- 1College of Food Science and Technology, Henan Agricultural University (HAU), Zhengzhou, China
- 2Department of Agricultural, Forest and Food Sciences (DISAFA), University of Turin (UNITO), Grugliasco, Italy
To maximize the initial quality going to storage and maintain the postharvest antioxidant property of the minimally processed corn salad, the synergistic effects of culture systems (traditional soil culture system [TCS] vs. soilless culture system [SCS]), washing practices (unwashed vs. washed), packaging methods (flow pack bag [FPB] vs. flow pack tray [FPT]), and storage temperatures (4°C vs. 8°C) on the antioxidant property of minimally processed corn salad (Valerianella olitoria L.) was investigated during 7 days of storage. The results showed that SCS-grown corn salad contained significantly higher levels of total ascorbic acid (TAA) (25.6%), ascorbic acid (AA) (17.5%), total phenols (TP) (57.0%), and antioxidant capacity (AC) (67.0%), but lower levels of chlorophyll and carotenoid than TCS. TAA, AA, and TP were the main contributors to AC, with highly positive correlations between them. Washing practice induced a transient increase in AC value only on processing day (d0). The AC value was significantly higher in SCS-grown leaves than in TCS-grown leaves during the storage period. FPT packaging had a considerable impact on pigments preservation and DHA generation, which was highly culture system-depended. The storage temperature showed no significant influence on overall quality. Browning enzyme (phenylalanine ammonia-lyase [PAL], polyphenol oxidase [PPO], peroxidase [POD]) activities were significantly influenced by factors tested and changed over time. However, no visible browning spots were observed at the end of storage. After 7 days of storage, all leaves were still fresh and marketable with the largest fresh weight loss of 0.38%. Conclusively, the synergism of SCS, washing practice, and FPT favored obtaining high-quality raw material at harvest and maintaining the postharvest antioxidant property of minimally processed corn salad during storage. This unique finding not only offers an innovative and feasible strategy for the fresh-cut industry to produce higher-quality products that meet the growing expectations and demands of modern consumers, but also emphasizes the significance of integrating preharvest and postharvest factors within the fresh supply chain.
1 Introduction
Corn salad (Valerianella Olitoria L.), also known as lamb’s lettuce, is one of the most promising minimally processed leafy vegetables with pleasant taste and dietary characteristics (Fontana and Nicola, 2009; Schmitzer et al., 2021; Skrypnik et al., 2021). Its rosette-opposing leaves are rich in bioactive compounds such as phenolic compounds carotenoids, ascorbic acid, and flavonoids (Schmitzer et al., 2021). A total of 35 types of phenolic compounds, including individual phenolic acids, flavanones, flavanols and flavanones, 7 xanthophyll, and 4 carotenes were identified in corn salad leaves (Hernández et al., 2021). In particular, corn salad contains more ascorbic acid than the most commonly consumed leafy vegetables, such as lettuce and spinach (Hernández et al., 2021). Corn salad is usually marketed with cut leaves or whole rosette formed by 5–6 leaves, known as minimally processed leafy greens. These single leaves or loose canopies are delicate and susceptible to rapid water loss and mechanical injuries, which accelerates postharvest quality deterioration. This leads to symptoms such as wilting, yellowing, browning/black spots, nutrient degradation, and a shortened shelf life (Ariffin et al., 2017; Zhan, 2009). In view of this, novel strategies must be implemented during preharvest raw material production, harvest processing, and postharvest storage to ensure the production of high-quality raw materials for storage and to minimize quality loss in the final product when it reaches consumers.
Preharvest factors affect the raw material’s visual quality, nutritional composition, and postharvest shelf life (Yang et al., 2022). Producers of leafy vegetables attempt to optimize yield and quality by enhancing cultural inputs, such as culture systems. Being an alternative to the traditional culture system (TCS) in soil, the soilless culture system (SCS) has been increasingly applied as an innovative cultural technology to produce many leafy vegetables with high production and enhanced postharvest shelf life (Nicola et al., 2016; Pignata et al., 2020; Zhan et al., 2022). Scientists and extension specialists are increasingly adopting this system as an efficient method for producing baby leafy vegetables with a short cultural cycle, high added value, and desirable characteristics for the fresh-cut supply chain (Nicola et al., 2016). Earlier studies demonstrated that corn salad grown in the SCS system had a greater fresh mass, reached the market earlier, and contained lower nitrate content than TCS (Fontana and Nicola, 2009; Manzocco et al., 2011). Despite these contributions, the knowledge of SCS affecting corn salad nutritional quality and postharvest behaviors is lacking.
Successful production is the first part of the supply chain; optimum processing practices and storage conditions are equally vital to keep minimally processed produce postharvest quality and extend the shelf life. Washing is a key process in preparing minimally processed produce before packaging. It aims to remove released cell fluids from the cutting surface, sanitize the materials, and lower the raw material temperature (Kenny and O’Beirne, 2009). The majority of the research studies focused on the effects of sanitizer type on minimally processed produce quality and safety (Martínez-Sánchez et al., 2006). Little attention has been paid to the washing operation itself, affecting material quality (Toivonen and Stan, 2004; Rux et al., 2017). We observed that washing water became slightly green after washing leafy vegetables, especially baby leaves, due to the dissolution of pigments, implying that washing has the potential to decrease pigments in delicate produce and thus deteriorate the visual quality. In addition, the washing may affect antioxidant levels through leaching into processing water or other means (Kenny and O’Beirne, 2009). However, little information has been published concerning the impact of washing treatments on the overall quality of minimally processed corn salad.
Following washing and drying operations, the produce is packaged to protect it from mechanical injuries and contamination, ensuring that it is presented as a convenient and efficient unit for consumers. Currently, the most widely used and studied method is modified atmosphere packaging (MAP), which can be generated either by the respiration of fresh-cut produce (passive MAP) or by gas flushing (active MAP) (Jasper et al., 2021). Minimally processed baby leaves are delicate and perishable and must be protected, particularly from mechanical damage during shipping and marketing. For this reason, packaging accessories such as plastic trays or bowls are usually used as supports to prevent compression injuries or reduce collision injuries during shipping and marketing. Despite the wide application of these packaging accessories in the fresh-cut industry, the evaluations of their benefits on produce over quality are still unclear according to existing literature.
The packaged fresh-cut products must be stored at low temperatures (<7°C) to slow the respiration rate, enzymatic processes, and microbial activity. Generally, the recommended storage temperature for many leafy vegetables is between 0 and 4°C, although the optimal storage temperature can vary from species to species. This temperature range helps maintain turgidity and slows microbial contamination (Jacxsens et al., 2002). Despite the well-documented benefit of consistently low temperatures throughout the supply chain, strict temperature control throughout the entire cold chain remains a challenge in real commercial circumstances. The wholesalers and retailers generally keep the produce slightly above the recommended temperature due to cost savings or lack of energy and refrigeration equipment. The open display cases, widely used in food supermarkets and grocery stores, maintained an average temperature of over 7°C (Brenes et al., 2020). Understanding the threshold of higher temperature tolerance is necessary to develop a good fit between produce values and expenditure.
Taking into account all of the above reasons and the lack of basic studies on the multifactor involved in the fresh-cut supply chain affecting minimally processed corn salad quality and postharvest behaviors, this study aimed to investigate the synergistic effects of the preharvest culture system (traditional culture system [TCS] vs. soilless culture system [SCS]), washing practice (washed vs. unwashed), package method (flow pack bag [FPB] vs. flow pack tray [FPT]), and postharvest storage temperature (4°C vs. 8°C) on the antioxidant property of minimally processed corn salad during 7 days of shelf life. Parameters related to antioxidant properties presented by total phenolics (TP), total ascorbic acid (TAA), ascorbic acid (AA), dehydroascorbic acid (DHA), and antioxidant capacity (AC) were analyzed at harvest (d0), 1-day (d1), and 7-day (d7) shelf life. In addition, phenolic compounds metabolic enzymes activity of PAL (phenylalanine ammonia-lyase), PPO (polyphenol oxidase), and POD (peroxidase) were assayed. The pigments and fresh weight loss were also detected to ensure the products were acceptable by marketed during 7 days of storage.
2 Materials and methods
2.1 Plant production and treatments
For the traditional culture system (TCS), the mechanical sowing in pressed peat cubes occurred in the local nursery Piovano Farm, Trofarello (44° 59′ 41” N; 7° 44′ 54″ E), Italy. The seedlings were transplanted with their peat cubes in the soil and arranged in a grid, spaced 0.10 m between rows and 0.10 m within rows, covering a total surface of 26 m2. The plant density was approximately 1,000 plants per m2. The plants were overhead irrigated and fertilized with organic rabbit manure during plant growing. The TCS was managed by the farmer. The harvest of the whole canopy occurred 90 days after sowing. For the soilless culture system (SCS), the manual sowing in a polystyrene 60-cell tray happened in the same local nursery. Each tray (size, 0.153 m2; 60-cell, 44-mm top, and 25-mm lower diameter, respectively) was filled with specific peat-based horticultural medium (“Neuhaus Huminsubstrat N17”; Klasmann-Deilmann, Geeste-Groß Hesepe, Germany), and contained 180 plants with three plants per hole. The trays were moved into the greenhouse after germination in a controlled chamber at 20°C. A total of 72 trays were arranged on a surface of 26 m2. Plant density was approximately 1,180 plants per m2. During plant growing, the trays were continuously floating in a nutrient solution containing macronutrients (NH4)2SO4 (0.8 mmol L−1), K2SO4 (1.0 mmol L−1), KH2PO4 (2.0 mmol L−1), Ca(OH)2 (1.0 mmol L−1), MgSO4·7H2O (2.0 mmol L−1), NH4NO3 (3.2 mmol L−1), and microelements (Micral® Aicar Spa, Ronco Scrivia, GE, Italy at 0.16 g L−1) with pH range 5.5–6.5. The pH and electrical conductivity of the nutrient solution were checked weekly and kept close to 6.0 and 2,000 μS cm−1, respectively. The harvest of the whole canopy occurred 81 days after sowing.
At harvest, for each culture system and replication, 2 kg of raw material sample was washed with tap water without any chemical sanitizers. The leaf excess water was removed by centrifugation. At the same time, another 2 kg sample was unwashed. Successively, both the washed and unwashed samples were packaged comparing two packages. An 80-g sample was packaged in (1) polypropylene flow pack bag (FPB) (size: 250 mm × 350 mm) with oxygen permeability of 1,330 cm3 m−2 d−1 (Alvapack s.r.l.; Bologna, Italy); (2) flow pack bag + trays (FPT) (top, 190 mm × 145 mm; bottom, 140 mm × 100 mm; high, 55 mm, respectively) and wrapped with the same polypropylene flow pack. Subsequently, the packaged samples were stored in refrigerators (Medika 600; Fiocchetti Cold Manufacturer, Luzzara, (RE), Italy) at 4°C and 8°C for a 7-day shelf life. Sampling happened at harvest (d0), d1, and d7 of storage. The statistical design was a randomized complete block design (RCBD) with three replications during plant growth and shelf life. The experimental design consisted of 2 culture systems × 2 washing practices × 2 packaging methods × 2 storage temperatures × 3 blocks × 3 sample days = 144 samples during shelf life.
2.2 Parameters analysis
2.2.1 Pigments
In brief, 2 g of fresh tissue was blended and extracted in 10 mL 80% acetone/water (v/v) solution overnight at 4°C in darkness. The extract was filtered and used for the spectrophotometric determination of chlorophyll a (Chl a), chlorophyll b (Chl b), and carotenoids at wavelengths of 662, 645, and 470 nm, respectively. The quantity of these pigments was calculated according to the Lichtenthaler and Wellburn (1983) formulas and expressed as milligrams per gram of fresh weight (mg g−1 FW).
2.2.2 Enzyme activities assay
PAL activity was carried out by homogenizing 0.5 g of fresh tissue in 4 mL of 50-mM iced sodium phosphate buffer solution (PBS, pH 8.0) (Sigma–Aldrich GmbH, Steinheim, Germany) containing 0.01-g polyvinylpyrrolidone (Merck KGaA, Darmstadt, Germany). The homogenate was centrifuged at 20,000 g at 4°C for 20 min. A 0.1-ml supernatant was incubated in 0.5-ml 50-mM L-phenylalanine (Sigma–Aldrich GmbH, Steinheim, Germany) and 1.40-ml 50 mM PBS for 1 h at 40°C. The absorbance was measured at 290 nm. The enzyme activity was expressed as micromole cinnamic acid (Sigma–Aldrich GmbH, Steinheim, Germany) per hour per gram of fresh weight (μmol cinnamic acid h−1 g−1 FW). PPO and POD activities were measured by homogenizing 0.5 g of tissue in 4 mL of 50-mM PBS (pH 7.0). The homogenate was centrifuged at 20,000 g at 4°C for 20 min, and the supernatant was used as the crude enzyme source. PPO activity was determined by incubating 0.1 mL extract in PBS containing 2.5 mM of pyrocatechol (Sigma–Aldrich GmbH, Steinheim, Germany) at 25°C for 30 min before absorbance was recorded at 480 nm. One unit of PPO activity was defined as the amount of enzyme that causes a change of 0.01 in absorbance per minute and expressed as a unit per gram of fresh weight (Unit g−1 FW). The POD reaction mixture contained 25 mM PBS (pH 7.0), 0.05% guaiacol (Sigma–Aldrich, Inc., St. Louis, MO, USA), 10 mM H2O2 (Sigma–Aldrich GmbH, Seelze, Germany) and crude enzyme extract. POD activity was measured as an increase in absorbance at 470 nm due to guaiacol oxidation and expressed as ∆Aλ per min per gram of fresh weight (∆Aλ min−1 g−1 FW).
2.2.3 Ascorbic acid and dehydroascorbic acid assay
AA and DHA were assayed by homogenizing 5 g of fresh leaves in 5 mL 6% of freezing trichloroacetic acid/water (w/v) (Sigma–Aldrich GmbH, Steinheim, Germany). The homogenate was centrifuged at 15,000 g at 4°C for 10 min, and the supernatant was collected. The precipitates were re-extracted twice using the same procedure. Three supernatants were mixed and made up to a final volume of 50 mL. Total AA and AA were analyzed according to the methodology of Kampfenkel et al. (1995). The DHA content was computed from the total AA and AA differences. The results were expressed as milligrams per 100 g of fresh weight (mg 100 g−1 FW) based on the calibrations that were compared to standard curves produced by freshly prepared L-ascorbic acid (Sigma–Aldrich Inc., St. Louis, MO, USA) (10–250 mg L−1) and dehydroascorbic acid (Sigma–Aldrich GmbH, Steinheim, Germany) (1–100 mg L−1).
2.2.4 Total phenols and antioxidant capacity assay
A 1 g of fresh tissue was homogenized in 5-ml iced methanol (assay ≥99.9% [GC], Fluka GmbH, Buchs, Switzerland), and the homogenates were centrifuged at 15,000 g at 4°C for 20 min. The supernatant was collected for both TP and AC assays. TP was measured using the Folin–Ciocalteu procedure (Singleton and Rossi, 1965). The assay was performed by mixing 0.1 mL methanol extract and 0.5 mL Folin–Ciocalteu reagent (Sigma–Aldrich, Inc., St. Louis, MO, USA). The mixture stood for 3 min before 0.4-ml 7.5% sodium carbonate/water (w/v) was added. Then, the tube content was thoroughly mixed and incubated at 20°C for 30 min. After that, the absorbance at 760 nm was recorded and the result was expressed as milligram gallic acid (Sigma–Aldrich, Inc., St. Louis, MO, USA) per gram of fresh weight (mg gallic acid g−1 FW) based on the standard curve produced by gallic acid.
The antioxidant capacity was assayed using the ferric-reducing antioxidant power (FRAP) method based on the study by Benzie and Strain (1996). The fresh FRAP working solution was prepared daily by mixing 300-mM sodium acetate–acetic acid buffer (pH 3.6), 20-mM FeCl3, and 10-mM 2,4,6tripyridyl-s-stiazine (TPTZ) in a ratio of 10:1:1. For AC assay, 0.95 mL FRAP working solution was mixed with 0.05-ml methanol extract. The mixture was maintained at 20°C for 4 min before absorbance at 593-nm wavelength was read. The AC values were calculated from a standard curve produced by the fresh ammonium ferrous sulfate and expressed as micromole ferrous ion per gram of fresh weight (μmol Fe2+ g−1 FW).
2.2.5 Fresh weight loss
Fresh weight loss was assayed by monitoring the sample’s weight daily from the start to the end of storage and expressed as a percent of fresh weight (%).
2.3 Statistical analysis
All data were submitted to the analysis of variance (ANOVA) with repeated measurements using a Mixed Model to analyze the trends over time using the Statistical Package for Social Science (SPSS version 16.0, SPSS, Inc., Chicago, IL, USA). When ANOVA was significant, the culture systems, washing practices, packaging methods, and storage temperature effects were tested using the F-test, and the interaction effect between factors was tested using Tukey’s multiple range test.
3 Results and discussion
3.1 Pigments
Chlorophyll a (Chl a), chlorophyll b (Chl b), total chlorophyll (total Chl), and carotenoids were significantly influenced by the interaction between the culture system and washing practice with p-values of 0.042, 0.033, 0.034, and 0.003, respectively (Table 1). The highest pigment content was in unwashed TCS-grown corn salad, and the lowest in washed SCS-grown samples. TCS produces corn salad leaves with higher pigment content than SCS (Figure 1). The washing practice accelerated the pigment decay, which was more significant in production from TCS than from SCS. In washed TCS-grown corn salad, Chl a, Chl b, total Chl, and carotenoids content decreased by 13.9, 9.1, 12.6, and 11.0% compared to unwashed samples, while these parameters only reduced by 5.4, 3.1, 4.9, and 4.4% in washed SCS-grown plants, respectively (Table 1).
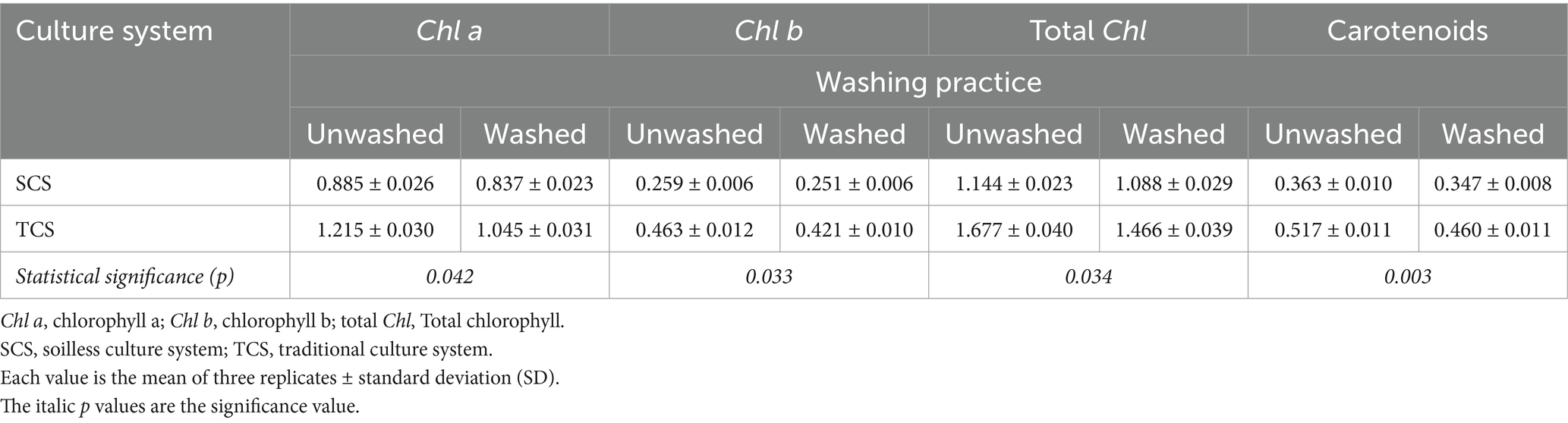
Table 1. Interaction between culture system and washing practice on the content (mg g−1 FW) of Chl a, Chl b, total Chl, and carotenoids in minimally processed corn salad at both 4°C and 8°C.
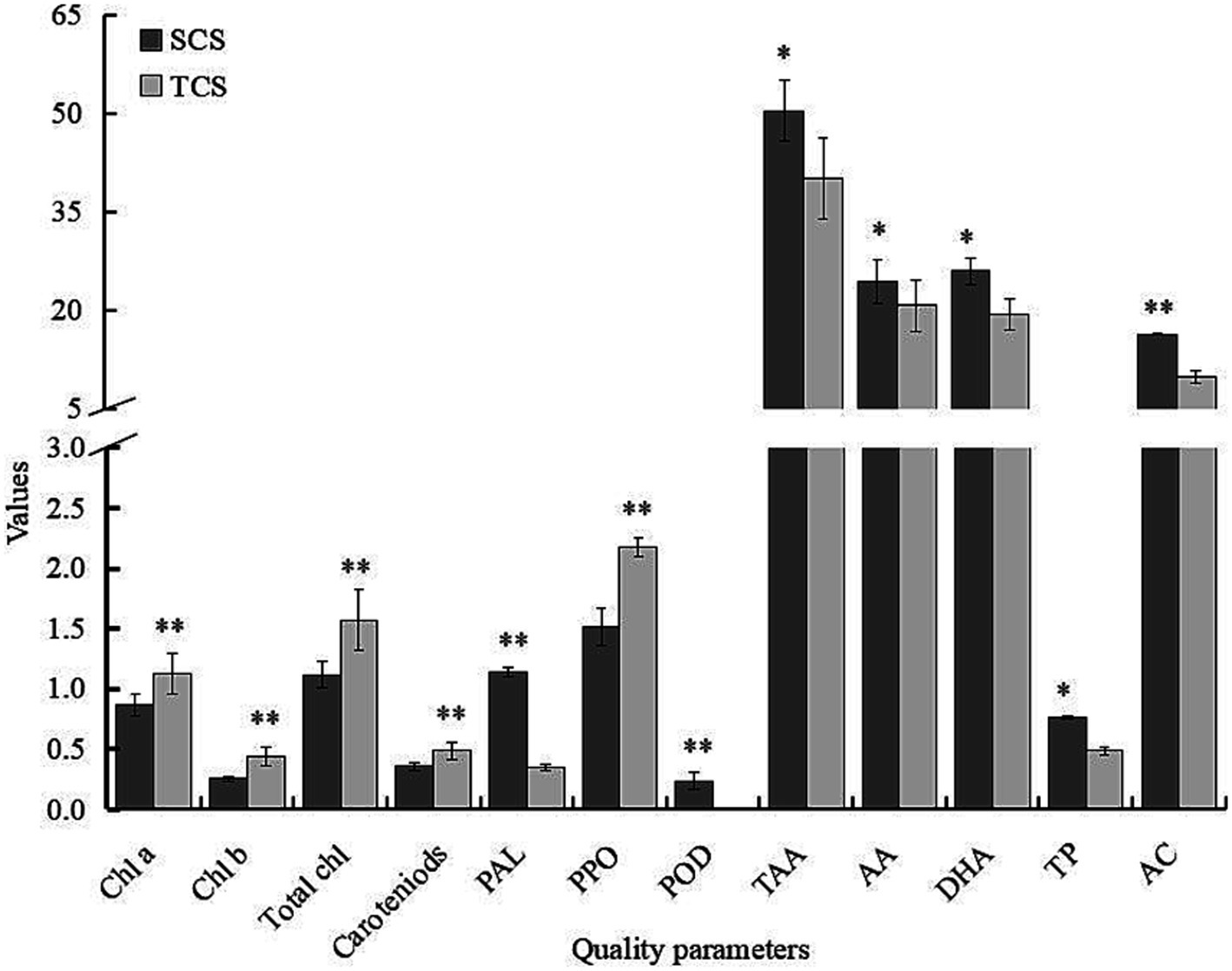
Figure 1. The effect of culture systems on quality parameters of corn salad at harvest. SCS, soilless culture system; TCS, traditional culture system; Chl a, chlorophyll a; Chl b, chlorophyll b; total Chl, Total chlorophyll (mg g−1 FW); PAL, phenylalanine ammonia-lyase (μmol cinnamic acid g−1 FW h−1); PPO, polyphenol oxidase (U g−1 FW); POD, peroxidase (∆Aλ min−1 g−1 FW); TAA, total ascorbic acid; AA, ascorbic acid; DHA, dehydroascorbic acid (mg 100 g−1 FW); TP, total phenolics (mg gallic acid g−1 FW); AC, antioxidant capacity (μmol Fe2+ g−1 FW). **Significant at the 0.01 level; *Significant at the 0.05 level.
The interaction between the culture system and the packaging method significantly influenced pigment retention over time (Table 2), with the culture system having a more significant effect than the packaging method. The highest pigment retention was found in TCS samples packaged with FPB, while the lowest was observed in SCS samples packaged with FPB. In SCS samples, pigments decreased significantly over time regardless of packaging methods: with respect to the initial value at harvest (d0), FPT resulted in a reduction of 28.19% in total Chl and 29.08% in carotenoids, respectively, at the end of storage; and FPB resulted in a decrease of 32.44% in total Chl and 32.55% in carotenoids, respectively. FPT was more effective in preserving pigment content than FPB by the end of storage. However, in TCS samples, pigment changes were inconsistent across packaging methods: FPB caused a slight increase in pigment content, with a rise of 8.17% in total Chl and 10.15% in carotenoid content, respectively. Conversely, FPT led to a decrease of 8.98% in total Chl and 6.77% in carotenoids, respectively, after 7 days of storage. Consequently, the samples from TCS packaged with FPB contained more total Chl (1.641 vs. 1.392 mg g−1 FW) and carotenoid (0.521 vs. 0.443 mg g−1 FW) content than those packaged with FPT at the end of storage.
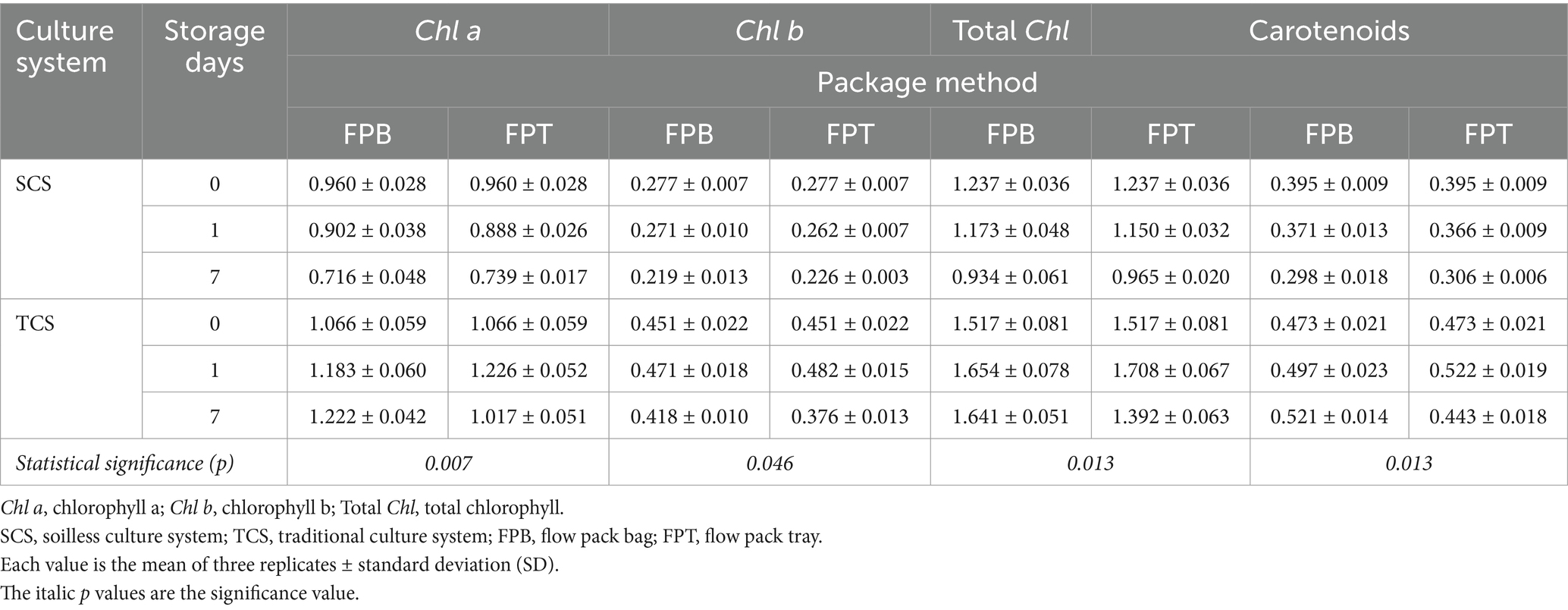
Table 2. Interaction between culture system and package method over time on content (mg g−1 FW) of Chl a, Chl b, total Chl, and carotenoids in minimally processed corn salad during 7 days of storage at both 4°C and 8°C.
The green color represented by pigments is a critical sensory quality and determinative parameter for consumers’ selection and purchase of leafy vegetables. The pigments generally tend to reduce after harvest, and the extent of this reduction largely varies depending on species, cultural technology, processing operation, and subsequent storage conditions (Sinkovič et al., 2023). In this study, TCS produced corn salad containing more pigments than SCS, which is in agreement with the results obtained by Siomos et al. (2001), who found higher Chl a, Chl b, and total Chl content in lettuce (Lactuca sativa L.) harvested from soil culture system than those harvested from soilless culture system. This is possibly attributed to the longer growing days of corn salad grown in TCS (90 days) in comparison to plants grown in SCS (81 days), as mature corn salad tends to accumulate more pigments than immature or “young” plants (Sinkovič et al., 2023). Furthermore, the different nutrient supply and growth medium were the primary technical inputs characterizing the two culture systems tested; thus, they could have influenced the synthesis and content of the pigments in corn salad. Washing is a common and critical practice. During fresh-cut product processing soil, pesticide residues, and debris are removed to reduce microbial load (Kenny and O’Beirne, 2009). This practice and the subsequent drying operation are proven to bring mechanical damage to plant tissues. As expected, our data showed that the washing practice accelerated pigment deterioration of corn salad from TCS and SCS. However, the extent of this deterioration was lower (Chl a, 5.4% vs. 13.9%; Chl b, 3.1% vs. 9.1%; total Chl, 4.9% vs. 12.6%; and carotenoids, 4.4% vs. 11.0%) in SCS-grown corn salad than TCS-grown corn salad (Table 1), implying SCS-grown salad had higher ability to withstand the washing practice in term of pigments degradation. Apart from leaching into washing water from cutting wound juice, the pigment reduction was mainly due to the oxidative degradation caused by mechanical damage brought by washing and the subsequent drying phase, which exposed the leaves tissue to ambient air. It is supposed that the smaller size in leaves and canopies from SCS than those from TCS (3.1 vs. 4.1 g/fresh plant) was beneficial to buffer the impact force of washing water and centrifugal basket extrusion pressure, thus reducing potential wounds and alleviating the deterioration of the pigments. However, due to the lack of comparable literature, further investigation is needed to determine the degree of leaf injuries.
Commercially, salad leaves are marketed in flow pack bags or plastic trays wrapped with flow pack film to prevent wilting (Rux et al., 2017). Placing a tray inside the bag as a support not only facilitates consumer selection and handling of the product but also protects it from crushing injuries during transporting, handling, and marketing. In this study, we found that the packaging method considerably impacted pigment preservation; however, this impact was highly dependent on the culture system. For SCS-grown corn salad, the FPT packaging method showed more beneficial pigment preservation than FPB. In contrast, for TCS-grown corn salad, the FPB packing method was more effective than FPT in preserving the pigments. This contrasting result was possibly explained by the fact that the SCS-grown leaves were “young” (81 days of growth period) and hydrated and tender; the tray acted as a protective barrier around the leaves, reducing crushing injuries. While TCS-grown leaves were mature and “old” (90 days of growth period), the protective effect of FPT was less pronounced. In addition, it is important to note that in the current study, all samples were packaged and placed on chambers’ shelves, simulating basic commercial market conditions, without further distribution, delivery, or display. This reduced the risk of compression and collision injuries, which in turn diminished the protective effect of the FPT packaging method.
3.2 Enzymes
The PAL was significantly influenced by the interaction between the culture system and washing practice over time (Table 3). The highest increase in PAL activity occurred in the washed SCS-grown corn salad, with a 30% increase from 0.971 μmol cinnamic acid g−1 FW h−1 at harvest to 1.262 μmol cinnamic acid g−1 FW h−1 at the end of shelf life. PAL activity in corn salad from SCS (1.131 μmol cinnamic acid g−1 FW h−1) was 3.8 times higher than that in leaves from TCS (0.296 μmol cinnamic acid g−1 FW h−1) at harvest (Figure 1), and it maintained significantly higher during the whole storage. The washing effect on PAL activity was observed on processing day (day 0), with notable differences between washed and unwashed samples. However, during the shelf life, these differences leveled off and diminished regardless of the culture system. The PAL activity was significantly influenced by the interaction between washing practice, packaging method, and storage temperature over time (Supplementary Table S1). While no clear conclusion could be drawn from this interaction due to a very high standard deviation (SD), this result must be clarified through further studies. When comparing data based only on the washing practice factor, the results showed a tendency for the washing practice to reduce PAL activity (−4.83%) on the processing day, regardless of treatments, and to induce higher PAL activity (+17.43%) in the washed samples compared to the unwashed samples (+10%) at the end of the shelf life. However, these differences were not statistically significant (Supplementary Table S2). This could be explained by the possibility that the washing practice removed the enzyme by removing the cell fluid on the processing day. At the same time, the washing practice and subsequent drying operations inevitably caused mechanical wounds, inducing the PAL activity to increase during the shelf life, as PAL is a wound-induced enzyme (López-Gálvez et al., 1996).
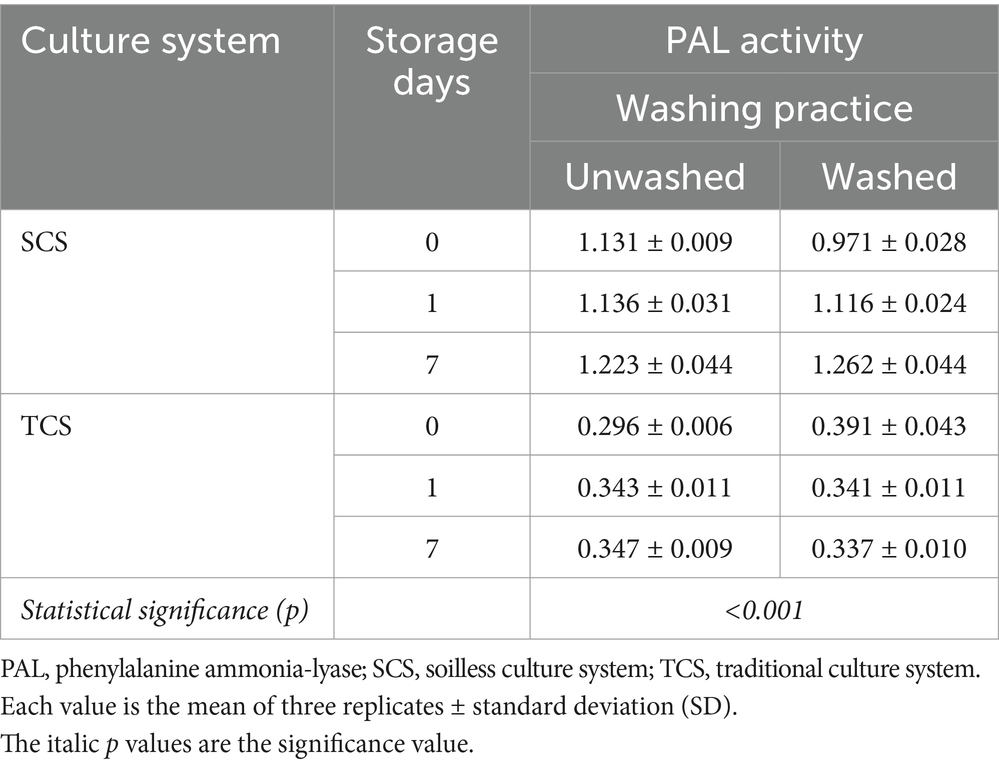
Table 3. Interaction between culture system and washing practice over time on PAL activity (μmol cinnamic acid g−1 FW h−1) in minimally processed corn salad during 7 days of storage at both 4°C and 8°C.
The PPO was significantly influenced by the interaction between the culture system, washing practice, and packaging method over time (Table 4). The highest PPO activity was observed at the end of the shelf life in washed samples from SCS-grown leaves packaged in FPT. The largest increase in PPO activity was observed in the SCS-grown leaves, unwashed, and packaged with FPB, with a nearly 4-fold increase compared to the initial value (Table 4). The PPO increased over time without reaching the peak in all samples. In the SCS-grown leaves, the washing practice increased the PPO activity (3.333 vs. 1.511 U g−1 FW) on the processing day; thereafter, the differences in PPO activity between unwashed and washed samples leveled off during the shelf life. No clear interaction with the package method was observed. However, no significant differences were observed between treatments in the TCS-grown plants. The PPO activity in all samples increased more than 2-fold (2.33–2.67 folds) at the end of the shelf life compared to the initial value. The enzyme activity of POD was significantly influenced by the interaction between the culture system and washing practice (p = 0.002) (Supplementary Table S3), as well as the culture system over time (p < 0.001) (Supplementary Table S4). The POD enzyme activity was 0.273 ∆Aλ min−1 g−1 FW in SCS samples but was not detected in TCS samples. The washing reduced POD activity by 30% in SCS samples (Supplementary Table S3). At the end of the shelf life, POD activity in SCS samples was more than 2-fold compared to the initial value (Supplementary Table S4).
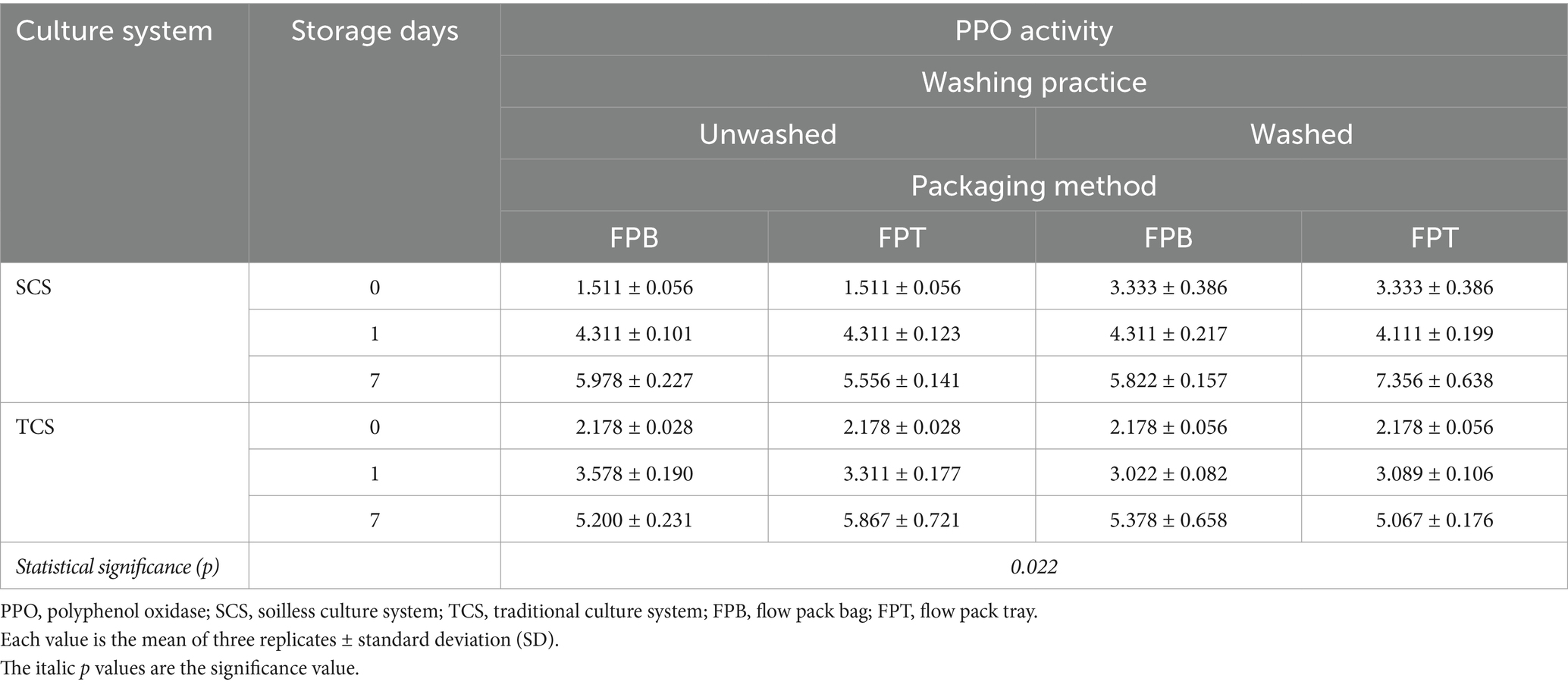
Table 4. Interaction between culture system, washing practice, and packaging method over time on PPO activity (U g−1 FW) in minimally processed corn salad during 7 days of storage at 4°C and 8°C.
PPO is the key enzyme responsible for plant-based food browning development. It can directly catalyze the oxidation of polyphenols into o-quinones in the presence of oxygen, which subsequently polymerize into browning or/ and black pigments, known as tissue browning (Degl’Innocenti et al., 2007). POD is normally considered an antioxidant enzyme for scavenging free radicals in plants under stress conditions. POD catalyzes the conversion of hydrogen peroxide to water using polyphenols as hydrogen donors, which produces red browning substances (Lee et al., 2021). Consequently, POD is often considered to cooperate with PPO to trigger food quality decay in polyphenol oxidation and browning color development (Shao and Zhang, 2015). Therefore, both enzymes have been evaluated to monitor the quality deterioration of fresh products during postharvest processing and preservation (Jang and Moon, 2011). In this study, PPO and POD displayed irregular behaviors when different treatments were applied. Overall, in comparison to TCS, SCS produces corn salad with lower PPO (1.511 vs. 2.178 U g−1 FW) but higher POD (0.232 ∆Aλ min−1 g−1 FW vs. not detected) at harvest (Figure 1). Such low PPO activity might be associated with higher AA content in leaves from SCS at harvest (Figure 1), as AA possesses strong inhibitory activity against PPO by forming an AA-PPO complex (Li et al., 2022). In addition, the plant maturity of the harvest also affects the PPO activity. Korus (2011) demonstrated that the PPO activity in three varieties of kale increased with the degree of plant maturity: the PPO activity was 19% higher at the 2nd stage (14 weeks growth) and 75% higher in the 3rd stage (18 weeks growth) in comparison to the 1st stage (10 weeks growth). In our study, the lower PPO activity observed in SCS-grown leaves could be attributed to their lower maturity (81 days of growth) than TCS-grown leaves (90 days of growth). The washing practice induced the PPO activity in SCS samples on the processing day (Table 4). A reasonable explanation could be that the washing and subsequent drying operations mechanically destroy the cell integrity of tissue, provoking cell lysis, which is highly related to the increase in PPO enzyme activity (Nogales-Delgado, 2021). During the postharvest phase, the largest increase (3.95-fold) in PPO activity was observed in unwashed SCS samples packaged with FPB (Table 4). Such an increase is likely the result of a synergistic effect of the factors tested. Washing is typically used to remove surface soil, microorganisms, and other contaminants. Unwashed samples may harbor a higher microbial population, which could activate PPO activity, as microorganisms can damage tissue integrity and increase enzyme activity (Nogales-Delgado, 2021). Additionally, modified atmosphere packaging with a high CO2 concentration has been shown to inhibit PPO activity (Galvis-Sánchez et al., 2006; Nogales-Delgado, 2021). Based on these facts, we speculated that the unwashed samples contained more microbial matter due to the absence of the washing procedure, which may activate the PPO activity. Furthermore, FPB packaging might have resulted in a lower CO2 concentration due to the larger bag space, which dilutes the headspace gas concentration compared to the smaller size of the tray used in FPT packaging (Supplementary Figure S1). Therefore, the conditions in the unwashed samples packaged with FPB likely favored PPO activation. POD, an antioxidant enzyme, plays an important role in protecting plant cells from oxidative damage caused by reactive oxygen species (ROS)—often produced under stress conditions. The activation of POD helps to mitigate this damage. As described in the “Materials and Methods” section, the SCS system consists of floating the plants in a nutrient solution; the lower temperature and/or greater temperature fluctuation of the nutrient solution in the winter would be an abiotic factor to stress the plant growth. In this context, the higher POD activity in SCS samples could be interpreted as a defensive mechanism triggered by the plant in response to this abiotic stress. In addition, the SCS system allows plant roots to be continuously immersed in a water solution, creating a hypoxic condition in the root zone. This low oxygen condition is another abiotic stress factor the plants can encounter. In response, the plant antioxidant system, including antioxidant enzymes such as POD, is activated and induced to act as a protective antioxidant to improve the plant’s tolerance to stress conditions by scavenging the oxygen-free radical (Liang et al., 2017; Pan et al., 2021). In tomato production, the growing systems of open fields and net houses had a remarkable impact on PPO and POD activity. However, this impact is highly genotype-specific (Lee et al., 2021), illustrating that both cultural systems and species gene background considerably impact PPO and POD activity. Unfortunately, studies on the culture system and postharvest factors affecting PPO and POD activity of leafy greens are very limited; thus, there is no equivalent data to compare. Therefore, further trial is imperative to clarify these enzyme behaviors in different corn salad varieties or other leafy green species before concluding. In addition, the activities in both PPO and POD showed a considerable increase over time regardless of the treatments. However, no browning symptoms were observed for all leaves at the end of storage, indicating corn salad is non-susceptible to tissue browning and still marketable after 7 days of storage in cold conditions.
3.3 Total ascorbic acid, ascorbic acid, and dehydroascorbic acid
The culture system significantly influenced the content of the overall total ascorbic acid (TAA), ascorbic acid (AA), and dehydroascorbic acid (DHA). In contrast to pigments, the SCS system allowed to produce corn salad leaves with a higher content of 25.6% TAA (50.47 vs. 40.19 mg 100 g−1 FW), 17.5% AA (24.47 vs. 20.84 mg 100 g−1 FW), and 34.4% DHA (26 vs. 19.36 mg 100 g−1 FW) than TCS system at harvest (Figure 1). These data were consistent with the results reported by Frezza et al. (2005), who found that the AA content in lettuce from the floating system was significantly higher (+72%) than that from greenhouse soil. Furthermore, the plant growth stage at harvest is a major factor determining the compositional quality of leafy vegetables (Skrypnik et al., 2021). The same authors found that lamb lettuce harvested at the short growth stage (52 days after sowing) contained significantly higher levels of AA content than those harvested at the longer growth stage (66 days after harvest sowing). Similarly, the spinach baby leaves exhibited higher AA and TAA when harvested at a younger stage (17–18 days after sowing) than those harvested at an older stage (23–24 days and 29–30 days) (Bergquist et al., 2006). In our study, corn salad from SCS had a shorter growth period (81 days) than that from TCS (90 days) at harvest; this reduced plant growth stage could be responsible for the higher TAA and AA content observed in SCS-grown leaves. As regards the variations of these parameters over time, TAA was influenced only by storage time, with 27.3% at the end of shelf life regardless of treatments (data not shown). DHA was significantly influenced by the interaction between cultural systems and packaging methods over time. In SCS-grown corn salad, DHA significantly increased over time, and this change was more gradual and less with FPT than with the FPB package. In TCS-grown corn salad, no differences resulted between the packaging methods, and at the end of shelf life the DHA increase values were similar (Table 5).
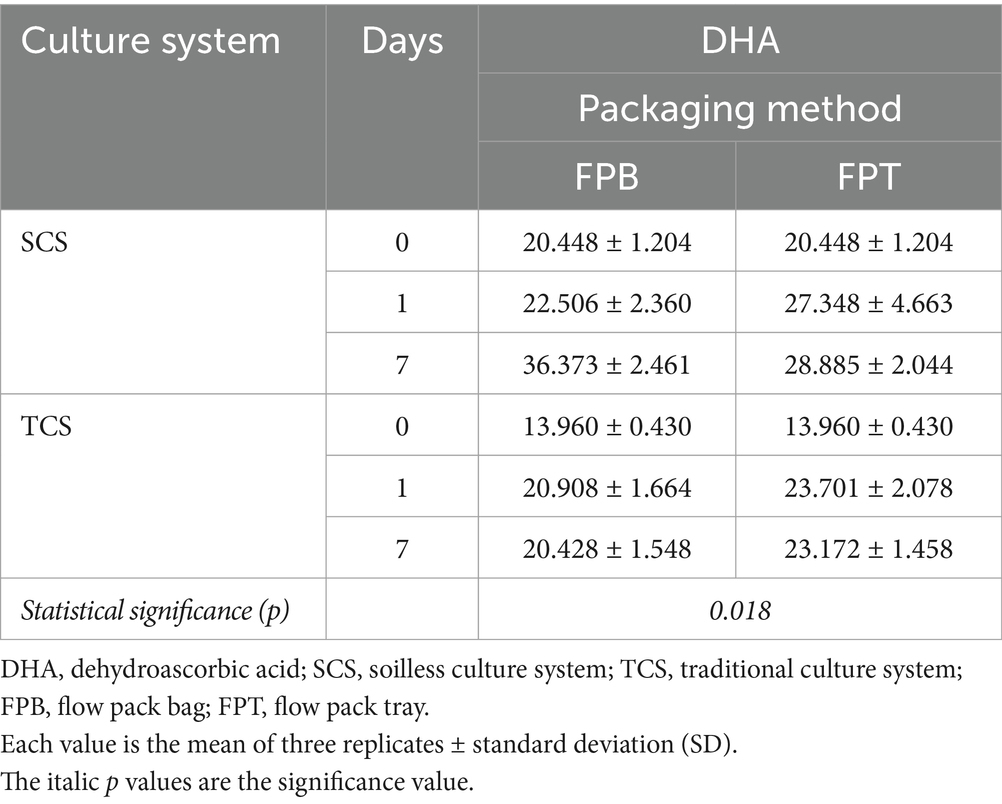
Table 5. Interaction between culture system and packaging method over time on dehydro-ascorbic acid (DHA) content (mg 100 g−1 FW) in minimally processed corn salad during 7 days of storage at 4°C and 8°C.
As known, AA, the reduced form of vitamin C, is prone to deterioration and oxidizes into DHA, the first oxidation product of AA, in the presence of molecular oxygen (Lee and Kader, 2000). The opposite direction of this reaction, which would regenerate AA, is not thermodynamically the most favored (Degl’Innocenti et al., 2007). Thus, AA tends to decrease, and meanwhile, DHA increases during postharvest storage of fresh fruit and vegetables. In our study, less DHA generation (28.89 vs. 36.37 mg 100 g−1 FW) was found in the SCS-grown produce packaged with FPT than FPB, illustrating less AA deterioration occurred in FPT. However, no significant change in AA content was observed. This could be attributed to the tray in PBT, which acted as a protective holder and oxygen barrier, reducing mechanical injuries and limiting oxygen availability, thereby preventing the oxidation of AA into DHA. Additionally, as shown in Supplementary Figure S1, compared to the larger size of the bag in FPB, the smaller size of the tray in FPT allowed for the creation of faster and intensive modified atmosphere conditions, characterized by high CO2 and low O2 levels resulting from leaf respiration. This low O2 content in the FPT alleviated the oxidation of AA to DHA. However, FPT showed no impact on DHA generation for leaves from TCS, which was possibly associated with the reduced movements and collisions of products during the whole storage period. In our study, although the materials were processed and stored to simulate commercial conditions, the absence of shipping, distribution, and marketing processes reduced the potential for friction injuries, possibly concealing the protective function of packaging accessories such as trays. However, in a real supply chain, the processors generally are not sellers, so the packaged produce has to be distributed to different retailers after packaging, in some cases via wholesales, during which the produce is supposed to suffer from injuries of compression or collision. Storage at low temperatures is highly recommended for leafy vegetable to preserve their visual quality and nutritional content. Storage of spinach baby leaves at 2°C reduced AA content less than storage at 10°C (Bergquist et al., 2006). In our study, unexpectedly, the storage temperature had no significant effect on AA. This may be attributed to the small temperature difference between the tested temperature (8°C vs. 4°C). Usually, the degradation rate of unprotected AA doubles with every 10°C increase in temperature (Pavlovska and Tanevska, 2013). Furthermore, unlike in the commercial supply chain, the absence of temperature fluctuation during the entire storage period was favorable for AA preservation. Therefore, further studies should focus on the complete commercial conditions simulation to clear this issue.
3.4 Total phenols and antioxidant capacity
Culture systems only affected TP content, of which SCS-grown corn salad had more than 57.1% TP than that grown in TCS at harvest (0.77 vs. 0.49-mg gallic acid g−1 FW) (Figure 1). TPs are a large group of plant secondary metabolites that play an important role in plant-derived food quality, representing quality characteristics and health-promoting properties (Hernández et al., 2021). In plant tissue, TP biosynthesis originates from trans-cinnamic acid that is formed via PAL catalyzing the non-oxidative deamination of L-phenylalanine; TP oxidative degradation via PPO that catalyzes/oxidizes TP to quinones in the presence of molecular oxygen (Tomás-Barberán and Espín, 2001). Thus, the actual assayed concentrations of TPs in plant tissue depend on the balance between the TP synthesis rate and their oxidation rate (Zhan et al., 2020). In this study, the differences in TP concentration from SCS and TCS followed this pattern, which was consistent with the trend of their respective synthetic and oxidative enzyme activity. That is, in comparison to TCS-grown leaves, SCS-grown corn salad had significantly higher PAL activity (1.14 vs. 0.34 μmol cinnamic acid g−1 FW h−1) and lower PPO activity (1.51 vs. 2.18 Ug−1 FW) (Figure 1). this implied that the TP biosynthesis rate was faster while its oxidization rate was slower, consequently leading to more accumulation of de novo TP. Actually, a positive correlation (R = 0.785, p < 0.001) between PAL and TP (Figure 2) further confirmed PAL’s critical responsibility in TP accumulation. Furthermore, the higher AA level in SCS-grown leaves might be partly responsible for the higher level of TP because AA could not only directly reduce quinones back to phenols but also inhibit PPO action and limit the oxygen availability, thus indirectly retard TP oxidation (Degl’Innocenti et al., 2007; Li et al., 2022). This explanation was supported by the positive relationship between AA and TP (R = 0.382, p < 0.001) (Figure 2). Similar trends were also reported by Reyes et al. (2006), who found that vegetables (e.g., white and red cabbage) with higher AA levels had a greater soluble phenols content. Conversely, vegetables (e.g., lettuce and celery) with lower AA levels had a lower soluble phenols content.
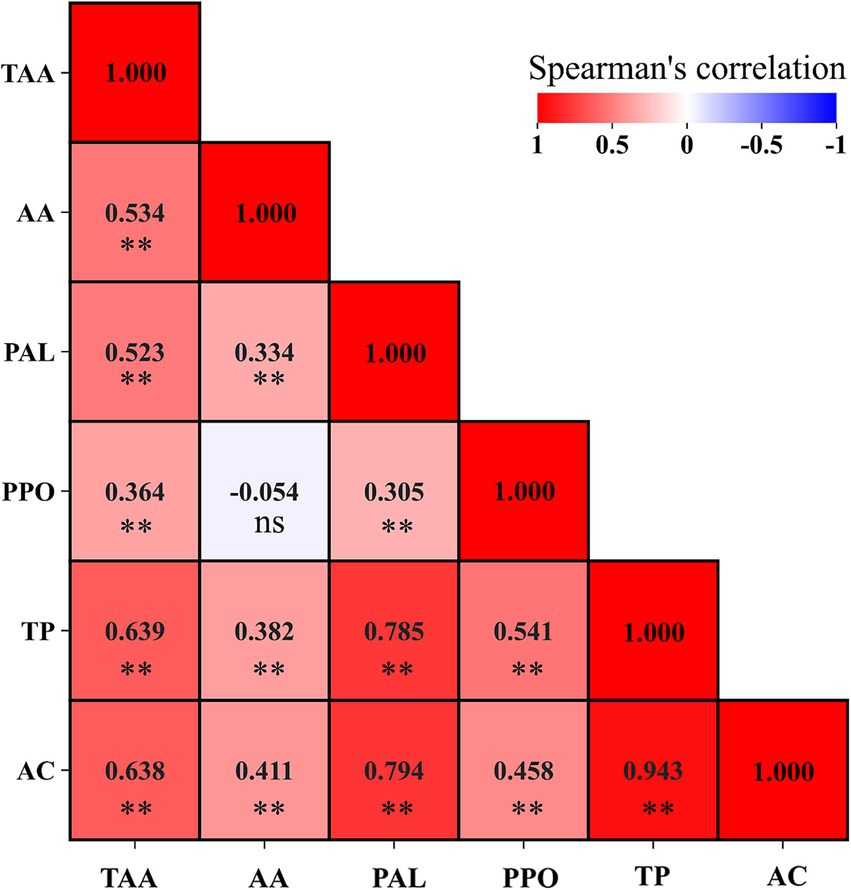
Figure 2. The Spearman’s correlations analysis among select variables. TAA, total ascorbic acid; AA, ascorbic acid; PAL, phenylalanine ammonia-lyase; PPO, polyphenol oxidase; TP, total phenolics; AC, antioxidant capacity. The total number of 144 replicates for each parameter. The correlation coefficients are proportional to the color intensity. Positive correlation is displayed in red and negative in blue color. **The correlation is significant at the 0.01 level (two-tailed). ns, not significant.
The AC was significantly influenced by both cultural systems over time (p < 0.001) and washing practices over time (p = 0.039) (Table 6). AC values were higher (+67%) in leaves from SCS than in TCS at harvest, and they increased by 66.1% at the end of shelf life without reaching the peak. Comparatively, in leaves from TCS, AC values were low at harvest, increased to a peak on the first day of storage (d1), and then decreased thereafter (Table 6). The AC in leafy vegetables is mainly derived from phytochemicals, including TP compounds, vitamins, and flavonoids (Rico et al., 2007). The present study also showed that SCS produced corn salad with significantly higher AC values than TCS at harvest and shelf life. Such a high level of AC was attributed to its high level of TAA, AA, and TP content, as the extremely significant correlations between AC and these bioactive substances were established regardless of treatments (Figure 2), indicating TAA, AA, DHA, and TP were the main contributors to AC value in corn salad. These findings were in agreement with those of Martínez-Sánchez et al. (2008), who reported the strong correlations between AC values and content of TP and vitamin C in baby leaves of Brassicaceae species, such as wild rocket and salad rocket, whose baby leaves are suggested to be good dietary sources of antioxidants with a variety of important bioactive compounds. Washing operation significantly increased AC values (+16.5%) only at processing day (d0). During the subsequent storage days, in both washed and unwashed samples, AC increased with similar dimensions and reached the same level (15.2 vs. 15.8 μmol Fe2+ g−1 FW) at the end of the shelf life (Table 6). This transient increase in AC values after washing could have been elicited by the mechanical wounds brought by the washing and consequent drying practices because wound, as an abiotic factor, could induce the increase in AC values of fresh-cut produce such as lettuce (Kang and Saltveit, 2002) and lotus root slices (Hu et al., 2014). However, inconsistent data was also reported by Kenny and O’Beirne (2009), who found no significant effect of washing practices (tap rinsed, water-dipped, chlorine dipped) on AC of ready-to-use iceberg lettuce during 8 days of storage.
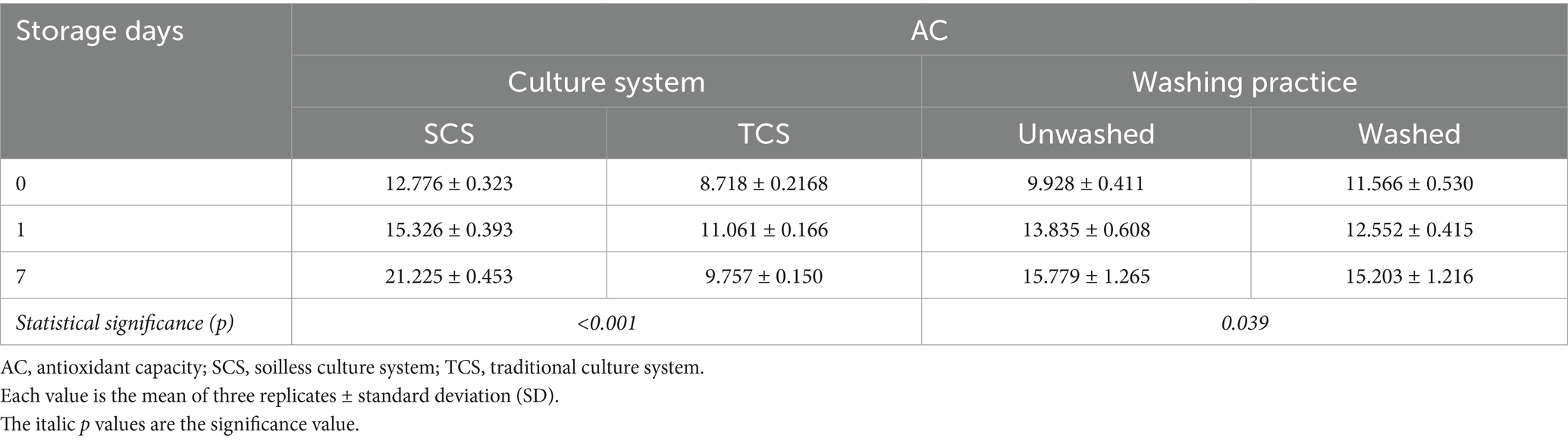
Table 6. The effect of culture system over time, washing practice over time on AC (μmol Fe2+ g−1 FW) in minimally processed corn salad during 7 days of storage at 4°C and 8°C.
3.5 Fresh weight loss
The minimally processed corn salad fresh weight loss was significantly influenced by the culture system (p < 0.001), package method (p = 0.003), and storage temperature (p < 0.001), respectively (Supplementary Tables S5–S7). However, despite these significant effects, the overall fresh weight loss was minimal in all samples. The highest fresh weight loss observed in TCS-grown leaves was only 0.38% (Supplementary Table S5), far below the 4–5% threshold that renders the leafy vegetable texture unacceptable (Nunes and Emond, 2007). Thus, all corn salad leaves were fresh and marketable after 7 days of storage. This result was in agreement with our previous finding, where corn salad grown in SCS lasted 8 days shelf life in the cool chamber at 4°C (Fontana and Nicola, 2009). Similar results were also reported in our recent studies of other leafy baby leaves, such as lettuce (Pignata et al., 2020), spinach (Zhan et al., 2020), and lettuce and rocket salad (Zhan et al., 2022), all of which were usually turgid and fresh after 7 days of storage at cool condition.
4 Conclusion
This study demonstrated that SCS grew corn salad with a higher concentration of phytochemicals presented by TAA, AA, and TP, thereby more AC than TCS. The TAA, AA, DHA, and TP were the main contributors to the AC of corn salad, as the highly positive correlations between them were established. The pigment content in SCS-grown leaves was less than in TCS-grown leaves but showed an enhanced ability to withstand washing operations. Washing practice using only tap water deteriorated pigments. Still, it induced a transient increase in AC values significantly higher in SCS-grown leaves than in TCS during the whole storage time. However, the packaging methods showed a considerable impact on pigment retention and DHA generation. However, this feature was highly associated with the culture system. Storage temperatures tested had no significant influences on the overall quality of minimally processed corn salad over time, suggesting that minimally-processed corn salad could be stored for 7 days at 8°C with acceptable qualitative characteristics. The narrow temperature range/interval and the absence of temperature abuse during the whole storage might conceal the potential effects between different storage temperatures, which need further clarification in future studies. The activities in enzymes of PAL, PPO, and POD, which are related to phenolic metabolism and tissue browning development, were affected by factors tested over time. However, no browning symptoms or subsequent visual quality decay were observed during the whole storage. After 7 days of storage, minimally processed corn salad was still fresh and marketable, with the most significant fresh weight loss of 0.38%. This study uniquely evaluated the interaction of multiple factors within the fresh-cut produce supply chain, including raw material production, processing practices (washing and packaging), and postharvest storage conditions. The results offer practical insight for improving preharvest nutritional quality and maintaining postharvest antioxidant characteristics of fresh-cut leafy vegetables. Moreover, the findings of this study highlighted the synergistic benefit of preharvest and postharvest factors in improving fresh-cut product quality.
Data availability statement
The original contributions presented in the study are included in the article/Supplementary material, further inquiries can be directed to the corresponding author.
Author contributions
LZ: Data curation, Formal analysis, Funding acquisition, Methodology, Writing – original draft, Writing – review & editing. YF: Data curation, Methodology, Software, Writing – original draft. EF: Data curation, Methodology, Software, Writing – review & editing. GT: Methodology, Software, Writing – review & editing. SN: Conceptualization, Funding acquisition, Project administration, Resources, Software, Supervision, Validation, Visualization, Writing – review & editing, Investigation.
Funding
The author(s) declare that financial support was received for the research, authorship, and/or publication of this article. This work partially was supported by the National Natural Science Foundation of China (NNSFC) Grant number: 31972135.
Conflict of interest
The authors declare that the research was conducted in the absence of any commercial or financial relationships that could be construed as a potential conflict of interest.
The author(s) declared that they were an editorial board member of Frontiers, at the time of submission. This had no impact on the peer review process and the final decision.
Publisher’s note
All claims expressed in this article are solely those of the authors and do not necessarily represent those of their affiliated organizations, or those of the publisher, the editors and the reviewers. Any product that may be evaluated in this article, or claim that may be made by its manufacturer, is not guaranteed or endorsed by the publisher.
Supplementary material
The Supplementary material for this article can be found online at: https://www.frontiersin.org/articles/10.3389/fsufs.2024.1468986/full#supplementary-material
References
Ariffin, S. H., Gkatzionis, K., and Bakalis, S. (2017). Leaf injury and its effect towards shelf-life and quality of ready-to-eat (RTE) spinach. Energy Procedia 123, 105–112. doi: 10.1016/j.egypro.2017.07.265
Benzie, I. F., and Strain, J. J. (1996). The ferric reducing ability of plasma (FRAP) as a measure of “antioxidant power”: the FRAP assay. Anal. Biochem. 239, 70–76. doi: 10.1006/abio.1996.0292
Bergquist, S. Å., Gertsson, U. E., and Olsson, M. E. (2006). Influence of growth stage and postharvest storage on ascorbic acid and carotenoid content and visual quality of baby spinach (Spinacia oleracea L.). J. Sci. Food Agr. 86, 346–355. doi: 10.1002/jsfa.2373
Brenes, A. L. M., Brown, W., Steinmaus, S., Brecht, J. K., Xie, Y., Bornhorst, E. R., et al. (2020). Temperature profiling of open- and closed doored produce cases in retail grocery stores. Food Control 113:107158. doi: 10.1016/j.foodcont.2020.107158
Degl’Innocenti, E., Pardossi, A., Tognoni, F., and Guidi, L. (2007). Physiological basis of sensitivity to enzymatic browning in ‘lettuce’, ‘escarole’ and ‘rocket salad’ when stored as fresh-cut products. Food Chem. 104, 209–215. doi: 10.1016/j.foodchem.2006.11.026
Fontana, E., and Nicola, S. (2009). Traditional and soilless culture systems to produce corn salad (Valerianella olitoria L.) and rocket (Eruca sativa mill.) with low nitrate content. J. Food Agric. Environ. 92, 1827–1410. doi: 10.3168/jds.2009-92-4-1827
Frezza, D., León, A., Logegaray, V., Chiesa, A., Desimone, M., and Diaz, L. (2005). Soilless culture technology for high quality lettuce. Acta Hortic. 697, 43–48. doi: 10.17660/ActaHortic.2005.697.3
Galvis-Sánchez, A. C., Fonseca, S. C., Gil-Izquierdo, A., Gil, M. I., and Malcata, F. X. (2006). Effect of different levels of CO2 on the antioxidant content and the polyphenol oxidase activity of ‘Rocha’ pears during cold storage. J. Sci. Food Agric. 86, 509–517. doi: 10.1002/jsfa.2359
Hernández, V., Botella, M. Á., Hellín, P., Cava, J., Flores, P., Mestre, T., et al. (2021). Phenolic and carotenoid profile of lamb’s lettuce and improvement of the bioactive content by preharvest conditions. Food Secur. 10:188. doi: 10.3390/foods10010188
Hu, J., Yang, L., Wu, W., Li, Y., and Zhan, L. (2014). Slicing increases antioxidant capacity of fresh-cut lotus root (Nelumbo nucifera G.) slices by accumulating total phenols. Int. J. Food Sci. Technol. 49, 2418–2424. doi: 10.1111/ijfs.12563
Jacxsens, L., Devlieghere, F., and Debevere, J. (2002). Temperature dependence of shelf-life as affected by microbial proliferation and sensory quality of equilibrium modified atmosphere packaged fresh produce. Postharvest Biol. Technol. 26, 59–73. doi: 10.1016/S0925-5214(02)00004-2
Jang, J. H., and Moon, K. D. (2011). Inhibition of polyphenol oxidase and peroxidase activities on fresh-cut apple by simultaneous treatment of ultrasound and ascorbic acid. Food Chem. 124, 444–449. doi: 10.1016/j.foodchem.2010.06.052
Jasper, J., Elmore, J. S., and Wagstaff, C. (2021). Determining the quality of leafy salads: past, present and future. Postharvest Biol. Technol. 180:111630. doi: 10.1016/j.postharvbio.2021.111630
Kampfenkel, K., Montagu, M. V., and Inze, D. (1995). Extraction and determination of ascorbic and dehydroascorbate from plant tissue. Anal. Biochem. 225, 165–167. doi: 10.1006/abio.1995.1127
Kang, H. M., and Saltveit, M. E. (2002). Antioxidant capacity of lettuce leaf tissue increases after wounding. J. Agric. Food Chem. 50, 7536–7541. doi: 10.1021/jf020721c
Kenny, O., and O’Beirne, D. (2009). The effects of washing treatment on antioxidant retention in ready-to-use iceberg lettuce. Int. J. Food Sci. Technol. 44, 1146–1156. doi: 10.1111/j.1365-2621.2009.01935.x
Korus, A. (2011). Level of vitamin c, polyphenols, and antioxidant and enzymatic activity in three varieties of kale (Brassica Oleracea L. Var. Acephala) at different stages of maturity. Int. J. Food Prop. 14, 1069–1080. doi: 10.1080/10942910903580926
Lee, S. K., and Kader, A. A. (2000). Preharvest and postharvest factors influencing vitamin C content of horticultural crops. Postharvest Biol. Technol. 20, 207–220. doi: 10.1016/S0925-5214(00)00133-2
Lee, J. H., Kasote, D. M., Jayaprakasha, G. K., Avila, C. A., Crosby, K. M., and Patil, B. S. (2021). Effect of production system and inhibitory potential of aroma volatiles on polyphenol oxidase and peroxidase activity in tomatoes. J. Sci. Food Agric. 101, 307–314. doi: 10.1002/jsfa.10644
Li, C., Li, J., Yan, S., and Wang, Q. (2022). The mechanism of interaction between lotus rhizome polyphenol oxidase and ascorbic acid: inhibitory activity, thermodynamics, and conformation analysis. J. Food Biochem. 46:e14047. doi: 10.1111/jfbc.14047
Liang, X., Chen, Q., Lu, H., Wu, C., Lu, F., and Tang, J. (2017). Increased activities of peroxidase and polyphenol oxidase enhance cassava resistance to Tetranychus urticae. Exp. Appl. Acarol. 71, 195–209. doi: 10.1007/s10493-017-0125-y
Lichtenthaler, H., and Wellburn, A. (1983). Determination of total carotenoids and chlorophyll a and b of leaf in different solvents. Biochem. Soc. Trans. 11, 591–592. doi: 10.1042/bst0110591
López-Gálvez, G., Saltveit, M., and Cantwell, M. (1996). Wound-induced phenylalanine ammonia lyase activity: factors affecting its induction and correlation with the quality of minimally processed lettuces. Postharvest Biol. Technol. 9, 223–233. doi: 10.1016/S0925-5214(96)00050-6
Manzocco, L., Foschia, M., Tomasi, N., Maifreni, M., Dalla Costa, L., Marino, M., et al. (2011). Influence of hydroponic and soil cultivation on quality and shelf life of ready-to-eat lamb's lettuce (Valerianella locusta L. Laterr). J. Sci. Food Agric. 91, 1373–1380. doi: 10.1002/jsfa.4313
Martínez-Sánchez, A., Allende, A., Bennett, R. N., Ferreres, F., and Gil, M. I. (2006). Microbial, nutritional and sensory quality of rocket leaves as affected by different sanitizers. Postharvest Biol. Technol. 42, 86–97. doi: 10.1016/j.postharvbio.2006.05.010
Martínez-Sánchez, A., Allende, A., Cortés-Galera, Y., and Gil, M. I. (2008). Respiration rate response of four baby leaf Brassica species to cutting at harvest and fresh-cut washing. Postharvest Biol. Technol. 47, 382–388. doi: 10.1016/j.postharvbio.2007.07.010
Nicola, S., Pignata, G., Casale, M., Turco, P. E. L., and Gaino, W. (2016). Overview of a lab-scale pilot plant for studying baby leaf vegetables grown in soilless culture. Horticult. J. 85, 97–104. doi: 10.2503/hortj.MI-R01
Nogales-Delgado, S. (2021). Polyphenoloxidase (PPO): effect, current determination and inhibition treatments in fresh-cut produce. Appl. Sci. 11:7813. doi: 10.3390/app11177813
Nunes, C. N., and Emond, J. P. (2007). Relationship between weight loss and visual quality of fruits and vegetables. Proc. Fla. State Hort. Soc. 120, 235–245.
Pan, J., Sharif, R., Xu, X., and Chen, X. (2021). Mechanisms of waterlogging tolerance in plants: research progress and prospects. Front. Plant Sci. 11:627331. doi: 10.3389/fpls.2020.627331
Pavlovska, G., and Tanevska, S. (2013). Influence of temperature and humidity on the degradation process of ascorbic acid in vitamin C chewable tablets. J. Therm. Anal. Calorim. 111, 1971–1977. doi: 10.1007/s10973-011-2151-z
Pignata, G., Ertani, A., Casale, M., Piano, S., and Nicola, S. (2020). Mixing fresh-cut baby green and red leaf lettuce from soilless cultivation preserves phytochemical content and safety. Agric. Food Sci. 29, 55–65. doi: 10.23986/afsci.88904
Reyes, L. F., Villarreal, J. E., and Cisneros-Zevallos, L. (2006). The increase in antioxidant capacity alter wounding depends on the type of fruit or vegetable tissue. Food Chem. 101, 1254–1262. doi: 10.1016/j.foodchem.2006.03.032
Rico, D., Martìn-Diana, A. B., Barat, J. M., and Barry-Ryana, C. (2007). Extending and measuring the quality of fresh-cut fruit and vegetables: a review. Trends Food Sci. Technol. 18, 373–386. doi: 10.1016/j.tifs.2007.03.011
Rux, G., Caleb, O. J., Geyer, M., and Mahajan, P. V. (2017). Impact of water rinsing and perforation-mediated MAP on the quality and off-odour development for rucola. Food Packag. Shelf Life 11, 21–30. doi: 10.1016/j.fpsl.2016.11.003
Schmitzer, V., Senica, M., Slatnar, A., Stampar, F., and Jakopic, J. (2021). Changes in metabolite patterns during refrigerated storage of lamb's lettuce (Valerianella locusta L. Betcke). Front. Nutr. 8:731869. doi: 10.3389/fnut.2021.731869
Shao, X., and Zhang, X. (2015). Characterisation of polyphenol oxidase and peroxidase and the role in browning of loquat fruit. Czech J. Food Sci. 33, 109–117. doi: 10.17221/384/2014-cjfs
Singleton, V. L., and Rossi, I. A. (1965). Colorimetry of total phenolics with phospho-molybdic-phosphotugstic acid reagents. Am. J. Enol. Vitic. 16, 144–158. doi: 10.5344/ajev.1965.16.3.144
Sinkovič, L., Sinkovič, D. K., and Ugrinović, K. (2023). Yield and nutritional quality of soil-cultivated crisphead lettuce (Lactuca sativa L. var. capitata) and corn salad (Valerianella spp.) harvested at different growing periods. Food Sci. Nutr. 11, 1755–1769. doi: 10.1002/fsn3.3205
Siomos, A. S., Beis, G., Papadopoulou, P. P., and Barbayiannis, N. (2001). Quality and composition of lettuce (cv. Plenty) grown in soil and soilless culture. Acta Hortic. 548, 445–450. doi: 10.17660/ActaHortic.2001.548.52
Skrypnik, L., Styran, T., Savina, T., and Golubkina, N. (2021). Effect of selenium application and growth stage at harvest on hydrophilic and lipophilic antioxidants in lamb's lettuce (Valerianella locusta L. Laterr.). Plants 10:2733. doi: 10.3390/plants10122733
Toivonen, P. M., and Stan, S. (2004). The effect of washing on physicochemical changes in packaged, sliced green peppers. Int. J. Food Sci. Technol. 39, 43–51. doi: 10.1111/j.1365-2621.2004.00744.x
Tomás-Barberán, F. A., and Espín, C. J. (2001). Phenolic compounds and related enzymes as determinants of quality in fruits and vegetables. J. Sci. Food Agric. 81, 853–876. doi: 10.1002/jsfa.885
Yang, X., Gil, M. I., and Yang, Q. (2022). Bioactive compounds in lettuce: highlighting the benefits to human health and impacts of preharvest and postharvest practices. Compr. Rev. Food Sci. Food Saf. 21, 4–45. doi: 10.1111/1541-4337.12877
Zhan, L. (2009). The qualitative and physiological responses of minimally processed leafy vegetables to pre/postharvest factors. Torino, Italy: Università degli Studi di Torino.
Zhan, L., Bulgari, R., Pignata, G., Casale, M., and Nicola, S. (2022). The mixing ratio and filling-amount affect the tissue browning and antioxidant properties of fresh-cut baby leaf lettuce (Lactuca sativa L.) and rocket (Eruca sativa mill.) grown in floating growing systems. Foods. 11:3515. doi: 10.3390/foods11213515
Zhan, L., Li, J., Huang, W., Song, C., Li, J., Pang, L., et al. (2020). Light irradiation affects the total antioxidant capacity, total phenolic compounds, phenolic acids, and related enzyme activities of minimally processed spinach (Spinacia oleracea L.). J. Food Process. Preserv. 44:e14825. doi: 10.1111/jfpp.14825
Keywords: culture system, fresh-cut chain, total phenols, antioxidant capacity, enzyme activity, shelf-life
Citation: Zhan L, Fan Y, Fontana E, Tibaldi G and Nicola S (2025) Preharvest culture systems and postharvest factors synergistically improved the antioxidant property of minimally processed corn salad (Valerianella olitoria L.) during cold storage. Front. Sustain. Food Syst. 8:1468986. doi: 10.3389/fsufs.2024.1468986
Edited by:
Debabandya Mohapatra, Indian Council of Agricultural Research (ICAR), IndiaReviewed by:
Hong Ru Liu, Shanghai Academy of Agricultural Sciences, ChinaVictor Escalona, University of Chile, Chile
Copyright © 2025 Zhan, Fan, Fontana, Tibaldi and Nicola. This is an open-access article distributed under the terms of the Creative Commons Attribution License (CC BY). The use, distribution or reproduction in other forums is permitted, provided the original author(s) and the copyright owner(s) are credited and that the original publication in this journal is cited, in accordance with accepted academic practice. No use, distribution or reproduction is permitted which does not comply with these terms.
*Correspondence: Silvana Nicola, c2lsdmFuYS5uaWNvbGFAdW5pdG8uaXQ=