- 1Shanxi Provincial Department-Municipal Key Laboratory Cultivation Base for Quality Enhancement and Utilization of Shangdang Chinese Medicinal Materials, Changzhi Medical College, Changzhi, China
- 2Shanxi Agriculture University, Taigu, China
Background: Forsythia suspensa (F. suspensa) is a plant of the Oleaceae family that is used as a medicinal plant. It is a traditional Chinese medicine that functions in clearing heat and detoxification. F. suspensa contains various phytochemicals, such as lignans, flavonoids, triterpenoids, and phenylethanolic glycosides; however, the main active phytochemical in F. suspensa is phillygenin, which has lipid-lowering, antioxidant, antihypertensive, and tyrosinase inhibitory effects. However, the content of phillygenin in F. suspensa is very low, limiting its application.
Methods: This study used wild F. suspensa leaves (FSL) from Lingchuan County (LC), Anze County (AC), Pingshun County (PS), and Pingding County (PD) in Shanxi Province as experimental materials. Through a single factor and orthogonal experimental design, the fermentation time, temperature, and humidity of F. suspensa leaf fermented tea (FSLFT) were optimized using a black tea fermentation machine. By comparing the differences in phillygrin and phillygenin content in FSL and FSLFT, the optimal production process for increasing phillygenin content in FSLFT was sought. This study also compared the differences in bioactive substance content and in vitro antioxidant and antibacterial functions of wild FSLFT from the four counties to evaluate the quality of wild FSLFT from the four counties.
Results: The optimal technological parameters of Forsythia suspensa leaf fermented tea (FSLFT): fermentation time of 2 h, fermentation humidity of 80%, fermentation temperature of 35°C, the experimental results showed that the order of influence of each factor on phillygenin content was C (fermentation temperature) > A (fermentation time) > B (fermentation humidity). The cellulase activity of FSLFT was significantly higher than that of FSL (P < 0.05). The total volatile compounds of the wild FSLFT from the four counties were 87 volatile aroma components, 13 of which were common aroma components, and the PCA scores ranged from high to low as follows: AZ-FSLFT > LC-FSLFT > PS-FSLFT > PD-FSLFT. The antioxidant effects of the FSLFT were as follows: AZ-FSLFT > LC-FSLFT > PS-FSLFT > PD-FSLFT. PD-FSLFT had the least inhibitory effect on both Gram-positive and Gram-negative bacteria, whereas AZ-FSLFT had the greatest inhibitory effect.
Conclusion: Fermentation promoted the conversion of phillygrin to phillygenin in FSL, which might be related to the increased cellulase activity in FSL during fermentation. The AZ-FSLFT had the best quality and functional activity, which made sense given its active ingredient content. The AZ-FSLFT had the best quality and functional activity, which made sense given its active ingredient content. This study contributes to the comprehensive development and utilization of F. suspensa leaves for economic and healthcare purposes.
1 Introduction
Forsythia suspensa is a well-known medicinal plant used in China, it has the effects of clearing away heat, detoxification, eliminating swelling and dispersing nodules, and evacuating wind heat, and is often used to treat diseases such as wind heat cold, the beginning of febrile disease, entering the camp with warm heat, high fever, thirst, hot shower and urinary closure, carbuncle, scrofula, breast carbuncle, erysipelas, and swelling toxins. It is known as the “holy medicine of the sore family” (Zhang et al., 2022; Yang et al., 2022). The self-pollination rate of wild F. suspensa is low; however, F. suspensa leaves are rich in resources. The main bioactive substances in F. suspensa leaves include lignans, phenylethanoid glycosides, terpenoids, and flavonoids. Additionally, F. suspensa leaves contain glucose, protocatechuic acid (EGCG), p-hydroxybenzoate (p-hydroxybenzoate), etc. F. suspensa leaves are highly safe, have low toxicity, and no mutagenic and teratogenic effects (Liu Y. et al., 2022). Studies have shown that F. suspensa leaves have antiviral (Wang et al., 2022b), antitumor (Wang et al., 2023b), antioxidant (Xiao et al., 2024), hypolipidemic the mechanism is related to the regulation of intestinal microbes (Gui et al., 2024), hypoglycemic (Wal, 2024), weight loss (Nishibe et al., 2021), anti-inflammatory, and analgesic effects (Guo et al., 2022a). In 2017, F. suspensa leaves were officially approved as a local characteristic food in Shanxi Province, which opened up broader prospects and research value for the development and utilization of F. suspensa leaves as a characteristic and advantageous resource in the field of food and health products.
Phillygenin is one of the main effective components of F. suspensa and is used as a marker for its quality control of F. suspensa (Liu Y. et al., 2024). Some studies have shown that phillyrin is not easily absorbed orally, and that it exerts its curative effect after decomposition into phillygenin in the body (Peng et al., 2020; Wang C. et al., 2023). It has been reported that phillygenin, which is more easily absorbed and has stronger pharmacological effects, is produced during fermentation of F. suspensa black tea (He et al., 2023). Phillygenin, the main pharmacodynamic base material of F. suspensa leaves, is produced by oxidative fermentation of phillgrin (Patyra et al., 2022). As a by-product of F. suspensa, most F. suspensa leaves have not been effectively used, except for a small part of the folk production of F. suspensa leaf tea, which has caused a great waste of resources. Few studies have been conducted on the enzymatic hydrolysis of phillyrin to produce phillygenin in F. suspensa leaves (Figure 1). Fungi in tea can produce cellulase, polyphenol oxidase, tannase, and other enzymes using tea as a substrate during the fermentation process (Yan K. et al., 2021). Cellulose is a multi-component complex enzyme system that is currently determined to contain three main components: endoglucanase, exoglucanase, and β-glucosidase; endoglucanase can randomly cleave cellulose chains to produce various sugars or oligosaccharides and generate additional exolytic sites; exoglucanase acts on cellulose chains to produce soluble cellulose dextrins and cellulose disaccharides; and β-glucosidase can hydrolyze cellulose disaccharides and oligosaccharides to produce glucose (Saldana-Mendoza et al., 2024). Liu S. et al. (2024) have found that the cellulase activity of Bacillus subtilis significantly increases during the post-fermentation tea heap process and is positively correlated with tea quality.
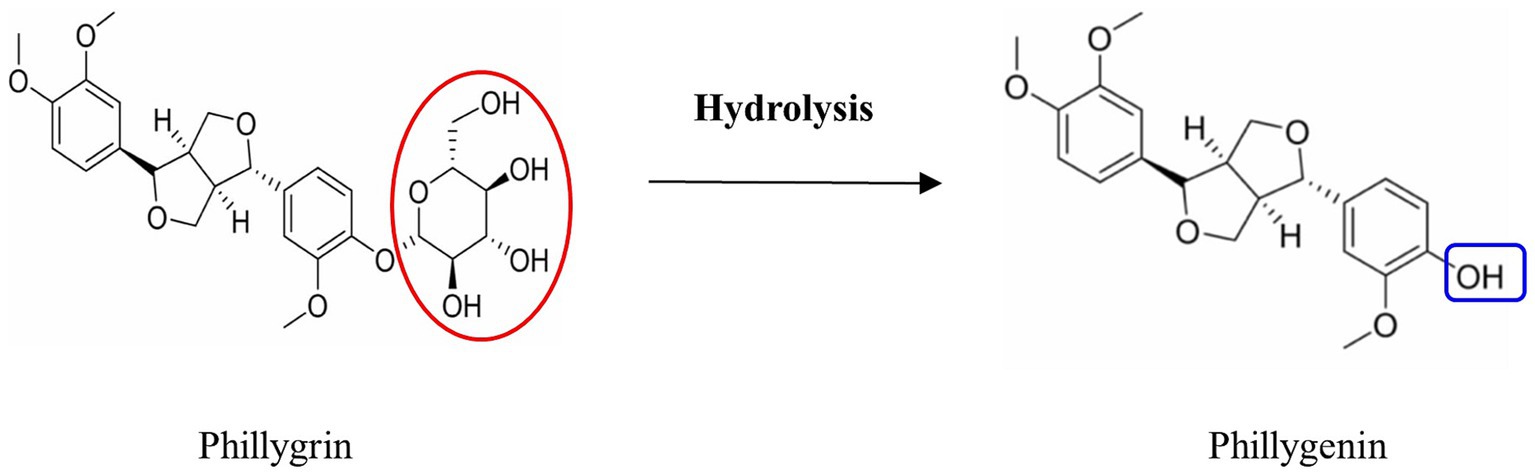
Figure 1. Schematic diagram of converting phillyrin to phillygenin. Image sourced from literature Zhou et al. (2022) and Wang et al. (2023).
This study utilized F. suspensa leaves as raw materials, employed fermented tea processing technology, and focused on the phillygenin content as the fermentation condition to determine the optimal fermentation process for FSLFT. By comparing the cellulase content of F. suspensa leaves and FSLFT, we sought to uncover the mechanism behind the conversion of phillygrin into phillygenin, thereby providing a reference for the preparation of phillygenin. Additionally, we investigated the aroma components and antioxidant and antibacterial properties of FSLFT, offering theoretical support for the development and production of FSLFT products and practical directions for enhancing the utilization rate of F. suspensa leaves.
2 Materials and methods
2.1 Plant material and strain
As raw materials for sample preparation, we collected a total of 60 wild and natural, pure, and sensory- normal wild Forsythia leaves from 30 sampling points in the main production areas of wild Forsythia suspensa in Shanxi Province: Lingchuan County (seven sampling points), Anze County (eight sampling points), Pingshun County (eight sampling points), and Pingding County (seven sampling points). Combine all the leaves gathered from each county in equal amounts and quarter them into approximately 500-gram portions. Sampling was conducted during the Qingqiao Era. The experiments were conducted at the Economic Crop Research Institute and the Horticultural Medicinal Tea Practice Center of the Shanxi Agricultural University.
2.1.1 Sample pretreatment
Four different Forsythia suspensa leaves (0.5 g) were accurately weighed and placed in a 50-mL centrifuge tube. Next, a 70% ethanol aqueous solution preheated to 70°C was used as the extraction solvent. Extraction was carried out for 60 min at an ultrasonic power of 300 W, solid–liquid ratio of 1 g/50 mL, and temperature of 60°C. Once the extraction was complete, the samples were transferred to a centrifuge and centrifuged them at 3500 rpm for 10 min. Subsequently, the filtrate was filtered with filter paper, transferred to a 25-mL volumetric flask, and shaken well after reaching the scale for subsequent use.
2.1.2 Strain
Escherichia coli (E. coli), Staphylococcus aureus, Bacillus subtilis (Henan Industrial Microbial Engineering Technology Research Center).
2.2 Regents
The main reagents used in this study were sodium carbonate (analytical grade, Shanghai Hengxing Chemical Reagent Manufacturing Co., Ltd.), Folin-phenol reagent, anhydrous ethanol, sodium nitrite (analytical grade, Beijing Solaibao Technology Co., Ltd.), ethyl acetate, aluminum nitrate (analytical grade, Tianjin Damao Chemical Reagent Factory), anthrone, concentrated sulfuric acid, sodium hydroxide, DPPH, potassium persulfate, ABTS, phosphate buffer solution, potassium ferrocyanide, trichloroacetic acid, FeCl3 (analytical grade, Beijing Solaibao Technology Co., Ltd.), methanol, glacial acetic acid (chromatographic grade, Beijing Solaibao Technology Co., Ltd.), beef paste, peptone, agar (Beijing Solaibao Technology Co., Ltd.), phillygenin, and phillyrin standard samples purchased from Tianjin Damao Chemical Reagent Factory.
2.3 The main instruments
The main instruments used in this study included a withering tank 6CWD (Zhejiang Shangyang Machinery Co., Ltd.), a rolling machine 6CR-40 (Zhejiang Shangyang Machinery Co., Ltd.), a 6CST-50 drum withering machine (Zhejiang Shangyang Machinery Co., Ltd.), a DL-6CHZ-9B tea baking machine (Quanzhou Deli), and a drying machine (01-2AB), Zhejiang Fuyang Tea Machinery General Factory, light incubator (303-5A, Shanghai Sunshine Experimental Instrument Co., Ltd.), constant temperature water bath (DK-S24, Shanghai Jinghong Co., Ltd.), electronic balance (Tianjin Taist Instrument Co., Ltd.), high-performance liquid chromatography (Agilent 1260LC, Agilent Technologies Co., Ltd.), ultraviolet spectrophotometer (UV-1750, Shimadzu Co., Ltd., Japan), rotary evaporator (RE-3000, Shanghai Yarong Biochemical Instrument Factory), circulating water vacuum pump (SHZ-III, Shanghai Yarong Biochemical Instrument Factory), enzyme-linked immunosorbent assay (ELISA) reader (Infinite M200, Deacon, Switzerland), TRACE 1300 ISDDQ gas chromatography–mass spectrometry (Thermo Fisher Scientific, United States), SH-R-RD, USA TX-5MS chromatographic column (30 m × 0.25 mm × 0.25 μ m), manual SPME injector, etc.
2.4 Single factor experiment and orthogonal experimental design of FSLFT
Fresh leaves of F. suspensa were selected according to a unified standard and processed into F. suspensa tea through withering, rolling, fermentation, and drying processes. Taking “AZ” F. suspensa leaves as raw materials, the optimal extraction conditions were explored by single factor test and orthogonal optimization test, taking phillygenin content in fermented tea as the index. The processing technology of the FSLFT was as follows: fresh F. suspensa leaves were evenly spread on a perforated stainless-steel tray with a thickness of 2–3 cm, and naturally spread and withered indoors. The room temperature was between 35°C, the relative humidity was between 50–80%, and it was flipped every 0.5 h. When the moisture content of fresh leaves dropped to 60%, the leaves were removed and kneaded for 40 min using alternating light pressure-heavy pressure-light pressure kneading; the rolled leaves were removed for fermentation and placed in a light incubator at 25–45°C for 1–5 h; Then, the tea was dried twice in sections and the fragrance was increased at 95°C for 25 min to produce FSLFT (Hao et al., 2024).
We conducted a single factor experiment with a fixed fermentation time of 3 h, fermentation temperature of 30°C, and fermentation humidity of 70% to analyze the effects of different fermentation times (1, 2, 3, 4, and 5 h), temperatures(20°C, 25°C, 30°C, 35°C, and 40°C), and humidity (55, 65, 75, 85, and 95%) on the content of phillygenin in FSLFT, and determined the optimization intervals for each influencing factor.
Based on a single-factor experiment, orthogonal experiments were carried out for fermentation time (h), fermentation temperature (°C), and fermentation humidity (%). The experimental factors and level design are listed in Table 1 and were repeated three times. The optimal technological conditions for the FSLFT were determined using the phillygenin content in the FSLFT as an index.
2.5 Determination of aroma and functional components of FSLFT
2.5.1 Determination of aroma components
We used Head Space-Solid Phase Microextraction (HS-SPME) to extract the scents from the tea and then used GC–MS to identify and quantify the fragrance composition (Qu et al., 2021).
The HS-SPME method consisted of placing a 0.5 g sample in an empty top bottle and inserting it into a manual input sample with PDMS extraction head fibers. The extraction heads were heated to 90°C and allowed to extract for 30 min. Following extraction, we promptly withdrawn the extractive head and inserted the GC–MS into the sample, maintaining a temperature of 250°C for 5 min.
The gas chromatography (GC) settings consisted of an HP-5MS color column with a flow rate of 1.0 mL/min. The input sample was then heated to 250°C. The heating process began at 80°C and was increased by 4°C per minute until it reached 200°C. It subsequently increased at a rate of 8°C/min until it reached 50°C in 5 min. The flow ratio was 20:1.
The conditions for the mass spectrometry (MS) analysis were as follows: the electron impact (EI) ion source used had an ionization voltage of 70 electron volts (eV). The ion source temperature was set at 230°C. The scan range for the analysis was 10–500 atomic mass units (aum). A solvent delay of 3 min was used.
We determined the relative peak areas of the volatile components of leaf-fermented tea subjected to various treatments. We then used the NIST14.L spectrum to determine the structure and names of the compounds, successfully matching more than 800 results.
2.5.2 The determination of phillgenin and phillygrin
Please refer to Nhu-Trang et al. (2023) for the techniques used to ascertain the phillygenin and phillygrin concentrations. Using an Agilent 1,260 instrument, we analyzed phillygenin and phillygrin in the FSLFT. Phillygenin and phillygrin were separated using an Agilent TC-C18 column (5 μm, 4.6 × 250 mm). The mobile phase consisted of methanol [1] and 0.1% acetic acid [3]. The linear gradient was as follows: flow rate, 1.0 mL/min. The injection volume was 10 μL, detection time was 45 min, and wavelength was 229 nm. The column temperature was maintained at 28°C.
2.5.3 Determination of cellulase activity
Extraction method of cellulase solution from F. suspensa leaves and FSLFT (Oanh et al., 2023): We took 1.5 g of F. suspensa leaves or FSLFTs, added 0.6 g polyvinylpyrrolidone, 0.2 g quartz sand, and an appropriate amount of precooled pH 5.0 citric acid buffer. After thorough grinding and mixing on ice, added the corresponding buffer to a volume of 10 mL, let it stand at 4°C for 24 h, we took it out, centrifuged at 8000 r/min for 10 min at 4°C. The supernatant was used as the crude enzyme solution.
Determination of cellulase activity (Liu L. et al., 2022): we took a 0.5 mL enzyme solution into a 10 mL centrifuge tube, then added 2 mL preheated mass fraction 1.0% carboxymethyl cellulose sodium (CMCS) (pH 4.8), placed it in a constant temperature water bath at 45°C for 30 min, immediately removed the centrifuge tube and added 2 mL of 3,5-dinitrosalicylic acid solution (DNS) to terminate the reaction. The centrifuge tube was boiled in boiling water for 5 min, immediately removed, and cooled with running water, and the optical density (OD) was measured at a wavelength of 540 nm using a spectrophotometer. We added 2.0 mL of CMCS was added to the control tube, 2 mL of DNS solution was added, and enzyme solution (0.5 mL) was added. The other operations were the same as the sample determination method mentioned above. Enzyme activity definition: Under specific reaction conditions, the amount of enzyme required to hydrolyze a substrate to produce 1 μmol of reducing sugar in 1 min is 1 U.
2.5.4 Determination of total polyphenol content
We determined the total polyphenol content in the sample using a spectrophotometer (Tian et al., 2022) and moderately adjusted it. In summary, 1 mL of diluted tea extract was added to a 10 mL volumetric flask, added 5.0 mL of 10% Folin phenol reagent was added to the centrifuge tube, and the mixture was gently shaken. After allowing the reaction mixture to stand for approximately 5 min, we added 4.0 mL of 7.5% Na2CO3 solution was added, and the volume was fixed to the scale with distilled water. The mixture was gently shaken and allowed to react for approximately 60 min to complete the color reaction, and absorbance was measured at 765 nm using a Shimadzu UV-1800 UV/visible scanning spectrophotometer. We expressed the TPCS in tea as gallic acid equivalents or extracts (mg/mL).
2.5.5 The method for determination of total flavonoid content
We used the NaNO2-Al (NO3) 3-NaOH method to determine the total flavone content of the samples. Refer to the study (Tian et al., 2022) for a brief description. We added 0.50 mL of the extract was added to 10 mL volumetric flasks, added 0.3 mL of a 5% NaNO2 solution was added, shaken well, and allowed to stand for 6 min. We then added 0.3 mL of 10% Al (NO3) 3 solution was added to each volumetric flask, shaken well again, and allowed to stand for 6 min. Finally, we added 4.0 mL of 4% NaOH solution was added to each volumetric flask, diluted with water to the scale line, shaken well, and allowed to stand for 10 min to complete the color reaction. Finally, absorbance was measured at 510 nm using a Shimadzu UV-1800 UV/visible scanning spectrophotometer. The extract was prepared using the rutin equivalent extract method (mg/mL).
2.5.6 Determination method for soluble sugar content
To determine the soluble sugar concentration, we used anthrone colorimetry as described previously. We combined sulfuric acid solution containing 4 mL of 0.033% anthrone. After heating for 7 min at 100°C, the mixture was cooled for 30 min. Next, we measured the absorbance at 620 nm using a Shimadzu UV-1800 UV/visible scanning spectrophotometer, with glucose as the standard. The results were expressed in milligrams of glucose per milliliter of extract.
2.6 Determination of in vitro antioxidant capacity and antibacterial activity of FSLFT
2.6.1 Determination of antioxidant capacity
2.6.1.1 DPPH radical scavenging rate
Based on the study (Setiawan et al., 2021), we mixed the sample solution with the same amount of DPPH ethanol solution, kept it out of the light for 30 min, measured its absorbance at 517 nm, and labeled it as a samp. An equal volume mixture of the sample solution and ethanol solution was used as the control group, while DPPH and ethanol solutions were used as the blank group. The DPHH clearance was calculated using the following formula:
2.6.1.2 Iron reduction force determination
Refer to the study (Zhou et al., 2022). As soon as we mixed 0.5 mL the sample solution, 2.5 mL, phosphoric acid buffer solution (2.5 mL), and potassium ferricyanide solution, we heated them to 50°C and allowed them to sit there for 20 min. To cool them down quickly, we put them in an ice bath and added 2.5 mL of 10% trichloroacetic acid. We mixed it and then put 2.5 mL of the supernatant, distilled water (2.5 mL), and 0.5 mL of 0.1% ferric chloride in a centrifuge. The reaction was set at 25°C for 10 min, the absorbance was measured at 700 nm, and the results were recorded as an OD700 sample.
2.6.1.3 ABST+ radical-scavenging rate
In a previous study (Wu et al., 2021), we combined 7 mmol/L ABST+ solution with a volume of 2.45 mmol/L mercury peroxide solution, after which the mixture underwent an ophthalmic reaction for 12 h. Prior to the experiment, phosphate buffer was used to thin out the reserve liquid so that it had an absorption value of 0.70 ± 0.03 at a wavelength of 734 nm. We mixed the 0.2 mL sample and 0.8 mL ABST+ diluted solution in a 37°C water bath for 10 min, and then measured the intensity of their absorption at that wavelength. The clearance rate was calculated using the following formula:
2.6.2 Determination of antibacterial activity
We used the disk diffusion method to determine the effect of tea extract on Escherichia coli, Staphylococcus aureus, and Bacillus subtilis, with reference to the study (Widatalla et al., 2022) and some modifications. To prepare the bottom plate, approximately 20 mL Mueller-Hinton agar was poured into a sterile Petri dish. Using a sterile cotton swab, we streaked approximately 0.1 mL of the standard bacterial stock suspension (108–109 CFU/mL) onto the substrate and prepared 20 mg/mL of tea extract stock solution with distilled water, using three dilutions of 5, 10, and 20 mg/mL. Additionally, we immersed a sterilized filter paper tray with a diameter of 6 mm in the tea extract solution before placing it on the surface of the test bacterial plate. The diameter of the inhibition zone was measured after incubating the plates for 24 h.
2.7 Data analysis
Data were expressed as “mean ± standard error of mean ( ± SEM),” analyzed by one-way ANOVA, and compared by Tukey’s method for multiple comparisons; p < 0.05, significant difference; p < 0.01 an extremely significant difference.
3 Results
3.1 Optimization of fermented tea process with Forsythia suspensa leaf
Figure 2A showed that the phillygenin concentration of FSLFT progressively increased as the fermentation duration increased, peaking at 3 h, and then gradually declining. Figure 1B showed that as the fermentation temperature increased, the phillygenin concentration gradually increased until it reached its optimal level at 35°C, after which it decreased. During this process, phillygenin content fluctuated over time, particularly at 40°C, where it declined significantly. Figure 1C demonstrated a positive link between the FSLFT phillygenin concentration and an increase in relative humidity, as long as the relative humidity remained below 75%. When the relative humidity reached 75 percent, FSLFT phillygenin content peaked. We chose 70, 75, and 80% as the orthogonal optimization intervals for fermentation relative humidity.
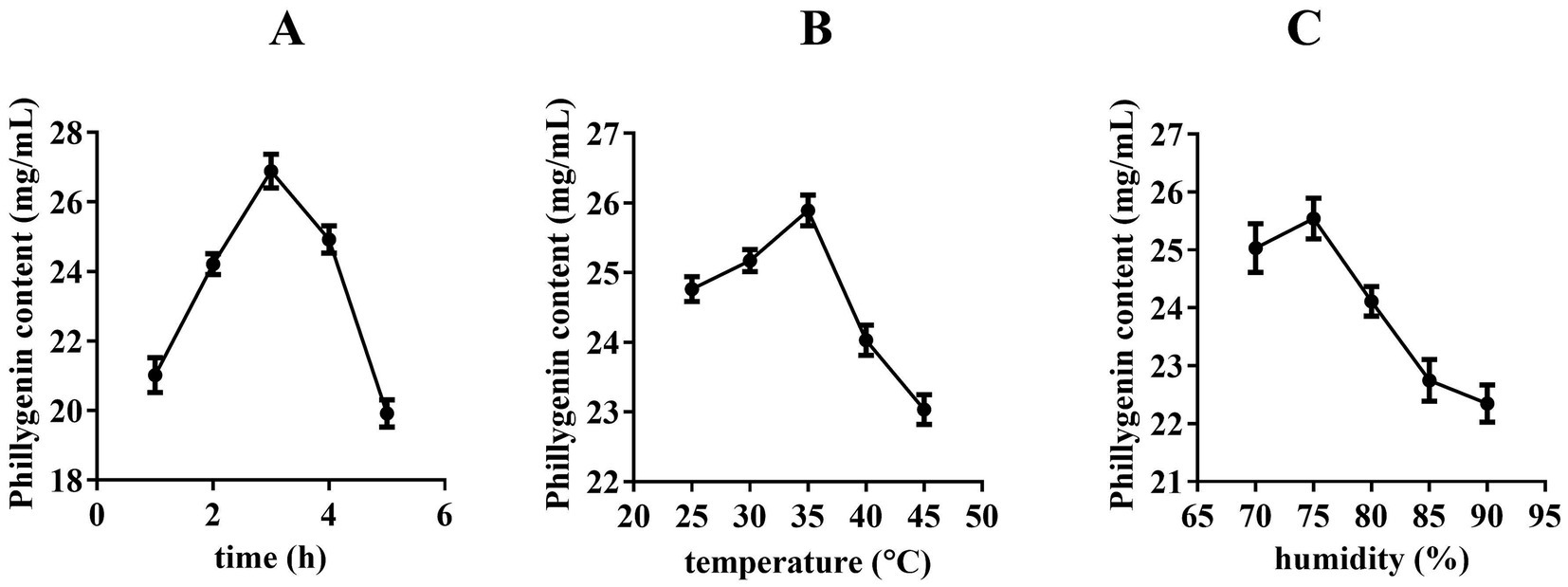
Figure 2. Effect of fermentation time, temperature, humidity on phillygenin content (A) Time, (B) Temperature, (C) Humidity.
Three factors were selected for the L9 (33) orthogonal experiment based on a single-factor experiment: fermentation time, relative humidity, and fermentation temperature. We used phillygenin content as an inspection index to identify the factors that significantly influenced the test results and established the ideal technological conditions for FSLFT. By comparing the R values (range) of each index in Table 1, we determined the order in which each factor influenced phillygenin content: C > A > B. This indicated that fermentation temperature was the most important factor, followed by fermentation time, and fermentation humidity was the least influential factor. Based on the values of k1, k2, and k3 for each index in Supplementary Table S1, we determined the optimal combination for each factor and found that A2B3C3 was the best combination. Supplementary Table S2 shows that the variance analysis results indicated that fermentation time and temperature had a significant effect on phillygenin content. Ultimately, we selected A2B3C3 as the optimal combination, which involved a 2-h fermentation time, 8 fermentation humidity of 80%, and a fermentation temperature of 35°C. We conducted three repeated trials of the process condition, with phillygenin levels of 25.82 mg/g, 25.86 mg/g, and 25.93 mg/g, respectively, with an average of 25.87 mg/g, indicating the excellent stability of this process.
3.2 The contents of phillyrin and phillygenin and the cellulase activity
From Table 2, we can see that the concentrations of phillyrin and phillygenin in the leaves were AZ-FSLFT > LC-FSLF > PS-FSLFT > PD-FSLFT; the concentrations of phillyrin in fermentation tea were LC-FSLFT > PD-FSLFT > AZ-FSLFT > PS-FSLFT; and the concentrations of phillygenin in fermentation tea were AZ-FSLFT > LC-FSLFT > PS-FSLFT > PD-FSLFT. Compared to the leaves, the phillyrin content of fermented tea in the four counties decreased by 71.70, 74.36, 70.94, and 74.03%, respectively, and the phillygenin content increased by 12.02, 12.21, 12.92, and 12.03 times.
Interestingly, there was no significant difference in cellulase activity in fresh leaves of F. suspensa from the four regions (Figure 3A). After fermentation, cellulase activity in the FSLFTs from the four regions was significantly increased (Figure 3C). The cellulase activity in FSLFTs from the four regions was in the order AZ-FSLFT > LC-FSLFT > PS-FSLFT > PD-FSLFT, and the cellulase activity in FSLFTs from the AZ and PS regions was significantly higher than that from LC and PD (Figure 3B). These results suggest that the phillygenin content in FLFT from AZ county was the highest, which may be related to the high content of phillyrin in F. suspensa leaves from this region and the highest cellulase activity during the fermentation process.
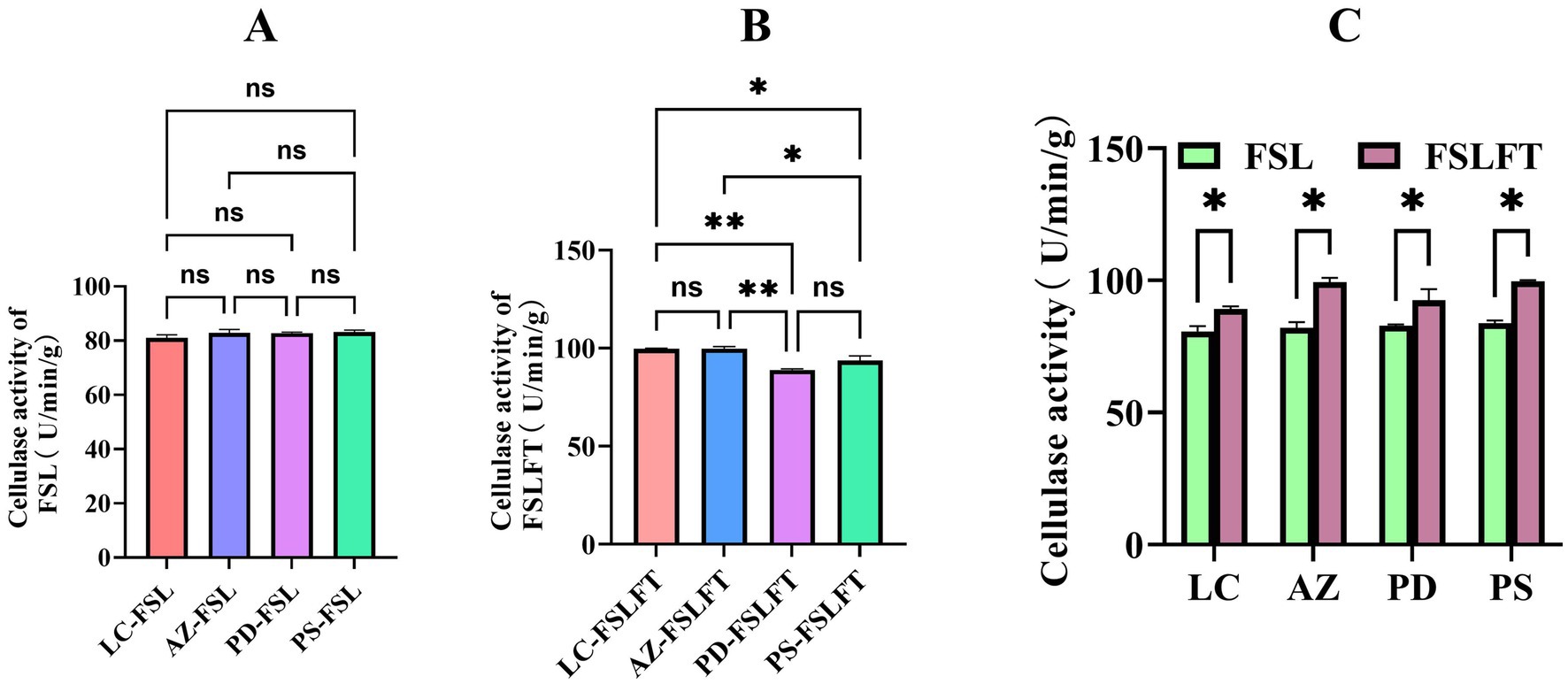
Figure 3. The cellulase activities of FSL and FSLFT (A) cellulase activity of leaves, (B) cellulase activity of tea, (C) comparison of cellulase activity of leaf and tea.
3.3 Analysis of functional components in FSLFT
Figure 4A showed that AZ-FSLFT had a significantly higher total flavonoid content than LC. Both AZ-FSLFT and PD-FSLFT had significantly higher total flavonoid contents than PS-FSLFT, with LC-FSLFT having a significantly higher total flavonoid content than PS-FSLFT. The total amount of polyphenols in the LC-FSLFT was much higher than that in the PS-FSLFT, as shown in Figure 3B. Figure 3C shows that the amount of soluble sugar in the AZ-FSLFT was much higher than that in the LC-FSLFT and PS-FSLFT. Similarly, the amount of soluble sugar in the PL-FSLFT was much higher than that in the PS-FSLFT.
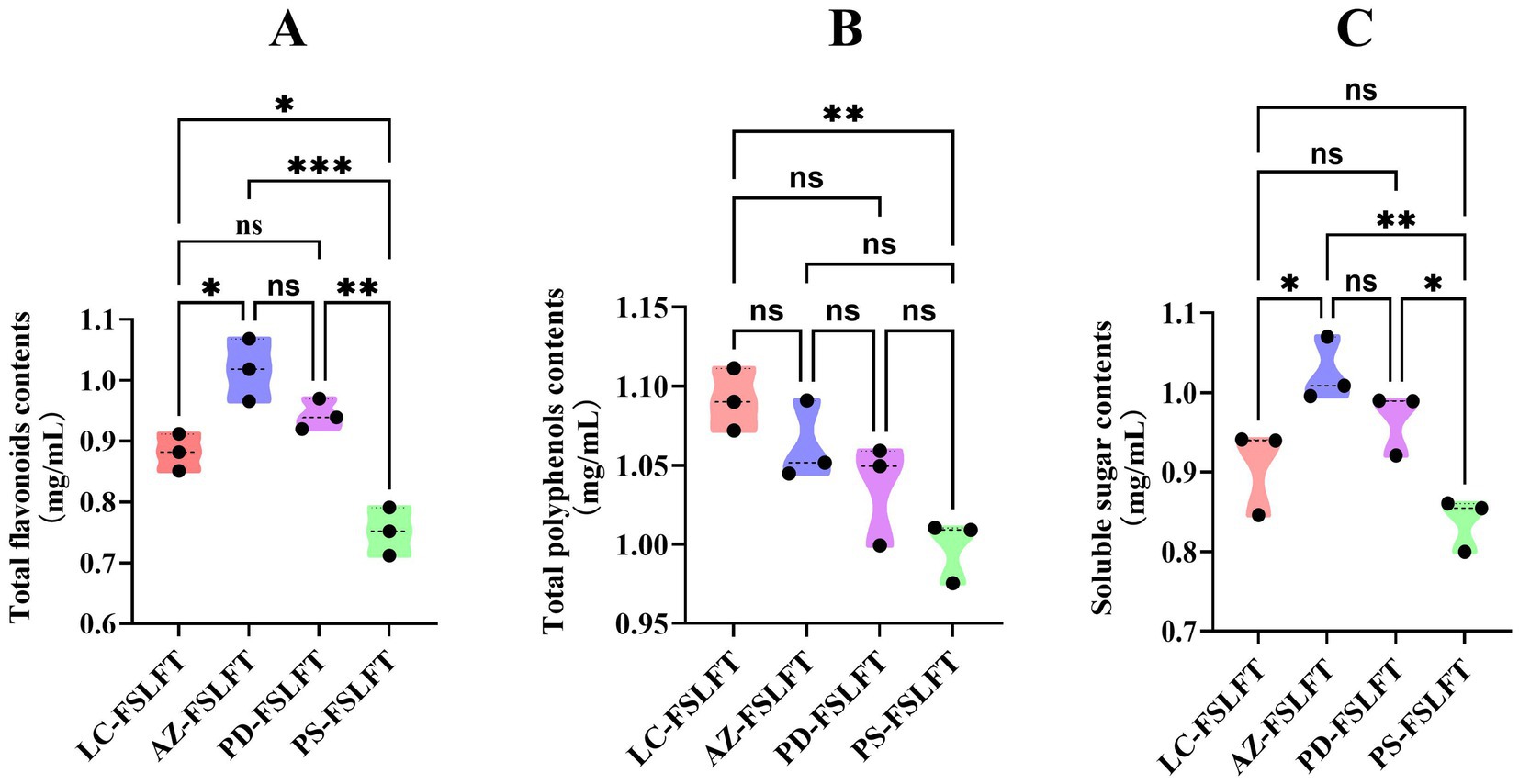
Figure 4. functional components in FSLFT (A) The total flavonoids contents, (B) The total polyphenols contents, (C) Soluble sugar contents.
3.4 Analysis of aroma components in FSLFT
Supplementary Table S3 showed that four distinct varieties of fermented tea contained 87 volatile compounds. The AZ-FSLFT found 41 volatile substances with an absolute content of 114.82 mg/g; the PL-FSLFT found 38 volatile substances with a total content of 102.36 mg/g; the LC-FSLFT found 46 volatilizers with an absolute content of 91.48 mg/g; and the PS-FSLFT found 50 volatilizers with a composite content of 115.94 mg/g. The total volatile compounds of the four FSLFTs from high to low were as follows: PS-FSLFT > LC-FSLFT > AZ-FSLFT > PL-FSLFT, and the absolute content of the total components from high to low was PS-FSLFT > AZ-FSLFT > PL-FSLFT > LC-FSLFT. The gas chromatography–mass spectrometry (GC–MS) gas chromatograms of the FSLFTs from different origins are shown in Supplementary Figure S1.
The four fermentation teas contained 13 volatile aroma ingredients (see Supplementary Table S4). We used principal component analysis to evaluate the volatility of the ingredients in different fermenting teas, as illustrated in Figure 5. The AZ-FSLFT fragrances mostly contained 2-methylbutanal, (+)-limonene, and isobutyraldehyde. The majority of PL-FSLFT fragrances contained 2-Ethylfuran, β-Cyclocitral, isovaleraldehyde, and (E, E)-2,4-heptadienaldehyd. The main components of the LC-FSLFT were linalool, 2,2,6-trimethycyclohexanome, β-Lonone, β-pinene, and (E, E)-2-4-heptadienaldyde. PS-FSLFT primarily contained linalool, 2,2,6-trimethycyclohexanome, lonone, pinene, 1-diethoxyethane and hexanal.
As shown in Table 3, AZ-FSLFT had the highest scores on the second and third main ingredients, 2.639 and 1.316, respectively. LC fermented tea had a maximum score of 3.348 for the first main ingredient. Based on the fragrance composition, we ranked the combined score of the fermented tea as AZ-FSLFT > LC-FSLFT > PS-FSLFT > PD-FSLFT, suggesting that AZ-FSLFT had the highest fragrance quality.
3.5 Analysis of antioxidant capacity of FSLFT
Figure 6 showed the antioxidant capacity of the FSLFT extract. As the mass concentration went from 0.1 mg/mL to 0.5 mg/mL, the DPPH free radical scavenging rate (Figure 6A), FRAP iron ion reducing power (Figure 6B), and ABTS scavenging rate (Figure 6C) all went up. This showed that the four tea extracts are related in a good manner. At 0.5 mg/mL, LC-FSLFT, AZ-FSLFT, and PD-FSLFT fermented tea scavenged more DPPH-free radicals than PS-FSLFT (Figure 6D). The order of FRAP-reduced iron ions was AZ-FSLFT > LC-FSLFT > PS-FSLFT > PD-FSLFT, and significant differences were observed (Figure 6E). AZ-FSLFT and LC-FSLFT had significantly higher ABTS scavenging rates than PD-FSLFT and PS-FSLFT (Figure 6F).
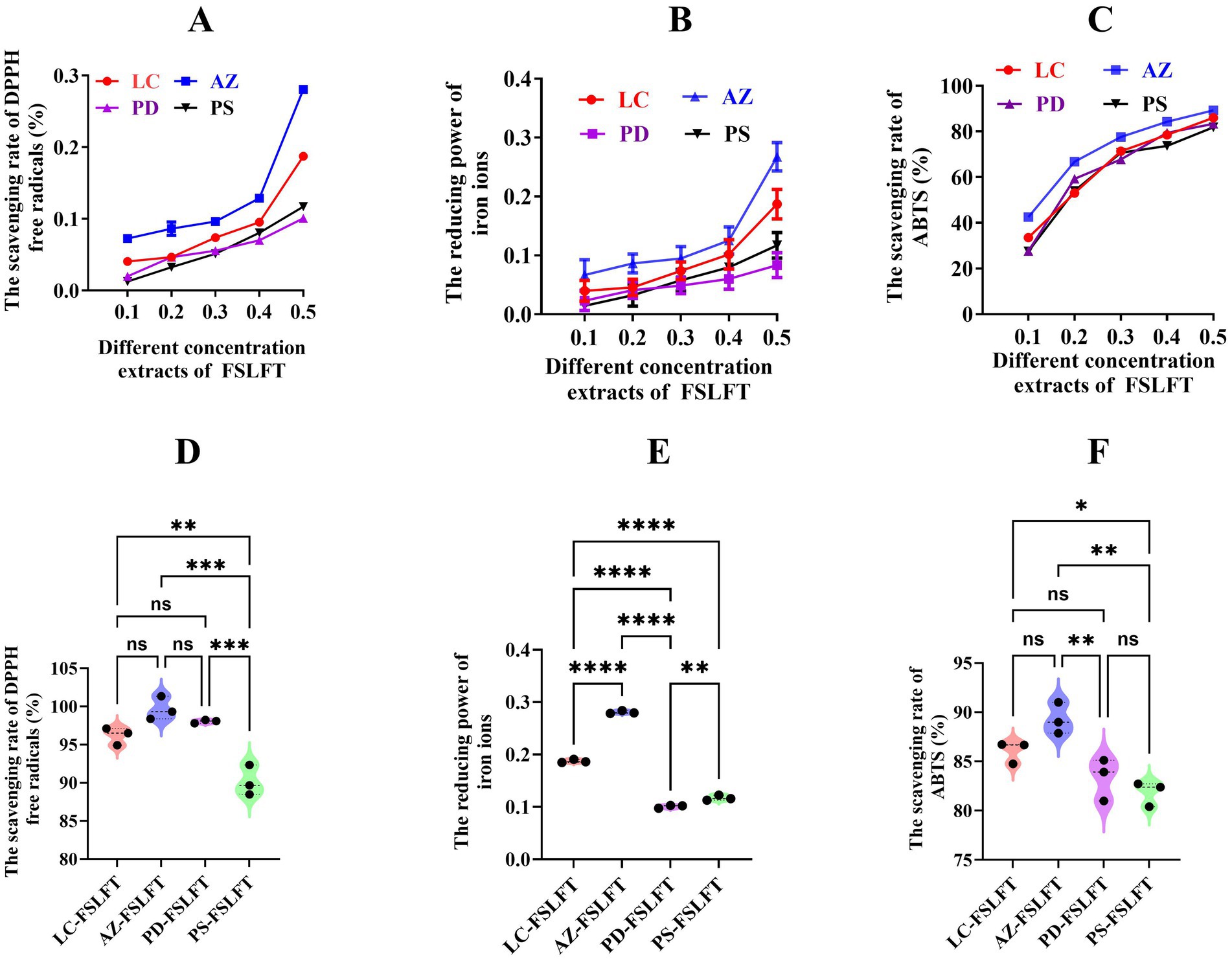
Figure 6. The antioxidant capacity of FSLFT in the four counties. (A) The scavenging rate of DPHH in the different concentration extracts. (B) The reducing power of iron ions in the different concentration extracts. (C) The scavenging rate of ABTS in the different concentration extracts. (D) The scavenging rate of DPHH in 0.5 mg/mL extracts. (E) The reducing power of iron ions in 0.5 mg/mL extracts. (F) The scavenging rate of ABTS in in 0.5 mg/mL extracts.
3.6 Analysis of antibacterial function of FSLFT
Figure 7A showed notable variations among the four fermented teas, with their inhibitory effect on Staphylococcus aureus rated in descending order as AZ-FSLFT > LC-FSLFT > PS-FSLFT > PD-FSLFT. Figure 7B showed a significant difference between the four fermented teas, with no significant difference between LC-FSLFT and PS-FSLFT, and a descending order for the inhibitory effect of teas on Escherichia coli: AZ-FSLFT > LC-FSLFT > PS-FSLFT > PD-FSLFT. Figure 7C showed the descending ranking of the inhibitory effects of the four fermented teas on Bacillus subtilis: AZ-FSLFT > LC-FSLFT > PS-FSLFT > PD-FSLFT. The differences between the various fermented teas were significant but not between PD-FSLFT, PS-FSLFT, or LC-FSLFT. According to these findings, among the four fermented tea extracts, PD-FSLFT had the least inhibitory effect on both Gram-positive and Gram-negative bacteria, whereas AZ-FSLFT had the greatest inhibitory effect.
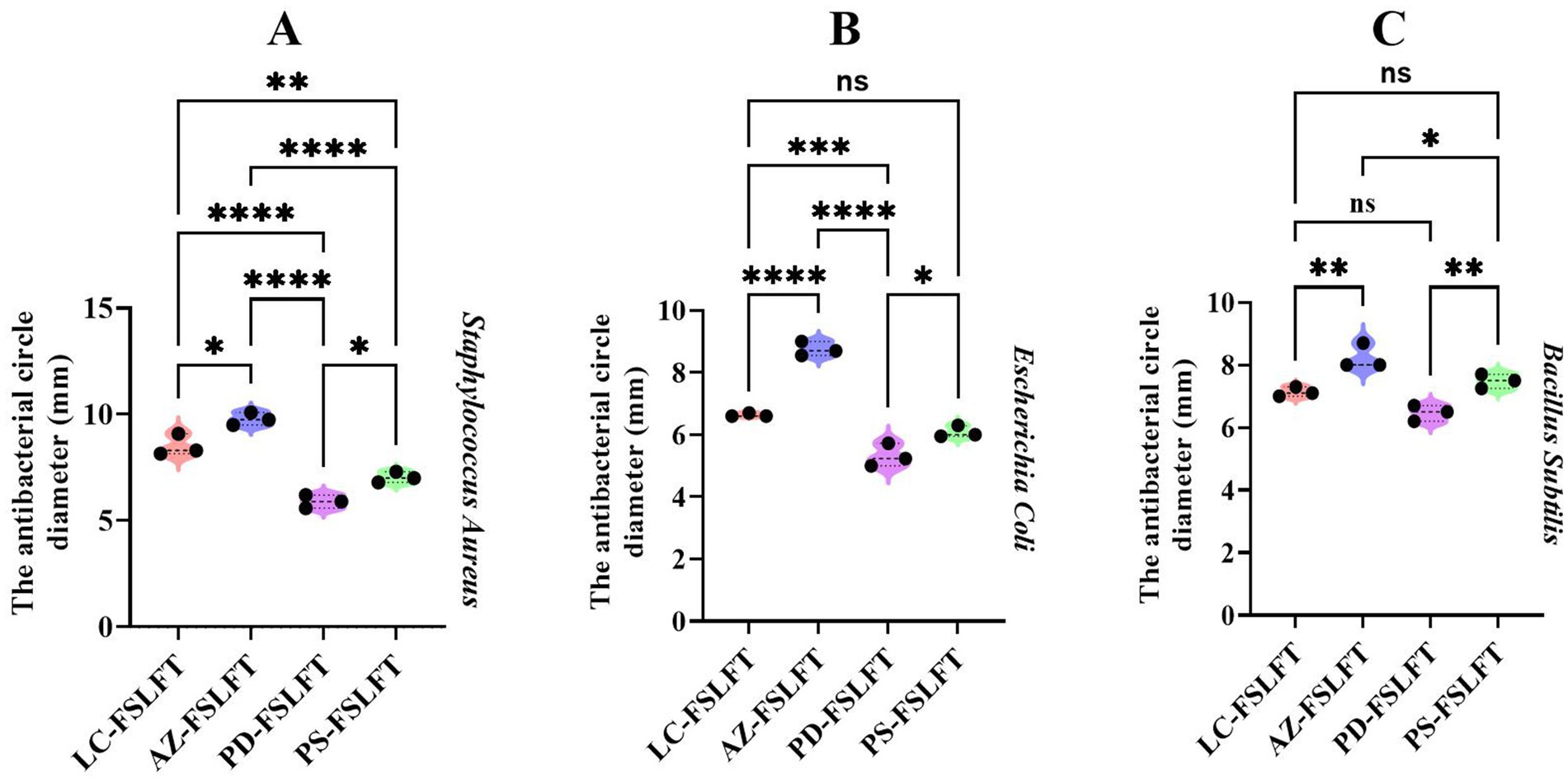
Figure 7. The antibacterial function of FSLFT in the four counties. (A) The antibacterial circle diameter of Staphylococcus aureus. (B) The antibacterial circle diameter of Escherichia coli. (C) the antibacterial circle diameter of Bacillus subtilis.
4 Discussion
Fermentation is the core link in black tea processing and plays a decisive role in the brightness of the black tea color and the formation of a unique taste. In this study, we realized that the quality of fermentation was not determined by a single factor, but was affected by multiple key parameters, such as fermentation temperature, humidity, and time, which is consistent with Rahman et al. (2020) reported. Wang et al. demonstrated that the volatile components in tea samples fermented for 3 h were the most abundant, the integrated sensor response to sweet and umami tastes was strong, and the sensory quality was the best (Wang et al., 2022). Control of the degree of fermentation should focus on changes in leaf color and aroma, and the fermentation time should be adjusted according to the actual situation (Wang et al., 2022). Zhu et al. found that low-temperature fermentation was conducive to maintaining the orange appearance and bright red liquor of Yunnan Congou black tea (YCBT), and high-temperature fermentation was conducive to increasing the bright appearance and orange red liquor color of YCBT. 3.5 ~ 4.5 h was the appropriate fermentation time to obtain a YCBT appearance and bright red soup color (Zhu et al., 2022), which was consistent with the fermentation time of FSLFT in this experiment. The results showed that fermentation time, fermentation temperature, and fermentation humidity had obvious effects on forsythin production in FSLFT. An FSLFT with a higher phillygenin content was obtained only under the appropriate process conditions. Orthogonal test analysis is a reliable test method that is widely used to optimize the process or determine the formula. Li et al. used orthogonal tests to adjust the fermentation conditions and discovered that moisture had the greatest impact (Li Y. et al., 2022). Ning et al. explored the influence of fresh leaf tenderness and withering mode on the quality of dandelion black tea through a single factor experiment and optimized its processing parameters using exogenous enzyme technology. Based on a single-factor experiment, orthogonal analysis was used to optimize the extraction process of the FSLFT (Ning et al., 2020). Based on a single-factor experiment, the orthogonal analysis method was used to optimize the extraction process of the FSLFT. The results showed that FSLFT had the highest phillygenin content when the fermentation time was 2 h, fermentation humidity was 80%, and fermentation temperature was 35°C. In addition, this study did not conduct a comprehensive sensory evaluation comparison of the FSLFT in the four regions. To obtain high-quality and optimal varieties of FSLFT, further exploration of the impact of different resources on the quality of the FSLFT is needed. The main component of F. suspensa leaves, phillygenin, has good application value; however, its content in F. suspensa leaves is extremely low, and its direct utilization value is low. Research has found that Biotransformation can convert phillyrin into phillygenin, which is related to an increase enzyme activity (Liu X. et al., 2022). This study found that the phillygenin content in FSLFT was significantly higher than that in F. suspensa leaves (Table 2). Correspondingly, the cellulase activity in FSLFT was significantly higher than that in F. suspensa leaves (Figure 2C), which is consistent with the results of Lu et al. (2024). This may be related to the promotion of cellulase secretion by relevant fungi or bacteria during the fermentation process (Unban et al., 2020a,b). Cellulase can hydrolyze cellulose in tea leaves, break down cell walls, and form more soluble sugars, which is beneficial for the dissolution of active ingredients, such as tea polyphenols, amino acids, caffeine, and soluble sugars that are enclosed within. According to previous reports, β-glucosidase can improve the quality of black tea by hydrolyzing glycoside compounds (Yan Z. et al., 2021; Supriyadi et al., 2021).
As an efficient and accurate mathematical tool, principal component analysis has significant advantages in comprehensive evaluation. It aims to select a few major and representative indicators from among many indicators for a comprehensive evaluation. This method not only effectively reduces the complexity and workload of multi-index comprehensive evaluation but also highlights the key role of the main indicators in the overall evaluation. In recent years, principal component analysis has been widely used in the comprehensive evaluation of tea and other scented teas. Widatall et al. found PCA could effectively differentiate between different grades of Pu-Erh tea, with a more pronounced distinction between high-grade and low- grade Pu-Erh tea (Widatalla et al., 2022). The grade of tea depends largely on the quality of the tea, and aroma are particularly important factors (Zhai et al., 2022). The aroma components of black tea are composed of volatile compounds from fresh leaves and precursor substances produced during processing (Chen et al., 2022), including alcohols, esters and hydrocarbon (Fangling et al., 2023). Under the PCA and PLS-DA modes, black tea (FBT) can be divided into different classes (Wu et al., 2023). In this study, the volatile aroma components of FSLFT were determined and analyzed using gas chromatography–mass spectrometry. A total of 87 volatile aroma components were identified, including alcohols, terpenes, alkanes, aldehydes, aldehydes, ketones, esters, acids, furans, and other oxides. There were 13 types of volatile aroma components (Supplementary Table S4) in FSLFT from the four counties, and the main components included alcohols, esters, aldehydes, ketones, olefins, acids, terpenes, alkanes, and so on. AZ-FSLFT had the highest score in the principal component analysis.
Previous studies have shown that fermented tea has antioxidant activity. Wang et al. found the antioxidant capacity of black tea fermentation groups was higher than that of the unfermented group (Wang et al., 2022a). Li J. et al. (2022) discovered a close relationship between the antioxidant activity of F. suspensa leaves and its forsythoside A, phillyrin, and phillygenol. According to Guo et al., phillygenin has beneficial effects on AFB1-induced oxidative damage, inflammatory response, apoptosis, and immunotoxicity by inhibiting NF-κB and activating the Nrf2 signaling pathway in chickens (Guo et al., 2022b). AZ- FSLFT demonstrated a significant antioxidant capacity in this study, potentially due to its higher content of bioactive components such as forsythin (Figure 2A), soluble sugar (Figure 2C), and total flavonoids (Figure 2E). However, the DPPH free radical scavenging power, ABTS scavenging power, and FRAP reducing power of LC-, PL-, and PS-fermented tea did not significantly differ, and further exploration is necessary to understand the specific reasons behind this. In recent years, the antibacterial activity of fermented tea has been a popular topic of research for many scholars. Talawa et al. found that during the fermentation period, the pH of tea decreased from approximately 5 to 2, and the OD600 of tea soup significantly increased from approximately 0 to 1.5. Black tea broth had the greatest inhibitory activity; Vibrio parahaemolyticus was the most sensitive to fermented tea, whereas pathogenic bacteria in the human body seemed to be less sensitive. These results may stem from the gradual production of key organic acids, such as succinic acid and gluconic acid, during tea fermentation, demonstrating their primary role in inhibiting microbial growth. Zhang et al. found that phillyrin has an antibacterial effect on Escherichia coli, Bacillus subtilis, Staphylococcus aureus, and Salmonella (Zhang et al., 2022).
This study found that the FSLFT exhibited an inhibitory effect on Staphylococcus aureus, Bacillus subtilis, and Escherichia coli. This effect was attributed to the presence of a variety of functional active ingredients, including total flavonoids, polyphenols, and phillygenin. For example, phillygenin inhibits Helicobacter pylori by preventing biofilm formation and inducing ATP leakage (Zhu et al., 2022). There were significant differences in the content of bioactive components in Forsythia suspensa leaves from different regions, which directly led to their varying antibacterial effects. The antibacterial activity test results confirmed the presence of multiple bioactive components and volatile substances in the AZ-FSLFT, which significantly enhanced its antibacterial effect.
5 Conclusion
Our research revealed that Fermentation causes an increase in cellulase activity in tea, promoting the hydrolysis of phillyrin into phillygenin. Additionally, FSLFT contained a number of aroma components and functional active ingredients that worked as antioxidants and antibacterials. The AZ-FSLFT had the best quality and functional activity, which made sense given its active ingredient content.
Data availability statement
The datasets presented in this study can be contained within the article and Supplementary material (doi: 10.6084/m9.figshare.26386279).
Author contributions
LL: Formal analysis, Methodology, Writing – original draft, Writing – review & editing. YZ: Conceptualization, Investigation, Resources, Software, Writing – original draft. SZ: Conceptualization, Data curation, Methodology, Writing – original draft. XZ: Data curation, Methodology, Writing – original draft. GD: Formal analysis, Funding acquisition, Project administration, Supervision, Writing – review & editing.
Funding
The author(s) declare that financial support was received for the research, authorship, and/or publication of this article. The research was funded by the Doctoral Research Initiation Fund of Changzhi Medical College, grant no. 2024BS08.
Acknowledgments
The authors are thankful to the Institute of Economic Crop Research at the University of Shanxi Agriculture and the Garden Pharmacy Tea Practice Center at the Shanxi Agricultural University for their support.
Conflict of interest
The authors declare that the research was conducted in the absence of any commercial or financial relationships that could be construed as a potential conflict of interest.
Publisher’s note
All claims expressed in this article are solely those of the authors and do not necessarily represent those of their affiliated organizations, or those of the publisher, the editors and the reviewers. Any product that may be evaluated in this article, or claim that may be made by its manufacturer, is not guaranteed or endorsed by the publisher.
Supplementary material
The Supplementary material for this article can be found online at: https://www.frontiersin.org/articles/10.3389/fsufs.2024.1482782/full#supplementary-material
References
Chen, Q., Zhu, Y., Liu, Y., Liu, Y., Dong, C., Lin, Z., et al. (2022). Black tea aroma formation during the fermentation period. Food Chem. 374:131640:131640. doi: 10.1016/j.foodchem.2021.131640
Fangling, Z., Shiya, G., Lei, Z., Yingqi, C., Xiaoyi, Z., Qing, J., et al. (2023). Characteristic aroma and molecular sensory analysis of black teas from different regions by gas chromatography-mass spectrometry and gas chromatography-Olfactometry. Shipin Kexue 44, 262–268. doi: 10.7506/spkx1002-6630-20230110-073
Gui, L., Wang, S., Wang, J., Liao, W., Chen, Z., Pan, D., et al. (2024). Effects of forsythin extract in Forsythia leaves on intestinal microbiota and short-chain fatty acids in rats fed a high-fat diet. Food Sci. Human Wellness 13, 659–667. doi: 10.26599/FSHW.2022.9250055
Guo, J., Tang, J. K., Wang, B. F., Yan, W. R., Li, T., Guo, X. J., et al. (2022a). Phillygenin from Forsythia suspensa leaves exhibits analgesic potential and anti-inflammatory activity in carrageenan-induced paw edema in mice. J. Food Biochem. 46:e14460. doi: 10.1111/jfbc.14460
Guo, J., Yan, W.-R., Tang, J.-K., Jin, X., Xue, H.-H., Wang, T., et al. (2022b). Dietary phillygenin supplementation ameliorates aflatoxin B1-induced oxidative stress, inflammation, and apoptosis in chicken liver. Ecotoxicol. Environ. Saf. 236:113481. doi: 10.1016/j.ecoenv.2022.113481
Hao, M., Lai, X., Li, Q., Cao, J., Sun, L., Chen, R., et al. (2024). Widely targeted metabolomic analysis reveals metabolite changes induced by incorporating black tea fermentation techniques in oolong tea processing for quality improvement. Food Chem. 459:140433. doi: 10.1016/j.foodchem.2024.140433
He, C., Zhou, J., Li, Y., Ntezimana, B., Zhu, J., Wang, X., et al. (2023). The aroma characteristics of oolong tea are jointly determined by processing mode and tea cultivars. Food Chem. 18:100730. doi: 10.1016/j.fochx.2023.100730
Li, Y., Hao, J., Zhou, J., He, C., Yu, Z., Chen, S., et al. (2022). Pile-fermentation of dark tea: conditions optimization and quality formation mechanism. LWT 166:113753. doi: 10.1016/j.lwt.2022.113753
Li, J., Qin, Q., Zha, S.-H., Zhao, Q.-S., Li, H., Liu, L.-P., et al. (2022). Green extraction of Forsythoside a, Phillyrin and Phillygenol from Forsythia suspensa leaves using a β-Cyclodextrin-assisted method. Molecules 27:7055. doi: 10.3390/molecules27207055
Liu, X., Li, F., and Zhang, L. (2022). Simultaneous detection of Phillyrin and its three metabolites in rat bile, excrement and urine via Hplc-Esi-Ms/Ms quantitative method. Curr. Pharm. Anal. 18, 88–100. doi: 10.2174/1573412917666210118115735
Liu, L., Shi, J., Yuan, Y., and Yue, T. (2022). Changes in the metabolite composition and enzyme activity of fermented tea during processing. Food Res. Int. 158:111428. doi: 10.1016/j.foodres.2022.111428
Liu, Y., Wang, C., Chen, Z., Yuan, H., Lei, R., Li, X., et al. (2024). Distribution of active ingredients and quality control of Forsythia suspensa with Ap-Maldi mass spectrometry imaging. J. Mass Spectrom. 59:e5073. doi: 10.1002/jms.5073
Liu, Y., Zhao, J., Guo, Y., Wang, M., Li, X., and Zhang, B. (2022). Mutagenic and teratogenic toxicity evaluation of Forsythia suspensa leaves aqueous extract. Drug Chem. Toxicol. 45, 1825–1832. doi: 10.1080/01480545.2021.1883645
Liu, S., Zhao, L., Li, M., Zhu, Y., Liang, D., Ma, Y., et al. (2024). Probiotic Bacillus as fermentation agents: status, potential insights, and future perspectives. Food Chem. 22:101465. doi: 10.1016/j.fochx.2024.101465
Lu, L., Liu, J., Zhang, W., Cheng, X., Zhang, B., Yang, Y., et al. (2024). Key factors of quality formation in Wuyi black tea during processing timing. Food Secur. 13:1373. doi: 10.3390/foods13091373
Nhu-Trang, T.-T., Nguyen, Q.-D., Cong-Hau, N., Anh-Dao, L.-T., and Behra, P. (2023). Characteristics and relationships between total polyphenol and flavonoid contents, antioxidant capacities, and the content of caffeine, gallic acid, and major catechins in wild/ancient and cultivated teas in Vietnam. Molecules 28:3470. doi: 10.3390/molecules28083470
Ning, Y., Wu, Z., Li, Z., Meng, R., Xue, Z., Wang, X., et al. (2020). Optimization of fermentation process enhancing quality of dandelion black tea on the functional components, activity and sensory quality. Open Access Libr. J. 7, 1–11. doi: 10.4236/oalib.1106323
Nishibe, S., Mitsui-Saitoh, K., Sakai, J., and Fujikawa, T. (2021). The biological effects of Forsythia leaves containing the cyclic amp phosphodiesterase 4 inhibitor phillyrin. Molecules 26:2362. doi: 10.3390/molecules26082362
Oanh, D. T. Y., Hien, L. T. M., Duyen, N. K., Hieu, N. H., and Dao, D. T. A. (2023). Effect of enzyme – assisted extraction on total polyphenol content and antioxidant capacity from fresh tea leaves (Camellia sinensis). Vietnam J. Chem. 61, 551–562. doi: 10.1002/vjch.202300109
Patyra, A., Kołtun-Jasion, M., Jakubiak, O., and Kiss, A. K. (2022). Extraction techniques and analytical methods for isolation and characterization of Lignans. Plan. Theory 11:2323. doi: 10.3390/plants11172323
Peng, H., Lu, P., Liu, Y., Liu, C., Gao, Y., Nwafor, E., et al. (2020). Research progress on chemical composition, pharmacological effects of Forsythia suspensa (Thunb.) Vahl and predictive analysis on Q-marker. Tmr Mod. Herb. Med 3, 86–112. doi: 10.53388/TMRmhm202003069
Qu, Q., Li, Y., Dong, Q., Li, S., Du, H., Wang, Z., et al. (2021). Comparative evaluation of forsythiae fructus from different harvest seasons and regions by Hplc/Nir analysis and anti-inflammatory and antioxidant assays. Front. Pharmacol. 12:737576. doi: 10.3389/fphar.2021.737576
Rahman, M., Hossain, M., Das, R., and Ahmad, I. (2020). Changes in phytochemicals and determination of optimum fermentation time during black tea manufacturing. J. Sci. Res. 12, 657–664. doi: 10.3329/jsr.v12i4.45452
Saldana-Mendoza, S. A., Palacios-Ponce, A. S., Ruiz, H. A., Ascacio-Valdés, J. A., and Aguilar, C. N. (2024). Revalorization of green tea waste through the production of cellulases by solid-state fermentation using a aspergillus Niger 28A. Biomass Convers. Biorefinery 14, 16711–16724. doi: 10.1007/s13399-023-03919-1
Setiawan, V., Phangestu, S., Soetikno, A. G., Arianti, A., and Kohar, I. (2021). Rapid screening analysis of antioxidant activities in green tea products using Dpph and Frap. Pharm. J. Indones. 7, 9–14. doi: 10.21776/ub.pji.2021.007.01.2
Supriyadi, S., Nareswari, A. R., Fitriani, A., and Gunadi, R. (2021). Enhancement of black tea aroma by adding the β-glucosidase enzyme during fermentation on black tea processing. Int. J. Food Sci. 2021:5542109, 1–9. doi: 10.1155/2021/5542109
Tian, Z., Tan, Z., Li, Y., and Yang, Z. (2022). Rapid monitoring of flavonoid content in sweet tea (Lithocarpus litseifolius (Hance) Chun) leaves using Nir spectroscopy. Plant Methods 18:44. doi: 10.1186/s13007-022-00878-y
Unban, K., Khatthongngam, N., Pattananandecha, T., Saenjum, C., Shetty, K., and Khanongnuch, C. (2020a). Microbial community dynamics during the non-filamentous fungi growth-based fermentation process of Miang, a traditional fermented tea of North Thailand and their product characterizations. Front. Microbiol. 11:1515. doi: 10.3389/fmicb.2020.01515
Unban, K., Kodchasee, P., Shetty, K., and Khanongnuch, C. (2020b). Tannin-tolerant and extracellular tannase producing Bacillus isolated from traditional fermented tea leaves and their probiotic functional properties. Food Secur. 9:490. doi: 10.3390/foods9040490
Wal, P. (2024). Phytochemicals and their potential mechanisms against insulin resistance. Curr. Diabetes Rev. 20:113:125. doi: 10.2174/0115733998262924231020083353
Wang, X.-F., Chen, L., Gui, R., Ning, E.-J., Wang, W., Wang, X.-B., et al. (2023b). Study on the response surface optimization of extraction technology of total triterpenes from Forsythia suspensa leaves and its anti-tumor activity. Hubei Agric. Sci. 62:113. doi: 10.14088/j.cnki.issn0439-8114.2023.09.021
Wang, H., Shen, S., Wang, J., Jiang, Y., Li, J., Yang, Y., et al. (2022). Novel insight into the effect of fermentation time on quality of Yunnan congou black tea. LWT 155:112939. doi: 10.1016/j.lwt.2021.112939
Wang, X., Wang, P., Du, H., Li, N., Jing, T., Zhang, R., et al. (2022b). Prediction of the active components and mechanism of Forsythia suspensa leaf against respiratory syncytial virus based on network pharmacology. Evid. Based Complement. Alternat. Med. 2022, 1–14. doi: 10.1155/2022/5643345
Wang, X., Wang, D., Wang, H., Jiao, S., Wu, J., Hou, Y., et al. (2022a). Chemical profile and antioxidant capacity of Kombucha tea by the pure cultured Kombucha. LWT 168:113931. doi: 10.1016/j.lwt.2022.113931
Wang, C., Wu, R., Zhang, S., Gong, L., Fu, K., Yao, C., et al. (2023). A comprehensive review on pharmacological, toxicity, and pharmacokinetic properties of phillygenin: current landscape and future perspectives. Biomed. Pharmacother. 166:115410. doi: 10.1016/j.biopha.2023.115410
Widatalla, H. A., Yassin, L. F., Alrasheid, A. A., Rahman Ahmed, S. A., Widdatallah, M. O., Eltilib, S. H., et al. (2022). Green synthesis of silver nanoparticles using green tea leaf extract, characterization and evaluation of antimicrobial activity. Nanoscale Adv. 4, 911–915. doi: 10.1039/d1na00509j
Wu, L.-G., Wang, A., Shen, R., and Qu, L. (2021). Effect of heating under pressure treatment on the antioxidant of quinoa. Int. J. Food Eng. 17, 795–804. doi: 10.1515/ijfe-2020-0282
Wu, Q., Zhou, Z., Zhang, Y., Huang, H., Ou, X., and Sun, Y. (2023). Identification of key components responsible for the aromatic quality of Jinmudan black tea by means of molecular sensory science. Food Secur. 12:1794. doi: 10.3390/foods12091794
Xiao, X., Zhang, Y., Sun, K., Liu, S., Li, Q., Zhang, Y., et al. (2024). Enzymatic and ultrasound assisted β-cyclodextrin extraction of active ingredients from Forsythia suspensa and their antioxidant and anti-inflammatory activities. Ultrason. Sonochem. 108:106944. doi: 10.1016/j.ultsonch.2024.106944
Yan, K., Abbas, M., Meng, L., Cai, H., Peng, Z., Li, Q., et al. (2021). Analysis of the fungal diversity and community structure in Sichuan dark tea during pile-fermentation. Front. Microbiol. 12:706714. doi: 10.3389/fmicb.2021.706714
Yan, Z., Cao, X., Yang, X., Yang, S., Xu, L., Jiang, X., et al. (2021). A novel β-glucosidase from Chryseobacterium scophthalmum 1433 for efficient Rubusoside production from Stevioside. Front. Microbiol. 12:744914. doi: 10.3389/fmicb.2021.744914
Yang, H.-X., Liu, Q.-P., Zhou, Y.-X., Chen, Y.-Y., An, P., Xing, Y.-Z., et al. (2022). Forsythiasides: a review of the pharmacological effects. Front. Cardiovasc. Med. 2022:971491. doi: 10.3389/fcvm.2022.971491
Zhai, X., Zhang, L., Granvogl, M., Ho, C. T., and Wan, X. (2022). Flavor of tea (Camellia sinensis): a review on odorants and analytical techniques. Compr. Rev. Food Sci. Food Saf. 21, 3867–3909. doi: 10.1111/1541-4337.12999
Zhang, J., Gao, M., Luo, J., Guo, Y., Bao, Y., and Yang, T. (2022). Antibacterial activity and mechanism of phillyrin against selected four foodborne pathogens. Food Sci. Technol. 42:e32922. doi: 10.1590/fst.32922
Zhou, C., Lu, M., Cheng, J., Rohani, E. R., Hamezah, H. S., Han, R., et al. (2022). Review on the pharmacological properties of phillyrin. Molecules 27:3670. doi: 10.3390/molecules27123670
Zhou, H., Wang, Z., Ma, H., Wang, S., Xie, W., Chen, Y., et al. (2022). Characterisation and antioxidant activity of polysaccharide iron (iii) complex in Qingzhuan dark tea. Food Sci. Technol. 42:119421. doi: 10.1590/fst.119421
Keywords: Forsythia suspensa leaf fermented tea, fermentation process, aroma components, antibacterial function, antioxidant activity
Citation: Li L, Zhao Y, Zhang S, Zhang X and Duan G (2024) Comparison of Forsythia suspensa leaf fermented tea in the four different regions of Shanxi Province: quality, functional ingredients, and bioactivity. Front. Sustain. Food Syst. 8:1482782. doi: 10.3389/fsufs.2024.1482782
Edited by:
Sui Kiat Chang, Universiti Tunku Abdul Rahman, MalaysiaReviewed by:
Miguel Ángel De León Zapata, Autonomous University of Coahuila, MexicoMohamad Shazeli Che Zain, University of Science Malaysia, Malaysia
Copyright © 2024 Li, Zhao, Zhang, Zhang and Duan. This is an open-access article distributed under the terms of the Creative Commons Attribution License (CC BY). The use, distribution or reproduction in other forums is permitted, provided the original author(s) and the copyright owner(s) are credited and that the original publication in this journal is cited, in accordance with accepted academic practice. No use, distribution or reproduction is permitted which does not comply with these terms.
*Correspondence: Guofeng Duan, NDQ4MDUyMzM5QHFxLmNvbQ==