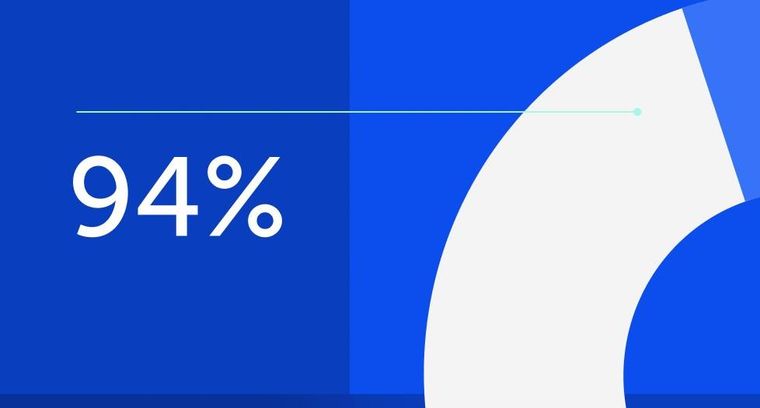
94% of researchers rate our articles as excellent or good
Learn more about the work of our research integrity team to safeguard the quality of each article we publish.
Find out more
REVIEW article
Front. Sustain. Food Syst., 24 September 2024
Sec. Aquatic Foods
Volume 8 - 2024 | https://doi.org/10.3389/fsufs.2024.1470683
This article is part of the Research TopicDetection, Risk Analysis and Monitoring of Chemical Contaminants from Agro-Aqua Food Production and Processing: Implications on the One Health TriadView all 9 articles
Mercury (Hg) is a persistent pollutant highly bioaccumulated in the aquatic environment through the food chain reaching high concentration levels in the tissues of predator fishes. Among the relevant sources of anthropogenic mercury emissions in Latin America and the Caribbean (LAC), mining is one of the most important along with soil erosion due to deforestation and agricultural activities where pesticides are intensively used. Several reports have demonstrated an association between a fish-based diet with elevated Hg levels in the blood and neurotoxic effects in humans. In this systematic review with quantitative analysis data from 92 articles were compiled, providing evidence of Hg concentration in fishes that are commonly consumed in LAC. An assessment was conducted using three indices for health risk: the Minamata Initial Assessment (MIA), the Target Hazard Quotient (THQ), and the Meals per Week (MPW) index. Of the 410 fish species studied, 5.4% had concentrations above 0.95 μg g−1 of wet weight (ww), which is the recommended limit for Total Hg (THg) ingestion through fish consumption according to the MIA index, regardless of the water habitat (i.e., marine or freshwater). Additionally, the Target Hazard Quotient (THQ) calculation indicated high-risk values (THQ ≥ 1) in 15 out of the 19 countries studied, and very high-risk values (THQ ≥ 10) were obtained from Hg concentrations measured in 5 fish species inhabiting watersheds in Trinidad and Tobago, Suriname and Peru. Finally, recommendations on fish consumption were made based on the MPW index. This study reveals the need for updated biomonitoring studies of Hg concentrations in fish to perform more accurate human health risk analyses.
Mercury (Hg), in its various chemical forms, is widely distributed in the environment. It is found in different ecosystems due to its many natural sources such as volcanic emissions, soil and rock erosion, and wildfire, but also due to anthropogenic activities such as fossil fuel burning and mining extraction (Pirrone et al., 2010). According to the UN Environment (2019). Latin America and the Caribbean (LAC) in 2015 released 20.5% of global anthropogenic Hg air emissions, of which small-scale gold mining contributed to 354.3 t of Hg emissions, followed by the industry sectors (66.4 t of Hg) and intentional use (including product waste; 20.21 t of Hg). In addition, South America alone accounted for 53% of the estimated 1,220 t of Hg released globally from small-scale gold mining to aquatic and terrestrial environments in 2015 (Canham et al., 2021).
Aquatic ecosystems are one of the most relevant environments for the cycling of Hg deposited from the atmosphere since its methylation is carried out mainly by benthic microorganism transformations that produce methylmercury (MeHg), an organic form of Hg with higher toxicity (Al-Sulaiti et al., 2022). More than 85–90% of the Hg found in fish tissues is MeHg and its concentration depends on its trophic position, age, and size (Grieb et al., 1990; Southworth et al., 1995). Higher MeHg is found in those predator species with the highest trophic position in the food chain (Lavoie et al., 2013).
In an ecotoxicological report from Brazil, it was found that top predator fish, which are locally consumed, present high levels of Total mercury, THg, (1.2–2.1 μg g−1 wet weight, ww; Hacon et al., 2020), well above the risk threshold level of 0.95 μg g−1 ww according to MIA recommendations (MIAs in the Caribbean, 2017). This and other studies (Rice et al., 2014; Gribble et al., 2015) evidence that in LAC humans are exposed to risky levels of Hg and its associated harmful effects through a diet based on fish consumption. Furthermore, reported evidence obtained in LAC indicates associations between consumption of marine and freshwater fishes displaying high levels of Hg (THg and MeHg) concentrations and detectable amounts of this metal in human hair (Bravo et al., 2019; Valdelamar-Villegas and Olivero-Verbel, 2020).
It is widely known that human exposure to high levels of MeHg/THg is associated with neurotoxicity (Castoldi et al., 2001). One of the most well-known cases of high-scale poisoning with fish and seafood contaminated with MeHg was the Minamata disaster in the 1950s at Minamata Bay in Japan. This compound was generated as a by-product in reaction chambers for manufacturing acetaldehyde and discharged directly to the bay, resulting in fish and seafood bioaccumulation that later was consumed by the local inhabitants. The intake of contaminated marine products produced a syndrome called Minamata disease, with numerous severe neurological and physical sequelae on the muscle, sight, and hearing systems (Eto, 2000; Hachiya, 2006). Besides these high exposure levels to MeHg, chronic exposure to lower levels can cause hepatic diseases and reproductive toxicity (Tan et al., 2009; Rice et al., 2014). In 2013, the Minamata Convention was created, and to date, 137 countries have adopted the agreement, of which 20 are countries from LAC (Coulter, 2016). Therefore, it is of major importance to implement a risk assessment method that can be applied in decision-making and the generation of environmental regulations in vulnerable countries such as those of LAC. This literature review and meta-analysis seeks to assess the risk of Hg exposure and consequently health hazards due to fish consumption in LAC by gathering available data from THg levels in fish and fish consumption rates per country.
This systematic review with a quantitative analysis focus was conducted following the PRISMA guideline (Figure 1; Page and Left, 1996). A literature search on peer-reviewed journal articles and published reports documenting THg and/or MeHg concentrations in fish in LAC countries was conducted on Web of Science1 and Google Scholar2 according to Sheehan et al. (2014). Studies published from 2005 until 2024 were selected. Exceptionally some reports published before 2005 were included since for some countries there was no information after this year (Table 1).
Figure 1. Schema of the literature search on peer-reviewed journal articles and published reports documenting THg and/or MeHg concentrations in fish in Latin American and Caribbean countries.
Studies were first selected based on the title and abstract screening, followed by full-text review and exclusion criteria. Studies that did not state if the concentrations were dry weight (dw) or wet weight (ww) were excluded. In addition, a secondary search was made on FishBase (Froese and Pauly, 2023) to determine if the fish species were human-consumed and exclude reports on non-consumed species. When the same species or site, was reported on multiple articles, the most recently published was chosen.
Data were classified by fish species, country, sample size, habitat (marine or freshwater environment), and fish diet (herbivore, omnivore, and carnivore; Supplementary Table S1). In addition, the classification considered the type of mercury species measured (THg and/or MeHg) and the analytical methods used. All data reported in dw were converted to ww using the Equation 1, as described by Cresson et al. (2017).
Where, Cwet and Cdry correspond to the substance concentration of interest measured in wet and dry tissue respectively, and %H is the percentage of humidity in wet tissues (~80% for most of the species).
As previously mentioned, data were filtered by (1) fish species that are consumed by humans, and (2) THg and/or MeHg concentration quantified in the muscle that corresponds to consumed fish tissue. Additional data was obtained for human health risk assessment such as average body weight by sex and average fish consumption per country and day. Average body weight was obtained from the Eglitis dataset (Eglitis, 2021) for adult women (BWW) and men (BWM) from each country analyzed. Average fish consumption per country and day (FCR) was estimated from the “Fish and seafood consumption per capita” dataset via www.ourworldindata.org and the National Oceanic and Atmospheric Administration (NOAA, 2014) which are expressed in kg per year.
The Target hazard quotient (THQ) is a non-carcinogenic index that assesses the health risk from food consumption by residents and is calculated based on Salazar-Camacho et al. (2022):
In Equation 2, EF corresponds to the exposure frequency (365 days/year); ED is the total duration of fish consumption, considering an average human life of 70 years (Roser et al., 2013); FCR is the fish consumption rate (g per day), and CHg is the MeHg concentration expressed as μg g−1 (ww). Due to some reports included in the survey informing of THg instead of MeHg concentrations, a MeHg conversion factor has been applied according to the Guidance for Assessing Chemical Contaminant Data for Use in Fish Advisories (USEPA, 2011) which assumes that 95% of the Hg concentration measured in fish tissue is MeHg. BW is human body weight (kg); AT is the average time of food consumption (EF × 70 years); and RfD is the reference dose which is the daily dosage that enables an individual to sustain a long-term level of exposure without experiencing any harmful effects (USEPA, 2011). A RfD of 0.1 μg per kg per day was used, according to EPA’s guidelines (USEPA, 2001).
Recommendations for fish consumption per week (MPW) were calculated with Equation 3, based on (Vieira et al., 2021):
We have considered a fish meal size of 226.8 g of fish per week based on a 2000 cal diet according to the Dietary Guidelines for Americans (2015–2020). Body weight (BW) was obtained from the Eglitis data set from 2021 and is based on the BW average for the adult population in each country analyzed according to sex (BWW: women’s body weight and BWM: men’s body weight).
THg concentration is presented as median ± range (μg g−1 ww, Supplementary Figure S1). The Mood’s median test was used to estimate statistical differences (p = 0.05) in THg concentrations among dietary preferences, habitat type (marine or freshwater), and countries (Supplementary Table S2). Further analyses were applied (Mood’s pairwise median tests) for comparison between aquatic environments (S3), diet preferences (S4), and countries (S5). The analysis was made using the R-packages (R version 4.1.2). Species showing a THg content >0.95 μg g−1 (ww) were analyzed to determine their distribution according to the habitat (marine or freshwater) and diet preferences (carnivores, detritivores, herbivores, or omnivores). With these classifications, a Sankey plot was built using the Sankey Network function from the networkD3 R-package (Allaire et al., 2017).
To compare the risk (THQ) differences between women and men, a Mann–Whitney U-test was performed (Supplementary Table S6). To observe the geographic distribution of the Hg exposure risk by fish consumption among inhabitants of LAC countries, the obtained risk values for women and men were mapped using the geographic coordinates reported in the respective paper of origin. For this, the rgdal (Bivand et al., 2023) and ggplot2 (Wickham, 2016) R-packages (R Core Team, 2021) were used. All plots resulting from the information gathered were created with ggplot2 and R-packages (R version 4.1.2).
Studies concerning THg bioaccumulation in human-consumed fishes were found in around 55% of LAC countries including, from north to south: Mexico, Cuba, Jamaica, Belize, Anguilla, Nicaragua, Costa Rica, Colombia, Trinidad and Tobago, Venezuela, Suriname, Ecuador, Brazil, Peru, Bolivia, Chile, Paraguay, Uruguay and Argentina with a total data set of 1,046 samples. Countries reporting a higher number of fish species in which Hg bioaccumulation was measured (Table 2) were Brazil (n = 194) and Mexico (n = 58).
Table 2. Median and range of the THg concentration (μg g−1 ww) in fish muscle by country and number of species (n), and the proportion of fish species with median concentrations ≥0.95 μg g−1 ww.
A total of 410 fish species were analyzed from studies reporting THg and/or MeHg levels in muscle. THg concentrations in freshwater fish range from 0.003 to 3.570 μg g−1 ww; meanwhile marine fish range from 0.002 to 2.218 μg g−1 ww (Supplementary Table S1). Most of the species were found in freshwater ecosystems; specifically, rivers (45%), lakes (7.4%), reservoirs (1.3%), and wetlands (1.5%). A lower number of species were from marine ecosystems and were distributed as follows: species from coastal environments (36.9%), coastal/oceanic environments (3.7%), oceanic species (2%), and estuaries (2.2%). The distribution of the fish species in the water column is mainly benthopelagic (45.6%) and benthic (36.4%), and a smaller portion is pelagic (18%).
The distribution of the species in the water column does not have a significant impact on the THg (Mood’s median test, p-value = 0.058), contrary to the habitat (freshwater or marine, Mood’s median test, p-value = 3.63e−8) and aquatic environment where we found significant differences between the Coastal, Coastal/Oceanic and River fish (Mood’s median test, p-value <0.001; Supplementary Tables S2, S3).
There are significant differences in THg content in fish muscle between species with different diet preferences. Carnivorous fish had significantly higher THg content than detritivores, herbivores, or omnivorous fishes (Mood’s median test p-value <0.001, Supplementary Tables S2, S4). No differences were detected within the last three groups, regardless of the habitat. Fish species showing THg concentrations above MIA’s standard (≥0.95 μg g−1 ww) mostly correspond to carnivore species.
The Sankey plot (Figure 2) shows a subsample of those fish species with a THg concentration ≥ 0.95 μg g−1 ww (5.4% of the data). This includes 44 fish species from 9 countries. According to MIA’s the remaining samples are considered to have concentrations that are safe to be consumed unrestrictedly (<0.22 μg g−1 ww, 70.9%) or to be consumed in a moderated diet (0.22–0.95 μg g−1 ww, 23.7%).
Figure 2. Sankey diagram representing the distribution of THg concentrations in fishes from LAC. THg concentration ranges are represented by the width of grey ribbons. To the right, bars indicate the distribution between water habitats (marine or freshwater, respectively). Food preferences (carnivorous, omnivorous, detritivore, or herbivore) are indicated by coloured bars in the middle. *: highlighted those species most consumed. Only species displaying THg concentrations above MIA’s regulations (≥ 0.95 μg g−1 ww) were considered in this diagram.
The plot showed that carnivorous species present the largest range and highest concentrations of THg (0.954 to 3.570 μg g−1 ww), independently of the water habitat. However, three omnivorous (1.560 to 2.140 μg g−1 ww), two detritivores (1.325 and 2.131 μg g−1 ww), and three herbivores (1.000 to 1.630 μg g−1 ww) also displayed THg concentrations that might deserve attention since most of the recommendations declare not to intake THg concentrations above 0.95 μg g−1 ww.
On the other hand, specimens showing high THg concentrations, and considered hazardous for human consumption (1.2 to 1.7 μg g−1 ww) by the regulation of FAO and WHO (2018) were five marine species and 31 freshwater species, were predators C. porosus (2.218 μg g−1 ww) a marine fish from Trinidad and Tobago, and the freshwater B. rousseauxii (3.570 μg g−1 ww) from Brazil the highest THg concentration.
Brazil, Bolivia, Ecuador, Colombia, Peru, Suriname, Trinidad and Tobago, Uruguay, and Venezuela showed the fish species with the highest Hg levels. Belize, Paraguay, and Venezuela had the highest median values of THg concentration on fish muscle (> 0.3 μg g−1 ww; Table 2). Statistical tests showed significant differences in the THg median between countries (Mood’s median test, p-value <0.001, Supplementary Table S2).
In Figure 3, 77.2–79.3% of the total species studied showed to have a THQ < 1, from which 68.7–69.2% showed THg concentrations lower than 0.22 μg g−1 ww, and 18.9–20.5% present concentrations above this value without exceeding the threshold value of 0.95 μg g−1 ww. Of the remaining species with THQ values ≥1, only 5.6% have THg levels ≥0.95 μg g−1 ww. From this subset, only 0.8–0.9% have THQ values ≥10. The group of species with THg concentrations above the MIA’s recommended levels (≥ 0.95 μg g−1 ww) were found in Brazil, Bolivia, Ecuador, Colombia, Peru, Suriname, Trinidad and Tobago, Uruguay, and Venezuela and should not be recommended for frequent human consumption. The distribution of the THQ index in the 19 LAC countries is in Supplementary Figure S2.
Figure 3. Venn diagram summarizing the distribution of THg concentration (range THg < 0.22 μg g−1 ww; 0.22 < THg < 0.95 μg g−1 ww; and THg < 0.95 μg g−1 ww) and its interaction with Target Hazard Quotient (THQ) ranges (THQ < 1; 1 > THQ > 10; and THQ > 10) in the total species assessed in this study. The data is presented in percentages and is sex-specific, indicated as ♂ (men) and ♀ (women).
The results of risk estimation for fish consumption are displayed in Figure 4, showing that THg concentrations ≥0.95 μg g−1 ww and THQ ≥ 1 were from Brazil, Uruguay, Perú, Suriname, Trinidad and Tobago, and Venezuela. However, some species with THg concentrations below 0.95 μg g−1 ww also show a THQ ≥ 1 in almost every analyzed country (Supplementary Table S1). Finally, considering all the species studied, the distribution by country of those showing a THQ ≥ 1 remained as follows: Anguilla (4 species), Argentina (2 species), Belize (1 species), Brazil (77 species), Chile (6 species), Colombia (16 species), Ecuador (9 species), Jamaica (2 species), Mexico (7 species), Paraguay (1), Peru (16 species), Suriname (12 species), Trinidad and Tobago (3 species), Uruguay (2 species), and Venezuela (10 species). The list of fish species is in Supplementary Table S1.
Figure 4. Geolocation of the health risk estimation related to total mercury (THg) contaminated fish consumption from LAC. The adult human consumption level (HCL) of fish is indicated by color, where fish with a high consumption rate are those of high commercial interest (magenta), while fish with a low consumption rate are those of the local diet (green). Circle sizes indicate the average Target Hazard Quotient (THQ) value, associated with a fish species. The bar graphs illustrate the frequency of risky THQ values (≥1) and safe THQ values (<1) for the men population (THQM, up) and women population (THQW, down).
There is a slight trend difference in the risk estimation regarding women (excluding lactating or pregnant women) and men, with women the group displaying higher THQ values, however, no statistical differences between both groups were found (Figure 4, Mann–Whitney U-test, p-value = 0.131, mean difference 0.020, Supplementary Table S6).
The countries estimated to have the greatest health risk were Brazil, Colombia, Peru, Suriname, and Venezuela due to more than 1/3 of the species studied from these countries presenting a THQ ≥ 1 (n = 77 from 203, 16 from 46, 16 from 43, 12 from 18, and 10 from 17, respectively). Fewer species contributed to very high THQ values (THQ > 10) namely A. microlepis, H. malabaricus, S. rhombeus, C. ocellaris, P. eigenmanni, and L friderici from Suriname; C. porosus from Trinidad and Tobago; P. flavipinnis and P. pirinampu from Peru; and B. rousseauxii from Brazil.
Additionally, considering that a THQ ≥ 1 is considered hazardous for human health, the frequency plot shows that most studied fish species have no risk for adult human consumption (68.7% for women and 69.2% for men).
The latest Dietary Guidelines for Americans from the U.S. Department of Agriculture and U.S. Department of Health and Human Services advise eating around 226.8 g of fish per week, based on a 2,000-calorie diet USDA and HHS (2020). This recommendation would be acceptable for 70.9% of the data collected in the present study based on the MPW estimation. MPW < 1 (29.06%) estimation corresponded in most of the cases to concentrations >0.22 μg g−1 ww THg, which is recommended to eat once a month or to avoid in the species with levels higher than 0.95 μg g−1 ww (MIAs in the Caribbean, 2017). Only 71.7% of the MPW lower than 1 had a THQ above 1. In Supplementary Table S1 there is a recommendation chart for those species to avoid consuming, keep consuming with moderation (good choice), and encourage their consumption (best choice) based on their THg levels and THQ values obtained during this study. This classification is based on the MPW index, where less than 1 MWP is recommended to avoid, 1 or 2 MPW is a good choice, and more than 2 MPW is the best choice. Specifications are described by country.
Fisheries are one of the most important economic activities in LAC countries, and in some of these nations, fish is a significant component of the population’s diet. Consequently, it is essential to evaluate the risk of mercury intake through this food source. In this study, THQ values were obtained to perform the risk assessment associated with fish consumption in LAC countries. These values varied widely across species, ecosystems, and populations from different countries, highlighting the need for region-specific assessments considering consumption rates, body weight, and cultural preferences. The human risk analysis of Hg intake by fish consumption indicated that ~70% of the fish analyzed were considered safe and non-risky for human consumption. However, it is recommended to avoid consuming fishes from some genera, such as Acestrorhynchus, Brachyplatystoma, Cichla, Hoplias, Myloplus, Pinirampus, Pseudoplatystoma, Pygocentrus, and Salminus due to the occurrence of high THg concentrations in some countries.
Due to Hg biomagnification across trophic levels, the highest THg levels were found in carnivore species which is consistent with previous studies (Kidd and Batchelar, 2011; Lavoie et al., 2013; Chiang et al., 2021). The same species had a significant contribution to the estimated risk showing a correlation between elevated THg concentrations and THQ values above 1. Nevertheless, in some cases, low THg levels caused high THQ values. This was observed in Peru where despite most species having safe THg concentrations (<0.95 μg g−1 ww), the THQ values were above 1. This is due to the high rate of fish consumption by the Peruvian population (68.6 g day−1) compared for example to Argentina whose fish species have similar THg levels to Peru’s, but its consumption rate is among the lowest in LACs (15.6 g day−1) leading to safe THQ values. This exemplifies that regardless of having safe THg concentrations, the fish consumption rates (> 65 g day−1) and body weight (>76 kg per adult woman and > 80 kg per adult man) are important factors in determining the safety of human fish consumption. On the contrary, in countries like Anguilla, Cuba, Nicaragua, and Costa Rica we observed a direct correlation between THg concentrations and THQ levels, indicating that the amount of fish consumption and body weight distribution in these countries does not alter this correlation. Most of their fish species have acceptable THg levels, and THQ values lower than 1. Due to the variety of species, ecosystems, and demographic factors, we have found in this a wide range of THQ values (THQW = 0.006–17.008; THQM = 0.006–15.934), slightly different from those reported in other places in the world like Augusta Bay in southern Italy (THQ = 0.31–15.8; Bonsignore et al., 2013); Azores archipelago in Portugal (THQ = 0.1–8.6; Vieira et al., 2021) or Atrato River basin at the west of Colombia (THQ = 0.09–21.6; Salazar-Camacho et al., 2022), reflecting the importance of this kind of assessment to be specific for different parts of the world. It is interesting to highlight the low value of the lower limit of the reported ranges of the THQ for men and women in Chile, which is several indices of magnitude smaller than the lower limits of the ranges reported in the other regions described above. This could suggest that there is greater variability in the risk associated with fish consumption for the Chilean population.
There are several approaches to estimating the risk associated with food consumption contaminated with Hg. If only the MIAs in the Caribbean (2017) recommendations were considered, 5.35% of the total fish species would be recommended to avoid their consumption. On the other side, regarding the U.S. EPA guidelines, in which a THQ value above 1 is considered risky for human consumption, the percentage of fish species to be avoided increased to 22.56% for adult women and 20.45% for adult men, depending on average body weight, rates of fish consumption, and also considering the RfD and other variables like a cooking adjustment factor that would alter the MeHg concentration in the fish muscle depending on if it is raw or cook (USEPA, 2011). Finally, Vieira et al. (2021) employed the MPW variable and categorized the recommendations as choices to avoid, good choices, and best choices. These authors were based on the Joint Expert Committee on Food Additives (JECFA) recommendations for the maximum amount of fish meals per week. The JECFA recommendations also consider body weight, RfD, and THg concentrations in fish, in addition to the quantity and frequency of the consumption of fish species. According to these antecedents and our MPW calculation, 29.06% of the fish species from our study should be avoided, similar to the percentages obtained using the THQ.
It is important to highlight that this study only considers a general estimation of fish consumption by country and does not consider regional differences within the country. For geographical reasons, fish consumption in coastal living populations is higher than in non-coastal communities. Therefore, in an ideal scenario, the risk analysis should be performed by geographical areas sharing features like frequency of fish consumption and other demographic variables. However, it is difficult to carry out an approach with this level of precision to design public policies, regulations, and health safety guidelines.
The nutritional benefits of fish consumption are undeniable. A diet that frequently includes fish provides proteins, energy, and nutrients like vitamin D, selenium (Se), omega-3/n-3 long-chain, and polyunsaturated fatty acids (n-3 LC-PUFA). However, Hg contamination related to anthropogenic activities has raised concerns about healthy options for fish consumption. Nevertheless, Se is known to provide defense against the toxicity of different metals such as Hg. Se and Hg exhibit a strong affinity for binding to each other, thereby diminishing each other’s toxic effects (Gochfeld and Burger, 2021). Studies must consider then measuring Se content to calculate the real toxicity of fish consumption. In addition, (FAO and WHO, 2018) made a risk/benefit analysis between Hg and omega-3 intake which made recommendations on the frequency of fish consumption based on Hg and omega-3 concentrations.
Reducing the risks of Hg poisoning is not as simple as avoiding fish entirely, but optimizing fish choices. Fish lower on the food chain should be more likely to be consumed than those on the high trophic level. There is a need for updated information to help people make rational decisions about their choices for fish consumption. In terms of public health, some actions should be considered to mitigate the risks of mercury exposure: (a) Surveillance and monitoring of mercury levels in fish and the population through consumption patterns; (b) Establishing safety limits and applying regulations; (c) Risk communication: to provide information about the risks associated to fish consumption with high Hg content and provide the recommended intake per week; (d) Nutritional interventions, education and training for the safe consumption of fish; (e) Exposure assessment, conduct epidemiological studies and evaluate possible health effects.
Most of the countries considered in this study do not have updated data on levels of Hg contamination in their fish species. This is a matter of concern since mining is one of the most important economic activities in Latin America (Alimonda, 2015) and one of the most relevant Hg contamination sources (Chalker and Esdaile, 2018). Although most of the global fishery and aquaculture activities are centered on marine species (FAO, 2020), only one-third of the data found during this research belonged to marine ecosystems. For instance, Trachurus murphyi, Engraulis ringens, and Strangomera bentincki make up 83% of Chilean fishery exports (SUBPESCA, 2020; OECD, 2021), and only two of these species were found in the studies analyzed.
To compare Hg pollution with other countries, East and Southeast Asia, a major responsible for Hg emissions from mining and industry (Soe et al., 2022) has a similar average of Hg bioaccumulation in fish as some LAC countries (Park et al., 2011; Jeevanaraj et al., 2019; Shalini et al., 2021; Soe et al., 2022).
Finally, the study identified some limitations to obtaining more accurate and representative estimations. Some issues were the outdated demographic and environmental information and the data’s homogenization. Almost half of the countries from LACs have no records of Hg pollution in fish, and some of them, like Nicaragua and Bolivia, were from fish samples from 30 or 20 years ago. Furthermore, this study only addressed the adult population. Consequently, in more vulnerable demographic groups, such as children and pregnant/lactating people, the risk could be underestimated. This topic is crucial, because along with damage from acute or chronic exposure to the brain, lungs, kidneys, and immune system (Tan et al., 2009; Rice et al., 2014; Guzzi et al., 2021), Hg exposure also relates to neuropsychological decline (Corral et al., 2013). Jacobson et al. (2015) found a link between Hg in pregnant women’s hair and lower children’s IQ. Specifically, for each part per million (ppm) increase in a mother’s hair mercury, a child’s IQ drops by 0.7 points. This evidence has proved the urge to enhance the development of local Hg monitoring systems in fish that are most consumed in LAC countries.
In many countries, there is a dearth of recent information on the mercury content in fish, as highlighted in this review. The rise in mining activities, deforestation, and other environmental issues in LAC necessitates an improvement in environmental regulations to prevent health problems among the population. Differences in the distribution of mercury emissions, population behavior, and demographic characteristics make it unfeasible to regulate under the standards of other countries. Therefore, we propose utilizing strategies that consider various parameters and incorporate local data to ensure a more precise risk assessment analysis.
EV: Conceptualization, Data curation, Formal analysis, Funding acquisition, Investigation, Methodology, Project administration, Resources, Software, Supervision, Validation, Visualization, Writing – original draft, Writing – review & editing. FP: Conceptualization, Formal analysis, Methodology, Visualization, Writing – review & editing. LZ: Conceptualization, Data curation, Formal analysis, Methodology, Software, Writing – review & editing. KF: Conceptualization, Data curation, Investigation, Methodology, Writing – review & editing. PB: Conceptualization, Data curation, Formal analysis, Funding acquisition, Investigation, Methodology, Project administration, Resources, Supervision, Validation, Visualization, Writing – original draft, Writing – review & editing.
The author(s) declare that financial support was received for the research, authorship, and/or publication of this article. This work was funded by author P.B. grants FONDECYT Initiation Grant 11180914, FONDECYT 1230485, ANID Millennium Science Initiative NCN2021_056: Millennium Nucleus of Austral Invasive Salmonids (INVASAL), and Project CHIC ANID/BASAL FB210018. E.V. was funded by ANID Scholarship 21220155 and by the General Direction Research, University of Playa Ancha – Chile (P.B.).
The authors would like to thank Veronica Molina for her collaboration funding support and editing of the manuscript. We also thank Ian Acuña Rodríguez for his support in the representation of this work.
The authors declare that the research was conducted in the absence of any commercial or financial relationships that could be construed as a potential conflict of interest.
All claims expressed in this article are solely those of the authors and do not necessarily represent those of their affiliated organizations, or those of the publisher, the editors and the reviewers. Any product that may be evaluated in this article, or claim that may be made by its manufacturer, is not guaranteed or endorsed by the publisher.
The Supplementary material for this article can be found online at: https://www.frontiersin.org/articles/10.3389/fsufs.2024.1470683/full#supplementary-material
Alimonda, H. (2015). “Mining in Latin America: coloniality and degradation” in The international handbook of political ecology. Ed. E. Bryan. (Edward Elgar Publishing Limited), 149–161.
Allaire, J.J., Gandrud, C., Russell, K., and Yetman, C. (2017). networkD3: D3 JavaScript network graphs from R. R package, version 0.4.
Al-Sulaiti, M. M., Soubra, L., and Al-Ghouti, M. A. (2022). The causes and effects of mercury and methylmercury contamination in the marine environment: a review. Curr. Pollut. Rep. 8, 249–272. doi: 10.1007/s40726-022-00226-7
Bivand, R., Keitt, T., Rowlingson, B., Edzer, P., Sumner, M., Hijmans, R., et al. (2023). Package ‘rgdal.’
Bonsignore, M., Salvagio Manta, D., Oliveri, E., Sprovieri, M., Basilone, G., Bonanno, A., et al. (2013). Mercury in fishes from Augusta bay (southern Italy): risk assessment and health implication. Food Chem. Toxicol. 56, 184–194. doi: 10.1016/j.fct.2013.02.025
Bravo, M. A., Parra, S., Quiroz, W., and Neaman, A. (2019). Human exposure assessment to mercury through hair analysis in coastal villages of the Valparaiso region (Chile). J. Chil. Chem. Soc. 64, 4480–4483. doi: 10.4067/S0717-97072019000204480
Canham, R., González-Prieto, A. M., and Elliott, J. E. (2021). Mercury exposure and toxicological consequences in fish and fish-eating wildlife from anthropogenic activity in Latin America. Integr. Environ. Assess. Manag. 17, 13–26. doi: 10.1002/ieam.4313
Castoldi, A., Coccini, T., Ceccatelli, S., and Manzo, L. (2001). Neurotoxicity and molecular effects of methylmercury. Brain Res. Bull. 55, 197–203. doi: 10.1016/S0361-9230(01)00458-0
Chalker, J.M., and Esdaile, L.J. (2018). Chemistry A European J - 2018 - Esdaile - The mercury problem in artisanal and small-scale gold mining.pdf. 12.
Chiang, G., Kidd, K. A., Díaz-Jaramillo, M., Espejo, W., Bahamonde, P., O’Driscoll, N. J., et al. (2021). Methylmercury biomagnification in coastal aquatic food webs from western Patagonia and western Antarctic Peninsula. Chemosphere 262:128360. doi: 10.1016/J.CHEMOSPHERE.2020.128360
Corral, S., Sáez, D., Lam, G., Lillo, P., Sandoval, R., Lancellotti, D., et al. (2013). Neurological and neuropsychological deterioration in artisanal gold miners from the town of Andacollo, Chile. Toxicol. Environ. Chem. 95, 344–358. doi: 10.1080/02772248.2013.766191
Coulter, M. A. (2016). Minamata convention on mercury. Int. Leg. Mater. 55, 582–616. doi: 10.5305/intelegamate.55.3.0582
Cresson, P., Travers-Trolet, M., Rouquette, M., Timmerman, C. A., Giraldo, C., Lefebvre, S., et al. (2017). Underestimation of chemical contamination in marine fish muscle tissue can be reduced by considering variable wet: dry weight ratios. Mar. Pollut. Bull. 123, 279–285. doi: 10.1016/J.MARPOLBUL.2017.08.046
Eglitis, L . (2021). Average height of men and women worldwide. Available at: https://www.worlddata.info/average-bodyheight.php (Accessed August 04, 2021).
FAO (2020). The State of World Fisheries and Aquaculture 2020. Sustainability in action. Rome.. doi: 10.4060/ca9229en
FAO and WHO (2018). Joint FAO/WHO food standards Programme: CODEX ALIMENTARIUS COMMISSION, 41st session report of the 12th session of the Codex Committee on contaminants in foods.
Froese, R., and Pauly, D. (2023). FishBase, Available at: www.fishbase.org (Accessed February 2023).
Gochfeld, M., and Burger, J. (2021). Mercury interactions with selenium and sulfur and the relevance of the se: hg molar ratio to fish consumption advice. Environ. Sci. Pollut. Res. 28, 18407–18420. doi: 10.1007/s11356-021-12361-7
Gribble, M. O., Cheng, A., Berger, R. D., Rosman, L., and Guallar, E. (2015). Mercury exposure and heart rate variability: a systematic review. Curr. Environ. Health Rep. 2, 304–314. doi: 10.1007/s40572-015-0053-0
Grieb, T. M., Bowie, G. L., Driscoll, C. T., Gloss, S. P., Schofield, C. L., and Porcella, D. B. (1990). Factors affecting mercury accumulation in fish in the upper Michigan peninsula. Environ. Toxicol. Chem. 9, 919–930. doi: 10.1002/ETC.5620090710
Guzzi, G., Ronchi, A., and Pigatto, P. (2021). Toxic effects of mercury in humans and mammals. Chemosphere 263:127990. doi: 10.1016/j.chemosphere.2020.127990
Hachiya, N. (2006). The history and the present of Minamata disease-entering the second half a century-investigation of causative agent and spread of the pollution occurrence of Minamata disease. Jap. Med. Assoc. J. 49, 112–118.
Hacon, S. D. S., Oliveira-Da-Costa, M., Gama, C. D. S., Ferreira, R., Basta, P. C., Schramm, A., et al. (2020). Mercury exposure through fish consumption in traditional communities in the Brazilian Northern Amazon. Int. J. Environ. Res. Public Health 17, 1–15. doi: 10.3390/ijerph17155269
Jacobson, J. L., Muckle, G., Ayotte, P., Dewailly, É., and Jacobson, S. W. (2015). Relation of prenatal methylmercury exposure from environmental sources to childhood IQ. Environ. Health Perspect. 123, 827–833. doi: 10.1289/ehp.1408554
Jeevanaraj, P., Hashim, Z., Elias, S. M., and Aris, A. Z. (2019). Risk of dietary mercury exposure via marine fish ingestion: assessment among potential mothers in Malaysia. Expo. Health 11, 227–236. doi: 10.1007/s12403-017-0270-x
Lavoie, R. A., Jardine, T. D., Chumchal, M. M., Kidd, K. A., and Campbell, L. M. (2013). Biomagnification of mercury in aquatic food webs: a worldwide meta-analysis. Environ. Sci. Technol. 47, 13385–13394. doi: 10.1021/es403103t
NOAA (2014). Per capita consumption. 104–111. Available at: https://www.st.nmfs.noaa.gov/Assets/commercial/fus/fus14/documents/09_PerCapita2014.pdf (Accessed April 27, 2023).
Page, T., and Left, I. (1996). Chapter 1 Sampling, Comprehensive Analytical Chemistry, Elsevier, 30, 3–4.
Park, J. S., Jung, S. Y., Son, Y. J., Choi, S. J., Kim, M. S., Kim, J. G., et al. (2011). Total mercury, methylmercury, and ethylmercury in marine fish and marine fishery products sold in Seoul, Korea. Food Addit. Contam. Part B Surveill. 4, 268–274. doi: 10.1080/19393210.2011.638087
Pirrone, N., Cinnirella, S., Feng, X., Finkelman, R. B., Friedli, H. R., Leaner, J., et al. (2010). Global mercury emissions to the atmosphere from anthropogenic and natural sources, Atmos. Chem. Phys, 10, 5951–5964. doi: 10.5194/acp-10-5951-2010
R Core Team (2021). R: A language and environment for statistical computing (version de R, 4.2 creo que era). R Foundation for Statistical Computing. Available at: http://www.R-project.org/
Rice, K. M., Walker, E. M., Wu, M., Gillette, C., and Blough, E. R. (2014). Environmental mercury and its toxic effects. J. Prev. Med. Public Health 47, 74–83. doi: 10.3961/jpmph.2014.47.2.74
Roser, M., Ortiz-Ospina, E., and Ritchie, H. (2013). Life expectancy, Published online at OurWorldInData.org. Available at: https://ourworldindata.org/life-expectancy (Accessed October 19, 2023).
Salazar-Camacho, C., Salas-Moreno, M., Marrugo-Madrid, S., Paternina-Uribe, R., Marrugo-Negrete, J., and Díez, S. (2022). A human health risk assessment of methylmercury, arsenic, and metals in a tropical river basin impacted by gold mining in the Colombian Pacific region. Environ. Res. 212:113120. doi: 10.1016/J.ENVRES.2022.113120
Shalini, R., Jeyasekaran, G., Shakila, R. J., Sundhar, S., Arisekar, U., Jawahar, P., et al. (2021). Dietary intake of trace elements from commercially important fish and shellfish of Thoothukudi along the southeast coast of India and implications for human health risk assessment. Mar. Pollut. Bull. 173:113020. doi: 10.1016/J.MARPOLBUL.2021.113020
Sheehan, M. C., Burke, T. A., Navas-Acien, A., Breysse, P. N., McGready, J., and Fox, M. A. (2014). Exposition globale au méthylmercure par la consommation de poisson et fruits de mer et risque de neurotoxicité sur le développement: Un examen systématique. Bull. World Health Organ. 92, 254–269F. doi: 10.2471/BLT.12.116152
Soe, P. S., Kyaw, W. T., Arizono, K., Ishibashi, Y., and Agusa, T. (2022). Mercury pollution from artisanal and small-scale gold Mining in Myanmar and Other Southeast Asian Countries. Int. J. Environ. Res. Public Health 19:6290. doi: 10.3390/IJERPH19106290
Southworth, G. R., Turner, R. R., Peterson, M. J., and Bogle, M. A. (1995). Form of mercury in stream fish exposed to high concentrations of dissolved inorganic mercury. Chemosphere 30, 779–787. doi: 10.1016/0045-6535(94)00407-L
SUBPESCA (2020). Informe sectorial de Pesca y Acuicultura. Departamento de Analisis Sectorial. Available at: https://www.subpesca.cl/portal/618/articles-107685_documento.pdf
Tan, S. W., Meiller, J. C., and Mahaffey, K. R. (2009). The endocrine effects of mercury in humans and wildlife. Crit. Rev. Toxicol. 39, 228–269. doi: 10.1080/10408440802233259
UN Environment . (2019). Global Mercury Assessment 2018. UN Environment Programme, Chemicals and Health Branch Geneva, Switzerland.
USDA and HHS . (2020). Dietary guidelines for Americans, 2020–2025., 9th Edition. Available at: DietaryGuidelines.gov (Accessed October 19, 2023).
USEPA (2001). Methylmercury (MeHg) (CASRN 22967-92-6). Integrated risk information system (IRIS) chemical assessment summary National Center for environmental assessment, 1–43. Available at: https://cfpub.epa.gov/ncea/iris/iris_documents/documents/subst/0073_summary.pdf#nameddest=rfd
USEPA (2011). Revised technical support document: National-scale assessment of mercury risk to populations with high consumption of self-caught freshwater fish in support of the appropriate and necessary finding for coal and oil-fired electric generating units.
Valdelamar-Villegas, J., and Olivero-Verbel, J. (2020). High mercury levels in the indigenous population of the Yaigojé Apaporis National Natural Park, Colombian Amazon. Biol. Trace Elem. Res. 194, 3–12. doi: 10.1007/s12011-019-01760-0
Vieira, H. C., Ramirez, M. M. B., Bordalo, M. D., Rodrigues, A. C. M., Soares, A. M. V. M., Abreu, S. N., et al. (2021). Total and organic mercury in fish from different geographical areas in the North Atlantic Ocean and health risk assessment. Expo. Health 13, 361–373. doi: 10.1007/s12403-021-00388-7
Wickham, H . (2016). Wickham Hadley ggplot2 elegant graphics for data analysis second edition. Available at: http://www.springer.com/series/6991
Keywords: health risk assessment, mercury, Latin America and the Caribbean, fish consumption, mercury intake
Citation: Vergara E, Pancetti F, Zúñiga L, Fabres K and Bahamonde P (2024) Risk map of human intake of mercury through fish consumption in Latin America and the Caribbean. Front. Sustain. Food Syst. 8:1470683. doi: 10.3389/fsufs.2024.1470683
Received: 25 July 2024; Accepted: 11 September 2024;
Published: 24 September 2024.
Edited by:
Matthew Chidozie Ogwu, Appalachian State University, United StatesReviewed by:
Eduardo Esteves, University of Algarve, PortugalCopyright © 2024 Vergara, Pancetti, Zúñiga, Fabres and Bahamonde. This is an open-access article distributed under the terms of the Creative Commons Attribution License (CC BY). The use, distribution or reproduction in other forums is permitted, provided the original author(s) and the copyright owner(s) are credited and that the original publication in this journal is cited, in accordance with accepted academic practice. No use, distribution or reproduction is permitted which does not comply with these terms.
*Correspondence: Paulina Bahamonde, cGF1bGluYS5iYWhhbW9uZGVAdW1heW9yLmNs; Floria Pancetti, cGFuY2V0dGlAdWNuLmNs
Disclaimer: All claims expressed in this article are solely those of the authors and do not necessarily represent those of their affiliated organizations, or those of the publisher, the editors and the reviewers. Any product that may be evaluated in this article or claim that may be made by its manufacturer is not guaranteed or endorsed by the publisher.
Research integrity at Frontiers
Learn more about the work of our research integrity team to safeguard the quality of each article we publish.