- 1National Institute of Agricultural Research, Rabat, Morocco
- 2Laboratory of Agro-Alimentary and Health, Faculty of Sciences and Techniques, Hassan First University of Settat, Settat, Morocco
- 3Human Nutrition, Bioacives and Oncogenetics Team, Faculty of Sciences, Moulay Ismail University, Meknes, Morocco
- 4Higher Institute of Nursing Professions and Health Technical (ISPITS), Marrakech, Morocco
- 5Laboratory of Physical Chemistry of Processes and Materials, Faculty of Sciences and Techniques, Hassan First University, Settat, Morocco
- 6Doctoral School of Geosciences, Faculty of Geography and Geology, Alexandru Ioan Cuza University of Iasi, Iasi, Romania
- 7Preclinical Department, Apollonia University, Iasi, Romania
- 8Doctoral School of Biology, Faculty of Biology, “Alexandru Ioan Cuza” University of Iași, Iasi, Romania
- 9Department of Public Health, Faculty of Veterinary Medicine, Iasi University of Life Sciences, Iasi, Romania
- 10Department of Biology, Faculty of Biology, Alexandru Ioan Cuza University of Iasi, Iasi, Romania
- 11Ioan Haulica Institute, Apollonia University, Iasi, Romania
- 12Academy of Romanian Scientists, Bucharest, Romania
- 13CENEMED Platform for Interdisciplinary Research, “Grigore T. Popa” University of Medicine and Pharmacy of Iasi, Iasi, Romania
Introduction: Phenacoccus solenopsis Tinsley (Hemiptera: Pseudococcidae) is a major pest that causes significant damage to many crops worldwide, necessitating effective control methods.
Methods: This study evaluated five treatments under screenhouse conditions: untreated control, Harmonia convergens (HC) alone, Alternaria murispora (AM) alone, AM+HC, and imidacloprid (positive control). Conducted over two experiments, the study involved weekly monitoring for 5 weeks to assess P. solenopsis and HC densities and treated plants’ visual quality.
Results and discussion: In Experiment 1, AM+HC reduced mealybug densities to 2.0 by week 5, compared to 184.2 in the untreated control. Similarly, in Experiment 2, AM+HC decreased densities to 0.8, while the control had 189.6 mealybugs. Egg counts reflected these trends, with AM+HC showing the lowest counts (1.8 in Experiment 1 and 0.8 in Experiment 2) versus the control’s high counts (282.0 in Experiment 1 and 287.3 in Experiment 2). AM+HC had the highest plant visual quality scores (9.8 in Experiment 1 and 9.9 in Experiment 2), while the control scores were much lower (1.2 and 0.3, respectively). Predator densities peaked at 28.1 for HC alone in Experiment 1 and 32.1 in Experiment 2, with AM+HC reaching 14.7 and 18.2, respectively. Mummy counts were initially higher in AM alone but increased significantly in AM+HC by weeks 3 and 4. Experiment 2 had a higher mean temperature (22.17°C) compared to Experiment 1 (20.78°C), though this did not affect treatment efficacy. In conclusion, AM+HC proved most effective in reducing P. solenopsis densities and maintaining plant health, demonstrating its potential as a sustainable alternative to chemical pesticides.
1 Introduction
The sap-sucking pest Phenacoccus solenopsis Tinsley (Hemiptera: Pseudococcidae) is a highly polyphagous insect that infests a wide variety of cultivated and wild plants, affecting over 200 species across 60 botanical families (Abbes et al., 2024). Originally reported in North America (United States), this species has now spread to more than 50 countries and is recognized as a significant pest in Asia and Africa (Fand and Suroshe, 2015). Its impact is due to its ability to cause direct damage by sucking plant sap and indirect damage by transmitting phytopathogens such as the cotton leaf curl virus (Saeed et al., 2007; Xi et al., 2019), and by secreting honeydew, which leads to sooty mold growth on host plants (Shankarganesh et al., 2022). Indeed, after its first record on tomato plants in Egypt in Ibrahim et al. (2015), P. solenopsis has quickly become an important pest of many economically important crops across North Africa such as Citrus lemon (L.) Osbeck (Rutaceae) in Algeria (Aroua et al., 2020), Opuntia spp. in Morocco (El Aalaoui and Sbaghi, 2021), and Lantana camara L. (Verbenaceae), Lycopersicon esculentum Mill. (Solanaceae), Solanum tuberosum L. (Solanaceae), Bougainvillea glabra Choisy (Nyctaginaceae), Capsicum annuum L. (Solanaceae), and Hibiscus mutabilis L. (Malvaceae) in Tunisia (Abbes et al., 2024). This rapid spread underscores the urgent need for effective control measures.
Integrated Pest Management (IPM) strategies, incorporating a combination of all possible control methods, have garnered increasing attention in recent years. Particularly, natural enemies (predators and parasitoids) are considered crucial for suppressing P. solenopsis populations in nature (Fand and Suroshe, 2015; Chen et al., 2021). Coccinellids are the major predators of P. solenopsis worldwide (Tong et al., 2019). Various ladybirds have successfully impacted P. solenopsis in natural settings worldwide, including Brumoides suturalis (Fabricius) (Ali et al., 2014), Coccinella undecimpunctata Linnaeus (Hameed et al., 2013), Cryptolaemus montrouzieri Mulsant (Khan et al., 2012), Hippodamia convergens Guerin-Meneville (Arif et al., 2011), and Scymnus nubilus Mulsant (Sankar et al., 2011). In Morocco, three ladybird predators—identified as Hippodamia convergens Guérin-Méneville, Scymnus nubilus Mulsant, and Scymnus interruptus (Goeze)—were observed actively feeding on the mealybug in the field (El Aalaoui and Sbaghi, 2021), with H. convergens effectively contributing to sustainable P. solenopsis management in field conditions (El Aalaoui and Sbaghi, 2024).
Enhancing the effectiveness of naturally occurring predators, especially H. convergens, by integrating them with other biological control agents, such as entomopathogenic fungi, can offer a safe and cost-effective method for managing P. solenopsis populations (Pell and Vandenberg, 2002). The potential of entomopathogenic fungi to control P. solenopsis has been demonstrated in numerous laboratory (Nagrare et al., 2011) and field (Sahayaraj and Namachivayam, 2011) studies. Also, combining Alternaria murispora (Ascomycota: Pleosporaceae) with Chilocorus bipustulatus and Exochomus nigripennis (Coleoptera: Coccinellidae) significantly reduced Diaspis echinocacti (Bouché) (Hemiptera: Diaspididae) infestations on Opuntia ficus-indica (L.) Mill without affecting plant quality (El Aalaoui et al., 2025). In the present study, we evaluated the effect of combining the application of the entomopathogenic fungus A. murispora and the predatory ladybird H. convergens for the control of the polyphagous mealybug P. solenopsis on potato under screenhouse conditions.
2 Materials and methods
2.1 Phenacoccus solenopsis culture
The P. solenopsis specimens used in this study were obtained from a colony maintained at the insectarium of the National Institute of Agricultural Research (INRA) in Zemamra, Morocco (32°37′48″ N, 8°42′0″ W, Elevation 165 m). They were reared on sprouted potatoes (Solanum tuberosum L.) under controlled conditions of 25 ± 2°C, 60 ± 10% relative humidity, and a 12:12 h light–dark photoperiod. The initial culture originated from mealybug-infested branches, stems, and leaves of purslane [Portulaca oleracea L. (1753) (Portulacaceae)] collected from a field in the Beni Mellal region, Morocco (32°20′ N, 6°21′ W) in 2020 (El Aalaoui and Sbaghi, 2021).
2.2 Study site and plant growth
The study was conducted in a screenhouse at the INRA’s experimental station in Zemamra. The screenhouse dimensions were 11 m in length, 9 m in width, and 3 m in height, supported by iron pillars, with its sides covered with white fine mesh netting (0.4–1.0 mm). Temperature and relative humidity data inside the screenhouse were recorded at 1-h intervals, starting from the day when natural enemies were released at the height of 2 m above ground throughout the experiment using a thermohygrometer (Testo, Germany). The night temperature was determined from the three lowest daily values (Vinogradova and Reznik, 2013). Before starting the experiments, sprouted potatoes (Solanum tuberosum L.) obtained from the zemamra market were planted in plastic pots (33 cm in diameter and 12 cm in height), with one sprouted potato per pot. The pots were filled with a mixture of fine sand (2/3) and peat (1/3) and placed in a screenhouse to grow. The plants were irrigated as needed. Experiments were conducted on one-month-old potted potato plants (20–35 cm tall). Each plant was artificially infested with 60 adult P. solenopsis of both sexes (approximately 40 females and 20 males) directly on the leaves using a fine hairbrush and allowed to establish and multiply for 21 days.
2.3 Entomopathogenic fungi
The Alternaria murispora isolate (NCBI GenBank Acc. No: PP264308), sourced from the EPF collection at the INRA insectarium in Zemamra, was used in this study. Originally isolated from sterilized cadavers of Dactylopius opuntiae (Cockerell) (Hemiptera: Dactylopiidae), this isolate has previously shown effectiveness in controlling D. opuntiae in both laboratory and greenhouse settings. For cultivation, the fungus was grown on potato dextrose agar (PDA) for 2 weeks at 27°C. Afterward, the cultures were dried, and the resulting conidia were stored in glass tubes following the method outlined by Hansen and Steenberg (2007) at 5°C. prior to the experiment, the viability of the conidia was verified by inoculating PDA plates with a conidial suspension and then incubating them for 24 h at 27°C. After adding sterile 0.02% Tween 80, germination was assessed based on the length of the germ tube, which needed to be at least as long as the conidia diameter for confirmation. The viability test showed 100% germination. For application in the study, a concentration of 108 conidia mL−1 was prepared based on cell counts using a hemocytometer (HGB, Germany) under a binocular microscope (Motic) at 40× magnification.
2.4 Harmonia convergens rearing
Hippodamia convergens individuals used in this research were sourced from a colony raised on P. solenopsis-infested P. oleracea plants at the INRA insectarium in Zemamra. The colony was kept under controlled conditions at a temperature of 26 ± 2°C, relative humidity of 60 ± 10%, and subjected to a photoperiod of 8 h light: 16 h dark (El Aalaoui and Sbaghi, 2024). Prior to release, the predatory ladybirds were starved for 24 h. Ten ovipositing adult females were released per plant. The screenhouse was partitioned into five chambers using insect-proof nets, with treatments randomly assigned to the chambers to prevent movement of H. convergens, P. solenopsis, and A. murispora among treatments.
2.5 Insecticide
In this study, Imipower (imidacloprid 35% SC, Nanjing Red Sun Co. Ltd. – China) was used as a positive control. It was applied at the recommended field rate of 0.75 cm3 per liter of water (Abd El-Mageed et al., 2018).
2.6 Treatments
The experimental treatments included the following: T1 - untreated control, T2 - H. convergens (HC) alone, T3 - A. murispora (AM) alone, T4 - AM+ HC, and T5 - imidacloprid (ICP). Alternaria murispora and imidacloprid were applied at a rate of 50 mL per plant using a 1.5 L Garden Pressure Spray Bottle (Mesto Spritzenfabrik Ernst Stockburger GmbH, Germany). To prevent contamination of neighboring plants, potted plants were treated outside the screenhouse and returned afterwards. Treatments were arranged in a randomized complete block design with each treatment replicated three times, and each replicate consisted of 20 potted potato plants, totaling 300 plants per experiment. Predatory ladybirds were released 5 days after fungus application to mitigate any observed repellency effects noted in earlier laboratory observations (El Aalaoui M, pers. obs.). The experiment was conducted from April 21 to Jun 21, 2023 (Experiment 1), and repeated from April 18 to Jun 18, 2024 (Experiment 2).
2.7 Data collection
Before applying treatments, three randomly selected potted plants per treatment replicate were destructively sampled to assess the initial density of P. solenopsis across all treatments. Monitoring began 1 week after releasing H. convergens predatory ladybirds and continued weekly for five consecutive weeks. For each treatment replicate, three plants were randomly chosen and subjected to destructive sampling. Each plant was carefully examined under a dissecting binocular loupe (Motic) to count eggs and active stages (larvae, nymphs, and adults) of both P. solenopsis and H. convergens found on the apex and all leaves. Additionally, the number of mummified P. solenopsis (whether sporulated or non-sporulated) resulting from A. murispora infection was recorded. All stages of H. convergens were collected and identified to species using specific identification keys (Romero-Nápoles, 1988; Rhoades, 2010). At the end of each experiment (after 5 weeks), the damage caused by P. solenopsis was assessed using a numerical scale from 0 to 10, as outlined by Gettys et al. (2021). This scale measures plant visual quality, with 0 indicating dead plants and 10 indicating plants in excellent condition. This assessment method has been previously used to evaluate the effects of herbicides, salt stress, and other factors (Gettys and Haller, 2012; Smith et al., 2014; Tootoonchi et al., 2020).
2.8 Statistical analysis
For statistical analyzes, we focused on the density of P. solenopsis on the first eight leaves of each potted potato plant. These leaves were selected for their youth, high photosynthetic activity, and preference as feeding sites for mealybugs. They typically harbor the highest densities of P. solenopsis and are the primary foraging area for the nocturnally active predator H. convergens, making them optimal for assessing predation effects. The impact of treatments and exposure time on P. solenopsis densities and plant visual quality was assessed using one-way analysis of variance (ANOVA) in R software version 4.3.2. Significant treatment differences identified by ANOVA were further evaluated using the Student–Newman–Keuls (SNK) multiple range test (Onzo et al., 2013). Additionally, we investigated the effects of A. murispora (AM) alone and A. murispora (AM) + H. convergens (HC) treatments on the number of mummies (i.e., P. solenopsis killed by A. murispora), and the effects of H. convergens (HC) alone and A. murispora (AM) + H. convergens (HC) treatments on H. convergens density. A two-sample t-test was used for comparisons, and ANOVA with SNK post-hoc tests were employed to analyze predator density and mummy counts across different treatments over the weeks. Furthermore, a two-sample t-test was applied to compare temperature and relative humidity (RH) between the two experiments and assess their influence on organism densities. To ensure variance homogeneity, ladybird counts were transformed using log10(x + 1), and proportions were transformed using the arcsine square root transformation before statistical analysis. All statistical analyzes were conducted using R software version 4.3.2.
3 Results
3.1 Experiment 1: efficacy of treatments on Phenacoccus solenopsis density and plant visual quality
Statistical analyzes over multiple weeks revealed significant variations in treatment efficacy for controlling mealybug active stages (Figure 1). Before treatment application, non-significant differences among treatments were observed (F4, 40 = 0.004, p > 0.05). By week 1 after treatment, significant treatment effects were evident (F4, 40 = 1416.000, p < 0.001). The control had the highest mean mealybug active stages, significantly higher than HC, AM, imidacloprid, and AM+HC treatments. This pattern continued into week 2 (F4, 40 = 3277.000, p < 0.001), with imidacloprid and AM+HC treatments showing the lowest mealybug active stages. Throughout weeks 3–5, AM+HC consistently demonstrated the lowest mealybug active stages among treatments (week 3 F4, 40 = 2671.000, p < 0.001; week 4 F4, 40 = 3108.000, p < 0.001; week 5 F4, 40 = 5394.000, p < 0.001). The untreated control exhibited a substantial increase in mealybug active stages over time (F1, 52 = 677.800, p < 2.0 × 10−16). HC treatment significantly reduced the mealybug active stages (F1, 52 = 498.900, p < 2.0 × 10−16) from 113.2 before treatment to 10.8 in week 5. AM treatment also significantly reduced the mealybug active stages (F1, 52 = 96.910, p = 1.8 × 10−13) from 113.11 to 9.8 over the same period. Combined AM+HC treatment maintained consistent control (F1, 52 = 77.580, p = 6.9 × 10−12), decreasing from 113.0 to 2.0. Imidacloprid showed efficacy (F1, 52 = 14.620, p = 3.5 × 10−4), decreasing from 113.1 to 4.7.
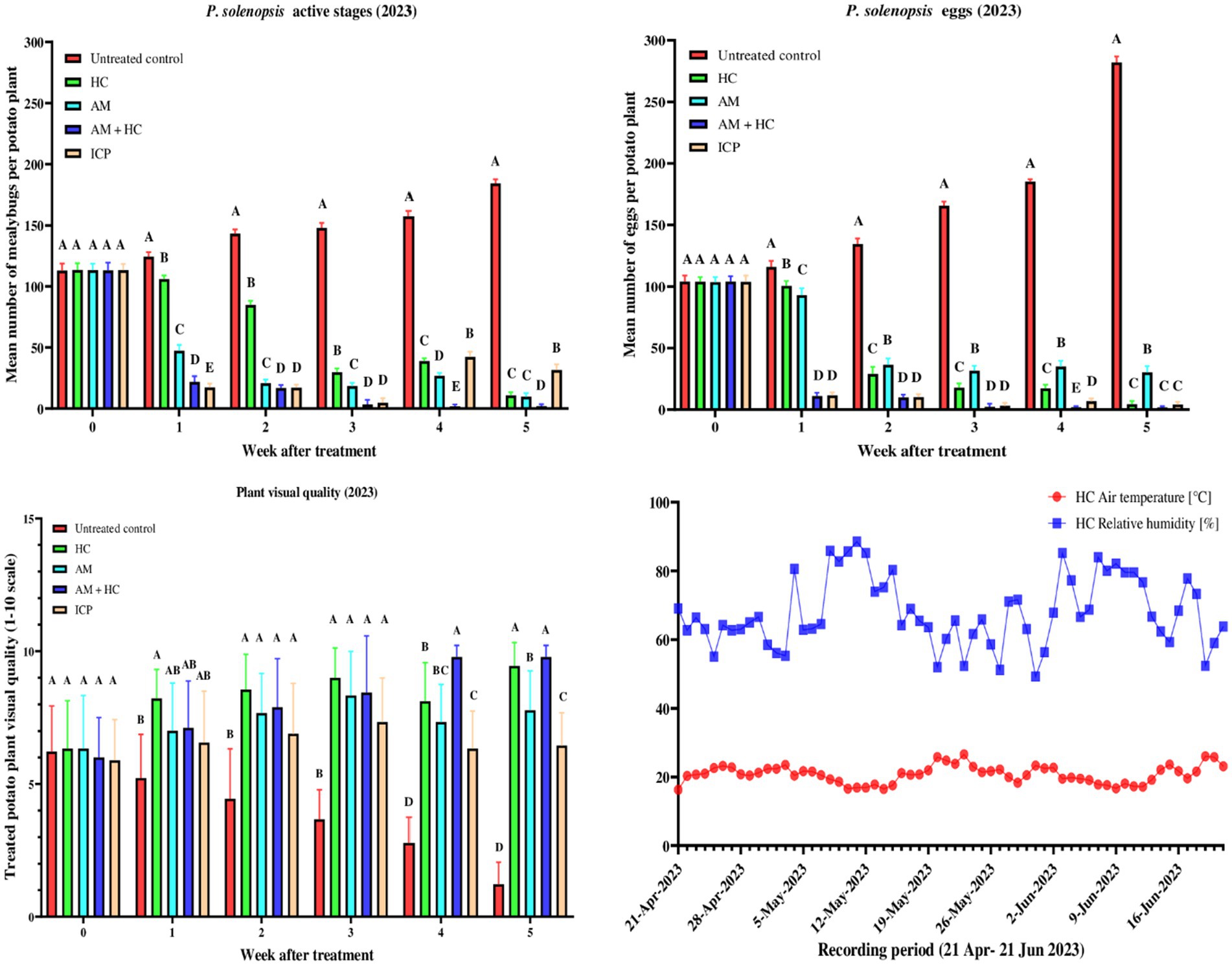
Figure 1. Mean (±SE) active stages and egg densities of P. solenopsis, and treated potato plants’ visual quality following spray application of A. murispora (MA) and imidacloprid (ICP), and release of predatory ladybirds Hippodamia convergens (HC) in the screenhouse during experiment 1 (April 21 to June 21, 2023). Different letters above bars indicate statistical differences (based on Student–Newman–Keuls test, α = 0.05).
Mealybug egg counts also varied significantly across treatments (Figure 1). Before treatment, no significant differences were observed (F4, 40 = 0.020, p = 0.999). By week 1 post-treatment, significant differences emerged (F4, 40 = 1418.000, p < 2.0 × 10−16), with the untreated control having the highest egg count, followed by HC and AM, while imidacloprid and AM+HC showed reduced counts. Significant differences persisted in week 2 (F4, 40 = 1321.000, p < 2.0 × 10−16), week 3 (F4, 40 = 3953.000, p < 2.0 × 10−16), week 4 (F4, 40 = 6461.000, p < 2.0 × 10−16), and week 5 (F4, 40 = 10751.000, p < 2.0 × 10−16). The control consistently had the highest counts, whereas AM+HC treatment consistently maintained the lowest. For the control treatment, time significantly increased egg counts, rising from 104.0 before treatment to 282.0 by week 5 (F1, 52 = 311.000, p < 2.0 × 10−16). HC, AM, AM+HC, and imidacloprid treatments all showed significant decreases in egg counts over time (HC: F1, 52 = 233.400, p < 2.0 × 10−16; AM: F1, 52 = 139.700, p = 2.4 × 10−16; AM+HC: F1, 52 = 55.970, p = 8.5 × 10−10; imidacloprid: F1, 52 = 49.680, p = 4.1 × 10−9).
Plant visual quality scores varied significantly across treatments (Figure 1). Before treatment, no significant differences were observed (F4, 40 = 0.124, p = 0.973). By week 1 post treatment, significant differences emerged (F4, 40 = 3.774, p = 0.0107), with HC treatment scoring highest and the control lowest. Differences intensified in week 2 (F4, 40 = 7.866, p = 8.9 × 10−5), week 3 (F4, 40 = 16.570, p = 4.4 × 10−8), week 4 (F4, 40 = 42.300, p = 7.4 × 10−14), and week 5 post treatment (F4, 40 = 100.100, p < 2 × 10−16). AM+HC and HC treatments consistently had the highest scores (9.78 and 9.44, respectively), while the control had the lowest (1.22). Scores for the control treatment significantly decreased over time (F1, 52 = 74.23, p = 1.4 × 10−11), from 6.2 before treatment to 1.2 at week 5 post treatment. HC treatment showed a significant effect (F1, 52 = 15.900, p = 2.1 × 10−4), maintaining high scores from 6.3 before treatment to 9.4 at week 5 post treatment. AM treatment had a marginally significant effect (F1, 52 = 3.697, p = 0.06), with stable scores from 6.33 before treatment to 8.33 at week 3. AM+HC treatment had a significant effect (F1, 52 = 44.700, p = 1.6 × 10−8), achieving scores of 9.8 at weeks 4 and 5, starting at 6.0 before treatment. Imidacloprid showed no significant effect (F1, 52 = 0.316, p = 0.577), maintaining stable scores from 5.9 before treatment to 7.3 at week 3. These findings underscore the effectiveness of AM+HC and HC treatments in maintaining superior plant visual quality compared to other treatments and the control.
3.2 Experiment 1: impact of Alternaria murispora spray application on Harmonia convergens density
In week 1 post-treatment, there was no significant difference between treatments (Table 1). By week 2, HC density was significantly higher in the HC treatment compared to AM+HC treatment and this trend continued in weeks 3 and 4. In week 5, HC densities were still significantly higher in the HC treatment than in the AM+HC treatment (Table 1). The results showed a significant effect of exposure time after treatment on HC density in both treatments (HC alone, F1, 52 = 4.120, p = 0.0475; AM+HC, F1, 52 = 4.962, p = 0.0303). The highest densities were observed in week 3 for both treatments (28.1 for HC treatment and 14.7 for AM+HC treatment) (Table 1).
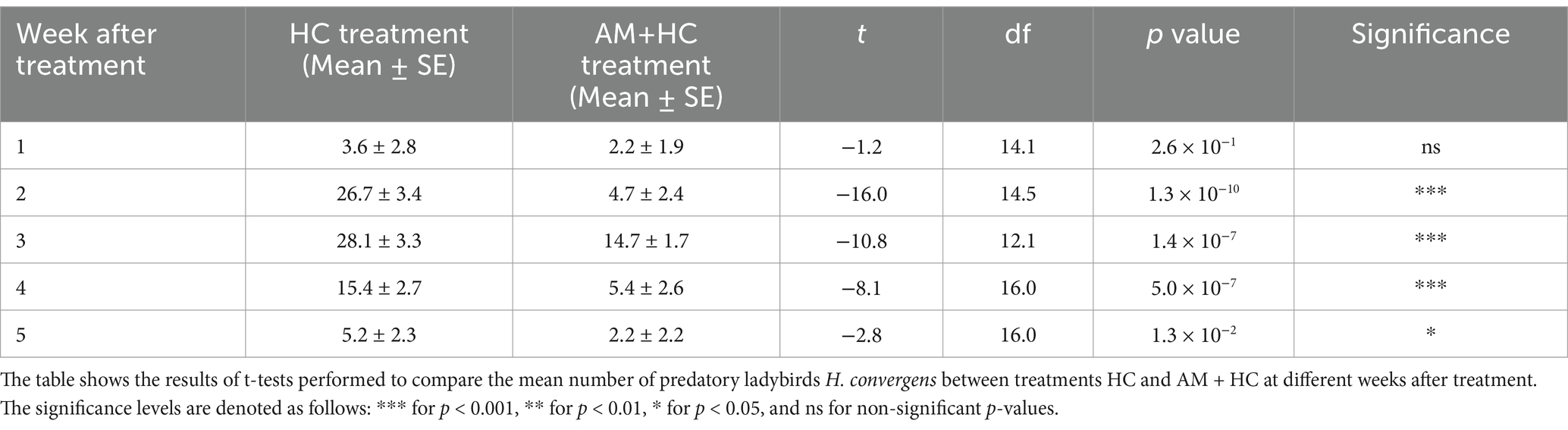
Table 1. Impact of A. murispora (AM) spray application on H. convergens (HC) density released for controlling P. solenopsis under screenhouse conditions from April 21 to June 21, 2023 (Experiment 1).
3.3 Experiment 1: impact of Alternaria murispora alone and Alternaria murispora + Harmonia convergens on Phenacoccus solenopsis mummy
In week 1 post-treatment, significantly more mummies (P. solenopsis killed by AM) were observed in the AM treatment compared to the AM+HC treatment (Table 2). Week 2 post-treatment also showed a significantly higher number of mummies in the AM treatment. In weeks 3 and 4, the AM+HC treatment had significantly more mummies than the AM treatment. By week 5, there was no significant difference between treatments. The results indicated a significant effect of exposure time after treatment on the number of mummies for both treatments (AM: F1, 52 = 155.400, p < 2.0 × 10−16; AM+HC: F1, 52 = 82.960, p = 2.4 × 10−12) (Table 2). These results highlight that the AM+HC treatment initially reduces mummy counts but becomes more effective in weeks 3 and 4 post-treatment compared to AM alone.
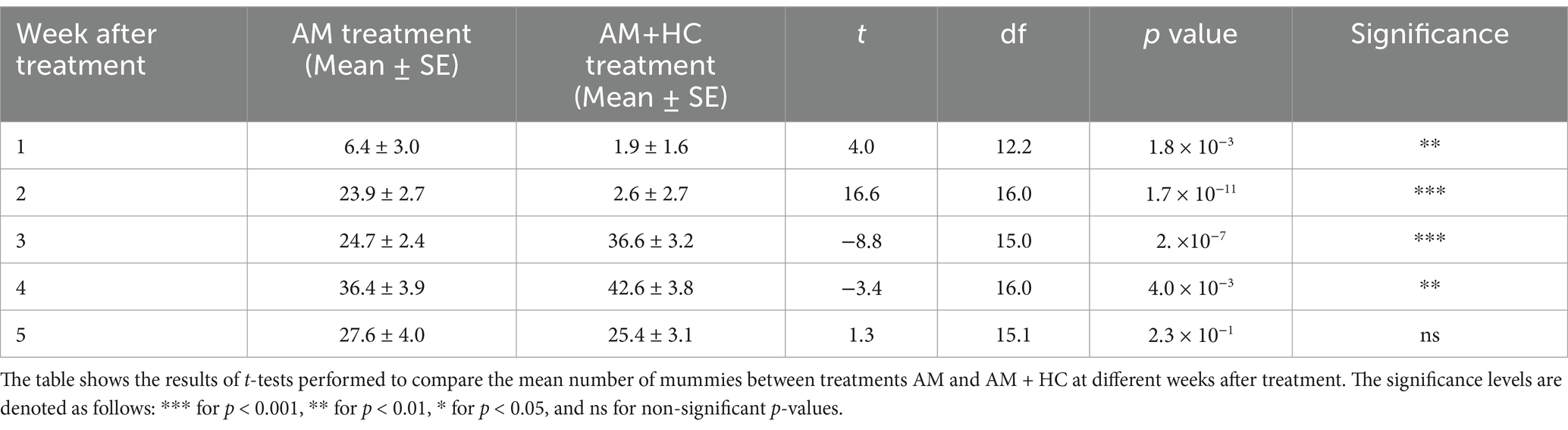
Table 2. Impact of A. murispora (AM) alone and A. murispora (AM) + H. convergens (HC) treatments released for controlling P. solenopsis on the number of mummies (i.e., P. solenopsis killed by A. murispora) under screenhouse conditions from April 21 to June 21, 2023 (Experiment 1).
3.4 Experiment 2: efficacy of treatments on Phenacoccus solenopsis density and plant visual quality
Significant differences in mealybug active stages among treatments were observed throughout the study period (Figure 2). Before treatment application, there were no significant differences among treatments (F4, 40 = 0.007, p = 1). By week 1 post-treatment, treatments showed significant variation (F4, 40 = 1703, p < 2.0 × 10−16), with the control treatment exhibiting significantly higher mean mealybug active stages compared to HC, AM, AM+HC, and Imidacloprid treatments. Weeks 2 to 5 post-treatment similarly showed significant differences (Week 2: F4, 40 = 3385.000, p < 2.0 × 10−16; Week 3: F4, 40 = 4451.000, p < 2.0 × 10−16; Week 4: F4, 40 = 4725.000, p < 2.0 × 10−16; Week 5: F4, 40 = 8126.000, 2.0 × 10−16), with the control treatment consistently exhibiting the highest mean mealybug active stages, followed by HC, AM, Imidacloprid, and AM+HC treatments. The untreated control showed a substantial increase in mealybug active stages over time (F1, 52 = 673.100, 2.0 × 10−16). HC treatment significantly decreased from pre-treatment levels to week 5 post-treatment (F1, 52 = 590.800, 2.0 × 10−16). AM treatment initially had the highest mealybug active stages (F1, 52 = 90.230, p = 6.0 × 10−13) but decreased significantly in later weeks. AM+HC treatment saw a dramatic drop from pre-treatment to week 1 post-treatment and remained consistently low through week 5 (F1, 52 = 57.970, p = 5.2 × 10−10). Imidacloprid treatment had the highest mealybug active stages before treatment, with significantly lower stages in subsequent weeks (F1, 52 = 35.450, p = 2.3 × 10−7). For mealybug eggs, there were no significant variations among treatments before treatment application (F4, 40 = 0.034, p = 0.998). By week 1 post-treatment, significant differences were found (F4, 40 = 1688.000, p < 2.0 × 10−16), with the control having the highest mean egg count, followed by HC, AM treatments, and the lowest in Imidacloprid and AM+HC treatments. Weeks 2–5 also showed significant variation (Week 2: F4, 40 = 1710.000, p < 2.0 × 10−16; Week 3: F4, 40 = 5707.000, p < 2.0 × 10−16; Week 4: F4, 40 = 9895.000, p < 2.0 × 10−16; Week 5: F4, 40 = 15486.000, p < 2.0 × 10−16), with the control consistently having the highest counts, while AM+HC treatment consistently had the lowest. For the control treatment, the mean mealybug eggs were highest in week 5 and significantly lower in earlier weeks (F1, 52 = 315.500, p < 2.0 × 10−16). HC treatment showed significant differences, with the highest mean egg count before treatment and the lowest in week 5 (F1, 52 = 240.300, p < 2.0 × 10−16). AM treatment initially had the highest egg count (F1, 52 = 399.800, p < 2.0 × 10−16) but showed significant reductions in later weeks. AM+HC treatment displayed a notable drop from pre-treatment to week 1 and remained consistently low through Week 5 (F1, 52 = 42.770, p = 2.7 × 10−8). Imidacloprid treatment started with the highest egg count (F1, 50 = 33.750, p = 4.3 × 10−7) but had significantly lower counts in subsequent weeks.
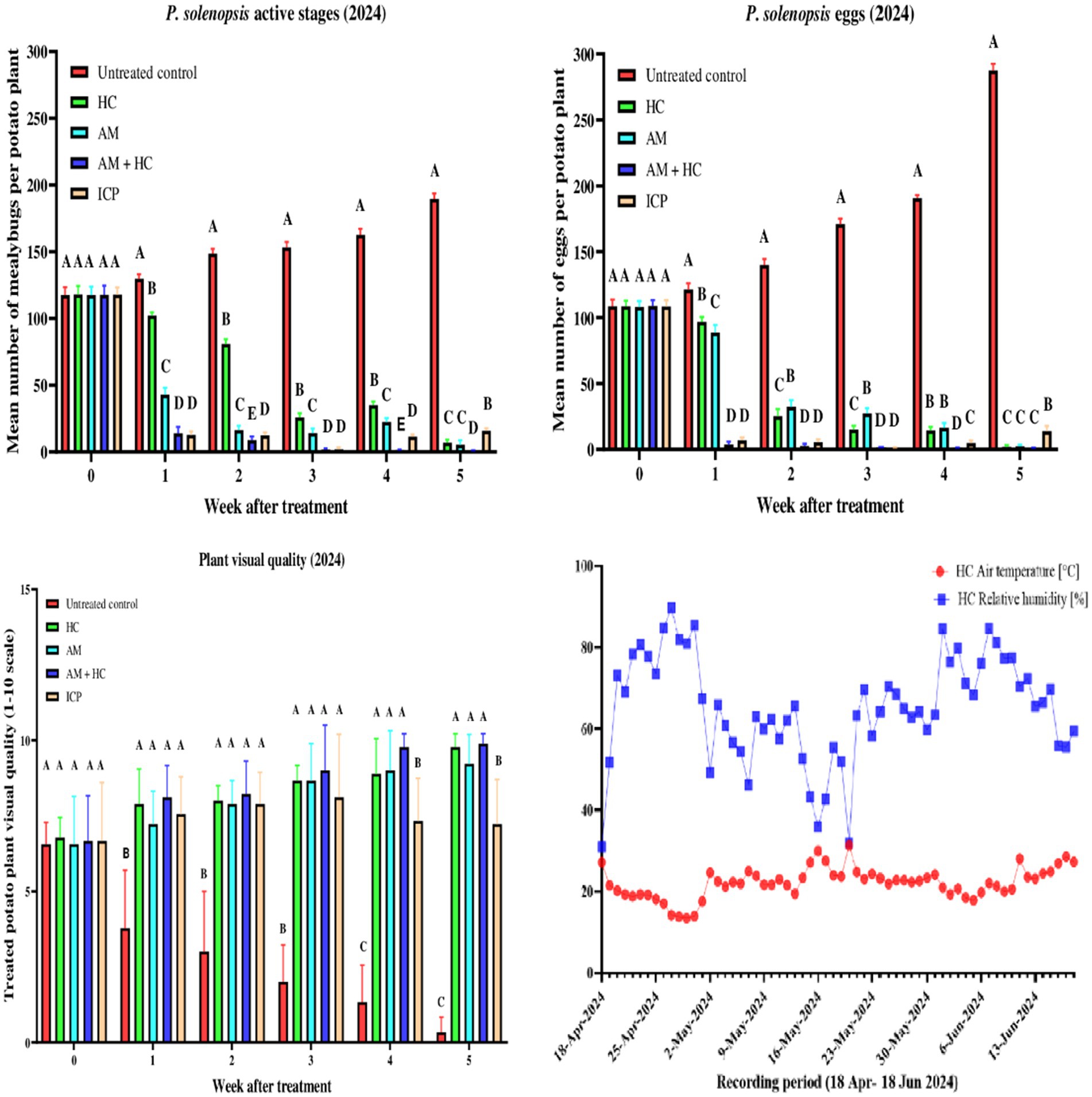
Figure 2. Mean (±SE) active stages and egg densities of P. solenopsis, and treated potato plants’ visual quality following spray application of A. murispora (MA) and imidacloprid (ICP), and release of predatory ladybirds Hippodamia convergens (HC) in the screenhouse during experiment 2 (April 18 to June 18, 2024). Different letters above bars indicate statistical differences (based on Student–Newman–Keuls test, α = 0.05).
Regarding plant visual quality scores, significant differences were observed among treatments from week 1 onwards (F4, 40 = 16.110, p < 6.2 × 10−8). Before treatment application, there were no significant differences among treatments (F4, 40 = 0.041, p = 0.997). Significant differences persisted in weeks 2 (F4, 40 = 31.510, p < 7.0 × 10−12), 3 (F4, 40 = 40.35, p < 1.6 × 10−13), 4 (F4, 40 = 77.940, p < 2.0 × 10−16), and 5 (F4, 40 = 198.200, p < 2.0 × 10−16), with AM+HC and HC treatments maintaining high scores while the control had significantly lower scores. The control treatment exhibited a significant decline in scores from an initial high before treatments to week 5 (F1, 52 = 96.800, p < 0.001). Conversely, HC treatment showed an overall improvement trend, with scores peaking in week 5 (F1, 52 = 70.170, p < 0.001) from its initial score. AM treatment showed relatively stable scores with minor fluctuations (F1, 52 = 36.150, p < 0.001), while AM+HC treatment displayed a similar pattern to HC treatment albeit with slightly lower initial scores (F1, 52 = 52.310, p < 0.001). Imidacloprid treatment did not show significant variations across weeks (F1, 52 = 0.278, p = 0.6), maintaining consistent scores throughout.
3.5 Experiment 2: impact of Alternaria murispora spray application on Harmonia convergens density
In week 1 post-treatment, there were no significant differences in HC densities between treatments (Table 3). By week 2, HC densities were markedly higher in the HC treatment compared to the AM+HC treatment, a trend that persisted through weeks 3 and 4. Even in week 5, HC densities remained significantly elevated in the HC treatment compared to AM+HC treatment (Table 3). The results showed a significant effect of exposure time after treatment on HC density for both HC alone (F1, 52 = 6.347, p = 1.5 × 10−2) and AM+HC (F1, 52 = 10.150, p = 2.4 × 10−3) treatments. Peak densities were observed during week 3 for both treatments, with HC alone reaching 32.1 and AM+HC reaching 18.2 (Table 3).
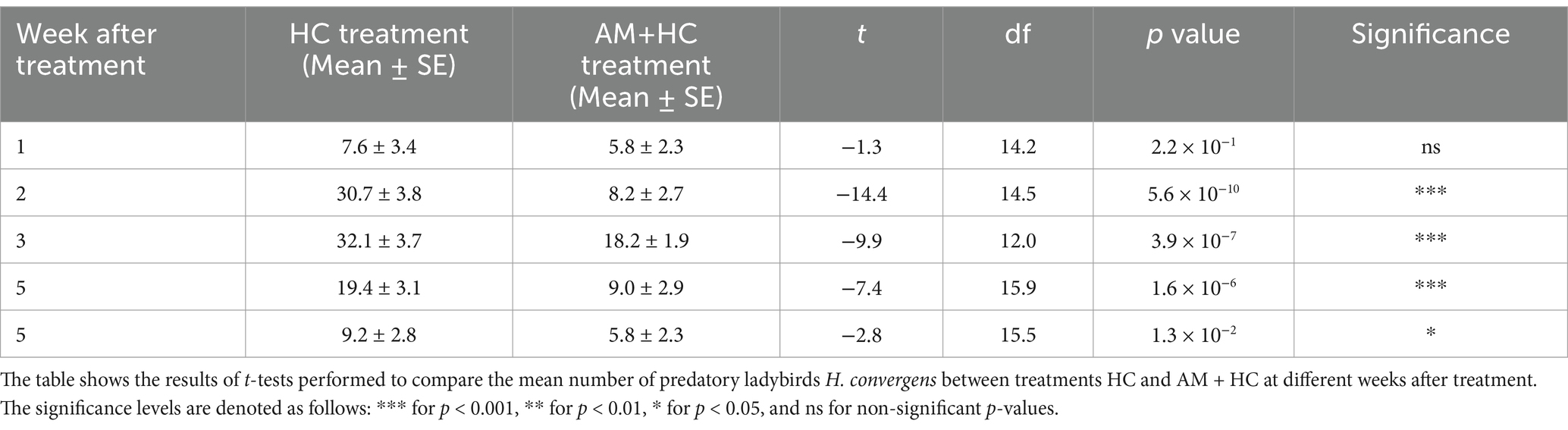
Table 3. Impact of A. murispora (AM) spray application on H. convergens (HC) density released for controlling P. solenopsis under screenhouse conditions from April 18 to June 18, 2024 (Experiment 2).
3.6 Experiment 2: impact of Alternaria murispora alone and Alternaria murispora + Harmonia convergens on Phenacoccus solenopsis mummy
In the initial week post-treatment, no significant difference in mummy counts was observed between the AM and AM+HC treatments (Table 4). However, by week 2, mummy counts were significantly higher in the AM treatment compared to AM+HC. In weeks 3 and 4, the AM+HC treatment exhibited higher mummy counts than AM alone. Week 5 post-treatment showed no significant difference between treatments. Statistical analysis confirmed a significant effect of exposure time after treatment on mummy counts for both AM (F1, 52 = 155.400, p < 0.001) and AM+HC (F1, 52 = 93.370, p < 0.001) treatments, with peak mummy counts observed in weeks 3 and 4 for the AM+HC treatment, suggesting a synergistic effect of combined treatments on pest control efficacy (Table 4).
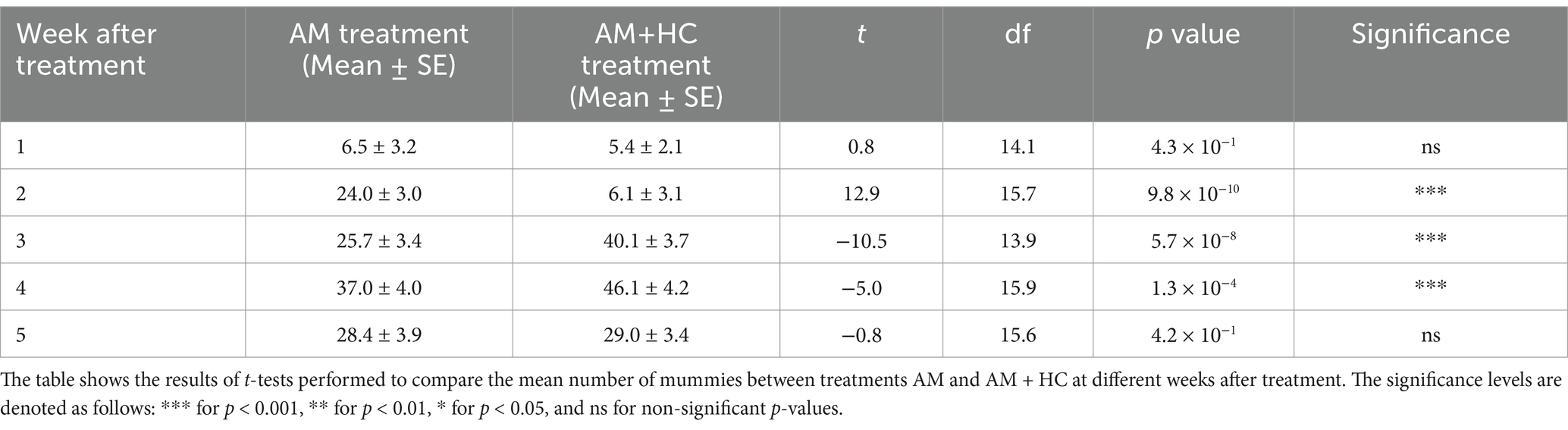
Table 4. Impact of A. murispora (AM) alone and A. murispora (AM) + H. convergens (HC) treatments released for controlling P. solenopsis on the number of mummies (i.e., P. solenopsis killed by A. murispora) under screenhouse conditions from April 18 to June 18, 2024 (Experiment 2).
3.7 Variation of climatic conditions among the two experiments
Comparison of air temperature and relative humidity between Experiment 1 and Experiment 2 revealed distinct differences. The results for air temperature indicated a significant difference (t = −2.4072, df = 107.82, p = 1.8 × 10−2). The mean air temperature was 20.78°C in Experiment 1 and 22.17°C in Experiment 2, with a 95% confidence interval for the difference in means ranging from −2.53°C to −0.25°C, suggesting that Experiment 2 had a higher average temperature. In contrast, the results for relative humidity showed no significant difference between the two experiments (t = 1.2364, df = 114.12, p = 2.2 × 10−1). The mean relative humidity was 67.86% in Experiment 1 and 65.26% in Experiment 2, with the 95% confidence interval for the difference in means between −1.57 and 6.77%. This indicates that the relative humidity levels were similar in both experiments.
4 Discussion
The efficacy of various treatments against P. solenopsis in potato plants was evaluated in this study, focusing on both pest population dynamics and plant health parameters. The experimental treatments, including H. convergens (HC) alone, A. murispora (AM) alone, AM+HC combined treatment, and imidacloprid (ICP), demonstrated varying degrees of effectiveness in controlling mealybug populations and maintaining plant visual quality. Across both experiments, significant differences in mealybug active stages were observed among treatments throughout the study periods. Treatments such as HC alone, AM alone, AM+HC, and imidacloprid used as a positive control consistently showed lower mealybug active stages compared to the untreated control. The combined AM+HC treatment exhibited the most consistent and significant reduction in mealybug active stages across weeks, indicating a synergistic effect of the combined treatment. These results confirm the effectiveness of H. convergens against P. solenopsis as reported in prior studies (Arif et al., 2011; El Aalaoui and Sbaghi, 2024). The effectiveness of the entomopathogenic fungus A. murispora in reducing P. solenopsis populations has also been reported elsewhere in screenhouse conditions (El Aalaoui and Sbaghi, 2024). This also confirms the findings of several authors regarding the potential entomopathogenic properties of Alternaria spp. Alternaria spp., particularly A. alternata, have shown promising entomopathogenic properties against various pests, including Zyginidia pullula (Boheman) (Hemiptera: Cicadellidae), thrips, Oulema gallaeciana (Heyden) (Coleoptera: Chrysomelidae), and Corythucha ciliata (Say) (Hemiptera: Tingidae) (Sharma and Sharma, 2014). Also, Ouguas et al. (2022) reported that Ulocladium spp., Alternaria spp., and their combinations showed substantial Dactylopius opuntiae (Cockerell) (Hemiptera: Dactylopiidae) nymphal mortality ranging from 70 to 90% under laboratory conditions. The differences in treatment efficacy stem from the distinct mechanisms of HC and AM as biological control agents against P. solenopsis. AM’s superior effectiveness in reducing pest populations compared to HC in the present study may be attributed to its specific infection and spread mechanisms, which gradually diminish pest numbers over time (Hajek, 2001). In contrast, HC relies on immediate predation, quickly consuming pests but potentially lacking sustained control, particularly evident across varying prey densities (Omkar and Pervez, 2004). Predators like HC exhibit varied feeding behaviors: at low prey densities, they may not achieve satiation, resulting in partial consumption, while at high densities, they feed until satiation without fully consuming all prey (Omkar and Pervez, 2004). This variability highlights how prey abundance influences predator efficacy in pest management. In addition, our experimental conditions likely facilitated AM’s effectiveness by providing optimal environments for transmission and host infection, supporting its long-term pest management capabilities. Conversely, HC’s rapid predatory activity may offer initial advantages but could be less consistent in achieving sustained pest control efforts over time (Agboton et al., 2013). Alternaria murispora serves as both a foliar endophyte in olive trees (Nicoletti et al., 2020) and an entomopathogenic fungus, highlighting its ecological versatility. Regarding the potential production of mycotoxins by Alternaria fungi, which are found in processed plant foods and agricultural products at low levels without specific regulatory guidelines (Chen et al., 2021); further research into the metabolic profiles of A. murispora is necessary to ensure its safe and effective use in agricultural applications. In the present study, the mealybug egg counts also varied significantly among treatments, with the untreated control consistently having the highest counts throughout the study periods. HC, AM, AM+HC, and imidacloprid treatments all significantly reduced egg counts over time, with the AM+HC treatment consistently maintaining the lowest counts. This underscores the effectiveness of the combined treatment AM+HC in suppressing mealybug reproduction. Generally, in pest management programs, combining natural enemies like predators and parasitoids with entomopathogenic microorganisms presents challenges and varying outcomes. The integration of the predator Typhlodromalus aripo De Leon (Acari: Phytoseiidae) with the fungus Neozygites tanajoae Delalibera (Zygomycetes: Entomophthorales) shows potential for controlling the cassava green mite, Mononychellus tanajoa Bondar (Acari: Tetranychidae), but can negatively affect N. tanajoae populations (Onzo et al., 2013). Success factors include timing, dosage, and the interval between releasing natural enemies and microbial infection (Tamayo-Mejía et al., 2015). Applying Bacillus thuringiensis var. kurstaki 2 days before releasing Habrobracon hebetor (Say) (Hymenoptera: Braconidae) is recommended (Allahyari et al., 2020). Similarly, Beauveria bassiana (Bals.) (Hypocreales: Cordycipitaceae) should be applied before releasing Trichogramma dendrolimi Matsumura (Hymenoptera: Trichogrammatidae) to optimize egg parasitization (Wu et al., 2022). In some cases, spatially separating microbial treatments and natural enemy releases has proven effective (Presa-Parra et al., 2021). Imidacloprid, while effective initially, showed less sustained control over mealybug populations, possibly due to resistance (Fand and Suroshe, 2015).
In both experiments, plant visual quality scores indicated that the HC and AM+HC treatments achieved higher ratings compared to other treatments and the control group. Over time, the control group’s scores decreased, whereas the HC and AM+HC treatments either maintained or enhanced their scores, underscoring their effectiveness in protecting against mealybug damage. Similarly, da Silva et al. (2010) observed reduced damage levels on tomato plants treated with Phytoseiulus longipes Evans (Acari: Phytoseiidae).
Differences in experimental conditions did not significantly impact P. solenopsis populations, as mealybug densities in control treatments were comparable between the two experiments. In both experiments, HC densities were higher in the HC-alone treatment from week 2 onward. Initially, AM treatment produced more mummies than AM+HC treatment, but from weeks 3 to 4, AM+HC exceeded AM alone in mummy counts. The reduction in HC density in the combined treatments could be attributed to HC consuming infected P. solenopsis, which reduces its own reproductive success and longevity due to the lower nutritional quality of infected prey (Agboton et al., 2013). Furthermore, toxins from the entomopathogenic fungi may harm predators consuming infected mealybugs (Boucias and Pendland, 1998). However, our personal observations did not reveal any direct physical antagonism between AM and HC, supporting the hypothesis that this interference is prey-mediated rather than a result of direct fungal toxicity to HC. This supports previous findings that predators are less effective when consuming entomopathogens (Ariori and Dara, 2007). Our results indicate that the competition between HC and AM is asymmetric, with significant effects on HC but not on AM densities. While AM-alone treatments initially produced more mummies, from weeks 3 to 4, mummy counts in the combined AM+HC treatment exceeded those in the AM-alone treatment. This finding suggests that HC might inadvertently contribute to the spread of fungal conidia while preying on P. solenopsis. Such interactions could enhance fungal infection rates, as has been hypothesized in other studies where predators facilitate entomopathogen dissemination (Ariori and Dara, 2007; Onzo et al., 2013). This interference is likely indirect and mediated through their shared prey, P. solenopsis, since AM does not appear to directly harm HC (personal observation). Overall, while HC densities were higher in Experiment 1, the combined AM+HC treatment resulted in more mummies in Experiment 2, likely due to warmer temperatures. Relative humidity was similar across experiments. Thus, using both AM and HC together can enhance mealybug control, even though it may reduce HC populations.
This study has some limitations that should be acknowledged. First, the experiments were conducted under controlled laboratory conditions, which may not fully replicate the complexity of field environments. Factors such as natural prey density, environmental variability, and alternative prey availability were not accounted for, which could influence predator–prey dynamics and fungal efficacy. Second, the study did not directly quantify the nutritional quality of fungal-infected prey or measure toxin levels in HC after consumption of infected prey. Future studies should incorporate biochemical analyzes to confirm the indirect effects observed here. Lastly, while predator-mediated conidial dissemination was hypothesized, we did not explicitly measure the extent to which HC contributed to the spread of AM. Further research is needed to validate this mechanism.
5 Conclusion
Overall, the findings highlight the importance of integrated pest management strategies that combine biological control agents like HC and AM for sustainable pest management in agricultural systems. Further research could explore optimizing application timings and rates for enhanced efficacy and evaluating long-term effects on non-target organisms and ecosystem dynamics.
Data availability statement
The original contributions presented in the study are included in the article/supplementary material, further inquiries can be directed to the corresponding authors.
Ethics statement
Ethical approval was not required for the studies on animals in accordance with the local legislation and institutional requirements because only commercially available established cell lines were used.
Author contributions
MA: Conceptualization, Data curation, Formal analysis, Funding acquisition, Investigation, Methodology, Project administration, Resources, Software, Supervision, Validation, Visualization, Writing – original draft, Writing – review & editing. SR: Conceptualization, Data curation, Formal analysis, Funding acquisition, Investigation, Methodology, Project administration, Resources, Software, Supervision, Validation, Visualization, Writing – original draft. FK: Conceptualization, Data curation, Formal analysis, Funding acquisition, Investigation, Methodology, Project administration, Resources, Software, Supervision, Validation, Visualization, Writing – review & editing. GC: Conceptualization, Data curation, Formal analysis, Funding acquisition, Investigation, Methodology, Project administration, Resources, Software, Supervision, Validation, Visualization, Writing – review & editing. VR: Conceptualization, Data curation, Formal analysis, Funding acquisition, Investigation, Methodology, Project administration, Resources, Software, Supervision, Validation, Visualization, Writing – review & editing. LH: Conceptualization, Data curation, Formal analysis, Funding acquisition, Investigation, Methodology, Project administration, Resources, Software, Supervision, Validation, Visualization, Writing – review & editing. AC: Conceptualization, Data curation, Formal analysis, Funding acquisition, Investigation, Methodology, Project administration, Resources, Software, Supervision, Validation, Visualization, Writing – review & editing. MS: Conceptualization, Data curation, Formal analysis, Funding acquisition, Investigation, Methodology, Project administration, Resources, Software, Supervision, Validation, Visualization, Writing – review & editing.
Funding
The author(s) declare that no financial support was received for the research, authorship, and/or publication of this article.
Conflict of interest
The authors declare that the research was conducted in the absence of any commercial or financial relationships that could be construed as a potential conflict of interest.
Generative AI statement
The authors declare that no Generative AI was used in the creation of this manuscript.
Publisher’s note
All claims expressed in this article are solely those of the authors and do not necessarily represent those of their affiliated organizations, or those of the publisher, the editors and the reviewers. Any product that may be evaluated in this article, or claim that may be made by its manufacturer, is not guaranteed or endorsed by the publisher.
References
Abbes, K., Harbi, A., Wanassi, T., Ricupero, M., Mazzeo, G., Russo, A., et al. (2024). The cotton mealybug Phenacoccus solenopsis Tinsley (Hemiptera: Pseudococcidae) is spreading in North Africa: first report in Tunisia. Orient. Insects 58, 172–186. doi: 10.1080/00305316.2023.2253237
Abd El-Mageed, A., Youssef, N., and Mostafa, M. (2018). Efficacy of some different insecticides against cotton mealybug, Phenacoccus solenopsis Tinsley (Hemiptera: Pseudococcidae) and its associated predators. J. Plant Prot. Pathol. 9, 351–355. doi: 10.21608/jppp.2018.41677
Agboton, B. V., Hanna, R., Onzo, A., Vidal, S., and von Tiedemann, A. (2013). Interactions between the predatory mite Typhlodromalus aripo and the entomopathogenic fungus Neozygites tanajoae and consequences for the suppression of their shared prey/host Mononychellus tanajoa. Exp. Appl. Acarol. 60, 205–217. doi: 10.1007/s10493-012-9630-1
Ali, M., Perveen, R., Yousuf, M. J., Khawja, S., and Amin, M. (2014). Predatory potential of five coccinellid predators against cotton mealy bug Phenacoccus solenopsis (Tinsley) in laboratory and field conditions from Sindh, Pakistan. Pak. Entomol. 36, 7–12.
Allahyari, R., Aramideh, S., Safaralizadeh, M. H., Rezapanah, M., and Michaud, J. P. (2020). Synergy between parasitoids and pathogens for biological control of Helicoverpa armigera in chickpea. Entomol. Exp. Appl. 168, 70–75. doi: 10.1111/eea.12866
Arif, M. J., Gogi, M. D., Abid, A. M., Muhammad Imran, M. I., Shahid, M. R., Sabir Hussain, S. H., et al. (2011). Predatory potential of some native coccinellid predators against Phenacoccus solenopsis, Tinsely (Pseudococcidae: Hemiptera). Pak. Entomol. 33, 97–103.
Ariori, S. L., and Dara, S. K. (2007). Predation of Neozygites tanajoae-infected cassava green mites by the predatory mite, Typhlodromalus aripo (Acari: Phytoseiidae). Agric. Conspec. Sci. 72, 169–172.
Aroua, K., Kaydan, M. B., Ercan, C., and Biche, M. (2020). First record of phenacoccus solenopsis tinsley (Hemiptera: Coccoidea: Pseudococcidae) in Algeria. Entomol. News 129, 63–66. doi: 10.3157/021.129.0109
Boucias, D. G., and Pendland, J. C. (1998). Principles of insect pathology. Boston, MA, USA: Springer.
Chen, H.-Y., Li, H.-L., Pang, H., Zhu, C. D., and Zhang, Y. Z. (2021). Investigating the parasitoid community associated with invasive mealybug Phenacoccus solenopsis in southern China. Insects 12:290. doi: 10.3390/insects12040290
da Silva, F. R., de Moraes, G. J., Gondim, M. G. C., Knapp, M., Rouam, S. L., Paes, J. L. A., et al. (2010). Efficiency of Phytoseiulus longipes Evans as a control agent of Tetranychus evansi baker & pritchard (Acari: Phytoseiidae: Tetranychidae) on screenhouse tomatoes. Neotrop. Entomol. 39, 991–995. doi: 10.1590/S1519-566X2010000600022
El Aalaoui, M., Rammali, S., Bencharki, B., and Sbaghi, M. (2025). Integrated biological control of Diaspis echinocacti (Bouché) on Opuntia ficus-indica (L.) mill (Cactaceae) using predatory ladybirds and fungal pathogens. Crop Prot. 187:106950. doi: 10.1016/j.cropro.2024.106950
El Aalaoui, M., and Sbaghi, M. (2021). First record of the mealybug Phenacoccus solenopsis Tinsley (Hemiptera: Pseudococcidae) and its seven parasitoids and five predators in Morocco. EPPO Bull. 51, 299–304. doi: 10.1111/epp.12745
El Aalaoui, M., and Sbaghi, M. (2024). The feeding and foraging behaviour of three ladybird beetles on Phenacoccus solenopsis (hem.: Pseudococcidae) at different temperatures. Biocontrol Sci. Tech. 34, 452–468. doi: 10.1080/09583157.2024.2351824
Fand, B. B., and Suroshe, S. S. (2015). The invasive mealybug Phenacoccus solenopsis Tinsley, a threat to tropical and subtropical agricultural and horticultural production systems – A review. Crop Prot. 69, 34–43. doi: 10.1016/j.cropro.2014.12.001
Gettys, L. A., and Haller, W. T. (2012). Effect of herbicide-treated irrigation water on four vegetables. Weed Technol. 26, 272–278. doi: 10.1614/WT-D-11-00120.1
Gettys, L. A., Thayer, K. L., and Sigmon, J. W. (2021). Evaluating the effects of acetic acid and d-limonene on four aquatic plants. HortTechnology 31, 225–233. doi: 10.21273/HORTTECH04769-20
Hajek, A. E. (2001). Larval behavior in Lymantria dispar increases risk of fungal infection. Oecologia 126, 285–291. doi: 10.1007/s004420000509
Hameed, A., Saleem, H. M., Ahmad, S., Iqbal, J., Karar, H., and Amin, A. (2013). Predatory potential and life history characteristics of eleven spotted beetle, coccinella undecimpunctata l. reared on cotton mealybug, Phenacoccus solenopsis Tinsley. Pak. J. Zool. 45, 1555–1562.
Hansen, L. S., and Steenberg, T. (2007). Combining larval parasitoids and an entomopathogenic fungus for biological control of Sitophilus granarius (Coleoptera: Curculionidae) in stored grain. Biol. Control 40, 237–242. doi: 10.1016/j.biocontrol.2006.09.012
Ibrahim, S. S., Moharum, F. A., and Abd El-Ghany, N. M. (2015). The cotton mealybug Phenacoccus solenopsis Tinsley (Hemiptera: Pseudococcidae) as a new insect pest on tomato plants in Egypt. J. Plant Prot. Res. 55, 48–51. doi: 10.1515/jppr-2015-0007
Khan, H. A. A., Sayyed, A. H., Akram, W., Raza, S., and Ali, M. (2012). Predatory potential of Chrysoperla carnea and Cryptolaemus montrouzieri larvae on different stages of the mealybug, Phenacoccus solenopsis: A threat to cotton in South Asia. J. Insect Sci. 12, 1–12. doi: 10.1673/031.012.14701
Nagrare, V. S., Kranthi, S., Kumar, R., Dhara Jothi, B., Amutha, M., Deshmukh, A. J., et al. (2011). Compendium of cotton mealybugs. Nagpur: Central Institute for Cotton Research, 42.
Nicoletti, R., Di Vaio, C., and Cirillo, C. (2020). Endophytic fungi of olive tree. Microorganisms 8:1321.
Omkar,, and Pervez, A. (2004). Functional and numerical responses of Propylea dissecta (Col., Coccinellidae). J. Appl. Entomol. 128, 140–146. doi: 10.1111/j.1439-0418.2004.00824.x
Onzo, A., Bello, I. A., and Hanna, R. (2013). Effects of the entomopathogenic fungus Neozygites tanajoae and the predatory mite Typhlodromalus aripo on cassava green mite densities: Screenhouse experiments. BioControl 58, 397–405. doi: 10.1007/s10526-013-9508-0
Ouguas, Y., Cherkaoui, B., Bouchtalla, A., Chakhmani, A., and Ilham, M. (2022). Larvicidal effect of three entomopathogenic fungi on the carmine scale of the prickly pear. Acta Horticult. 1349, 195–202. doi: 10.17660/ActaHortic.2022.1349.27
Pell, J. K., and Vandenberg, J. D. (2002). Interactions among the aphid Diuraphis noxia, the entomopathogenic fungus Paecilomyces fumosoroseus and the coccinellid Hippodamia convergens. Biocontrol Sci. Tech. 12, 217–224. doi: 10.1080/09583150120124478
Presa-Parra, E., Hernández-Rosas, F., Bernal, J. S., Valenzuela-González, J. E., Martinez-Tlapa, J., and Birke, A. (2021). Impact of Metarhizium robertsii on adults of the parasitoid Diachasmimorpha longicaudata and parasitized Anastrepha ludens larvae. Insects 12:125. doi: 10.3390/insects12020125
Rhoades, M. H. (2010). Key to first and second instars of six species of Coccinellidae (Coleoptera) from alfalfa in Southwest Virginia author(s): Mary H. Rhoades source. J. N. Y. Entomol. Soc. 104, 83–88.
Romero-Nápoles, J. (1988). Características morfológicas útiles para diferenciar Hippodamia convergens Guerin de H. koebelei Timberlake (Coleoptera: Coccinellidae), en el área de Chapingo, México. Folia Entomol. Mex. 76, 45–54.
Saeed, S., Ahmad, M., Ahmad, M., and Kwon, Y. J. (2007). Insecticidal control of the mealybug Phenacoccus gossypiphilous (Hemiptera: Pseudococcidae), a new pest of cotton in Pakistan. Entomol. Res. 37, 76–80. doi: 10.1111/j.1748-5967.2007.00047.x
Sahayaraj, K., and Namachivayam, S. (2011). Field evaluation of three entomopathogenic fungi on groundnut pests. Tropicultura 29, 143–147.
Sankar, C., Marimuthu, R., Saravanan, P., Jeyakumar, P., Tanwar, R. K., Sathyakumar, S., et al. (2011). Predators and parasitoids of cotton mealybug, Phenacoccus solenopsis Tinsley (Hemiptera: Pseudococcidae) in Perambalur district of Tamil Nadu. J. Biol. Control. 25, 242–245.
Shankarganesh, K., Ricupero, M., and Sabtharishi, S. (2022). Field evolved insecticide resistance in the cotton mealybug Phenacoccus solenopsis and its direct and indirect impacts on the endoparasitoid Aenasius arizonensis. Sci. Rep. 12, 16764–16713. doi: 10.1038/s41598-022-20779-3
Sharma, I., and Sharma, A. (2014). Use of Alternaria spp. as a Pest control agent: A review. World Appl. Sci. J. 31, 1869–1872. doi: 10.5829/idosi.wasj.2014.31.11.83163
Smith, H. C., Ferrell, J. A., and Koschnick, T. J. (2014). Flurprimidol performance on ornamental species in relation to trimming time and method of application. HortScience 49, 1305–1308. doi: 10.21273/hortsci.49.10.1305
Tamayo-Mejía, F., Tamez-Guerra, P., Guzmán-Franco, A. W., and Gomez-Flores, R. (2015). Can Beauveria bassiana Bals. (Vuill)(Ascomycetes: Hypocreales) and Tamarixia triozae (Burks)(Hymenoptera: Eulophidae) be used together for improved biological control of Bactericera cockerelli (Hemiptera: Triozidae)? Biol. Control 90, 42–48. doi: 10.1016/j.biocontrol.2015.05.014
Tong, H.-J., Yan, A. O., Li, Z.-H., Ying, W., and Jiang, M.-X. (2019). Invasion biology of the cotton mealybug, Phenacoccus solenopsis Tinsley: current knowledge and future directions. J. Integr. Agric. 18, 758–770. doi: 10.1016/S2095-3119(18)61972-0
Tootoonchi, M., Gettys, L. A., Thayer, K. L., Markovich, I. J., Sigmon, J. W., and Sadeghibaniani, S. (2020). Ecotypes of aquatic plant vallisneria americana tolerate different salinity concentrations. Diversity 12:65. doi: 10.3390/d12020065
Vinogradova, E. B., and Reznik, S. Y. (2013). Induction of larval diapause in the blowfly, Calliphora vicina R.-D. (Diptera, Calliphoridae) under field and laboratory conditions. Entomol. Rev. 93, 935–941. doi: 10.1134/S0013873813080010
Wu, Y., Fang, H., Liu, X., Michaud, J. P., Xu, H., Zhao, Z., et al. (2022). Laboratory evaluation of the compatibility of Beauveria bassiana with the egg parasitoid Trichogramma dendrolimi (Hymenoptera: Trichogrammatidae) for joint application against the oriental fruit moth Grapholita molesta (Lepidoptera: Tortricidae). Pest Manag. Sci. 78, 3608–3619. doi: 10.1002/ps.7003
Keywords: biological control, integrated pest management, mealybug infestation, plant health, pest density
Citation: El Aalaoui M, Rammali S, Kamal FZ, Calin G, Rarinca V, Hritcu LD, Ciobică A and Sbaghi M (2025) Effectiveness of the entomopathogenic fungus Alternaria murispora and the predatory ladybird Harmonia convergens in controlling Phenacoccus solenopsis (Tinsley). Front. Sustain. Food Syst. 8:1469247. doi: 10.3389/fsufs.2024.1469247
Edited by:
Anindya Chanda, Mycologics LLC, United StatesReviewed by:
Xiang-Shun Hu, Northwest A&F University, ChinaFarhan Mahmood Shah, Bahauddin Zakariya University, Pakistan
Copyright © 2025 El Aalaoui, Rammali, Kamal, Calin, Rarinca, Hritcu, Ciobică and Sbaghi. This is an open-access article distributed under the terms of the Creative Commons Attribution License (CC BY). The use, distribution or reproduction in other forums is permitted, provided the original author(s) and the copyright owner(s) are credited and that the original publication in this journal is cited, in accordance with accepted academic practice. No use, distribution or reproduction is permitted which does not comply with these terms.
*Correspondence: Gabriela Calin, bV9nYWJyaWVsYTIwMDRAeWFob28uY29t; Viorica Rarinca, cmFyaW5jYV92aW9yaWNhQHlhaG9vLmNvbQ==