- 1School of Life Science, Institute of Cellular and Molecular Biology, Jiangsu Normal University, Xuzhou, China
- 2College of Biological and Pharmaceutical Engineering, West Anhui University, Lu'an, China
Introduction: The demand for sustainable food packaging materials has led to the exploration of bioactive composite films. This study aimed to prepare and evaluate a composite film made from sweet potato peel polyphenols extract and sweet potato starch (SPS) for its potential use in food packaging.
Methods: The composite film was prepared by uniformly dispersing 0.4% sweet potato peel polyphenols in the SPS matrix. Physicochemical properties and functional characteristics were assessed, including mechanical properties, UV barrier, water and gas barrier properties, antioxidant activity, and antimicrobial abilities against Bacillus subtilis, Escherichia coli, and Staphylococcus aureus. The film's efficacy in food packaging was tested using fresh cherry tomatoes, stored at 4°C, to determine its impact on shelf life.
Results: The starch-based sweet potato peel polyphenols film demonstrated enhanced mechanical properties and excellent UV barrier properties. It showed improved water and gas barrier properties and strong antioxidant activity, with clearance rates above 90% for DPPH and ABTS radicals. The film also exhibited effective antimicrobial abilities against the tested bacteria. Food packaging experiments indicated that the film could extend the shelf life of fresh cherry tomatoes to 7 days when stored at 4°C.
Discussion: The results suggest that the developed composite film has significant potential as an eco-friendly food packaging material. Its multifunctional properties, including UV protection, barrier enhancement, antioxidant activity, and antimicrobial capabilities, make it a promising candidate for extending the shelf life of perishable foods. The film's performance in slowing spoilage and extending the shelf life of cherry tomatoes highlights its practical application prospects in the food industry.
1 Introduction
In recent years, with the increasing global awareness of environmental protection and the continuous development of food packaging technology, the search for eco-friendly materials to replace traditional plastic packaging has become an important issue in food science and technology. The widespread use of non-degradable plastics has led to high carbon emissions and serious “white pollution,” posing a threat to the environment and public health. In China, there is a growing emphasis on low-carbon environmental protection, and people's requirements for food packaging are shifting toward environmental and safety aspects. Therefore, there is an urgent need to replace traditional plastic packaging with more sustainable, green, active packaging. As green packaging, edible films maintain food quality by controlling oxygen ingress and moisture transfer. Due to their ability to effectively address preservation, environmental protection, and packaging issues, research and application of edible films are becoming increasingly widespread.
Starch, as a natural renewable resource, has garnered increasing attention in the field of food packaging materials due to its abundant and eco-friendly, biodegradable properties, presenting promising development prospects (Madadi et al., 2021; Channab et al., 2023). Corn starch, sweet potato starch (SPS), and potato starch are widely used to prepare edible films (Dhull et al., 2021; Tafa et al., 2023). Studies have shown that pure starch films have limitations in mechanical performance, antioxidant properties, and antibacterial activity, necessitating improving their properties by incorporating other components (Narvaez-Gomez et al., 2021; Sun et al., 2023). For instance, edible films made by Ghasemlou et al. (2013) lack the mechanical properties required for traditional packaging but can be effectively improved by adding other components. Research by Liu et al. found that adding glycerol to SPS can effectively plasticize the film and enhance its mechanical properties. Additionally, composite films prepared by Wei et al., using cauliflower leaf polyphenols and cassava starch/pectin, demonstrate improvements in various aspects compared to pure cassava starch films (Li et al., 2023; Sarka et al., 2023).
Sweet potato (Ipomoea batatas (L.) Lam.) is a herbaceous plant that belongs to the Convolvulaceae family and is an annual or perennial dicotyledonous plant. Commonly known as sweet potato, yam, sweet yam, sweet potato, sweet potato yam, white sweet potato, and white yam, it is recommended as one of the best foods by the World Health Organization (Gao et al., 2021; Han et al., 2021). Consumers highly favor potato sticks, chips, and candied fruits made from fresh sweet potatoes. However, the production process of sweet potato products generates a significant amount of sweet potato peel by-products. While these by-products have been mainly utilized for feed and biogas production in recent years, a large portion still ends up as waste, leading to resource wastage and environmental pollution. Sweet potato peels are rich in polyphenolic compounds, which possess functions such as antioxidation, free radical scavenging, metal ion chelation, and antibacterial activity. Consequently, extracting polyphenols from sweet potato peels can enhance the added value of sweet potatoes and promote the development and utilization of agricultural processing waste.
Fresh fruits, such as cherry tomatoes, as ready-to-eat fruit and vegetable products, have gradually entered consumers' vision with changes in consumer lifestyles and the rapid development of cold chain logistics. However, the shelf life of fresh cherry tomatoes is often short (approximately 1–3 days at 4°C), and factors such as mechanical damage, nutrient loss, and dehydration have limited the development of the fresh fruit ready-to-eat industry. This study aims to develop a new food packaging material—sweet potato skin polyphenol composite film using sweet potato skin polyphenols and sweet potato starch. The study explores the application potential of sweet potato skin polyphenol composite film in fresh fruit preservation using fresh cherry tomatoes as the experimental subject. By adding different concentrations of sweet potato peel polyphenol extracts to SPS and using solution casting to prepare composite films, the effects of the amount of sweet potato peel polyphenols on the physical and chemical properties of the composite films, including optical, mechanical, gas barrier, and thermal stability properties, were investigated. The antioxidant and antibacterial properties of the composite films were also evaluated.
The ultimate goal of this study is to develop an environmentally friendly and efficient food packaging material by fully utilizing by-products from sweet potato processing, promoting the sustainable development of food packaging technology. In the future, further exploration of the applicability of sweet potato peel polyphenol composite films in different types of food and the global promotion of their applications contribute to environmental protection and sustainable resource utilization.
2 Materials and methods
2.1 Materials
Sweet potatoes, yams, SPS, corn starch, and cassava starch were purchased from a local supermarket. Glycerol of food-grade quality was obtained from Lianyungang Xinai Food Technology Co., Ltd. Strains of Staphylococcus aureus, Escherichia coli, and Bacillus subtilis were provided by the Microbial Laboratory of Biology and Pharmaceutical Engineering at Wannan Medical College. Anhydrous analytical grade ethanol was procured from Shanghai Aladdin Chemical Co., Ltd. The DPPH free radical scavenging assay kit and ABTS free radical scavenging assay kit were purchased from Beijing Solabo Technology Co., Ltd. All other chemicals used were of analytical grade and purchased from Shanghai Aladdin Chemical Co., Ltd.
2.2 Extraction of sweet potato peel polyphenols and determination of total phenolic content
Initially, sweet potatoes were treated with a 65% ethanol solution at a 1:25 (g/mL) ratio for 1 h to extract polyphenols from the peel using ultrasonic assistance. The extracted mixture was then filtered, and the filtrate was collected and evaporated to remove ethanol using a vacuum rotary evaporator at 50°C. Subsequently, freeze-drying was performed to obtain a polyphenol-rich dry extract stored at −80°C (Lyly et al., 2004; Wang and Weller, 2006; Dai and Mumper, 2010). The total phenolic content of sweet potato peel was determined using the folin-ciocalteu method. A certain amount of the dry extract was dissolved in deionized water to prepare a sample solution of a specific concentration. Taking 0.5 mL of the sample solution, it was added to a 10 mL test tube along with 2.5 mL folin-ciocalteu reagent (folin-ciocalteu reagent: water = 1:10), mixed, and allowed to stand for 5 min before adding 2.0 mL 7.5% sodium carbonate solution. After thorough mixing, the mixture was left in the dark for 30 min, and the absorbance of the sample was measured at 760 nm using a UV-Vis spectrophotometer. By preparing a series of standard solutions of known gallic acid concentrations and measuring their absorbance, a standard curve was plotted to calculate the gallic acid equivalent (GAE) concentration in the sample solution, eventually converting it to the total phenolic content per gram of the sample. The results indicated a total phenolic content of 19.92 ± 0.13 mg/g in sweet potato peel.
2.3 Preparation of edible starch-sweet potato peel polyphenols films
Edible starch-sweet potato peel polyphenols composite films were fabricated using a solution casting method. Initially, 4.5 g of SPS was dissolved in 100 mL of distilled water, and 2.0 g of glycerol was added as a plasticizer. The mixture was heated at 60°C for 30 min with continuous stirring. Subsequently, a specific amount of sweet potato peel polyphenols powder (at varying w/w ratios of 0%, 0.1%, 0.2%, 0.3%, 0.4%, 0.5%, and 0.6%) was added to the mixture, and the film-forming solution was stirred magnetically at 100°C for 10 min. The solution was then subjected to ultrasonic treatment for 5 min to remove air bubbles and left to stand for 30 min. Following homogenization for 10 min, 15 mL of the film-forming solution was cast on a 9 cm diameter polystyrene dish and dried in a hot air oven at 60°C for 3 h. After drying, the film was gently peeled off from the polystyrene dish. The prepared films were labeled based on the content of sweet potato peel polyphenols as SPS film, SPS/sweet potato peel polyphenols 0.1% (SPS/SPPP 0.1%) film, SPS/sweet potato peel polyphenols 0.2% (SPS/SPPP 0.2%) film, SPS/sweet potato peel polyphenols 0.3% (SPS/SPPP 0.3%) film, SPS/sweet potato peel polyphenols 0.4% (SPS/SPPP 0.4%) film, SPS/sweet potato peel polyphenols 0.5% (SPS/SPPP 0.5%) film, and SPS/sweet potato peel polyphenols 0.6% (SPS/SPPP 0.6%) film. Before preparing the SPS/sweet potato peel polyphenols films, pure starch films of sweet potato, corn, and cassava were prepared using the same procedure (except not adding sweet potato peel polyphenols) to determine the suitable starch type for film preparation through a comparison of the properties of the three pure starch films.
2.4 Characterization of films
2.4.1 Scanning electron microscope
The surface morphology of the film samples was characterized using an SEM, FEI Quanta 250 SEM (FEI Company, USA). Prior to testing, the film samples underwent gold sputter coating to enhance their conductivity.
2.4.2 FT-IR analysis
The surface chemical groups of the edible film samples were characterized using a Fourier Transform Infrared Spectrometer (thermo scientific nicolet iS50 FT-IR, thermo fisher scientific inc., USA) scanning in the wavelength range of 400–6,000 cm−1 with a resolution of 4 cm−1.
2.4.3 XRD
The crystallinity of the films was determined using an X-ray diffraction (XRD) instrument (XRD, Ultima IV, Rigaku, Japan) with Cu-Kα radiation source (λ = 0.154 nm) within the 2θ range of 5° to 85°.
2.4.4 Thickness and moisture content
Smooth, dry, and uniform film samples were overlapped in stacks of four, and five random measurement points were selected to measure the total thickness using a digital caliper. The average thickness of each film was calculated by dividing the total thickness by four. The water content was determined using the direct drying method per the standard procedure (Chinese National Standard GB 5009.3-2010). Film samples cut into approximately 2 cm × 2 cm squares were initially weighed, dried in a hot air oven at 100°C until a constant weight was achieved (indicating a difference of <0.002 g between consecutive weighings), and the moisture content was determined by calculating the percentage of water loss. Each sample was measured in triplicate, and the average was calculated.
2.4.5 Mechanical properties
Flat, uniform, and defect-free film samples were tested for tensile strength using the standard method (Chinese National Standard GB/T 1040.1-2018). The film samples were left in ambient conditions for 24 h before being cut into standard test strips measuring 25 mm × 5 mm, and the width and thickness were recorded. The samples were fixed in the grips of a universal testing machine (Instron 3343, USA), subjected to a tensile force at a rate of 100 mm/min, and the stress-strain curve was recorded until sample fracture to determine the maximum tensile force (Fmax) and elongation at break. Each sample was tested in quintuplicate, and the average values were calculated. The tensile strength (σ) was calculated using Equation (1), and the elongation at break (ε) was calculated using formula.
Where A0 is the initial cross-sectional area of the sample.
Where ε represents the elongation at the film's break, L0 is the initial grip length of the film before stretching, and L is the length at film fracture.
2.4.6 Optical properties
Even defect-free film samples were measured for their average thickness (mm) using a thickness gauge. Then, the transparency of the film samples was determined in the 300 nm to 800 nm wavelength range using a UV-visible spectrophotometer (Ultrospec 200; Scinteck, UK). The transparency value was calculated using Equation (3).
Where absorbance value A = –log T600, T600 is the light transmittance of the film at 600 nm, and d is the average thickness of the film (mm).
2.4.7 Water resistance
The water barrier properties of different films were determined by measuring WS and water contact angle (WCA). The film samples were cut into 1 cm × 1 cm squares for separate WS and WCA testing. In the WS test, the films containing 500 mg were dried at 100°C for 24 h to determine the initial weight. Subsequently, each film was immersed in distilled water at room temperature for 24 h. After drying the samples for another 24 h at 100°C, the non-soluble residue was measured to calculate the WS of the film using formula.
Where W0 (g) and W (g) represent the initial and final dried weights of the film samples, respectively. For the WCA test, the WCA of the film samples was measured using a contact angle analyzer (JC2000D1, Shanghai Zhonghao, China). A volume of approximately 5 μL of distilled water was dispensed onto the film surface using a high-precision syringe or microsyringe, and the WCA was measured after the water droplet stood still on the film surface for 30 seconds. The WCA value for each film sample was the average of at least three measurements at different positions.
2.4.8 Gas barrier properties
The films' water vapor permeability (WVP) testing was performed according to the literature with slight modifications. Using a weighing dish with a diameter of 6.0 cm and a depth of 3.0 cm, 25 mL of water was placed into the dish to provide 100% relative humidity (RH), and the cup was covered with a film layer and sealed with petroleum jelly. The cup and its contents were weighed and kept in a dry incubator at 25°C. The weight loss was measured every 12 h, and the WVP of the film was calculated using formula (5).
Where Δm/Δt is the weight increase (g/s) per unit time, d is the average film thickness (mm), A represents the exposed area of the film surface (m2), and Δp denotes the pressure difference on both sides of the water vapor film (Pa). For each type of film, three replicate samples were measured for WVP. The oxygen permeability (OP) of the films was determined according to the ASTM D1434 standard using a differential pressure gas permeation apparatus (VAC-V2, labthink, China). The film samples were cut into appropriate sizes, ensuring a flat and defect-free surface. The samples were mounted in the permeation device, ensuring good sealing. The experiment conditions were set to 23°C and 50% RH, the equipment was activated, and the oxygen permeation rate was recorded. Three replicate measurements were performed for each film sample, and the average value was taken as the final result.
2.4.9 Antioxidant activity
The antioxidant properties of the films were evaluated using the DPPH and ABTS free radical scavenging methods. The most optimal starch-sweet potato peel edible film was selected based on physical and sensory properties and prepared as a film solution with a concentration of 0.1 mg/mL. Specifically, the film was added to 14 mL of deionized water and dissolved at 60°C. If any insoluble particles were present, the solution was ground using a homogenizer, and different concentrations of the sample solution were prepared using deionized water.
For the DPPH solution, 11.82 mg of DPPH was dissolved in anhydrous ethanol and diluted to 250 mL to obtain a 120 μmol/L DPPH solution, stored in the dark at low temperature. For the ABTS solution, 0.0384 g of ABTS was dissolved in deionized water and diluted to 10 mL to prepare a 7 mmol/L ABTS solution, which was stored in the dark at a low temperature.
The determination of DPPH free radical scavenging activity was referenced with slight modifications. 1.0 mL of sample solution at different concentrations was pipetted into cuvettes, followed by adding 3.0 mL of 120 μmol/L DPPH solution. After thorough mixing, the mixture was left to stand at room temperature for 30 min, and the absorbance was measured at 517 nm, denoted as A1. The absorbance value measured with ethanol instead of the DPPH solution was denoted as A2, and the absorbance measured with deionized water instead of the sample solution was denoted as A0. A blank was prepared by mixing 1.0 mL of deionized water with 3.0 mL of ethanol, and VC was used as a positive control. The calculation formula for the DPPH free radical scavenging rate is as follows:
The method for determining the ABTS free radical scavenging capacity was referenced with slight modifications. Before use, the ABTS stock solution was diluted with deionized water to achieve an absorbance of 0.70 ± 0.02, resulting in the ABTS working solution. Samples of 0.15 mL at different concentrations were pipetted into cuvettes, followed by the addition of 2.85 mL of freshly prepared ABTS working solution with deionized water. After thorough mixing, the reactions were carried out in the dark at room temperature for 10 min, and the absorbance was measured at 734 nm wavelength, denoted as A1. The absorbance value obtained using a deionized water solution instead of the sample solution was denoted as A2. A blank was prepared by mixing a deionized water solution with deionized water, with VC as the positive control. The formula for calculating the ABTS free radical scavenging rate is as follows:
2.4.10 Antimicrobial properties
To assess the antibacterial efficacy of film samples against Staphylococcus aureus, Escherichia coli, and Bacillus subtilis, a 1% fully activated bacterial inoculum was first cultured in LB broth at 37°C with constant shaking (150 rpm) for 12 h. The culture was then diluted with sterile water to a concentration of 106 cfu/mL−1 and stored at 4°C for later use. Following the method described by de Azevedo et al., the cross-streaking technique was employed for testing. The specific steps are as follows: Film strips measuring 10 mm × 60 mm were sterilized under a UV lamp in a laminar flow hood for 30 min and set aside. A loop was used to transfer a small amount of the prepared cultures of Bacillus subtilis, Escherichia coli, and Staphylococcus aureus, and streaked onto the center of an agar nutrient medium plate. Sterilized film strips were then placed in the center of the plate. After incubating the plates at a constant temperature for 24 h, bacterial growth was observed and recorded.
2.5 Fresh tomato preservation application
Fresh small tomatoes that are uniform in size and free from mechanical damage and insect infestation are washed with distilled water and dried. Subsequently, the tomatoes are randomly divided into four groups and packaged in uncovered polyethylene terephthalate (PET) boxes, with the top covered tightly with either low-density polyethylene (LDPE), pure SPS film, or SPS with 4% polyphenols film, while the group left uncovered serves as the control. All samples are stored in a refrigerator at 4°C. Changes in the visual appearance of fresh tomatoes are captured using a digital camera. At 0, 1, 3, 5, and 7 days, the weight loss rate, hardness, and total bacterial count, as three storage indicators, are measured and tracked with three repetitions for each test.
2.5.1 Weight loss rate
The weight loss rate is determined using the weighing method. The weight loss rate is calculated according to formula 8:
In the equation, M0 (g) represents the initial mass of the sample, and Mt (g) represents the remaining mass of the sample after treatment for t days.
2.5.2 Hardness
Hardness measurement was performed using the P/2N type test probe of a texture analyzer in TPA mode. Two points on the cross-section of the sample were sequentially measured, with the maximum stress value recorded during the puncture process as the hardness value. The specific operational procedure included securing the sample on the test platform of the texture analyzer to ensure stability. The P/2N type test probe punctured two points on the sample in TPA mode, recording the maximum stress value during each puncture process. Each sample was measured five times in parallel, and the average was taken as the final hardness value (N).
2.5.3 Total bacterial count
The total bacterial count was determined with slight modifications based on the standard method (Chinese National Standard GB 4789.2-2022). The specific steps were as follows: homogenize the sample, prepare a series of dilutions, apply an appropriate amount of dilution on agar plates, and culture at 37°C for 48 h to count the resulting colonies. The total bacterial count in each gram of the sample was calculated based on dilution factors and colony-forming units per gram (cfu/g).
2.6 Statistical analysis
Data analysis was conducted using SPSS 22.0 software (SPSS Inc., Chicago, Illinois, USA). All experiments were repeated at least three times, and the experimental data were expressed as mean ± standard deviation (Mean ± SD). Analysis of variance (ANOVA) was performed using the Duncan multiple comparison test for significance testing, with the significance level set at p < 0.05.
3 Results
3.1 Determination of the optimal starch type for edible film preparation
To assess the performance of edible films prepared from different starch sources, the microstructure of various starch film surfaces was observed through SEM. As shown in Figures 1a–c, pure corn starch films exhibited a smooth, uniform, dense surface without voids, cracks, or obvious aggregates. In contrast, pure cassava starch films showed unevenness and the presence of granular substances (Figures 1d–f). On the other hand, pure SPS films displayed a relatively uniform microstructural surface (Figures 1g–i). The thickness, moisture content, mechanical properties, WVP, and transparency of the edible films made from different starch sources are presented in Table 1. There were no significant differences in thickness and moisture content among the edible films prepared from the three different starch sources. Regarding mechanical properties, the tensile strength of sweet potato starch film is approximately 139.29 MPa, significantly higher than that of corn starch film (63.83 MPa) and cassava starch film (44.05 MPa), indicating higher mechanical performance. The water vapor transmission rate test results show that the water vapor transmission rate of sweet potato starch film is 23.34 g/(m2·24 h), significantly lower than that of corn starch film (46.29 g/(m2·24 h) and cassava starch film [25.23 g/(m2·24 h)]. Furthermore, the light transmittance at 600 nm of sweet potato starch film (T600) is 92.04%, higher transparency than corn starch and cassava starch films (Table 1). In summary, sweet potato starch film exhibits excellent performance in surface structure, mechanical properties, water vapor transmission rate, and light transmittance, making it the optimal starch material for preparing edible films.
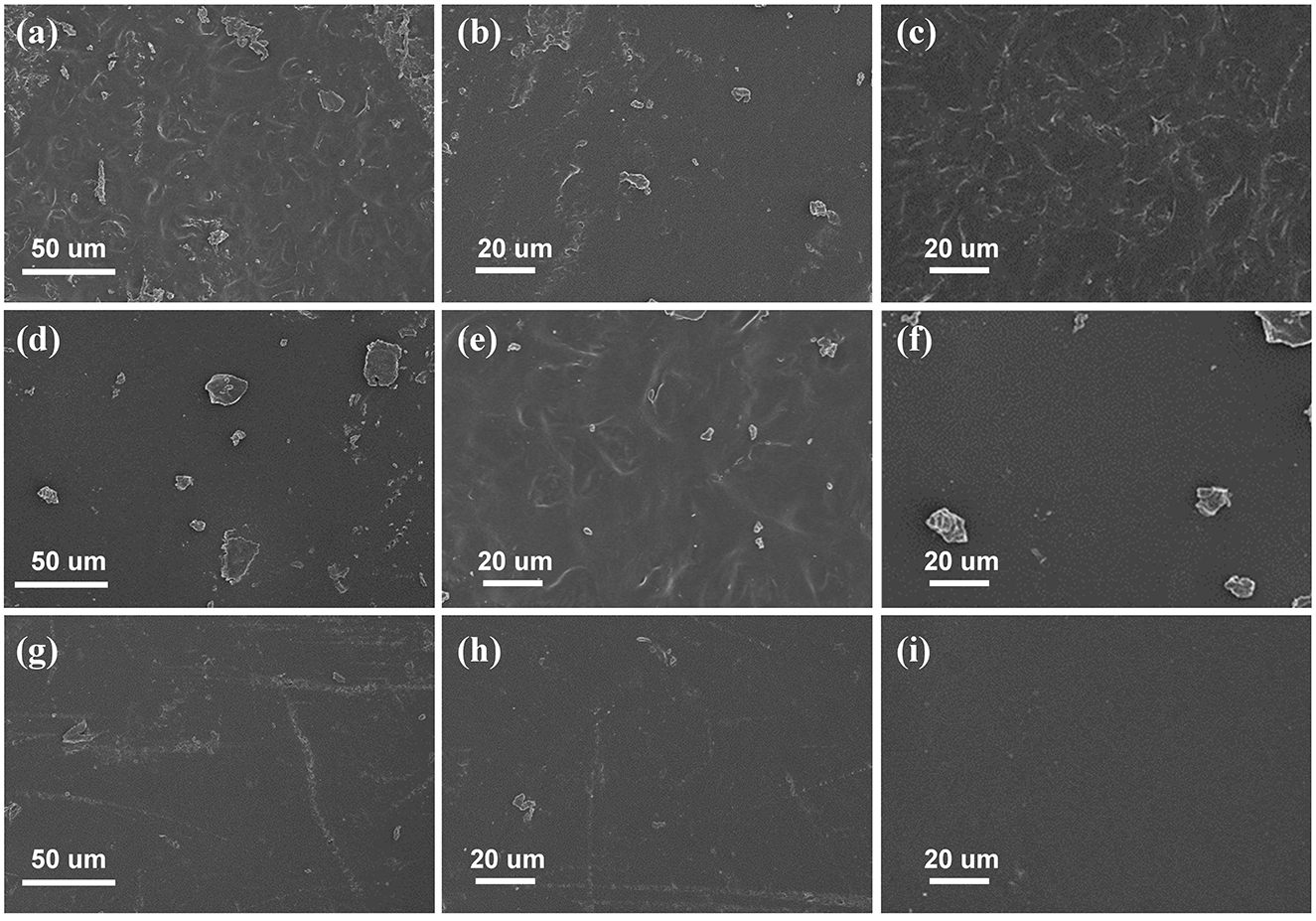
Figure 1. SEM images of various starch film surfaces. (a–c) Corn starch film, (d–f) Cassava starch film, and (g–i) SPS film.

Table 1. Thickness, moisture content, mechanical properties, water vapor permeability, and light transmittance of different types of edible starch films.
3.2 Characterization of SPS-sweet potato peel polyphenols edible film
3.2.1 Surface morphology
The microstructure of SPS-sweet potato peel polyphenols edible films with varying concentrations of sweet potato peel polyphenols was observed using SEM. As shown in Figure 2a, the surface of the pure SPS film appeared smooth, uniform, and dense, without voids, cracks, or aggregates. After adding a small amount of sweet potato skin polyphenols (0.1%) to the sweet potato starch matrix, the film surface remains uniformly flat in appearance (Figure 2b). With increased added sweet potato skin polyphenols, non-uniformity gradually appears on the film surface (Figures 2c–g). The sweet potato starch film containing 0.4% skin polyphenols exhibits a uniform surface microstructure (Figure 2e). In contrast, granular substances are observed at concentrations of 0.5% and 0.6% (Figures 2f, g).
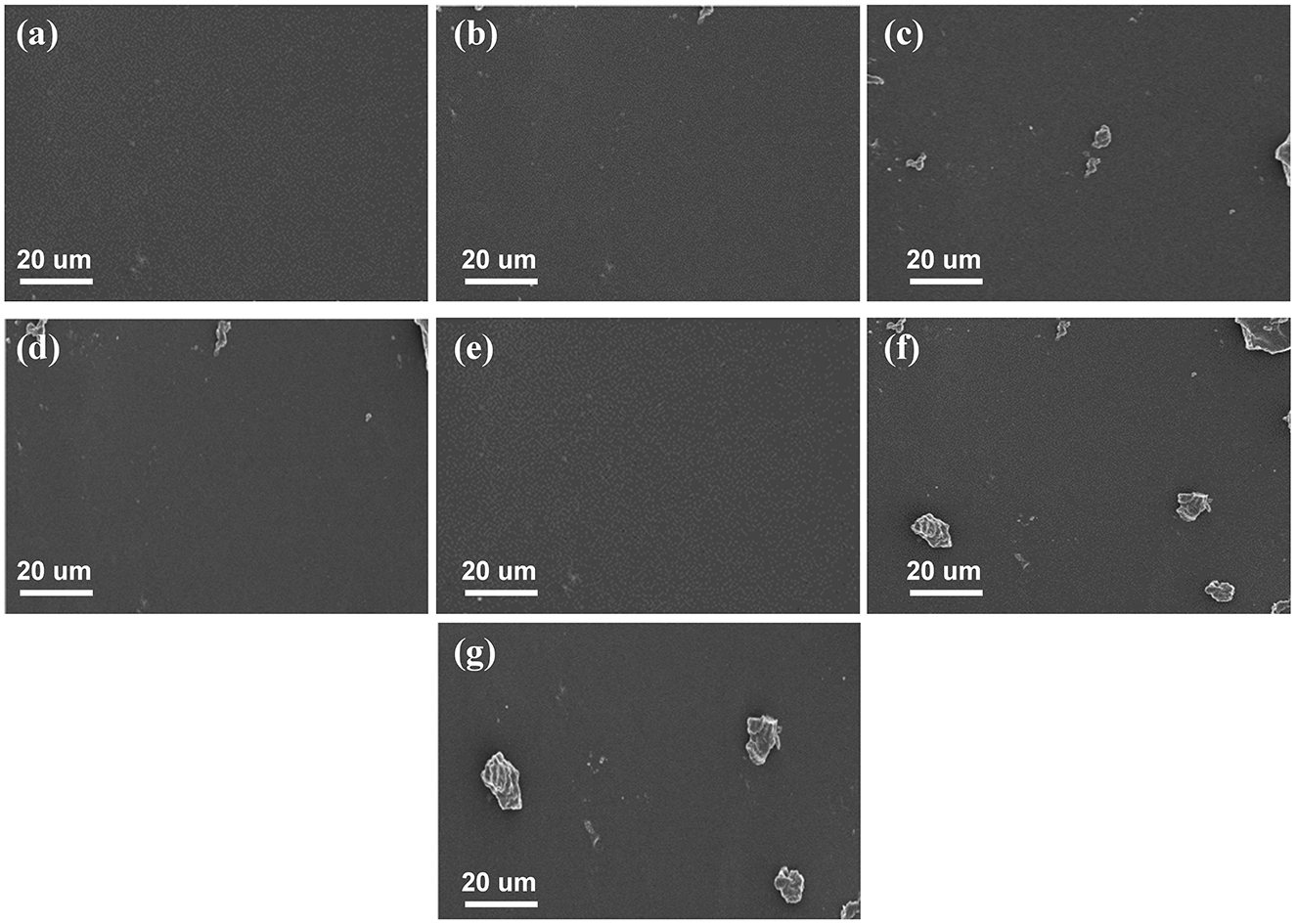
Figure 2. SEM surface morphology image of SPS film with various concentrations of sweet potato peel polyphenols. (a) SEM image of pure SPS film, (b–g) SEM images of SPS edible film incorporating 0.1% to 0.6% sweet potato peel polyphenols.
3.2.2 FT-IR analysis
The chemical group changes in the edible film samples of SPS with different concentrations of sweet potato peel polyphenols were studied through FT-IR analysis, as shown in Figure 3a. The FT-IR spectrum of pure SPS film exhibits a broad absorption peak at 3,400~3,500 cm−1, corresponding to the stretching vibration of O-H groups (Fu et al., 2021). The peaks at 2,844 and 2,855 cm−1 are attributed to the C-H stretching vibration of methyl in starch (Roy and Rhim, 2020; Higashi et al., 2021). The peaks at 1,416 and 1,598 cm−1 correspond to the asymmetric and symmetric stretching vibrations of -COOH in SPS molecules (Li et al., 2024a). The peaks at 1,303 and 1,320 cm−1 belong to the stretching vibration of C-O-C, which is a characteristic peak of the polysaccharide backbone (Liu et al., 2022). After incorporating sweet potato peel polyphenols into the SPS film, compared to the pure SPS film, the functional groups did not show significant changes, but the O-H characteristic peak slightly widened and showed a slight redshift. With the increase in the amount of sweet potato peel polyphenols added, the O-H characteristic peak further shifted to lower wavenumbers, and the shift increased until reaching a minimum at a content of 0.4%, possibly due to the rich -OH and -COOH groups on sweet potato peel polyphenols forming hydrogen bonds with oxygen-containing groups in SPS (Khoshkalampour et al., 2023). When the content of sweet potato peel polyphenols further increased to 0.5% and 0.6%, the hydroxyl peak slightly shifted to higher wavenumbers.
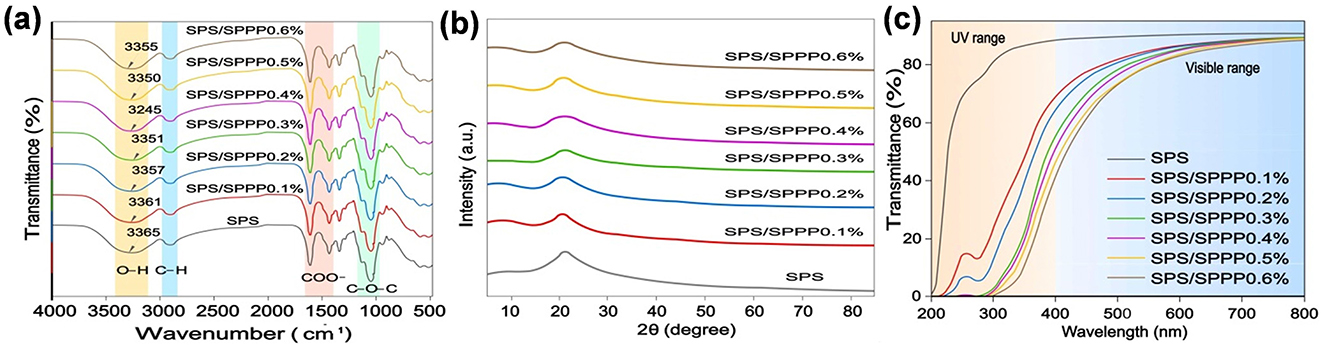
Figure 3. Spectra of SPS edible film samples incorporated with different sweet potato peel polyphenols concentrations. (a) FT-IR spectra, (b) XRD spectra, and (c) UV-visible transmittance spectra.
3.2.3 XRD analysis
XRD was used to analyze the crystal structure of edible film samples of SPS with different concentrations of sweet potato peel polyphenols. The results are shown in Figure 3b, where the pure SPS film exhibits a distinct broad diffraction peak near 2θ = 25.1°, indicating the presence of an amorphous phase in the SPS film (Yue et al., 2023). Adding sweet potato polyphenols to sweet potato starch edible films results in lower characteristic amorphous peak intensity. As the concentration of sweet potato polyphenols increases from 0.1% to 0.4%, the characteristic diffraction peaks of sweet potato starch edible films gradually widen and decrease in intensity. After adding 0.5% and 0.6% sweet potato polyphenols, there is a slight increase in the intensity of the characteristic diffraction peaks. These results indicate that the addition of sweet potato polyphenols has a significant impact on the crystalline structure of sweet potato starch films.
3.2.4 Mechanical properties
The mechanical properties of edible films assess their ability to withstand physical damage during transportation and storage. The results show (Table 2) that the thickness of pure sweet potato starch film is approximately 0.097 mm. When sweet potato polyphenols are added at concentrations of 0.1%, 0.2%, 0.3%, and 0.4%, there is no significant change in the thickness of the edible film. With an increase in sweet potato polyphenol concentration to 0.5% and 0.6%, there is a slight increase in the impact on the thickness of the edible film. In terms of mechanical strength, after adding 0.1% sweet potato polyphenols, the fracture elongation, tensile strength, and elastic modulus of sweet potato starch edible films increased. As the concentration of sweet potato peel polyphenols increases, the mechanical strength of the SPS edible film is further enhanced. The optimal mechanical strength is achieved when the concentration of sweet potato peel polyphenols reaches 0.4%. Specifically, compared to the pure SPS film, adding 0.4% Sweet potato peel polyphenols increases the fracture elongation by 104.45%, tensile strength by 6.273%, and elastic modulus by 24.502%.
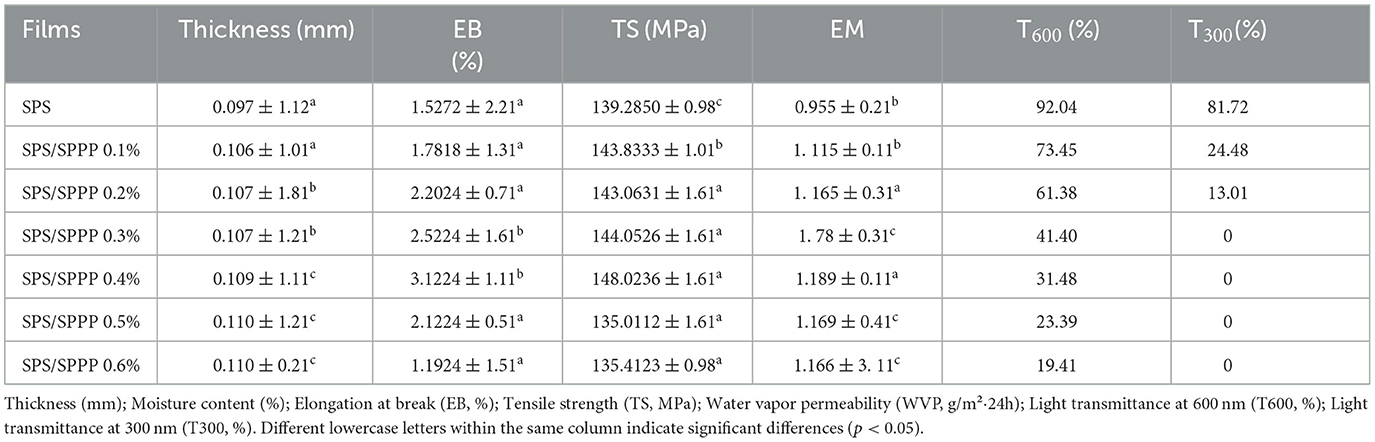
Table 2. Comparison of properties of sweet potato starch films with different concentrations of sweet potato peel polyphenols.
3.2.5 Optical properties
Packaging materials with low UV transmittance are essential for delaying nutrient loss and food deterioration due to photooxidation, while materials with high visible light transmittance are crucial for transparent packaging applications. This study evaluated the effect of incorporating different concentrations of sweet potato peel polyphenols into SPS edible films on the UV-visible transmittance spectra in the wavelength range of 200–800 nm to assess the film's light transmittance at different wavelengths. As shown in Figure 3c, the transmittance of the pure SPS film is highest, and it significantly decreases (p < 0.05) after the addition of sweet potato peel polyphenols, particularly in the 200–400 nm range. Evaluating the transmittance at 300 nm and 600 nm wavelengths (denoted as T300 and T600, respectively) for film samples assessed the UV-blocking ability and transparency of the edible films. The results (Table 2) show that the T300 and T600 values for the pure SPS film are 81.72% and 92.04%, respectively. After adding 0.1% Sweet potato peel polyphenols, these values decrease to 24.48% and 73.45%, respectively. Significantly (p < 0.05), they decrease to 0% and 19.41% as the sweet potato peel polyphenols content is further increased to 0.6%.
3.2.6 WS
Researching the WS and WCA of biopolymer films is crucial for understanding their hydrophilicity and practical applications (Mousazadeh et al., 2021). Figures 4a, b present the WS and WCA of various edible film samples. The pure SPS film exhibits the highest WS at 90.40% and the lowest WCA at 51.72°, attributed to the inherent hydrophilicity of SPS (de Castro et al., 2024). Upon introducing sweet potato peel polyphenols, the WS of the edible film significantly decreases (p < 0.05) while the WCA increases. Specifically, the optimal WS is observed in the SPS film containing 0.4% Sweet potato peel polyphenols, with a WS of 45.89% and a WCA of 82.32°.
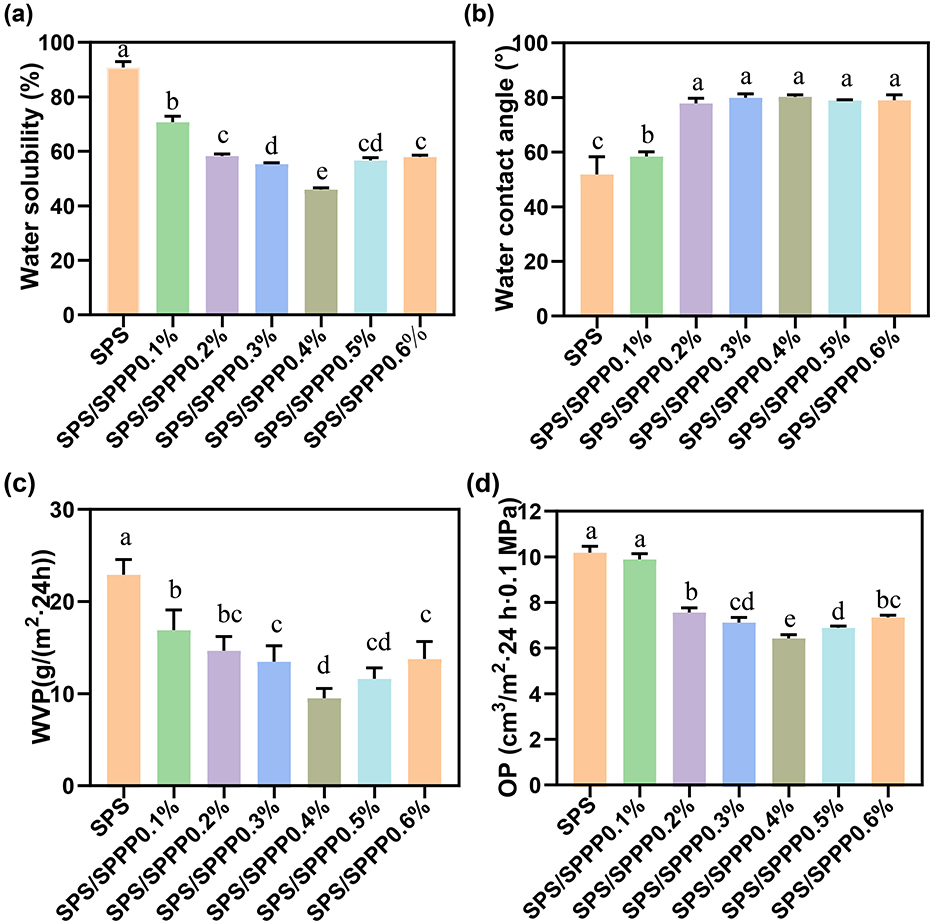
Figure 4. SPS edible film samples incorporated with different concentrations of sweet potato peel polyphenols. (a) WS, (b) WCA, (c) WVP, and (d) OP. Different lowercase letters indicate statistical significance (p < 0.05) in the observed differences.
3.2.7 Gas barrier properties
We further investigated the gas barrier properties. Figures 4c, d depict the WVP and OP of sweet potato peel polyphenols-incorporated SPS edible films at different concentrations. The pure SPS film exhibits the lowest barrier properties against water vapor and oxygen, with a WVP of 22.92 g/(m2·24 h) and an OP of 10.21 cm3/m2·24 h·0.1 MPa. With an increase in sweet potato peel polyphenols concentration, the WVP and OP sharply decrease, followed by a slight increase. When 0.4% of sweet potato peel polyphenols are added, the WVP and OP reach their lowest values of 9.45 g/(m2·24 h) and 6.43 cm3/m2·24 h·0.1 MPa, respectively.
3.2.8 Antioxidant activity
The antioxidant activity of food packaging films plays a significant role in reducing the adverse effects of free radicals on food nutrients and extending the shelf life of packaged foods. The antioxidant performance of starch-based films was evaluated by clearing DPPH and ABTS free radicals, as shown in Figure 5. The pure SPS film exhibits very weak antioxidant activity, with clearance rates of 3.3% for DPPH and 10.2% for ABTS. In contrast, the SPS with sweet potato peel polyphenols edible film shows a significantly enhanced (p < 0.05) clearance ability against DPPH and ABTS, reaching up to 90.32% and 93.6%, respectively.
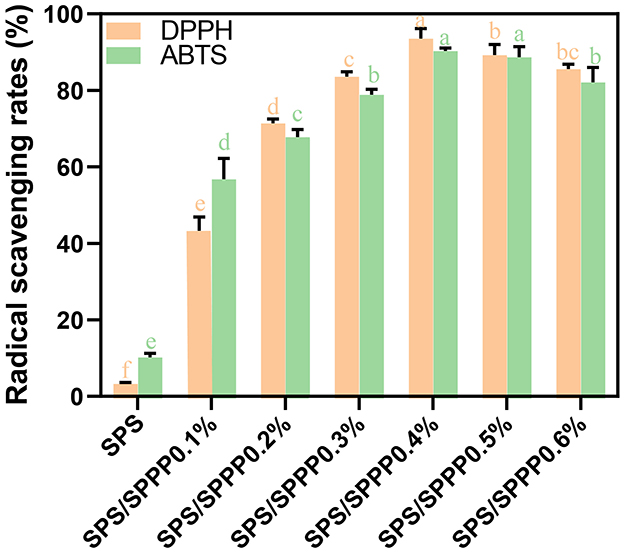
Figure 5. Free radical scavenging rate of DPPH and ABTS in SPS edible film samples incorporated with different concentrations of sweet potato peel polyphenols. Different lowercase letters indicate statistical significance (p < 0.05) in the observed differences.
3.2.9 Antimicrobial activity
This study employed a total viable count method to investigate the bacteriostatic effects of film samples against Gram-negative bacteria (Escherichia coli) and Gram-positive bacteria (Bacillus subtilis, Staphylococcus aureus). As shown in Figure 6, the bacteriostatic effect of pure SPS film is negligible, while SPS edible film incorporated with sweet potato peel polyphenols exhibits a significantly enhanced bacteriostatic effect against the three types of bacteria. Among them, the SPS film with 0.4% sweet potato peel polyphenols demonstrates the optimal bacteriostatic efficacy, with inhibition rates of 76.6% for Escherichia coli, 91.2% for Bacillus subtilis, and 86.6% for Staphylococcus aureus. When 0.4% sweet potato peel polyphenols are incorporated, the bacteriostatic effect is the best.
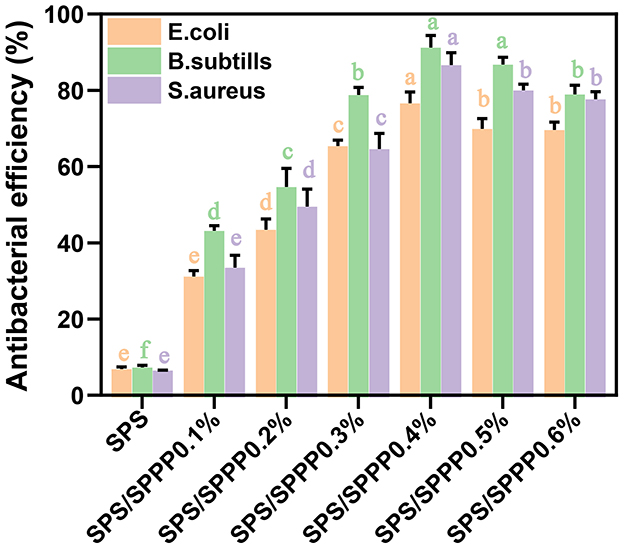
Figure 6. Antimicrobial activity of SPS edible film samples incorporated with different concentrations of sweet potato peel polyphenols. Different lowercase letters indicate statistical significance (p < 0.05) in the observed differences.
3.3 Packaging application of fresh cherry tomatoes
Fresh cherry tomatoes packaged with various films gradually lose water during storage due to respiration and evaporation (Figure 7a). On the 7th day, the control group exhibited a weight loss of 17.98%, significantly higher than the packaging groups (5.5% to 12.8%) (p < 0.05). The SPS/SPPP 0.4% film group demonstrated a weight loss rate below 15.0% on day 7. Hardness is also a critical criterion for determining the quality and freshness of fresh cherry tomatoes. The impact of different packaging on the hardness of fresh cherry tomatoes is illustrated in Figure 7b. The hardness of fresh cherry tomatoes in all groups gradually decreased with storage time. The primary factors causing the softening of fresh cherry tomatoes include respiration, water loss, cell wall deformation due to cell wall-degrading enzymes, and structural damage caused by bacterial growth (Xie et al., 2022). At the end of preservation, the control group exhibited the lowest hardness for fresh cherry tomatoes (7.5 N), while the fresh cherry tomatoes in the SPS film, LDPE film, and SPS/SPPP 0.4% film groups had hardness values of 14.5, 23.6, and 26.3 N, respectively, indicating the presence of protective packaging. Regarding total bacterial count, the SPS/SPPP 0.4% film demonstrated the most significant antibacterial effect, with a total bacterial count of approximately 3 log CFU/g (Figure 7c).
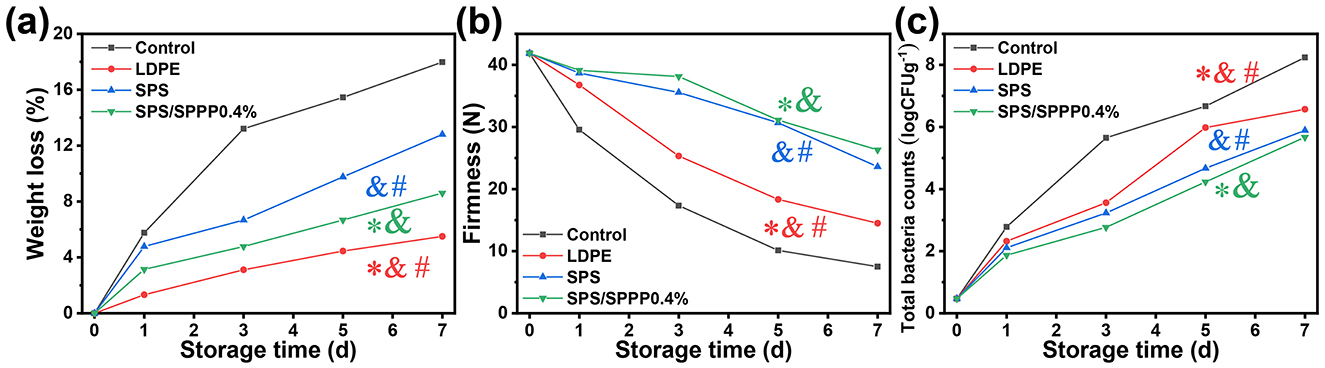
Figure 7. Changes in weight loss rate (a), hardness (b), and total bacterial count (c) of fresh cherry tomatoes packaged in different films during storage period. *Denotes p < 0.05 compared to the Control group; &denotes p < 0.05 compared to the LDPE group; #denotes p < 0.05 compared to the SPS group. Differences with p < 0.05 are deemed statistically significant.
4 Discussion
In recent years, the field of food packaging has increasingly focused on the development of environmentally friendly and functional materials. Traditional synthetic packaging materials have been gradually abandoned by researchers due to their non-biodegradability and environmental pollution. Sweet potato peel, as a by-product of sweet potato processing, is rich in polyphenols and other bioactive substances, with potential antioxidant and antimicrobial properties (Das et al., 2019; Lado et al., 2021). Combining sweet potato peel polyphenols with SPS to prepare edible composite films not only achieves waste utilization but also provides a new solution for food preservation.
In this study, we developed sweet potato polyphenol composite films and evaluated their potential application in preserving fresh fruits. Mechanical property tests showed that after adding 0.4% sweet potato polyphenols, the tensile strength and elongation of the composite film significantly increased. This may be due to the dense molecular network structure formed by the -OH and -COOH groups in polyphenols bonding through hydrogen bonds with oxygen-containing groups in sweet potato starch, enhancing the film's rigidity and toughness. Similar to findings in other studies, natural polyphenols can enhance the film's structural stability and mechanical strength by forming hydrogen bonds with starch molecules. However, as the polyphenol concentration increases to 0.5% and 0.6%, there is a slight decrease in mechanical strength, possibly because high concentrations of polyphenols aggregate in the matrix, forming uneven microstructures that weaken the interaction between polyphenols and starch matrix, leading to uneven stress distribution within the film.
Our optical property studies have revealed a significant reduction in ultraviolet transmittance of the composite film with the addition of sweet potato polyphenols, especially in the 200–400 nm range, which is crucial for protecting food from UV-induced oxidation (Abdullah et al., 2022; Singh et al., 2022; Li et al., 2024b). This reduction can be attributed to the ability of sweet potato polyphenols, containing many benzene rings and phenolic hydroxyl groups, to improve UV absorption through π → π and n → π transitions. Additionally, while the addition of polyphenols slightly decreases the visible light transmittance of the film, transparency effects are not significant below 0.4%, making it suitable for visual food packaging.
Furthermore, our research has shown that the addition of sweet potato polyphenols significantly enhances the gas barrier properties of the composite film. As the polyphenol concentration increased from 0.1% to 0.4%, the water vapor and oxygen permeability of the composite film decreased significantly, mainly attributed to the hydrogen bond interaction between polyphenols and the starch matrix, resulting in a denser internal structure of the film (Chen et al., 2023; Li Y. et al., 2024). However, when the polyphenol concentration exceeded 0.4%, a slight decrease in barrier properties was observed due to the formation of polyphenol aggregation areas in the film. This phenomenon is consistent with the trend of crystalline structural changes observed in XRD analysis.
Antioxidant and antibacterial properties are critical indicators for assessing the application of edible films in food preservation. Experimental results demonstrate that the composite film exhibits over 90% removal rates for DPPH and ABTS free radicals, comparable to or even better than results from using different natural antioxidants in other studies (Ahammed et al., 2023; Liu et al., 2023). This significant enhancement is mainly attributed to the abundant phenolic hydroxyl groups in sweet potato peel. These groups can eliminate free radicals by generating phenoxyl radicals, thus slowing down food oxidation and deterioration (Argenziano et al., 2022; Chen et al., 2023; Xu et al., 2023). Furthermore, antibacterial circle experiments have shown that after adding 0.4% sweet potato peel polyphenols, the composite film exhibits significantly enhanced inhibitory effects on Escherichia coli, Bacillus subtilis, and Staphylococcus aureus. Some sweet potato peel polyphenol components may disrupt the integrity of bacterial cell membranes, inhibiting their growth and reproduction (Riaz et al., 2018). These results further demonstrate the practical application value of sweet potato peel polyphenol composite films in extending the shelf life of food, highlighting significant advantages in food preservation.
Testing in food packaging applications is a crucial step to validate the actual performance of the composite film (Huang et al., 2022; Venkatesan et al., 2023; Wiszumirska et al., 2023). Results show that cherry tomatoes packaged in composite films with sweet potato peel polyphenols maintained good freshness after 7 days, while the control group exhibited significant decay under the same conditions. This may be attributed to the comprehensive performance advantages of the composite film, including mechanical strength, ultraviolet barrier properties, water vapor barrier properties, and antioxidant and antibacterial activities. The packaging material's low water vapor transmission rate indicates good moisture resistance, extending the shelf life of the food, which is more suitable for the preparation of starch-based edible films. Compared to traditional synthetic materials such as low-density polyethylene (LDPE), LDPE film groups have excellent water vapor barrier properties. Although its water vapor and oxygen barrier properties are slightly lower, its environmental friendliness and biodegradability give it a significant advantage in future packaging material development.
Traditional synthetic materials such as polyethylene and polypropylene, despite their good mechanical and barrier properties, pose serious environmental pollution due to their non-biodegradability (Sopher et al., 2015). At the same time, the excellent transparency of sweet potato polyphenol composite film is suitable for visual display in food packaging, which is related to the degree of starch molecular gelatinization with different compositions. After considering the differences in elemental composition, surface characteristics, mechanical properties, water vapor transmission rate, and light transmittance of three types of starch, it was found that sweet potato starch edible film forms a dense structure without gaps and protrusions, with significantly higher tensile strength, water vapor transmission rate, and light transmittance compared to corn starch and cassava starch edible films. Therefore, sweet potato starch is the most suitable material among these three sources for preparing starch edible films. Furthermore, by utilizing by-products from food processing like sweet potato peel, resources wastage is effectively reduced through waste utilization. This eco-friendly feature positions sweet potato peel polyphenol composite films with significant application prospects in the future development of food packaging materials.
From a scientific perspective, this study presents an innovative approach to utilizing sweet potato peel as a by-product in food processing, contributing to resource recycling and enhancing the functionality of food packaging materials through the natural antioxidant and antimicrobial properties of polyphenols. Developing this eco-friendly edible composite film provides an effective solution for food preservation, reducing food spoilage and waste, and mitigating environmental pollution. While the application of this film has been limited to a small variety of fruits in the experimental phase, it theoretically holds promise for extending to the preservation of various fruits and other perishable foods.
Although this study achieved positive results in cherry tomato preservation, its limitations are apparent. Firstly, the research was confined to a limited range of fruit types (cherry tomatoes) and a short preservation period (7 days). Furthermore, despite the impressive preservation enhancement demonstrated by sweet potato peel polyphenols extracts, challenges related to cost and extraction efficiency may arise during actual production and commercialization. Thus, further research and optimization are required regarding cost-effectiveness and the feasibility of large-scale production. Lastly, more research is needed on the composite film's biodegradability and long-term environmental impacts to ensure its sustainable widespread application.
Future research directions could encompass various aspects. Firstly, the scope of research should be expanded to include a broader range of fruit types and perishable food categories to comprehensively assess the preservation effects and applicability of the composite film. Secondly, exploring more efficient polyphenol extraction and membrane preparation methods to reduce production costs and optimize processes. Additionally, evaluating and optimizing the biodegradability of the composite film to ensure safe decomposition after practical use, minimizing environmental impacts, which is crucial for promoting its commercial application. Lastly, conducting long-term preservation studies and market acceptance surveys to better meet market demands and consumer expectations, thus advancing the development of sustainable packaging materials.
5 Conclusion
This study successfully prepared sweet potato peel polyphenols-starch composite film and comprehensively evaluated its application effectiveness in fresh fruit preservation. Sweet potato starch edible film containing sweet potato polyphenols showed excellent performance in various aspects, especially the sweet potato starch film with 0.4% sweet potato polyphenol exhibited the best optical properties, mechanical properties, gas barrier properties, and antimicrobial properties. Therefore, the sweet potato starch film with 0.4% sweet potato polyphenol was selected as the optimal film sample for fresh preservation packaging application, abbreviated as SPS/SPPP 0.4%. This composite film has outstanding antioxidant activity and antimicrobial effects, effectively extending the shelf life of fresh cherry tomatoes under low-temperature conditions. These findings suggest that the sweet potato peel polyphenols-starch composite film, a novel eco-friendly food packaging material, possesses excellent preservation performance and potential commercial value (Figure 8).
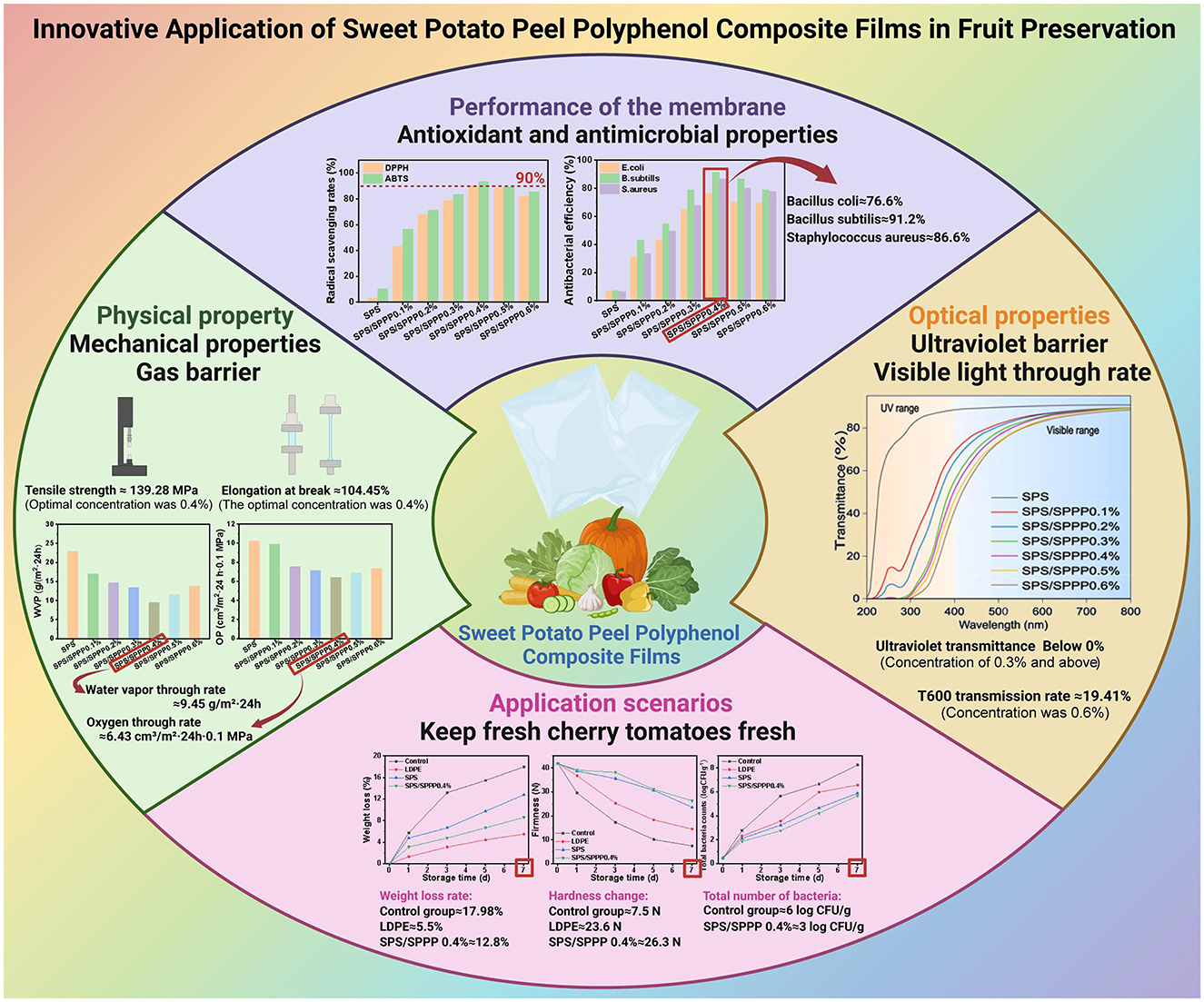
Figure 8. Innovative application of sweet potato peel polyphenols composite film in fruit preservation.
Data availability statement
The original contributions presented in the study are included in the article/supplementary material, further inquiries can be directed to the corresponding author.
Author contributions
ZZ: Conceptualization, Data curation, Methodology, Validation, Visualization, Writing – original draft. YW: Data curation, Formal analysis, Investigation, Methodology, Validation, Writing – original draft. XF: Data curation, Formal analysis, Investigation, Resources, Visualization, Writing – review & editing. XC: Data curation, Formal analysis, Investigation, Methodology, Visualization, Writing – original draft. ZY: Data curation, Formal analysis, Validation, Visualization, Writing – review & editing. CZ: Conceptualization, Funding acquisition, Project administration, Resources, Software, Supervision, Validation, Writing – review & editing.
Funding
The author(s) declare financial support was received for the research, authorship, and/or publication of this article. This study was supported by the Fund of Traditional Chinese Medicine Institute of Anhui Dabie Mountain (National Key R&D Program of China) 2023YFC3503804.
Conflict of interest
The authors declare that the research was conducted in the absence of any commercial or financial relationships that could be construed as a potential conflict of interest.
Publisher's note
All claims expressed in this article are solely those of the authors and do not necessarily represent those of their affiliated organizations, or those of the publisher, the editors and the reviewers. Any product that may be evaluated in this article, or claim that may be made by its manufacturer, is not guaranteed or endorsed by the publisher.
References
Abdullah Cai, J., Hafeez, M. A., Wang, Q., Farooq, S., Huang, Q., et al. (2022). Biopolymer-based functional films for packaging applications: a review. Front. Nutr. 9:1000116. doi: 10.3389/fnut.2022.1000116
Ahammed, S., Easdani, M., Liu, F., and Zhong, F. (2023). Encapsulation of tea polyphenol in zein through complex coacervation technique to control the release of the phenolic compound from gelatin-zein composite film. Polymers (Basel) 15:2882. doi: 10.3390/polym15132882
Argenziano, R., Alfieri, M. L., Arntz, Y., Castaldo, R., Liberti, D., Maria Monti, D., et al. (2022). Non-covalent small molecule partnership for redox-active films: beyond polydopamine technology. J. Colloid Interface Sci. 624, 400–410. doi: 10.1016/j.jcis.2022.05.123
Channab, B. E., El Idrissi, A., Zahouily, M., Essamlali, Y., and White, J. C. (2023). Starch-based controlled release fertilizers: a review. Int. J. Biol. Macromol. 238:124075. doi: 10.1016/j.ijbiomac.2023.124075
Chen, N., Gao, H. X., He, Q., and Zeng, W. C. (2023). Potato starch-based film incorporated with tea polyphenols and its application in fruit packaging. Polymers (Basel) 15:588. doi: 10.3390/polym15030588
Dai, J., and Mumper, R. J. (2010). Plant phenolics: extraction, analysis and their antioxidant and anticancer properties. Molecules 15, 7313–7352. doi: 10.3390/molecules15107313
Das, G., Patra, J. K., Basavegowda, N., Vishnuprasad, C. N., and Shin, H. S. (2019). Comparative study on antidiabetic, cytotoxicity, antioxidant and antibacterial properties of biosynthesized silver nanoparticles using outer peels of two varieties of Ipomoea batatas (L.) Lam. Int. J. Nanomedicine 14, 4741–4754. doi: 10.2147/IJN.S210517
de Castro, L., Silva, L. G. L., Abreu, I. R., Braz, C. J. F., Rodrigues, S. C. S., Moreira-Araujo, R., et al. (2024). Biodegradable PBAT/PLA blend films incorporated with turmeric and cinnamomum powder: a potential alternative for active food packaging. Food Chem. 439:138146. doi: 10.1016/j.foodchem.2023.138146
Dhull, S. B., Bangar, S. P., Deswal, R., Dhandhi, P., Kumar, M., Trif, M., et al. (2021). Development and characterization of active native and cross-linked pearl millet starch-based film loaded with fenugreek oil. Foods 10:3097. doi: 10.3390/foods10123097
Fu, X., Li, G., Cai, S., Yang, H., Lin, K., He, M., et al. (2021). Color-switchable hybrid dots/hydroxyethyl cellulose ink for anti-counterfeiting applications. Carbohydr. Polym. 251:117084. doi: 10.1016/j.carbpol.2020.117084
Gao, Z., Hu, Y., Han, M., Xu, J., Wang, X., Liu, L., et al. (2021). Effects of continuous cropping of sweet potatoes on the bacterial community structure in rhizospheric soil. BMC Microbiol. 21:102. doi: 10.1186/s12866-021-02120-6
Ghasemlou, M., Aliheidari, N., Fahmi, R., Shojaee-Aliabadi, S., Keshavarz, B., Cran, M. J., et al. (2013). Physical, mechanical and barrier properties of corn starch films incorporated with plant essential oils. Carbohydr. Polym. 98, 1117–1126. doi: 10.1016/j.carbpol.2013.07.026
Han, B., Jiang, P., Jiang, L., Li, X., and Ye, X. (2021). Three phytosterols from sweet potato inhibit MCF7-xenograft-tumor growth through modulating gut microbiota homeostasis and SCFAs secretion. Food Res. Int. 141:110147. doi: 10.1016/j.foodres.2021.110147
Higashi, B., Mariano, T. B., Alves de Abreu Filho, B., Goncalves, R. A. C., and Braz de Oliveira, A. J. (2021). Corrigendum to: Effects of fructans and probiotics on the inhibition of Klebsiella oxytoca and the production of short-chain fatty acids assessed by NMR spectroscopy. Carbohydr. Polym. 260:117568. doi: 10.1016/j.carbpol.2020.117568
Huang, X., Ge, X., Zhou, L., and Wang, Y. (2022). Eugenol embedded zein and poly(lactic acid) film as active food packaging: Formation, characterization, and antimicrobial effects. Food Chem. 384:132482. doi: 10.1016/j.foodchem.2022.132482
Khoshkalampour, A., Ghorbani, M., and Ghasempour, Z. (2023). Cross-linked gelatin film enriched with green carbon quantum dots for bioactive food packaging. Food Chem. 404:134742. doi: 10.1016/j.foodchem.2022.134742
Lado, J., Vicente, E., Moltini, A. I., Alcaire, F., and Ares, G. (2021). Integrating consumer perception in the selection of purple-skin sweet potato cultivars. J. Sci. Food Agric. 101, 3620–3629. doi: 10.1002/jsfa.10991
Li, L., Zhang, L., Han, Y., Wen, L., Shao, S., and Zong, X. (2023). Effect of the addition of mung bean, corn, and sweet potato starch on the properties of konjac gel. J. Food Sci. Technol. 60, 3094–3101. doi: 10.1007/s13197-023-05821-0
Li, X., Liu, Y., Luo, B., Xiang, W., and Chen, Z. (2024a). Effect of apple polyphenols on physicochemical properties of pea starch/pulp cellulose nanofiber composite biodegradable films. Int. J. Biol. Macromol. 257:128480. doi: 10.1016/j.ijbiomac.2023.128480
Li, X., Zhang, X., Lv, J., Zhang, X., Li, Y., Han, X., et al. (2024b). Development of starch-based films reinforced with curcumin-loaded nanocomplexes: characterization and application in the preservation of blueberries. Int. J. Biol. Macromol. 264:130464. doi: 10.1016/j.ijbiomac.2024.130464
Li, Y., Duan, Q., Yue, S., Alee, M., and Liu, H. (2024). Enhancing mechanical and water barrier properties of starch film using chia mucilage. Int. J. Biol. Macromol. 274:133288. doi: 10.1016/j.ijbiomac.2024.133288
Liu, Y. W., Ma, Y., Liu, Y., Zhang, J., Hossen, M. A. E, et al. (2022). Fabrication and characterization of pH-responsive intelligent films based on carboxymethyl cellulose and gelatin/curcumin/chitosan hybrid microcapsules for pork quality monitoring. Food Hydrocoll. 124:107224. doi: 10.1016/j.foodhyd.2021.107224
Liu, Z., Zhao, M., Zhang, Z., Li, C., Xia, G., Shi, H., et al. (2023). Chitosan-based edible film incorporated with wampee (Clausena lansium) seed essential oil: Preparation, characterization and biological activities. Int. J. Biol. Macromol. 253:127683. doi: 10.1016/j.ijbiomac.2023.127683
Lyly, M., Salmenkallio-Marttila, M., Suortti, T., Autio, K., Poutanen, K., and Lähteenmäki, L. (2004). The sensory characteristics and rheological properties of soups containing oat and barley β-glucan before and after freezing. LWT-Food Sci. Technol. 37, 749–761. doi: 10.1016/j.lwt.2004.02.009
Madadi, R., Maljaee, H., Serafim, L. S., and Ventura, S. P. M. (2021). Microalgae as contributors to produce biopolymers. Mar. Drugs 19:466. doi: 10.3390/md19080466
Mousazadeh, S., Ehsani, A., Moghaddas kia, E., and Ghasempour, Z. (2021). Zinc oxide nanoparticles and periodate oxidation in developing pH-sensitive packaging film based on modified gelatin. Food Pack. Shelf Life 28:100654. doi: 10.1016/j.fpsl.2021.100654
Narvaez-Gomez, G., Figueroa-Florez, J., Salcedo-Mendoza, J., Perez-Cervera, C., and Andrade-Pizarro, R. (2021). Development and characterization of dual-modified yam (Dioscorea rotundata) starch-based films. Heliyon 7:e06644. doi: 10.1016/j.heliyon.2021.e06644
Riaz, A., Lei, S., Akhtar, H. M. S., Wan, P., Chen, D., Jabbar, S., et al. (2018). Preparation and characterization of chitosan-based antimicrobial active food packaging film incorporated with apple peel polyphenols. Int. J. Biol. Macromol. 114, 547–555. doi: 10.1016/j.ijbiomac.2018.03.126
Roy, S., and Rhim, J. W. (2020). Carboxymethyl cellulose-based antioxidant and antimicrobial active packaging film incorporated with curcumin and zinc oxide. Int. J. Biol. Macromol. 148, 666–676. doi: 10.1016/j.ijbiomac.2020.01.204
Sarka, E., Sinica, A., Smrckova, P., and Slukova, M. (2023). Non-traditional starches, their properties, and applications. Foods 12:3794. doi: 10.3390/foods12203794
Singh, G. P., Bangar, S. P., Yang, T., Trif, M., Kumar, V., and Kumar, D. (2022). Effect on the properties of edible starch-based films by the incorporation of additives: a review. Polymers 14:1987. doi: 10.3390/polym14101987
Sopher, C. J., Adamson, B. J., Andrasik, M. P., Flood, D. M., Wakefield, S. F., Stoff, D. M., et al. (2015). Enhancing diversity in the public health research workforce: the research and mentorship program for future HIV vaccine scientists. Am. J. Public Health 105, 823–830. doi: 10.2105/AJPH.2014.302076
Sun, C., Wei, Z., Xue, C., and Yang, L. (2023). Development, application and future trends of starch-based delivery systems for nutraceuticals: a review. Carbohydr. Polym. 308:120675. doi: 10.1016/j.carbpol.2023.120675
Tafa, K. D., Satheesh, N., and Abera, W. (2023). Mechanical properties of tef starch based edible films: development and process optimization. Heliyon 9:e13160. doi: 10.1016/j.heliyon.2023.e13160
Venkatesan, R., Alagumalai, K., and Kim, S. C. (2023). Preparation and performance of biodegradable poly(butylene adipate-co-terephthalate) composites reinforced with novel AgSnO(2) microparticles for application in food packaging. Polymers 15:554. doi: 10.3390/polym15030554
Wang, L., and Weller, C. L. (2006). Recent advances in extraction of nutraceuticals from plants. Trends Food Sci. Technol. 17, 300–312. doi: 10.1016/j.tifs.2005.12.004
Wiszumirska, K., Czarnecka-Komorowska, D., Kozak, W., Bieganska, M., Wojciechowska, P., Jarzebski, M., et al. (2023). Characterization of biodegradable food contact materials under gamma-radiation treatment. Materials (Basel) 16:859. doi: 10.3390/ma16020859
Xie, J., Wang, R., Li, Y., Ni, Z., Situ, W., Ye, S., et al. (2022). A novel Ag(2)O-TiO(2)-Bi(2)WO(6)/polyvinyl alcohol composite film with ethylene photocatalytic degradation performance towards banana preservation. Food Chem. 375:131708. doi: 10.1016/j.foodchem.2021.131708
Xu, Q. D., Jing, Z., He, Q., and Zeng, W. C. (2023). A novel film based on gluten, pectin, and polyphenols and its potential application in high-fat food. J. Sci. Food Agric. 103, 6119–6127. doi: 10.1002/jsfa.12682
Keywords: bioactive packaging material, edible film, fresh-keeping packaging, antioxidant activity, antimicrobial effect, eco-friendly materials
Citation: Zhang Z, Wang Y, Fang X, Chen X, Yin Z and Zhang C (2024) Preparation of edible film from sweet potato peel polyphenols: application in fresh fruit preservation. Front. Sustain. Food Syst. 8:1470732. doi: 10.3389/fsufs.2024.1470732
Received: 26 July 2024; Accepted: 05 November 2024;
Published: 22 November 2024.
Edited by:
Rakesh Bhardwaj, Indian Council of Agricultural Research (ICAR), IndiaReviewed by:
Jun Liu, Yangzhou University, ChinaSapna Langyan, Indian Council of Agricultural Research (ICAR), India
Copyright © 2024 Zhang, Wang, Fang, Chen, Yin and Zhang. This is an open-access article distributed under the terms of the Creative Commons Attribution License (CC BY). The use, distribution or reproduction in other forums is permitted, provided the original author(s) and the copyright owner(s) are credited and that the original publication in this journal is cited, in accordance with accepted academic practice. No use, distribution or reproduction is permitted which does not comply with these terms.
*Correspondence: Chunlei Zhang, Y2x6aGFuZ0Bqc251LmVkdS5jbg==