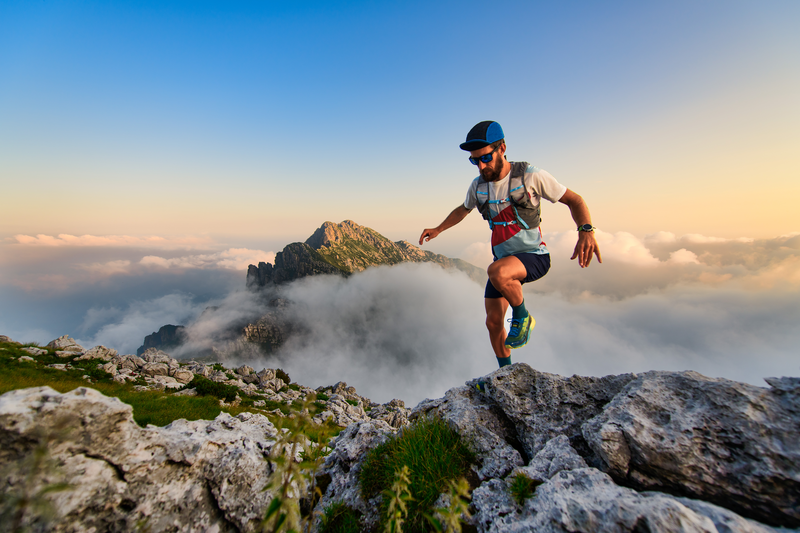
94% of researchers rate our articles as excellent or good
Learn more about the work of our research integrity team to safeguard the quality of each article we publish.
Find out more
ORIGINAL RESEARCH article
Front. Sustain. Food Syst. , 15 January 2025
Sec. Nutrition and Sustainable Diets
Volume 8 - 2024 | https://doi.org/10.3389/fsufs.2024.1476134
This article is part of the Research Topic Trends on Valorization of Agri-Food Waste Through Green Technologies View all articles
The development of peptides derived from plants, which have potential anti-angiotensin converting enzyme (ACE) activity and other bioactivities, are of scientific interest. ACE inhibitory peptide (CLPP, the ACE inhibitory rate is 76.52 ± 1.07%) was obtained by hydrolysis of tiger nut (Cyperus esculentus) protein with alkaline protease. The plastein product (PCLPP, the ACE inhibitory rate is 85 ± 2.33%) was prepared by modifying CLPP with a plastein reaction. The results of SEM, IR, and XRD show that the structure of PCLPP is different from that of CLPP. The results of differential scanning calorimetry, fluorescence, and free amino analysis showed that the reaction was carried out by aggregation and condensation. These results suggest that the plastein reaction may be an effective method to increase the variety of bioactive peptides.
Cardiovascular diseases are the number one cause of death around the world, and the rise of cardiovascular diseases is strongly associated with the increased value of blood pressure. Inhibition of angiotensin I-converting enzyme (ACE) was deemed as an effective method in the treatment of hypertension (Han et al., 2023; Wang et al., 2008). Therefore, there is an urgent need for studying the inhibition of ACE to prevent and control hypertension.
At present, chemically synthetic ACE inhibitors, such as captopril, enalapril, and lisinopril, are widely used as anti-hypertensive drugs in clinical applications (Taskiran and Avci, 2021; Yasmin and Joerg, 2018). However, they have been reported to lead to certain side effects, including changes in taste, coughs, and rashes (Ye et al., 2022; Suo et al., 2022). Thus, it is important to find new and safe natural substitutes to lower the application of synthetic drugs. ACE-inhibitory peptides, which are safer than synthetic ACE inhibitors, are as effective antihypertensive drugs (Yu et al., 2006; Kaori et al., 2009). The methods for the preparation of ACE inhibitory peptide included enzymatic production, chemical synthesis, extraction of natural active peptide, and recombination technology. Recently, food-derived bioactive peptides have been revealed to serve a significant role in decreasing blood pressure because of their advantage of high safety, specificity, and no side effects (Xu et al., 2021; Zhao and Li, 2009). Therefore, there is an increasing interest in ACE-inhibitory peptides. In particular, the production and characterization of ACE inhibitory peptides from food sources is an emerging area of biochemical research.
Cyperus esculentus belongs to the perennial herb mainly distributed in tropical and temperate regions. Its underground tubers (tiger nuts) are rich in nutrients, including 25–30% of oil, and the quality of extracted oil is excellent (Mohammed et al., 2022). Tiger nuts are consumed throughout China in dairy, bakery, candy, and cooking oil products. The tiger nuts dregs take the most parts of the by-products of oil processing, which contain abundant valuable proteins that could be the precursor of some functional peptides by the means of enzymatic hydrolysis, which contained abundant hydrophobic amino acids. In addition, it has been found that the tiger nut peptides can help to improve blood circulation, reduce cardiovascular disease and heart disease, and prevent stroke and respiratory inflammation (Sánchez-Zapata et al., 2012; Parker et al., 2000; Chinma et al., 2010). Therefore, the tiger nut is a particularly good source of bioactive peptides and is rich in proteins that result in high yields of peptides. It has previously been revealed that peptides rich in hydrophobic residues demonstrated a greater ACE inhibition and proteins that contained abundant hydrophobic amino acids were able to produce ACE inhibitory peptides (Yuka et al., 2008). Thus, it was suggested that the tiger nut proteins may be a promising candidate for producing ACE inhibitory peptides.
Recently, it has been reported that the plastein reaction can enhance the activity of ACE inhibitory peptides (Zhao and Li, 2009; Jiang et al., 2018; Wei et al., 2013). This reaction can also bring about bioactivity changes in protein hydrolysates related to human health promotion. Therefore, the plastein reaction has been used widely for some purposes, including the modification of some protein hydrolysates to improve their antioxidant properties or ACE-inhibitory activity. Plastein reactions were first discovered by Danilevski and Okuneffin in 1902, when the addition of rennet to a protein hydrolysate produced a precipitate that was thought to be an inverse enzymatic reaction (Zhou et al., 2024). The plastein reaction contained a process in which a high substrate concentration (20–50%, w/v) of protein hydrolysate or polypeptide was catalyzed by peptide bonding mode in the presence of appropriate proteases under optimum conditions to form an insoluble, high molecular weight, aggregated macromolecular structure, plastein precipitate. More importantly, the reaction can modify the amino acid sequences and spatial structures of proteins or peptides, and thus change the nutritional values of proteins via enforcing the restrictive amino acids or removing the unwished amino acids from the specific products (Gao and Zhao, 2012). Meanwhile, the reaction can also affect several properties of proteins, including surface hydrophobicity, thermal stability, and so on. Some researchers believe that transpeptide is the main mechanism to maintain the reaction, and new covalent bonds are formed during the reaction. On the other hand, many have suggested that hydrophobic interactions are the main force forming the plastein reaction (Jiang et al., 2018; Zhang and Zhao, 2014).
During the formation of plastein products, the sequence changes caused by peptide modifications induced by proteases make their products have many potential applications. Hazelnut plastein peptides inhibit ACE activity (anti-hypertensive) (Song et al., 2023). Adding exogenous glutamic acid to prepare efficient zinc-binding peptides from modified hydrolysates of oysters (Crassostrea gigas) promotes intestinal absorption of zinc (Li et al., 2019). Gastric protease catalyzes the conversion to tryptophan, which enhances the inhibitory activity of lactoferrin hydrolysate on cancer cell BGC−823 in vitro (Ma et al., 2019). The plastein reaction enhances the bile acid binding ability of soy protein hydrolysate and whey protein hydrolysate (Qian et al., 2018), and the aggregation of hydrophobic residues during peptide aggregation makes it possible for class proteins to be used as peptide de bitters and bile acid chelating agents, which may help reduce endogenous cholesterol.
This study aimed to determine the effect of the plastein reaction on The ACE inhibitory peptide and to elucidate the possible chemical mechanism of the plastein reaction. In the present study, the ACE inhibitory activity, structural changes, and thermal stability of plastein reaction-modified PCLPP were determined, and as well as the possible interaction involved in the plastein reaction was also explored.
Tiger nut proteins were obtained according to the described methods (Gao and Zhao, 2012). Tiger nut powder was mixed with water in a ratio of 1:10, pH was adjusted to 11, stirred for 60 min, and centrifuged (20 min, 4,000 g). The supernatant was used to remove the upper layer of oil using a separatory funnel, then the pH of the aqueous layer was adjusted to the isoelectric point and left to stand overnight. Then centrifuged (20 min, 4,000 g), the precipitate was washed three times with water, the pH was adjusted to neutral, lyophilized, and stored to obtain tiger nut protein.
The tiger nut protein was mixed with distilled water at a concentration of 2%, the pH was adjusted to 11 with 1 mol/L NaOH, and then 6,000 U/g alkaline protease (Melun Biotechnology Co., LTD) was added to initiate enzymatic hydrolysis. After hydrolysis in a water bath at 57°C for 3 h, the reaction was terminated by heating at 90°C for 15 min. The reaction mixture was centrifuged at 10,000 g for 20 min at 4°C to remove the precipitate. The supernatant was freeze-dried to obtain CLPP, which was stored in a well-sealed self-sealing bag in a desiccator for subsequent analysis.
CLPP was dissolved in distilled water to form a solution with a mass fraction of 40%, and then trypsin (2,500 U/g) (Meilun Biotechnology Co., Ltd.) was added, and heated at 45°C for 1 h to cause a plastein reaction, and then heated at 100°C for 5 min to terminate the reaction, and then the solution was freeze-dried to obtain the plastein product PCLPP.
The structure of the sample was analyzed using a Fourier-transform infrared (FT-IR) spectrometer (VERTEX70, Bruker, Germany). For each measurement, 32 scans were coded with a resolution of 4 cm−1 and a wave number ranging from 500 to 4,000 cm−1 (Doucet et al., 2014).
The structure was evaluated via Scanning Electron Microscopy (SEM). Before the investigation, good conductivity of the samples was achieved with a thin layer of gold sputter-coated using a sputter coater (Quorum, Q150V ES) (Li et al., 2020).
The crystalline structure of samples was evaluated by X-ray diffraction (D8 Advance, Bruker, Germany) in the 2θ range 5°-80°, using the Cu Kα radiation and operating at 45 KV with 30 mA current (Sun et al., 2021).
The sample was dissolved in phosphate buffer solution (0.01 mol/L, pH 7.0) at a concentration of 2 mg/mL, 8,000 r/min, centrifuged for 30 min, and the supernatant was taken for determination. The excitation wavelength of the fluorescence spectrum is 290 nm, the range of the scanning emission spectrum is 300–400 nm, and the width of the excitation and emission slit is 5 nm (Spanneberg et al., 2010).
ANS was used as a probe to measure the hydrophobicity change in the process of plastein reaction by fluorescence method (Zhang et al., 2018). The samples were analyzed with excitation and emission wavelengths of 390 and 470 nm, respectively. Surface hydrophobicity was determined as the initial slope (S0) of the fluorescence intensity vs. concentration (%) plot. The sample was dissolved in phosphate buffer solution (0.01 mol/L, pH = 7.0) with a final concentration of 2 mg/mL. Then the hydrolyzed solution was separated by centrifugation at 10,000 g for 30 min and then the supernatant was determined. The absorbance of the sample was measured at 290 nm. The excitation and emission slit widths are both at 5 nm.
The free amino group (–NH2) contents of ACE inhibitory peptides and its modified products were determined using the o-phthaladehyde (OPA) assay (Doucet et al., 2003). The OPA solution was prepared by dissolving 2.0 g of sodium dodecyl sulfate in 30 mL of 0.4 mol/L sodium borate buffer (pH 9.5). Then, 200 μL β-mercaptoethanol, 80 mg OPA in 1 mL ethanol (ETOH), and borate buffer were mixed to obtain a final solution volume of 100 mL. A volume of 3 mL OPA reagent was mixed with 3 mL sample solution and kept for 2 min before measuring the absorbance at 340 nm (Gao and Zhao, 2012).
The thermal properties of the samples were analyzed by DSC (Q2000, Ta Instruments, USA) under a continuous N2 gas flow with a flow rate of 20 mL/min. The sample (3 mg) was weighed and placed on the DSC cell for analysis. A blank aluminum crucible was used as a control. Each analyzed sample was heated to a range of 40–160 with a flow rate of dry nitrogen set to 20 mL/min and a heating rate of 10°C/min (Zheng et al., 2022).
To determine the effect of sealing amino termini on the yield of plastein products (Jiang et al., 2018). The freeze-dried antihypertensive peptide (5 g) was dissolved in 0.2 mol/L phosphate buffer solution (pH 7.5). After adding 1.2 mL of acetic anhydride, the volume was increased to 12.5 mL and the reaction was carried out at 20°C for 48 h. Then, 1 g of solid ammonium acetate was added and the mixture was titrated to pH 6.0 with 0.5 mol/L HCl. Furtherly, alkaline protease was added to initiate the plastein reaction as described above. At last, the protein was precipitated by absolute ethanol. The precipitate was then freeze-dried and weighed to assess the amount of amino block to the proteinoid response.
The effect of blocking the carboxyl terminus on the yield of the plastein product was also examined. The lyophilized antihypertensive peptide (5 g) was mixed with 10 mL methanol (4°C) and 0.75 mL HCl (12 mol/L) at 25°C by stirring for 48 h. After the reaction was completed, the methanol and HCl were removed using a rotary vaporizer at 35°C. Then, the alkaline protease was added to begin the plastein reaction. When the reaction was finished, 10 mL of absolute ethanol was added to precipitate the proteins and ended the reaction. The precipitate was collected and freeze-dried to measure the amount of carboxyl block to the plastein reaction.
The yield of plastein product after blocking the amino or carboxyl terminus was calculated as follows:
5 mol/l HHL (sigma chemical co) solution 100 μl (ph = 8.3, containing 0.3 mol/l nacl) mixed with 20 μl CLPP or PCLPP, preheated at 37°C for 5 min, reacted with 20 μl ACE solution (0.1 μ/ml) for 60 min at the same temperature. the reaction was terminated by the addition of 1.0 m HCl (100 μl). the absorbance of hippuric acid in the incubated solution was determined at 228 nm using a UV-spectrophotometer. the absorbance value of the extracted hippuric acid was measured at 228 nm (Gao and Zhao, 2012).
Where Ab is the absorbance value of the sample tube with buffer added; As is the absorbance value of the sample tube with inhibitor; Ac is the absorbance value of the sample tube of inactivating enzyme before reaction.
All experiments were repeated in triplicate independently. The representative data were manifested by mean ± standard (SD). One-way analysis of variance (ANOVA) was used to analyze the differences between groups. A p-value of < 0.05 was considered a statistical significance.
Infrared spectroscopy was used as an effective tool for analyzing the structure of peptides and proteins. As shown in Figure 1, the characteristic peaks of CLPP and PCLPP at 3,408 cm−1 (N-H stretching vibration characteristic absorption peak) and 3,070 cm−1 (-CONH- characteristic absorption peak) indicate that they have both protein and polypeptide properties. The spectra both show broad and strong absorption peaks around 3,400 cm−1, indicating that many intramolecular or intermolecular hydrogen bonds may be present in the polymers of CLPP and PCLPP protein multimers (Gong et al., 2025). The absorption peaks of 2,923 and 2,808 cm−1 represent the C-H stretching vibration of -CH2 and -CH3. Compared with CLPP, the C=O stretching vibration peak of PCLPP shifted to 1,610 cm−1, with a spectral range of 1,700–1,500 cm−1 for amide I and II bands. The fingerprint region of 1,350–400 cm−1 shows the main difference between CLPP and PCLPP. The spectra of PCLPP show many new small absorption peaks, which indicate that the plastein reaction produces some new secondary structures (Doucet et al., 2014).
The surface morphology of CLPP and PCLPP was observed under a scanning electron microscope at a magnification of 5,000 times as shown in Figure 2. It can be seen that the substrate CLPP is granular (Figure 2A). However, the product PCLPP appeared to exhibit a relatively smooth structure with a combination of blocky and lamellar surfaces and appeared to be more porous than CLPP, a morphology that is typical of the plastein reaction product (Figure 2B), which is distinctly different from that of the substrate CLPP. The formation of the blocky structure of PCLPP can be attributed to the aggregation of peptides in the plastein reaction (Zhou et al., 2024). Furthermore, these dense and non-porous structures contribute to the increased surface hydrophobicity observed in the plastein product PCLPP.
Figure 2. SEM images of the surface structure of (A) Cyperus esculentus hydrolysates (CLPP) and (B) its modified products after plastein reaction (PCLPP). (B) Scale bars = 10.0 μm.
XRD analysis was used to study the formation of plastein reaction products. The spectra of CLPP and PCLPP are shown in Figure 3. As can be seen from the figure, CLPP shows a strong characteristic crystalline peak near 19.3°; PCLPP is weaker compared to the peak at this position, but shows a weak diffraction peak at 2θ = 8.9°, which is the diffuse scattering caused by the multiple structural layers of the polypeptide (Qu et al., 2022). These variations in the XRD spectra confirm the formation of a new crystal structure (Sun et al., 2021).
Differential scanning calorimetry (DSC) is an effective tool for studying the structural and thermodynamic properties of substances and can be used to determine the denaturation of proteins and changes in structural organization. As shown in Figure 4, the exothermic peak of PCLPP was 108.8°C, which was slightly higher than that of CLPP (104.25°C). After the plastein reaction, the enthalpy change (ΔH) of PCLPP decreased from 40.95 to 27.09 J/g, which indicated that the plastein reaction significantly improved the thermal stability of PCLPP. The Differential Scanning Calorimetry (DSC) results showed only broad peaks indicating the presence of a heterogeneous reaction mixture. This result is in complete agreement with the conclusion that the plastein reaction is formed via the protein aggregation pathway (Zheng et al., 2022).
Aromatic amino acids in proteins such as L-phenylalanine, tyrosine, and tryptophan are fluorescent substances, which exhibit fluorescent properties when excited by excitation light (Xu et al., 2016), so fluorescence spectroscopy can be used to study the environment of aromatic amino acids. As can be seen in Figure 5, the fluorescence intensity of PCLPP is significantly higher than that of the substrate CLPP, and the maximum fluorescence emission wave of PCLPP undergoes a blue shift of 7 nm, indicating that when the plastein reaction occurs, due to the action of trypsin, the CLPP protein unfolds, and tryptophan residues and other residues are exposed on the surface, and the fluorescence intensity increases (Spanneberg et al., 2010). However, the exposure of hydrophobic groups enhances intermolecular interactions, leading to molecular reaggregation, whereupon new products are generated. This echoes the results of surface hydrophobicity, which are shown in Section 3.6.
Surface hydrophobicity is an important indicator of protein denaturation, reflecting the degree of exposure of amino acid residues within a protein molecule in a polar environment (Xie et al., 2022). To investigate the effect of hydrophobicity on the plastein reaction, the change in hydrophobicity during the reaction was measured. The results (Figure 6) confirmed that the surface hydrophobicity gradually increased during the plastein reaction. This may be due to the role of enzymes in the reaction, which expose hydrophobic groups that then interact to form polymers. As the reaction time increased, the hydrophobicity index did not continue to increase when the potential hydrophobic groups were exposed to a maximum extent, indicating that the reaction reached an equilibrium. Thus, hydrophobic interactions are one of the main forces in the formation of the plastein reaction, which is consistent with a related report (Sun et al., 2021).
The reduction in the content of –NH2 is an important feature of the plastein reaction (Sun et al., 2021). In the present study, the changes in free amino groups during the reaction are shown in Figure 7. The results showed that the content of free amino acids decreased, indicating that peptide condensation occurred. Moreover, the decrease of free amino acids fluctuated between 45 and 55 μmol/L when the reaction time exceeded 4 h, which suggested the reaction may have reached a dynamic equilibrium. This might be due to the concentration of the substrate involved in the reaction being relatively high, the synthesis reaction occupies a dominant position in the reaction process, the free amino terminal can react with the carboxyl-terminal, and thus the content of free amino acids in the downward trend of reduction. The reduction of free amino groups is attributed to peptide condensation before and after the plastein reaction, and similar results have also been reported in silver carp protein hydrolysate and hazelnut peptide (Zhou et al., 2024; Song et al., 2023).
The free amino and carboxyl groups of CLPP were blocked to judge whether the plastein reaction involved condensation (Jiang et al., 2018). The yield of the plastein reaction product of CLPP without a closed-end was used as a control. As shown in Figure 8, the plastein reaction product yield decreased from 74.93 to 55.91% after blocking the amino end and from 74.93 to 63.88% after blocking the carboxyl end compared with the control group. Therefore, it can be considered that peptide bond condensation occurred during the plastein reaction of CLPP.
Figure 8. Effect of amino and carboxyl termini of blocked hydrolysates on the yield of plastein products.
The use of plant-derived proteins to prepare peptides with ACE inhibitory effects, thereby lowering blood pressure, is one of the popular research directions at present. There are two ways to lower blood pressure: one is to prevent substrate binding by competitive binding to the active site of ACE; the other is to lower blood pressure by combining with other sites to form enzyme-inhibitor complexes, changing the conformation of ACE and preventing it from catalyzing the production of active angiotensin II (Ang II) (Jiang et al., 2018). Currently, available ACE inhibitors are non-peptide analogs of Ang I, which work by decreasing ACE enzyme activity, thereby reducing Ang II production (Acharya et al., 2024). Computer simulation techniques were used in conjunction with bioassays to identify ACE inhibitors, and selective structural domain docking revealed that 4-O-caffeoylquinic acid, isolated from Moringa, has a strong affinity for the C structural domain of ACE enzyme (Darwish et al., 2024).
The ACE inhibitory activity of CLPP and PCLPP at a concentration of 0.4 mg/mL was evaluated in vitro. The results (Figure 9) showed that the ACE inhibitory activity of PCLPP was 85 ± 2.33%, which was 11.08% higher than that of CLPP. This indicates that the ACE inhibitory activity of specific proteins can be enhanced by plastein reaction. Similar results, such as adding leucine to chicken plasma protein hydrolysate (CPPH) and using trypsin-catalyzed plastein reaction, increased the ACE inhibitory activity of the product by 28.57%, reaching 82.07 ± 0.03% (Gao et al., 2020). Plastein reaction with hazelnut protein hydrolysate and hazelnut peptide YLVR as substrates enhances the activity of ACE inhibitory peptide (Song et al., 2023). Molecular dynamics simulations showed that three non-competitive ACE inhibitory peptides were isolated from Chlorella vulgaris, which interacted with unconventional binding sites on ACE to inhibit ACE activity (Suo et al., 2024). PCLPP has the same ACE inhibitory effect, and its mechanism of action is not very different from those reported so far, which needs to be further investigated.
Plastein reaction is an important enzymatic reaction with important applications in protein or peptide research. Plastein reaction is an important enzymatic reaction with important applications in protein or peptide research. Plastein has been shown to have potential food and nutraceutical applications such as antihypertensive (Xu et al., 2014), antioxidant (Zhao et al., 2014), antithrombotic, enhancement of the nutritional value of proteins, and enhancement of chemosensory properties of protein hydrolysates and peptides (Gong et al., 2015). In this study, the plastein product, PCLPP, was prepared by trypsin catalysis with CLPP as substrate, and the ACE inhibitory activity of PCLPP was 85 ± 2.33%. The structure of PCLPP was analyzed using SEM, FT-IR, XRD, and fluorescence spectra. The results showed that the structure of PCLPP was different from that of the substrate. However, differential scanning calorimetry, blocking of the amino and carboxyl ends showed that aggregation and peptide condensation was involved in the plastein reaction. Moreover, the surface hydrophobicity increases gradually during the plastein reaction, indicating that the hydrophobic interaction is also one of the forces of the plastein reaction. Overall, our study shows that the plastein reaction is a complex process involving physical aggregation, hydrophobicity, condensation, and peptide transformation. Future studies will further isolate specific ACE inhibitory peptides and validate the activity and biostability of plastein reaction-modified products in cells and in vivo. Plastein reaction shows great potential for improving some biological activities of protein hydrolysates.
The original contributions presented in the study are included in the article/supplementary material, further inquiries can be directed to the corresponding authors.
JS: Writing – review & editing. ZY: Writing – original draft, Writing – review & editing. MX: Writing – original draft, Writing – review & editing. GZ: Writing – original draft, Writing – review & editing. YG: Writing – original draft, Writing – review & editing. HZ: Writing – original draft, Writing – review & editing. JF: Writing – original draft, Writing – review & editing.
The author(s) declare that no financial support was received for the research, authorship, and/or publication of this article.
The authors declare that the research was conducted in the absence of any commercial or financial relationships that could be construed as a potential conflict of interest.
All claims expressed in this article are solely those of the authors and do not necessarily represent those of their affiliated organizations, or those of the publisher, the editors and the reviewers. Any product that may be evaluated in this article, or claim that may be made by its manufacturer, is not guaranteed or endorsed by the publisher.
Acharya, K. R., Gregory, K. S., and Sturrock, E. D. (2024). Advances in the structural basis for angiotensin-1 converting enzyme (ACE) inhibitors. Biosci. Rep. 44, 1–17. doi: 10.1042/BSR20240130
Chinma, C. E., Abu, J. O., and Abubakar, Y. A. (2010). Effect of tiger nut (Cyperus esculentus) flour addition on the quality of wheat-based cake. Int. J. Food Sci. Technol. 45, 1746–1752. doi: 10.1111/j.1365-2621.2010.02334.x
Darwish, M. M., Ibrahim, R. S., Metwally, A. M., and Mahrous, R. S. (2024). In-depth in silico and in vitro screening of selected edible plants for identification of selective C-domain ACE-1 inhibitor and its synergistic effect with captopril. Food Biosci. 59:104115. doi: 10.1016/j.fbio.2024.104115
Doucet, D., Gauthier, S. F., Otter, D. E., and Foegeding, E. A. (2003). Enzyme-induced gelation of extensively hydrolyzed whey proteins by alcalase: comparison with the plastein reaction and characterization of interaction. J. Agric. Food Chem. 51, 6036–6042. doi: 10.1021/jf026041r
Doucet, D., Gauthier, S. F., Otter, D. E., and Foegeding, E. A. (2014). In vitro calcium-chelating and platelet anti-aggregation activities of soy protein hydrolysate modified by the alcalase-catalyzed plastein reaction. J. Food Biochem. 38, 374–380. doi: 10.1111/jfbc.12063
Gao, B., and Zhao, X. H. (2012). Modification of soybean protein hydrolysates by alcalase-catalyzed plastein reaction and the ACE-inhibitory activity of the modified product in vitro. Int. J. Food Prop. 15, 982–996. doi: 10.1080/10942912.2010.511755
Gao, D. D., Guo, P. H., Cao, X., Ge, L. L., Ma, H. X., Cheng, H., et al. (2020). Improvement of chicken plasma protein hydrolysate angiotensin I-converting enzyme inhibitory activity by optimizing plastein reaction. Food Sci. Nutr. 8, 2798–2808. doi: 10.1002/fsn3.1572
Gong, M., Mohan, A., Gibson, A., and Udenigwe, C. C. (2015). Mechanisms of plastein formation, and prospective food and nutraceutical applications of the peptide aggregates. Biotechnol. Rep. 5, 63–69. doi: 10.1016/j.btre.2014.12.003
Gong, Y., Feng, M. Q., and Sun, J. (2025). Effect of different thermal processing methods and thermal core temperatures on the protein structure and in vitro digestive characteristics of beef. Food Chem. 464:141751. doi: 10.1016/j.foodchem.2024.141751
Han, X. X., Jia, Y. Q., Liu, C. Y., Wang, H. Y., and Zhu, Z. Y. (2023). Structure identification and inhibitory mechanism evaluation of three novel angiotensin converting enzyme (ACE) inhibitory peptides from small-aroma chicken. Process Biochem. 132, 86–96. doi: 10.1016/j.procbio.2023.07.011
Jiang, S. S., Zhao, Y. H., Shen, Q., Zhu, X. J., Dong, S. Y., Liu, Z. Y., et al. (2018). Modification of ACE-inhibitory peptides from Acaudina molpadioidea using the plastein reaction and examination of its mechanism. Food Biosci. 26, 1–7. doi: 10.1016/j.fbio.2018.08.008
Kaori, I., Takanobu, G., Hirokuni, K., Seiichi, M., Takeshi, N., and Naoyuki, Y. (2009). Release of antihypertensive peptides in miso paste during its fermentation, by the addition of casein. J. Biosci. Bioeng. 108, 111–115. doi: 10.1016/j.jbiosc.2009.03.007
Li, J. P., Gong, C., Wang, Z. Y., Gao, R. C., Ren, J. Y., Zhou, X. D., et al. (2019). Oyster-derived zinc binding peptide modified by plastein reaction via zinc chelation promotes the intestinal absorption of zinc. Mar. Drugs 17:341. doi: 10.3390/md17060341
Li, Q., Fu, Y., Zhang, L. T., Otte, J., and Lametsch, R. (2020). Plastein from hydrolysates of porcine hemoglobin and meat using Alcalase and papain. Food Chem. 320:126654. doi: 10.1016/j.foodchem.2020.126654
Ma, C. L., Li, T. J., and Zhao, X. H. (2019). Pepsin-catalyzed plastein reaction with tryptophan increases the in vitro activity of lactoferrin hydrolysates with BGC-823 cells. Food Biosci. 28, 109–115. doi: 10.1016/j.fbio.2019.01.013
Mohammed, A., Sanusi, K., and Haruna, U. Y. (2022). Tiger nut (Cyperus esculentus L.) and date palm (Phoenix dactylifera L.) fruit blend mitigates hyperglycemia, insulin resistance and oxidative complications in type-2 diabetes models. J. Food Biochem. 46, e14423–e14423. doi: 10.1111/jfbc.14423
Parker, M. L., Ng, A., Smith, A. C., and Waldron, K. W. (2000). Esterified phenolics of the cell walls of chufa (Cyperus esculentus L.) tubers and their role in texture. J. Agric. Food Chem. 48, 6284–6291. doi: 10.1021/jf0004199
Qian, F., Wang, Y., Wen, Z. J., Jiang, S. J., Tuo, Y. F., and Mu, G. Q. (2018). Plastein reaction enhanced bile-acid binding capacity of soybean protein hydrolysates and whey protein hydrolysates. J. Food Sci. Technol. 55, 1021–1027. doi: 10.1007/s13197-017-3015-8
Qu, W. J., Li, Y. H., Xiong, T., Feng, Y. H., Ma, H. L., Dzidzorgbe, K., et al. (2022). Calcium-chelating improved zein peptide stability, cellular uptake, and bioactivity by influencing the structural characterization. Food Res. Int. 162:112033. doi: 10.1016/j.foodres.2022.112033
Sánchez-Zapata, E., Fernández-López, J., and Pérez-Alvarez, J. A. (2012). Tiger nut (Cyperus esculentus) commercialization: health aspects, composition, properties, and food applications. Caliph. J. Sci. Technol. 11, 366–377. doi: 10.1111/j.1541-4337.2012.00190.x
Song, W. T., Fu, J. X., Zeng, Q., Lu, H. Y., Wang, J., Fang, L., et al. (2023). Improving ACE inhibitory activity of hazelnut peptide modified by plastein: physicochemical properties and action mechanism. Food Chem. 402:134498. doi: 10.1016/j.foodchem.2022.134498
Spanneberg, R., Osswald, F., Kolesov, I., Anton, W., Radusch, H. J., and Glomb, M. A. (2010). Model studies on chemical and textural modifications in gelatin films by reaction with glyoxal and glycolaldehyde. J. Agric. Food Chem. 58, 3580–3585. doi: 10.1021/jf9039827
Sun, X. H., Acquah, C., Gazme, B., Boachie, R. T., Nwachukwu, I. D., and Udenigwe, C. C. (2021). Mechanisms of plastein formation influence the IgE-binding activity of egg white protein hydrolysates after simulated static digestion. Food Chem. 345, 128783. doi: 10.1002/9781119792130
Suo, Q. S., Wang, J., Wu, N., Geng, L. H., Zhang, Q. B., and Yue, Y. (2024). Discovery of a novel nanomolar angiotensin-I converting enzyme inhibitory peptide with unusual binding mechanisms derived from Chlorella pyrenoidosa. Int. J. Biol. Macromol. 280:135873. doi: 10.1016/j.ijbiomac.2024.135873
Suo, Q. S., Yue, Y., Wang, J., Wu, N., Geng, L. H., and Zhang, Q. B. (2022). Isolation, identification and in vivo antihypertensive effect of novel angiotensin I-converting enzyme (ACE) inhibitory peptides from Spirulina protein hydrolysate. Food Funct. 13, 9108–9118. doi: 10.1039/D2FO01207C
Taskiran, A. S., and Avci, O. (2021). Effect of captopril, an angiotensin-converting enzyme inhibitor, on morphine analgesia and tolerance in rats, and elucidating the inflammation and endoplasmic reticulum stress pathway in this effect. Neurosci. Lett. 741:135504. doi: 10.1016/j.neulet.2020.135504
Wang, J. P., Hu, J. E., Cui, J. Z., Bai, X. F., Du, Y. G., Miyaguchi, Y. J., et al. (2008). Purification and identification of a ACE inhibitory peptide from oyster proteins hydrolysis and the antihypertensive effect of hydrolysis in spontaneously hypertensive rats. Food Chem. 111, 302–308. doi: 10.1016/j.foodchem.2008.03.059
Wei, X., Li, T. J., and Zhao, X. H. (2013). Coupled neutrase-catalyzed plastein reaction mediated the ACE-inhibitory activity in vitro of casein hydrolysates prepared by alcalase. Int. J. Food Prop, 16, 429–443. doi: 10.1080/10942912.2011.553759
Xie, Y. T., Cai, L. L., Zhao, D., Liu, H., Xu, X. L., Zhou, G. H., et al. (2022). Real meat and plant-based meat analogues have different in vitro protein digestibility properties. Food Chem. 387:132917. doi: 10.1016/j.foodchem.2022.132917
Xu, W., Kong, B. H., and Zhao, X. H. (2014). Optimization of some conditions of neutrase-catalyzed plastein reaction to mediate ACE-inhibitory activity in vitro of casein hydrolysate prepared by Neutrase. J. Food Sci. Technol. 51, 276–284. doi: 10.1007/s13197-011-0503-0
Xu, X. F., Liu, W., Liu, C. M., Luo, L. P., Chen, J., Luo, S. J., et al. (2016). Effect of limited enzymatic hydrolysis on structure and emulsifying properties of rice glutelin. Food Hydrocoll. 61, 251–260. doi: 10.1016/j.foodhyd.2016.05.023
Xu, Z. Q., Wu, C. P., Sun, D. X., Zhao, T. T., Zhao, M. M., and Su, G. W. (2021). Identification of post-digestion angiotensin-I converting enzyme (ACE) inhibitory peptides from soybean protein Isolate: their production conditions and in silico molecular docking with ACE. Food Chem. 345:128855. doi: 10.1016/j.foodchem.2020.128855
Yasmin, T., and Joerg, B. (2018). Investigation of applicability and compatibility of enalapril minitablets in various beverages. Int. J. Pharm. 536:505. doi: 10.1016/j.ijpharm.2017.08.031
Ye, S. F., Chen, Q. L., Li, D. Y., Zhou, H. Y., Chen, Y. B., Meng, C., et al. (2022). Isolation and identification of novel angiotensin I-converting enzyme (ACE) inhibitory peptides from Pony Seed and evaluation of the inhibitory mechanisms. J. Funct. Foods 95:105151. doi: 10.1016/j.jff.2022.105151
Yu, Y., Hu, J., Miyaguchi, Y., Bai, X., Du, Y., and Lin, B. (2006). Isolation and characterization of angiotensin I-converting enzyme inhibitory peptides derived from porcine hemoglobin. Peptides 27, 2950–2956. doi: 10.1016/j.peptides.2006.05.025
Yuka, K., Tadashi, Y., Tomohisa, K., Hideki, Y., and Shigeo, K. (2008). Angiotensin-I converting enzyme (ACE) inhibitory mechanism of tripeptides containing aromatic residues. J. Biosci. Bioeng. 106, 310–312. doi: 10.1263/jbb.106.310
Zhang, Y., Liu, D. S., Liu, X. M., Hang, F., Zhou, P., Zhao, J. X., et al. (2018). Effect of temperature on casein micelle composition and gelation of bovine milk. Int. Dairy J. 78, 20–27. doi: 10.1016/j.idairyj.2017.10.008
Zhang, Y., and Zhao, X. H. (2014). In vitro angiotensin I-converting enzyme inhibition of casein hydrolysate responsible for plastein reaction in ethanol-water medium, solvent fractionation, and protease digestion. Int. J Food Prop. 17, 1577–1590. doi: 10.1080/10942912.2013.768269
Zhao, X. H., Fu, Y., and Yue, N. (2014). In vitro cytoprotection of modified casein hydrolysates by plastein reaction on rat hepatocyte cells. CyTA J. Food 12, 40–47. doi: 10.1080/19476337.2013.792296
Zhao, X. H., and Li, Y. Y. (2009). An approach to improve ACE-inhibitory activity of casein hydrolysis with plastein reaction catalyzed by Alcalase. Eur. Food Res. Technol. 229, 795–805. doi: 10.1007/s00217-009-1110-4
Zheng, L. J., Xu, H. J., Hu, H., Ruan, J. X., Shi, C. H., Cao, J. Q., et al. (2022). Preparation, characterization and antioxidant activity of inclusion complex loaded with puerarin and corn peptide. Food Biosci. 49:101886. doi: 10.1016/j.fbio.2022.101886
Zhou, Y. J., Ding, N., Zhang, Y., Zhang, H. J., Hong, H., Luo, Y. K., et al. (2024). Plastein reaction augments the metal chelating capabilities of silver carp (Hypophthalmichthys molitrix) hydrolysates: unlocking the chemical modification mechanism. Food Chem. 438:138030. doi: 10.1016/j.foodchem.2023.138030
Keywords: plastein reaction, trypsin, peptides, angiotensin converting enzyme, tiger nut
Citation: Shi J, Yang Z, Xu M, Zhao G, Gao Y, Zheng H and Feng J (2025) Structure characterization and mechanism of angiotensin I-converting enzyme (ACE) inhibitory peptides modified by plastein reaction derived from tiger nut (Cyperus esculentus). Front. Sustain. Food Syst. 8:1476134. doi: 10.3389/fsufs.2024.1476134
Received: 05 August 2024; Accepted: 13 December 2024;
Published: 15 January 2025.
Edited by:
Nataša Nastić, University of Novi Sad, SerbiaReviewed by:
Michael Ukwuru Ukwuru, Federal Polytechnic Idah, NigeriaCopyright © 2025 Shi, Yang, Xu, Zhao, Gao, Zheng and Feng. This is an open-access article distributed under the terms of the Creative Commons Attribution License (CC BY). The use, distribution or reproduction in other forums is permitted, provided the original author(s) and the copyright owner(s) are credited and that the original publication in this journal is cited, in accordance with accepted academic practice. No use, distribution or reproduction is permitted which does not comply with these terms.
*Correspondence: Yawen Gao, Z2FveWF3ZW5AamxhdS5lZHUuY24=; Hongyan Zheng, emhlbmdob25neWFuNjgxMUAxNjMuY29t
Disclaimer: All claims expressed in this article are solely those of the authors and do not necessarily represent those of their affiliated organizations, or those of the publisher, the editors and the reviewers. Any product that may be evaluated in this article or claim that may be made by its manufacturer is not guaranteed or endorsed by the publisher.
Research integrity at Frontiers
Learn more about the work of our research integrity team to safeguard the quality of each article we publish.