- 1Key Laboratory of Agricultural Soil and Water Engineering in Arid and Semiarid Areas at Ministry of Education, Northwest A&F University, Yangling, China
- 2College of Water Resources and Architectural Engineering, Northwest A&F University, Yangling, China
- 3Institute of Soil Fertilizer and Agricultural Water Saving, Xinjiang Academy of Agricultural Sciences, Urumqi, China
- 4School of Environmental Sciences, University of Guelph, Guelph, ON, Canada
The issue of soil salinization poses a significant barrier to sustainable agricultural development, particularly in arid and semi-arid regions. Finding methods to enhance the quality of salinized soils while conserving water resources has become a pressing challenge. In arid and semi-arid environments, conserving water resources while maintaining soil health is a critical challenge. This study, conducted from 2021 to 2023, aimed to explore the combined effects of irrigation and biochar application on soil physicochemical properties, such as bulk density, porosity, and pH, as well as on Weighted Plane Soil Water Storage (WPSWS), soil temperature, and soil water evaporation. The experimental design included four irrigation levels, based on actual crop evapotranspiration (ETc): I1 (0.6 ETc), I2 (0.8 ETc), I3 (1.0 ETc), and I4 (1.2 ETc), coupled with four amounts of biochar application (AOBA) of 0, 10, 20, and 30 t ha−1, designated as C0, C10, C20, and C30, respectively. Through binary quadratic regression analysis, we sought to identify the optimal combination of irrigation amount and AOBA for enhancing soil quality. The results revealed that as AOBA increased from 10 to 30 t ha−1, soil bulk density decreased by 1.31–8.58% and soil pH by 0.23–1.31%. However, higher levels of AOBA adversely affected WPSWS, with the C10 treatment showing the maximum improvement in WPSWS, registering an average increase of 6.77, 7.49, and 11.16% compared to the C0, C20, and C30 treatments, respectively. We observed that an increase in irrigation amount significantly elevated accumulated soil evaporation (ASE) and WPSWS but led to a reduction in accumulated soil temperature (AST). The most notable soil quality improvements were recorded when irrigation levels were between 340 and 380 mm and AOBA ranged from 10 to 25 t ha−1. This study provides insights into the effective combination of biochar application and irrigation for optimizing soil resilience, thereby offering a sustainable approach to soil management in water-limited environments.
1 Introduction
Sugar beet, a cornerstone of the global sugar economy, plays a pivotal role in meeting approximately 30% of the world’s annual sugar demand (Ahmad et al., 2015). Its significance extends beyond traditional uses, contributing substantially to the biofuel sector as a primary source for ethanol and glycerol production (Misra et al., 2023; Li et al., 2022a). Favored for its resilience to cold, simplicity in cultivation, and shorter production cycles, sugar beet has emerged as a globally dominant sugar crop (Yu et al., 2020). Despite these advantages, challenges such as soil salinization and water scarcity are increasingly undermining its yield and sugar content, necessitating urgent solutions to secure stable production (Xiao et al., 2023a; Li et al., 2023a; Sun et al., 2023).
In this context, biochar emerges as a transformative solution. Its application has been shown to enhance soil quality and sustain crop productivity, thanks to its high specific surface area influencing soil density and porosity (Asai et al., 2009; Xiao et al., 2016). Biochar’s ability to modulate soil temperature and minimize inefficient water evaporation is increasingly recognized, although the balance between its benefits and potential reduction in soil water storage capacity remains a topic of active research (Liang et al., 2021; Xu et al., 2016; Wang et al., 2022a). Liu et al. (2021) showed that the application of biochar could offset the crop yield reduction caused by deficit irrigation and thus improve water use efficiency. However, in a faba bean-ryegrass intercropping system, it was found that although biochar application improved soil water storage, total biomass decreased under deficit irrigation (Liu et al., 2022a). Beyond physical properties, biochar’s rich active functional groups facilitate nutrient conversion and absorption, enhancing soil ion exchange rates and reducing nutrient loss (Qi et al., 2024; Li et al., 2018). Its typically weakly alkaline nature, rich in mineral salts and organic carbon, positions biochar as a potent means for soil organic matter enrichment and carbon sequestration (Butt et al., 2018). Empirical evidence supports biochar’s role in bolstering growth and yield in various crops, even in soils distressed by heavy metals (Almaroai and Eissa, 2020; Aguirre et al., 2021; Liu et al., 2014). However, the optimal application rate of biochar, particularly under varying degrees of soil salinization and across different crop types, remains an area ripe for exploration.
Concurrently, the strategic allocation of water resources is imperative, especially in arid and semi-arid regions grappling with water scarcity (Xu et al., 2019; Kang et al., 2017). Developing efficient irrigation systems is not just a matter of enhancing crop growth and yield; it is critical for maximizing water use efficiency and conserving this vital resource (Zhang et al., 2022a). Some studies have shown that a low irrigation volume (1200–1800 m3 ha−1) leads to poor desalination and is prone to secondary salinization disasters, while a high irrigation volume (4,200 m3 ha−1) reduces water use efficiency (Li et al., 2023b). The balance between insufficient and excessive irrigation is delicate, as both extremes can detrimentally impact crop health (Zhang et al., 2016). Emerging research underscores the complexity of this balance, highlighting the need for optimized irrigation strategies tailored to specific crop requirements and environmental conditions (Guo et al., 2021b; Himanshu et al., 2021; Liu et al., 2024). Sugar beet as a high-water demand crop, it is necessary to develop a rational irrigation strategy. Proper irrigation strategies not only increased the rate of nutrient uptake by sugar beet, but also effectively reduced soil salinity (Yan et al., 2021). This study gains urgency and relevance in the context of Xinjiang, China’s primary sugar beet cultivation region. Xinjiang’s unique climatic and light conditions are conducive to sugar accumulation, yet it faces the daunting challenge of extensive soil salinization, covering an area of 8.5 × 104 km2, posing a significant threat to sugar beet productivity (Wang et al., 2018; Feng et al., 2017). Understanding the interplay between irrigation and biochar application in such environments is crucial. While previous studies have independently examined the effects of irrigation and biochar on soil properties, the synergistic impact of these two factors on soil water dynamics, temperature, and physicochemical properties, especially in salinized soils, is less understood.
Therefore, this study aims to fill these critical gaps by: (1) investigating the combined effects of various irrigation levels and biochar application rates on soil water storage, evaporation, temperature, and other key soil properties throughout the growth stages of sugar beet during 2021, 2022, and 2023; and (2) identifying the optimal combination of irrigation and biochar application to enhance soil quality. This research not only expected to advance the understanding of the theory of salinized soil improvement and water conservation and salt control, but also has important implications for sustainable agricultural development.
2 Materials and methods
2.1 Experimental site description
This study was conducted over three consecutive sugar beet growing seasons (2021–2023) in Beiquan Town, Shihezi, Xinjiang Uygur Autonomous Region, China (85°97′05″E; 44°47′83″N). This location, at the southern fringe of the Junggar Basin and on the western bank of the Manas River, is characterized by a perennially dry climate with potent evaporation, abundant solar and thermal resources, and a typical temperate continental climate. Notably, the area receives an average annual precipitation of 142 mm, predominantly between June and August, and experiences an average annual temperature of 6.2°C with a frost-free period extending from 168 to 171 days. The active accumulated temperatures (≥0°C and ≥ 10°C) range from 4,023–4,118°C and 3,570–3,729°C, respectively. The region predominantly supports irrigated agriculture, with sugar beet and cotton as the principal economic crops. Soil type was dominated by sandy loam, with clay, silt, and sand accounting for 3.1, 69.1, and 27.8% of the 0–100 cm soil layer, respectively. The groundwater level consistently remains below 2 meters, underscoring the region’s semi-arid nature.
2.2 Experimental design
2.2.1 Experimental plot design
Biochar, derived from palm fruit branches and pyrolyzed under anaerobic conditions at 500–600°C, was sourced from Zhengzhou Yongbang New Energy Equipment Technology Co. Ltd., China. Its physicochemical properties were characterized by an initial electrical conductivity of 11.02 mS cm−1, organic carbon content of 472.2 g kg−1, pH 6.7, total nitrogen content of 2.30 g kg−1, specific surface area of 116.6 m2 g−1, and a bulk density of 0.5 g cm−3.
The experimental design incorporated 16 treatments across four irrigation gradients and four biochar application rates. Each treatment was replicated thrice, resulting in a total of 48 plots. The experimental plots were arranged in a randomized complete factorial block design, with each plot measuring 48 m2 (6 m × 8 m). A buffer zone of 50 cm was maintained between plots to prevent cross-contamination. Biochar application rates were set at 0, 10, 20, and 30 t ha−1. The application was executed once before sowing in 2021, the biochar was uniformly spread on the soil surface with specified amounts, thoroughly mixed with the soils using a wooden rake, and then ploughed down to 30 cm (Liang, 2021b; Wang et al., 2022a) depth using a rotary cultivator, and this regime was maintained throughout the three-year experiment.
Over the course of 3 years, the planting and harvesting of sugar beets were scheduled from April 19 to September 28 in 2021, April 15 to September 25 in 2022, and April 18 to September 23 in 2023. The chosen cultivar for these trials was “De tian 2” a variety commonly grown by local agriculturists. The prevalent planting methodology in the region involved a system termed “one plastic film, two drip pipes, and four rows.” The drip tape utilized in this study was an in-line system with drippers spaced at 30 cm intervals. Each dripper had a flow rate of 2.4 L h−1 and operated at a pressure of 0.1 MPa. In this arrangement, sugar beets were systematically sown to maintain uniform inter-row and intra-row spacing of 40 cm each (Figure 1a).
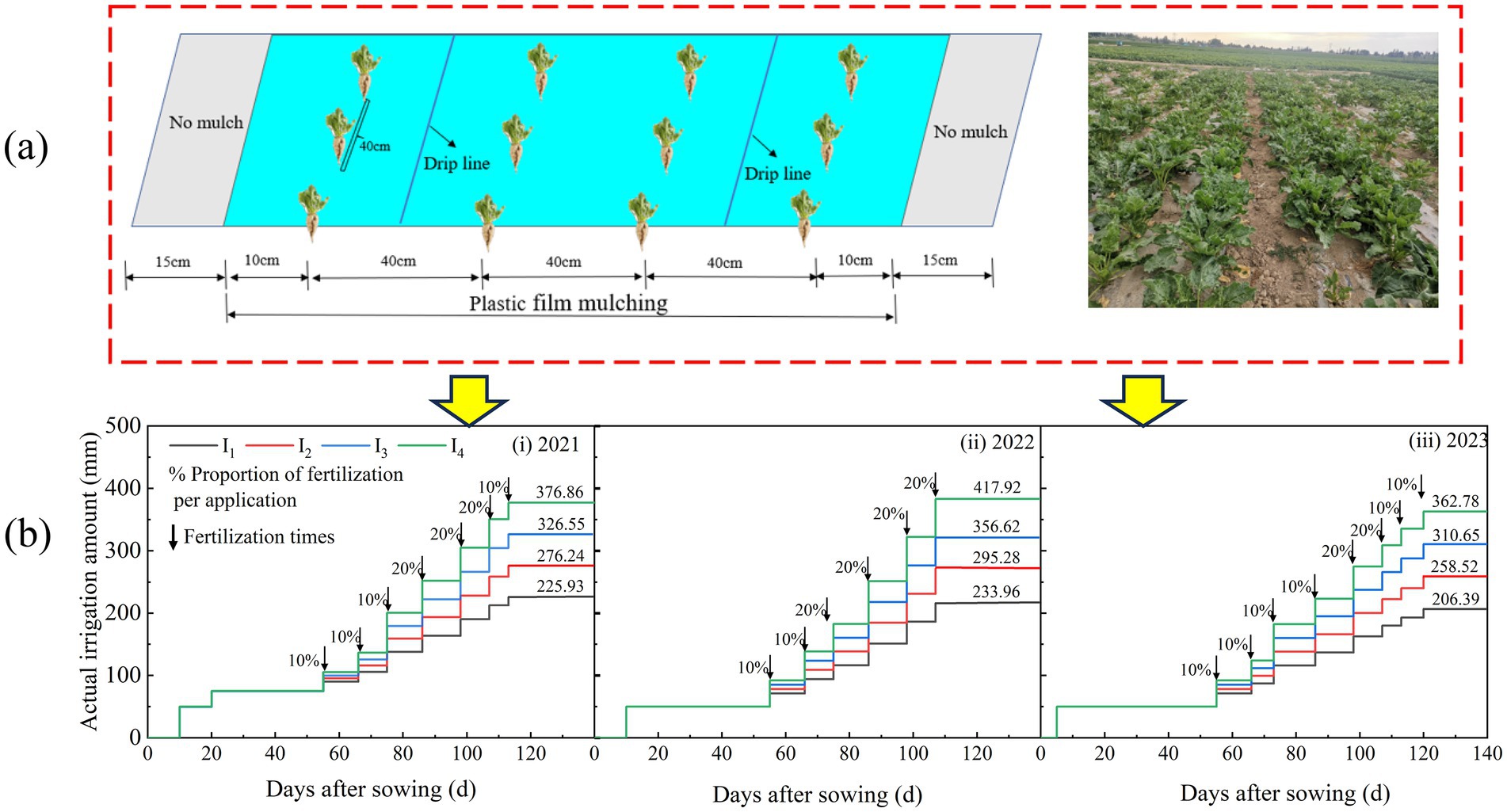
Figure 1. Schematic diagram (left) and experimental planting (right) of sugar-beet planting under plastic mulched drip irrigation (a), diagram of irrigation cycle and fertilization times (b). I1, I2, I3, and I4 represent irrigation quota at 60, 80, 100, and 120% ETc, respectively.
2.2.2 Irrigation schedule
Field meteorological data, including rainfall, wind speed, temperature, sunshine intensity, and humidity, were monitored using a portable automatic weather station (HOBO U30, United States). The total precipitation and average daily temperatures during the sugar beet growth periods for 2021, 2022, and 2023 were recorded.
Irrigation quotas were determined as fractions (0.6ETc, 0.8ETc, 1.0ETc, and 1.2ETc) of the actual daily crop evapotranspiration (ETc), as calculated using the formula by Allen et al. (1998), where . The crop coefficient (Kc) of sugar beet varied across different growth stages, while the daily reference crop evapotranspiration (ET0) was computed using the standard formula incorporating parameters like net radiation, soil heat flux density, humidity meter constant, daily average temperature, wind speed, and vapor pressure deficit Equation 1;
where Rn is net radiation amount (MJ⋅m−2⋅d−1); G is soil heat flux density (MJ⋅m−2⋅d−1); γ is the humidity meter constant (kPa⋅C−1); T is daily average temperature (°C); μ2 is the wind speed at 2 m above the ground (m⋅s−1); es and ea are saturated and actual water vapor pressure (kPa); Δ is the slope of the saturation vapor pressure curve at air temperature (kPa C−1).
Irrigation commenced 5 days post-sowing, initially at a volume of 50 mm according to the experience of local farmers, a practice termed “dry sowing and wet seedling.” Throughout 2021, a total of 10 irrigation events were executed, with respective quotas of 226, 276, 327, and 377 mm for the treatments I1, I2, I3, and I4. In the subsequent year, 2022, the number of irrigation events was reduced to eight due to the COVID-19 pandemic, setting the quotas at 214, 275, 336, and 398 mm for the same respective treatments. In 2023, the irrigation frequency was adjusted to nine events, with quotas of 207, 259, 311, and 363 mm allocated to treatments I1 through I4.
Fertilization, integrated with the irrigation process, mirrored the local agricultural practices in terms of quantities and types of fertilizers used. Specifically, nitrogen, phosphorus, and potassium were applied at rates of 450, 265, and 100 kg ha−1, respectively. This was achieved using urea (with a nitrogen content of at least 46%), diammonium phosphate (boasting a minimum of 46% P2O5), and potassium sulfate (containing no less than 52% K2O). A detailed depiction of the fertilization and irrigation protocol is presented in Figure 1(B).
2.3 Measurements and methods
2.3.1 Soil properties
For each treatment, during the harvest stages of sugar beet in the years 2021, 2022, and 2023, soil cores were extracted from a depth of 40 cm using a steel corer, which had a capacity of 100 cm3. These samples were then transported to the laboratory to assess soil bulk density (BD) and porosity ( ) (Danielson and Sutherland, 1986). Utilizing spiral soil drills to collect additional soil samples were collected from varying depths: 0–10 cm, 10–20 cm, 20–30 cm, and 30–40 cm. These samples were air-dried, ground, and then utilized to prepare a soil-water mixture in the ratio of 1:5. The pH levels of this mixture were determined using a pH meter (model PHS – 2F).
2.3.2 The weighted planar soil water storage (WPSWS)
Soil samples were systematically collected at various stages of sugar beet growth (Seedling stage, rapid growth stage, expansion stage, sugar accumulation stage and harvest stage) post-sowing - utilizing spiral soil drills. These samples were extracted from depths of 0, 10, 20, 40, 60, 80, and 100 cm. Soil water content was measured by drying soil samples at a temperature of 105°C until a constant weight was achieved. Sampling was conducted at three distinct points: the area devoid of film coverage (LNM), directly beneath the drip irrigation belt (LU), and at the central point of the drip irrigation belt (LM). The Weighted Planar Soil Water Storage (WPSWS) was calculated using the moisture content data obtained from these various locations, both along the rows and under the plastic mulch, to evaluate the vertical distribution of soil moisture storage. The formula for WPSWS (cm3 cm−3) is Equation 2:
where, LNM, LU and LM represent the areas without film coverage, the areas under the drip irrigation belt, and the middle of the drip irrigation belt, respectively. NM , U , and M denote the average soil water content (cm3 cm−3) in the areas without film coverage, under the drip irrigation belt, and in the middle of the drip irrigation belt, respectively.
2.3.3 Soil water evaporation
Throughout the years 2021, 2022, and 2023, the daily rate of soil water evaporation was closely observed during the period of rapid growth of the sugar beet. This measurement was conducted using a micro soil lysimeter, consisting of two PVC drum cylinders: an outer cylinder measuring 10 cm in length and 20 cm in height, and an inner cylinder with dimensions of 9 cm in length and 15 cm in height. The outer cylinder was embedded in the soil, while the inner cylinder, lined at the bottom with breathable gauze for aeration, was filled with soil. An electronic balance was employed for the precise measurement of daily soil evaporation, recorded at 8:00 PM each day. Following any incidence of rainfall or irrigation, the soil within the inner cylinder was replaced with fresh soil to maintain consistency in measurements.
2.3.4 Soil temperature
Daily soil temperatures at depths of 5, 10, 15, 20, and 25 cm were recorded using a right-angle geothermometer (Hong Kong thermal instruments, Wuqiang County, Hebei Province, China) from 8:00 AM to 8:00 PM (Tan et al., 2017).
2.4 Statistical analysis
Data were analyzed using a two-way analysis of variance (ANOVA) in SPSS18.0 to assess the impacts of biochar application and irrigation on soil properties. Statistical significance was determined at the 0.05 or 0.01 confidence levels. Graphical illustrations were created using Origin 9.0 software.
3 Results
3.1 Effects of biochar on soil bulk density, porosity, and pH
Reduction in Soil Bulk Density and Increase in Porosity: The application of biochar significantly decreased soil bulk density while simultaneously enhancing soil porosity (Figure 2). In the top 40 cm of soil, the C10, C20, and C30 treatment groups experienced a reduction in soil bulk density by 2.63–8.58%, 1.32–7.75%, and 1.31–5.26%, respectively. Correspondingly, soil porosity in these groups increased by 3.66–10.49%, 2.14–10.09%, and 1.41–6.57% compared to the control group (C0). It was observed that with the application of consistent biochar amounts, the soil bulk density tended to increase as the soil depth increased. For example, at the 0–10 cm depth, the decrease in soil bulk density was 1.41–3.24%, 6.67–8.57%, and 5.89–9.03% in comparison to the 10–20 cm, 20–30 cm, and 30–40 cm depths, respectively. Inversely, soil porosity showed a tendency to decrease with increasing soil depth. Specifically, soil porosity at the 0–10 cm depth increased by 1.59–3.08%, 7.71–10.68%, and 2.89–11.37% relative to the 10–20 cm, 20–30 cm, and 30–40 cm depths.
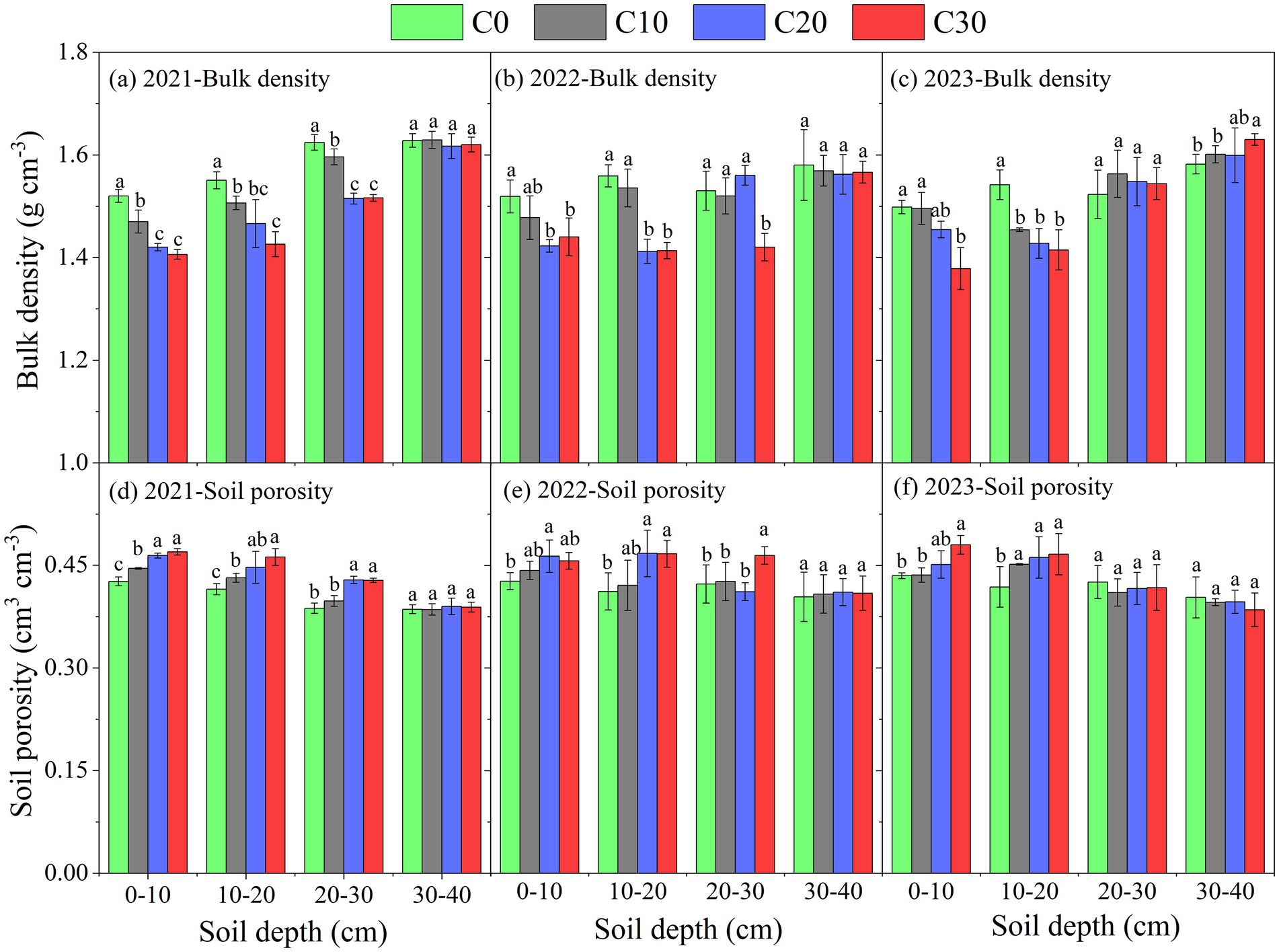
Figure 2. Effect of different biochar application rates on bulk density and porosity at the depth of 0–40 cm in 2021, 2022, and 2023 (pre-planting). C0, C10, C20, and C30 represent biochar application rates of 0, 10, 20, and 30 t ha−1, respectively. Error bars represent standard errors. Different letters above the bars indicate statistical differences among treatments at the significance level p < 0.05 with an LSD test.
Change in Soil pH: Supplementary Figure S1 presents a comparison of soil pH values within a depth of 0–40 cm, under varying irrigation levels and biochar application rates, using data from the year 2021 as an illustrative case. The introduction of biochar led to a significant decrease in soil pH at the 0–30 cm depth, while its impact was negligible on the 30–40 cm layer. Specifically, the soil pH in the C10, C20, and C30 treatment groups showed an average decrease of 0.23, 0.62, and 1.31% respectively, in comparison to the control group (C0). An inverse relationship was observed between soil pH and the amount of biochar applied (AOBA); higher biochar levels corresponded to lower pH values. Furthermore, when the biochar application rate was constant, an increase in irrigation quantity resulted in a further reduction in soil pH. The pH values recorded for the years 2022 and 2023 are displayed in Supplementary Figures S2,S3.
3.2 Influences biochar application and irrigation on WPSWS
Variation of WPSWS with Biochar and Irrigation: The 2021 data, depicted in Supplementary Figure S4, illustrates the trend of WPSWS under various levels of biochar application and irrigation. WPSWS initially showed an increase followed by a decrease as the sowing days progressed. Additionally, deeper soil layers exhibited a narrower fluctuation range in WPSWS. When considering the same irrigation level, an increase in biochar application initially raised the WPSWS but subsequently led to a reduction. The highest WPSWS was observed under the C10 treatment, whereas the C30 treatment registered the lowest. The WPSWS variations for the years 2022 and 2023 are presented in Supplementary Figures S5,S6. The trend was consistent with that of 2021, positively correlated with irrigation water, and greatest in the C10 treatment. Statistical Analysis of Influences on WPSWS: The study, spanning over 3 years, revealed that both the AOBA and the irrigation level significantly influenced WPSWS (Table 1). An analysis of variance indicated that irrigation predominantly affected WPSWS at depths of 0–10 cm (p < 0.01), 0–40 cm, and 0–100 cm (p < 0.05). Biochar had a notable impact on WPSWS at the 0–10 cm depth but showed no significant difference at the 0–100 cm depth range. Considering the interaction between irrigation and biochar (I × C), no significant differences were found in WPSWS across the 0–10, 0–40, and 0–100 cm depths.

Table 1. The variance significance level of WPSWS at 0–10 cm, 0–40 cm, and 0–100 cm depth ranges in 2021, 2022, and 2023, compared between four irrigation amounts (at the same biochar application rates), four biochar application rates and 16 biochar-irrigation treatments.
Cumulative WPSWS under Various Treatments: The cumulative WPSWS at a depth of 0–100 cm over the 3 years, under different irrigation and biochar levels, is showcased in Supplementary Figure S7. The I4C10 treatment consistently demonstrated a higher accumulated WPSWS compared to the other 15 treatments. Under identical biochar levels, the I4 treatment exhibited an average increase of 5.96, 14.33, and 19.86% relative to the I1, I2, and I3 treatments, respectively. When irrigation levels were constant, the maximum accumulated WPSWS was achieved under the C10 treatment, surpassing the C0, C20, and C30 treatments by 6.77, 7.49, and 11.16%, respectively.
3.3 Influences of biochar application and irrigation on soil temperature
Influence of Air Temperature on Soil Temperature: The soil temperature during the sugar beet growth period in 2021, 2022, and 2023 was significantly influenced by air temperature. The daily maximum air temperatures ranged from 14.0 to 40.1°C, 12.7 to 39.8°C, and 13.1 to 41.2°C, respectively, across these years. Similarly, the daily minimum air temperatures varied between −0.9 to 25.9°C, 0.7 to 24.7°C, and 1.2 to 23.6°C.
Soil Temperature Dynamics: In 2021, the peak soil temperature was observed around 60 days after sowing, as shown in Supplementary Figure S8. In the subsequent years, the highest soil temperatures were recorded on the 75th day in 2022 and the 60th day in 2023 (Supplementary Figures S9,S10). A consistent trend was noted where soil temperature decreased with increasing depth. The average daily maximum soil temperature at 5 cm depth showed a relative increase by 1.22–5.79%, 2.19–6.99%, 2.56–4.84%, and 0.79–6.28% compared to the temperatures at depths of 10, 15, 20, and 25 cm, respectively (Table 2). Biochar application effectively elevated soil temperatures under the same irrigation conditions. The highest and lowest soil temperatures in the C0 treatment increased by an average of 0.54–7.35% and decreased by −1.99–10.89%, respectively, when compared to the C10, C20, and C30 treatments. Moreover, increasing irrigation amounts resulted in lower soil temperatures within the same biochar treatment category.
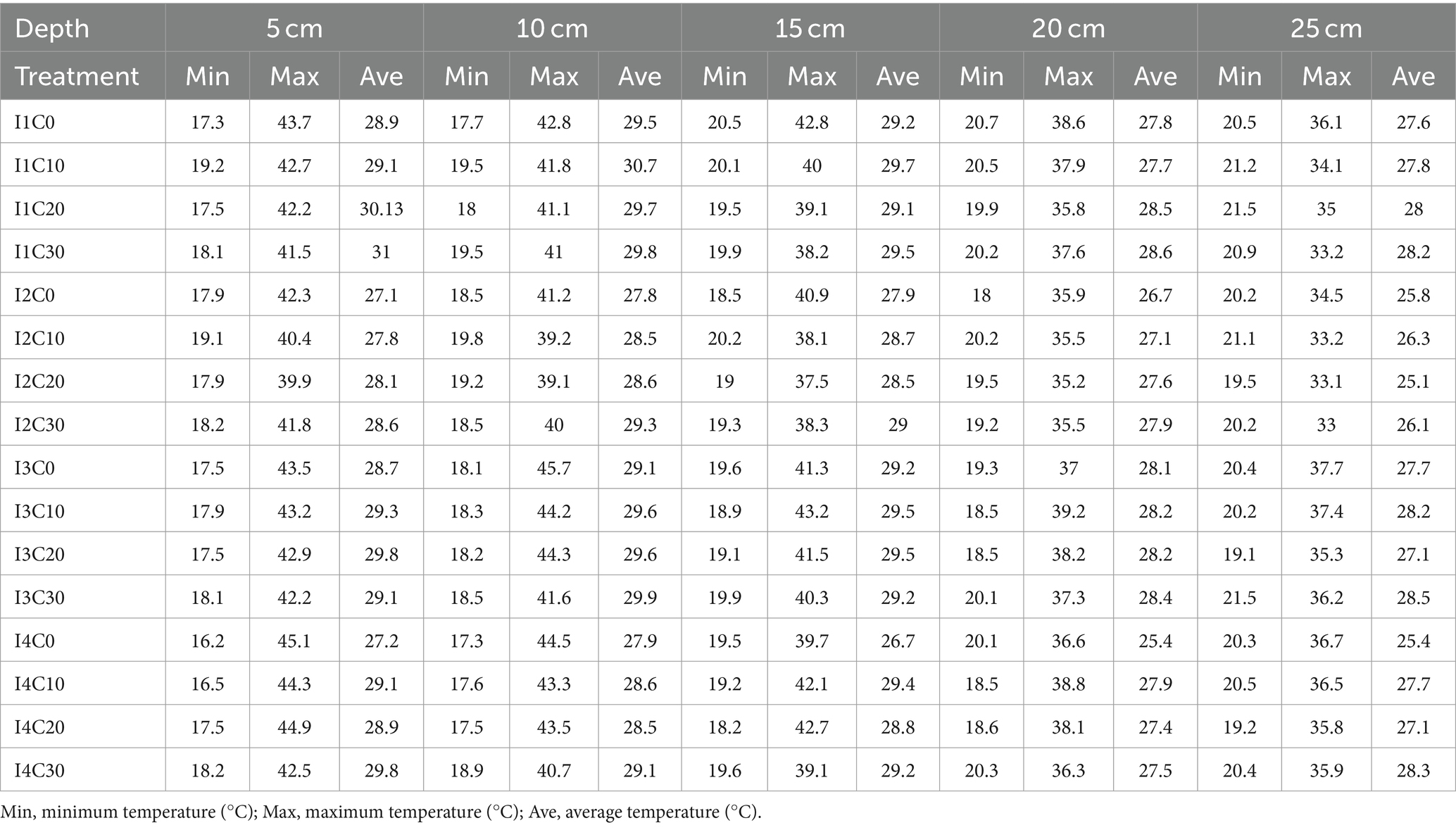
Table 2. The statistical characteristics of soil temperature (°C) at 5, 10, 15, 20, and 25 cm for different treatments at the growth stages of sugar-beet in 2021, 2022, and 2023.
Total Accumulated Soil Temperature (AST) Variation: The patterns of AST over the years 2021, 2022, and 2023 were consistent in Supplementary Figure S11. Increases in biochar application and reductions in irrigation amounts led to an increase in AST. For instance, in 2021 with constant biochar levels, the AST in the I1 treatment showed an average increase of 1.14, 3.56, and 6.58% compared to the I2, I3, and I4 treatments, respectively. In 2022 and 2023, the average increase in AST was 0.25, 1.09, 5.47 and 2.54%, 5.41, 8.58%, respectively. The effect of biochar on AST was most pronounced in the C30 treatment, followed by C20 and C10 when compared to the control (C0). In 2021, the AST of the C30, C20, and C10 treatments rose by an average of 2.89, 5.05, and 8.86%, respectively, relative to the C0 treatment. The corresponding increases in 2022 and 2023 were 3.64, 6.99, 10.56 and 3.08%, 5.74, 7.57%, respectively. The data also revealed that higher irrigation levels resulted in lower AST values. When irrigation levels were constant, the soil temperature under the C30 treatment increased significantly, underscoring those higher levels of biochar application effectively enhance soil temperature.
3.4 Effects of biochar application and irrigation on soil evaporation
Impact on Daily Soil Evaporation (DSE): The combination of biochar application, irrigation amount, and irrigation frequency significantly influenced DSE. In 2021, under the same irrigation conditions, the DSE for the C10, C20, and C30 treatments were 10.68, 19.81, and 42.48% higher, respectively, than that of the C0 treatment, as illustrated in Figure 3. In the following years, the increases in DSE for these treatments compared to C0 were 7.53, 11.91, and 16.66% in 2022, and 15.01, 33.80, and 43.04% in 2023, respectively (Supplementary Figures S12,S13). Furthermore, under a constant biochar application rate, the DSE escalated with an increase in irrigation amount. For instance, compared to the I1 treatment, the I2, I3, and I4 treatments showed increases in DSE by 4.24, 19.72, and 34.78% in 2021, 21.19, 37.78, and 61.93% in 2022, and 16.24, 37.33, and 43.27% in 2023, respectively.
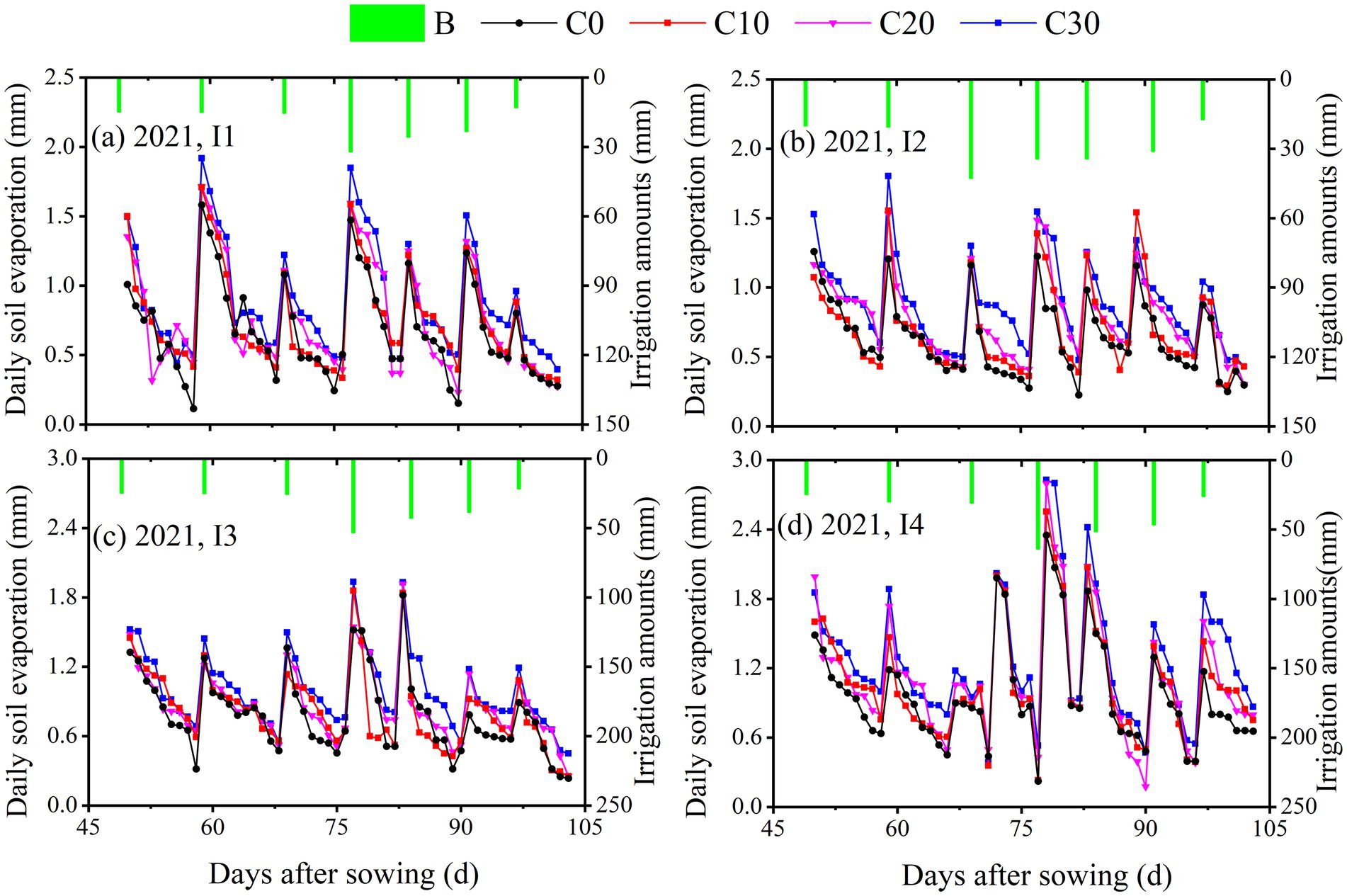
Figure 3. Daily soil evaporation in non-film mulched areas under different biochar application rate and irrigation amount combinations in 2021. I1, I2, I3, and I4 represent irrigation quota at 60, 80, 100, and 120% ETc, respectively; C0, C10, C20, and C30 represent biochar application rates of 0, 10, 20, and 30 t ha−1, respectively.
Accumulated Soil Evaporation (ASE) Patterns: Over the years 2021, 2022, and 2023, the ASE in non-mulched areas under different irrigation and biochar levels is presented in Figure 4. The highest ASE was recorded in the I4C30 treatment, while the lowest was in the I1C0. Under identical irrigation levels, ASE rose with an increase in biochar application. During the harvest stages of 2021, ASE in the C10, C20, and C30 treatments surged by 9.71–26.97%, 6.06–13.50% in 2022, and 12.92–29.71% in 2023 compared to the C0 treatment. Under the same biochar application, the I4 irrigation treatment exhibited the highest ASE, while the I1 treatment showed the lowest. There was a notable difference in ASE across the 3 years under varying biochar applications, as shown in Supplementary Figure S14. Specifically, when irrigation levels were constant, the ASE in the C30 treatment was higher by 31.09, 18.38, and 9.71% compared to the C0, C10, and C20 treatments, respectively. Similarly, under a fixed biochar application, the ASE under the I4 irrigation treatment over 3 years increased by 45.21, 32.11, and 14.18% compared to the I1, I2, and I3 treatments. These findings suggest that while increasing irrigation and biochar application can enhance ASE, there are limits to the extent they can improve soil hydraulic conditions.
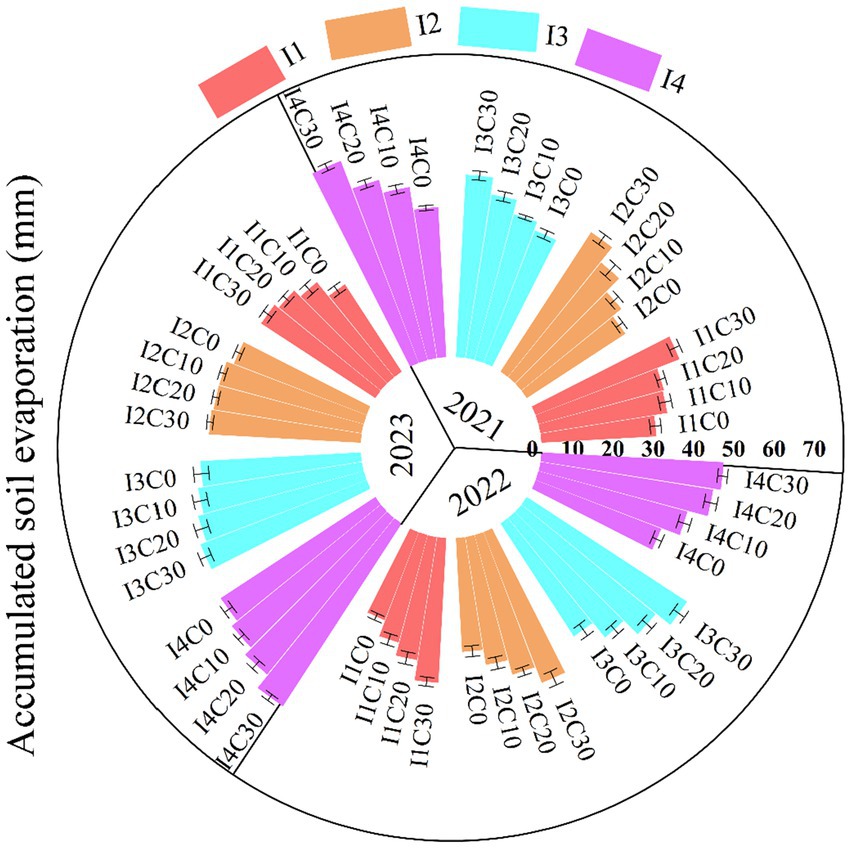
Figure 4. Accumulated soil water evaporation in non-film mulched areas under different biochar application rates and irrigation amounts combinations in 2021, 2022, and 2023. I1, I2, I3, and I4 represent irrigation quota at 60, 80, 100, and 120% ETc, respectively; C0, C10, C20, and C30 represent biochar application rates of 0, 10, 20, and 30 t ha−1, respectively.
3.5 Relationship between soil properties and AOBA and irrigation amount
Multivariate Analysis of Soil Properties: Using MATHEMATIC 10.0 software, a three-dimensional binary quadratic regression analysis was conducted to examine the interplay between biochar application, irrigation amount, and various soil properties (AST, pH, WPSWS, and ASE). Increasing irrigation improved WPSWS; however, it also led to a decrease in AST and an increase in ASE. The application of biochar mitigated these effects, as illustrated in Figure 5.
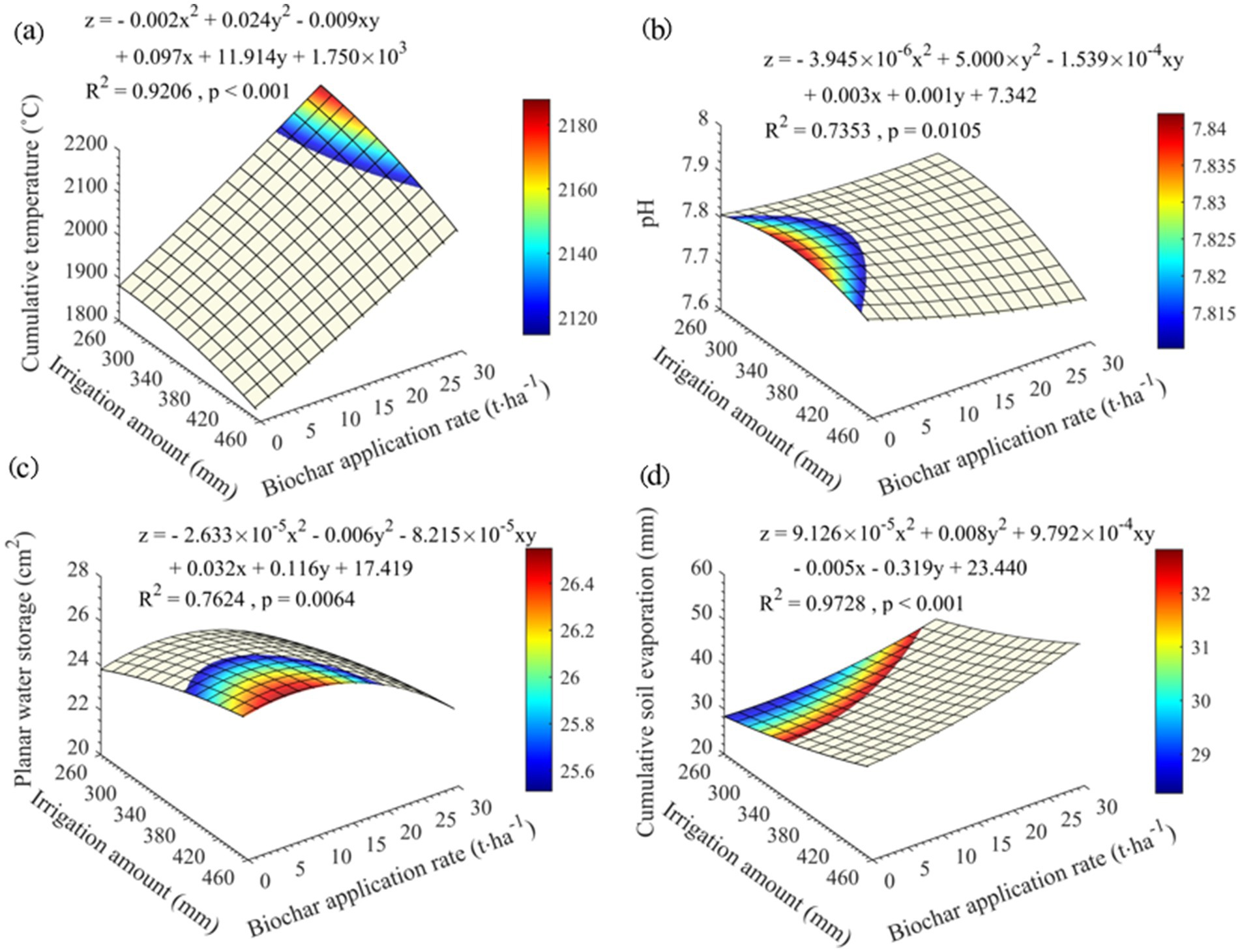
Figure 5. Relationship of accumulated soil daily temperature, pH, weighted plane soil water storage, and accumulated soil evaporation with irrigation amount and biochar application rates. The colored region represents the 90% confidence interval.
Influence on AST: The analysis revealed that both an increase in biochar application and a reduction in irrigation amount positively affected the AST. A suggested irrigation range of 260–390 mm was identified as optimal, considering its correlation with suitable soil temperatures (p < 0.05). Notably, biochar applications in the range of 20–30 t ha−1 demonstrated enhanced soil warming effects. However, it’s crucial to consider that soil temperature is influenced by multiple factors, including warming pathways and the positive impact of temperature on crop growth.
Effect on Soil pH: Soil pH demonstrated a downward trend with higher biochar application and irrigation amounts. An irrigation range of 260–380 mm and a biochar application of 0–10 t ha−1 were optimal for reducing pH while conserving water resources and minimizing biochar usage.
Impact on WPSWS: The WPSWS was observed to be directly proportional to the irrigation amount. It increased initially and then decreased with rising biochar application. The optimal range for irrigation was identified as 340–460 mm, and for biochar application, it was 0–25 t ha−1. This suggests that a certain level of biochar application enhances soil water retention, but excessive application might lead to increased water loss.
Changes in ASE: The ASE escalated with both increased biochar application and irrigation amounts. The minimal ASE was achieved with an irrigation volume of 260–300 mm and biochar application ranging from 0 to 25 t ha−1, indicating a balance between irrigation efficiency and biochar usage for optimal soil moisture conservation.
4 Discussion
4.1 Continuous vs. single biochar application on soil properties
The method of biochar application, whether it be continuous or a single instance, plays a critical role in enhancing soil properties. Research by Liang et al. (2021) indicated that ongoing biochar application over 3 years substantially enhanced soil physical and hydraulic properties in Kuerle, Xinjiang. The improvements were noticeable from the first year, although they did not significantly vary over the three-year period. However, it was observed that soil water retention capacity declined when the Amount of Biochar Applied (AOBA) exceeded 25 t ha−1. In a separate four-year study by Shi et al. (2022) in Bei’an, Heilongjiang Province, an increase in AOBA led to a consistent decrease in soil bulk density and an increase in soil porosity. This study also highlighted that soil water retention capacity followed a unimodal curve, reaching an optimum at 50 t ha−1 of biochar.
Contrasting these findings, Zhang et al. (2022b) compared the effects of long-term continuous biochar application with single applications in Wuhan City, Hubei Province, and found that continuous application yielded superior improvements in soil physicochemical properties. On the other hand, Li et al. (2022b) observed in degraded alpine grasslands that a single biochar application significantly altered soil physical properties, but the benefits diminished in the second year. These variations suggest that the environmental context and the type of biochar can influence the longevity of its beneficial effects. Continuous application of biochar over several years can enhance soil porosity (Zheng et al., 2022), though it may also lead to reduced soil hydraulic attributes (Baronti et al., 2022) and higher input costs (Joseph et al., 2020).
In this study, biochar was applied only in the first year (2021), resulting in notable improvements in soil physicochemical and hydrothermal properties. The most effective soil water retention was observed at an AOBA of 10 t ha−1, and this level of performance was consistent across three consecutive years.
4.2 Effects of irrigation and biochar application on soil physical properties
Biochar has been consistently recognized for its capacity to enhance soil physical properties, as noted by Qi et al. (2024). Studies such as those by Burrell et al. (2016) and Hardie et al. (2014) have demonstrated that the application of low-density biochar significantly reduces soil bulk density. These studies also revealed that an increase in the Amount of Biochar Applied (AOBA) not only improves soil efficiency and permeability but also enhances soil porosity. Wang et al. (2022b) observed a substantial decrease in soil bulk density with an AOBA of 100 t ha−1. Similarly, Li et al. (2018) reported a 22% reduction in soil bulk density for the top 20 cm of soil with an AOBA of 60 t ha−1. The low bulk weight and multiple voids of biochar were the main reasons for this phenomenon, but irrigation had no significant effect on soil physical properties (Liu et al., 2022b). Conversely, Ahmed et al. (2016) discovered that finer biochar particles could potentially clog soil pores, resulting in reduced soil porosity. Igaz et al. (2018) did not find significant changes in soil bulk density after biochar application in powdery soil types. These findings highlight that the effects of biochar are influenced by its type, the amount applied, and the specific experimental conditions (Liang et al., 2021). Selecting appropriate biochar types is crucial to prevent exacerbating soil acidification or alkalization, as indicated by Xiao et al. (2023b). However, Qian et al. (2022) found a 3% increase in soil pH after adding 3 g L−1 of biological carbon. In an experiment using acidified biochar (pH = 6.7) similar to this study, Wang et al. (2022b) applied it to alkaline soils (pH = 7.82) in South Xinjiang, China, and noted that increasing AOBA and pyrolysis level led to an elevation in soil pH. In this study, the application of biochar resulted in a 0.23–1.31% decrease in soil pH, and the reasons for this phenomenon were mainly related to the type of biochar and the fact that it had been subjected to acidification (Wang et al., 2022b). This research corroborates these findings, showing that biochar application significantly reduces soil bulk density and pH while concurrently increasing soil porosity.
4.3 Effects of irrigation combining with biochar application on soil hydro-thermal properties
The dynamics of soil hydrothermal properties, including WPSWS, ASE, and soil temperature, are significantly influenced by both irrigation levels and the AOBA. Xiao et al. (2023a) observed that enhancing irrigation volume, frequency, and total amount resulted in elevated soil moisture levels. Liang et al. (2021) noted a rapid increase in ASE post-irrigation. Furthermore, Yang et al. (2023) found that increased irrigation not only promotes plant growth but also contributes to a reduction in soil temperature, due to decreased soil light intensity and increased soil specific heat capacity, as corroborated by Mahdavi et al. (2016). This study supports these findings, revealing that both WPSWS and ASE amplify with increased irrigation, while higher irrigation volumes lead to a reduction in soil temperature, with the accumulated temperature reaching its peak under the I1C30 treatment over the three-year period.
Biochar, due to its high specific surface area, is effective in enhancing soil water storage capacity (Lee et al., 2022; Zhang et al., 2020). Contrarily, Li et al. (2018) discovered that higher AOBA levels might cause soil internal looseness and promote water evaporation. Excessive biochar application, as shown by Yan et al. (2019) and Wang et al. (2022b), can adversely affect soil water retention, particularly when AOBA surpasses 25 t ha−1. The application of biochar modifies soil water retention behavior and raises soil temperature, with the effect on temperature becoming more pronounced at higher AOBA levels (Feng et al., 2021). According to He et al. (2016) and Zhao et al. (2018), biochar not only increases soil temperature but also stabilizes its fluctuation. Our findings align with these observations; with constant irrigation, WPSWS initially increased and then decreased with rising biochar application, peaking at the C10 level. Furthermore, an increase in AOBA led to heightened ASE and soil temperature, suggesting that while biochar application can improve soil water retention, it also presents limitations in enhancing soil hydraulic properties.
4.4 Optimal combination of irrigation amounts and biochar application rate
The synergistic impact of combining irrigation with biochar application has been evident across various crop types, enhancing growth and yield. Agbna et al. (2017) discovered that an AOBA of 25 t ha−1 effectively compensated for yield losses under deficit irrigation conditions (50% ET0). In research by Guo et al. (2021a), tomato plants grown in soil with a 2% biochar content showed no marked difference in dry matter quality between deficit (60%) and full (90%) irrigation. Similarly, Hou et al. (2023) noted that wheat straw pellet biochar, in conjunction with partial root-zone drying irrigation, significantly boosted cotton growth and water use efficiency. Liu et al. (2021) observed that biochar application under adequate irrigation (90% water holding capacity) favored tobacco biomass accumulation. However, Faloye et al. (2019) indicated that while biochar at 20 t ha−1 enhanced maize’s water use efficiency and yield, the combined effects of biochar and irrigation did not significantly impact yield.
These studies collectively suggest optimal combinations of irrigation and biochar application for various crops. Our findings indicate that an irrigation range of 340–380 mm and an AOBA between 10 and 25 t ha−1 can maximize soil resilience while ensuring sustainable crop production. The enhancement of soil properties through biochar application is a gradual process. This study’s conclusions are based on 3 years of observation, highlighting the need for prolonged experiments to fully comprehend the long-term influences of biochar on soil resilience. Continued research and extended observational studies are crucial for a deeper understanding of biochar’s enduring effects on soil properties.
4.5 Impacts and implications
The interplay between biochar application, irrigation strategies, and soil properties necessitates a comprehensive understanding that spans beyond individual studies. This research contributes to a growing body of knowledge, affirming the potential of biochar as a soil amendment tool. It underscores the necessity of a balanced approach to biochar application, considering both its immediate and lasting effects on soil health. Moreover, this study’s insights are particularly relevant for regions facing similar climatic and soil conditions as the study area. The findings offer practical guidelines for sustainable agricultural practices, emphasizing the importance of customizing biochar application and irrigation strategies to specific environmental conditions and crop requirements.
Advancing Agricultural Practices: The findings of this study have important implications for modern agricultural practices, particularly in the context of sustainable soil management. By elucidating the effects of biochar application in combination with irrigation strategies, this research provides actionable insights that could alter current farming practices. The demonstration of biochar’s ability to improve soil physical properties, enhance water retention, and modulate soil temperature paves the way for more efficient use of water resources and improved crop resilience against climate variability.
Environmental Sustainability: This study contributes to the discourse on environmental sustainability in agriculture. The use of biochar, a product of biomass recycling, aligns with eco-friendly practices by promoting carbon sequestration and reducing the reliance on chemical fertilizers. Furthermore, the optimization of irrigation practices highlighted in this research can lead to more judicious use of water, a critical resource in many agrarian societies facing water scarcity and drought conditions.
Economic Implications: For farmers and agricultural stakeholders, the implications of this research are both practical and economic. The optimization of biochar application and irrigation amounts can lead to better crop yields and quality, which in turn can have a positive impact on the economic viability of farms. By enhancing soil health and crop productivity, farmers can achieve better returns, contributing to the economic sustainability of agricultural communities.
Policy and Educational Aspects: The insights gained from this study can inform policymaking in agriculture, especially in regions where soil degradation and water scarcity are prevalent. Policies that encourage the adoption of sustainable practices like biochar application can have significant impacts on regional and global food security. Additionally, these findings can be incorporated into educational programs and extension services to train the next generation of farmers and agricultural professionals in sustainable farming techniques.
Future Research Directions: While this research advances our understanding of biochar’s impact on soil properties, several questions remain unanswered. Future research should focus on the long-term dynamics of biochar in soil, its interaction with different soil types and climatic conditions, and its cumulative effects on a broader range of soil properties. Additionally, there is a need to explore the economic feasibility and scalability of biochar application in various agricultural settings, ensuring that its benefits are accessible to a wider range of farmers and practitioners.
5 Conclusion
The research presented in this paper provided compelling evidence that biochar application substantially enhances soil properties, with significant implications for agricultural productivity and sustainability. Notably, applying biochar increased soil porosity in the 0–30 cm depth layer. As the Amount of Biochar Applied (AOBA) increased from 10 to 30 t ha−1, we observed a decrease in soil bulk density by 1.31–8.58% and a reduction in soil pH by 0.23–1.31%. These changes underscore biochar’s role in improving soil structure and potentially alleviating soil acidity. Furthermore, the study revealed that increasing irrigation amounts significantly raised Accumulated Soil Evaporation (ASE) and Weighted Planar Soil Water Storage (WPSWS) across various soil depths (0–10, 0–40, and 0–100 cm), particularly when the AOBA remained constant. Specifically, the Accumulated Soil Temperature (AST) under the I1 irrigation treatment exhibited increases ranging from 0.25–2.54% to 5.47–8.58% compared to the I2, I3, and I4 treatments, illustrating the influence of irrigation on soil thermal dynamics. Importantly, we found that with consistent irrigation amounts, an increase in biochar application not only raised AST and ASE but also resulted in a decrease in WPSWS. The optimal biochar application rate, particularly under the C10 treatment, significantly improved WPSWS, with average increases of 6.77, 7.49, and 11.16% compared to the C0, C20, and C30 treatments, respectively. Examining the relationship between various soil properties (including WPSWS, AST, ASE, and soil pH) and the combined effect of irrigation amount and biochar application rate, this study identifies an optimal range for soil improvement conducive to agricultural production. The most favorable outcomes were observed when the irrigation amount ranged between 340 and 380 mm and the AOBA was maintained between 10 and 25 t ha−1.
In conclusion, this research highlights the potential of biochar as a sustainable soil amendment strategy, offering a path to enhance soil health and agricultural productivity. The findings provide valuable insights for farmers, agronomists, and policymakers seeking to optimize soil management practices in an environmentally sustainable manner. The synergistic effect of carefully calibrated irrigation and biochar application opens new avenues for advancing sustainable agriculture in various agroecological contexts.
Data availability statement
The raw data supporting the conclusions of this article will be made available by the authors, without undue reservation.
Author contributions
FJ: Data curation, Methodology, Writing – original draft. YL: Methodology, Supervision, Writing – review & editing. YJ: Conceptualization, Writing – original draft. LL: Data curation, Writing – original draft. RG: Investigation, Writing – original draft. AB: Methodology, Writing – review & editing.
Funding
The author(s) declare that financial support was received for the research, authorship, and/or publication of this article. This research was jointly supported by the National Key Research and Development Program of China (No. 2022YDF1900401), the Key Research and Development Program of Xinjiang (No. 2022B02020-2), NYHXGG project (No. 2023AA303), and the High-end Foreign Experts Introduc-tion Project (G2022172025L).
Conflict of interest
The authors declare that the research was conducted in the absence of any commercial or financial relationships that could be construed as a potential conflict of interest.
The author(s) declared that they were an editorial board member of Frontiers, at the time of submission. This had no impact on the peer review process and the final decision.
Publisher’s note
All claims expressed in this article are solely those of the authors and do not necessarily represent those of their affiliated organizations, or those of the publisher, the editors and the reviewers. Any product that may be evaluated in this article, or claim that may be made by its manufacturer, is not guaranteed or endorsed by the publisher.
Supplementary material
The Supplementary material for this article can be found online at: https://www.frontiersin.org/articles/10.3389/fsufs.2024.1480991/full#supplementary-material
Abbreviations
WPSWS, Weighted planar soil water storage; ET0, Daily reference crop evapotranspiration; ETc, Actual crop evapotranspiration; AOBA, Amount of biochar application; AST, Accumulated soil temperature; DSE, Daily soil evaporation; ASE, Accumulated soil evaporation.
References
Agbna, G. H. D., Dongli, S., Zhipeng, L., Elshaikh, N. A., Guangcheng, S., and Timm, L. C. (2017). Effects of deficit irrigation and biochar addition on the growth, yield, and quality of tomato. Sci. Hortic. 222, 90–101. doi: 10.1016/j.scienta.2017.05.004
Aguirre, J. L., Martín, M. T., González, S., and Peinado, M. (2021). Effects and economic sustainability of biochar application on corn production in a Mediterranean climate. Molecules 26:3313. doi: 10.3390/molecules26113313
Ahmad, M., Akbar, H., Jan, M. T., Khattak, M. J. K., and Bari, A. (2015). Effect of seeding depth, nitrogen placement method and biochar on the growth, yield and its related parameters of sugar beet. SJA 31, 224–231. doi: 10.17582/journal.sja/2015/31.4.224.231
Ahmed, A. S., Kurian, J. K., and Raghavan, V. (2016). Biochar influences on agricultural soils, crop production, and the environment: a review. Environ. Rev. 24, 495–502. doi: 10.1139/er-2016-0008
Allen, R. G., Pereira, L. S., Raes, D., and Smith, M. (1998). Crop evapotranspiration-Guidelines for computing crop water requirements-FAO Irrigation and drainage paper 56. Rome: Food and Agriculture Organization of the United Nations.
Allohverdi, T., Mohanty, A. K., Roy, P., and Misra, M. (2021). A review on current status of biochar uses in agriculture. Molecules 26:5584. doi: 10.3390/molecules26185584
Almaroai, Y. A., and Eissa, M. A. (2020). Effect of biochar on yield and quality of tomato grown on a metal-contaminated soil. Sci. Hortic. 265:109210. doi: 10.1016/j.scienta.2020.109210
Amede, T., Konde, A. A., Muhinda, J. J., and Bigirwa, G. (2023). Sustainable farming in practice: building resilient and profitable smallholder agricultural Systems in sub-Saharan Africa. Sustain. For. 15:5731. doi: 10.3390/su15075731
Asai, H., Samson, B. K., Stephan, H. M., Songyikhangsuthor, K., Homma, K., Kiyono, Y., et al. (2009). Biochar amendment techniques for upland rice production in northern Laos: 1. Soil physical properties, leaf SPAD and grain yield. Field Crop Res. 111, 81–84. doi: 10.1016/j.fcr.2008.10.008
Ayaz, M., Feizienė, D., Tilvikienė, V., Akhtar, K., Stulpinaitė, U., and Iqbal, R. (2021). Biochar role in the sustainability of agriculture and environment. Sustain. For. 13:1330. doi: 10.3390/su13031330
Babu, S., Singh, R., Kumar, S., Rathore, S. S., Yadav, D., Yadav, S. K., et al. (2023). Biochar implications in cleaner agricultural production and environmental sustainability. Environ. Sci. 2, 1042–1059. doi: 10.1039/d2va00324d
Baronti, S., Magno, R., Maienza, A., Montagnoli, A., Ungaro, F., and Vaccari, F. P. (2022). Long term effect of biochar on soil plant water relation and fine roots: results after 10 years of vineyard experiment. Sci. Total Environ. 851:158225. doi: 10.1016/j.scitotenv.2022.158225
Biederman, L. A., and Harpole, W. S. (2013). Biochar and its effects on plant productivity and nutrient cycling: a meta-analysis. GCB Bioenergy 5, 202–214. doi: 10.1111/gcbb.12037
Bo, X., Zhang, Z., Wang, J., Guo, S., Li, Z., Lin, H., et al. (2023). Benefits and limitations of biochar for climate-smart agriculture: a review and case study from China. Biochar 5:77. doi: 10.1007/s42773-023-00279-x
Burrell, L. D., Zehetner, F., Rampazzo, N., Wimmer, B., and Soja, G. (2016). Long-term effects of biochar on soil physical properties. Geoderma 282, 96–102. doi: 10.1016/j.geoderma.2016.07.019
Butt, F. I., Yaqub, G., and Khurshid, F. (2018). Role of biochar products towards environmental management and technologies: a brief review. ChemBioEng Rev. 5, 104–110. doi: 10.1002/cben.201700006
Danielson, R. E., and Sutherland, P. L. (1986). “Porosity” in Methods of soil analysis. Part I. Physical and mineralogical methods. ed. A. Klute, vol. 9 (Madison, WI: American Society of Agronomy), 443–461.
Faloye, O. T., Alatise, M. O., Ajayi, A. E., and Ewulo, B. S. (2019). Effects of biochar and inorganic fertiliser applications on growth, yield and water use efficiency of maize under deficit irrigation. Agric. Water Manag. 217, 165–178. doi: 10.1016/j.agwat.2019.02.044
Feng, L., Dai, J., Tian, L., Zhang, H., Li, W., and Dong, H. (2017). Review of the technology for high-yielding and efficient cotton cultivation in the northwest inland cotton-growing region of China. Field Crop Res. 208, 18–26. doi: 10.1016/j.fcr.2017.03.008
Feng, W., Wang, T., Yang, F., Cen, R., Liao, H., and Qu, Z. (2023). Effects of biochar on soil evaporation and moisture content and the associated mechanisms. Environ. Sci. Eur. 35:66. doi: 10.1186/s12302-023-00776-7
Feng, W., Yang, F., Cen, R., Liu, J., Qu, Z., Miao, Q., et al. (2021). Effects of straw biochar application on soil temperature, available nitrogen and growth of corn. J. Environ. Manag. 277:111331. doi: 10.1016/j.jenvman.2020.111331
Guo, L., Bornø, M. L., Niu, W., and Liu, F. (2021a). Biochar amendment improves shoot biomass of tomato seedlings and sustains water relations and leaf gas exchange rates under different irrigation and nitrogen regimes. Agric. Water Manag. 245:106580. doi: 10.1016/j.agwat.2020.106580
Guo, Q., Huang, G., Guo, Y., Zhang, M., Zhou, Y., and Duan, L. (2021b). Optimizing irrigation and planting density of spring maize under mulch drip irrigation system in the arid region of Northwest China. Field Crop Res. 266:108141. doi: 10.1016/j.fcr.2021.108141
Hardie, M., Clothier, B., Bound, S., Oliver, G., and Close, D. (2014). Does biochar influence soil physical properties and soil water availability? Plant Soil 376, 347–361. doi: 10.1007/s11104-013-1980-x
He, X., Du, Z., Wang, Y., Lu, N., and Zhang, Q. (2016). Sensitivity of soil respiration to soil temperature decreased under deep biochar amended soils in temperate croplands. Appl. Soil Ecol. 108, 204–210. doi: 10.1016/j.apsoil.2016.08.018
Himanshu, S. K., Fan, Y., Ale, S., and Bordovsky, J. (2021). Simulated efficient growth-stagebased deficit irrigation strategies for maximizing cotton yield, crop water productivity and net returns. Agric. Water Manag. 250:106840. doi: 10.1016/j.agwat.2021.106840
Hossain, M. Z., Bahar, M. M., Sarkar, B., Donne, S. W., Ok, Y. S., Palansooriya, K. N., et al. (2020). Biochar and its importance on nutrient dynamics in soil and plant. Biochar 2, 379–420. doi: 10.1007/s42773-020-00065-z
Hou, J., Zhang, J., Liu, X., Ma, Y., Wei, Z., Wan, H., et al. (2023). Effect of biochar addition and reduced irrigation regimes on growth, physiology and water use efficiency of cotton plants under salt stress. Ind. Crop. Prod. 198:116702. doi: 10.1016/j.indcrop.2023.116702
Hu, Y., Cao, Y., Ma, C., and Yan, W. (2024). Nano-biochar as a potential amendment for metal(loid) remediation: implications for soil quality improvement and stress alleviation. J. Environ. Manag. 351:119658. doi: 10.1016/j.jenvman.2023.119658
Igaz, D., Šimanský, V., Horák, J., Kondrlová, E., Domanová, J., Rodný, M., et al. (2018). Can a single dose of biochar affect selected soil physical and chemical characteristics? J. Hydrol. Hydromech. 66, 421–428. doi: 10.2478/johh-2018-0034
Joseph, S., Pow, D., Dawson, K., Rust, J., Munroe, P., Taherymoosavi, S., et al. (2020). Biochar increases soil organic carbon, avocado yields and economic return over 4 years of cultivation. Sci. Total Environ. 724:138153. doi: 10.1016/j.scitotenv.2020.138153
Kang, S., Hao, X., Du, T., Tong, L., Su, X., Lu, H., et al. (2017). Improving agricultural water productivity to ensure food security in China under changing environment: from research to practice. Agric. Water Manag. 179, 5–17. doi: 10.1016/j.agwat.2016.05.007
Lee, X., Yang, F., Xing, Y., Huang, Y., Xu, L., Liu, Z., et al. (2022). Use of biochar to manage soil salts and water: effects and mechanisms. Catena 211:106018. doi: 10.1016/j.catena.2022.106018
Li, L., Liu, H., Gong, P., Lin, E., Bai, Z., Li, P., et al. (2023a). Multi-objective optimization of winter irrigation for cotton fields in salinized freeze-thaw areas. Eur. J. Agron. 143:126715. doi: 10.1016/j.eja.2022.126715
Li, J., Shao, X., Huang, D., Liu, K., Shang, J., Zhang, Q., et al. (2022a). Short-term biochar effect on soil physicochemical and microbiological properties of a degraded alpine grassland. Pedosphere 32, 426–437. doi: 10.1016/S1002-0160(21)60084-X
Li, T., Wang, M., Cui, R., Li, B., Wu, T., Liu, Y., et al. (2023b). Waterlogging stress alters the structure of sugar beet rhizosphere microbial community structure and recruiting potentially beneficial bacterial. Ecotox. Environ Safe. 262:115172. doi: 10.1016/j.ecoenv.2023.115172
Li, C., Xiong, Y., Qu, Z., Xu, X., Huang, Q., and Huang, G. (2018). Impact of biochar addition on soil properties and water-fertilizer productivity of tomato in semi-arid region of Inner Mongolia, China. Geoderma 331, 100–108. doi: 10.1016/j.geoderma.2018.06.014
Li, Y., Yao, N., Liang, J., Wang, X., Jia, Y., Jiang, F., et al. (2022b). Optimum biochar application rate for peak economic benefit of sugar beet in Xinjiang, China. Agric. Water Manag. 272:107880. doi: 10.1016/j.agwat.2022.107880
Liang, J. (2021b). Improvement mechanism of biochar on saline-alkali soil in cotton and sugar beet field under mulched drip irrigation in arid area of Xinjiang. [dissertation/master’s thesis]. Yang ling (Shaanxi): Northwest A&F University.
Liang, J., Li, Y., Si, B., Wang, Y., Chen, X., Wang, X., et al. (2021). Optimizing biochar application to improve soil physical and hydraulic properties in saline-alkali soils. Sci. Total Environ. 771:144802. doi: 10.1016/j.scitotenv.2020.144802
Liu, J., Li, H., Yuan, Z., Feng, J., Chen, S., Sun, G., et al. (2024). Effects of microbial fertilizer and irrigation amount on growth, physiology and water use efficiency of tomato in greenhouse. Sci. Hortic. 323:112553. doi: 10.1016/j.scienta.2023.112553
Liu, X., Ma, Y., Manevski, K., Andersen, M. N., Li, Y., Wei, Z., et al. (2022b). Biochar and alternate wetting-drying cycles improving rhizosphere soil nutrients availability and tobacco growth by altering root growth strategy in Ferralsol and Anthrosol. Sci. Total Environ. 806:150513. doi: 10.1016/j.scitotenv.2021.150513
Liu, X., Manevski, K., Liu, F., and Andersen, M. N. (2022a). Biomass accumulation and water use efficiency of faba bean-ryegrass intercropping system on sandy soil amended with biochar under reduced irrigation regimes. Agric. Water Manag. 273:107905. doi: 10.1016/j.agwat.2022.107905
Liu, X., Wei, Z., Ma, Y., Liu, J., and Liu, F. (2021). Effects of biochar amendment and reduced irrigation on growth, physiology, water-use efficiency and nutrients uptake of tobacco (Nicotiana tabacum L.) on two different soil types. Sci. Total Environ. 770:144769. doi: 10.1016/j.scitotenv.2020.144769
Liu, X., Ye, Y., Liu, Y., Zhang, A., Zhang, X., Li, L., et al. (2014). Sustainable biochar effects for low carbon crop production: a 5-crop season field experiment on a low fertility soil from Central China. Agric. Syst. 129, 22–29. doi: 10.1016/j.agsy.2014.05.008
Mahdavi, S. M., Neyshabouri, M. R., and Fujimaki, H. (2016). Assessment of some soil thermal conductivity models via variations in temperature and bulk density at low moisture range. Eurasian Soil Sci. 49, 915–925. doi: 10.1134/S1064229316080068
Misra, V., Mall, A. K., and Singh, D. (2023). Rhizoctonia root-rot diseases in sugar beet: pathogen diversity, pathogenesis and cutting-edge advancements in management research. Microbe 1:100011. doi: 10.1016/j.microb.2023.100011
Mohamed, B. A., Ellis, N., Kim, C. S., Bi, X., and Emam, A. E. (2016). Engineered biochar from microwave-assisted catalytic pyrolysis of switchgrass for increasing water-holding capacity and fertility of sandy soil. Sci. Total Environ. 566-567, 387–397. doi: 10.1016/j.scitotenv.2016.04.169
Premalatha, R. P., Poorna Bindu, J., Nivetha, E., Malarvizhi, P., Manorama, K., and Parameswari, E. (2023). A review on biochar’s effect on soil properties and crop growth. Front. Energy Res. 11:1092637. doi: 10.3389/fenrg.2023.1092637
Qi, X., Yang, G., Li, Y., Hou, Z., Shi, P., Wang, S., et al. (2024). Optimizing biochar application rates for improved soil chemical environments in cotton and sugar-beet fields under trickle irrigation with plastic mulch. Soil Tillage Res. 235:105893. doi: 10.1016/j.still.2023.105893
Qian, W., Liang, J. Y., Zhang, W. X., Huang, S. T., and Diao, Z. H. (2022). A porous biochar supported nanoscale zero-valent iron material highly efficient for the simultaneous remediation of cadmium and lead contaminated soil. J. Environ. Sci. 113, 231–241. doi: 10.1016/j.jes.2021.06.014
Schmidt, H., Kammann, C., Hagemann, N., Leifeld, J., Bucheli, T. D., Sánchez Monedero, M. A., et al. (2021). Biochar in agriculture – a systematic review of 26 global meta-analyses. GCB Bioenergy 13, 1708–1730. doi: 10.1111/gcbb.12889
Shi, G., Hou, R., Li, T., Fu, Q., Wang, J., Zhou, W., et al. (2023). Effects of biochar and freeze–thaw cycles on the bacterial community and multifunctionality in a cold black soil area. J. Environ. Manag. 342:118302. doi: 10.1016/j.jenvman.2023.118302
Shi, G., Wu, Y., Li, T., Fu, Q., and Wei, Y. (2022). Mid- and long-term effects of biochar on soil improvement and soil erosion control of sloping farmland in a black soil region. China J. Environ. Manag. 320:115902. doi: 10.1016/j.jenvman.2022.115902
Singh, A. (2021). Soil salinization management for sustainable development: a review. J. Environ. Manag. 277:111383. doi: 10.1016/j.jenvman.2020.111383
Sun, F., Dong, X., Li, S., Sha, H., Gao, W., Bai, X., et al. (2023). Genome-wide identification and expression analysis of SUT gene family members in sugar beet (Beta vulgaris L.). Gene 870:147422. doi: 10.1016/j.gene.2023.147422
Tan, S., Wang, Q., Xu, D., Zhang, J., and Shan, Y. (2017). Evaluating effects of four controlling methods in bare strips on soil temperature, water, and salt accumulation under film-mulched drip irrigation. Field Crop Res. 214, 350–358. doi: 10.1016/j.fcr.2017.09.004
Wang, X., Li, Y., Wang, H., Wang, Y., Biswas, A., Wai Chau, H., et al. (2022a). Targeted biochar application alters physical, chemical, hydrological and thermal properties of salt-affected soils under cotton-sugar-beet intercropping. Catena 216:106414. doi: 10.1016/j.catena.2022.106414
Wang, Y., Ma, X., Saleem, M., Yang, Y., and Zhang, Q. (2022b). Effects of corn stalk biochar and pyrolysis temperature on wheat seedlings growth and soil properties stressed by herbicide sulfentrazone. Environ. Technol. 25:102208. doi: 10.1016/j.eti.2021.102208
Wang, R., Wan, S., Sun, J., and Xiao, H. (2018). Soil salinity, sodicity and cotton yield parameters under different drip irrigation regimes during saline wasteland reclamation. Agric. Water Manag. 209, 20–31. doi: 10.1016/j.agwat.2018.07.004
Xiao, C., Ji, Q., Zhang, F., Li, Y., Fan, J., Hou, X., et al. (2023a). Effects of various soil water potential thresholds for drip irrigation on soil salinity, seed cotton yield and water productivity of cotton in Northwest China. Agric. Water Manag. 279:108172. doi: 10.1016/j.agwat.2023.108172
Xiao, J., Li, X. G., Cao, Y. N., and Chen, G. C. (2023b). Does micro/nano biochar always good to phytoremediation? A case study from multiple metals contaminated acidic soil using Salix jiangsuensis “172”. Carbon Res. 2:21. doi: 10.1007/s44246-023-00053-5
Xiao, Q., Zhu, L., Shen, Y., and Li, S. (2016). Sensitivity of soil water retention and availability to biochar addition in rainfed semi-arid farmland during a three-year field experiment. Field Crop Res. 196, 284–293. doi: 10.1016/j.fcr.2016.07.014
Xu, L., Du, H., and Zhang, X. (2019). Spatial distribution characteristics of soil salinity and moisture and its influence on agricultural irrigation in the Ili River valley, China. Sustain. For. 11:7142. doi: 10.3390/su11247142
Xu, J., Niu, W., Zhang, M., Li, Y., Lu, W., Li, K., et al. (2016). Effect of biochar addition on soil evaporation. Chin. J. Appl. Ecol. 27, 3505–3513. doi: 10.13287/j.1001-9332.201611.018
Yan, Q., Dong, F., Li, J., Duan, Z., Yang, F., and Li, X. (2019). Effects of maize straw-derived biochar application on soil temperature, water conditions and growth of winter wheat. Eur. J. Soil Sci. 70, 1280–1289. doi: 10.1111/ejss.12863
Yan, F., Zhang, F., Fan, J., Hou, X., Bai, W., Liu, X., et al. (2021). Optimization of irrigation and nitrogen fertilization increases ash salt accumulation and ions absorption of drip-fertigated sugar beet in saline-alkali soils. Field Crop Res. 271:108247. doi: 10.1016/j.fcr.2021.108247
Yang, L., Heng, T., He, X., Yang, G., Zhao, L., Li, Y., et al. (2023). Spatial-temporal distribution and accumulation characteristics of residual plastic film in cotton fields in arid oasis area and the effects on soil salt transport and crop growth. Soil Tillage Res. 231:105737. doi: 10.1016/j.still.2023.105737
Yu, B., Chen, M., Grin, I., and Ma, C. (2020). Mechanisms of sugar beet response to biotic and abiotic stresses. Adv. Exp. Med. Biol. 1241, 167–194. doi: 10.1007/978-3-030-41283-8_10
Zhang, J., Li, K., Gao, Y., Feng, D., Zheng, C., Cao, C., et al. (2022a). Evaluation of saline water irrigation on cotton growth and yield using the AquaCrop crop simulation model. Agric. Water Manag. 261:107355. doi: 10.1016/j.agwat.2021.107355
Zhang, D., Luo, Z., Liu, S., Li, W., Tang, W., and Dong, H. (2016). Effects of deficit irrigation and plant density on the growth: yield and fiber quality of irrigated cotton. Field Crop Res. 197, 1–9. doi: 10.1016/j.fcr.2016.06.003
Zhang, R.-H., Xie, Y., Zhou, G., Li, Z., Ye, A., Huang, X., et al. (2022b). The effects of short-term, long-term, and reapplication of biochar on the remediation of heavy metal-contaminated soil. Ecotox. Environ. Safe 248:114316. doi: 10.1016/j.ecoenv.2022.114316
Zhang, Y., Yang, J., Yao, R., Wang, X., and Xie, W. (2020). Short-term effects of biochar and gypsum on soil hydraulic properties and sodicity in a saline-alkali soil. Pedosphere 30, 694–702. doi: 10.1016/S1002-0160(18)60051-7
Zhao, B., Connor, D., Zhang, J., Peng, T., Shen, Z., Tsang, D. C. W., et al. (2018). Effect of pyrolysis temperature, heating rate, and residence time on rapeseed stem derived biochar. J. Clean. Prod. 174, 977–987. doi: 10.1016/j.jclepro.2017.11.013
Keywords: biochar, irrigation, saline-alkali soil, soil evaporation, soil temperature
Citation: Jiang F, Li Y, Jia Y, Li L, Guan R and Biswas A (2024) Compound effects of biochar application and irrigation on soil water and temperature transport. Front. Sustain. Food Syst. 8:1480991. doi: 10.3389/fsufs.2024.1480991
Edited by:
Maria Pilar Bernal, Spanish National Research Council (CSIC), SpainCopyright © 2024 Jiang, Li, Jia, Li, Guan and Biswas. This is an open-access article distributed under the terms of the Creative Commons Attribution License (CC BY). The use, distribution or reproduction in other forums is permitted, provided the original author(s) and the copyright owner(s) are credited and that the original publication in this journal is cited, in accordance with accepted academic practice. No use, distribution or reproduction is permitted which does not comply with these terms.
*Correspondence: Yi Li, bGl5aUBud2FmdS5lZHUuY24=