra of microalgae. Life 12:1395. doi: 10.3390/life12091395
Sumi, E., Anandan, R., Rajesh, R., Ravishankar, C., and Mathew, S. (2018). Nutraceutical and therapeutic applications of squalene. Fish. Technol. 55, 229–237.
Tamasiga, P., Miri, T., Onyeaka, H., and Hart, A. (2022). Food waste and circular economy: challenges and opportunities. Sustain. For. 14:9896. doi: 10.3390/su14169896
Tassoni, A., Tedeschi, T., Zurlini, C., Cigognini, I. M., Petrusan, J. I., Rodríguez, Ó., et al. (2020). State-of-the-art production chains for peas, beans and chickpeas-valorization of agro-industrial residues and applications of derived extracts. Molecules 25:1383. doi: 10.3390/molecules25061383
Thomas, R. M., Falegan, C. R., Olojede, A. O., Oludipe, E. O., Awarun, O. D., and Daodu, G. O. (2023). Nutritional and sensory quality of Ofada rice sourdough bread made with selected lactic acid bacteria strains. Heliyon 9:e20828. doi: 10.1016/j.heliyon.2023.e20828
Ugwuanyi, J. O., and Okpara, A. N. (2019). Current status of alkaline fermented foods and seasoning agents of Africa. New Adv. Ferment. Process. :240.
Ukwubile, C. A., Ahmed, A., Katsayal, U. A., Ya’u, J., and Mejida, S. (2019). GC–MS analysis of bioactive compounds from Melastomastrum capitatum (Vahl) Fern. Leaf methanol extract: an anticancer plant. Sci. Afr. 3:e00059. doi: 10.1016/j.sciaf.2019.e00059
Venn-Watson, S., and Schork, N. J. (2023). Pentadecanoic acid (C15:0), an essential fatty acid, shares clinically relevant cell-based activities with leading longevity-enhancing compounds. Nutrients 15:4607. doi: 10.3390/nu15214607
Wang, X., Zhang, C., and Bao, N. (2023). Molecular mechanism of palmitic acid and its derivatives in tumor progression. Front. Oncol. 13:1224125. doi: 10.3389/fonc.2023.1224125
Yadeta, A. T. (2024). Chemical structures, biological activities, and medicinal potentials of amine compounds detected from Aloe species. Front. Chem. 12:1363066. doi: 10.3389/fchem.2024.1363066
Yuan, G.-F., Chen, X.-E., and Li, D. (2014). Conjugated linolenic acids and their bioactivities: a review. Food Funct. 5, 1360–1368. doi: 10.1039/C4FO00037D
Zahir, M., Fogliano, V., and Capuano, E. (2020). Effect of soybean processing on cell wall porosity and protein digestibility. Food Funct. 11, 285–296. doi: 10.1039/C9FO02167A
Zhong, L., Ali, H., Fang, Z., Wahlqvist, M. L., Hodgson, J. M., and Johnson, S. K. (2020). Lupin seed coat as a promising food ingredient: physicochemical, nutritional, antioxidant properties, and effect of genotype and environment. Int. J. Food Sci. Technol. 55, 1816–1824. doi: 10.1111/ijfs.14460
Keywords: food waste, valorization, Parkia biglobosa, chocolate flavor, waste to wealth, dietary fiber
Citation: Oludipe EO, Ajayi AO, Owolabi AO, Olojede AO, Afolabi YT, Nwonuma CO, Adewumi BG, Dada EO and Owa SO (2024) Food waste from Parkia biglobosa seed processing as a potential biomass resource for valorization. Front. Sustain. Food Syst. 8:1497536. doi: 10.3389/fsufs.2024.1497536
Edited by:
Guihun Jiang, Jilin Medical University, ChinaReviewed by:
Carolina Ramírez-López, National Polytechnic Institute (IPN), MexicoDi Chen, Zhengzhou University, China
Copyright © 2024 Oludipe, Ajayi, Owolabi, Olojede, Afolabi, Nwonuma, Adewumi, Dada and Owa. This is an open-access article distributed under the terms of the Creative Commons Attribution License (CC BY). The use, distribution or reproduction in other forums is permitted, provided the original author(s) and the copyright owner(s) are credited and that the original publication in this journal is cited, in accordance with accepted academic practice. No use, distribution or reproduction is permitted which does not comply with these terms.
*Correspondence: Emmanuel Olorunleke Oludipe, b2x1ZGlwZS5lbW1hbnVlbEBsbXUuZWR1Lm5n; Akinyomade Oladipo Owolabi, b3dvbGFiaS5ha2lueW9tYWRlQGxtdS5lZHUubmc=