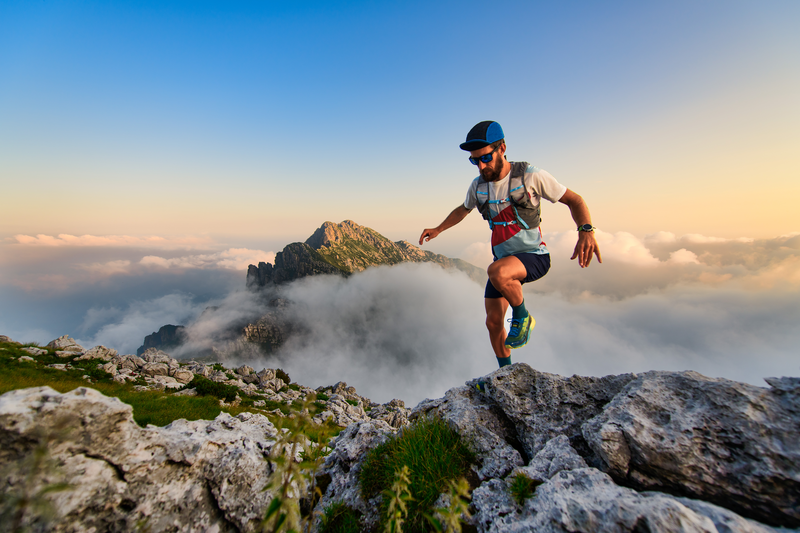
95% of researchers rate our articles as excellent or good
Learn more about the work of our research integrity team to safeguard the quality of each article we publish.
Find out more
ORIGINAL RESEARCH article
Front. Sustain. Food Syst. , 13 January 2025
Sec. Agroecology and Ecosystem Services
Volume 8 - 2024 | https://doi.org/10.3389/fsufs.2024.1457462
This article is part of the Research Topic Increasing Resilience through Multi-Species Intercropping, Agroforestry and Mixed Grazing Crop-Livestock Systems View all 10 articles
Mixed orchards, planted with different species of tree crops, are a form of a traditional cropping system that has been practiced for millennia in the Mediterranean and provides the important ecosystem service of carbon sequestration. We used six allometric equations (M1-M6) based on existing literature and data from 49 orchards for estimating tree total biomass (TB) and carbon sequestration, based on C content of dry biomass. A species/geographically-specific equation (M1), a genus-specific (M2), a genus/geographically-specific forest equation (M3), two generalized forest allometric equations (M4 and M5) and a generalized agricultural landscape equation (M6) were compared and yielded an average of 15.42, 10.80, 11.39, 6.12, 6.66, and 9.88 Mg C ha−1, respectively. Organic and conventional orchards at the same productive stage did not differ significantly from each other in CO2 sequestration (CO2seq) per tree per year (10.42 and 10 kg CO2eq, respectively). Equation M1, was considered as the most representative (species and environment) for use in perennial Mediterranean orchards. The use of allometric equations is proposed as a simple, effective, and efficient method to estimate CO2 sequestration from mixed orchards using easily measurable biometric characteristics of the trees. The findings are important for the future estimation of CO2 stocks of agricultural landscapes.
The challenge of achieving sustainably produced food and other agricultural commodities without compromising ecosystems resilience is receiving considerable global attention (FAO, 2017; Gerten et al., 2020). There are growing concerns regarding the intensifying impacts of global climate change on agriculture and other ecosystems (Mehmood et al., 2020; IPCC, 2021), such as the occurrence of extreme weather events and variations in climate conditions (heat waves, hail, drought and/or floods) (Raza et al., 2019). These events have adverse effects on various aspects of agricultural systems, such as plant growth and productivity (Rosenzweig et al., 2013; Zhao et al., 2017; Dhankher and Foyer, 2018). Mitigating climate change requires a comprehensive and collaborative effort in every sector to address and control greenhouse gas (GHG) emissions, leading to healthier and more resilient ecosystems. Agricultural sector activities in 2019 accounted for 22% (13 GtCO2-eq) of global total net anthropogenic GHG emissions (IPCC, 2021). The three atmospheric GHGs associated with the agri-food systems are carbon dioxide (CO2), methane (CH4) and nitrous oxide (N2O) (Snyder et al., 2009; Balafoutis et al., 2017). Due to the rapid global population growth, food security and global environmental stability are at risk and intensive agriculture leads to higher GHG emissions (Arora, 2019; Jhariya et al., 2024).
Carbon sequestration can be defined as any continuous accumulation of carbon either in soil, in plant materials or in the sea (Hutchinson et al., 2007), through processes that ultimately increase the carbon content of any carbon pool other than the atmosphere. Fruit tree orchards have an important role in the carbon sequestration process (Scandellari et al., 2016; Henry et al., 2020; Sharma et al., 2021), CO2 from the atmosphere is sequestered through photosynthesis and then stored as carbon in the biomass (tree trunks, branches, foliage and roots) and soil (IPCC et al., 2018; Sharma et al., 2021; Jhariya et al., 2024). Orchard biomass can significantly contribute to sequestration as an additional terrestrial carbon pool for the storage of atmospheric CO2 (Montanaro et al., 2021). Fruit trees orchards globally, account for ca. 22% of irrigated agricultural land (Yasin et al., 2021). However, the worldwide contribution of carbon sequestration by trees under agricultural land and their function as a potential carbon sink is so far poorly understood and likely significantly underestimated (Zomer et al., 2016; Plenet et al., 2022). Carbon sequestration and storage in perennial tree biomass can be considered as a sustainable, nature-based and cost-effective agricultural solution to mitigate the impacts associated with GHG emissions (Asbjornsen et al., 2013; Quiñones et al., 2013; Griscom et al., 2017).
A notable contribution to the carbon sequestration potential can be achieved where farmers adopt agricultural practices that maximize CO2 sequestration (Wu et al., 2012; Bithas and Latinopoulos, 2021). The potential to reduce and/or control greenhouse gas emissions through the application of agricultural management practices in different systems such as organic, conservation, integrated, regenerative and/or precision agriculture has been examined in several studies (Gomiero et al., 2011; Lal, 2015; Balafoutis et al., 2017; Du et al., 2022). All targeted management practices applied in the above-mentioned systems, fall under the umbrella of “carbon farming,” aiming at mitigating and compensating the negative impacts of climate change (Malhi et al., 2021). Certain management practices (e.g., organic fertilization, efficient irrigation management, low tillage, soil cover, tree planting) contribute to both emissions’ reduction and avoidance, compared to conventional practices, but also enhance carbon removal through its sequestration and long-term storage in tree biomass and soils (Pardo et al., 2017).
Evaluation of the importance of fruit trees in reducing atmospheric CO2 usually involves assessing the biomass contribution of monocultures such us apples (Panzacchi et al., 2012; Wu et al., 2012) and grapevines (Miranda et al., 2017). The cultivation of mixed orchards (orchards that comprise more than one crop species in the same plot of land, e.g., stone, pome, other fruits and nuts), together with further adoption of farming practices that contribute to carbon storage should be treated as a priority in agricultural climate mitigation initiatives, as they can increase carbon sequestration, offset and help reduce GHG emissions (Liu et al., 2016; Toensmeier, 2017) while providing additional benefits in terms of ecosystem services (Ioannidou et al., 2022). Mixed orchards genetic diversity enhances the ability to maintain biomass levels in agricultural landscapes, improving the resilience and resistance of agroecosystems to environmental variability (Hajjar et al., 2008).
As trees are a carbon sink for terrestrial ecosystems, with an important role in the global carbon cycle (Stephenson et al., 2014; Zomer et al., 2016), the amount of carbon they sequester depends significantly on the biomass they produce. A possible approach that could provide insight into the extent of benefits of atmospheric CO2 removal resulting from carbon sequestration by fruit trees is to estimate the aboveground and belowground biomass (total biomass) (IPCC et al., 2008). Thus, orchards can be monitored and inventoried in annual national GHG reports and reliable estimates should be obtained based on the accumulation of site-specific measurements (Montanaro et al., 2017; IPCC, 2019). On-site estimations are important, as tree biomass production and their CO2 sequestration capacity varies and depends on various parameters (tree species, soil and environmental conditions, management practices). However, this monitoring involves destructive sampling (Ledo et al., 2018).
Allometric equations are a fundamental tool in ecological research for estimating tree biomass. These equations establish a relationship between easily measurable tree parameters, such as diameter at breast height (DBH), height, and wood density, with the tree’s biomass (Brown, 2002; Chave et al., 2005). Allometric equations vary depending on tree species, geographical location, and environmental conditions, requiring specific models for accurate biomass estimation (Kuyah et al., 2012; Miranda et al., 2017). They are essential for calculating carbon stocks, understanding ecosystem productivity, and informing climate change models, as trees play a significant role in the global carbon cycle by sequestering carbon dioxide from the atmosphere.
The aim of this study is to quantify tree biomass and its potential of carbon sequestration in mixed orchards in the Mediterranean, using as a model the island of Cyprus. The specific objectives are to (1) identify suitable allometric equations from the literature, (2) quantify the total biomass of trees of 10 deciduous fruit tree species commonly planted in mixed orchards, after tree growth measurements, (3) compare the carbon sequestration and storage capacity in tree total biomass of organic and conventional orchards, and (4) compare six allometric equations and assess their suitability for use in the context of Mediterranean agroecosystems.
The climate of Cyprus is considered semi-arid Mediterranean according to Köppen climate classification (Peel et al., 2007). Soils are generally poor in organic matter and closely associated to parent material and landscape position (Camera et al., 2017). Thin (leptic) and stony (lithic) soils dominate the mountainous areas developing mostly as residuum while in coastal areas, soils form on transported materials (e.g., alluvial and colluvial deposits) (Camera et al., 2017).
Ten deciduous fruit tree species (fig, Ficus carica; walnut, Juglans regia; apple, Malus domestica; Pistachio, Pistacia vera; apricot, Prunus armeniaca; Cherry, Prunus avium; Plum, Prunus domestica; Almond, Prunus dulcis; Peach, Prunus persica; Pomegranate, Punica granatum) cultivated in Cyprus and supporting food supply and other ecosystem services (Ioannidou et al., 2022) were examined in the present study. The 10 different species are commonly found in mixed stands in orchards of the island and elsewhere in the Mediterranean.
Forty-nine mixed fruit orchards (25 conventional and 24 organic) located in mountainous and semi mountainous areas of Cyprus were selected for the study (Supplementary Table S1; Figure 1). The average orchard size was 0.58 ha, while the minimum and maximum size was 0.10 and 1.67 ha, respectively (Supplementary Table S1). Small and irregularly shaped parcels of agricultural land are the norm in Cyprus, especially on the mountains (Adamides et al., 2020). Most of the orchards were at full production stage, with an average tree age of 13 years, while the ages recorded ranged from 4 to 40 years, covering all stages of tree development (juvenile, productive and mature). Further information and characteristics of the orchards are presented in Supplementary Table S1. In all mixed orchards of the study 2–3 species coexisted in the same field, which is considered typical. According to the presence of the dominant crop cultivated in each orchard, (genus/species to which the dominant crop belongs), 10 types of orchards were identified (Supplementary Table S2). The grouping was considered necessary to estimate tree total biomass and the carbon sequestration capacity. The total biomass estimations were performed for the dominant species in each of the selected orchards.
For the determination of tree biomass and carbon sequestration, in situ measurements were carried out and the following data were obtained: tree age (in years), trunk girth at breast height (GBH) (in cm) and tree height (in m) (average data of five trees). Additional parameters required for the purposes of the study were then calculated. Specifically, tree trunk radius (r) (in cm), tree trunk diameter at breast height (DBH) (in cm) and tree basal area (BA) (in cm2) calculated based on the above GBH measurements. Orchards’ locations (coordinates) and orchards plot size (in hectares) were also recorded. Wood density (ρ) (in g/cm3) data for Malus domestica, Prunus persica, Prunus domestica, Prunus avium, Prunus armeniaca, Punica granatum and Juglans regia were obtained from the database of Zanne et al. (2009). Since wood data density were not available for Ficus carica and Pistacia vera mean values were extracted from the Zanne et al. (2009) using data on other species of the genera Ficus and Pistacia, respectively. Specifically, wood density for Ficus carica was calculated as the average of Ficus anthelmintica, Ficus citrifolia and Ficus eugenioides. Similarly, wood density for Pistacia vera was calculated as the average of Pistacia chinensis and Pistacia integerrima. For Prunus dulcis, where data also were not available in the database, wood density was calculated as the average of the results of the relevant studies on the same species by Fathi et al. (2022) and Nabi et al. (2017).
Allometric equations were used to calculate aboveground biomass per tree species, based on a literature review for specific and generalized allometric equations for the 10 cultivated species in the study. We used the following query in Scopus database to search for allometric equations suitable for temperate and/or Mediterranean environment and included the genera and common names for the fruit species under study: “allometric equations” AND (“Europe” OR “Mediterranean”) AND (“prunus” OR “malus” OR “pistachia” OR “juglans” OR “ficus carica” OR “punica” OR “apples” OR “peach” OR “almonds” OR “plums” OR “cherries” OR “apricots” OR “figs” OR “walnuts” OR “pistachios” OR “pomegranates”) AND (LIMIT-TO (SUBJAREA, “AGRI”) OR LIMIT-TO (SUBJAREA,"ENVI”). The search resulted in 177 studies. These were further limited geographically to the countries of the Mediterranean basin (France, Spain, Italy, Portugal, Greece, Egypt, Israel, Spain) and 45 relevant studies were identified. The review revealed limited availability of species and/or site specific allometric equations for agricultural fruit species in the Mediterranean. In particular, the results exhibited specific equations for the cultivated species Malus domestica (apple tree) and Prunus persica (peach tree). Furthermore, it became apparent that allometric equations for biomass estimation of trees are widely used methods for forest species, in contrast to agricultural species. The above results were considered and then the most relevant studies in the literature were used to identify further information (Daniel, 2012; Yadav et al., 2019). The selection of allometric equations originating from other countries was deemed necessary due to the absence of any specific and/or generalized allometric equations for the 10 fruit species under study in Cyprus.
Six allometric equations (methods M1-6) were used (Table 1) to calculate Above Ground Biomass (AGB) in the studied orchards. Selection was based on similarity to the characteristics of the current study in terms of the cultivated species (e.g., apple, peach, almond), the parameters (tree biometrics) used by each equation (trunk diameter at breast height; DBH and tree height; H), the range of DBH and H dimensions, and their geographical distribution (Mediterranean-Europe-Global).
The below ground biomass in each orchard (root system biomass) was calculated using a factor adopted by IPCC (2008), which takes a value of 0.28 (percentage of below ground biomass to above ground tree biomass). Subsequently, as tree biomass is a measure of the dry mass of woody and leaf mass (kg), aboveground biomass and belowground biomass were combined to determine total biomass (TB) per tree for each orchard (Equation 7). Leaf biomass usually contributes less than 5% of the total aboveground biomass (Delitti et al., 2006) and due to the deciduous nature of species included in the present study, this parameter was not considered. Therefore, a multiplication factor of 1.28 was applied to quantify the total biomass per tree corresponding to each method employed. TB was calculated in kg dry weight/tree and then expressed in the study in Mg/ha, (a planting density of 300 trees per hectare was typically observed).
Where:
• TΒΜ1-6: total biomass per tree (kg dry weight/tree) per method
• 1.28: IPCC proportional factor for calculating below ground biomass
• AGBΜ1-6: aboveground biomass (kg dry weight/tree) per method
The carbon content of a tree’s biomass represents approximately 50% of its total dry biomass (IPCC, 2019). For the purposes of this study, carbon fraction (Cf, % carbon) values for each cultivated species were obtained from Bilanzdija et al. (2012) where the percentage of carbon for each species was determined using the weight fraction on dry basis (wt. % on dry basis). For orchards with pomegranate (Punica granatum L.) and pistachios (Pistacia vera) as the dominant cultivated species, since no published data were found to justify a different value, the default value of 0.50 according to IPCC guidelines (2019) was applied. The carbon content for each tree was calculated by multiplying the total dry weight biomass by the carbon fraction of each tree species.
Carbon sequestration in orchards is attributed to CO2 captured in permanent structures (e.g., wood and roots). The stored carbon in total tree biomass is then converted into a stoichiometric carbon dioxide (CO2) ‘stock’ with a molecular weight ratio (CO2:C 44/12 = 3.67), as referred to in Litskas et al. (2020). Determining the weight of carbon dioxide sequestered per tree (in kg) was calculated per method according to Equation 8 (Fransen, 2019). The results were then expressed as an annual value (CO2seq/tree/year) after dividing the calculated value by tree age for each orchard.
• Where
• CO2 seqΜ1-6: Carbon sequestration per tree per method, (kg/tree)
• TB Μ1-6: Total biomass per tree per method, (kg dry weight/tree)
• Cf: Carbon fraction of tree biomass, (% carbon)
Summary statistics were calculated for the results TBM1-6, CO2seqM1-6/tree/year that were obtained by applying the six allometric equations (Table 1). One-factor analysis of variance (ANOVA) was conducted to examine whether there were statistically significant differences in the mean values of carbon sequestration (CO2 seq) produced by M1 between the two cropping systems (organic and conventional) for all (n = 49) orchards. A second analysis was performed, comparing organic and conventional orchards of the same productive stage. Therefore, 10 organic and 11 conventional orchards (n = 21) were selected (after excluding orchards less than 8 years old and more than 18 years old). In this context, the Kruskal-Wallis statistical test was used, as the assumption of normal distribution was not met. Data analysis was performed in SPSS v.20.
Supplementary Table S3 presents descriptive statistics of biometric characteristics of sampled trees from the 49 orchards in the study. Mean orchard age was 13.9 years, mean trunk diameter was 11.1 cm (with a range from 3.2 to 31.8 cm) and mean tree height was 4.6 m.
The TB (in dry weight; d.w.) as estimated by M1-M6 allometric equations is illustrated in Figure 2. The highest mean TB was predicted by equation M1 (31.89 Mg ha−1) and the lowest by equation M4 (12.60 Mg ha−1). Four equations, M1-3 and M6 predicted biomass above 20 Mg ha−1, while equations M4 and M5 provided lower values. The mean predicted TB for all methods was 20.74 Mg ha−1. The results showed that the total biomass carbon of all methods yielded an average value of 10.04 Mg C ha−1, while the values per Method (M1-6) were 15.42, 10.80, 11.39, 6.12, 6.66, and 9.88 Mg C ha−1, respectively (Supplementary Table S5).
Figure 2. Total biomass (Mg dry weight ha−1) for all the study orchards (conventional and organic) per method. M1–M6, corresponding to equations 1–6, respectively. In the boxplot: the x point indicates the mean value, the line in the box shows the median, the box shows the interquartile range (IQR), the whiskers show 1.5xIQR, and the points are the outliers.
We selected M1 as most representative for the case of Cypriot orchards, as it was closer to the cultivated species and climate, and compared C sequestration in organic and conventional orchards, per dominant species. A one-factor analysis of variance (ANOVA) was applied where the dependent variable defined was “carbon dioxide sequestration (CO2seq)” and the independent variable was “agricultural management practices,” (two levels: organic-conventional). The analysis of variance was preceded by testing the assumptions of normality and homoscedasticity of residuals. Statistical analysis of the 49 observations (24 organic orchards, 25 conventional orchards) obtained from the proxy M1 method revealed a statistically significant effect of the factor “agricultural management practices” and therefore a statistically significant difference between its two levels (F = 12.12, df = 1, p < 0.05) was recorded (Figure 3). The mean carbon sequestration values obtained for each system were 9.09 and 13.19 kg CO2/tree/year for organic and conventional orchards, respectively.
Figure 3. Carbon sequestration in studied orchards by agricultural management system. CO2 sequestration: (kg CO2/tree/year), agricultural management system: Org: organic, Con: conventional orchards. In the boxplot: the x point indicates the mean value, the line in the box shows the median, the box shows the interquartile range (IQR), the whiskers show 1.5xIQR, and the points are the outliers.
Since the study sample consisted of orchards in three stages (young, productive, and mature), the carbon sequestration results were further analyzed with the sample consisting of 10 organic and 11 conventional orchards (n = 21) in the productive phase (removing orchards less than 8 years old and more than 18 years old). In this context, the Kruskal-Wallis statistical test was used, as the assumption of normal distribution was not met. The test examines the null hypothesis that the mean rankings of the dependent variable “CO2 seq” (kg CO2/tree/year), at each of the two levels of the factor “agricultural management practices “, organic (Org) and conventional (Con) are equal. The results revealed that there was no statistically significant difference between the two systems (p > 0.05), at a 95% confidence interval (Figure 4). The mean carbon sequestration values obtained for each agricultural management system were 10.42 and 10.00 kg CO2/tree/year for organic and conventional orchards, respectively.
Figure 4. Distribution of carbon sequestration in studied orchards by agricultural management system in productive orchards. CO2 sequestration: (kg CO2/tree/year), agricultural management system: Org: organic, Con: conventional orchards. In the boxplot: the x point indicates the mean value, the line in the box shows the median, the box shows the interquartile range (IQR), the whiskers show 1.5xIQR, and the points are the outliers.
The results of the six methods (M1-M6) used to estimate tree biomass are illustrated in Figure 5. The mean carbon sequestration values obtained for each method in kg CO2 per tree per year were M1:11.18, M2:6.61, M3:7.28, M4:3.78, M5:3.58 and M6: 4.38. These values correspond to 3,354, 1983, 2,184, 1,134, 1,074, 1,314 kg CO2 ha−1 year−1, as the planting density was 300 trees per ha. The M1 method yielded the highest values, followed by M3 and M2 which received reduced values of 34.9, 40.8% respectively, compared to M1. The generalized allometric methods M6, M4 and M5 yielded lower carbon sequestration in tree biomass by 56.7, 66.2, and 68%, respectively, compared to the values obtained by the M1 method.
Figure 5. Carbon sequestration in orchards by method. Carbon sequestration: (kg CO2/tree/year), Method: M1-M6 refer to allometric equations 1–6, respectively. In the boxplot: the x point indicates the mean value, the line in the box shows the median, the box shows the interquartile range (IQR), the whiskers show 1.5xIQR, and the points are the outliers.
Limited information is currently available on the potential of Mediterranean agroecosystems for C storage, due to lack of data regarding the C content of biomass in perennial systems, such as orchards. Being perennial crops, orchards present significant sequestration potential (Funes et al., 2022) by storing atmospheric carbon in their vegetative biomass over a long term (Montanaro et al., 2017; Da Silva et al., 2022). Calculating above and below ground biomass of trees, by using models (such as allometric equations) allows generating important data on the ability of agroecosystems to mitigate the effects of climate change (Paustian et al., 2016; Vicente-Vicente et al., 2016) through carbon sequestration and storage (Sahoo et al., 2021; Sharma et al., 2021). In this work, the six models used for estimating the annual total biomass in orchards located in Cyprus, yielded an average value of 20.74 Mg dry weight (d.w.) ha−1 (Figure 2). Using the carbon fraction (approx. 48%) derived from literature for each species, the C content of trees in the evaluated orchards was estimated using M1-6 with an average of 10.48 Mg C ha−1, while M1 (proxy method) yielded 15.42 Mg C ha−1 (Supplementary Table S5).
The selected models estimate total biomass based on parameters such as tree species, age, tree growth and wood density (Table 1). Comparable results are available in the current literature, from olive, nuts, and fruit trees in Spain, where the C content in trees ranged between 13.86 and 27.40 Mg C ha−1, after estimations based on allometric equations (Almagro et al., 2010; Funes et al., 2022). Carbon content in total biomass values in citrus crops, (assessed by allometric equations through a non-destructive approach) ranged from 6.98 to 10.28 Mg C ha−1 in Pakistan (Yasin et al., 2021), while the use of destructive methods in citrus orchards in Spain (Iglesias et al., 2013), resulted in a value of approximately 10 Mg C ha−1 per year, indicating comparative proximity between the two different methods. In addition, in Germany Winzer et al. (2017), using a destructive method recorded values of total biomass of 18.3 Mg d.w. ha−1 in apple orchards at 10–15 years old, results that are in accordance with both the average TB recorded in the present study (20.74 Mg d.w. ha−1) and the average age of the orchards (13.9 years). Similarly using destructive methods, slightly higher range of 22.72 to 28.70 Mg d.w. ha−1 was estimated in a study by Scandellari et al. (2016) in high density apple orchards in Italy.
In contrast to perennial, annual crops have a lower potential for C sequestration in vegetation, which is typically removed from the system after harvesting (Winzer et al., 2017). In Denmark Chen et al. (2022) studied a system of 10 different crop types, including two monocultures (maize and triticale), crop rotation species (e.g., sugar beet, hemp), five intensively fertilized perennial grasses (tall fescue, festulolium, reed canary, cocksfoot and miscanthus) and two grass/legume mixtures, where biomass yield ranged from 9.0 Mg d.w. ha−1 to 18.5 Mg d.w. ha−1. Lower values, as expected, were recorded in other crops such as cotton (3.11 Mg d.w. ha−1) and soybean (3.1 Mg d.w. ha−1) (Nouri et al., 2019). In addition, in a study of ground cover crops by Wendling et al. (2019), biomass production was reported to be highly variable, ranging from 1 Mg d.w. ha−1 to about 7 Mg d.w. ha−1. More particularly mean values were between 2 and 3.2 Mg d.w. ha−1 for individual crops (Brassica juncea, Pisum sativum, Avena strigosa, Phacelia tanacetifolia, Guizotia abyssinica, and Raphanus sativus longipinnatus) and about 3.5 Mg d.w. ha−1 for mixtures (50% legumes and 50% other species). Compared to the above-mentioned crop types, the results of the present study indicate a greater contribution to the ecosystem service of carbon storage by perennial crops.
When considering CO2 sequestration in the biomass, significantly higher values were observed for conventional orchards (Figure 3). This is mainly due to the younger stage (average tree age 11 years) of development in the organic orchards than what was observed in conventional (average tree age 17 years) (Supplementary Table S4), which results in lower biomass in permanent plant structures, ultimately resulting in lower C content in biomass. Although this is obvious, it is a picture for the state of C storage in the orchards, which involve younger trees in organic orchards. A comparison of orchards belonging to the same production age (Figure 4), resulted in no difference between organic and conventional orchards, regarding CO2 sequestration per tree per year. This is an important finding, in terms of managing the orchards of the island and shifting toward organic production, which results in numerous benefits in terms of ecosystems services and environmental protection (Ioannidou et al., 2022). Tree age as expected influences biomass production and C storage therein. Plenet et al. (2022) observed significantly lower biomass growth and carbon sequestration in orchards with peach trees at the juvenile stage compared to trees in their productive phase. Considering intensive management practices, Khalsa et al. (2020), reported that intensive nitrogen fertilization as compared to reduced fertilization increased biomass and total carbon storage in a seven-year-old almond orchard. Furthermore, total carbon sequestration in the standing biomass of peach trees was not significantly affected by the influence of organic and conventional farming practices (Montanaro et al., 2017), a result consistent with the results of the analysis on orchards of the same age in the present study. However, in a previous study in apple orchards of the same age, trees under organic management showed higher biomass compared to trees grown under conventional management (Roussos and Gasparatos, 2009) and therefore showed an increased capacity for CO2seq. Regarding the results of our study, in comparison to the available literature, we stress the need for validating the models that were selected to field studies related to the C content of trees. This unavoidably will require the implementation of experiments involving destructive sampling, which was not possible during the time of this research.
In the context of the study, six methods were examined as possible ways of estimating the carbon sequestration potential of orchards of 10 different species. In particular, the M1 method of Ledo et al. (2018) differs in the CO2seq values obtained compared to the other methods (M2–M6). Quantification of biomass and carbon sequestration with this method employs an allometric equation as a function of age (year), a parameter not used in any of the other methods that calculate CO2seq based on biometric characteristics of the trees. The results of the variable CO2seq by M1 are also the highest obtained among the six methods (M.O.: CO2seq 11.18 kg/tree/year). The quantification of carbon sequestration using the allometric equation M2 of Johnson and Gerhold (2001) was performed to compare its results with the M1 equation, as they concern the same genus (Malus spp.), yet under different climatic conditions. The CO2seq results, compared between M1-M2 methods, differed by about 40% (reduction in the M2 method), even if they were developed for the same genus. This fact strongly suggests that field validation should be tailored to the use of allometric equations. The M3 method of Annighöfer et al. was adopted in the study for comparison with the M1 method, as both apply to Temperate and Mediterranean conditions (Annighöfer et al., 2012). In addition, M3 is consider a genus-specific equation relative to the cultivated species in the study. However, M3 shows 34.9% decrease in the mean CO2seq values obtained compared to M1. The importance of the species over the genus when developing and applying allometric equations to better estimate biomass and carbon stocks was also observed in deciduous forests in dry areas (Abich et al., 2018).
Additionally, the three methods (M4–6) by Chave et al. (2014), Brown (1997) and Kuyah et al. (2012) showed similar carbon sequestration values, underestimating M1 results by 66.2, 68, and 56.7%, respectively. M4 and M5 are related to forest species and yielded the lowest values in the study (M4: CO2seq 3.78 and M5: 3.58 Kg/tree/year, mean values). These equations are calibrated at the regional level and therefore might not function very well at the micro- (tree) level (Zianis and Mencuccini, 2003; Kebede and Soromessa, 2018). Supportive to this, a study by Vorster et al. (2020) showed that local forest species equations performed better than equations applied at the national level. Another study also suggests that allometric equations (if available) developed at the tree species and local levels should be developed and used instead of those developed at the national, or larger level (Ameztegui et al., 2022). Our study supports that when using models at the species or genus level yields higher values for C sequestration in tree biomass. In addition, larger scale forest species equations (M4 and M5) may not be applicable to agricultural species as they underestimate biomass and carbon sequestration. Relevant are the findings of Daba and Soromessa (2019) and Pandey et al. (2021) where a comparison of allometric equations revealed the need to develop allometric models for biomass prediction based on plant species and site conditions.
The present study only considered the carbon balance at the tree biomass level, whereas an ecosystem-level carbon balance should consider, among other things, the distribution within the plant (part stored in perennial organs), outputs to fruits and transport to the soil (prunings, leaves, dead roots) (Panzacchi et al., 2012). One important limitation in this study was the lack of validation of the allometric equations used for calculating total tree biomass for several of the cultivated species in the study. Validation would result in more robust comparisons and conclusions between methods for estimating the CO2seq potential of orchards. Nevertheless, the comparison of the six CO2 seq calculation methods yielded different results depending on the allometric equation. In the absence of validated allometric equations, the conditions prevailing in each case (area, climate, genus/species) should be considered when choosing an allometric equation to obtain the most accurate estimations and reduce the uncertainty of carbon sequestration measurements.
Allometric equations were selected and used in this study to estimate C sequestration in tree biomass. The method of Ledo et al. (2018) was chosen as a proxy method for all deciduous species in the study, due to its proximity to the climatic conditions and the species considered. The adoption and application of allometric equations provides the opportunity to create baseline scenarios of estimated biomass, carbon sequestration and storage in agricultural landscapes, thus providing easy and cost-effective information to climate change mitigation strategies. The contribution of orchards to carbon emissions as a terrestrial carbon sink should be considered by policy makers, through support for tree growers (government subsidies, payments, training, etc.) to strengthen and develop their operations. There is a high potential for improving our estimations. For more accurate estimates of the potential of mixed orchards to sequester carbon and function mainly as carbon pools, further research is needed considering the biometric characteristics of each individual plant species, soil and climatic conditions and the variation in biomass production during the different phenological phases of the trees to maintain a balance between yield and vegetative growth. The acquisition of the above data for several years, combined with laboratory analyses of carbon content in tree biomass, could validate the allometric equations selected and produce more accurate results for the tree species present in the orchards of Cyprus.
The datasets generated and/or analyzed during the current study are available from the corresponding author on reasonable request.
SI: Conceptualization, Data curation, Investigation, Methodology, Writing – original draft, Writing – review & editing. VL: Conceptualization, Methodology, Writing – original draft, Writing – review & editing. MS: Supervision, Writing – review & editing. IV: Conceptualization, Methodology, Supervision, Writing – review & editing.
The author(s) declare that no financial support was received for the research, authorship, and/or publication of this article.
VL was employed by the VL Sustainability Metrics Ltd.
The remaining authors declare that the research was conducted in the absence of any commercial or financial relationships that could be construed as a potential conflict of interest.
The author(s) declared that they were an editorial board member of Frontiers, at the time of submission. This had no impact on the peer review process and the final decision.
All claims expressed in this article are solely those of the authors and do not necessarily represent those of their affiliated organizations, or those of the publisher, the editors and the reviewers. Any product that may be evaluated in this article, or claim that may be made by its manufacturer, is not guaranteed or endorsed by the publisher.
The Supplementary material for this article can be found online at: https://www.frontiersin.org/articles/10.3389/fsufs.2024.1457462/full#supplementary-material
Abich, A., Mucheye, T., Tebikew, M., Gebremariam, Y., and Alemu, A. (2018). Species-specific allometric equations for improving aboveground biomass estimates of dry deciduous woodland ecosystems. J. For. Res. 30, 1619–1632. doi: 10.1007/s11676-018-0707-5
Adamides, G., Kalatzis, N., Stylianou, A., Marianos, N., Chatzipapadopoulos, F., Giannakopoulou, M., et al. (2020). Smart farming techniques for climate change adaptation in Cyprus. Atmos 11:557. doi: 10.3390/atmos11060557
Almagro, M., López, J., Boix-Fayos, C., Albaladejo, J., and Martínez-Mena, M. (2010). Belowground carbon allocation patterns in a dry Mediterranean ecosystem: a comparison of two models. Soil Biol. Biochem. 42, 1549–1557. doi: 10.1016/j.soilbio.2010.05.031
Ameztegui, A., Rodrigues, M., and Granda, V. (2022). Uncertainty of biomass stocks in Spanish forests: a comprehensive comparison of allometric equations. Eur. J. For. Res. 141, 395–407. doi: 10.1007/s10342-022-01444-w
Annighöfer, P., Mölder, I., Zerbe, S., Kawaletz, H., Terwei, A., and Ammer, C. (2012). Biomass functions for the two alien tree species Prunus serotina Ehrh. and Robinia pseudoacacia L. in floodplain forests of northern Italy. Eur. J. For. Res. 131, 1619–1635. doi: 10.1007/s10342-012-0629-2
Arora, N. K. (2019). Impact of climate change on agriculture production and its sustainable solutions. Environ. Sustain. 2, 95–96. doi: 10.1007/s42398-019-00078-w
Asbjornsen, H., Hernandez-Santana, V., Liebman, M., Bayala, J., Chen, J., Helmers, M., et al. (2013). Targeting perennial vegetation in agricultural landscapes for enhancing ecosystem services. Renew. Agric. Food Syst. 29, 101–125. doi: 10.1017/s1742170512000385
Balafoutis, A., Beck, B., Fountas, S., Vangeyte, J., Wal, T., Soto, I., et al. (2017). Precision agriculture technologies positively contributing to GHG emissions mitigation. Farm Prod. Econ. Sustain. 9:1339. doi: 10.3390/su9081339
Bilanzdija, N., Voca, N., Kricka, T., Matin, A., and Jurisic, V. (2012). Energy potential of fruit tree pruned biomass in Croatia. Spanish. J Agri. Res. 10, 292–298. doi: 10.5424/sjar/2012102-126-11
Bithas, K., and Latinopoulos, D. (2021). Managing tree-crops for climate mitigation. An economic evaluation trading-off carbon sequestration with market goods. Sustain. Prod. Consumpt. 27, 667–678. doi: 10.1016/j.spc.2021.01.033
Brown, S. (1997). “Estimating biomass and biomass change of tropical forests: a Primer”, in FAO Forestry Paper 134. Rome, Italy: Food and Agriculture Organization of the United Nations.
Brown, S. (2002). Measuring carbon in forests: current status and future challenges. Environ. Pollut. 116, 363–372. doi: 10.1016/s0269-7491(01)00212-3
Camera, C., Zomeni, Z., Noller, J. S., Zissimos, A. M., Christoforou, I. C., and Bruggeman, A. (2017). A high resolution map of soil types and physical properties for Cyprus: a digital soil mapping optimization. Geoderma 285, 35–49. doi: 10.1016/j.geoderma.2016.09.019
Chave, J., Andalo, C., Brown, S., Cairns, M. A., Chambers, J. Q., Eamus, D., et al. (2005). Tree allometry and improved estimation of carbon stocks and balance in tropical forests. Oecologia 145, 87–99. doi: 10.1007/s00442-005-0100-x
Chave, J., Réjou-Méchain, M., Búrquez, A., Chidumayo, E., Colgan, M. S., Delitti, W. B. C., et al. (2014). Improved allometric models to estimate the aboveground biomass of tropical trees. Glob. Chang. Biol. 20, 3177–3190. doi: 10.1111/gcb.12629
Chen, J., Manevski, K., Lærke, P. E., and Jørgensen, U. (2022). Biomass yield, yield stability and soil carbon and nitrogen content under cropping systems destined for biorefineries. Soil Tillage Res. 221:105397. doi: 10.1016/j.still.2022.105397
Da Silva, L., Souza, T., Laurindo, L. K., Nascimento, G., de, E., and Freitas, H. (2022). Aboveground biomass, carbon sequestration, and yield of Pyrus pyrifolia under the management of organic residues in the subtropical ecosystem of southern Brazil. Agronomy 12:231. doi: 10.3390/agronomy12020231
Daba, D. E., and Soromessa, T. (2019). The accuracy of species-specific allometric equations for estimating aboveground biomass in tropical moist montane forests: case study of Albizia grandibracteata and Trichilia dregeana. Carbon Balance Manag. 14:18. doi: 10.1186/s13021-019-0134-8
Daniel, J. (2012). Sampling essentials: practical guidelines for making sampling choices. Thousand Oaks, CA: SAGE Publications, Inc.
Delitti, W. B. C., Meguro, M., and Pausas, J. G. (2006). Biomass and mineral mass estimates in a “cerrado” ecosystem. Rev. Bras. Bot. 29, 531–540. doi: 10.1590/s0100-84042006000400003
Dhankher, O. P., and Foyer, C. H. (2018). Climate resilient crops for improving global food security and safety. Plant Cell Environ. 41, 877–884. doi: 10.1111/pce.13207
Du, C., Li, L., and Effah, Z. (2022). Effects of straw mulching and reduced tillage on crop production and environment: a review. Water 14:2471. doi: 10.3390/w14162471
FAO (2017). The future of food and agriculture – trends and challenges. Available at: https://www.fao.org/3/i6583e/I6583E.pdf (Accessed May 20, 2023).
Fathi, L., Hasanagić, R., Iranmanesh, Y., Dahmardeh Ghalehno, M., Humar, M., and Bahmani, M. (2022). Physical and chemical properties of three wild almond wood species grown in Zagros forests. Les 71, 23–30. doi: 10.26614/les-wood.2022.v71n01a03
Fransen, B. (2019). How to calculate CO2 sequestration. EcoMatcher. Available at: https://test6.ecomatcher.com/how-to-calculate-co2-sequestration/ (Accessed January 1, 2022).
Funes, I., Molowny-Horas, R., Savé, R., De Herralde, F., Aranda, X., and Vayreda, J. (2022). Carbon stocks and changes in biomass of Mediterranean woody crops over a six-year period in NE Spain. Agron. Sustain. Dev. 42. doi: 10.1007/s13593-022-00827-y
Gerten, D., Heck, V., Jägermeyr, J., Bodirsky, B. L., Fetzer, I., Jalava, M., et al. (2020). Feeding ten billion people is possible within four terrestrial planetary boundaries. Nat. Sustain. 3, 200–208. doi: 10.1038/s41893-019-0465-1
Gomiero, T., Pimentel, D., and Paoletti, M. G. (2011). Environmental impact of different agricultural management practices: conventional vs. organic agriculture. Crit. Rev. Plant Sci. 30, 95–124. doi: 10.1080/07352689.2011.554355
Griscom, B. W., Adams, J., Ellis, P. W., Houghton, R. A., Lomax, G., Miteva, D. A., et al. (2017). Natural climate solutions. Proc. Natl. Acad. Sci. U. S. A. 114, 11645–11650. doi: 10.1073/pnas.1710465114
Hajjar, R., Jarvis, D. I., and Gemmill-Herren, B. (2008). The utility of crop genetic diversity in maintaining ecosystem services. Agric. Ecosyst. Environ. 123, 261–270. doi: 10.1016/j.agee.2007.08.003
Henry, R. J., Furtado, A., and Rangan, P. (2020). Pathways of photosynthesis in non-leaf tissues. Biology 9:438. doi: 10.3390/biology9120438
Hutchinson, J. J., Campbell, C. A., and Desjardins, R. L. (2007). Some perspectives on carbon sequestration in agriculture. Agric. For. Meteorol. 142, 288–302. doi: 10.1016/j.agrformet.2006.03.030
Iglesias, D. J., Quiñones, A., Font, A., Martínez-Alcántara, B., Forner-Giner, M. Á., Legaz, F., et al. (2013). Carbon balance of citrus plantations in eastern Spain. Agric. Ecosyst. Environ. 171, 103–111. doi: 10.1016/j.agee.2013.03.015
Ioannidou, S. C., Litskas, V. D., Stavrinides, M. C., and Vogiatzakis, I. N. (2022). Linking management practices and soil properties to ecosystem Services in Mediterranean mixed orchards. Ecosyst. Serv. 53:101378. doi: 10.1016/j.ecoser.2021.101378
IPCC (2019). “2019 refinement to the 2006 IPCC guidelines for national greenhouse gas inventories,” in The intergovernmental panel on climate change. eds. C. Buendia, K. Tanabe, A. Kranjc, J. Baasansuren, M. Fukuda, and S. Ngarize, et al. Switzerland: IPCC.
IPCC (2021). Climate change 2021: the physical science basis. Contribution of working group I to the sixth assessment report of the intergovernmental panel on climate change. eds. V. Masson-Delmotte, P. Zhai, A. Pirani, S. Connors, C. Péan, and Y. Chen, et al. (Cambridge, United Kingdom and New York, NY, USA: Cambridge University Press). In press.
IPCC (2008). 2006 IPCC guidelines for national greenhouse gas inventories – A primer, prepared by the national greenhouse gas inventories programme. eds. H. S. Eggleston, K. Miwa, N. Srivastava, and K. Tanabe. (Japan: IGES).
IPCCMasson-Delmotte, V., Zhai, P., Pörtner, H. O., Roberts, D., Skea, J., et al. (2018). Summary for policymakers. In: global warming of 1.5°C. An IPCC special report on the impacts of global warming of 1.5°C above pre-industrial levels and related global greenhouse gas emission pathways, in the context of strengthening the global response to the threat of climate change, sustainable development, and efforts to eradicate poverty : Cambridge University Press.
Jhariya, M. K., Singh, L., and Toppo, S. (2024). Wildfires and carbon budget of certain seasonally dry forests in India. Land Degrad. Dev. 35, 3771–3789. doi: 10.1002/ldr.5166
Johnson, A., and Gerhold, H. (2001). Carbon storage by utility-compatible trees. Arboricult. Urban For. 27, 57–68. doi: 10.48044/jauf.2001.008
Kebede, B., and Soromessa, T. (2018). Allometric equations for aboveground biomass estimation of Olea europaea L. subsp. cuspidata in Mana Angetu Forest. Ecosyst. Health Sustain. 4, 1–12. doi: 10.1080/20964129.2018.1433951
Khalsa, S. D. S., Smart, D. R., Muhammad, S., Armstrong, C. M., Sanden, B. L., Houlton, B. Z., et al. (2020). Intensive fertilizer use increases orchard N cycling and lowers net global warming potential. Sci. Total Environ. 722:137889. doi: 10.1016/j.scitotenv.2020.137889
Kuyah, S., Dietz, J., Muthuri, C., Jamnadass, R., Mwangi, P., Coe, R., et al. (2012). Allometric equations for estimating biomass in agricultural landscapes: I. Aboveground biomass. Agric. Ecosyst. Environ. 158, 216–224. doi: 10.1016/j.agee.2012.05.011
Lal, R. (2015). Sequestering carbon and increasing productivity by conservation agriculture. J. Soil Water Conserv. 70, 55A–62A. doi: 10.2489/jswc.70.3.55a
Ledo, A., Heathcote, R., Hastings, A., Smith, P., and Hillier, J. (2018). Perennial-GHG: a new generic allometric model to estimate biomass accumulation and greenhouse gas emissions in perennial food and bioenergy crops. Environ. Model Softw. 102, 292–305. doi: 10.1016/j.envsoft.2017.12.005
Litskas, V., Mandoulaki, A., Vogiatzakis, I. N., Tzortzakis, N., and Stavrinides, M. (2020). Sustainable viticulture: first determination of the environmental footprint of grapes. Sustain. MDPI. 12:8812. doi: 10.3390/su12218812
Liu, C., Cutforth, H., Chai, Q., and Gan, Y. (2016). Farming tactics to reduce the carbon footprint of crop cultivation in semiarid areas. A review. Agron. Sustain. Dev. 36. doi: 10.1007/s13593-016-0404-8
Malhi, G. S., Kaur, M., and Kaushik, P. (2021). Impact of climate change on agriculture and its mitigation strategies: a review. Sustain. MDPI. 13:1318. doi: 10.3390/su13031318
Mehmood, I., Bari, A., Irshad, S., Khalid, F., Liaqat, S., and Anjum, H., et al. (2020). Carbon cycle in response to global warming,” in Environment, climate, plant and vegetation growth, eds. S. Fahad, M. Hasanuzzaman, M. Alam, H. Ullah, M. Saeed, and A. Khan, et al. (Springer International Publishing), 1–15.
Miranda, C., Santesteban, L. G., Escalona, J. M., De Herralde, F., Aranda, X., Nadal, M., et al. (2017). Allometric relationships for estimating vegetative and reproductive biomass in grapevine (Vitis vinifera L.). Aust. J. Grape Wine Res. 23, 441–451. doi: 10.1111/ajgw.12285
Montanaro, G., Amato, D., Briglia, N., Russo, C., and Nuzzo, V. (2021). Carbon fluxes in sustainable tree crops: field, ecosystem and global dimension. Sustainability 13:8750. doi: 10.3390/su13168750
Montanaro, G., Tuzio, A. C., Xylogiannis, E., Kolimenakis, A., and Dichio, B. (2017). Carbon budget in a Mediterranean peach orchard under different management practices. Agric. Ecosyst. Environ. 238, 104–113. doi: 10.1016/j.agee.2016.05.031
Nabi, S., Qaisar, K. N., Rather, S. A., Khan, P. A., and Nabi, B. (2017). Fuelwood characteristics of some important tree species in prevalent agroforestry Systems of District Budgam, Kashmir Valley. Int. J. Curr. Microbiol. App. Sci. 6, 3801–3806. doi: 10.20546/ijcmas.2017.611.445
Nouri, A., Lee, J., Yin, X., Saxton, A. M., Tyler, D. D., Sykes, V. R., et al. (2019). Crop species in no-tillage summer crop rotations affect soil quality and yield in an Alfisol. Geoderma 345, 51–62. doi: 10.1016/j.geoderma.2019.02.026
Pandey, H. P., Bhandari, S. K., and Harrison, S. (2021). Comparison among allometric models for tree biomass estimation using non-destructive trees’ data. Trop. Ecol. 63, 263–272. doi: 10.1007/s42965-021-00210-0
Panzacchi, P., Tonon, G., Ceccon, C., Scandellari, F., Ventura, M., Zibordi, M., et al. (2012). Belowground carbon allocation and net primary and ecosystem productivities in apple trees (Malus domestica) as affected by soil water availability. Plant Soil 360, 229–241. doi: 10.1007/s11104-012-1235-2
Pardo, G., del Prado, A., Martínez-Mena, M., Bustamante, M. A., Martín, J. A. R., Álvaro-Fuentes, J., et al. (2017). Orchard and horticulture systems in Spanish Mediterranean coastal areas: is there a real possibility to contribute to C sequestration? Agric. Ecosyst. Environ. 238, 153–167. doi: 10.1016/j.agee.2016.09.034
Paustian, K., Lehmann, J., Ogle, S., Reay, D., Robertson, G. P., and Smith, P. (2016). Climate-smart soils. Nature 532, 49–57. doi: 10.1038/nature17174
Peel, M. C., Finlayson, B. L., and McMahon, T. A. (2007). Updated world map of the Köppen-Geiger climate classification. Hydrol. Earth Syst. Sci. 11, 1633–1644. doi: 10.5194/hess-11-1633-2007
Plenet, D., Borg, J., Barra, Q., Bussi, C., Gomez, L., Ould-Sidi Memah, M.-M., et al. (2022). Net primary production and carbon budget in peach orchards under conventional and low input management systems. Eur. J. Agron. 140:126578. doi: 10.1016/j.eja.2022.126578
Quiñones, A., Martínez-Alcántara, B., Font, A., Forner-Giner, M. Á., Legaz, F., Primo-Millo, E., et al. (2013). Allometric models for estimating carbon fixation in Citrus trees. Agron. J. 105, 1355–1366. doi: 10.2134/agronj2013.0015
Raza, A., Razzaq, A., Mehmood, S. S., Zou, X., Zhang, X., Lv, Y., et al. (2019). Impact of climate change on crops adaptation and strategies to tackle its outcome: A review. Plants. 8:34. doi: 10.3390/plants8020034
Rosenzweig, C., Elliott, J., Deryng, D., Ruane, A. C., Müller, C., Arneth, A., et al. (2013). Assessing agricultural risks of climate change in the 21st century in a global gridded crop model intercomparison. Proc. Natl. Acad. Sci. 111, 3268–3273. doi: 10.1073/pnas.1222463110
Roussos, P. A., and Gasparatos, D. (2009). Apple tree growth and overall fruit quality under organic and conventional orchard management. Sci. Hortic. 123, 247–252. doi: 10.1016/j.scienta.2009.09.011
Sahoo, U. K., Nath, A. J., and Lalnunpuii, K. (2021). Biomass estimation models, biomass storage and ecosystem carbon stock in sweet orange orchards: implications for land use management. Acta Ecol. Sin. 41, 57–63. doi: 10.1016/j.chnaes.2020.12.003
Scandellari, F., Caruso, G., Liguori, G., Meggio, F., Palese, A. M., Zanotelli, D., et al. (2016). A survey of carbon sequestration potential of orchards and vineyards in Italy. Eur. J. Hortic. Sci. 81, 106–114. doi: 10.17660/ejhs.2016/81.2.4
Sharma, S., Rana, V. S., Prasad, H., Lakra, J., and Sharma, U. (2021). Appraisal of carbon capture, storage, and utilization through fruit crops. Front. Environ. Sci. 9. doi: 10.3389/fenvs.2021.700768
Snyder, C. S., Bruulsema, T. W., Jensen, T. L., and Fixen, P. E. (2009). Review of greenhouse gas emissions from crop production systems and fertilizer management effects. Agric. Ecosyst. Environ. 133, 247–266. doi: 10.1016/j.agee.2009.04.021
Stephenson, N. L., Das, A. J., Condit, R., Russo, S. E., Baker, P. J., Beckman, N. G., et al. (2014). Rate of tree carbon accumulation increases continuously with tree size. Nature 507, 90–93. doi: 10.1038/nature12914
Toensmeier, E. (2017). “Perennial staple crops and agroforestry for climate change mitigation,” in Integrating landscapes: Agroforestry for biodiversity conservation and food sovereignty. Advances in Agroforestry, vol 12. ed. F. Montagnini (Cham: Springer).
Vicente-Vicente, J. L., García-Ruiz, R., Francaviglia, R., Aguilera, E., and Smith, P. (2016). Soil carbon sequestration rates under Mediterranean woody crops using recommended management practices: a meta-analysis. Agric. Ecosyst. Environ. 235, 204–214. doi: 10.1016/j.agee.2016.10.024
Vorster, A. G., Evangelista, P. H., Stovall, A. E. L., and Ex, S. (2020). Variability and uncertainty in forest biomass estimates from the tree to landscape scale: the role of allometric equations. Carbon Balance Manag. 15:8. doi: 10.1186/s13021-020-00143-6
Wendling, M., Charles, R., Herrera, J., Amossé, C., Jeangros, B., Walter, A., et al. (2019). Effect of species identity and diversity on biomass production and its stability in cover crop mixtures. Agric. Ecosyst. Environ. 281, 81–91. doi: 10.1016/j.agee.2019.04.032
Winzer, F., Kraska, T., Elsenberger, C., Kötter, T., and Pude, R. (2017). Biomass from fruit trees for combined energy and food production. Biomass Bioenergy 107, 279–286. doi: 10.1016/j.biombioe.2017.10.027
Wu, T., Wang, Y., Yu, C., Chiarawipa, R., Zhang, X., Han, Z., et al. (2012). Carbon sequestration by fruit trees - Chinese apple orchards as an example. PLoS One 7:e38883. doi: 10.1371/journal.pone.0038883
Yadav, S. K., Singh, S., and Gupta, R. (2019). “Sampling methods,” in Biomedical statistics. Singapore: Springer. 71–83.
Yasin, G., Farrakh Nawaz, M., Zubair, M., Qadir, I., Saleem, A. R., Ijaz, M., et al. (2021). Assessing the contribution of Citrus orchards in climate change mitigation through carbon sequestration in Sargodha District, Pakistan. Sustainability 13:12412. doi: 10.3390/su132212412
Zanne, A. E., Lopez-Gonzalez, G., Coomes, D. A., Ilic, J., Jansen, S., Lewis, S. L., et al. (2009). Data from: towards a worldwide wood economics spectrum [Dataset]. Dryad. doi: 10.5061/dryad.234
Zhao, C., Liu, B., Piao, S., Wang, X., Lobell, D. B., Huang, Y., et al. (2017). Temperature increase reduces global yields of major crops in four independent estimates. Proc. Natl. Acad. Sci. 114, 9326–9331. doi: 10.1073/pnas.1701762114
Zianis, D., and Mencuccini, M. (2003). Aboveground biomass relationships for beech (Fagus moesiaca Cz.) trees in Vermio Mountain, northern Greece, and generalised equations for Fagus sp. Ann. For. Sci. 60, 439–448. doi: 10.1051/forest:2003036
Keywords: orchards, agricultural management practices, carbon sequestration, climate action, Cyprus
Citation: Ioannidou S, Litskas VD, Stavrinides M and Vogiatzakis IN (2025) The role of mixed orchards in carbon sequestration and climate change mitigation in a Mediterranean island environment. Front. Sustain. Food Syst. 8:1457462. doi: 10.3389/fsufs.2024.1457462
Received: 30 June 2024; Accepted: 16 December 2024;
Published: 13 January 2025.
Edited by:
Erik Harry Murchie, University of Nottingham, United KingdomReviewed by:
Luciano Gebler, Brazilian Agricultural Research Corporation (EMBRAPA), BrazilCopyright © 2025 Ioannidou, Litskas, Stavrinides and Vogiatzakis. This is an open-access article distributed under the terms of the Creative Commons Attribution License (CC BY). The use, distribution or reproduction in other forums is permitted, provided the original author(s) and the copyright owner(s) are credited and that the original publication in this journal is cited, in accordance with accepted academic practice. No use, distribution or reproduction is permitted which does not comply with these terms.
*Correspondence: Menelaos Stavrinides, bS5zdGF2cmluaWRlc0BjdXQuYWMuY3k=; Vassilis D. Litskas, bGl0c2thc0BnbWFpbC5jb20=
Disclaimer: All claims expressed in this article are solely those of the authors and do not necessarily represent those of their affiliated organizations, or those of the publisher, the editors and the reviewers. Any product that may be evaluated in this article or claim that may be made by its manufacturer is not guaranteed or endorsed by the publisher.
Research integrity at Frontiers
Learn more about the work of our research integrity team to safeguard the quality of each article we publish.