- 1Terrestrial Ecosystem Management Laboratory, Graduate School of Global Environmental Studies, Kyoto University, Kyoto, Japan
- 2International Rice Research Institute South Asia Regional Centre, Varanasi, Uttar Pradesh, India
- 3International Maize and Wheat Improvement Centre (CIMMYT), NASC Complex, Pusa New Delhi, India
- 4International Crops Research Institute for the Semi-Arid Tropics, Patancheru, Telangana, India
- 5Borlaug Institute of South Asia (BISA), International Maize and Wheat Improvement Center, Pusa, Bihar, India
This study evaluates the impact of conservation agriculture (CA) on soil carbon sequestration and crop productivity in the Indo-Gangetic Plains, focusing on short-term effects over 3 and 5 years. Conducted at two distinct sites, Karnal and Samastipur, the research compares zero tillage, permanent raised beds, and conventional tillage systems across diverse cropping patterns. Initial findings after 3 years showed no significant differences in carbon and nitrogen stocks at Karnal, while Samastipur’s maize-mustard-mungbean rotation on permanent raised beds showed increased carbon stocks. Notably, after 5 years, significant differences in soil carbon stocks emerged at both sites, with improved organic matter input indicated by coarse particulate organic matter (cPOM) formation. The study confirms the potential of POXC and POC as early indicators for carbon sequestration in CA systems, highlighting the role of CA practices in enhancing soil health and crop productivity sustainably.
Highlights
• CA systems in the Indo-Gangetic Plains enhanced crop yields with zero tillage and raised beds.
• After 5 years, significant soil carbon changes were observed, not after 3 years.
• POXC and POC are effective short-term carbon sequestration indicators in CA systems.
• The study underscores short-term carbon stock accumulation indicators in CA practices.
1 Introduction
The Indo-Gangetic Plain (IGP) has been facing the challenge of land degradation and the crisis of stagnant crop productivity for decades. These issues largely owe their origin to the overexploitation of resources and the practice of rice-wheat cropping system in the region (Gathala et al., 2014; Mishra et al., 2018). The intensive tillage technique in rice-wheat (RW) systems calls for a great deal of labor, water, and energy, making it more expensive. Thus, when applied in RW systems, intensive tillage results in escalating production costs and dwindling profitability (Jat et al., 2019; Aryal et al., 2015). Burning crop residues is a practice commonly adopted by farmers in India particularly northern states (Punjab and Haryana), as it helps to prepare seedbeds (Kaur et al., 2022; Chaudhary et al., 2022). Such practices contribute to the degeneration of the soil quality, directly caused by the loss of soil carbon, and give rise to environmental hazards, eventually leading to lower productivity of RW cropping systems (Jha et al., 2014; Kumar et al., 2022). This also leads to the problem of lower productivity and making sustainability of this cropping system a major threat to food security (Gathala et al., 2014). So, looking at an alternative practice for sustainable agriculture in South Asia is very important (Mishra et al., 2018). Moreover, the massive population growth coupled with climate change, particularly prevalent in the IGP region, can be addressed by the shift from conventional agricultural practices to CA, which can function as a new approach or intervention to sustainably transform extensive agriculture into intensive agriculture (Jat et al., 2019). Conservation agriculture is steadily gathering momentum in the IGP region, as it is an effective approach for ameliorating the state of resource preservation and combating soil nutrient mining. At the same time, it also substantially contributes to improving the quality of the environment (Mishra et al., 2016; Jat et al., 2019).
CA embracing minimal tillage, residue recycling, and crop diversification, enhances soil carbon sequestration and fertility (Dey et al., 2020), which is pivotal for balancing carbon inputs with outputs. This approach, bolstered by the strategic management of carbon inputs—type, quantity, quality, and placement-aims to strengthen soil carbon stocks and maintain fertility, which is critical for promoting plant growth and soil health (Franzluebbers, 2010).
Notably, introducing maize into the rice-wheat (RW) systems addresses the intensive water and labor demands, presenting a sustainable alternative with lower water requirements and higher profitability. The adoption of permanent raised beds (PB) further epitomizes the CA ethos by reducing cultivation costs and environmental impact, supporting soil carbon dynamics, especially in short-term trials where understanding carbon stock and fractions becomes essential (Govaerts et al., 2009; Chauhan et al., 2012).
While CA’s benefits on soil health and productivity are well documented, the specific impact of PB on these aspects remains under-explored, warranting this study’s focus on carbon dynamics within PB-based CA systems. This investigation is critical, given the nuanced responses of carbon fractions to CA practices, which vary with agroecological conditions, highlighting the need for a deeper understanding of short-term carbon dynamics under PB (Das et al., 2018; Parihar et al., 2018).
Crop diversification, integral to CA, enriches microbial substrate heterogeneity and chemical complexity, fostering a diverse soil decomposer community that enhances carbon sequestration through the formation of recalcitrant residues (Hooper et al., 2005; Parihar et al., 2018). Additionally, incorporating mungbean into rotations increases total organic carbon (TOC) due to its effects on belowground biomass, residue recycling, and carbon rhizodeposition, underscoring the importance of legumes in CA systems for improving soil carbon dynamics (Ganeshamurthy and Srinivasarao, 2009; Hazra et al., 2018).
This study’s exploration of carbon dynamics under PB systems and the inclusion of crop diversification, mainly through mungbean, presents an innovative approach to understanding the short-term impacts of CA practices on soil carbon sequestration, addressing gaps in current knowledge and contributing valuable insights into sustainable agricultural practices in the Indo-Gangetic Plains. It is well recognized that changes in total organic C (TOC) by residue management in a short-term experiment (i.e., <5 years) are somewhat challenging to observe because of its slower turnover rate and large soil organic carbon (SOC) pool (De Oliveira Ferreira et al., 2018; Guo and Gifford, 2002; West and Post, 2002; Lal, 2020; Rumpel et al., 2018). However, labile carbon fractions, namely permanganate oxidizable carbon (Bongiorno et al., 2019; Culman et al., 2012; Hazra et al., 2018), microbial biomass carbon (Awale et al., 2017; Kumar et al., 2019), hot water extractable carbon and dissolved organic carbon (Awale et al., 2017; Bongiorno et al., 2019; Xue et al., 2013), particulate organic carbon (Awale et al., 2017; Bongiorno et al., 2019; Kumar et al., 2019) and carbon fraction based on different oxidisability (Nandan et al., 2019; Parihar et al., 2018; Samal et al., 2017; Singh et al., 2015) are more responsive towards the CA-based management practices in the short-term. The difference in their magnitude, however, is subject to changes in factors such as climate, soil type, crop type and rotation, crop residue, and agronomic management (Chaudhari et al., 2015; Prasad et al., 2016; Somasundaram et al., 2018).
While many studies in the tropical regions of South Asia have concentrated on crop productivity within CA, particularly in RW cropping systems (Sharma et al., 2020), our research broadens this focus. We investigate both productivity and the indicators of soil carbon accumulation in short-term CA trials. This approach not only provides insights into immediate agricultural outcomes but also explores the sustainability aspects of CA practices through the lens of soil health. Several studies have reported positive changes in the TOC during long-term experiments under CA systems in the IGP region (Das et al., 2013; Ghimire et al., 2017; Choudhury et al., 2014; Jat et al., 2014, 2019). Soil organic matter (SOM) is the important parameters and the key driving force that impacts the soil ecosystem’s physical and chemical processes, shaping an agricultural system’s productivity, resilience, and sustainability. The SOC and soil mineral N play a significant role in the agroecosystem’s long-term sustainable crop productivity, directly impacting the soil’s physical, chemical, and biological properties (Jha et al., 2014; Tian and Shi, 2014). However, scanty information is available on soil carbon dynamics in terms of lability and stabilization under CA management practices in the IGP region of India. This study posits that the combination of diversified biomass carbon input and no-tillage practices, influenced by variations in agro-ecological conditions, plays a pivotal role in determining the extent of carbon sequestration across different agro-ecologies. Thus, it is necessary to identify the changes in carbon fractions that respond to the short-term (3 and 5 years, to be precise) CA management practices; for this purpose, two sites (1,300 km apart) in the IGP, India, have been selected. The reason for selecting two contrasting sites lies in the differences in landholding size, cropping intensity, climate, and soil type, which can significantly alter the carbon dynamics even after applying similar treatments.
This study aims to evaluate the impact of resource conservation practices on crop yield and total organic carbon (TOC) stocking in Karnal (West Indo-Gangetic Plains) and Samastipur (Middle Indo-Gangetic Plains). It further seeks to examine temporal changes in soil carbon’s physical fractionation and explore the relationships between soil carbon stock, its labile fractions, and the input of crop residues. This comprehensive approach quantifies the immediate agricultural benefits and delves into the underlying soil carbon dynamics that support long-term soil health and productivity.
2 Materials and methods
2.1 Experimental location and description
This study was conducted at two sites of IGP, i.e., Taraori, Karnal, India (Lat. 29°48’ N and Long. 76°55′ E; called Karnal hereinafter) and Borlaug Institute of South Asia (BISA) farm, Samastipur, India (Lat. 25°57’ N and Long. 85°40′ E; called Samastipur hereinafter; Figure 1). In both the sites, the trial was initiated in 2012 (June in Karnal and November in Samastipur) on a well level (0–1% slope) having loam and silty loam soil texture, respectively. In Karnal, the climate is semiarid with hot, dry to wet summers (May–October) and cool, dry winters (November–April) having 24°C average annual temperature and 670 mm mean annual rainfall, of which 75–80% is received usually during southwest monsoon (July to September). Karnal is classified as humid subtropical, dry winter climate (Classification: Cwa). In Samastipur, the climate is monsoon-influenced humid subtropical climate, characterized by hot and humid summers and cold winters with an average annual temperature and rainfall of 25.5°C and 1,200 mm, respectively, 70% of which is received between July to September. The dominant soils at the study sites in the Indo-Gangetic Plains, specifically in Karnal and Samastipur, are characterized as Anthraquic Haplustepts and Oxyaquic Haplustepts, respectively. These soils feature low organic carbon content and an alkaline reaction. The texture of Karnal’s soil is loam, while Samastipur’s soil is silty loam. Such soil properties are crucial for understanding the impacts of conservation agriculture practices on soil health and carbon sequestration in these regions.
2.2 Experimental design and treatments
The experiment’s design using a randomized block with six treatments in Karnal (K1-K6) and four in Samastipur (S1-S4) was chosen to effectively account for the inherent variability within and across these contrasting agro-ecological sites. This methodological approach allows for a nuanced examination of CA practices’ impacts on soil carbon sequestration by accommodating site-specific conditions such as soil type, climate, and cropping systems. The plot size in both, Karnal and Samastipur, was same as 350 m2, with three replications in each site (Figure S1). Both sites’ treatments were based on conventional and conservation agriculture systems of rice, wheat, and maize-based cropping systems (Tables 1, 2). In addition, a mustard-based cropping system in Samastipur seems more promising and suitable in this climatic region. The diversified cropping systems were designed to include various cereals and legumes with different C:N ratios to study their effect on soil carbon and nitrogen dynamics. The sowing and harvesting time of all crops in other treatments is depicted in Figures 2a,b. The crops in different treatments were grown according to the recommended agronomic practices. Figure 3 illustrates the innovative cropping systems employed on permanent raised beds (PB) in two distinct regions: Karnal and Samastipur. In Karnal, the cropping system of maize-wheat-mungbean is adopted, showcasing a diversification strategy aimed at optimizing crop yields and improving soil health through varied crop rotations. Samastipur experiments with both maize-wheat-mungbean and maize-mustard-mungbean systems, reflecting an effort to tailor cropping patterns to local agro-ecological conditions while enhancing sustainability.
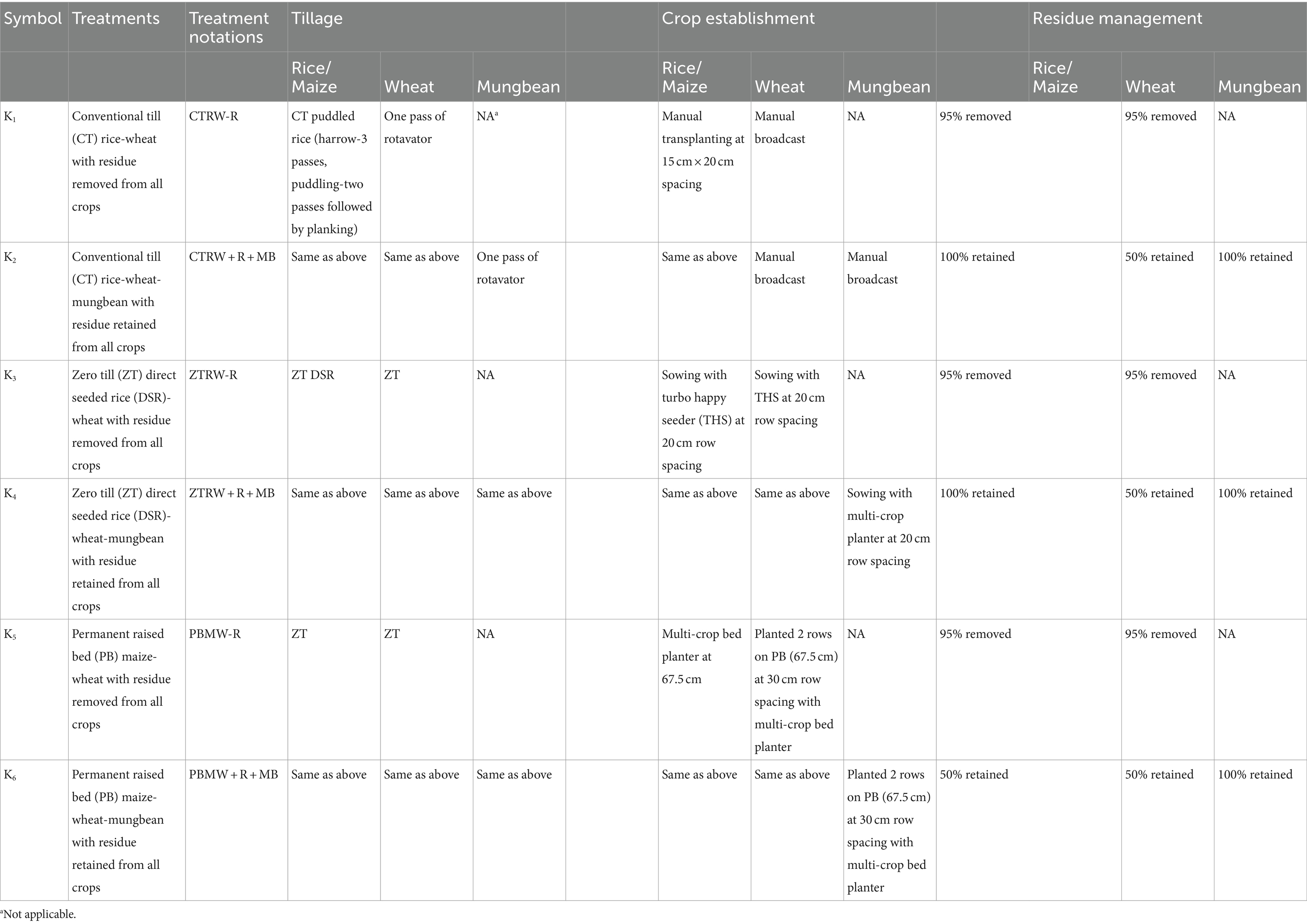
Table 1. Treatments abbreviation and description of management practices for rice, maize, wheat and mungbean crops in Karnal, India.
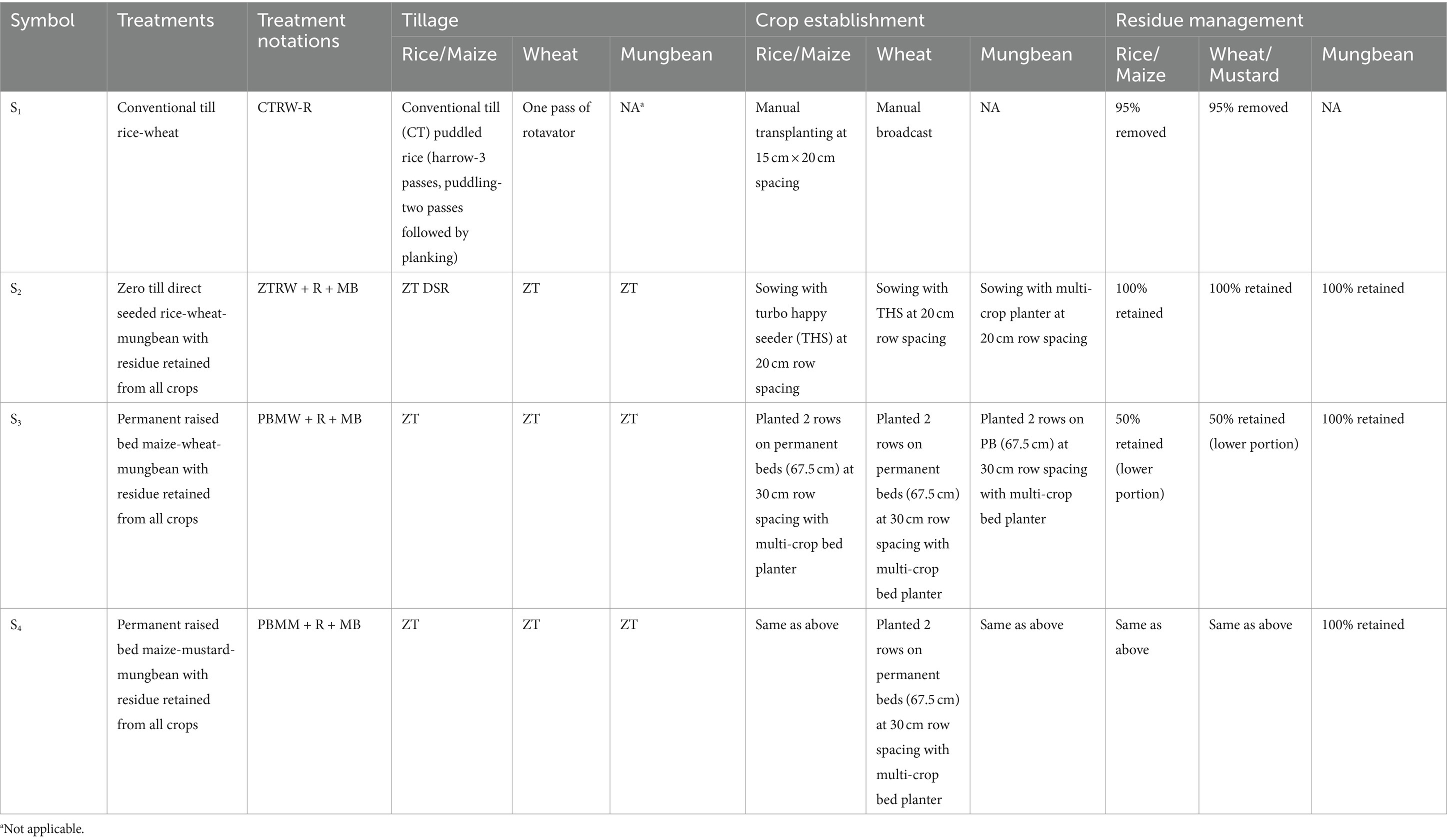
Table 2. Treatments abbreviation and description of management practices for rice, maize, wheat and mungbean crops in Samastipur, India.
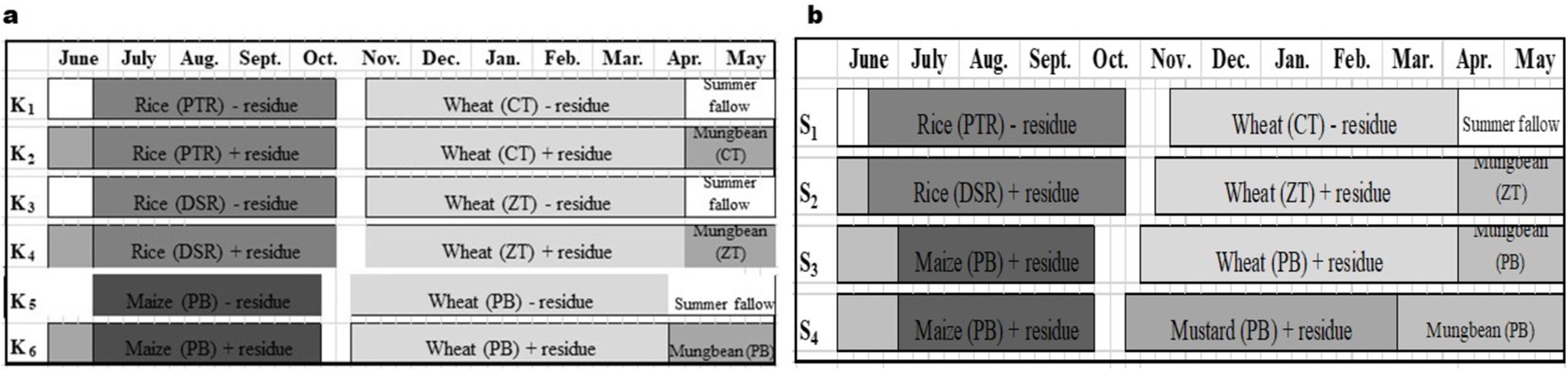
Figure 2. (a,b) Calendar of sowing, harvesting and residue management practices followed in cropping systems from 2012 to 2017 in Taraori, Karnal, Haryana, India and in BISA Farm, Samastipur, Bihar, India; PTR: puddle transplanted rice; ZT-DSR: zero-till direct-seeded rice; CT: conventional tillage; *Nursery seeding for transplanted rice was done on the same day as on DSR was sown. In the case of transplanted rice, 30 days old seedling was used for transplanting. Where, K1-Conventional till (CT) rice-wheat with residue removed from all crops, K2-Conventional till (CT) rice-wheat-mungbean with residue retained from all crops, K3-Zero till (ZT) direct-seeded rice (DSR)-wheat with residue removed from all crops, K4-Zero till (ZT) direct-seeded rice (DSR)-wheat-mungbean with residue retained from all crops, K5-Permanent raised bed (PB) maize-wheat with residue removed from all crops, K6-Permanent raised bed (PB) maize-wheat-mungbean with residue retained from all crop.
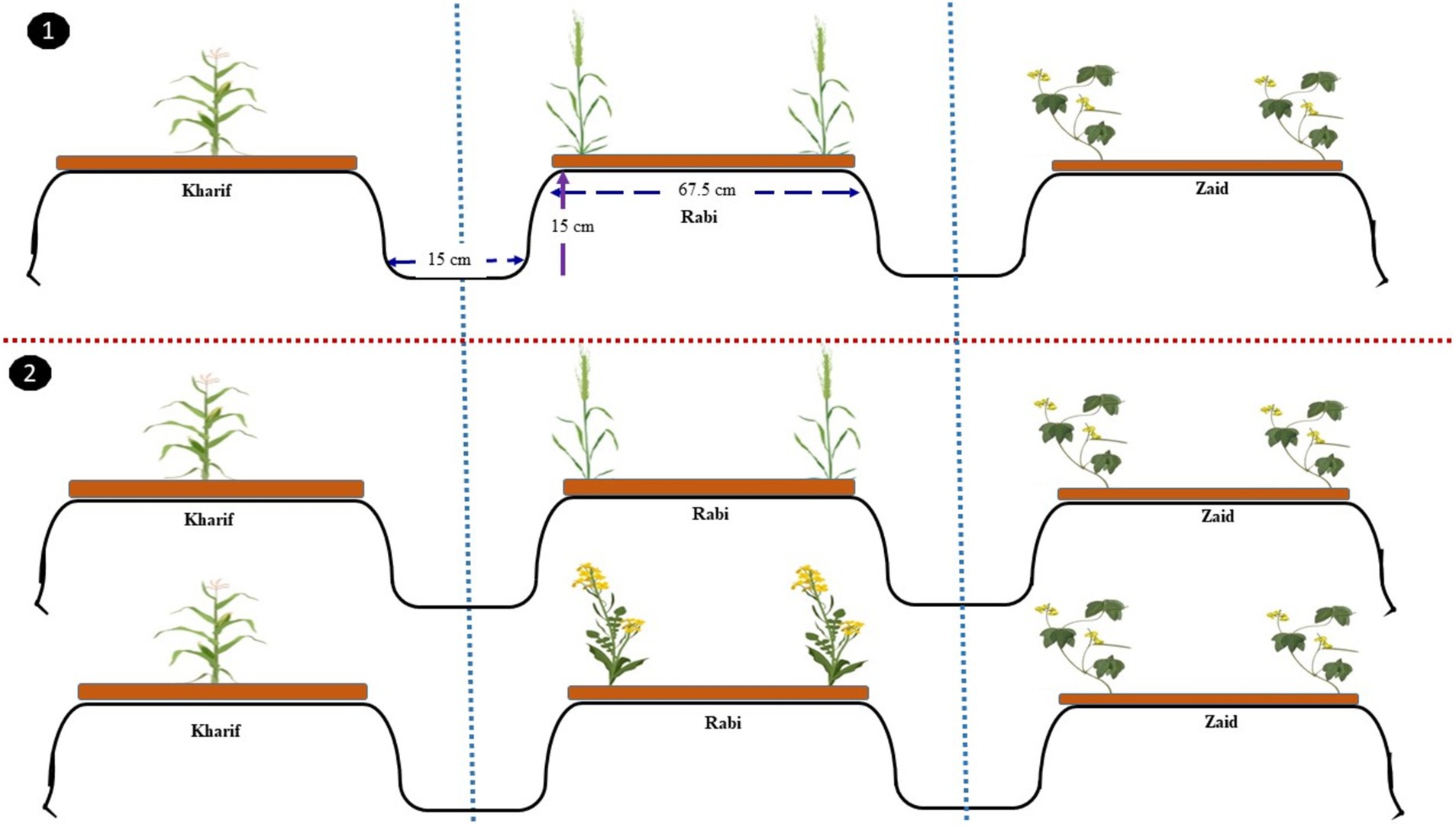
Figure 3. Different cropping systems on permanent raised beds (PB) in Karnal: maize-wheat-mungbean (1) and Samastipur (2): maize-wheat-mungbean and maize-mustard-mungbean.
2.3 Crop yield, cropping system productivity and plant-derived C inputs
Rice and wheat were harvested and threshed at crop maturity by a combined harvester. Mustard, maize and mungbean were harvested manually. In mungbean, pods were picked manually and the biomass was retained in the plots. For grain and biomass yield, crops were harvested manually from randomly selected 4 m × 2.7 m quadrate from three places within each field. The crop yield was recorded at 12–14% moisture content, while biomass yield was calculated after drying in an oven at 50°C for 72 h. The mustard yield was converted to wheat equivalent yield (WEY) to compare the treatments using Equation 1. System yield (SY) was calculated on rice equivalent yield (REY) basis for wheat, maize, mustard and mungbean grain yield by using Equation 2.
Where, MSP is the Minimum Support Price set by Govt. of India; INR is the India National Rupee (rice: 1,360 ± 85.4, wheat: 1,547 ± 135.0, maize: 1,297 ± 71.8, mustard: 3,440 ± 405.3 and mungbean: 4,950 ± 447.6).
After the crop harvest, the total biomass yield of roots and stubble was estimated using 0.5 m × 0.5 m quadrate, throwing randomly three places within each plot and extrapolated to a hectare basis at 0–15 cm soil depth. After 5 years of experimentation, samples from each crop were taken for total carbon (C) and nitrogen (N) in the crop residue, stubble and root by dry combustion method with an NC analyzer (Vario Max CHN, Elementar). The average total C and N in the crop residue, stubble and root at both sites are presented in Supplementary Tables S1, S2.
2.4 Soil sampling and analysis
After 3 and 5 years of experimentation (in 2015 and 2017 for Karnal and 2016 and 2018 for Samastipur), composite soil samples (five randomly selected points within each plot) were collected from two depths (0–5 cm and 5–15 cm) with a soil augur of 5 cm internal diameter.
The selection of two soil depths (0–5 cm and 5–15 cm) for sampling in this study is strategic, aimed at capturing the most significant changes in carbon (C) and nitrogen (N) content within the plough layer, which is generally up to 15 cm. This depth aligns with standard ploughing practices and encompasses the majority of root activity, ensuring that the assessment of soil health and nutrient dynamics is both relevant and comprehensive. The methodology for soil sampling, involving composite samples from five random points within each plot, and the subsequent analysis, including the use of an improved chromic acid digestion method for total organic carbon (TOC) determination, is designed to provide a detailed understanding of soil carbon and nitrogen changes attributable to conservation agriculture practices over the 3 and 5-year experimentation periods. To quantify the overall changes in C and N content up to plough layer (0–15 cm), Equation 3 was used that quantifies the weighted mean of the soil C and the soil N for the layer 0–15 cm. The soil samples were air-dried and passed through a 2 mm sieve for analysis. As the soil samples of both sites contain a substantial amount of carbonates, total organic carbon (TOC) was analyzed by an improved chromic acid digestion method (Tyurin, 1966). Soil bulk density (BD) was determined with a core sampler and cores of 5 cm height and 5 cm diameter (Blake and Hartge, 1986).
2.5 Particulate organic matter (POM) fractionation
POM fractionation provides insights into the soil’s ability to sequester carbon and maintain fertility, highlighting the effectiveness of CA practices in enhancing soil health. Additionally, understanding POM dynamics allows for assessing soil organic matter turnover and stability, critical factors in evaluating the sustainability of agricultural systems.
Twenty grams of air-dried and sieved soil (2 mm), five glass beads and 50 mL of distilled water were put in a 100 mL plastic bottle and shaken for 16 h at 20°C in an end-over-end shaker at 40 rotations per minute to ensure physical fractionation of soil organic matter (Koutika et al., 2017). The soil was wet-sieved to separate the suspension into three fractions: 2,000–250 μm, 250–53 μm and 0–53 μm. The following fractions were collected: coarse (cPOM, 2,000–250 μm) and fine POM fractions (fPOM, 250–53 μm) fractions and the organo-mineral fraction (OMF, < 53 μm). All fractions were dried at 65°C and weighed. The recovery of fractionation (sum of all fraction mass divided by soil mass) was 1.0047. Proportional soil mass (%) was calculated by using Equation 4
The C in cPOM fraction was analyzed by dry combustion method with the NC analyzer, whereas C in fPOM and OMF fractions was analyzed by Tyurin method to avoid overestimation as these fractions contain carbonates. Total N in all the fractions were analyzed by NC analyzer.
2.6 TOC stock and TN stock in different soil fractions of POM
The size of the TOC and TN in each POM fraction was calculated by multiplying their respective TOC and TN concentration with soil mass (mentioned as SM in equation) in each depth (0–5 cm and 5–15 cm) and mass of soil in each fraction using Equation (5).
Where, soil mass in Mg (depth 0–0.05 m) = BD (Mg m−3) × 500; Soil mass in Mg (depth 0.05–0.15 m) = BD (Mg m−3) × 1,000. TOC stock and TN stock up to plough layer (0–15 cm) were simply adding stock in 0–5 cm and 5–15 cm.
2.7 Permanganate oxidizable carbon (POXC)
The modified procedure for determining permanganate oxidizable carbon (POXC) is adapted from Culman et al. (2012). Briefly, 2.5 g of air-dried soil were dispersed in 20 mL of KMnO4 solution (0.02 mol L−1), with shaking on a reciprocating shaker (120 strokes minute−1) for exactly 2 min. After shaking, the soil solution was allowed to settle for 10 min, then 1 mL of the supernatant was transferred into a 50 mL centrifuge tube and 49 mL of deionized water was added. A spectrophotometer read the absorbance of the diluted supernatant at 550 nm. The change in the concentration of KMnO4 was used to estimate the amount of oxidized C. Sample POXC was calculated as in Weil et al. (2003) using Equation 6
Where 0.02 mol L−1 is the initial concentration of the KMnO4 reactant, a and b are the intercept and slope of the standard curve, respectively, Abs is the sample absorbance, 9,000 mg C mol−1 is the amount of C (0.75 mol) oxidized by 1 mol of MnO4, changing Mn7+ to Mn4+, and 0.0025 kg soil is the amount of soil reacted with KMnO4. The POXC stock was calculated by multiplying the respective POXC concentration with BD and soil depth.
2.8 Statistical analysis
The analysis of variance (ANOVA) was carried out separately for both locations to determine the statistically significant differences among the treatments in both sites using SPSS (17.0) statistical package (SPSS Inc.). Tukey’s post hoc test was used for multiple comparisons among the treatments (p < 0.05). Pearson correlation coefficient was used to evaluate the relationship between the carbon inputs and soil properties.
3 Results
3.1 Crop grain yield and system yield
After 5 years, significant yield differences emerged among tillage and residue management treatments in both sites (Table 3). In Karnal, maize in K5 and K6 (PB) outperformed ZT (K3, K4) and CT (K1, K2). Wheat and mungbean yields favored CA practices, particularly in ZT and PB systems. System yield was notably higher in treatments incorporating crop residue and mungbean (K2, K4, K6), with K6 leading (20.2% higher than K1). In Samastipur, rice yields in S1 and S2 were comparable, but PB treatments (S3, S4) yielded lower for maize compared to CT (S1). Wheat yields showed no significant difference. The highest system yields were in S2 (15.0 Mg ha−1) and S4 (13.7 Mg ha−1), with ZT (S2) achieving a 23.0% increase over CT (S1). Plant-derived C input data are detailed in Supplementary Table S3.
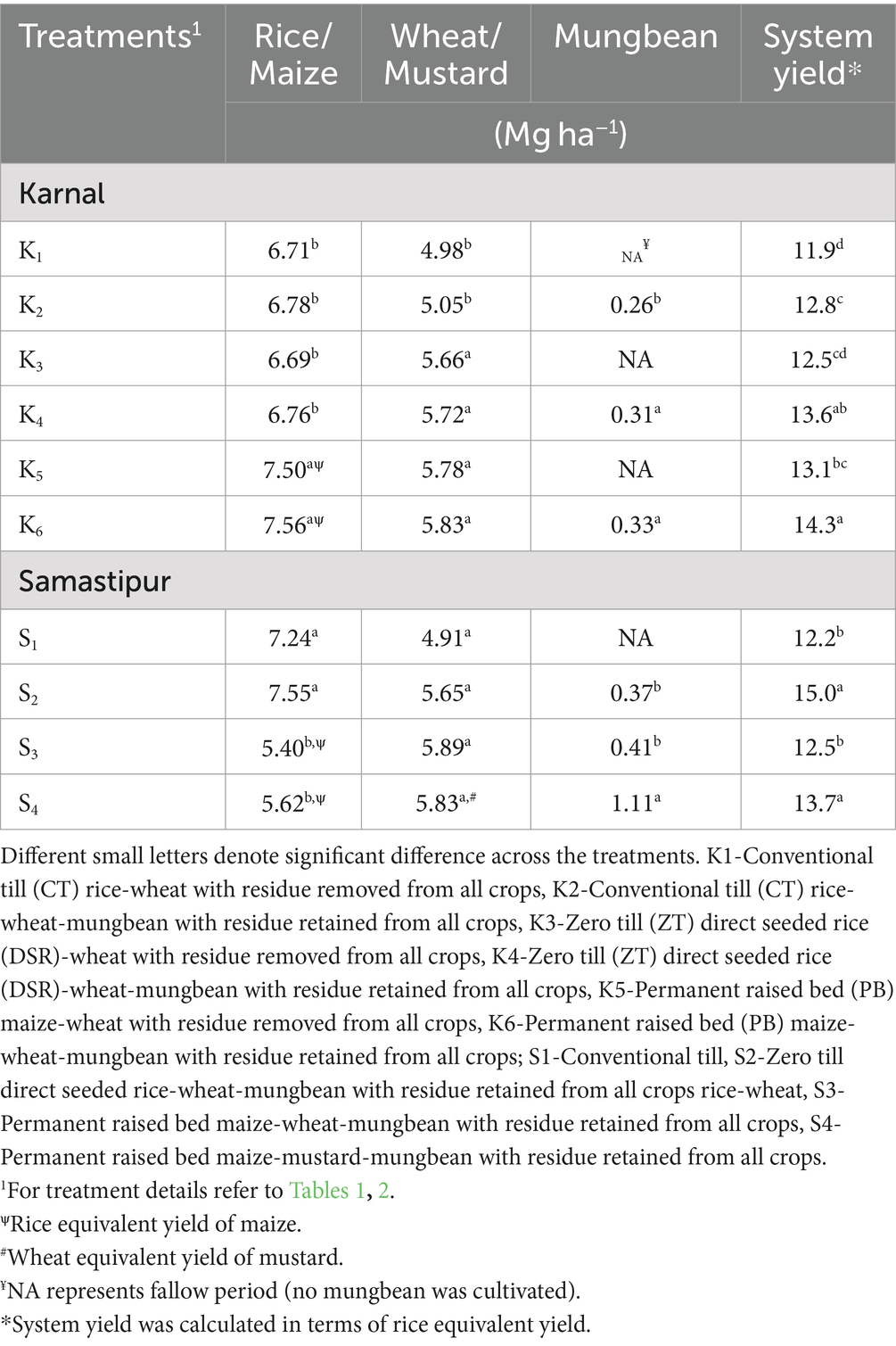
Table 3. Means of 5 years crop grain yield and system yield as affected by tillage and residue management practices.
3.2 Stocks of TOC and TN in two different agroecology of IGP
In Karnal, significant differences in TOC stocks at 0–5 cm depth were observed among treatments in both 2015 and 2017, with K4 and K6 showing the highest stocks, 17 and 11% higher than K1, respectively, in 2015; and 17 and 20% higher than K1 in 2017 (Table 4). TN stocks differed significantly in 2015 but not in 2017, with K6 (≈21% and ≈13% in 2015 and 2017 respectively) and K4 (≈12 and ≈15% in 2015 and 2017, respectively) showing the highest increases over K1. At 5–15 cm depth, TOC stocks showed significant change in 2015 and 2017 (8–11% (2015) and 5–10% (2017) in K4 to K6 over K1). While TN stocks differed in K4 (23%) in 2015 and, K4 (42%) and K6 (37%) in 2017 (Table 4).
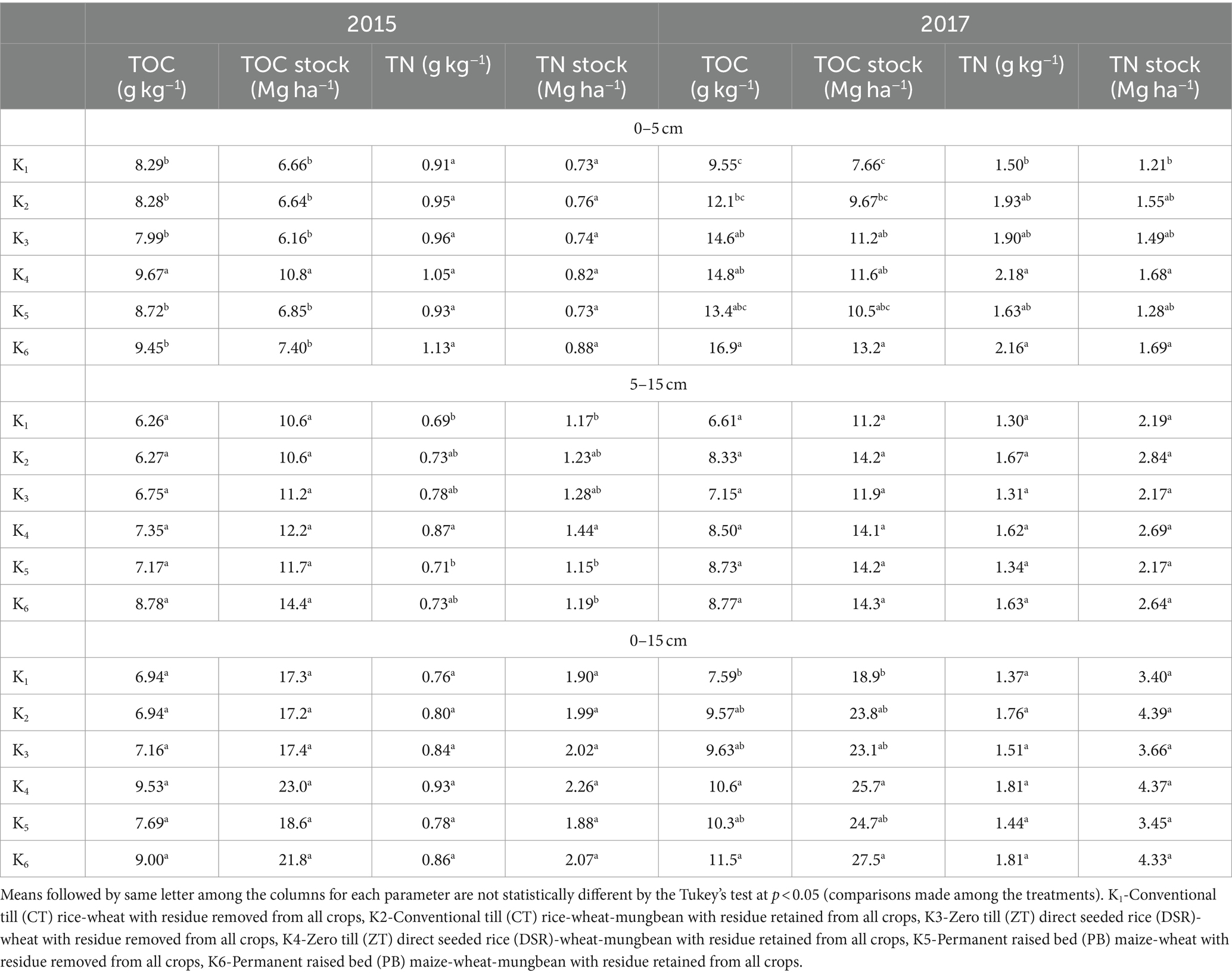
Table 4. Carbon and nitrogen stock under different tillage and residue management practices in Karnal.
TOC stocks in the 0–15 cm layer significantly differed in 2015 and 2017, with K6 and K4 substantially higher by 8 to 13% than K1. TN stocks showed significant differences in K4 and K6 2015 (18 and 9%) and 2017 (31 and 27%).
In Samastipur, TOC stocks at 0–5 cm depth differed significantly in both sampling periods, with the highest in S2 (24%) and S3 (26%) in 2016 and S2 (20%) in 2018 showing significantly higher stocks than others (Table 5). TN stocks at 0–5 cm showed significant differences in all the treatments (S2 to S4) over S1. At 5–15 cm depth, TOC and TN stocks in 2016 and 2018 showed no significant changes. Overall, TOC stocks at 0–15 cm depth varied significantly, with the highest values recorded in S3 (11%) in 2016 and 2018 (8%), while TN stocks showed improvement in 2016 with highest level in S2 (28%) and S4 (30%) but there was no change or variation in 2018.
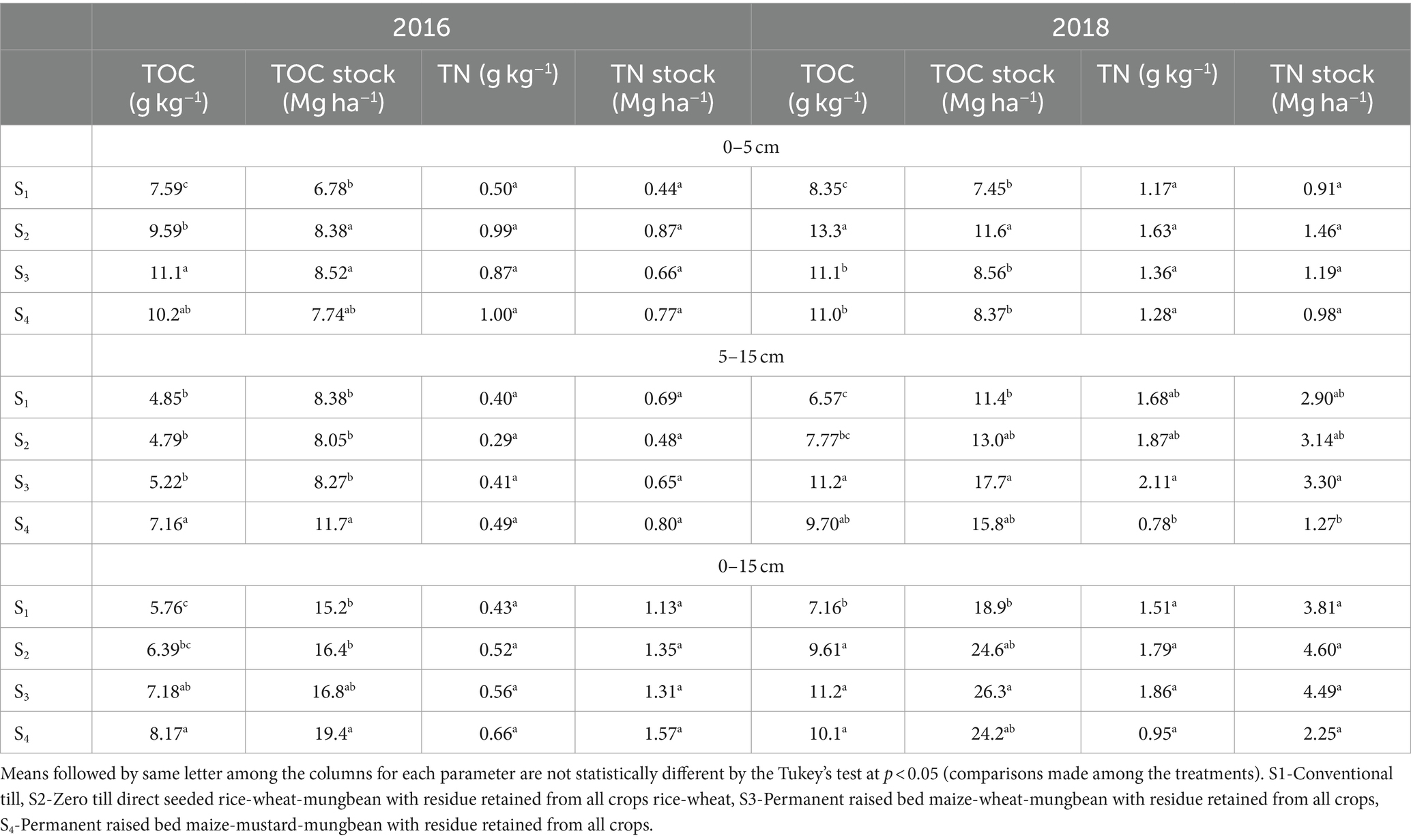
Table 5. Carbon and nitrogen stock under different tillage and residue management practices in Samastipur.
3.3 POXC as a labile pool of TOC and its stock
In Karnal, the POXC content and stock exhibited no significant differences among the treatments in 2015 at all the soil depths (0–5 cm, 5–15 cm, and 0–15 cm; Table 6). In 2017, POXC stock was 37.0% higher in K4 than in K1 at 0–5 cm soil depth. No significant differences were observed among the treatments in 2017 at 5–15 cm depth. In 0–15 cm depth, POXC content and stock showed a similar trend as 0–5 cm depth, with the highest value in K4, which was 32.5% higher than K1 after the 2018 sampling. In Samastipur, POXC stock at 0–5 cm depth recorded no significant difference in 2016, whereas, in 2018, S2 showed 93.8% higher POXC stock than S1 at 0–5 cm depth (Table 7).
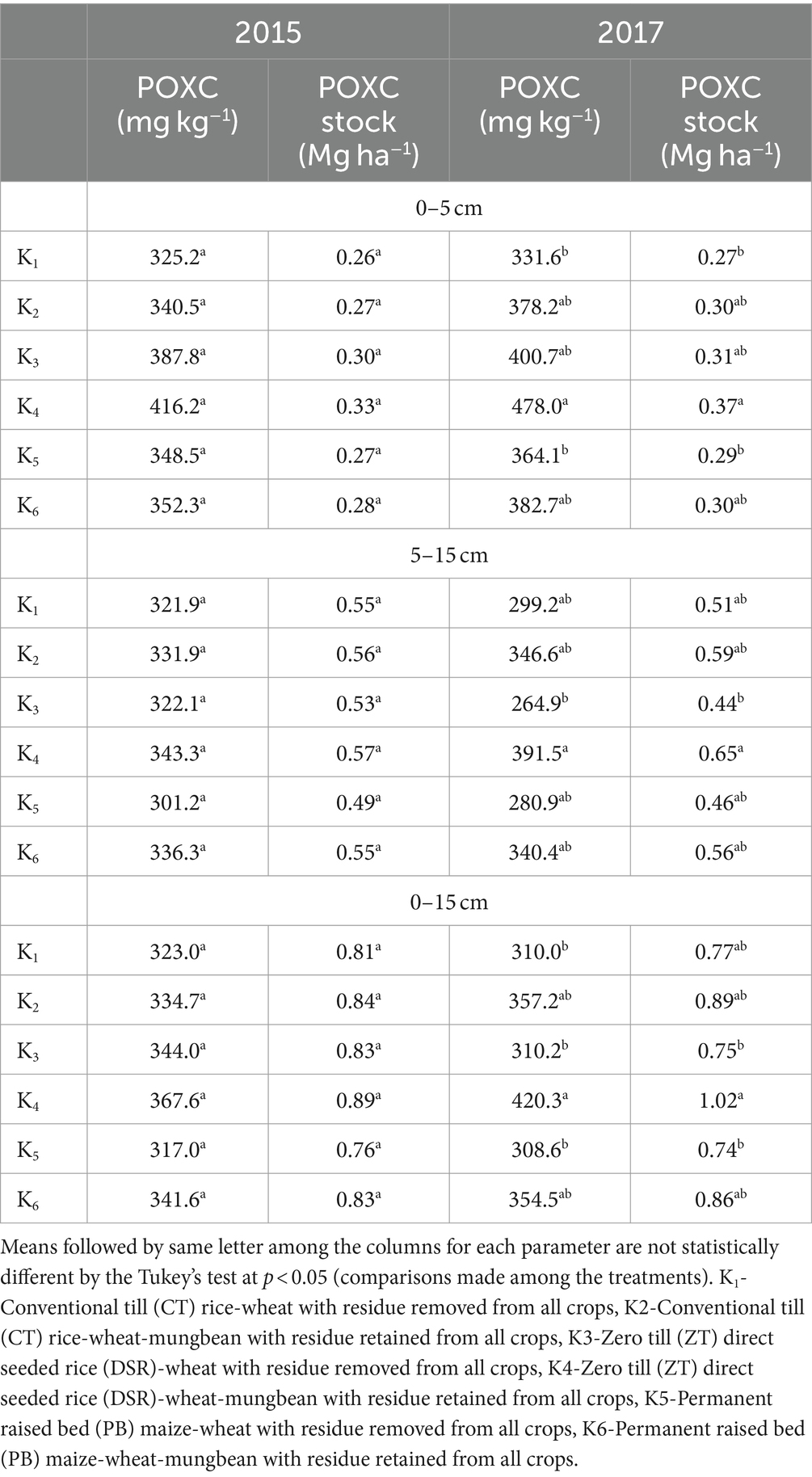
Table 6. Permanganate oxidisable carbon (POXC) content and stock in different tillage and residue management in Karnal.
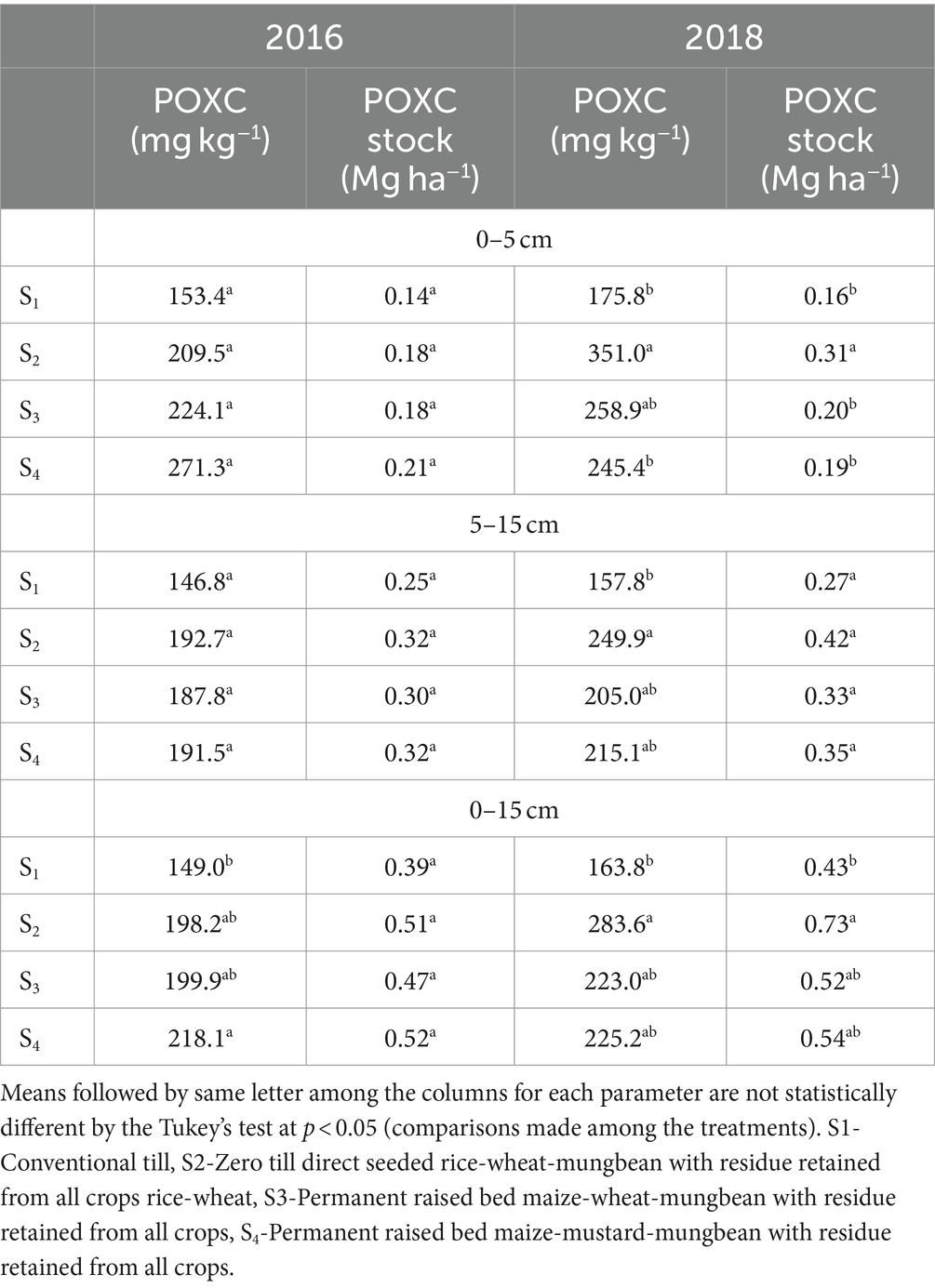
Table 7. Permanganate oxidisable carbon (POXC) stock under different tillage and residue management practices in Samastipur.
3.4 Characteristics of POM fractions
3.4.1 Physical fractionation and associated C and N stock in Karnal
Crop residue significantly increased cPOM mass at 0–5 cm depth but had minimal impact on fPOM and OMF (Table 8). In 2015, cPOM mass in K4 and K6 was 78.8 and 79.7% higher than K1. By 2017, K4’s cPOM was 3.90 times higher than K1. TOC and TN in cPOM significantly differed in 2017, with K4 highest at 3.42 Mg C ha−1 and 0.37 Mg N ha−1. No difference was noted at 5–15 cm in cPOM, fPOM, and OMF mass or stocks (Supplementary Table S4). By 2017, only cPOM showed significant differences in mass, TOC, and TN at 0–15 cm depth, with K4’s TOC highest at 4.94 Mg C ha−1, 3.53 times above K1 (Supplementary Table S5), mirroring the trend in TN stock. PB treatments (K5 and K6) under maize-wheat with or without residue and mungbean showed no significant difference.
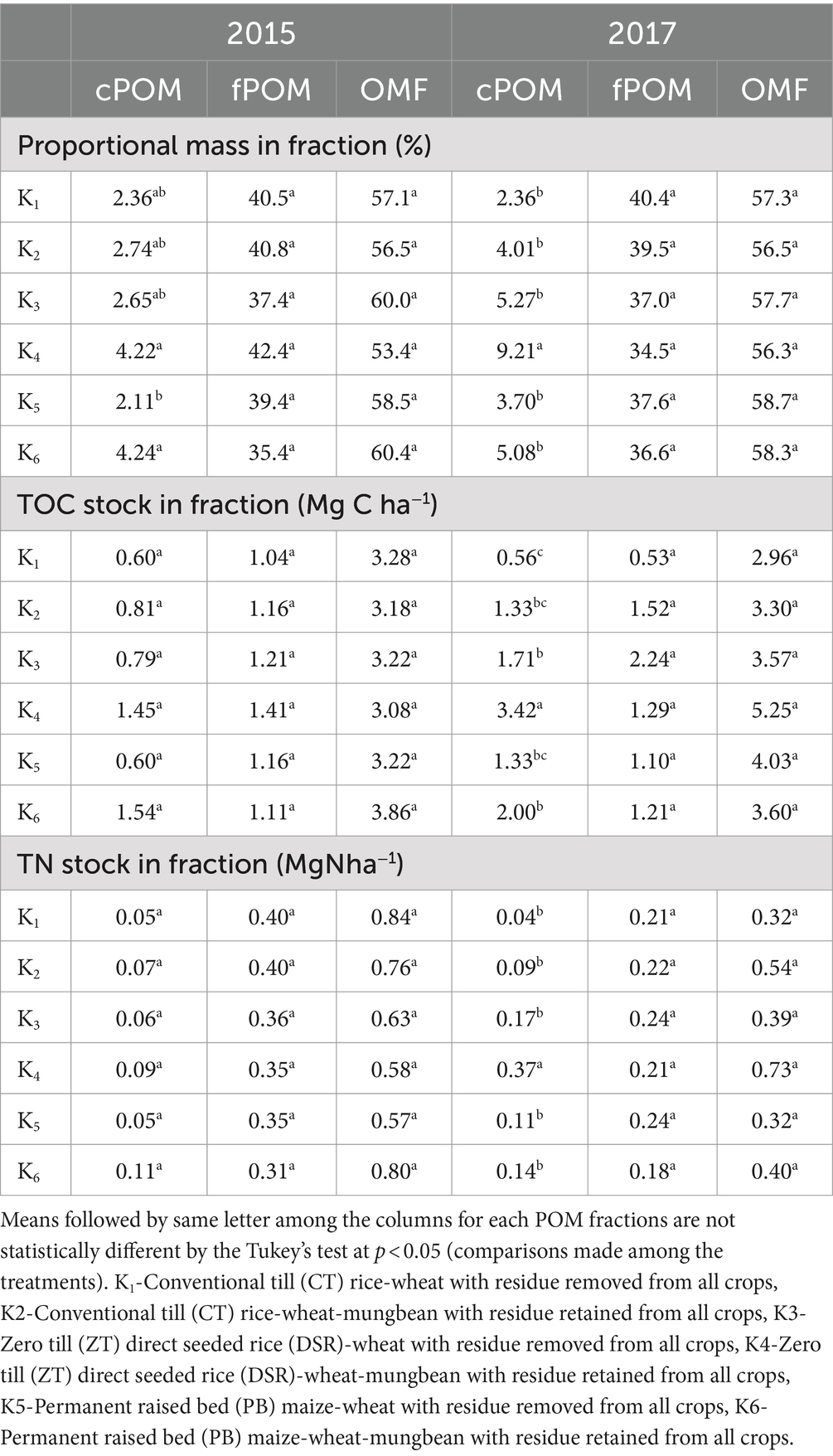
Table 8. Characterization of different particulate fraction under different tillage and residue management in Karnal at 0–5 cm soil depth.
3.4.2 Physical fractionation and associated C and N stock in Samastipur
At 0–5 cm depth, significant differences in mass proportions were observed among treatments, except for fPOM in 2018 (Table 9). CA management significantly increased cPOM mass compared to CT (S1), with S2 showing 3.19 and 2.41 times higher cPOM mass in 2016 and 2018, respectively. TOC and TN stocks in cPOM also significantly differed, with notable increases in S2 in 2018. At 5–15 cm depth, no significant TOC stock differences were found. At 0–15 cm, cPOM mass showed significant variations, with S2 and S4 being the highest compared to S1. In both years, TOC stock in cPOM was highest in S2, significantly outperforming S1 (Supplementary Table S4). fPOM and OMF varied less significantly, except for a notable increase in OMF TOC stock in S2 in 2018.
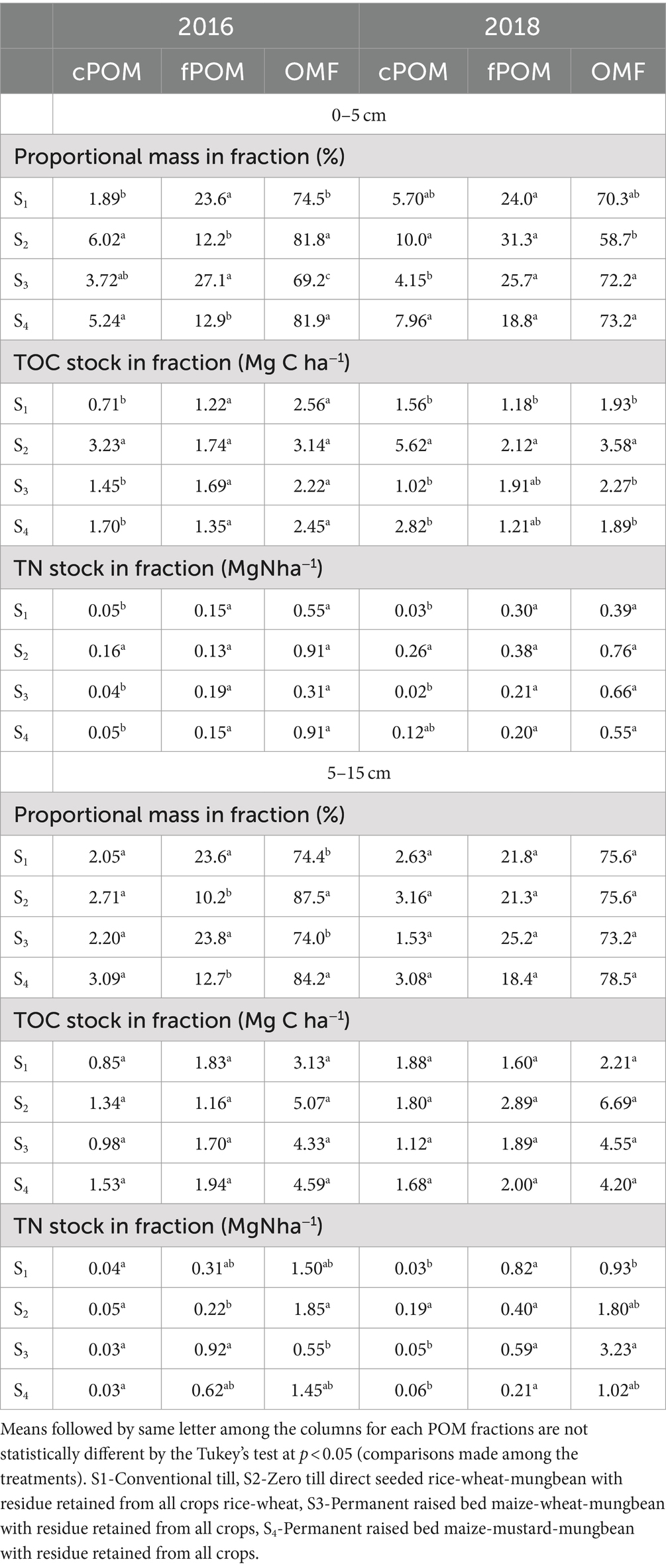
Table 9. Characterization of different particulate fraction under different tillage and residue management in Samastipur.
3.5 The relationship among the factors controlling carbon stock build-up
A Pearson’s correlation matrix indicated varying correlations between TOC stock, POXC stock, carbon input, and POM fractions across depths and times (Table 10). Table 10 shows the comparison of data at the same location. Initially, no significant correlation was found between carbon stock and cPOM fraction after 3 years. However, after 5 years, a notable correlation emerged (r = 0.63 and r = 0.76) at 0–5 cm depth in both sites. In Karnal, a significant correlation was found between soil carbon stock and total carbon input after both 3 and 5 years (r = 0.48 and r = 0.55) at 0–5 cm depth, while in Samastipur, this was observed only after 3 years (r = 0.78). No correlations except for carbon input were noted at 5–15 cm depth. At 0–15 cm depth, significant correlations between carbon stock and cPOM emerged after 5 years in Karnal (r = 0.57), with soil carbon stock significantly associated with total carbon input in both sites over time, except after 3 years in Samastipur.
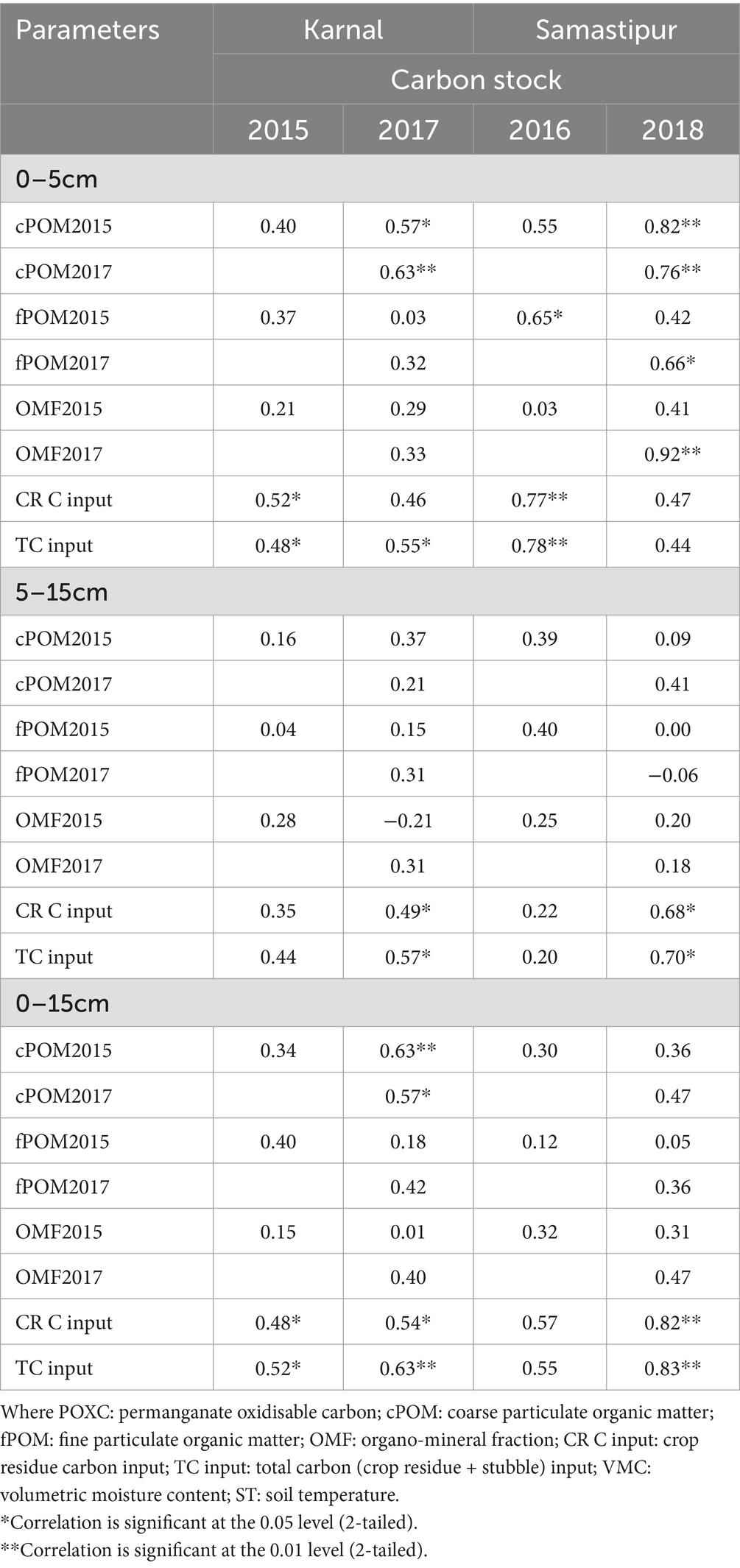
Table 10. Pearson’s correlation coefficients of organic carbon stock with soil fractions and carbon input.
4 Discussion
4.1 Crop grain yield and system yield
Our study highlights the significant influence of tillage and crop establishment methods, residue recycling/incorporation, and legume integration on crop grain yield and system yield (SY), as seen in the substantial SY increase in Karnal for the maize-wheat-mungbean rotation under permanent beds (PB). This finding aligns with the combined effects of yield increments across these crops, improved soil moisture and temperature regimes, enhanced aeration and light availability, and better soil physicochemical properties (Choudhary et al., 2018; Kumar et al., 2018; Choudhury et al., 2014; Parihar et al., 2016; Jat et al., 2014; Nandan et al., 2019; Parihar et al., 2018; Samal et al., 2017). Additionally, the increased nutrient availability and effective recycling of crop residues contribute to an enhanced total organic carbon (TOC) stock, which facilitates better soil aggregation (Blanco-Canqui and Lal, 2007; Jat H. S. et al., 2018; Chaudhary et al., 2022; Mishra et al., 2018; Kumar et al., 2019; Samal et al., 2017). In Samastipur, the treatments S2 (ZT-DSR and ZT-wheat with mungbean) and S4 (PB-maize and PB-mustard with mungbean (MM)) exhibited comparable SY, significantly higher than S3 (PB-maize and PB-wheat with mungbean) and S1 (CT-rice and CT-wheat), highlighting the benefits of conservation agriculture (CA) practices. The higher SY achieved through ZT and PB with the integration of mungbean in RW and MM systems is consistent with previous findings in the IGP region, reinforcing the value of CA systems in sustainable agriculture (Jat et al., 2019; Kumar et al., 2018). The lower SY observed in the poorest-performing treatments (K1 in Karnal and S1 in Samastipur) can be attributed to suboptimal soil and crop management practices, particularly the rapid decomposition of organic matter (Powlson et al., 2014; Singh et al., 2015; Stewart et al., 2007).
4.2 Soil organic carbon pools and stock
Exploring carbon stocking and lability in short-term trials of CA-based management practices over 3 and 5 years is crucial for identifying optimal practices for specific tropical soil sites. In the loamy soils of Karnal and the silty loams of Samastipur, the lability of TOC plays a vital role in carbon sequestration and stabilization. Our analysis of TOC and POXC stocks, particularly under permanent raised bed systems with maize-wheat-mungbean rotations and full residue retention at 0–15 cm soil depth, reveals the beneficial impacts of reduced tillage and enhanced crop residue turnover on carbon stocks (Tables 6–9). CT systems, conversely, may expedite organic matter decomposition through aggregate disruption and increased microbial exposure (Jat M. L. et al., 2018). Notably, after 5 years, K6 in Karnal exhibited the highest carbon stock increase at 72.3% over K1 at 0–5 cm depth, underscoring the efficiency of CA practices in enhancing soil carbon levels (Table 9). Similarly, in Samastipur, S2 and S3 treatments demonstrated significant carbon stock increases after 3 years, with S2 showing the most substantial rise after 5 years (Table 5). This suggests that ZT and PB practices, especially when including crop rotations with legumes like mungbean, significantly contribute to soil organic carbon accumulation, corroborating findings by Govaerts et al. (2007), Meurer et al. (2018), and Parihar et al. (2018). Our results align with the broader literature, indicating that CA practices, particularly those involving diverse crop rotations and residue management, are crucial for enhancing soil carbon sequestration in tropical soils (Awale et al., 2017; Bongiorno et al., 2019; Moharana et al., 2012).
Additionally, the observed increase in total organic carbon (TOC) across all soil layers under conventional management (K1 and S1) over 2 years may be explained by several mechanisms (Awale et al., 2017). Although conventional tillage (CT) typically accelerates organic matter decomposition by disrupting soil aggregates and enhancing microbial activity, it can also lead to short-term increases in TOC, particularly when crop residues are incorporated into the soil or when fertilization promotes greater biomass production (Rahman et al., 2021). In the short-term timeframe of this study, organic inputs from crop residues under CT may temporarily increase TOC before decomposition rates surpass carbon accumulation. Additionally, the lability of TOC, influenced by site-specific soil properties such as the loamy soils of Karnal and silty loams of Samastipur, may contribute to transient carbon sequestration even in conventional systems (Dey et al., 2020). This underscores the need for long-term evaluations to fully assess the impacts of tillage practices on carbon stabilization in tropical soils.
4.3 POM fractionation and C stabilization
The study’s examination of particulate organic matter (POM) fractionation and carbon stabilization across different conservation agriculture (CA) management practices provides compelling evidence of the pivotal role of short-term agronomic strategies in enhancing soil carbon stocks. Specifically, the significant increase in coarse particulate organic matter (cPOM) at the 0–5 cm soil depth within treatments K4 and K6 in Karnal, as observed in both 2015 and 2017, underscores the synergistic effects of reduced tillage and diverse crop residue management on soil structure and carbon dynamics (Table 6). This finding is particularly noteworthy as it highlights how strategic residue management and tillage reduction can lead to enhanced carbon sequestration by promoting the formation of macroaggregates, which serve as protective niches for organic carbon, thereby reducing its susceptibility to microbial decomposition.
In Samastipur, the superior performance of S2 and S4 treatments regarding cPOM accumulation further corroborates the efficacy of integrating legumes such as mungbean into cropping systems. This integration not only diversifies the cropping system but also contributes to a more complex soil organic matter composition through the addition of legume-derived residues, which are known for their rapid decomposition and nutrient release, enhancing soil fertility and structure (Benbi and Senapati, 2010; Parihar et al., 2018). Such practices, indicative of CAB34’s principles, foster a conducive environment for sustainable soil health management by improving the soil’s physical, chemical, and biological properties.
Moreover, the observed correlations between carbon stock and cPOM over time (Table 10) suggest a dynamic and responsive soil system under CA management, with implications for long-term soil health and productivity. The data indicate that as CA practices mature, their impact on soil carbon stocks becomes increasingly pronounced, highlighting the importance of time in assessing the outcomes of agricultural management practices on soil carbon dynamics.
This study’s findings align with and extend the work of Govaerts et al. (2007), Meurer et al. (2018), and others, situating them within the broader discourse on soil carbon sequestration and the sustainability of agricultural systems (Rahman et al., 2021). By demonstrating the potential of CA practices to influence soil carbon stocks in the short term significantly, this research contributes valuable insights into the mechanisms through which agricultural management practices can be optimized to enhance carbon sequestration, soil health, and, ultimately, the sustainability of farming systems. This underscores the critical need for continued research in this area, particularly long-term studies that can further elucidate soil carbon dynamics under different agricultural management practices and their implications for addressing global challenges such as climate change and food security.
4.4 The relationship between different SOC pools and carbon inputs
Pearson’s correlation matrix revealed that soil carbon stock was significantly and positively correlated with cPOM and carbon input from crop residues in both sites, mainly after 5 years of experimentation. The correlation is robust at 0–5 cm and weaker at 0–15 cm soil depth. Such a reduction in correlation might be due to no significant correlation in 5–15 cm soil depth. The results indicate that soil carbon stock build-up is strongly influenced by the continuous flow of crop residues into the system (Choudhury et al., 2018), especially the cPOM fraction. Barreto et al. (2010) have observed that the more significant variation in the labile fraction (cPOM) would be a consistent indicator that would monitor the variation in soil quality under different management practices. However, the period of experimentation is crucial for identifying such changes in CA-based management practices. The results of the present study support earlier findings (Moharana et al., 2012; Somasundaram et al., 2018; Choudhary et al., 2018). Thus, it can be stated by the final analysis that cPOM helps in understanding soil carbon dynamics under different climate regimes.
Additionally, study was done at two site in two different states sharing different agro-climatic zones and ecology, so these practices and findings are applicable to similar regions with similar climatic conditions following rice-wheat cropping systems. Apart from this, study is short term but longer term studies will also be rewarding based on the findings of the study and significance of the practices.
5 Conclusion
This study demonstrates that conservation agriculture (CA) practices, including no-till, residue management, and crop diversification, significantly promote carbon sequestration. However, the outcomes are intricately influenced by the study’s duration, site-specific conditions, and the soil fractions examined. Notably, integrating diversified residue management and including mungbean in crop rotations have emerged as pivotal factors in modulating carbon and nitrogen dynamics within CA-based systems. In Karnal, soil carbon accumulation was primarily supported by a synergistic effect of tillage practices, residue management, and crop diversity. Conversely, in Samastipur, the emphasis on residue recycling influenced the slightly lower observed soil carbon enhancements. Moreover, the variations observed in the total organic carbon (TOC) and total nitrogen (TN) within the coarse particulate organic matter (cPOM) fraction underscore its potential as a critical indicator for assessing the efficacy of short-term CA interventions. The impact of these practices on soil carbon and nitrogen stocks is contingent upon multiple factors, including soil depth, the duration of the CA implementation, soil type, crop rotation patterns, and overall management strategies. It was found that a 3-year period was insufficient to elicit detectable changes in soil carbon and nitrogen fractionation in Karnal. In contrast, in Samastipur, the exact duration was adequate for observing significant alterations in the soil’s physical fractionation. This highlights the necessity for tailored CA practices that consider local soil characteristics and climatic conditions to optimize carbon sequestration and soil health over time.
Data availability statement
The original contributions presented in the study are included in the article/supplementary material, further inquiries can be directed to the corresponding author.
Author contributions
AM: Writing – review & editing, Writing – original draft, Resources, Methodology, Investigation, Formal analysis, Conceptualization. HS: Writing – review & editing, Writing – original draft. HJ: Writing – review & editing, Writing – original draft. MJ: Writing – review & editing, Writing – original draft. RK: Writing – review & editing, Visualization. SF: Writing – review & editing.
Funding
The author(s) declare that no financial support was received for the research, authorship, and/or publication of this article.
Acknowledgments
AM appreciate the MEXT Scholarship for providing funds for a Doctoral degree program at Kyoto University, Kyoto, Japan. The authors wish to acknowledge the funding support from Prof. Shinya Funakawa, Dean, Graduate School of Global Environmental Studies, Kyoto University, Kyoto, Japan, and Kyoto University Foundation, Kyoto, Japan. We appreciate the support from all the farmers, key communicators, and local officials who contributed their knowledge to this study and helped build rapport with the community. We are highly thankful to the International Maize and Wheat Improvement Center (CIMMYT)-CGIAR Research Program on Climate Change, Agriculture and Food Security (CCAFS) and Borlaug Institute for South Asia (BISA) for providing an essential platform in various climate-smart villages of Haryana and Bihar, India and scientific contributions for the study. The authors also thank Deepak Bijarniya and Love K. Singh of CIMMYT India for their support while visiting the sites and data collection.
Conflict of interest
The authors declare that the research was conducted in the absence of any commercial or financial relationships that could be construed as a potential conflict of interest.
Publisher’s note
All claims expressed in this article are solely those of the authors and do not necessarily represent those of their affiliated organizations, or those of the publisher, the editors and the reviewers. Any product that may be evaluated in this article, or claim that may be made by its manufacturer, is not guaranteed or endorsed by the publisher.
Supplementary material
The Supplementary material for this article can be found online at: https://www.frontiersin.org/articles/10.3389/fsufs.2024.1476292/full#supplementary-material
References
Aryal, J. P., Sapkota, T. B., Jat, M. L., and Bishnoi, D. K. (2015). On-farm economic and environmental impact of zero-tillage wheat: a case of north-West India. Exp. Agric. 51, 1–16. doi: 10.1017/S001447971400012X
Awale, R., Emeson, M. A., and Machado, S. (2017). Soil organic carbon pools as early indicators for soil organic matter stock changes under different tillage practices in inland Pacific northwest. Front. Ecol. Evol. 5:96. doi: 10.3389/fevo.2017.00096
Barreto, P. A. B., Gama-Rodrigues, E. F., and Gama-Rodrigues, A. C. (2010). Carbon and nitrogen mineralization in soils under an eucalyptus plantation chronosequence. Revista Brasileira de Ciência do Solo 34, 735–745.
Benbi, D. K., and Senapati, N. (2010). Soil aggregation and carbon and nitrogen stabilization in relation to residue and manure application in rice-wheat systems in northwest India. Nutr. Cycl. Agroecosys. 87, 233–247.
Blake, G. R., and Hartge, K. H. (1986). “Bulk density” in Methods of soil analysis, part 1-physical and mineralogical methods, 2nd edition, agronomy monograph 9. ed. A. Klute (Madison: American Society of Agronomy, Soil Science Society of America), 363–382.
Blanco-Canqui, H., and Lal, R. (2007). Soil structure and organic carbon relationships following 10 years of wheat straw management in no-till. Soil Tillage Res. 95, 240–254. doi: 10.1016/j.still.2007.01.004
Bongiorno, G., Bünemann, E. K., Oguejiofor, C. U., Meier, J., Gort, G., Comans, R., et al. (2019). Sensitivity of labile carbon fractions to tillage and organic matter management and their potential as comprehensive soil quality indicators across pedoclimatic conditions in Europe. Ecol. Indic. 99, 38–50. doi: 10.1016/j.ecolind.2018.12.008
Chaudhari, S. K., Bardhan, G., Kumar, P., Singh, R., Mishra, A. K., Rai, P., et al. (2015). Short-term tillage and residue management impact on physical properties of a reclaimed sodic soil. J. Indian Soc. Soil Sci. 63, 30–38. doi: 10.5958/0974-0228.2015.00005.5
Chaudhary, A., Venkatramanan, V., Kumar Mishra, A., and Sharma, S. (2022). Agronomic and environmental determinants of direct seeded Rice in South Asia. Circ. Econ. Sustain. 3, 253–290. doi: 10.1007/s43615-022-00173-x
Chauhan, B. S., Mahajan, G., Sardana, V., Timsina, J., and Jat, M. L. (2012). Productivity and sustainability of the rice-wheat cropping system in the Indo-Gangetic Plains of the Indian subcontinent: problems, opportunities, and strategies. Adv. Agron. 117, 315–369. doi: 10.1016/B978-0-12-394278-4.00006-4
Choudhary, K. M., Jat, H. S., Nandal, D. P., Bishnoi, D. K., Sutaliya, J. M., Choudhary, M., et al. (2018). Evaluating alternatives to rice-wheat system in western Indo-Gangetic Plains: crop yields, water productivity and economic profitability. F. Crop. Res. 218, 1–10. doi: 10.1016/J.FCR.2017.12.023
Choudhury, S. G., Srivastava, S., Singh, R., Chaudhari, S. K., Sharma, D. K., Singh, S. K., et al. (2014). Tillage and residue management effects on soil aggregation, organic carbon dynamics and yield attribute in rice-wheat cropping system under reclaimed sodic soil. Soil Tillage Res. 136, 76–83. doi: 10.1016/j.still.2013.10.001
Choudhury, S. G., Yaduvanshi, N. P. S., Chaudhari, S. K., Sharma, D. R., Sharma, D. K., Nayak, D. C., et al. (2018). Effect of nutrient management on soil organic carbon sequestration, fertility, and productivity under rice-wheat cropping system in semi-reclaimed sodic soils of North India. Environ. Monit. Assess. 190:117. doi: 10.1007/s10661-018-6486-9
Culman, S. W., Snapp, S. S., Freeman, M. A., Schipanski, M. E., Beniston, J., Lal, R., et al. (2012). Permanganate oxidizable carbon reflects a processed soil fraction that is sensitive to management. Soil Sci. Soc. Am. J. 76, 494–504. doi: 10.2136/sssaj2011.0286
Das, T. K., Bhattacharyya, R., Sharma, A. R., Das, S., Saad, A. A., and Pathak, H. (2013). Impacts of conservation agriculture on total soil organic carbon retention potential under an irrigated agroecosystem of the western Indo-Gangetic Plains. Eur. J. Agron. 51, 34–42. doi: 10.1016/j.eja.2013.07.003
Das, T. K., Saharawat, Y. S., Bhattacharyya, R., Sudhishri, S., Bandyopadhyay, K. K., Sharma, A. R., et al. (2018). Conservation agriculture effects on crop and water productivity, profitability and soil organic carbon accumulation under a maize-wheat cropping system in the North-Western Indo-Gangetic Plains. F. Crop. Res. 215, 222–231. doi: 10.1016/j.fcr.2017.10.021
De Oliveira Ferreira, A., Amado, T. J. C., Rice, C. W., Ruiz Diaz, D. A., Briedis, C., Inagaki, T. M., et al. (2018). Driving factors of soil carbon accumulation in Oxisols in long-term no-till systems of South Brazil. Sci. Total Environ. 622-623, 735–742. doi: 10.1016/j.scitotenv.2017.12.019
Dey, A., Dwivedi, B. S., Bhattacharyya, R., Datta, S. P., Meena, M. C., Jat, R. K., et al. (2020). Effect of conservation agriculture on soil organic and inorganic carbon sequestration, and their lability: a study from a rice–wheat cropping system on a calcareous soil of eastern Indo-Gangetic Plains. Soil Use Manag. 36, 429–438. doi: 10.1111/sum.12577
Franzluebbers, A. J. (2010). Achieving soil organic carbon sequestration with conservation agricultural systems in the Southeastern United States. Soil Sci. Soc. Am. J. 74, 347–357. doi: 10.2136/sssaj2009.0079
Ganeshamurthy, A. N., and Srinivasarao, C. (2009). Assessment of field indicators of soil quality following long-term cultivation of pulses on Entisols in Indo Gangatic plain. J. Indian Soc. Soil Sci. 57, 76–80.
Gathala, M. K., Kumar, V., Sharma, P. C., Saharawat, Y. S., Jat, H. S., Singh, M., et al. (2014). Reprint of "optimizing intensive cereal-based cropping systems addressing current and future drivers of agricultural change in the Northwestern Indo-Gangetic Plains of India.". Agric. Ecosyst. Environ. 187, 33–46. doi: 10.1016/j.agee.2013.12.011
Ghimire, R., Lamichhane, S., Acharya, B. S., Bista, P., and Sainju, U. M. (2017). Tillage, crop residue, and nutrient management effects on soil organic carbon in rice-based cropping systems: a review. J. Integr. Agric. 16, 1–15. doi: 10.1016/S2095-3119(16)61337-0
Govaerts, B., Mezzalama, M., Unno, Y., Sayre, K. D., Luna-Guido, M., Vanherck, K., et al. (2007). Influence of tillage, residue management, and crop rotation on soil microbial biomass and catabolic diversity. Appl. Soil Ecol. 37, 18–30. doi: 10.1016/j.apsoil.2007.03.006
Govaerts, B., Sayre, K.D., Goudeseune, B., Corte, P.De, Lichter, K., Dendooven, L., et al. (2009). Conservation agriculture as a sustainable option for the central Mexican highlands. Soil Tillage Res. 103, 222–230. doi: 10.1016/j.still.2008.05.018
Guo, L. B., and Gifford, R. M. (2002). Soil carbon stocks and land use change: a meta-analysis. Glob. Chang. Biol. 8, 345–360. doi: 10.1046/j.1354-1013.2002.00486.x
Hazra, K. K., Ghosh, P. K., Venkatesh, M. S., Nath, C. P., Kumar, N., Singh, M., et al. (2018). Enzymes and C pools as indicators of C build up in short-term conservation agriculture in a savanna ecosystem in Cambodia. Soil Tillage Res. 177, 125–133. doi: 10.1016/J.STILL.2017.11.015
Hooper, D. U., Chapin, F., Stuart, I., Hector, A., Inchausti, P., Lavorel, S., et al. (2005). Effects of biodiversity on ecosystem functioning: a consensus of current knowledge. Ecol. Monogr. 75, 3–35. doi: 10.1890/04-0922
Jat, H. S., Datta, A., Sharma, P. C., Kumar, V., Yadav, A. K., Choudhary, V., et al. (2018). Assessing soil properties and nutrient availability under conservation agriculture practices in a reclaimed sodic soil in cereal-based systems of North-West India. Arch. Agron. Soil Sci. 64, 531–545. doi: 10.1080/03650340.2017.1359415
Jat, R. K., Sapkota, T. B., Singh, R. G., Jat, M. L., Kumar, M., and Gupta, R. K. (2014). Seven years of conservation agriculture in a rice-wheat rotation of Eastern Gangetic Plains of South Asia: yield trends and economic profitability. F. Crop. Res. 164, 199–210. doi: 10.1016/j.fcr.2014.04.015
Jat, R. K., Singh, R. G., Kumar, M., Jat, M. L., Parihar, C. M., Bijarniya, D., et al. (2019). Ten years of conservation agriculture in a rice – maize rotation of Eastern Gangetic Plains of India: yield trends, water productivity and economic profitability. F. Crop. Res. 232, 1–10. doi: 10.1016/j.fcr.2018.12.004
Jat, M. L., Singh, B., Stirling, C. M., Jat, H. S., Tetarwal, J. P., Jat, R. K., et al. (2018). Soil processes and wheat cropping under emerging climate change scenarios in South Asia. Adv. Agron. 148, 111–171. doi: 10.1016/BS.AGRON.2017.11.006
Jha, P., Lakaria, B. L., Biswas, A. K., Saha, R., Mahapatra, P., Agrawal, B. K., et al. (2014). Effects of carbon input on soil carbon stability and nitrogen dynamics. Agric. Ecosyst. Environ. 189, 36–42. doi: 10.1016/j.agee.2014.03.019
Kaur, M., Malik, D. P., Malhi, G. S., Sardana, V., Bolan, N. S., Lal, R., et al. (2022). Rice residue management in the indo-Gangetic Plains for climate and food security. A review. Agron. Sustain. Dev. 42:92. doi: 10.1007/s13593-022-00817-0
Koutika, L. S., Tchichelle, S. V., Mareschal, L., and Epron, D. (2017). Nitrogen dynamics in a nutrient-poor soil under mixed-species plantations of eucalypts and acacias. Soil Biol. Biochem. 108, 84–90. doi: 10.1016/j.soilbio.2017.01.023
Kumar, V., Jat, H. S., Sharma, P. C., Balwinder-Singh, G., Gathala, M. K., Malik, R. K., et al. (2018). Can productivity and profitability be enhanced in intensively managed cereal systems while reducing the environmental footprint of production? Assessing sustainable intensification options in the breadbasket of India. Agric. Ecosyst. Environ. 252, 132–147. doi: 10.1016/j.agee.2017.10.006
Kumar, P., Mishra, A. K., Chaudhari, S., Singh, R., Yadav, K., Rai, P., et al. (2022). Conservation agriculture influences crop yield, soil carbon content and nutrient availability in rice-wheat system of Northwest India. Soil Res. 60, 624–635. doi: 10.1071/SR21121
Kumar, N., Nath, C. P., Hazra, K. K., Das, K., Venkatesh, M. S., Singh, M. K., et al. (2019). Soil & Tillage Research Impact of zero-till residue management and crop diversification with legumes on soil aggregation and carbon sequestration. Soil Tillage Res. 189, 158–167. doi: 10.1016/j.still.2019.02.001
Lal, R. (2020). Managing soils for resolving the conflict between agriculture and nature: the hard talk. Eur. J. Soil Sci. 71, 1–9. doi: 10.1111/ejss.12857
Meurer, K. H. E., Haddaway, N. R., Bolinder, M. A., and Kätterer, T. (2018). Tillage intensity affects total SOC stocks in boreo-temperate regions only in the topsoil—a systematic review using an ESM approach. Earth-Sci. Rev. 177, 613–622. doi: 10.1016/j.earscirev.2017.12.015
Mishra, A. K., Mahinda, A. J., Shinjo, H., Jat, M. L., Singh, A., and Funakawa, S. (2018). “Role of conservation agriculture in mitigating soil salinity in Indo-Gangetic Plains of India” in Engineering practices for Management of Soil Salinity. eds. S. K. Gupta, M. R. Goyal, and A. Singh (Taylor & Francis: Apple Academic Press Inc.), 87–114.
Mishra, A.K., Shinjo, H., Jat, H.S., Jat, M.L., Jat, R.K., and Funakawa, S., (2016). “Farmer's perspective on adaptation and up-scaling of conservation agriculture-based management practices in indo-Gangetic plains of India.” in Int Conf Cons Agri Sustain Land Use. Szarka, Laszlo Csaba (Hungarian Academy of Sciences), Budapest, Hungary. pp. 40–46.
Moharana, P. C., Sharma, B. M., Biswas, D. R., Dwivedi, B. S., and Singh, R. V. (2012). Long-term effect of nutrient management on soil fertility and soil organic carbon pools under a 6-year-old pearl millet-wheat cropping system in an Inceptisol of subtropical India. F. Crop. Res. 136, 32–41. doi: 10.1016/j.fcr.2012.07.002
Nandan, R., Singh, V., Shankar, S., Kumar, V., Krishna, K., Prasad, C., et al. (2019). Geoderma impact of conservation tillage in rice – based cropping systems on soil aggregation, carbon pools and nutrients. Geoderma 340, 104–114. doi: 10.1016/j.geoderma.2019.01.001
Parihar, C. M., Parihar, M. D., Sapkota, T. B., Nanwal, R. K., Singh, A. K., Jat, S. L., et al. (2018). Long-term impact of conservation agriculture and diversified maize rotations on carbon pools and stocks, mineral nitrogen fractions and nitrous oxide fluxes in inceptisol of India. Sci. Total Environ. 640-641, 1382–1392. doi: 10.1016/J.SCITOTENV.2018.05.405
Parihar, C. M., Yadav, M. R., Jat, S. L., Singh, A. K., Kumar, B., Pradhan, S., et al. (2016). Long-term effect of conservation agriculture in maize rotations on total organic carbon, physical and biological properties of a sandy loam soil in north-western indo-Gangetic Plains. Soil Tillage Res. 161, 116–128. doi: 10.1016/j.still.2016.04.001
Powlson, D. S., Stirling, C. M., Jat, M. L., Gerard, B. G., Palm, C. A., Sanchez, P. A., et al. (2014). Limited potential of no-till agriculture for climate change mitigation. Nat. Clim. Chang. 4, 678–683. doi: 10.1038/nclimate2292
Prasad, J. V. N. S., Rao, C. S., Srinivas, K., Jyothi, C. N., Venkateswarlu, B., Ramachandrappa, B. K., et al. (2016). Effect of ten years of reduced tillage and recycling of organic matter on crop yields, soil organic carbon and its fractions in Alfisols of semi-arid tropics of southern India. Soil Tillage Res. 156, 131–139. doi: 10.1016/j.still.2015.10.013
Rahman, M. M., Aravindakshan, S., Hoque, M. A., Rahman, M. A., Gulandaz, M. A., Rahman, J., et al. (2021). Conservation tillage (CT) for climate-smart sustainable intensification: assessing the impact of CT on soil organic carbon accumulation, greenhouse gas emission and water footprint of wheat cultivation in Bangladesh. Environ. Sustain. Indicat. 10:100106. doi: 10.1016/j.indic.2021.100106
Rumpel, C., Amiraslani, F., Koutika, L. S., Smith, P., Whitehead, D., and Wollenberg, E. (2018). Put more carbon in soils to meet Paris climate pledges. Nature 564, 32–34. doi: 10.1038/d41586-018-07587-4
Samal, S. K., Rao, K. K., Poonia, S. P., Kumar, R., Mishra, J. S., Prakash, V., et al. (2017). Evaluation of long-term conservation agriculture and crop intensification in rice-wheat rotation of Indo-Gangetic Plains of South Asia: carbon dynamics and productivity. Eur. J. Agron. 90, 198–208. doi: 10.1016/j.eja.2017.08.006
Sharma, S., Singh, P., and Kumar, S. (2020). Responses of soil carbon pools, enzymatic activity, and crop yields to nitrogen and straw incorporation in a Rice-wheat cropping system in North-Western India. Front. Sustain. Food Syst. 4:532704. doi: 10.3389/fsufs.2020.532704
Singh, P., Heikkinen, J., Ketoja, E., Nuutinen, V., Palojärvi, A., Sheehy, J., et al. (2015). Tillage and crop residue management methods had minor effects on the stock and stabilization of topsoil carbon in a 30-year field experiment. Sci. Total Environ. 518-519, 337–344. doi: 10.1016/j.scitotenv.2015.03.027
Somasundaram, J., Chaudhary, R. S., Awanish Kumar, D., Biswas, A. K., Sinha, N. K., Mohanty, M., et al. (2018). Effect of contrasting tillage and cropping systems on soil aggregation, carbon pools and aggregate-associated carbon in rainfed Vertisols. Eur. J. Soil Sci. 69, 879–891. doi: 10.1111/ejss.12692
Stewart, C. E., Paustian, K., Conant, R. T., Plante, A. F., and Six, J. (2007). Soil carbon saturation: concept, evidence and evaluation. Biogeochemistry 86, 19–31. doi: 10.1007/s10533-007-9140-0
Tian, L., and Shi, W. (2014). Soil peroxidase regulates organic matter decomposition through improving the accessibility of reducing sugars and amino acids. Biol. Fertil. Soils 50, 785–794. doi: 10.1007/s00374-014-0903-1
Tyurin, I.V. (1966). metodike analiza deje sravniteľnogo izučenija sostava počvennogo peregnoja ili gumusa. In: Voprosy genezisa i plodorodija počv. Nauka, Moskva.
Weil, R. R., Islam, K. R., Stine, M. A., Gruver, J. B., and Samson-Liebig, S. E. (2003). Estimating active carbon for soil quality assessment: a simplified method for laboratory and field use. Am. J. Altern. Agric. 18, 3–17. doi: 10.1079/ajaa2003003
West, T. O., and Post, W. M. (2002). Soil organic carbon sequestration rates by tillage and crop rotation: a global data analysis. Soil Sci. Soc. Am. J. 66, 1930–1946. doi: 10.2136/sssaj2002.1930
Keywords: crop residue recycling, particulate organic matter, physical fractionation, permanent raised bed, resource conservation practices, system productivity
Citation: Mishra AK, Shinjo H, Jat HS, Jat ML, Kumar Jat R and Funakawa S (2024) Conservation agriculture enhances crop productivity and soil carbon fractions in Indo-Gangetic Plains of India. Front. Sustain. Food Syst. 8:1476292. doi: 10.3389/fsufs.2024.1476292
Edited by:
Johann G. Zaller, University of Natural Resources and Life Sciences Vienna, AustriaReviewed by:
Carlos A. Alexandre, University of Evora, PortugalVesna Vasić, Educons University, Serbia
Copyright © 2024 Mishra, Shinjo, Jat, Jat, Kumar Jat and Funakawa. This is an open-access article distributed under the terms of the Creative Commons Attribution License (CC BY). The use, distribution or reproduction in other forums is permitted, provided the original author(s) and the copyright owner(s) are credited and that the original publication in this journal is cited, in accordance with accepted academic practice. No use, distribution or reproduction is permitted which does not comply with these terms.
*Correspondence: Ajay Kumar Mishra, akm8cest@gmail.com; a.k.mishra@irri.org