- 1Key Laboratory of Agricultural Biosafety and Green Production of Upper Yangtze River (Ministry of Education), College of Horticulture and Landscape Architecture, Southwest University, Chongqing, China
- 2School of Design, Chongqing Industry Polytechnic College, Chongqing, China
- 3The Southwest Institute of Fruits Nutrition, Chongqing, China
Introduction: Currently, the large-scale consumption of fresh citrus fruits in the form of juices, jams, and purees results in significant quantities of waste consisting of citrus peels, pulp, and seeds.
Methods: To improve the utilization rate of whole citrus fruits and reduce the generation of processing waste, the best pre-optimized enzymatic fermentation conditions were used to treat whole citrus fruits and to analyse the changes in nutritional and active components (Enzymatic: pectinase, cellulase, hemicellulase added at a ratio of 1:1:1 for 63.326 min at a temperature of 60°C, with 0.506% of each enzyme added; Fermentation: Lacticaseibacillus rhamnosus TR08, Lactiplantibacillus plantarum subsp. Plantarum CICC 6257, and Limosilactobacillus fermentum CCFM1139 were added at a ratio of 1:1:1 for 30 h, with the concentration was 108 CFU/mL, and the total amount added was 6%).
Results: Results showed that after enzymatic hydrolysis, the mineral elements (Nitrogen, phosphorus), total amino acid, vitamin C, and organic acid content increased. For soluble sugars, the contents of fructose and glucose increased, whereas the sucrose content decreased. Fermentation had a minimal effect on the mineral content; however, the total amino acid, vitamin C, and soluble sugar contents decreased. Additionally, when comparing the samples after enzymatic fermentation to those after enzymatic hydrolysis alone, the total phenols, total flavonoids, carotenoids and antioxidant activities were significantly increased by 1.39, 1.49, 1.21 and 3.79 folds, respectively.
Discussion: This study addresses the challenges of utilizing fruit residue in the current market, including high treatment costs and environmental pollution, by providing a novel perspective on the enzymatic hydrolysis and fermentation of whole red oranges. Furthermore, it aims to improve the nutritional, functional, and health benefits of citrus fruit products.
1 Introduction
Citrus fruits are highly favored for their unique flavor and high nutritional value, making them suitable for fresh consumption or processing into juice, jam, and puree. These products are rich in soluble sugars, organic acids, vitamin C (Vc), minerals, amino acids, and functional substances such as flavonoids and carotenoids (Yuasa et al., 2021). The global production of citrus fruits is approximately 158.5 million tons and continues to increase annually. However, citrus fruit processing generates a significant amount of waste, comprising approximately 50% of the mass of fresh fruit, leading to resource waste and environmental burdens (Zema et al., 2018). Citrus fruit waste is considered an effective bioresource material for various applications in the food and nonfood industries because it contains valuable compounds (Santos et al., 2022). Therefore, novel, environmentally friendly processing methods are needed to increase the overall utilization rate of citrus fruits and increase the value added to the citrus industry.
Enzymatic hydrolysis refers to the use of active enzymes to hydrolyze specific substances, achieving the hydrolysis and biotransformation of polysaccharides and other macromolecules (Rasera et al., 2023). It is widely used in the food processing industry to convert raw materials into products, optimize the nutritional structure of products, adjust product functional properties, and increase product yield (Mok et al., 2023). The cell walls of plant in fruit pomace mainly consist of cellulose, hemicellulose, and pectin, which severely hinder the conversion and reuse of available substances (Bashline et al., 2014; Mok et al., 2023). Different types of enzymes can be used to degrade structures in plant cell walls, increasing cell wall porosity and allowing the release of nutrients and active substances, which is the primary purpose and means of food processing (Santos et al., 2022). The most common enzymes used to degrade cell wall components are cellulase, hemicellulase, and pectinase, which act synergistically to relax the cell structure, facilitating the extraction and utilization of nutrients (Marić et al., 2018). Compared with single enzymes, mixed enzymes are used at lower doses to promote the production of reducing sugars, the release of polyphenolic compounds, and the extraction of active substances (e.g., naringin and hesperidin) from citrus jam (Ruviaro et al., 2019; Tao et al., 2022).
Probiotics, primarily lactobacilli, bifidobacteria, yeasts, and lactococci, are defined as live microorganisms that confer health benefits to the host (Cosme et al., 2022). The functions of pro-biotics include improving gut health, enhancing immune responses, suppressing lactose intolerance, preventing cancer, reducing serum cholesterol levels, lowering the risk of colon cancer, and enhancing the immune system of consumers (Kechagia et al., 2013; Sionek et al., 2023). Through the life activities of probiotics, these beneficial microorganisms can directly or indirectly produce metabolites during fermentation, improving product quality, enhancing product flavor, increasing product functional activity, and extending shelf-life (Ruviaro et al., 2019; Guan et al., 2021). Probiotics can also produce active enzymes such as amylase, peptidase, protease, dehydrogenase, decarboxylase, and β-glucosidase during fermentation, which can improve the digestibility and nutritional value of various foods and convert major food substrates into functional bioactive substances (Cheirsilp et al., 2023).
In addition, the application of enzymatic hydrolysis combined with probiotic fermentation in the processing of fruits such as peaches, apples, citrus fruits, and plums has become a current hotspot for researchers (Fernandes Pereira and Rodrigues, 2018). In previous studies, it was found that after enzymatic hydrolysis and fermentation of whole citrus fruits, the total content of polyethoxylated flavonoids (PMFs) and flavanones increased significantly, the total content of organic acids decreased significantly, and the antioxidant capacity improved (Tao et al., 2022). Combining the methods of first acid hydrolysis of citrus extracts followed by fermentation with lactobacilli can accelerate the conversion of flavonoid glycosides with lower biological activity into aglycones with higher activity (König et al., 2023). The processing waste of citrus juice, after being subjected to both enzymatic hydrolysis and fermentation methods, has resulted in an increased content of dietary fiber, with fermentation emerging as the optimal method (Yi et al., 2014). Therefore, the combined method of enzymatic hydrolysis and fermentation for processing citrus fruits is an effective way to enhance their value. However, there are currently limited studies on the nutritional and health benefits of red mandarin after whole fruit enzymatic hydrolysis and fermentation, which restricts the application of red mandarin fruits.
In summary, this study explores the impact of a combined method of enzymatic hydrolysis and probiotic fermentation on the nutritional quality (mineral elements, amino acids, and Vc), flavor compounds (soluble sugars and organic acids), phytochemicals (total phenols, total flavonoids, and carotenoids), and health benefits (antioxidant activity) of whole red mandarin. The aim is to enhance the flavor, improve the nutrition, and provide health benefits for red mandarin products. The research results can improve the utilization rate of whole red mandarin during processing, thereby reducing resource waste while further enhancing the economic value of red mandarin fruits. This provides a theoretical basis for further developing red mandarin resources and exploring deep processing methods.
2 Materials and methods
2.1 Plant materials
At commercial maturity, the red mandarin (Citrus reticulata Blanco cv. ‘Dahongpao’) materials used in this study were harvested from Dazhou Town, Wanzhou District, Chongqing Municipality, in late October 2022. After being harvested, they were immediately transported to the Key Laboratory of Agricultural Biosecurity and Green Production of the Ministry of Education of the Upper Yangtze River at Southwest University. Fruits free from pests, diseases, and other damage were selected and then soaked in 1.0% (v/v) sodium hypochlorite solution for 2 min, rinsed with running tap water, air-dried at 25°C, and stored in a freezer at-20°C for later use.
Pectinase (100 kU/g), cellulase (100 kU/g), and hemicellulase (100 kU/g) were purchased from Henan Wanbang Chemical Technology Co., Ltd. Lacticaseibacillus rhamnosus TR08 (LR)、Lactiplantibacillus plantarum subsp. Plantarum CICC 6257 (LP), and Limosilactobacillus fermentum CCFM1139 (LF) were purchased from Zhenjiang Tianyi Biotechnology Co., Ltd.
2.2 Preparation of citrus whole fruit homogenate, enzymatic hydrolysis, and postenzymatic fermentation
2.2.1 Preparation of Citrus reticulata Blanco cv. ‘Dahongpao’ whole-fruit homogenate
Frozen Citrus reticulata Blanco cv. ‘Dahongpao’ fruits were thawed at 25°C. The fruit stems were removed, and the fruits were then crushed and homogenized in a blender (MJ-PB12Easy219, Midea, China) for 5 min until a uniform fruit pulp was achieved. This homogenate was stored in a-80°C freezer for further processing. The juice sample obtained by filtering the homogenate through 200-mesh gauze was labeled CK.
2.2.2 Enzymatic hydrolysis the Citrus reticulata Blanco cv. ‘Dahongpao’ whole-fruit homogenate
The pH of the red mandarin homogenate prepared in Section 2.2.1 was adjusted to 3.42 using food-grade citric acid. Subsequently, 1.5% (w/v) of a mixed enzyme solution (cellulose: hemicellulase: pectinase at a ratio of 1:1:1, 100 kU/g) was added. The mixture was subjected to enzymatic hydrolysis in a water bath at 60°C for 63.326 min Following hydrolysis, the enzyme-treated homogenate was inactivated at 90°C for 10 min. The inactivated homogenate was then filtered through 200-mesh gauze, and the resulting juice sample was labeled AEH.
2.2.3 Fermented the Citrus reticulata Blanco cv. ‘Dahongpao’ whole-fruit homogenate
The prepared enzyme-hydrolyzed red mandarin homogenate was divided into 200 g portions and added to 250 mL sterilized conical flasks, and sealed properly. The sealed flasks were then subjected to pasteurization (70°C for 30 min). Once cooled to 25°C, 6% (w/v) pre-activated mixed lactic acid bacteria culture (LR: LP: LF) fermentum at a ratio of 1:1:1, each with a concentration of 108 CFU/mL was inoculated onto the homogenate under aseptic conditions. Lactic acid fermentation was carried out at 37°C for 30 h. Following fermentation, the homogenate was filtered through 200-mesh gauze to obtain the red mandarin juice sample, which was labeled AF.
CK, AEH, and AF were stored in an ultralow-temperature freezer (UF3410, Thermo Fisher Scientific, USA) at-80°C for future analysis.
2.3 Nutrient determination
2.3.1 Mineral elements
Following a method proposed by McKenzie et al. (2010) with slight modifications, 30 mL of mixed acid solution (HNO3:HClO4 = 4:1) was added to 10 mL of CK, AEH, AF (prepared in Section 2.2). The digested CK, AEH, and AF were cooled to 25°C, transferred to 50 mL volumetric flasks, and left at 25°C for 12 h. The mixture was subsequently heated at 120°C for 2–3 h on a DB–B adjustable hotplate (produced by Jiangsu Jintan Shuibeixing Instrument Factory, China) until black smoke no longer appeared. Digestion was continued at 150–200°C until the residual digestion solution was reduced to 1–2 mL and became colorless and transparent. After cooling, the volume of the digested solution was adjusted to 10 mL with distilled water (Milli–Q, Millipore Corporation, USA). Elemental analysis of kalium (K), calcium (Ca), natrium (Na), ferrum (Fe), and zinc (Zn) was performed via flame atomic absorption spectroscopy (FAAS) with an atomic absorption spectrometer (AA–7000, Shimadzu Corporation, Japan), following the method outlined by Karaaslan et al. (2018). Additionally, the determination of cuprum (Cu) and manganese (Mn) contents was conducted via graphite furnace atomic absorption spectroscopy (GFAAS) according to a procedure described by Costa et al. (2021). The concentrations of K, Ca, Na, Fe, Zn, Cu, and Mn were calculated via a standard curve, and the results were reported in mg/L FW (fresh weight) along with their standard deviations.
The molybdenum blue spectrophotometric method for phosphorus (P) determination (Huo and Li, 2012) was used with slight modifications. Briefly, 1 mL of CK, AEH, and AF (prepared in Section 2.2) was mixed with 10 mL of HNO3, 1 mL of HClO4, and 2 mL of H2SO4, respectively, and subjected to digestion on an adjustable hotplate until the digestion solution became colorless and transparent. After cooling, 4 mL of distilled water was added to remove excess acid, followed by further cooling and volume adjustment to 10 mL with distilled water. Subsequently, 200 μL of the resulting solution was transferred to a test tube and mixed with 200 μL of ammonium molybdate solution (50 g/L), 100 μL of sodium sulfite solution (200 g/L), and 100 μL of phenol solution (5 g/L). The mixture was then adjusted to a total volume of 2.5 mL with distilled water and allowed to react for 30 min. Configuration of the phosphorus standard solution: 0.4394 g KH2PO4 was weighed, an appropriate amount of water was added to dissolve the chemical, which was subsequently transferred to a 1,000 mL volumetric flask, water was added to bring it to the final volume, and the mixture was mixed well. A total of 10 mL of phosphorus standard stock solution (100.0 mg/L) was added, and the mixture was placed in a 100 mL volumetric flask, diluted with water to the final volume, and mixed well. The phosphorus content was determined at 660 nm using a spectrophotometer (Lambda 25, PerkinElmer Inc., USA). The P content was calculated via a standard curve. The results are expressed as mg/L FW plus standard deviation.
2.3.2 Amino acids
Following a methodology described in previous studies (Wistaff et al., 2021), the content of 15 amino acids was determined. A total of 2.5 mL of CK, AEH, and AF (prepared in Section 2.2) were placed in an amino acid hydrolysis tube, to which 20 mL of HCl (6 mol/L) and 1 mL of phenol (1%, w/v) were added. The tube was flushed with N2 until all of the air was displaced, and the tube was tightly sealed with a cap. Hydrolysis was conducted at 110°C for 24 h, followed by cooling and centrifugation at 4000 rpm for 10 min. The supernatant was evaporated to near dryness at 80°C, and then 2 mL of distilled water was added before evaporation was carried out again. This process was repeated twice, and finally, the volume was adjusted to 6 mL.
To 1 mL of the resulting solution, 500 μL of phenyl isothiocyanate-acetonitrile solution (0.1 mol/L) and 500 μL of triethylamine-acetonitrile solution (0.1 mol/L) were added, and the mixture was placed in a 50°C water bath for 45 min. Subsequently, 2 mL of n-hexane was added, and the solution was vortexed for 1 min before leaving it undisturbed until phase separation occurred.
The lower layer was filtered through a 0.45 μm organic membrane filter and subjected to amino acid analysis via high-performance liquid chromatography (HPLC) (Waters, USA). The separation was performed on a Sunfire C18 column (250 mm × 4.6 mm, 5 μm) at 36°C with mobile phase A: 0.1 mol/L sodium acetate buffer solution (pH 6.5) and mobile phase B: 80% acetonitrile at a flow rate of 1 mL/min, and the injection volume was 10 μL. The detection wavelength was 254 nm. The amino acid concentrations were quantified according to the peak area via an external standard method. The results are expressed as mg/L FW plus standard deviation.
2.3.3 Vitamin C (Vc)
The determination of the Vc content was conducted following a method outlined by Nováková et al. (2008) with slight modifications. A total of 6 mL of CK, AEH, and AF (prepared in Section 2.2) was mixed with 24 mL of metaphosphoric acid solution (20 g/L) and subjected to ultrasonic extraction at room temperature for 5 min. After centrifugation at 4000 rpm for 5 min, the supernatant was filtered through a 0.45 μm aqueous membrane filter and analyzed via HPLC.
Column: Waters Sunfire C18 column (250 mm × 4.6 mm, 5 μm). Mobile phase: A: 6.8 g KH2PO4 and 0.91 g C19H42BrN were dissolved in water, and the volume was adjusted to 1 L (pH adjusted to 2.5 ~ 2.8 with H3PO4); B: 100% methanol. B: 100% methanol. A:B = 98:2 was mixed, passed through a 0.45 μm filter membrane, and degassed via ultrasonication. The flow rate was 0.7 mL/min, the detection wavelength was 245 nm, and the column temperature was 25°C. The amount of Vc was calculated via a standard curve. The results are expressed as mg/L FW plus standard deviation.
2.4 Flavor substance determination and soluble sugar and organic acid contents
The contents of soluble sugars (glucose, fructose, sucrose) and organic acids (tartaric acid, malic acid, citric acid, oxalic acid, lactic acid, and acetic acid) were determined via methods described in a previous study by Santos et al. (2022). Briefly, 1 mL of CK, AEH, or AF (prepared in Section 2.2) was centrifuged at 5000 × g for 2 h, evaporated at 45°C and then resuspended in 1 mL of distilled water. The aqueous solution was filtered through a 0.22 μm hydrophilic polytetrafluoroethylene (PTFE) membrane filter (Ampel Laboratory Technologies, Shanghai, China) and loaded into HPLC vials. The soluble sugars were separated using an amino-phase C18 column (Waters, SunFire). The mobile phases were 85% (v/v) acetonitrile (phase A) and 15% (v/v) water (phase B) at a flow rate of 1.0 mL/min. The temperature of the column was 40°C, the flow rate of the carrier gas was 40 P, and the temperature of the drift tube was 65°C. The separation of the organic acids was carried out on a Waters Sunfire C18 column (5 μm particle size, 150 × 4.6 mm). The mobile phase was 40 mM KH2PO4-H3PO4 buffer (pH 2.4). The flow rate and column temperature were set at 0.8 mL/min and 30°C, respectively, and the detection wavelength was 210 nm. The contents of soluble sugars and organic acids were calculated using a standard curve. The results are expressed as mg/L FW plus standard deviation.
2.5 Determination of phytochemical substances
2.5.1 Total phenolics (TPC) and total flavonoids (TFC)
The extraction method for TPC and TFC was adapted from Zhao et al. (2017) with slight modifications. In brief, 0.40 g of sample (prepared in Section 2.2) was mixed with 8 mL of methanol (80%, v/v) and subjected to ultrasonic extraction at 50°C for 45 min. The mixture was then centrifuged at 5000 rpm for 10 min, after which the supernatant was collected. This extraction process was repeated twice, and the supernatants were combined and adjusted to a final volume of 25 mL to obtain the extract for further analysis.
The determination of the TPC was conducted using the Folin–Ciocalteu method (Liu et al., 2022) with slight modifications. A volume of 120 μL of the extract was mixed with 4 mL of distilled water and 400 μL of Folin–Ciocalteu reagent, followed by incubation in the dark for 5 min. Subsequently, 2 mL of Na2CO3 solution (5%, w/v) was added, and the volume was adjusted to 10 mL with distilled water. The mixture was then allowed to react in the dark for 60 min, after which the absorbance was measured at 765 nm with a spectrophotometer. The TPC content was expressed as milligrams of gallic acid equivalents (GAE) per gram of FW (mg GAE/g FW).
The TFCs were determined via the NaNO2-Al(NO3)3 colorimetric method (Liu et al., 2022) with slight modifications. A volume of 500 μL of the extract was sequentially mixed with 200 μL of NaNO2 solution (5%, w/v) and 700 μL of distilled water, followed by incubation in the dark for 6 min. Then, 300 μL of Al(NO3)3 solution (10%, w/v) was added, and the mixture was incubated in the dark for another 6 min. Subsequently, 2 mL of NaOH solution (1 mol/L) was added, and the volume of the mixture was adjusted to 5 mL with distilled water. The mixture was further incubated in the dark for 15 min, after which the solution absorbance was measured at 500 nm with a spectrophotometer. The TFC content was expressed as milligrams of rutin equivalents (RE) per gram of FW (mg RE/g FW).
2.5.2 Carotenoids
The carotenoid content was determined according to the method outlined by Wei et al. (2023), with slight modifications. Specifically, 50 mL of extraction solvent (hexane: acetone: ethanol = 2:1:1, v/v) was added to a 10 mL sample (prepared in Section 2.2), followed by ultrasonic extraction at 25°C for 40 min. This extraction process was repeated twice, and the supernatants were combined. The collected supernatant was centrifuged at 6500 rpm for 5 min at 4°C, and the organic phase was collected after phase separation. The collected liquid was evaporated to near dryness at 40°C and redissolved in methyl tert-butyl ether (MTBE), and the liquid volume was adjusted to 2 mL.
Subsequently, 2 mL of 10% methanol-KOH solution was added, and the mixture was saponified in the dark for 12 h. Afterward, 5 mL of distilled water and 2 mL of MTBE were added for extraction, and the organic phase was collected. This extraction process was repeated twice with 2 mL of MTBE each time. The organic phases were combined, dried with 0.25 g of anhydrous Na2SO4 for 30 min to 1 h, and evaporated to dryness under nitrogen gas. The residue was redissolved in MTBE, and the liquid volume was adjusted to 1.5 mL, followed by filtration through a 0.22 μm organic mem-brane filter (model) before analysis by HPLC.
The carotenoid content was determined via HPLC on a YMC C30 column (250 mm × 4.6 mm, 5 μm). Mobile phase A was MTBE, mobile phase B was methanol, mobile phase C was H2O, and a gradient elution program was used. The elution program was as follows: 60% B/40% C initially; 80% B/20% C from 0 to 5 min; 15% A/81% B/4% C from 5 to 10 min; 85% A/11% B/4% C from 10 to 60 min; 100% B from 60 to 71 min; and 71–72 min before returning to the initial state to re-equilibrate. The flow rate was 1.0 mL/min, the injection volume was 10 μL, the detection wavelength was 450 nm, the column temperature was 27°C, and the detection time was 50 min. The carotenoid content was calculated via a standard curve. The results are expressed as mg/L FW plus standard deviation.
2.6 Determination of antioxidant activity
The antioxidant activity was determined via three methods, namely, ABTS radical scavenging activity, DPPH radical scavenging activity, and FRAP ferric ion reduction activity, following methods described by Wei et al. (2023) with slight modifications. Standard curves were prepared using Trolox solution in 80% methanol. The results are expressed as Trolox equivalent (TE) per mL of FW (μmol/mL TE FW). All of the samples were diluted 5-fold with distilled water and left to be measured.
For ABTS analysis, 5 mL of ABTS aqueous solution (7 mmol/L) was mixed with 88 μL of K2S2O4 solution (140 mmol/L) and left to react in the dark for 12–16 h. Before use, the ABTS solution was diluted with anhydrous ethanol to achieve an absorbance of 0.70 ± 0.02 at 734 nm. This diluted solution was used as the ABTS reaction mixture. Then, 40 μL of the sample was mixed with 3.90 mL of the ABTS reaction mixture under dark conditions and allowed to react for 10 min. Subsequently, the solution absorbance was measured at 734 nm.
DPPH analysis: 100 μL of the sample was mixed with 3.80 mL of DPPH solution (75 μmol/L) and allowed to react in the dark for 30 min. Subsequently, the solution absorbance was measured at 517 nm.
For FRAP analysis, acetate buffer (0.3 mol/L, pH 3.6), FeCl3 solution (20 mmol/L), and a working solution of TPTZ (10 mmol/L) prepared with HCl (40 mmol/L) were used. These three solutions were mixed at a certain ratio (10:1:1) to obtain the TPTZ solution. Then, 100 μL of the sample was mixed with 3.90 mL of the TPTZ solution under dark conditions and allowed to react for 30 min. Afterward, the solution absorbance was measured at 593 nm.
2.7 Statistical analysis
All collected data were processed via Excel 2018, and analysis and graphing were performed via Origin Pro 2021 software. Significant differences between samples were evaluated via one-way analysis of variance (ANOVA) followed by Duncan’s multiple range test, with p < 0.05 considered to indicate statistical significance. All the experiments were repeated three times, and the results are expressed as the mean ± standard deviation (SD).
3 Results
3.1 The effects of enzymatic hydrolysis and postenzymatic fermentation on the mineral contents of red mandarin juice
The influence of enzymatic hydrolysis and postenzymatic fermentation on the mineral element content of whole red mandarin juice is shown in Figure 1A. In the CK, the contents of Na, K, and Mg were relatively high, ranging from 40.77 mg/L FW to 78.76 mg/L FW; the contents of Fe, Ca, and P ranged from 1.89 mg/L FW to 11.03 mg/L FW; and the contents of Zn, Cu, and Mn were the lowest, ranging from 0.24 mg/L FW to 0.51 mg/L FW. After enzymatic hydrolysis, the contents of Na and P significantly increased. Compared with that of the CK, the Na content increased by 28-fold, whereas the P content increased by 9.6-fold. The high content of Na and P in the peel and seeds of red oranges results in a significant increase in the levels of these two elements after enzymatic hydrolysis of the whole fruit. The Zn and Fe contents increased by 1.4-fold and 1.3-fold respectively, compared with those of the control. Although the contents of Cu, Mg, K, and Mn also increased, the increase was relatively small. Ca was the only element whose content decreased after enzymatic hydrolysis, with a 2.2-fold reduction. Compared with enzymatic hydrolysis, fermentation had a minor effect on the mineral element content. Compared with those during enzymatic hydrolysis, the contents of Ca, Fe, K, Zn, Cu, and Mn increased. The element that showed the most significant increase was Mn, with a 1.3-fold increase. While the contents of Mg, Na, and P all decreased, the most significant decrease was in P, with a 0.5-fold reduction.
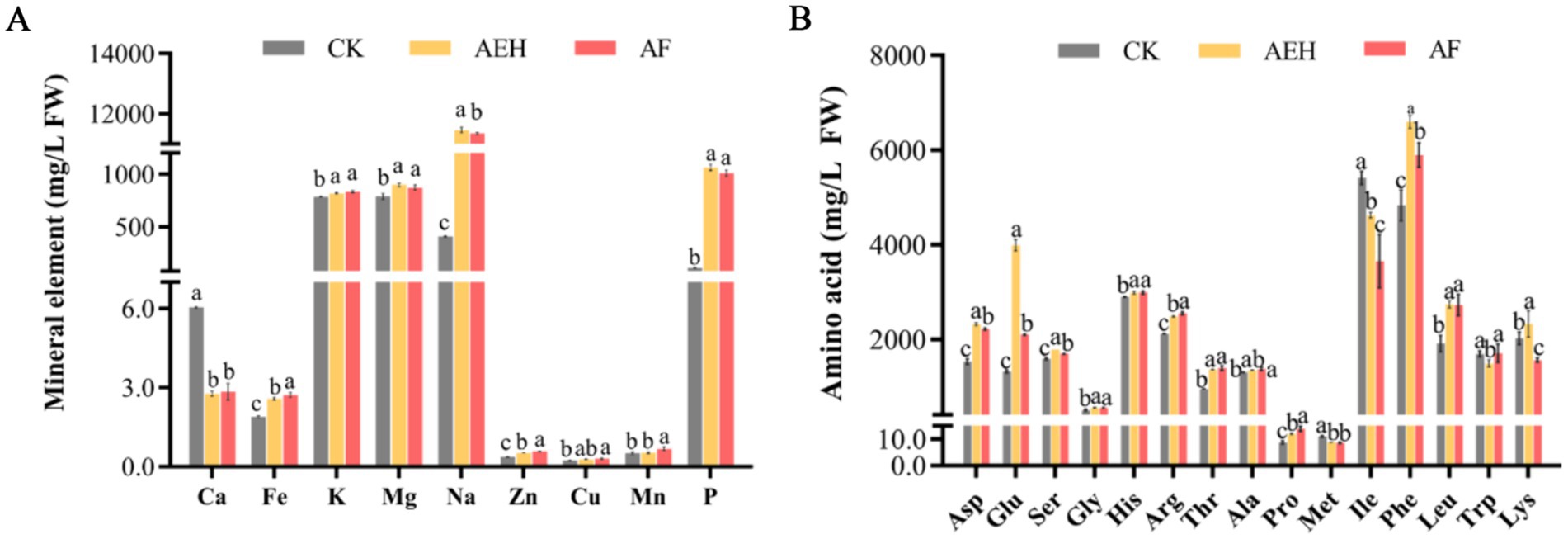
Figure 1. Effects of enzymatic and postenzymatic fermentation on the contents of mineral elements (A) and amino acids (B). Values followed by different superscripts (a–c) are significantly different (p < 0.05). The vertical bars represent the SDs of the means. CK: Juice obtained after filtration of whole fruit homogenate. AEH: Juice obtained by enzymatic hydrolysis and filtration of whole fruit homogenates. AF: Juice obtained by filtering after fermentation with AEH.
3.2 Influence of enzymatic hydrolysis and postenzymatic fermentation on the amino acid content in red mandarin juice
Determination of the amino acid content in red mandarin pulp revealed a total of 15 amino acids, including 8 amino acids essential to human health (histidine, threonine, methionine, isoleucine, phenylalanine, leucine, tryptophan, and lysine), 1 semiessential amino acid (arginine), and 7 nonessential amino acids (aspartic acid, glutamic acid, glycine, proline). The results are presented in Figure 1B. The total amino acid content in the CK was 2.82 ± 0.03 g/L FW, which increased to 3.48 ± 0.04 g/L FW after enzymatic hydrolysis but decreased to 3.06 ± 0.06 g/L FW after fermentation. Phenylalanine (Phe) had the highest content in the CK group, at 48.00 ± 26.48 mg/L FW, whereas proline (Pro) had the lowest, at 8.76 ± 0.46 mg/L FW. After enzymatic hydrolysis, the contents of methionine (Met), isoleucine (Ile), and tryptophan (Trp) decreased, whereas the contents of other amino acids increased. Among them, glutamic acid (Glu) and phenylalanine (Phe) showed the most significant increases, with increases of 266.89 mg/L FW and 176.68 mg/L FW, respectively. After fermentation, the contents of glutamic acid (Glu), isoleucine (Ile), phenylalanine (Phe), and lysine (Lys) significantly decreased.
3.3 Influence of enzymatic hydrolysis and postenzymatic fermentation on the contents of Vc, TPC, and TFC in red mandarin juice
The effects of enzymatic hydrolysis and postenzymatic fermentation on the Vc content in red mandarin pulp juice are shown in Figure 2A. The Vc content in the CK was 30.00 ± 0.62 mg/L FW. After enzymatic hydrolysis, the Vc content increased to 50.09 ± 0.41 mg/L FW, representing a 66.97% increase compared with that of CK. After fermentation, the Vc content slightly decreased to 46.62 ± 0.69 mg/L FW. These findings indicated that enzymatic hydrolysis and postenzymatic fermentation increased the Vc content in red mandarin pulp juice, enhancing its nutritional quality.
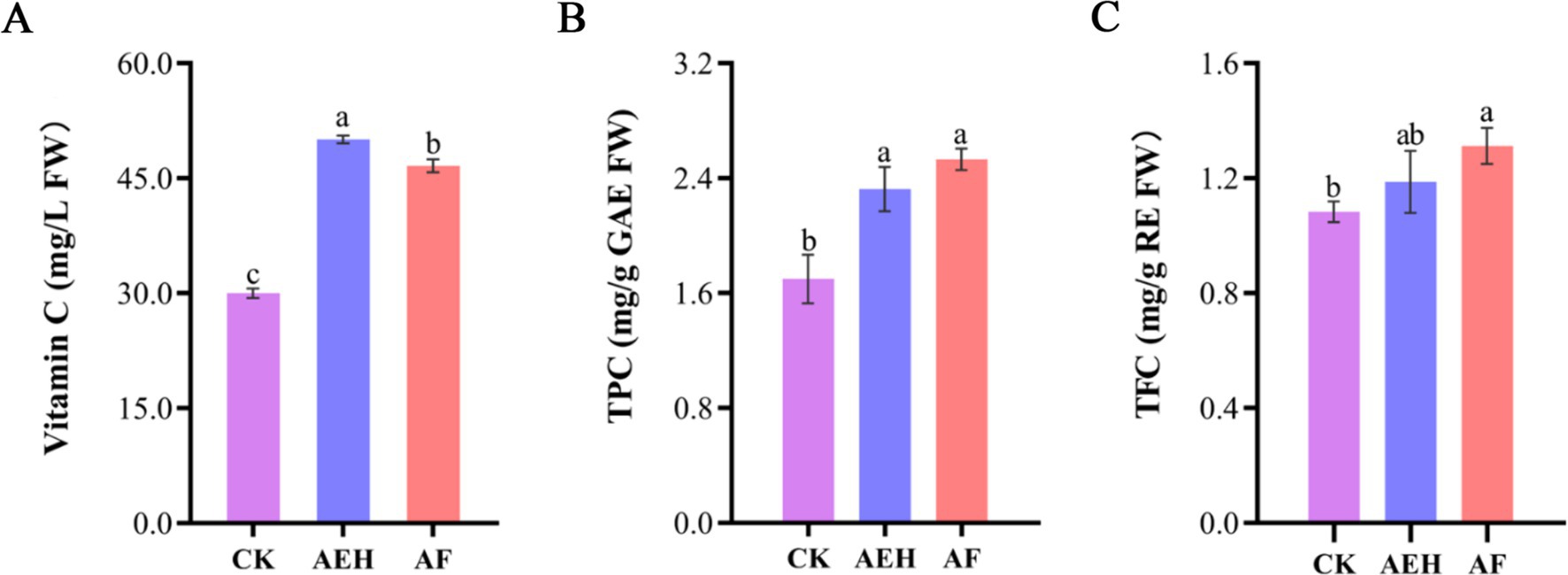
Figure 2. Effects of enzymatic and postenzymatic fermentation on the contents of the carotenoids Vc (A), TPC (B), and TFC (C). Values followed by different superscripts (a–b) are significantly different (p < 0.05). The vertical bars represent the SDs of the means. CK: Juice obtained after filtration of whole fruit homogenate. AEH: Juice obtained by enzymatic hydrolysis and filtration of whole fruit homogenates. AF: Juice obtained by filtering after fermentation with AEH.
Changes in the TPC and TFC of red mandarin pulp juice after enzymatic hydrolysis and postenzymatic fermentation were determined, and the results are shown in Figures 2B,C. The TPC and TFC increased after enzymatic hydrolysis and were further enhanced by fermentation. The TPC in CK was 1.7 ± 0.69 mg/g GAE FW. After enzymatic hydrolysis, the TPC increased to 2.32 ± 0.15 mg/g GAE FW, representing a 36.47% increase compared with that of CK. After fermentation, the TPC continued to increase to 2.53 ± 0.07 mg/g GAE FW. The TFC in the CK treatment was 1.08 ± 0.04 mg/g RE FW. After enzymatic hydrolysis, the TFC increased to 1.19 ± 0.11 mg/g RE FW, representing a 10.19% increase compared with that of CK. After fermentation, the TFC significantly increased to 1.31 ± 0.06 mg/g RE FW.
3.4 Influence of enzymatic hydrolysis and postenzymatic fermentation on carotenoids in red mandarin juice
Five types of carotenoids, namely, lutein, zeaxanthin, β-cryptoxanthin, α-carotene, and β-carotene, were measured in red mandarin pulp, as shown in Figure 3. β-Cryptoxanthin, lutein, and β-carotene were the major carotenoids in red mandarin juice, whereas α-carotene was not detected in the CK. α-Carotene was detected in the red mandarin pulp after enzymatic hydrolysis, and the contents of all five carotenoids significantly increased, ranging from 0.58 mg/L FW to 3.49 mg/L FW, representing increases of 58 to 275% compared with those in the CK. Fermentation also increased the contents of the five carotenoids, with lutein showing the greatest increase of 1.56 mg/L FW, whereas the increases in the other four carotenoids were relatively modest.
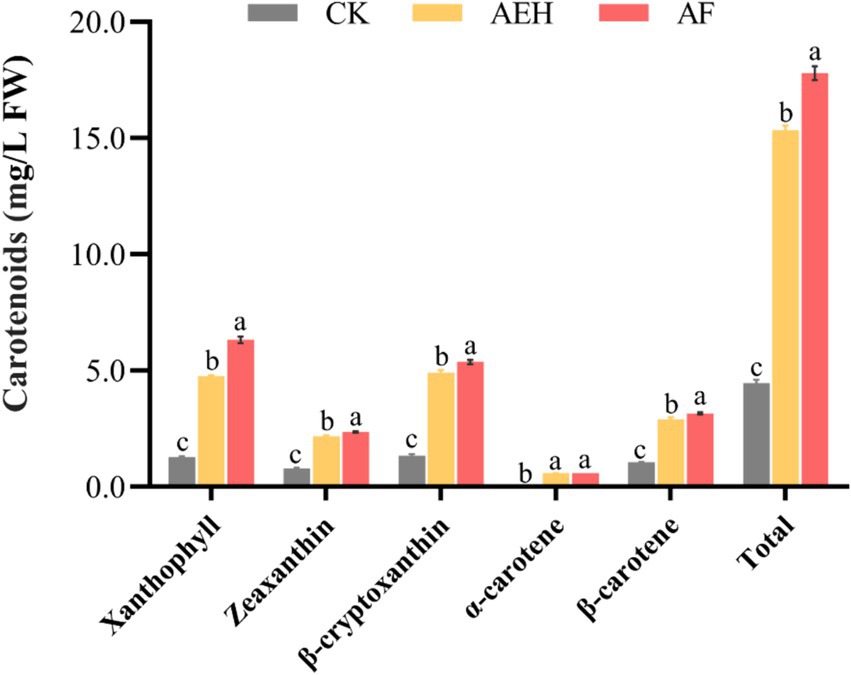
Figure 3. Effects of enzymatic and postenzymatic fermentation on the carotenoid content. Values followed by different superscripts (a–c) are significantly different (p < 0.05). The vertical bars represent the SDs of the means. CK: Juice obtained after filtration of whole fruit homogenate. AEH: Juice obtained by enzymatic hydrolysis and filtration of whole fruit homogenates. AF: Juice obtained by filtering after fermentation with AEH.
3.5 Influence of enzymatic hydrolysis and postenzymatic fermentation on soluble sugars and organic acids in red mandarin juice
The contents of three soluble sugars (fructose, glucose, sucrose) and seven organic acids (tartaric acid, malic acid, citric acid, oxalic acid, lactic acid, acetic acid, and succinic acid) were determined during the enzymatic hydrolysis and postenzymatic fermentation processes (Figure 4A). Soluble sugars are key factors affecting fruit quality, directly influencing sweetness and flavor. The highest content of sucrose was found in untreated red mandarin pulp juice (118.08 ± 1.50 g/L FW), followed by glucose (37.35 ± 1.38 g/L FW), and fructose had the lowest content (6.79 ± 1.50 g/L FW). Enzymatic hydrolysis increased the contents of glucose and fructose in red mandarin juice by 48.97 g/L FW and 17.19 g/L FW, respectively. After enzymatic hydrolysis and postenzymatic fermentation, the total decrease in sucrose content reached 117.40 g/L FW, indicating that sucrose was the most consumed sugar among the three soluble sugars. As shown in Figure 4B, acetic acid (26.58 ± 1.45 g/L FW) was the most abundant organic acid in red mandarin pulp, followed by citric acid, whereas oxalic acid had the lowest content (0.15 ± 0.00 g/L FW). After enzymatic hydrolysis, the contents of lactic acid and acetic acid significantly increased, with increases of 1.48 g/L FW and 10.18 g/L FW, respectively, whereas the changes in the contents of the other four organic acids were not significant. Fermentation significantly affected the contents of lactic acid, acetic acid, and oxalic acid. The lactic acid content increased by 0.85 g/L FW, the acetic acid content decreased by 14.13 g/L FW, and oxalic acid was not detected after fermentation.
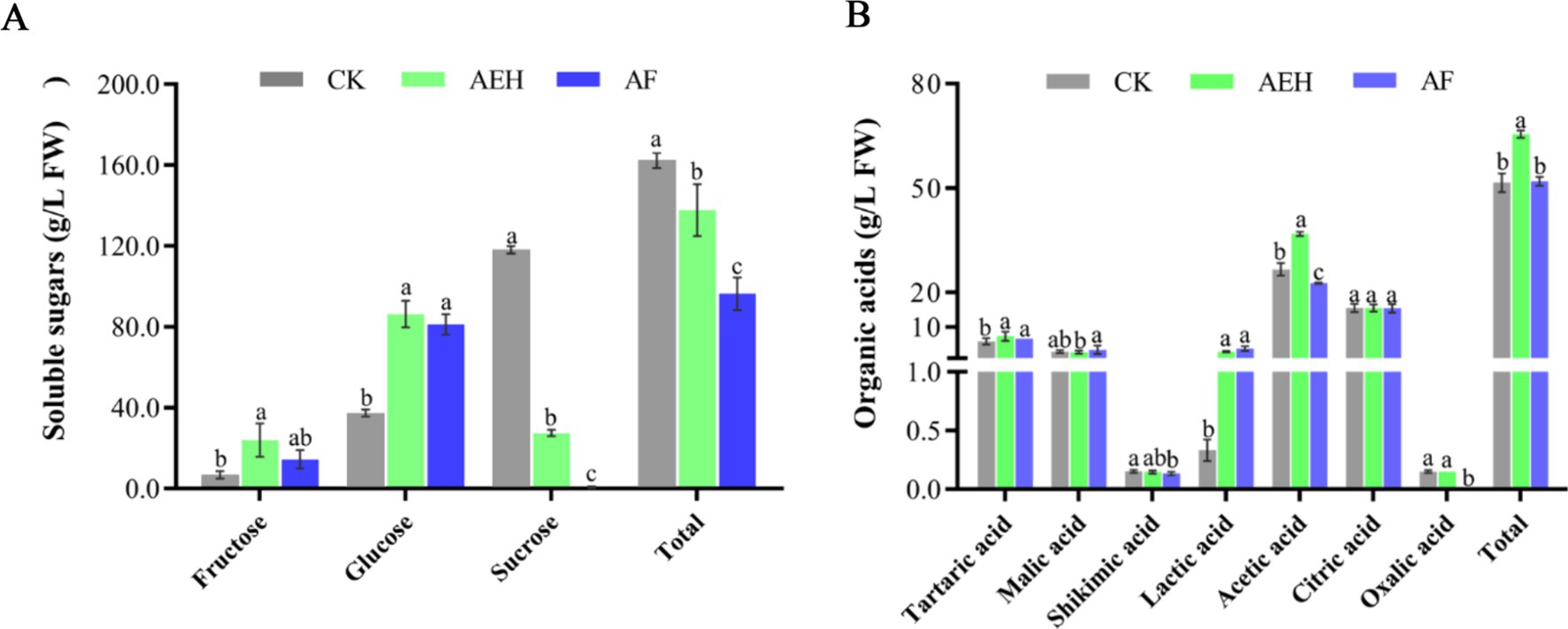
Figure 4. Effects of enzymatic and postenzymatic fermentation on the contents of soluble sugars (A) and organic acids (B). Values followed by different superscripts (a–c) are significantly different (p < 0.05). The vertical bars represent the SDs of the means. CK: Juice obtained after filtration of whole fruit homogenate. AEH: Juice obtained by enzymatic hydrolysis and filtration of whole fruit homogenates. AF: Juice obtained by filtering after fermentation with AEH.
3.6 Influence of enzymatic hydrolysis and postenzymatic fermentation on the antioxidant activity of red mandarin juice
The antioxidant capacity of the three samples was evaluated using the DPPH, ABTS, and FRAP methods, as shown in Table 1. The trends in the antioxidant capacity measured by the three methods were generally consistent across the different samples. The range of DPPH radical scavenging activity was 3.31–3.87 μmol/g TE FW; the range of ABTS radical scavenging activity was 1.72–1.92 μmol/g TE FW; and the FRAP values ranged from 3.52–3.61 μmol/g TE FW. To better evaluate the total antioxidant capacity of the samples, the comprehensive antioxidant performance index (APC index) was used in this study for evaluation. The APC of the studied samples ranged from 91.03 to 100%. The total antioxidant capacity of the three samples was ranked as follows: AF > AEH > CK. These results indicated that enzymatic hydrolysis and postenzymatic fermentation effectively enhanced the antioxidant capacity of red mandarin pulp (see Figure 5).
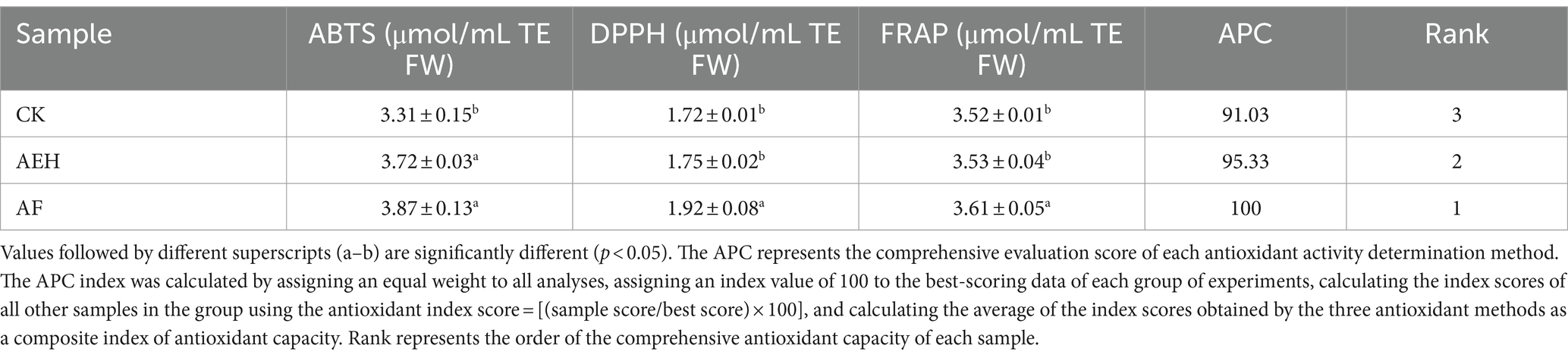
Table 1. Effects of enzymolysis and postenzymatic fermentation on the antioxidant activity of C. reticulata Blanco cv. Dahongpao juice.
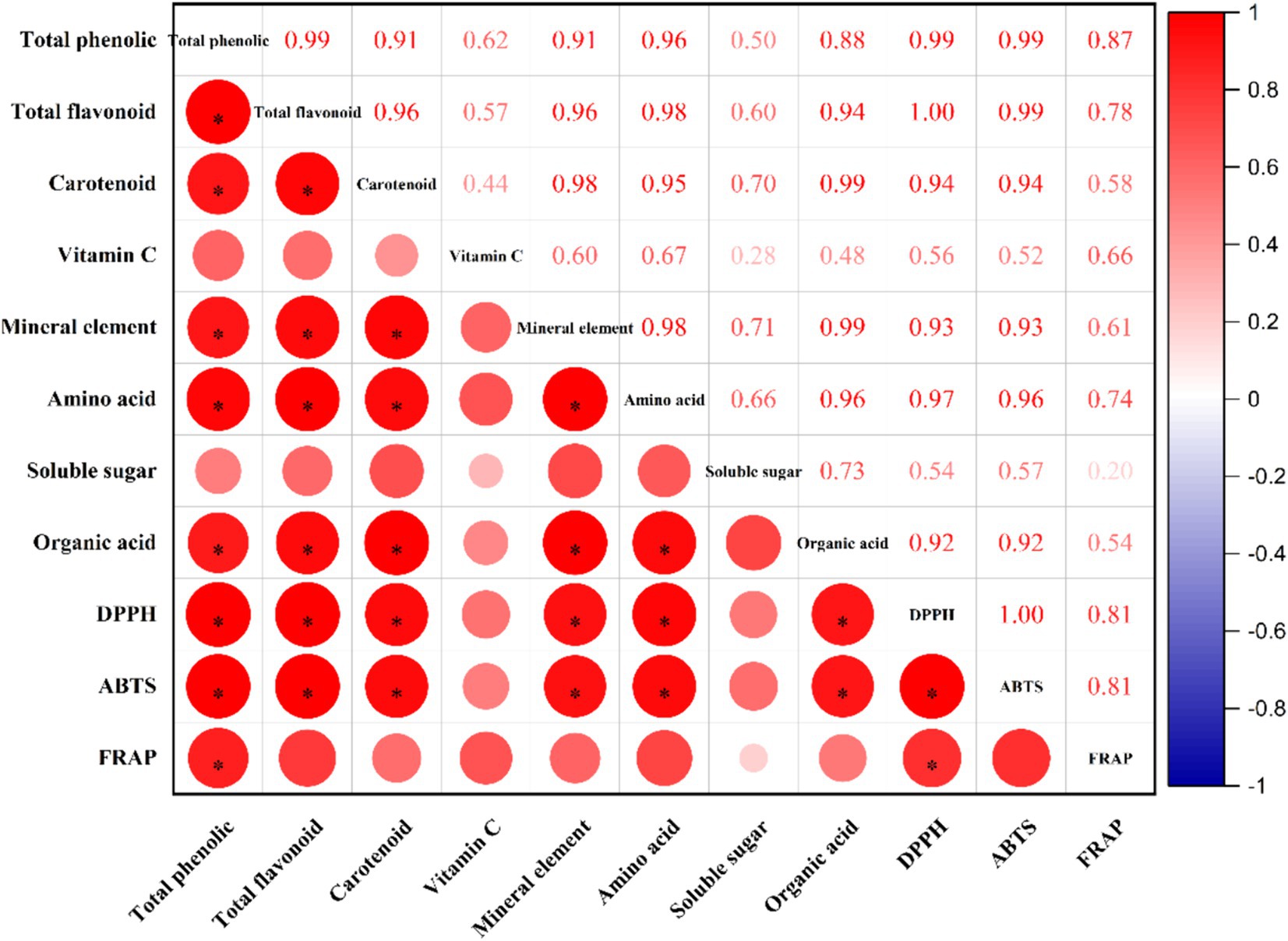
Figure 5. Correlation analysis between measured indicators and antioxidant activity. The asterisk indicates p < 0.05.
3.7 Pearson’s correlation analysis
Pearson’s correlation analysis was conducted to investigate the correlations between the levels of mineral elements, amino acids, Vc, carotenoids, soluble sugars, organic acids, total phenolics, total flavonoids, and antioxidant activity in red mandarin juice obtained after enzymatic hydrolysis and postenzymatic fermentation. The results from the three methods of antioxidant capacity analysis were positively correlated with eight indicators, with Pearson coefficients ranging from 0.20 to 1.00. According to the DPPH results, the Pearson coefficient between the TFC and antioxidant activity was the highest at 1.00 (p < 0.05), followed by the TFC with a Pearson coefficient of 0.99 (p < 0.05) and the third was amino acids with a Pearson coefficient of 0.97 (p < 0.05). According to the ABTS measurements, the TPC and TFC had the highest Pearson coefficients with antioxidant activity, both of which were 0.99 (p < 0.05). This was followed by amino acids, with a Pearson coefficient of 0.96 (p < 0.05). According to the FRAP results, the highest Pearson coefficient between TPC and antioxidant activity was 0.87 (p < 0.05), the second highest was TFC, with a Pearson coefficient of 0.78 (p < 0.05), and the third highest was amino acids, with a value of 0.74 (p < 0.05). These findings indicate that, compared with other substances, TPC, TFC, and amino acids make more significant contributions to the antioxidant capacity of red mandarin juice (Table 2.).
4 Discussion
Enzymes, as catalysts, bind to plant cell walls, causing partial bond breakage, thereby releasing active intracellular components (Sheldon and van Pelt, 2013). Dal Magro et al. (2016) reported that, compared with single enzyme formulations, the cooperative effect of multiple enzymes enhances plant cell wall degradation, increasing juice yield and the release of bioactive compounds. Fermented foods have been an essential part of the human diet since ancient times, extending shelf-life, enhancing the sensory properties of food, and improving the digestibility of proteins and carbohydrates, as well as the bioavailability of vitamins and minerals (Sanlier et al., 2019). In this study, enzymatic hydrolysis and fermentation of whole red mandarin pulp resulted in higher contents of mineral elements, total amino acids, Vc, organic acids, and soluble sugars in the AEH than in the CK. The synergistic action of pectinase, cellulase, and hemicellulase enzymes increased the release of juice and compounds, creating a favorable fermentation environment for subsequent cofermentation with Lacticaseibacillus rhamnosus TR08, Lactiplantibacillus plantarum subsp. Plantarum CICC 6257, and Limosilactobacillus fermentum CCFM1139, thereby increasing the nutritional value and flavor of red mandarin juice.
Mineral elements play crucial roles in regulating internal balance within the human body, contributing significantly to normal metabolism and reducing disease risk (Mohammadifard et al., 2019). The bioavailability of mineral nutrients in plant products is often low due to their complexation with compounds present in the plant matrix, such as oxalates, phytates, polyphenolic compounds, and dietary fibers, which bind or chelate metal ions in in-soluble forms (Suliburska and Krejpcio, 2014). In this study, the enzymatic hydrolysis of citrus pulp released metal ions, some of which are less bioavailable; in AEH, the contents of mineral elements increased, except for calcium, which decreased. Oxalate acts as a chelating agent for positively charged metal ions, including calcium ions (Peng et al., 2021). Postenzymatic hydrolysis of red mandarin pulp disrupts cell walls, increasing the oxalate content, which can precipitate as insoluble calcium oxalate, potentially contributing to the reduction in calcium content (Kim, 2017). In our study, the contents of elements such as magnesium, sodium, and phosphorus were lower in AF than in AEH, likely due to consumption by lactobacilli during fermentation to support their normal metabolism and growth (de Souza et al., 2015). Nevertheless, after enzymatic hydrolysis and fermentation of red mandarin pulp, the contents of mineral elements in the AF, excluding calcium, were greater than those in the CK.
Amino acids are crucial nutrients involved in regulating essential metabolic pathways and processes vital for growth and maintenance in organisms (Wu, 2009; D’Este et al., 2018) and are key nutrients found in citrus fruits and critical participants in the Maillard reaction (Liu et al., 2020). In this study, the total amino acid content in AEH significantly increased compared with that in CK. The enzymatic reactions catalyzed by the three enzymes decompose large protein molecules, releasing protein hydrolysates from whole red mandarin fruits and thereby increasing the total amino acid content (Lolli et al., 2023). However, due to heat inactivation at 90°C after enzymatic hydrolysis, Maillard reactions may have occurred, potentially leading to a decrease in the individual amino acid contents. The total amino acid content in AF was slightly lower than that in AEH, with glutamic acid showing the most significant reduction. Similar to our findings, Costa et al. (2021) reported de-creases in Ile, Phe, Lys, and total free amino acids after fermentation of citrus juice with lactic acid bacteria. We speculate that during the fermentation of whole red mandarin fruits, some amino acids may have been utilized as nitrogen sources for the growth and metabolism of lactic acid bacteria. Conversely, amino acid metabolism by lactic acid bacteria can produce volatile compounds contributing to the formation of aromas in fermented citrus juices (Castellano et al., 2012). Compared with the original citrus pulp CK, enzymatic hydrolysis and fermentation increased the total amino acid content in the juice, providing not only enhanced nutrition but also imparting a more flavorful taste to the juice.
Vc is one of the most important nutrients in citrus fruits (Suri et al., 2022). It is a water-soluble compound with excellent antioxidant properties that is capable of mitigating oxidative stress by maintaining the activity of ascorbate peroxidase and supporting immune function in the human body (Mditshwa et al., 2017). In addition to pulp, citrus peels also contain abundant Vc; enzymatic degradation of citrus peel cell walls and polysaccharides can increase the release rate of Vc (Ni et al., 2014; Suri et al., 2022). In this study, the Vc content in AEH increased by 66.97% after enzymatic hydrolysis (Di Cagno et al., 2009). Fermented tomato juice has been reported to have a higher Vc content than nonfermented juice. However, in our study, the Vc content in AF decreased after fermentation, possibly due to the lower total organic acid content in AF than in AEH, which reduces the acidity of the juice, thereby destabilizing Vc and causing oxidation and loss (Znamirowska et al., 2021). Nevertheless, the Vc content in the AF remained significantly greater than that in the CK.
Soluble sugars and organic acids are important nutritional components and flavor substances in fruit and vegetable juices (Li et al., 2023). In the enzymatic hydrolysis process of this study, the sucrose content in AEH decreased, whereas the glucose and fructose contents increased, resulting in a slight overall decrease in total sweetness perception substances but with significant compositional changes. Research has shown that mixed enzyme hydrolysis can increase the hydrolysis rate and amount of monosaccharides released after enzymatic hydrolysis, where sucrose is partially degraded, leading to a decreased content, whereas the contents of monosaccharides such as glucose and fructose increase (Lao et al., 2020), which is similar to our findings. During fermentation, plant lactic acid bacteria preferentially utilize simple carbohydrates such as sugars and glucose (Oguntoyinbo et al., 2016); in Costa et al. (2021)‘s study, all sugars significantly decreased after fermentation. In our study, the total soluble sugar content continued to decrease in AF, with sucrose being nearly completely consumed, whereas the glucose and fructose contents remained relatively stable. This may be because lactic acid bacteria use sugars from AEH as reaction substrates (Liu et al., 2023); early in fermentation, lactic acid bacteria likely utilize sucrose more than glucose and fructose because the breakdown rate of sucrose is faster than that of lactobacilli. Later, lactic acid bacteria utilized glucose and fructose as carbon sources for growth and reproduction (Cho et al., 2006; Yang et al., 2018). In terms of sweetness sub-stances, the levels of glucose and fructose increased in AF, replacing sucrose as the main sugar contributing to sweetness.
Postenzymatic hydrolysis, the contents of acetic acid in AF increased significantly, whereas the contents of malic acid and citric acid remained almost unchanged, possibly due to their involvement in the citric acid cycle and tricarboxylic acid cycle (Yang et al., 2022). The acetic acid content in AF significantly decreased, oxalic acid completely disappeared, and the lactic acid content substantially increased. Ghamry et al. (2023) reported that plant lactic acid bacteria have strong capabilities-in oxalic acid degradation. Therefore, it is speculated that during the fermentation of red mandarin juice, the three strains of lactic acid bacteria may have consumed acetic acid and oxalic acid as carbon sources, leading to a significant decrease in acetic acid and oxalic acid contents postfermentation; moreover, lactic acid bacteria converted glucose in the juice into lactic acid, resulting in increased lactic acid content. In terms of acidic substances, oxalic acid and acetic acid have pungent odors, whereas lactic acid provides mild acidity and a natural preservation effect. These changes in acidity undoubtedly contribute favorably to the final product’s taste.
Citrus fruits also contain many other antioxidant bioactive compounds, primarily carotenoids and phenolic compounds, which vary in quality and quantity depending on fruit species and varieties, collectively contributing to health benefits (Suri et al., 2022). In this study, TPC and TFC significantly increased in whole red mandarin pulp after enzymatic hydrolysis and fermentation. The enzymatic hydrolysis of CK by these three enzymes releases bound compounds such as PMFs and flavanones unique to citrus peels, increasing their bioavailability and converting flavonoid glycosides into more water-soluble glycosidic ligands (de Souza et al., 2015), thereby increasing the content of TPC and TFC in AEH. The continued increase in TPC and TFC in AF may be attributed to lactic acid bacteria producing enzymes such as β-glucosidase, α-glucosidase, and various glycosidases or esterases during fermentation, which hydrolyze glycosidic and ester bonds, releasing bound phenolic compounds into soluble or insoluble forms and increasing their content (Landete et al., 2014; Tao et al., 2022). Additionally, lactic acid bacteria can convert flavonoid glycosides into free flavonoid aglycones, significantly increasing the flavonoid content (Bisakowski et al., 2007).
Carotenoids can associate with stabilizing intermediates such as proteins, fatty acids, and sugars (Daruwalla et al., 2020). In our study, the carotenoid content increased significantly after mixed enzyme hydrolysis, with α-carotene detected in AEH, which was absent in CK. It has been shown that enzymatic hydrolysis softens citrus tissues, disrupts the cell walls of both the peel and pulp, reduces the lipid and lignin contents, and releases protein–carotenoid complexes, thereby increasing the extractability of carotenoid pigments (Santamaría et al., 2000; Nath et al., 2016). Santos et al. (2022) reported that lactic acid bacteria can increase the total carotenoid content during peach pulp fermentation. In our study, the increase in ca-rotenoid content in AF may be attributed to the ability of Lactiplantibacillus plantarum subsp. plantarum to produce the C30 carotenoid 4,4′-diaponeurosporene (Antognoni et al., 2019). Additionally, metabolites from the three strains of lactic acid bacteria can increase the antioxidant activity of red mandarin juice, reduce carotenoid oxidation and isomerization, and potentially reduce the specific accumulation of glutathione or related thiol compounds, thereby reducing lipid oxidation (Mapelli-Brahm et al., 2020).
Bioactive compounds offer health benefits to consumers by protecting against free radical-mediated deterioration and slowing lipid oxidation, demonstrating stronger antioxidant activity than synthetic antioxidants (Maisuthisakul et al., 2007). In this study, correlation analysis was conducted on amino acids, mineral elements, Vc, organic acids, soluble sugars, TPC, TFC, carotenoids, and antioxidant capacity, revealing that TPC, TFC, and amino acids significantly contribute to antioxidant activity. Compared with CK, AEH resulted in a decrease in total sugars but an increase in other substances. In contrast, after fermentation, AF presented decreased total levels of amino acids, mineral elements, Vc organic acids, and soluble sugars, whereas the TPC, TFC, and carotenoid contents significantly increased. TPC, TFC, and carotenoids are positively correlated with antioxidant capacity (Maisuthisakul et al., 2007; Laophongphit et al., 2023). Phenolic compounds possess structural forms capable of donating hydrogen atoms to free radicals (Aryal et al., 2019). Due to its relatively high TPC, TFC, and carotenoid contents, AF can help combat free radicals in the body, providing significant antioxidant health benefits.
In summary, through enzymatic hydrolysis and fermentation processes, this study has improved the utilization rate of the entire citrus fruit, utilizing the phytochemical properties in the peel and seeds of red mandarin, enhancing the nutritional and health benefits of the jam. Therefore, this study provides a theoretical basis for improving the utilization rate of the entire citrus fruit and enhancing the nutritional and health benefits of jam. Given that this study explores a product containing probiotics, preserving freshness while maintaining the activity of probiotics to the greatest extent possible is a topic worthy of further research in the future. In subsequent research, further exploration of the product specifications of ‘Dahongpao’ red mandarin jam should be conducted to provide more reliable data references for the development of red mandarin resources.
5 Conclusion
This study has developed a jam rich in nutrients and bioactive substances using the whole fruit of ‘Dahongpao’ red mandarin as the raw material through enzymatic hydrolysis and fermentation processes. After processing, the nutritional content and health benefits of red tangerine have increased significantly, and due to changes in soluble sugars and sour substances, the product has a delightful sweet-and-sour taste. Enzymatic hydrolysis followed by fermentation increased the content of vitamin C, total phenols, total flavonoids, and carotenoids, which were 1.39, 1.49, 1.21, and 3.79 folds higher than those in the original red mandarin, respectively. Therefore, this jam has advantag fold es in both nutritional and health benefits compared to fresh fruit, in addition to potential benefits in standardization. The optimized enzymatic hydrolysis and fermentation process parameters obtained in this study can be applied in industrial production, facilitating the transformation of laboratory data into large-scale factory production. This will enhance the utilization rate of the whole fruit on a larger scale and reduce waste of resources.
Data availability statement
The original contributions presented in the study are included in the article/supplementary material, further inquiries can be directed to the corresponding authors.
Author contributions
YL: Formal analysis, Methodology, Software, Writing – original draft. LG: Formal analysis, Software, Validation, Writing – review & editing. XM: Data curation, Formal analysis, Methodology, Writing – original draft. CJ: Data curation, Writing – review & editing. WL: Funding acquisition, Supervision, Writing – review & editing. ZZ: Funding acquisition, Project administration, Resources, Writing – review & editing.
Funding
The author(s) declare that financial support was received for the research, authorship, and/or publication of this article. This research was funded by the Municipal Technology Innovation and Application Development Special Key Projects of Chongqing (No. CSTB2022TIAD-KPX0086) and the Doctoral Fund of Chongqing Industry Polytechnic College (No. 2023GZYBSZK2-02) and The APC was funded by the Doctoral Fund of Chongqing Industry Polytechnic College (No. 2023GZYBSZK2-02).
Conflict of interest
The authors declare that the research was conducted in the absence of any commercial or financial relationships that could be construed as a potential conflict of interest.
Publisher’s note
All claims expressed in this article are solely those of the authors and do not necessarily represent those of their affiliated organizations, or those of the publisher, the editors and the reviewers. Any product that may be evaluated in this article, or claim that may be made by its manufacturer, is not guaranteed or endorsed by the publisher.
References
Antognoni, F., Mandrioli, R., Potente, G., Taneyo Saa, D. L., and Gianotti, A. (2019). Changes in carotenoids, phenolic acids and antioxidant capacity in bread wheat doughs fermented with different lactic acid bacteria strains. Food Chem. 292, 211–216. doi: 10.1016/j.foodchem.2019.04.061
Aryal, S., Baniya, M. K., Danekhu, K., Kunwar, P., Gurung, R., and Koirala, N. (2019). Total phenolic content, flavonoid content and antioxidant potential of wild vegetables from Western Nepal. Plan. Theory 8:96. doi: 10.3390/plants8040096
Bashline, L., Lei, L., Li, S., and Gu, Y. (2014). Cell Wall, cytoskeleton, and cell expansion in higher plants. Mol. Plant 7, 586–600. doi: 10.1093/mp/ssu018
Bisakowski, B., Atwal, A. S., Gardner, N., and Champagne, C. P. (2007). Effect of lactic acid fermentation of onions (Allium cepa) on the composition of flavonol glucosides. Int. J. Food Sci. Technol. 42, 783–789. doi: 10.1111/j.1365-2621.2006.01268.x
Castellano, P., Aristoy, M. C., Sentandreu, M. A., Vignolo, G., and Toldrá, F. (2012). Lactobacillus sakei CRL1862 improves safety and protein hydrolysis in meat systems. J. Appl. Microbiol. 113, 1407–1416. doi: 10.1111/jam.12005
Cheirsilp, B., Mekpan, W., Sae-ear, N., Billateh, A., and Boukaew, S. (2023). Enhancing functional properties of fermented Rice cake by using germinated black glutinous Rice, probiotic yeast, and enzyme technology. Food Bioprocess Technol. 16, 1116–1127. doi: 10.1007/s11947-022-02985-z
Cho, J., Lee, D., Yang, C., Jeon, J., Kim, J., and Han, H. (2006). Microbial population dynamics of kimchi, a fermented cabbage product. FEMS Microbiol. Lett. 257, 262–267. doi: 10.1111/j.1574-6968.2006.00186.x
Cosme, F., Inês, A., and Vilela, A. (2022). Consumer’s acceptability and health consciousness of probiotic and prebiotic of non-dairy products. Food Res. Int. 151:110842. doi: 10.1016/j.foodres.2021.110842
Costa, H. P., da Silva, M. G. C., and Vieira, M. G. A. (2021). Biosorption of aluminum ions from aqueous solutions using non-conventional low-cost materials: a review. J. Water Process Eng. 40:101925:101925. doi: 10.1016/j.jwpe.2021.101925
D’Este, M., Alvarado-Morales, M., and Angelidaki, I. (2018). Amino acids production focusing on fermentation technologies – a review. Biotechnol. Adv. 36, 14–25. doi: 10.1016/j.biotechadv.2017.09.001
Dal Magro, L., Dalagnol, L. M. G., Manfroi, V., Hertz, P. F., Klein, M. P., and Rodrigues, R. C. (2016). Synergistic effects of Pectinex ultra clear and Lallzyme Beta on yield and bioactive compounds extraction of Concord grape juice. LWT Food Sci. Technol. 72, 157–165. doi: 10.1016/j.lwt.2016.04.046
Daruwalla, A., Zhang, J., Lee, H. J., Khadka, N., Farquhar, E. R., Shi, W., et al. (2020). Structural basis for carotenoid cleavage by an archaeal carotenoid dioxygenase. Proc. Natl. Acad. Sci. USA 117, 19914–19925. doi: 10.1073/pnas.2004116117
de Souza, R. B., de Menezes, J. A. S., de Souza, R., Dutra, E. D., and de Morais Jr, M. A. (2015). Mineral composition of the sugarcane juice and its influence on the ethanol fermentation. Appl. Biochem. Biotechnol. 175, 209–222. doi: 10.1007/s12010-014-1258-7
Di Cagno, R., Surico, R. F., Paradiso, A., De Angelis, M., Salmon, J.-C., Buchin, S., et al. (2009). Effect of autochthonous lactic acid bacteria starters on health-promoting and sensory properties of tomato juices. Int. J. Food Microbiol. 128, 473–483. doi: 10.1016/j.ijfoodmicro.2008.10.017
Fernandes Pereira, A. L., and Rodrigues, S. (2018). “Chapter 15 - turning fruit juice into probiotic beverages” in Fruit Juices. eds. G. Rajauria and B. K. Tiwari (San Diego: Academic Press), 279–287.
Ghamry, M., Zhao, W., and Li, L. (2023). Impact of Lactobacillus apis on the antioxidant activity, phytic acid degradation, nutraceutical value and flavor properties of fermented wheat bran, compared to Saccharomyces cerevisiae and Lactobacillus plantarum. Food Res. Int. 163:112142. doi: 10.1016/j.foodres.2022.112142
Guan, Q., Xiong, T., and Xie, M. (2021). Influence of probiotic fermented fruit and vegetables on human health and the related industrial development trend. Engineering 7, 212–218. doi: 10.1016/j.eng.2020.03.018
Huo, J., and Li, Q. (2012). Determination of thiamazole in pharmaceutical samples by phosphorus molybdenum blue spectrophotometry. Spectrochim. Acta A Mol. Biomol. Spectrosc. 87, 293–297. doi: 10.1016/j.saa.2011.11.056
Karaaslan, M. G., Karaaslan, N. M., and Ates, B. (2018). Investigation of mineral components and antioxidant properties of a healthy red fruit: cornelian cherry (Cornus mas L.). J. Turkish Chem. Soc. 5, 1319–1326. doi: 10.18596/jotcsa.449593
Kechagia, M., Basoulis, D., Konstantopoulou, S., Dimitriadi, D., Gyftopoulou, K., Skarmoutsou, N., et al. (2013). Health benefits of probiotics: a review. ISRN Nutr. 2013:481651, 1–7. doi: 10.5402/2013/481651
Kim, S. Y. (2017). Production of fermented kale juices with Lactobacillus strains and nutritional composition. Prev. Nutr. Food Sci. 22, 231–236. doi: 10.3746/pnf.2017.22.3.231
König, A., Sadova, N., Dornmayr, M., Schwarzinger, B., Neuhauser, C., Stadlbauer, V., et al. (2023). Combined acid hydrolysis and fermentation improves bioactivity of citrus flavonoids in vitro and in vivo. Commun. Biol. 6, 1083–1018. doi: 10.1038/s42003-023-05424-7
Landete, J. M., Curiel, J. A., Rodríguez, H., de las Rivas, B., and Muñoz, R. (2014). Aryl glycosidases from Lactobacillus plantarum increase antioxidant activity of phenolic compounds. J. Funct. Foods 7, 322–329. doi: 10.1016/j.jff.2014.01.028
Lao, Y., Zhang, M., Li, Z., and Bhandari, B. (2020). A novel combination of enzymatic hydrolysis and fermentation: effects on the flavor and nutritional quality of fermented Cordyceps militaris beverage. LWT 120:108934. doi: 10.1016/j.lwt.2019.108934
Laophongphit, A., Siripornadulsil, S., and Siripornadulsil, W. (2023). Improvements in the functions of probiotic-based mango pulp rich in phenolic and proline antioxidants by treatment with pectinase and fermentation with lactic acid bacteria. LWT 181:114756. doi: 10.1016/j.lwt.2023.114756
Li, X., Li, Y., Gao, J., Mi, S., Mao, K., Zhang, T., et al. (2023). Chemical composition of naturally-fermented mixed fruit product and in vitro bioactivities. LWT 181:114771. doi: 10.1016/j.lwt.2023.114771
Liu, H.-M., Han, Y.-F., Wang, N.-N., Zheng, Y.-Z., and Wang, X.-D. (2020). Formation and antioxidant activity of Maillard reaction products derived from different sugar-amino acid aqueous model Systems of Sesame Roasting. J. Oleo Sci. 69, 391–401. doi: 10.5650/jos.ess19336
Liu, W., Mao, X., and Zhou, Z. (2022). Analysis of physicochemical properties, fatty acid composition and antioxidant activity of seed oil extracted from 12 citrus materials. Int. J. Food Sci. Technol. 57, 6480–6491. doi: 10.1111/ijfs.15973
Liu, G., Wu, M., Li, Y., Qayyum, N., Li, X., Zhang, J., et al. (2023). The effect of different pretreatment methods on jujube juice and lactic acid bacteria-fermented jujube juice. LWT 181:114692. doi: 10.1016/j.lwt.2023.114692
Lolli, V., Viscusi, P., Bonzanini, F., Conte, A., Fuso, A., Larocca, S., et al. (2023). Oil and protein extraction from fruit seed and kernel by-products using a one pot enzymatic-assisted mild extraction. Food Chem. X 19:100819. doi: 10.1016/j.fochx.2023.100819
Maisuthisakul, P., Suttajit, M., and Pongsawatmanit, R. (2007). Assessment of phenolic content and free radical-scavenging capacity of some Thai indigenous plants. Food Chem. 100, 1409–1418. doi: 10.1016/j.foodchem.2005.11.032
Mapelli-Brahm, P., Barba, F. J., Remize, F., Garcia, C., Fessard, A., Mousavi Khaneghah, A., et al. (2020). The impact of fermentation processes on the production, retention and bioavailability of carotenoids: an overview. Trends Food Sci. Technol. 99, 389–401. doi: 10.1016/j.tifs.2020.03.013
Marić, M., Grassino, A. N., Zhu, Z., Barba, F. J., Brnčić, M., and Rimac Brnčić, S. (2018). An overview of the traditional and innovative approaches for pectin extraction from plant food wastes and by-products: ultrasound-, microwaves-, and enzyme-assisted extraction. Trends Food Sci. Technol. 76, 28–37. doi: 10.1016/j.tifs.2018.03.022
McKenzie, J. S., Jurado, J. M., and de Pablos, F. (2010). Characterisation of tea leaves according to their total mineral content by means of probabilistic neural networks. Food Chem. 123, 859–864. doi: 10.1016/j.foodchem.2010.05.007
Mditshwa, A., Magwaza, L. S., Tesfay, S. Z., and Opara, U. L. (2017). Postharvest factors affecting vitamin C content of citrus fruits: a review. Sci. Hortic. 218, 95–104. doi: 10.1016/j.scienta.2017.02.024
Mohammadifard, N., Humphries, K. H., Gotay, C., Mena-Sánchez, G., Salas-Salvadó, J., Esmaillzadeh, A., et al. (2019). Trace minerals intake: risks and benefits for cardiovascular health. Crit. Rev. Food Sci. Nutr. 59, 1334–1346. doi: 10.1080/10408398.2017.1406332
Mok, I.-K., Jung, H., Kim, H., and Kim, D. (2023). Biotransformation of ginsenosides from Korean wild-simulated ginseng (Panax ginseng C. A. Mey.) using the combination of high hydrostatic pressure, enzymatic hydrolysis, and sonication. Food. Bioscience 53:102687. doi: 10.1016/j.fbio.2023.102687
Nath, P., Kaur, C., Rudra, S. G., and Varghese, E. (2016). Enzyme-assisted extraction of carotenoid-rich extract from red Capsicum (Capsicum annuum). Agric. Res. 5, 193–204. doi: 10.1007/s40003-015-0201-7
Ni, H., Yang, Y. F., Chen, F., Ji, H. F., Yang, H., Ling, W., et al. (2014). Pectinase and naringinase help to improve juice production and quality from pummelo (Citrus grandis) fruit. Food Sci. Biotechnol. 23, 739–746. doi: 10.1007/s10068-014-0100-x
Nováková, L., Solich, P., and Solichová, D. (2008). HPLC methods for simultaneous determination of ascorbic and dehydroascorbic acids. TrAC 27, 942–958. doi: 10.1016/j.trac.2008.08.006
Oguntoyinbo, F. A., Cho, G.-S., Trierweiler, B., Kabisch, J., Roesch, N., Neve, H., et al. (2016). Fermentation of African kale (Brassica carinata) using L. plantarum BFE 5092 and L. fermentum BFE 6620 starter strains. Int. J. Food Microbiol. 238, 103–112. doi: 10.1016/j.ijfoodmicro.2016.08.030
Peng, W., Meng, D., Yue, T., Wang, Z., and Gao, Z. (2021). Effect of the apple cultivar on cloudy apple juice fermented by a mixture of Lactobacillus acidophilus, Lactobacillus plantarum, and Lactobacillus fermentum. Food Chem. 340:127922. doi: 10.1016/j.foodchem.2020.127922
Rasera, G. B., de Vilhena Araújo, É., Pereira, A. K., Liszbinski, R. B., Pacheco, G., Fill, T. P., et al. (2023). Biotransformation of white and black mustard grains through germination and enzymatic hydrolysis revealed important metabolites for antioxidant properties and cytotoxic activity against Caco-2 cells. Food Res. Int. 169:112881. doi: 10.1016/j.foodres.2023.112881
Ruviaro, A. R., Barbosa, P. P. M., and Macedo, G. A. (2019). Enzyme-assisted biotransformation increases hesperetin content in citrus juice by-products. Food research international, SLACA 2017: food science and its role in a changing world. Food Res. Int. 124, 213–221. doi: 10.1016/j.foodres.2018.05.004
Sanlier, N., Gokcen, B. B., and Sezgin, A. C. (2019). Health benefits of fermented foods. Crit. Rev. Food Sci. Nutr. 59, 506–527. doi: 10.1080/10408398.2017.1383355
Santamaría, R. I., Reyes-Duarte, M. D., Bárzana, E., Fernando, D., Gama, F. M., Mota, M., et al. (2000). Selective enzyme-mediated extraction of capsaicinoids and caratenoids from chili guajillo puya (Capsicum annum L.) using ethanol as solvent. J. Agric. Food Chem. 48, 3063–3067. doi: 10.1021/jf991242p
Santos, K. L., Daccache, I. S. C., and de Castro, R. J. S. (2022). Synergistic action of multiple enzymes resulting in efficient hydrolysis of Banana bracts and products with improved antioxidant properties. PRO 10:1807. doi: 10.3390/pr10091807
Sheldon, R. A., and van Pelt, S. (2013). Enzyme immobilisation in biocatalysis: why, what and how. Chem. Soc. Rev. 42, 6223–6235. doi: 10.1039/c3cs60075k
Sionek, B., Szydłowska, A., Zielińska, D., Neffe-Skocińska, K., and Kołożyn-Krajewska, D. (2023). Beneficial Bacteria isolated from food in relation to the next generation of probiotics. Microorganisms 11:1714. doi: 10.3390/microorganisms11071714
Suliburska, J., and Krejpcio, Z. (2014). Evaluation of the content and bioaccessibility of iron, zinc, calcium and magnesium from groats, rice, leguminous grains and nuts. J. Food Sci. Technol. 51, 589–594. doi: 10.1007/s13197-011-0535-5
Suri, S., Singh, A., and Nema, P. K. (2022). Current applications of citrus fruit processing waste: a scientific outlook. Appl. Food Res. 2:100050. doi: 10.1016/j.afres.2022.100050
Tao, R., Chen, Q., Li, Y., Guo, L., and Zhou, Z. (2022). Physicochemical, nutritional, and phytochemical profile changes of fermented citrus puree from enzymatically hydrolyzed whole fruit under cold storage. LWT 169:114009. doi: 10.1016/j.lwt.2022.114009
Wei, J., Li, Y., Ye, Z., Li, Y., and Zhou, Z. (2023). Citrus carotenoid extracts exert anticancer effects through anti-proliferation, oxidative stress, and mitochondrial-dependent apoptosis in MCF-7 cells. Food Secur. 12:3469. doi: 10.3390/foods12183469
Wistaff, E. A., Beller, S., Schmid, A., Neville, J. J., and Nietner, T. (2021). Chemometric analysis of amino acid profiles for detection of fruit juice adulterations - application to verify authenticity of blood orange juice. Food Chem. 343:128452. doi: 10.1016/j.foodchem.2020.128452
Wu, G. (2009). Amino acids: metabolism, functions, and nutrition. Amino Acids 37, 1–17. doi: 10.1007/s00726-009-0269-0
Yang, W., Liu, J., Zhang, Q., Liu, H., Lv, Z., Zhang, C., et al. (2022). Changes in nutritional composition, volatile organic compounds and antioxidant activity of peach pulp fermented by lactobacillus. Food Biosci. 49:101894. doi: 10.1016/j.fbio.2022.101894
Yang, X., Zhou, J., Fan, L., Qin, Z., Chen, Q., and Zhao, L. (2018). Antioxidant properties of a vegetable-fruit beverage fermented with two Lactobacillus plantarum strains. Food Sci. Biotechnol. 27, 1719–1726. doi: 10.1007/s10068-018-0411-4
Yi, T., Wang, K., Zhuang, Z., and Huang, X. (2014). Comparative analysis of dietary fibre extract isolated from Citrus juice by-products using water extraction, fermentation and enzymatic treatment methods. Adv. J. Food Sci. Technol. 6, 1058–1066. doi: 10.19026/ajfst.6.160
Yuasa, M., Shimada, A., Matsuzaki, A., Eguchi, A., and Tominaga, M. (2021). Chemical composition and sensory properties of fermented citrus juice using probiotic lactic acid bacteria. Food Biosci. 39:100810. doi: 10.1016/j.fbio.2020.100810
Zema, D. A., Calabrò, P. S., Folino, A., Tamburino, V., Zappia, G., and Zimbone, S. M. (2018). Valorisation of citrus processing waste: a review. Waste Manag. 80, 252–273. doi: 10.1016/j.wasman.2018.09.024
Zhao, Z., He, S., Hu, Y., Yang, Y., Jiao, B., Fang, Q., et al. (2017). Fruit flavonoid variation between and within four cultivated Citrus species evaluated by UPLC-PDA system. Sci. Hortic. 224, 93–101. doi: 10.1016/j.scienta.2017.05.038
Keywords: citrus whole fruit utilization, citrus jam, probiotics, fermentation, enzymatically hydrolyzed
Citation: Li Y, Guo L, Mao X, Ji C, Li W and Zhou Z (2024) Changes in the nutritional, flavor, and phytochemical properties of Citrus reticulata Blanco cv. ‘Dahongpao’ whole fruits during enzymatic hydrolysis and fermentation. Front. Sustain. Food Syst. 8:1474760. doi: 10.3389/fsufs.2024.1474760
Edited by:
Pedro Ferreira Santos, University of Minho, PortugalReviewed by:
Lee Sin Chang, USCI University, MalaysiaMalgorzata Ziarno, Warsaw University of Life Sciences, Poland
Liliana Londono Hernandez, National Open and Distance University, Colombia
Copyright © 2024 Li, Guo, Mao, Ji, Li and Zhou. This is an open-access article distributed under the terms of the Creative Commons Attribution License (CC BY). The use, distribution or reproduction in other forums is permitted, provided the original author(s) and the copyright owner(s) are credited and that the original publication in this journal is cited, in accordance with accepted academic practice. No use, distribution or reproduction is permitted which does not comply with these terms.
*Correspondence: Zhiqin Zhou, emhvdXpoaXFpbkBzd3UuZWR1LmNu; Wenji Li, bGl3akBjcWlwYy5lZHUuY24=
†These authors have contributed equally to this work