- 1Center for Outcomes Research and Epidemiology, Department of Diagnostic Medicine and Pathobiology, College of Veterinary Medicine, Kansas State University, Manhattan, KS, United States
- 2Foreign Arthropod-Borne Animal Diseases Research Unit, National Bio and Agro-Defense Facility, Agricultural Research Services, United States Department of Agriculture, Manhattan, KS, United States
- 3National Feral Swine Damage Management Program, Animal and Plant Health Services, United States Department of Agriculture, Fort Collins, CO, United States
The United States is considered a susceptible region with great potential for the introduction of the Japanese encephalitis virus (JEV) given the presence of competent mosquito vector species, susceptible maintenance avian hosts, large populations of susceptible domestic and feral swine, intensive travel and trade activities to and from JEV-endemic countries, similar climatic and environmental conditions to epidemic countries, and no active JEV surveillance in place. As pigs are considered JEV’s primary amplifying host, comprehensively reviewing the available body of evidence, and respective knowledge gaps, pertaining to the role of swine in JEV transmission can provide valuable guidance to decision-makers. Our objectives were to synthesize scientific literature on the role of domestic and feral swine in the transmission of JEV via a rapid systematic review and identify knowledge gaps to determine potential areas amenable for future research. A total of 3,638 records were initially identified. Data were extracted from 227 reports. Transmission of JEV occurs primarily via infected mosquitoes; however, some evidence of direct oronasal transmission between pigs has been reported. Despite pigs exhibiting a short-lived viremia, JEV has been demonstrated to persist longer in their tonsils. In sows, JEV infection may cause reproductive disorders, and although maternal antibodies can confer protection for several months under field conditions, naïve piglets can exhibit neurologic signs which may progress to wasting disease. Studies evaluating breed or sex reported no association with JEV seropositivity. Application of biosecurity practices and vector control are recommended as preventive measures against introduction and spread of JEV in swine farms. Although there is no JEV vaccine licensed for pigs in the United States, live attenuated vaccines were reported to elicit superior immunogenicity compared to inactivated vaccines used in endemic countries. Summarizing the current understanding of JEV infection in swine can guide researchers, stakeholders, and policymakers in prioritizing research efforts and developing effective countermeasures. This is particularly crucial in the event of an outbreak in the United States, where preemptive measures can help minimize the spread of the virus, safeguard both human and animal populations, and ensure the long-term sustainability of the swine production sector.
1 Introduction
Japanese Encephalitis (JE) is an emerging, zoonotic disease transmitted by mosquitoes, primarily Culex but also from other genera including Aedes, carrying JEV (family Flaviviridae: genus Flavivirus). The virus is maintained and circulates among mosquito vectors and vertebrate hosts (Le Flohic et al., 2013). Ardeid wading birds, such as egrets and herons, and both domestic and feral swine are regarded as the primary natural reservoirs and amplifying hosts of JEV, respectively. Swine are considered amplifying hosts, as they can produce and maintain high levels of viremia, allowing for continued vector-borne transmission (Ricklin et al., 2016b; Ladreyt et al., 2019; Williams et al., 2019). Although the role of swine as key contributors to JEV amplification was questioned when their removal from specific endemic regions did not affect the broader transmission dynamics (van den Hurk et al., 2008), their involvement in the JEV cycle remains crucial due to its significant socioeconomic impact on humans. Clinical signs reported from JEV infection include reproductive issues in sows and boars, and fever, depression, and neurologic signs in piglets (Park et al., 2022). Other vertebrates (i.e., humans, horses, and cattle) are considered dead-end hosts, most displaying no signs of infection (except humans and horses), and producing levels of viremia that are insufficient to infect new mosquito vectors (Figure 1).
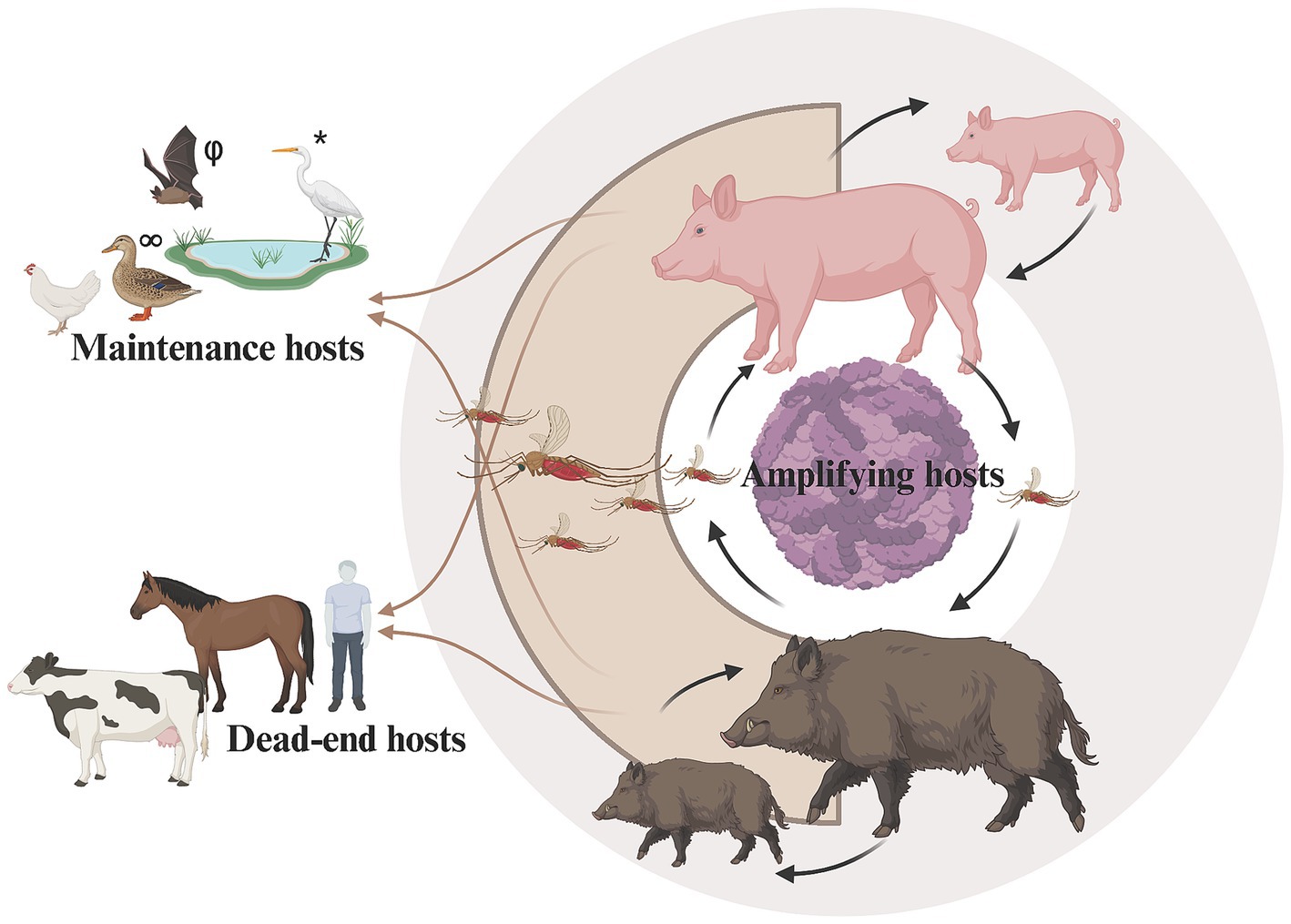
Figure 1. Schematic model highlighting the role of domestic and feral swine as amplifying hosts in the transmission cycle of Japanese encephalitis virus. *Ardeid wading birds (e.g., egrets) are maintenance hosts, but may have potential role as amplification hosts. φBats may have potential role as maintenance or amplifying hosts. ∞Other birds (e.g., ducks and chickens) may play a role as maintenance hosts. Host classifications from: Bhattacharya and Basu (2014). Created in BioRender (https://www.biorender.com).
In humans, JEV infection can cause inflammation of the brain (encephalitis), which can lead to fever, headache, respiratory distress, gastrointestinal pain, confusion, seizures, and, in some cases, death (Kumar et al., 1990; Campbell et al., 2011; Kliegman et al., 2015). Less than 1% of human cases are accompanied by symptoms, however 30% of symptomatic cases are fatal (Campbell et al., 2011). The global incidence of JE is uncertain due to variations in the effectiveness and quality of JE surveillance in endemic countries (Jayatilleke, 2020), and the availability of diagnostic testing worldwide. Researchers have estimated the yearly occurrence of human JE cases to range from 50,000 to 100,000 in countries where JEV is present (World Health Organization, 2006; Campbell et al., 2011; Quan et al., 2020). Although there are vaccines available to prevent JE, there is no specific antiviral treatment, and treatment consists of supportive care to relieve symptomology.
International trade and globalization, presence of competent vectors, susceptible amplifying and maintenance hosts, and optimal environmental conditions make the United States (U.S.) susceptible to the introduction and establishment of JEV (Oliveira et al., 2018a). As the world’s third-largest producer and consumer of pork and pork products [United States Department of Agriculture—Economic Research Service (ERS), 2024], the incursion of JEV into the United States could substantially impact the national economy. Concurrently, in the past decade, federal agencies have shown increased interest in funding research to enhance our understanding of the impact of invasive feral swine in the United States, whose population is estimated at over 6 million (United States Department of Agriculture, n.d.). This surge is driven by gaps in our knowledge about how this species affects swine disease transmission dynamics in the United States (Brown et al., 2024). Since pigs are considered an amplifying host of JEV, a systematic review of the literature and identification of knowledge gaps can inform researchers, stakeholders, and policymakers on effort prioritization, development of interventions to prevent JEV introduction, and evaluation of disease control measures in the event of a JEV incursion. While recent reviews have summarized existing knowledge regarding JEV transmission routes, patterns of viremia, and epidemiological cycles and systems (Ladreyt et al., 2019; Park et al., 2022), some knowledge gaps regarding the role of swine in JEV transmission remain. A robust understanding of the role of swine as an amplifying host for this virus is crucial for animal and public health authorities when devising interventions to curb JEV spread. The objectives of the present study were to synthesize scientific literature on the role of domestic and feral swine in the transmission of JEV, via a rapid systematic review, and identify gaps in the existing knowledge to determine potential areas amenable for future research.
2 Methods
A rapid review of the literature was conducted according to an a priori developed protocol (Veloso et al., 2023) incorporating streamlined systematic review methods proposed by Garritty et al. (2021). Post hoc changes to the protocol were incorporated as described in the protocol’s amendments section, and the updated version was made available in the same repository (K-Rex/CORE collection). This review was not registered a priori. The Preferred Reporting Items for Systematic Reviews and Meta-Analyses (PRISMA) guidelines (Page et al., 2021) were followed for reporting. We chose to utilize the systematic review label as our aim was to, not only map themes, but also synthesize data addressing specific questions which are essential to decision-makers. However, we recognize that this review’s methodology encompasses not only the principles of systematic reviews but also those of scoping reviews.
This rapid systematic review on the role of swine, both domestic and feral, in the transmission of JEV, was guided by the following research questions:
1. What are the routes of infection/transmission in swine?
2. Do swine exposed to different JEV genotypes present different macroscopic and microscopic pathological lesions?
3. Do swine exposed to different JEV genotypes exhibit variation in viral organotropism?
4. Do swine exposed to different JEV genotypes exhibit variations in clinical signs?
5. What diagnostic tests have been used to detect JEV in swine?
6. What is the sensitivity and specificity of diagnostic tests that have been evaluated for the detection of JEV in swine?
7. What is the JEV seroprevalence in swine?
8. Do swine demographics serve as risk factors for the proportion of JEV infection in swine?
9. Do the size, type, location, or other environmental characteristics of swine operations serve as risk factors for the proportion of JEV infection in swine?
10. What surveillance efforts have been conducted at the animal or farm level to detect JEV in swine?
11. Which JEV vaccines have been evaluated for their efficacy in swine?
12. What is the basic reproduction ratio (R0) for JEV in swine populations?
13. What biosecurity measures have been evaluated regarding the transmission of JEV in swine?
14. Can JEV be transmitted through/detected in pork products?
2.1 Expert and stakeholder engagement
Due to the broad scope of our objectives, expert opinion from swine producers, veterinarians, researchers, and other stakeholders from the United States and Australia with content expertise in the areas of commercial swine production and JEV was sought to prioritize research questions and identify critical outcomes. Expert opinions were gathered through emails and an electronic survey. Results from the electronic survey were strictly used to inform the review process and are not reported as a finding of this review.
2.2 Information sources
Searches of information sources were performed between August and October 2022. The bibliographic databases used were Scopus and Web of Science, which includes the following databases: Web of Science Core Collections, KCI-Korean Journal Database, MEDLINE, and SciELo Citation Index. Due to the limited peer-reviewed literature available on feral swine, we performed additional hand-searches to capture records on feral swine that may not have been indexed in the bibliographic databases. The other sources searched were United States government websites [United States Department of Agriculture (USDA) Animal and Plant Health Inspection Service (APHIS), Centers for Disease Control and Prevention (CDC), and USDA National Institute of Food and Agriculture (NIFA) Current Research Information System (CRIS)] and a repository (USDA Wildlife Services Digital Collection) as well as two citation searches. The first citation search was an author citation search of Dr. Vienna Brown’s google scholar profile, given her contributions to the field of feral swine management, and the second was a search of the reference list of the WHA fact sheet on Japanese encephalitis (Wildlife Health Australia, 2022), which reported information about JEV in wildlife in response to the latest JEV outbreak in Australia. All sources queried, dates searched, search terms, and the number of records are reported in Supplementary Table S1.
2.3 Eligibility criteria
Using a Population, Outcome, and Study design (POS) framework, two sets of eligibility criteria were designed, one for the reports from the bibliographic databases and one for reports gathered from the other sources, as the population of interest was different for the reports from the bibliographic databases (i.e., domestic and feral swine) and reports gathered from other sources (i.e., feral swine only). All criteria are summarized in Table 1.
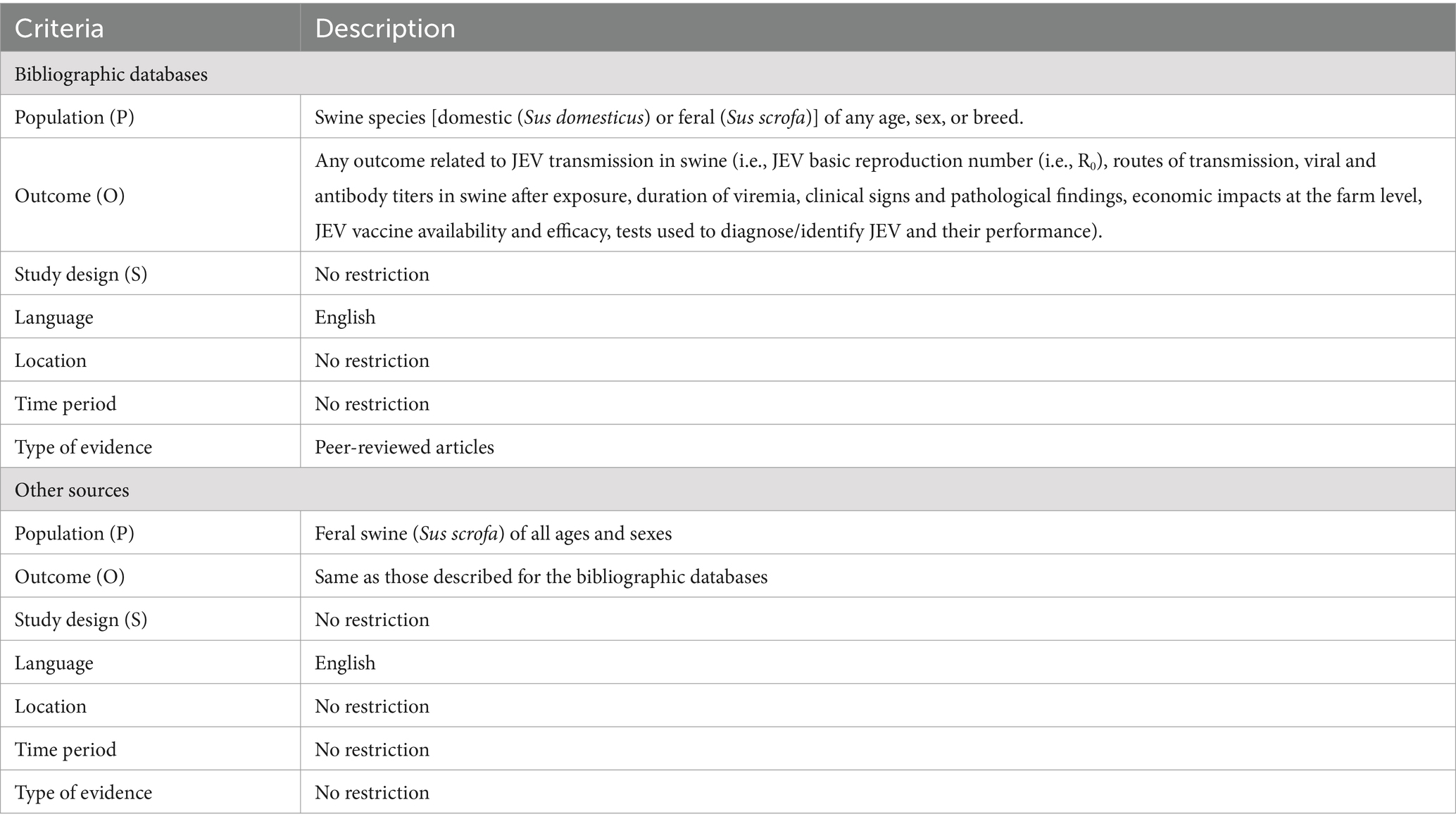
Table 1. Eligibility criteria for inclusion in the rapid systematic review on domestic and feral swine’s role in the transmission of Japanese encephalitis virus (JEV).
2.4 Search strategy
Our search strategies were informed by the POS framework described above and in Table 1. The full search strategies for the bibliographic databases are presented in Supplementary Table S1, but in brief, we searched for “Japanese encephalitis virus” and “swine” and restricted to reports written in English. Given the broad themes/questions covered and the limited search capabilities of some of the websites searched, we trialed multiple combinations of “Japanese encephalitis virus” and its synonyms (i.e., “Japanese encephalitis,” “Japanese B encephalitis,” “JEV,” “JE,” “summer encephalitis,” “viral encephalitis,” “viral meningitis,” “Russian autumnal encephalitis,” “viral encephalitis”) along with “feral swine” and synonyms for feral (i.e., “wild,” “game,” “free range,” “ranging,” “free-roaming,” “undomesticated,” “non-domesticated”) and swine (i.e., “pig,” “hog,” “boar,” “pork,” “Sus scrofa”). Based on these preliminary searches, we identified the combinations of search terms that yielded the most relevant records, unique to each website. For the hand-searching of citations, titles from reference lists were searched for language referring to “Japanese encephalitis virus” and “feral swine” or relevant synonyms.
2.5 Selection process
Records from the bibliographic databases were downloaded as Research Information Systems (RIS) files and then uploaded into Covidence (Covidence systematic review software, Veritas Health Innovation, Melbourne, Australia). Covidence’s deduplication tool was used to remove duplicate records before screening. Relevance screening of records was performed by two reviewers (VH, CH). One reviewer (VH) screened all records utilizing the following questions as a screening tool: (1) Are the title and abstract written in English? (2) Does the study population consist of swine (Sus scrofa or Sus domesticus)? (3) Does the abstract describe aspects of JEV? (4) Does the abstract describe a peer-reviewed article? (5) Does the abstract describe original research, a Systematic Review, or a Meta-analysis? (6) Does the study involve a transmission aspect of JEV in swine species, diagnostic test performance for JEV, or JEV vaccine efficacy? and (7) Is this abstract/article relevant? To each question, potential answers included “yes,” “no,” or “unclear.” A “no” answer for any question resulted in the exclusion of the record. If a record received an “unclear” answer for any question, the full report was retrieved and was screened with the previously described screening tool by another reviewer (CH). If a consensus about a record’s relevance could not be achieved, at any point in the screening process, then a tertiary reviewer (NC) was consulted.
To screen the records from the government websites and repository, a primary reviewer evaluated records for eligibility (ME) based on title only. The record title had to include a reference to JEV and feral swine, or their synonyms. If a record received an “unclear” or “yes” answer, the full report was retrieved and screened by two reviewers (ME, VH) using the previously described screening tool, except the population in question 2 was restricted to Sus scrofa. Disagreements between the primary and secondary reviewer were resolved via consensus, or through a tertiary reviewer (NC, CH) when a consensus could not be achieved.
2.6 Data collection process
A data collection tool was designed using Microsoft Excel (Microsoft Office Professional Plus 2019). Data were collected from eligible reports in duplicate by pairs of reviewers working independently (VH, CH, SE, NC, AT, and TM). Discrepancies were resolved by consensus or by a tertiary reviewer (VH, CH, and NC).
2.7 Data items
All key variables identified for data collection and any data simplifications are described in Supplementary Table S2. Citation information was extracted at the report level, while the remaining data items were extracted at the study level, considering that one report could contain descriptions of multiple studies. For each study, characteristics of the study population (breed, sex, and age), study characteristics (study type and location, sample size, design structure, and JEV case definition), and the study outcome measures that were relevant to our research questions were extracted. The extraction of the data items was tailored to the study design structure. For observational studies, risk factors were extracted. For experimental studies, interventions (e.g., vaccination, preventive/biosecurity measures, and surveillance strategy) and the route of exposure to JEV [i.e., challenge (along with dose, strain, and route) or natural] were extracted. Relevant outcomes including transmission routes, pathological lesions and viral organotropism, clinical signs, seroprevalence, and the basic reproduction ratio were extracted at the reported outcome level (i.e., animal or herd level). For studies evaluating diagnostic test performance, the outcomes of relevance included sample type, diagnostic sensitivity, diagnostic specificity, analytical sensitivity, analytical specificity and cross-reactivity.
2.8 Data synthesis methods
Data were summarized by outcomes for each research question by filtering on key data items using the R language or Microsoft Excel, and presented in either a tabular format or as text depending on the number of relevant reports. Below we describe the criteria used to synthesize data by theme/research question. If a research question was addressed by a systematic review identified through our search strategy, and no further substantial information was found, we refer the reader to the previous review. This was the case for the research questions regarding seroprevalence and routes of transmission for JEV in swine populations.
2.8.1 Pathological lesions and viral organotropism
Reports containing studies that described gross pathology, histopathology, and/or viral organotropism results were synthesized and organized by JEV genotype, system affected, type of exposure, inoculation route, and dose. Included in the synthesis table were reports that described vaccine efficacy studies; however, only animals that were challenged with JEV, but not given a vaccine (i.e., a positive control), were included in the synthesis table.
2.8.2 Clinical signs
Reports containing studies that reported clinical signs in unvaccinated swine infected with JEV were summarized. Synthesized data were organized by clinical sign category, by age category (i.e., nursing, weaning, finishing, or mature), then by JEV genotype, and within genotype, alphabetically by strain, and then inoculation route. Summarized data include report counts, population description, infection strain (genotype), infection route and dose, number and percentage of animals affected out of the total observed, and respective references.
2.8.3 Diagnostic tests
Two questions were evaluated regarding diagnostic tests for JEV. To evaluate the research question of “What diagnostic tests have been used to detect JEV in swine?,” any reported diagnostic test used to detect JEV in swine was summarized and organized by the diagnostic test method category. The categories used were adapted from the list of approved tests for detection of JEV as outlined in the World Organisation of Animal Health’s (WOAH) Manual of Diagnostic Tests and Vaccines for Terrestrial Animals (World Organisation for Animal Health, 2023). The table details the test name, test description, sample type used for the diagnostic test, and respective references.
To evaluate the research question “What is the sensitivity and specificity of diagnostic tests that have been evaluated for the detection of JEV in swine?,” we synthesized results from reports where the diagnostic sensitivity and specificity of novel (i.e., new) diagnostic tests for detecting JEV in swine were evaluated. For inclusion in the synthesis table, researchers needed to compare the novel test to a reference test. Reports were organized by the WOAH test method categories, and within each category reports were organized alphabetically by test name. Summarized information included the test method and name, sample type used, microbial species evaluated for test cross-reactivity, cross-reactivity of the test to Flavivirus spp., analytical sensitivity of the novel test, name of the reference test, diagnostic sensitivity and specificity of the novel test, and the respective references. A separate table was created for results from reports that met the inclusion criteria, but only reported analytical sensitivity and failed to report diagnostic sensitivity and specificity.
2.8.4 Risk factors
Reports including studies reporting a statistical test evaluating the association between animal-level (i.e., sex, breed, and/or age) or farm-level (e.g., environmental, management) characteristics with JEV seroprevalence, seroconversion, or viremia were synthesized. Studies evaluating swine demographic risk factors of breed, sex, and age were synthesized and organized in chronological order of publication, within each swine purpose category (domestic swine presented first followed by feral swine) by risk factor. Risk factors for domestic swine operations were grouped by farm location/geography, farm management, resources, and infrastructure, or host and vector presence around the farm. Studies are presented in chronological order of publication within each risk factor group. Summarized data include the total number of reports that evaluated each risk factor, risk factor level of stratification, swine population description, sample size, seroprevalence and/or odds ratio for JEV positivity, statistical significance of differences, and respective reference.
2.8.5 Surveillance and biosecurity
Reports including studies that statistically evaluated the effect of a surveillance strategy or biosecurity intervention on any component of JEV transmission in swine were synthesized. Results are discussed in the text.
2.8.6 Vaccine efficacy
Reports that assessed vaccine efficacy by comparing JEV vaccinated and challenged swine to positive controls, in experimental studies, were synthesized. Reports within the synthesis table are organized by type of vaccine evaluated (live-attenuated, killed, or other), then within each type in chronologically ascending order, and alphabetically by author within year. Summarized data include vaccine information, including strain and producer, study design and challenge strain, population and sample size of vaccinated swine, evidence used to determine efficacy, and respective references.
2.8.7 Basic reproduction ratio
Reports where authors modeled JEV transmission and estimated the basic reproduction ratio were synthesized. Information regarding model compartments, the control strategy evaluated (when applicable), the outcomes measured to evaluate the corresponding control strategy, the location, and the respective report references was included in the synthesis table. Reports are organized based on the similarity of the compartments evaluated across populations.
2.8.8 Pork products
Reports describing JEV detection in sample types of processed pork or pork products were sought for synthesis; however, no reports were obtained through our search strategy. Therefore, our findings regarding the existing knowledge gap pertaining to the safety and potential transmission of JEV via pork products are reported in the text.
2.9 Identification and characterization of knowledge gaps
Identification and characterization of knowledge gaps was performed using the framework proposed by Robinson et al. (2013), which was developed to identify and classify research gaps in systematic reviews. After adapting the knowledge gaps worksheet from Robinson et al. (2013) into a Microsoft excel worksheet (Supplementary material 2), reviewers (NC, AD, CH, and VH) identified knowledge gaps in the evidence synthesized for each research question and characterized the reasons for the gaps individually, each addressing a different research question. A knowledge gap synthesis was not performed when few or no reports were identified regarding the research question (i.e., surveillance, biosecurity, and pork products), or when the research question was not amenable for the knowledge gap tool utilization (i.e., diagnostic tests used, diagnostic tests evaluated, and vaccine efficacy). The following categories were utilized to classify the reason for the gap: insufficient or imprecise information, inconsistent or unknown consistency, and not the right information. Details of the explanation for each category can be found in Robinson et al. (2013) and in the Key section of the knowledge gap tool.
3 Results
3.1 Study selection
A total of 3,638 records were identified, including 3,163 from bibliographic databases and 475 from other sources. Two-hundred and twenty-six reports identified from the bibliographic database search and one report from other sources were included in the review for a total of 227 reports, describing 353 studies. A flow chart detailing the results of the screening process is presented in Figure 2.
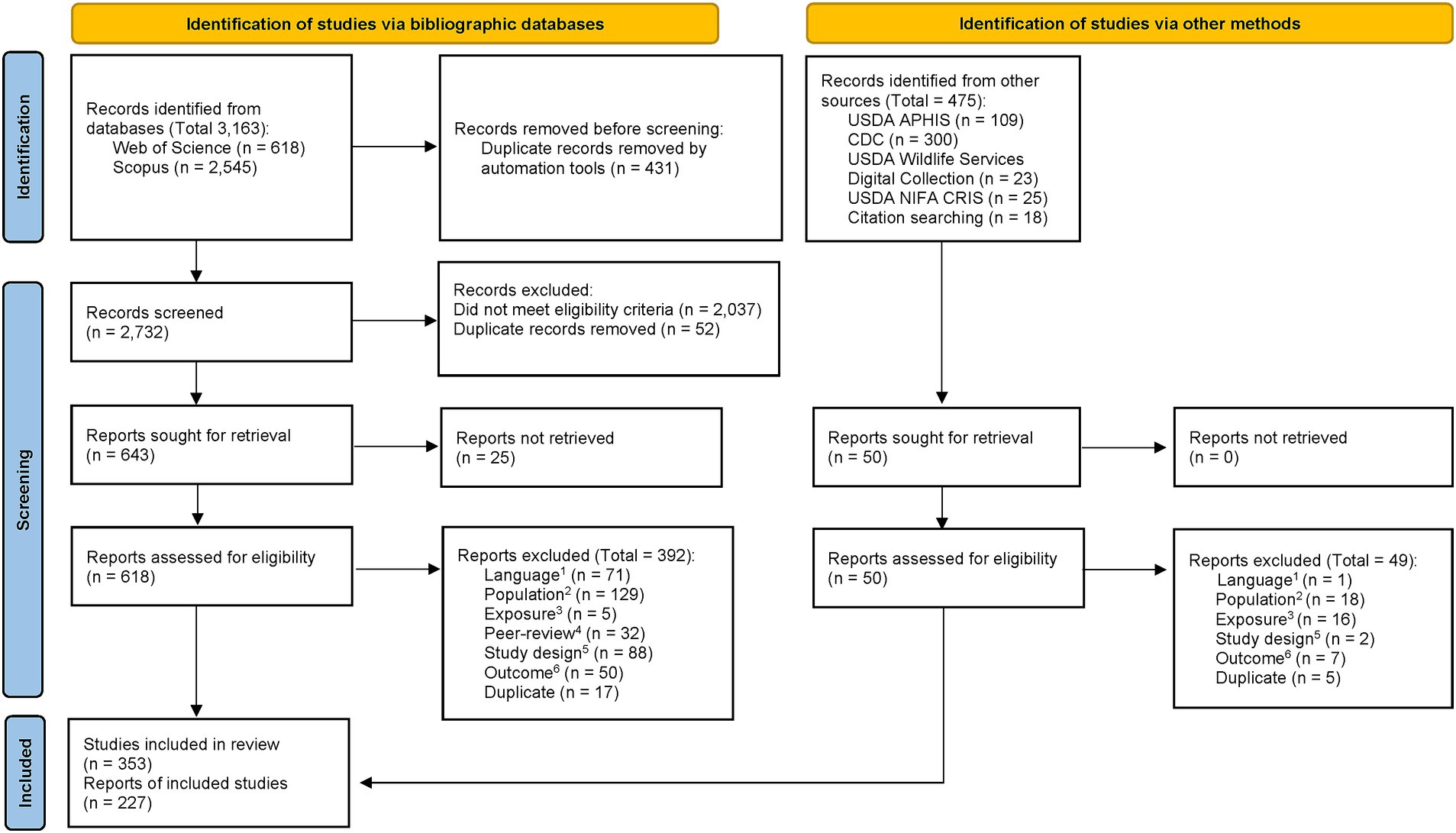
Figure 2. PRISMA flow diagram from Page et al. (2021). For more information, visit: http://www.prisma-statement.org/. 1Language: Report was not published in English. 2Population: Report did not describe outcomes of interest in swine. 3Exposure: Report did not describe JEV-related outcomes. 4Peer-review: Report was not published in a peer-reviewed publication. 5Study design: Report did not describe original research or a systematic review. 6Outcome: Report did not include data on an outcome of interest.
3.2 Study characteristics
All reports which were included in this rapid review are organized by study design in Supplementary Table S3 and, with detailed citation references listed in Supplementary material 3. Ninety-nine reports described 162 experimental studies, 135 described 180 observational studies, 10 detailed JEV modeling studies, and five reports were systematic reviews. The reports included in this review were published between 1947 and 2022 (median 2009). Nearly 40% of the reports originated from JEV endemic countries (Bangladesh, Cambodia, China, Indonesia, Laos, Malaysia, Myanmar, Philippines, Singapore, Sri Lanka, Thailand, and Vietnam), 37% were from JEV epidemic countries (Australia, Japan, North and South Korea, Nepal, and Taiwan), 16% were from India (where the northern region experiences JEV peak seasons, while the southern region observes year-round transmission), and the remaining reports were from JEV-free countries. All systematic reviews were published within the past 10 years (between 2015 and 2022). The oldest reports included in this review (i.e., 1947–1970) primarily focused on describing infection routes, pathological lesions, organotropism, and clinical signs. Reports evaluating risk factors associated with the proportion of JEV infection in swine populations were more recent, with the majority published between 2009 and 2021.
3.3 Syntheses of results
The information gathered on each research question of interest is described below and in synthesis tables, which are detailed in Table 2 along with the number of reports synthesized. Knowledge gaps associated with each research question are detailed in their respective sections, summarized in Table 2, and the completed tools are in Supplementary material 2.
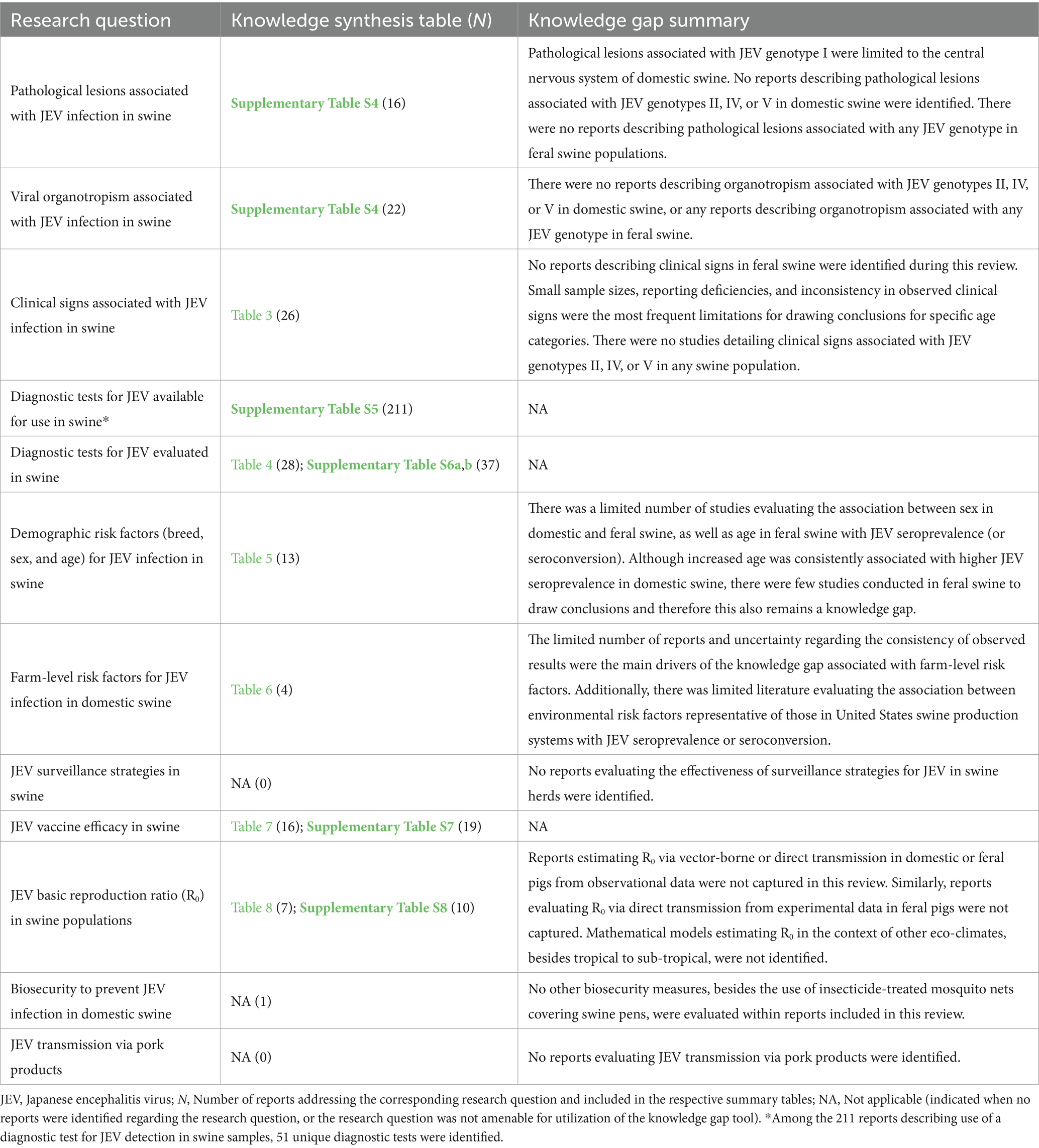
Table 2. Research questions, location of knowledge synthesis table and number of reports (N) identified and extracted per research question, and summary of knowledge gaps, identified as per Robinson et al. (2013), associated with each research question.
3.3.1 Transmission routes
The mechanisms of JEV transmission in pigs have been comprehensively summarized in the systematic review by Ladreyt et al. (2019), and our review of the literature did not uncover any additional research warranting an updated synthesis on transmission routes in swine. None of the reports included in this review described studies investigating JEV excretion and transmission through abortion fluid, a knowledge gap highlighted by Ladreyt et al. (2019). Lastly, the transmission of JEV through artificial insemination, via semen, or by the transfer of embryos derived from JEV positive animals, remains to be evaluated since the detection of JEV in swine semen and embryos by Ogasa et al. (1977) and Yoshida et al. (1981).
3.3.2 Pathological lesions and viral organotropism
Twenty-four reports describing macroscopic, microscopic pathological findings, and/or viral organotropism, associated with JEV genotype (GI, GIII, or unknown) and inoculation route and dose, were identified and are summarized in Supplementary Table S4. Pathological lesions and/or organotropism associated with JEV GI infection in swine was described in five reports, GIII infection was described in 14 reports, and nine reports described pathological and/or viral organotropic results from a JEV infection with a strain of unknown genotype.
3.3.2.1 Central nervous system
Hemorrhages in the brain and spinal cord of nursing pigs naturally exposed to either JEV GI or JEV of an unknown genotype were described by Cao et al. (2011) and Burns (1950), respectively. Yamada et al. (2004, 2009) reported brain edema in weaning aged pigs on days 3- or 7-day post inoculation, but this result was not consistent across studies or among JEV challenge strains (unknown genotype). Meiklejohn et al. (1947) also reported the inconsistent observance of hyperemia and necrosis in the brain of finishing aged pigs challenged with JEV (unknown genotype). No reports described macroscopic pathological lesions of the CNS system associated with JEV GIII infection.
After challenges with JEV GI intravenously (Park et al., 2018) and subcutaneously (Fan et al., 2018), CNS organs including nervous tissue, cerebellum, thalamus, and frontal and temporal lobe tested positive for JEV RNA 3-day post inoculation, and brain tested positive for JEV RNA 8-day post inoculation, respectively. Park et al. (2018) also isolated infectious virus from CNS tissues. However, at 11-day post inoculation, after both an intradermal and intravenous challenge, Ricklin et al. (2016a) reported that no viral RNA was detected in brain tissue. A similar pattern was found in the results of challenges with JEV GIII; JEV RNA detection and virus isolation in CNS organs varied depending on the organ, inoculation route, and dose. Sazawa et al. (1969a) reported viral isolation from both the brain and spinal cord after inoculation intracerebrally, just in the brain after intranasal inoculation, and in neither the brain nor spinal cord after a subcutaneous inoculation. Ricklin et al. (2016a) detected JEV RNA in the thalamus and basal nuclei after an oronasal inoculation, a result corroborated by the findings of García-Nicolás et al. (2017), however Ricklin et al. (2016a, 2016b) detected little to no viral RNA in the meninges, choroid plexus, and spinal cord after a joint intradermal, intravenous inoculation.
3.3.2.2 Lymphatic system
Only Burns (1950) described macroscopic pathological lesions in the lymphatic system, specifically lymphadenomegaly of the thoracic and mesenteric lymph nodes as well as splenic necrosis, following a natural JEV infection (unknown genotype) in nursing aged pigs. No reports described macroscopic pathological lesions of the lymphatic system associated with any specified JEV genotype infection.
All reports (Ricklin et al., 2016a; Fan et al., 2018; Park et al., 2018) describing viral organotropic results in organs of the lymphatic system after challenges (intradermal, intravenous, and subcutaneous) with JEV GI reported JEV RNA detection in bone marrow, lymph nodes, spleen, thymus, and/or tonsils 3–11-day post inoculation and at 25-day post inoculation in tonsils. Ricklin et al. (2016a) and Park et al. (2018) also isolated JEV from tonsils after intravenous inoculations at 106 and 107 TCID50, respectively. Similarly, all reports describing challenges with JEV GIII via intradermal, intranasal, both intradermal and intravenous, oronasal, and/or subcutaneous inoculation (Ricklin et al., 2016a, 2016b; García-Nicolás et al., 2017; Fan et al., 2018; Redant et al., 2020) reported the detection of JEV RNA in lymphatic organs, specifically bone marrow, lymph nodes, spleen, thymus, and/or tonsils 5–11-day post inoculation and at 21-day post inoculation in tonsils. Ricklin et al. (2016b) also reported isolating live virus in the tonsils up to 11-day post inoculation using a combination intradermal and intravenous inoculation method totaling 107 TCID50. Live JEV was isolated from lymph nodes, consistently, 2, 3, and 4 days after intracerebral, intranasal, and subcutaneous inoculation, and in spleens 2, 3, and 4 days after intracerebral inoculation, and up to 6 days after intranasal and subcutaneous inoculation (Sazawa et al., 1969a). However, Redant et al. (2020) reported no infectious virus in prescapular lymph nodes and spleen following intranasal inoculation at 105 TCID50.
3.3.2.3 Reproductive system
Shimizu et al. (1954) and Desingu et al. (2016) both described JEV GIII infection of pregnant sows resulting in the delivery of mummified fetuses or stillborn piglets with encephalitis and lymph node congestion in a portion of the affected litters. Piglets that were born alive from the infected sows were described as either normal or presenting with hydrocephalus and subcutaneous edema. Yoshida et al. (1981) described the recovery of affected embryos from JEV GIII infected sows. Similar macroscopic pathological findings were reported in sows infected with JEV strains of an unknown genotype along with reports of placental hemorrhage and necrosis (Fujisaki et al., 1975; Takashima et al., 1988). No reports described macroscopic pathological lesions of the reproductive system associated with JEV GI infection.
Only one report described organotropic results of GI infection in the reproductive system (Nie et al., 2022); they reported that testicular fluid and placenta and umbilical cords of aborted fetuses from naturally exposed, symptomatic boars and sows, respectively, tested positive for JEV RNA. Six reports described organotropic results of GIII infection in the reproductive system; three described studies with a natural exposure (Fan et al., 2010a; Teng et al., 2013; Desingu et al., 2016) and three described studies with a challenge of an unknown route (i.e., not reported clearly by authors) (Shimizu et al., 1954; Yoshida et al., 1981; Zheng et al., 2019). These reports describe similar results to those where viral GI RNA was detected. In addition, four reports reported isolation of JEV from fetuses and placentas from a challenged sow (Shimizu et al., 1954; Yoshida et al., 1981), fetal cerebral fluid from fetuses of naturally exposed sows (Fan et al., 2010a; Teng et al., 2013), and seminal fluid from naturally exposed boars (Teng et al., 2013). In addition, Zheng et al. (2019), reported that spermatogenic and Sertoli cells were positive for JEV E-antigen after a challenge.
3.3.2.4 Multiple systems
Pleural effusion was reported in five of 12 weaning aged pigs 5- and 7-day post inoculation with JEV GIII (Ricklin et al., 2016b), as well as in nursing aged pigs (Burns, 1950). Burns (1950) also reported subcutaneous edema, hepatic necrosis, and petechial hemorrhages of serosal membranes in nursing aged pigs.
Ricklin et al. (2016a) were the only authors to report on JEV GI RNA detection in other systems; detecting viral RNA in the ileum, kidney, liver, and skeletal muscle 3–11-day post inoculation either intradermally or intravenously at 106 TCID50. After inoculation (intracerebral, intranasal, and subcutaneous) with JEV GIII, infectious virus was isolated from lungs, livers, and/or kidneys (Sazawa et al., 1969a). However, Redant et al. (2020) reported that no JEV RNA was detected in liver and kidneys at 10- and 14-day post inoculation intranasally or intradermally with 105 TCID50. Whereas Ricklin et al. (2016b) reported JEV RNA detection in the distal ileum, liver, and kidneys up to 11-day post inoculation, low to negative JEV RNA detection in skeletal muscles at 3, 5, and 7 days post inoculation, and low RNA detection in peripheral blood at 3 days post inoculation with a combined intradermal and intravenous method at 107 TCID50. Ricklin et al. (2016a) also reported detecting JEV RNA in the ileum after oronasal inoculation. In addition to corroborating this data, García-Nicolás et al. (2017) detected JEV RNA in the jejunum, and detected low levels of RNA in urine from one animal.
3.3.2.5 Knowledge gaps
Our research question was to compare the pathological and organotropic effects between JEV genotypes in swine. The only identified genotypes used in laboratory challenge and natural exposure studies with domestic swine were genotypes I and III, although there were several challenge strains used with unknown genotypes. This creates a knowledge gap of the pathological and organotropic effects of infection with genotypes II, IV, and V in domestic swine of any age and breed. Additionally, we did not identify any reports describing pathological lesions or organotropism associated with any JEV infection in feral swine.
Only five reports identified the JEV genotype associated with the macroscopic pathological lesions described within the reports. No reports contained a direct comparison of macroscopic pathological lesions between genotypes I and III, nor did any reports describe lesions associated with JEV GI infection in any system apart from the CNS system. The limited number of reports creates a knowledge gap for comparing macroscopic pathological lesions among JEV genotypes.
There were a number of reports that examined organotropism in swine after JEV infection with genotypes I and III, however patterns from comparing the two could not be made descriptively as there likely are a number of confounding elements, such as inoculation route, dose, incubation period, and organ system investigated, that need to be considered. A further meta-analysis of this outcome is needed to close this knowledge gap.
3.3.3 Clinical signs
A total of 27 reports describing clinical signs associated with JEV infection in domestic swine were identified and are summarized in Table 3. The description of clinical signs was highly variable across swine age categories and among reported JEV genotypes (GI, GIII, and unknown). Fever observed at 2–11-days post infection was the most frequently reported clinical sign across age categories but was not reliably observed in all animals within a study population. Similarly, reproductive failure (including aborted litters and stillborn fetuses) in mature females was commonly reported, but also was not always observed in a large proportion of the study populations. Anorexia observed at 3–6-day post infection, neurological signs observed 3–27-day post infection, and depression lasting up to 13-day post infection were all reported in at least a portion of the study populations of nursing, weaning, and finishing-aged pigs with no discernible association with any JEV genotype. The occurrence of conjunctivitis (Meiklejohn et al., 1947), decreased fecal output (Ricklin et al., 2016a, 2016b), and weight loss (Park et al., 2018) were each only reported by a single research group within a single age category. In contrast to the other reports, four reports described a complete absence of clinical signs in all the pigs within their study populations (Shimizu et al., 1954; Yang et al., 2004a; Cappelle et al., 2016; Xie et al., 2022).
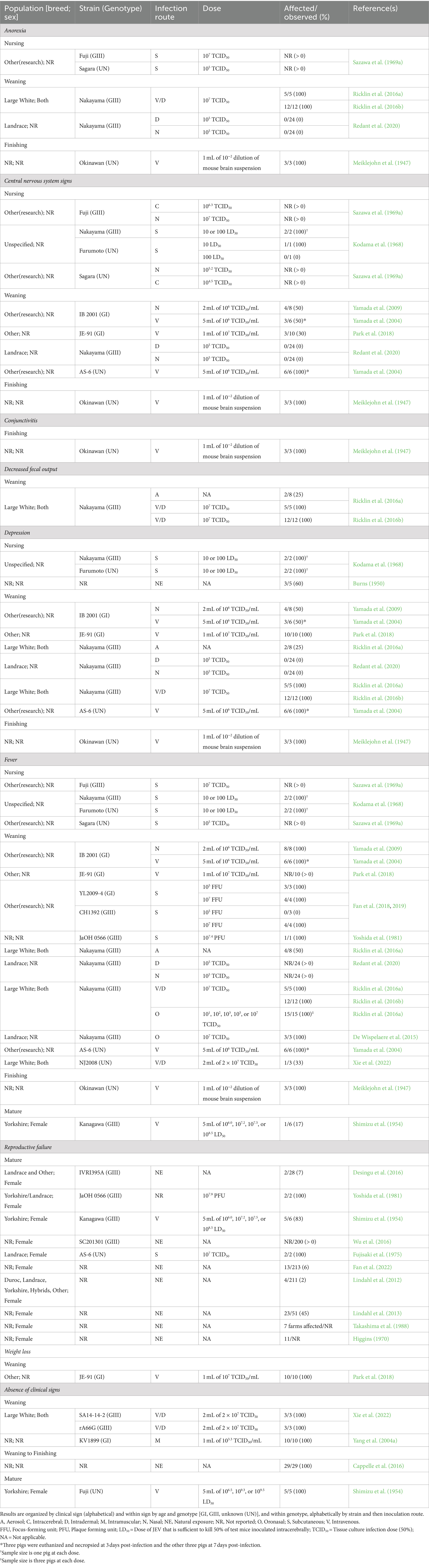
Table 3. Clinical signs associated with Japanese encephalitis virus (JEV) infection in swine from experimental and observational studies.
Only two reports directly compared differences in clinical signs in domestic swine infected with JEV GI versus GIII (Fan et al., 2018, 2019). In those reports, fever in weaning-aged pigs was consistently reported following JEV GI infection, whereas the presence of fever in weaning-aged pigs following JEV GIII infection was dependent on the challenge dose. However, sample sizes were small in these studies with three or four animals per treatment group.
No reports (0/27) describing clinical signs in feral swine were identified during this review. Small sample sizes, reporting deficiencies, and inconsistency in observed clinical signs were the most frequent limitations for drawing conclusions for specific age categories of swine. There were no studies detailing clinical signs associated with JEV genotypes II, IV, or V in any swine population.
3.3.4 Diagnostic tests
A total of 211 reports described the use of a diagnostic test (n = 51) to detect evidence of JEV exposure in biological samples from swine (Supplementary Table S5). According to WOAH (World Organisation for Animal Health, 2023), the hemagglutination inhibition test (HI) is the only recommended test for determining an animal’s freedom from infection, determining immune status post-vaccination, and surveilling for JEV. For confirmation of clinical cases, WOAH also recommends virus isolation, real time reverse-transcription PCR (RT-PCR), or a virus neutralization (VN)/plaque reduction neutralization test (PRNT), in addition to HI. Real time RT-PCR, enzyme-linked immunosorbent assay (ELISA), and VN and PRNT are recommended, with limitations, for surveillance and determination of an animal’s freedom from infection. Among reports included in this review, 97 utilized HI, with publication dates ranging from 1958 to 2021. This is the third longest method in use, surpassed only by virus isolation (1957–2022) and virus neutralization tests (1947–2022). ELISA tests have been reported in published literature since 1982 and RT-PCR since 2001. For virus detection, using a combination of testing methods is recommended. Among reports included in this review, 71 used multiple testing methods to detect JEV.
Thirty-seven reports evaluated the performance of 43 novel diagnostic tests against a reference test. Diagnostic test sensitivity and/or specificity were reported for the novel tests in 28 of these reports. The diagnostic test type, sample type tested, microbial species tested for cross-reactivity, cross-reactivity results to other flaviviruses, analytical sensitivity, the reference test name, and diagnostic sensitivity and specificity for the novel test are reported in Supplementary Table S6a, and a condensed table of these results is reported in Table 4. Nine reports only included results for analytical sensitivity and cross-reactivity of the novel test, and not diagnostic sensitivity and specificity. These results are summarized in Supplementary Table S6b.
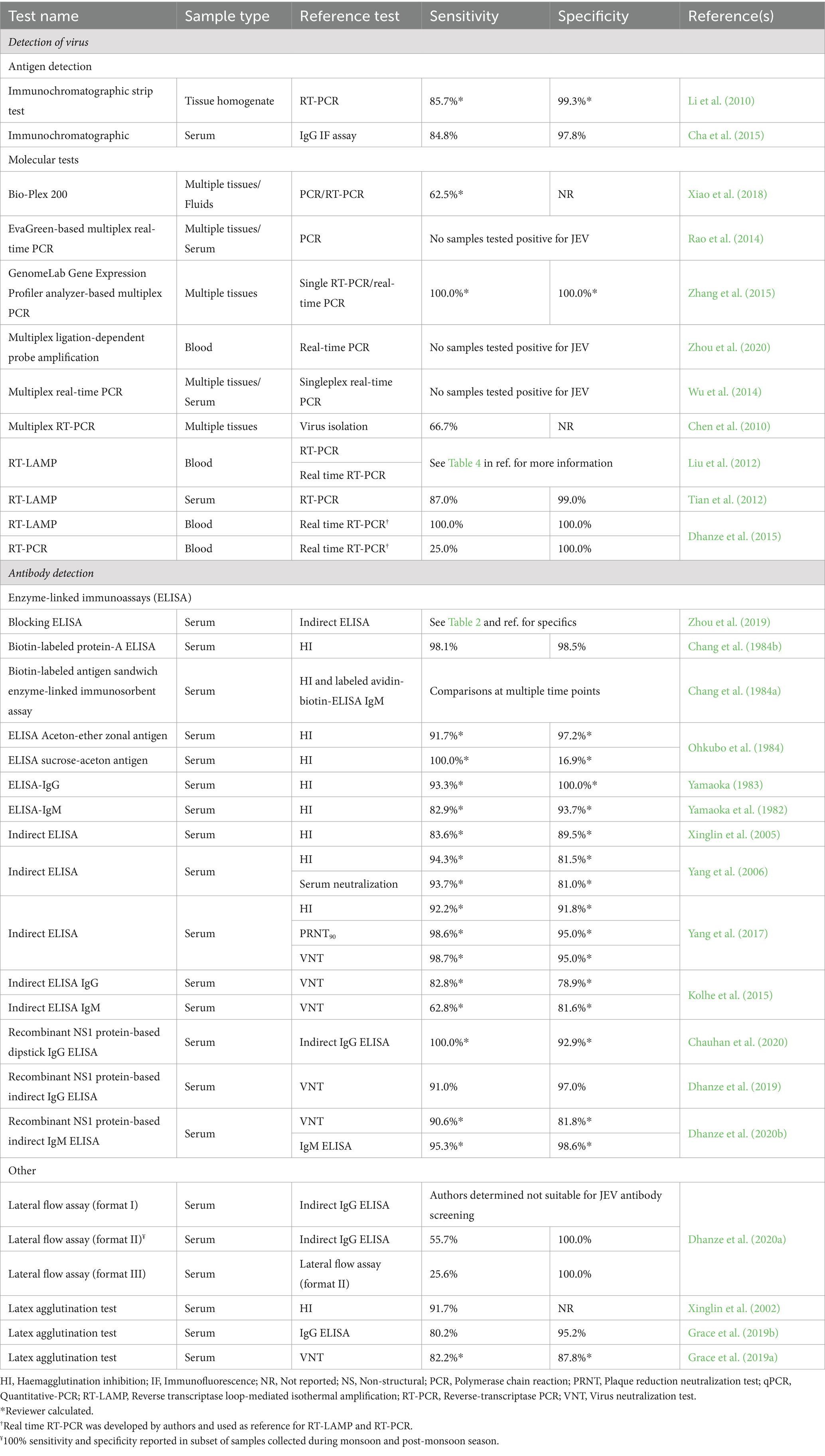
Table 4. Diagnostic sensitivity and specificity of diagnostic tests evaluated against a reference test for the detection of Japanese encephalitis virus (JEV) and JEV antibodies.
Thirteen novel tests were designed for virus detection, 85% (n = 11) of which utilized molecular methods. Twenty-one tests were designed to detect antibodies, 71% (n = 15) were ELISAs. Five reports published in the early 1980’s first described the evaluation of ELISAs and reported diagnostic sensitivity values ranging from 82.9 to 100% and diagnostic specificity values ranging from 16.9 to 100%. The remaining reports evaluating ELISAs were published after 2005 and report diagnostic sensitivity values of 62.8–100% and diagnostic specificity values of 78.9–98.6%. Reports that evaluated molecular tests have all been published since 2012 and report variable diagnostic sensitivity values.
Eight of the novel tests were assessed for cross-reactivity to other flaviviruses, specifically Dengue, West Nile, Yellow Fever, and Zika viruses (Dhanze et al., 2015; Kolhe et al., 2015; Wu et al., 2017; Pantawane et al., 2019; Wang et al., 2020), only one of which reported cross-reactivity to WNV (Kolhe et al., 2015). Twenty-six of the 43 evaluated tests reported the results of cross-reactivity assessment to other viruses and bacteria (Supplementary Tables S6a,b).
Two reports (Konishi and Yamaoka, 1982, 1983) used multiple different swine sample types to compare standard ELISA and HI methods with a rapid ELISA; however, because the authors only reported the correlation coefficients for these comparisons, these reports were not included in the summary tables.
3.3.5 Seroprevalence
The seroprevalence of JEV in swine populations, both domestic and feral, has been extensively examined, documented, and summarized elsewhere. This review identified five systematic reviews which have summarized JEV seroprevalence in swine populations, both domestic and feral, across different endemic regions and years (Lopez et al., 2015; Oliveira et al., 2017; Oliveira et al., 2018b; Ladreyt et al., 2019; Suresh et al., 2022).
Two of the 12 reports identified in this review, which described studies conducted in feral swine populations, were not included in prior reviews. These reports described observational studies, conducted in 2019, in JEV-endemic countries (China, Japan), with the primary outcome being evidence of JEV exposure in feral swine, as demonstrated by detecting JEV antibodies (Guo et al., 2019; Yonemitsu et al., 2019). While most studies on feral swine populations described the level of JEV antibody seroprevalence in serum samples, Yonemitsu et al. (2019) utilized heart and diaphragm meat juice as the diagnostic sample. After comparing results obtained from serum samples, the authors concluded that meat juice is a suitable specimen for detection of anti-JEV antibodies using ELISA (Yonemitsu et al., 2019). When evaluating knowledge gaps for this, and other, research questions of interest, it became evident that research on JEV in feral swine populations was largely unavailable.
3.3.6 Risk factors
3.3.6.1 Swine demographic risk factors
Summaries and specifics of the studies that evaluated the association between animal breed, sex, and/or age with JEV seroprevalence in swine are reported in Table 5. No significant association between domestic swine breeds and JEV seroprevalence was reported in any of the six studies in which it was evaluated. In five of the six studies, local or indigenous breeds were compared to unspecified commercial and cross breeds, whereas one study compared Vietnamese Duroc, Landrace, Yorkshire, and hybrid breeds. No significant association between sex and JEV seroprevalence was reported in any of the five studies where it was evaluated, two of which were conducted in feral swine populations (Table 5).
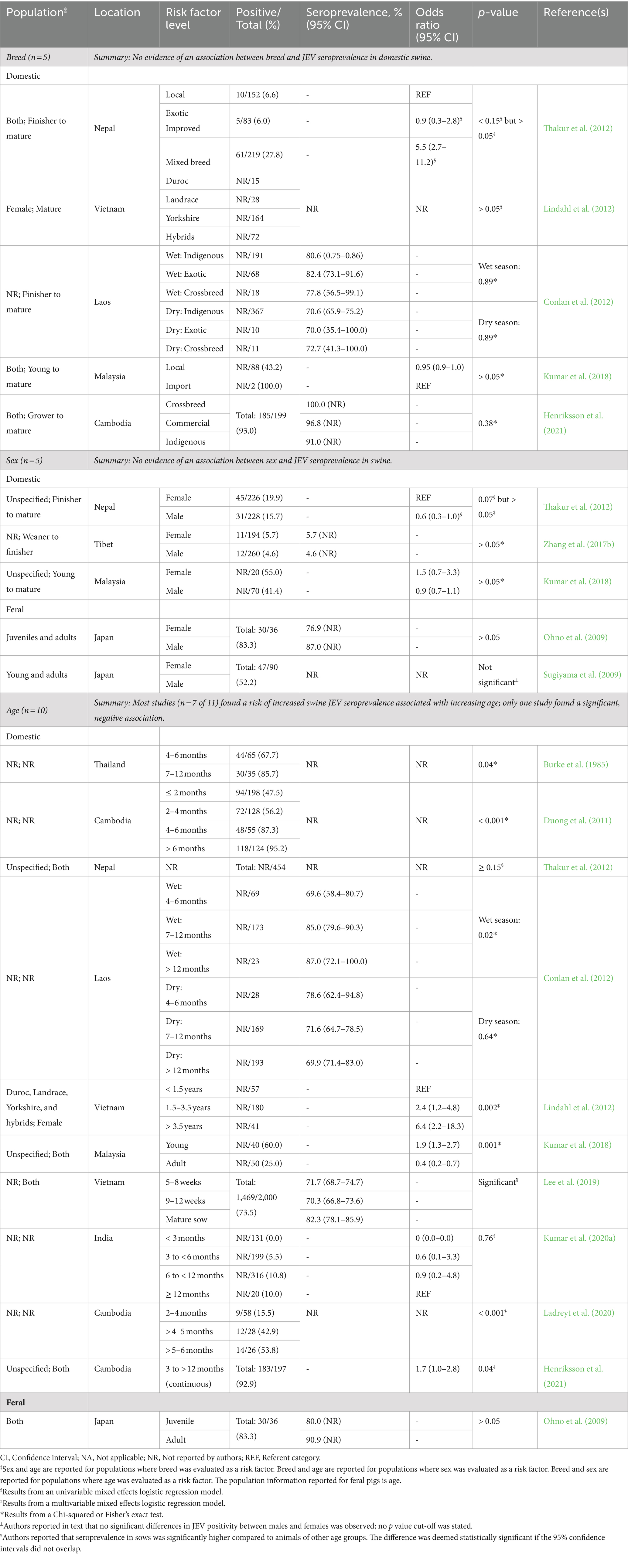
Table 5. Results from observational studies examining associations between population demographics (breed, sex, and age) and Japanese encephalitis virus (JEV) seropositivity in swine, including the number of reports that evaluated each risk factor, risk factor levels, population description, sample size, model-adjusted seroprevalence or odds ratio for JEV positivity, statistical significance of differences, and respective references.
Seven of 11 studies reported a risk of increased JEV seroprevalence with increasing age in domestic swine. Three studies compared groups of animals younger than 12 months, three compared animals younger than 12 months to animals 12 months and older, and one study evaluated age as a continuous variable (3 to >12 months; Henriksson et al., 2021). Alternatively, one study (Kumar et al., 2018) reported increased age as a protective factor for JEV seropositivity in domestic swine, comparing “young” and “adult” animals, defined by the authors as younger than 6 months and 6 months and older, respectively. One study (Ohno et al., 2009) evaluated the association of age and JEV seroprevalence in feral swine and reported no significant association. The age groups compared, “juvenile” and “adult,” were determined by animal weight, less than 50 kg and over 50 kg, respectively.
From our data synthesis, the associations between breed or age and JEV seroprevalence in swine are not knowledge gaps, although there are some specific caveats which limit external validity. Breed is not statistically associated with JEV seroprevalence in domestic swine when comparing local indigenous breeds versus imported breeds in JEV endemic areas. With limited studies (n = 5), the association between sex and JEV seroprevalence in feral and domestic swine remains inconclusive and therefore, a knowledge gap. Given the number of studies (n = 11) and consistency between them, we concluded that increased age is a risk factor for increased JEV seroprevalence in domestic swine in JEV endemic areas, however there are too few studies (n = 1) in feral swine to make any conclusions and this remains a knowledge gap. Reports on demographic risk factors in both domestic and feral swine focused solely on JEV seroprevalence. However, evaluating demographic risk factors using outcome measures that include a time component, such as incidence rate, remains necessary for a complete understanding of swine population susceptibility to JEV infection.
3.3.6.2 Swine operation risk factors
Only four reports included studies that statistically evaluated the association between farm-level risk factors and JEV seroprevalence in swine. The specific risk factors evaluated were: elevation, locality, proximity to a rice paddy, proximity to stagnant water (farm location), vehicular access, farm water source, animal source, toilet-related amenity, electricity in the home, piped water at home, waste management, feed type, swine housing type, size of operation, floor type, mosquito control (farm management, resources, and infrastructure), presence of mosquitoes, presence of ardeid birds, and presence of other animals (host and vector presence around the farm). Summaries of the associations between these risk factors and JEV seroprevalence in swine are reported in Table 6.
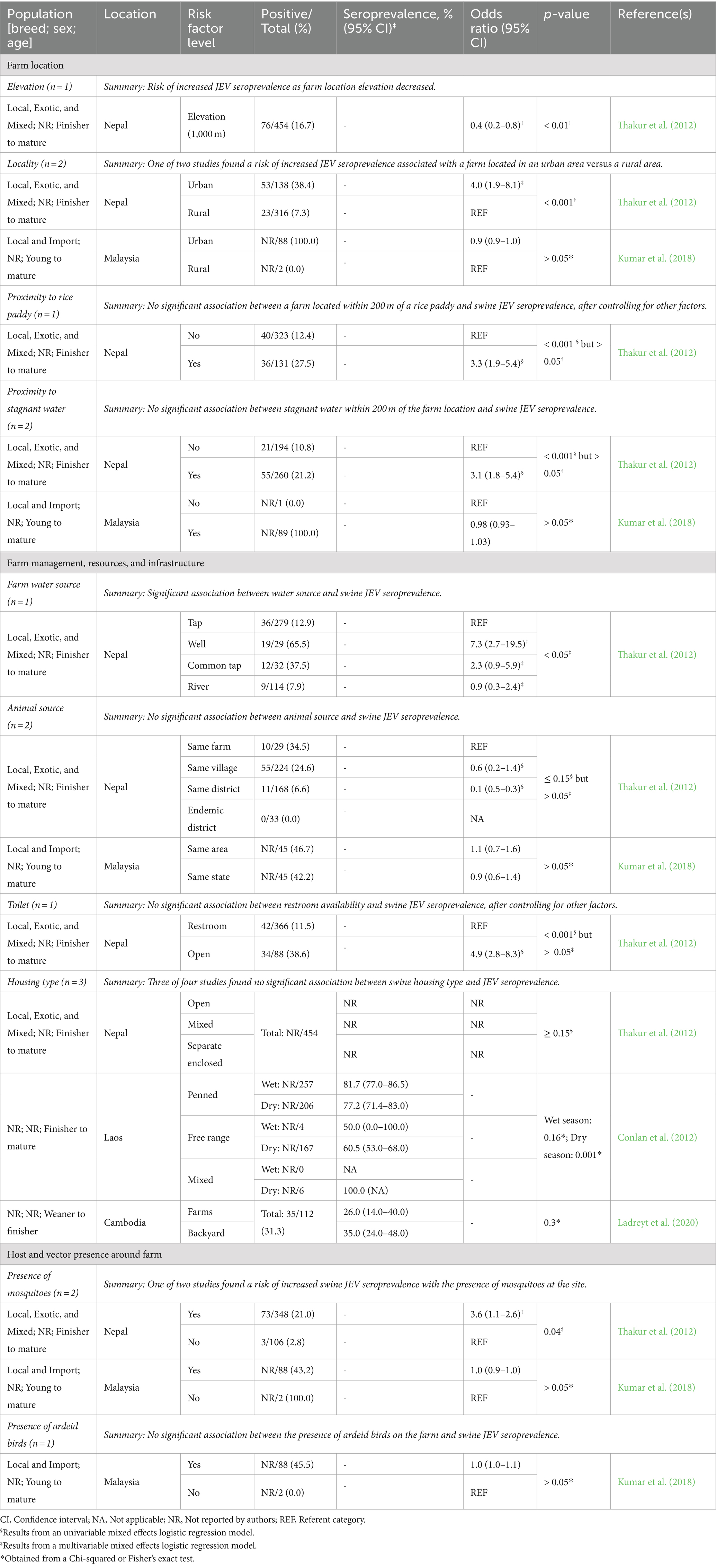
Table 6. Results from observational studies examining associations between farm location and management with Japanese encephalitis virus (JEV) seropositivity in domestic swine, including the number of reports that evaluated each risk factor, risk factor levels, population description, sample size, model-adjusted seroprevalence or odds ratio for JEV positivity, statistical significance of differences, and respective references.
Two reports (Thakur et al., 2012; Kumar et al., 2018) were responsible for most of the risk factor evaluations of farm location, farm management, resources, and infrastructure, and host and vector presence around the farm. The other two reports included studies evaluating housing types as a risk factor. Three of the four studies did not find a statistical association between swine housing type and JEV seroprevalence in swine (Table 6). Conlan et al. (2012) evaluated the association between housing type and JEV seroprevalence in the dry and wet seasons, reporting that during the dry season, free-range swine had lower JEV seroprevalence than penned swine. In addition to the associations reported in Table 6, Thakur et al. (2012) reported results on vehicular access, electricity in the home, piped water at home, waste management, swine feed type, size of operation, pen-floor type, mosquito control, and presence of other animals around the farm, but none were significantly associated with swine JEV seroprevalence in univariable models.
For all factors evaluated, the limited number of studies precludes general conclusions from being made. The sole risk factor with more than two studies, housing type, is relatively consistent, in that no significant association was found between housing type and JEV seroprevalence; however, the interaction of seasonality from the Conlan et al. (2012) studies and discrepancy between the types of housing utilized in Ladreyt et al. (2020) also limits the general conclusions that can be drawn from the broad category of housing type.
3.3.7 Surveillance
This review did not identify any reports evaluating the effectiveness of surveillance strategies (e.g., serological surveillance or systematic surveys of herds) for JEV detection in swine populations. Our search strategies and screening process would have precluded reports evaluating strategies that surveilled other species (i.e., mosquitoes) or other species used as sentinels to detect JEV presence/levels in swine populations. Although the lack of reports on the effectiveness of specific surveillance strategies in swine populations indicates a knowledge gap in this area for both domestic and feral swine in JEV endemic and non-endemic areas, a more specific search on surveillance strategies is needed to understand the scope of the gap.
3.3.8 Vaccine efficacy
Supplementary Table S7 describes the results of 19 reports evaluating the efficacy of 23 JEV vaccines in swine based on challenge studies. The results of the efficacious vaccines (n = 16), from 16 reports, are summarized in Table 7. The types of vaccines evaluated included live attenuated, killed, and other types such as recombinant, chimeric, and virus-like particles. Four reports described comparisons of the efficacy of multiple vaccine types, and a single report detailed the results of a passive immunization with monoclonal antibodies (Young et al., 2020).
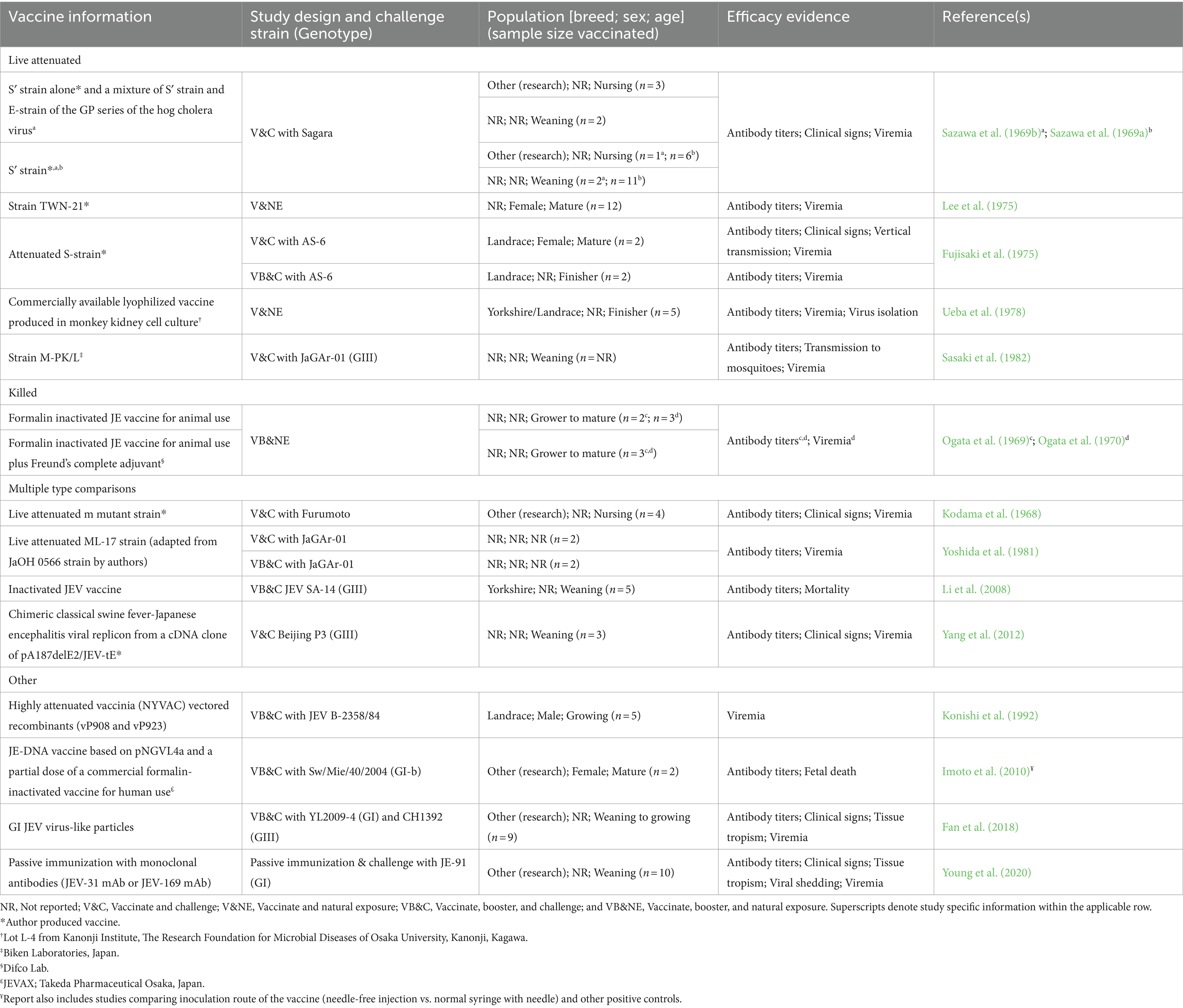
Table 7. Vaccine information, including strain and manufacturer, study design and challenge strain, population and sample size of vaccinated swine, evidence used to determine efficacy, and record information of efficacious vaccine studies for domestic swine organized by vaccine type (live attenuated, killed, multiple type comparisons, and other) and year of publication within vaccine type.
Six reports described challenges from natural exposure, while the remaining 13 challenges were conducted in a laboratory setting. The genotypes used for the laboratory challenges were GI (n = 3 reports), GIII (n = 6 reports), and unknown (n = 4 reports). Only two reports evaluated cross-protection between multiple genotypes. Fan et al. (2013) reported limited cross-protection of a vaccine derived from the GIII genotype against a GI challenge strain; Fan et al. (2018) reported that pigs vaccinated twice with a GI JEV virus-like particles vaccine had neutralizing antibodies against both GI and GIII JEV, with the GI antibody titer being almost 2x higher.
Sixteen vaccines were reported by the authors to be efficacious (live attenuated = 8, killed = 3, other = 5). Seven vaccines had inconclusive efficacy results (live attenuated = 2, killed = 3, other = 2), and one killed vaccine was reported as not efficacious. Fujisaki et al. (1975) found their live attenuated S- strain vaccine to be efficacious in finishing-aged Landrace pigs after vaccination and a booster, though its efficacy was inconclusive in finishing-aged Landrace and growing-aged research pigs after a single vaccination (no booster). However, they also reported that this vaccine was efficacious in mature, female, Landrace pigs after a single vaccination. Nine additional reports described the use of a JEV vaccine in swine, but failed to meet the inclusion criteria for the summary table, as the vaccinated pigs were not challenged with JEV (Sheng et al., 2016; De Wispelaere et al., 2015; Xu et al., 2011; Fei-fei et al., 2008; Xu et al., 2004; Nam et al., 2002; Konishi et al., 2000; Ogata et al., 1971; Yang et al., 2022). Authors in two of these reports indicated that disease challenge studies were not permitted at their institution (Sheng et al., 2016; De Wispelaere et al., 2015).
Commercially available vaccines were utilized in the studies from nine reports, but only five of these reports identified the vaccine manufacturer. Four reports indicated that the vaccine used was for veterinary use, though only Ueba et al. (1972) specified the manufacturer (tradename). Imoto et al. (2010) used a partial dose of a human-approved vaccine (JEVAX; Takeda Pharmaceutical Osaka, Japan), but no other tradenames were provided.
3.3.9 Basic reproduction ratio
Ten reports used mathematical equations and/or compartment models to describe JEV transmission and the results are summarized in Supplementary Table S8. However, only six of the 10 reports presented the basic reproduction ratio (R0) for JEV incorporated in swine as hosts, under specific modes of transmission (i.e., vector-borne and/or pig-to-pig) and different control strategies/scenarios (Table 8). All reports modeled vector-borne transmission routes; whereas only two reports included direct transmission (i.e., pig-to-pig), in addition to vector-borne transmission in their models, with the objective of modeling the contribution of direct transmission to the JEV epidemiological cycle in Cambodia (Diallo et al., 2018) or assessing the mechanism behind the JEV skip-and-resurgence patterns from 2003 to 2017 in Hong Kong (Zhao et al., 2018).
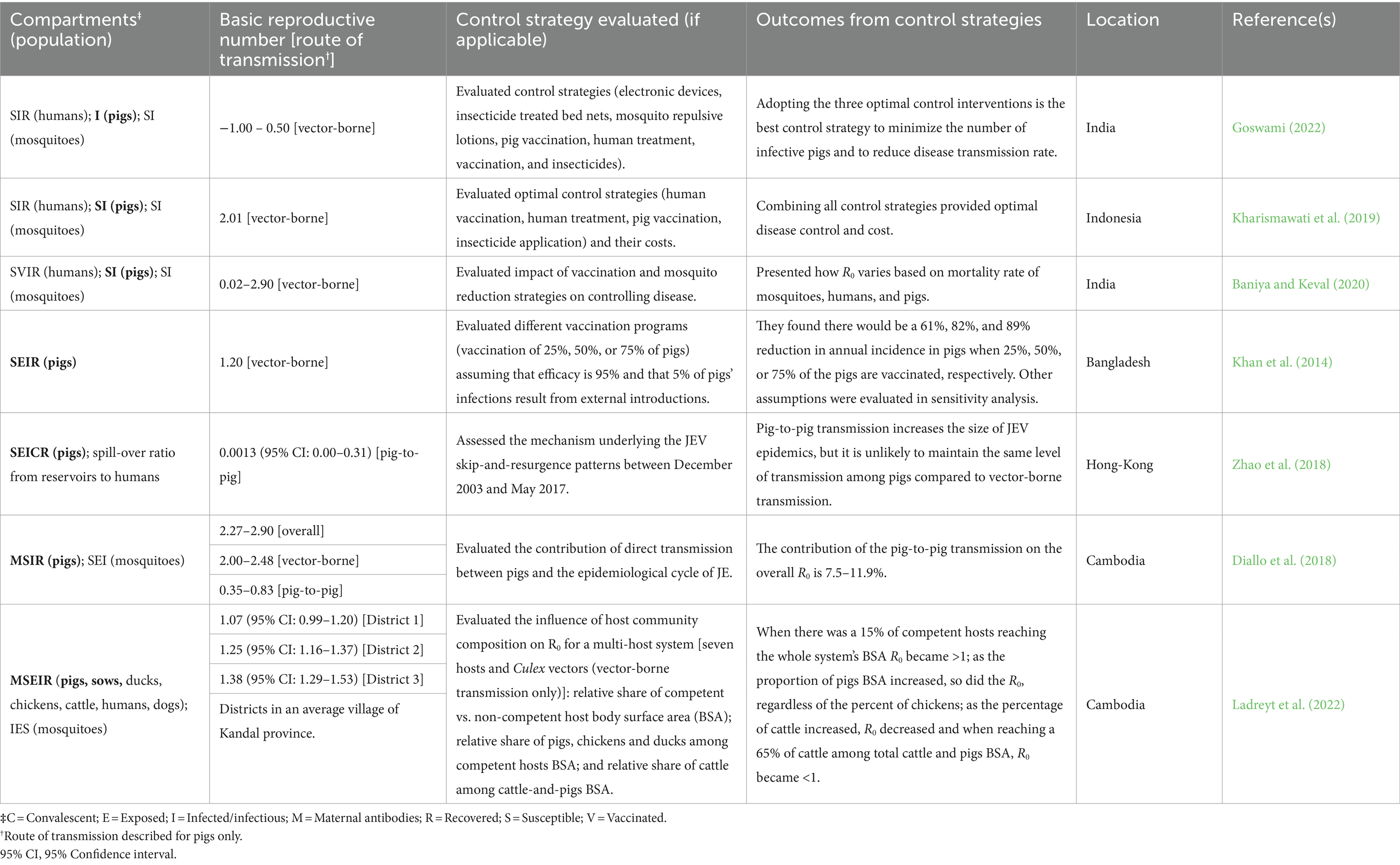
Table 8. Description of Japanese encephalitis virus (JEV) infectious disease models (compartments evaluated in pigs are highlighted in bold), control strategies evaluated, location and reported basic reproduction number.
Most of the reports (n = 5) evaluated different control strategies, primarily human and swine vaccination and mosquito control, with the goal of controlling disease in human populations. Only one report explicitly modeled the transmission of JEV in feral pigs using various bird migration parameters and mosquito vectorial capacities, however researchers did not report R0; this is the only report that modeled the transmission of JEV in the United States (Riad et al., 2017). All other reports modeled transmission in endemic or epidemic areas, specifically in Bangladesh, Cambodia, Hong Kong, India, Indonesia, and Japan.
For JEV R0 in domestic swine derived from vector-borne transmission scenarios, the range of effect sizes was very wide. However, given the specificity of R0 to the parameters and scenarios modeled, it is difficult to evaluate the consistency of these reports collectively. Yet, we were able to identify two knowledge gaps specific to JEV R0: a lack of reports evaluating R0 in domestic swine from direct transmission scenarios, and a lack of reports evaluating R0 in feral swine under any transmission scenarios.
3.3.10 Biosecurity
Only one study evaluating biosecurity measures was identified. Dutta et al. (2011) evaluated the effect of insecticide treated mosquito net placement (i.e., covering only human beds, only pig pens, human beds and pig pens, or no nets at all) on JEV seroconversion in mature domestic pigs. In this study authors reported that covering pig pens alone or both pig pens and human beds with insecticide treated mosquito nets significantly reduced the relative risk of JEV seroconversion in pigs. Given the single study on the use of insecticide treated mosquito nets as a biosecurity intervention, the use and efficacy of this and other biosecurity measures to reduce JEV transmission in domestic swine is a knowledge gap.
3.3.11 Pork products
No reports on JEV detection in processed pork or pork products, or transmission from raw by-products, were obtained through our search. The lack of literature evaluating or discussing the risk of JEV detection in, or transmission through processed pork or pork products is a critical knowledge gap for understanding introduction routes and economic impacts, when considering pork products derived from JEV-infected animals.
4 Discussion
In this review, we synthesized published literature on the role of domestic and feral swine in JEV transmission and identified knowledge gaps. Whereas swine have been questioned as key contributors to JEV amplification in some regions where their removal did not alter broader transmission dynamics (van den Hurk et al., 2008), their involvement in the JEV cycle remains critical due to their capacity to maintain high levels of viremia and the resulting socioeconomic implications of JEV outbreaks in swine herds. Analyzing the epidemiological features of JEV in the context of swine populations provides crucial insights into transmission dynamics that directly impact human health and economic stability. The unveiling of knowledge gaps from this synthesis can assist stakeholders with research prioritization, enhance preparedness, and develop countermeasures to mitigate the impact of JEV outbreaks on public health and the swine industry.
4.1 Transmission routes
Research has shown that JEV can be transmitted to pigs through either vertical or horizontal routes (both indirectly by mosquito vectors and potentially by direct contact). Transmission to swine primarily occurs after a pig is bitten by an infected mosquito. Although this vector-mediated transmission route (horizontal, indirect) of JEV is well described in the literature, and remains the most commonly reported means of transmission, evidence of direct vector-free transmission, described by Ricklin et al. (2016a), prompted a reevaluation of the role of swine in the JEV transmission cycle. Vertical transmission from infected sows to their offspring has been reported, although it is also considered a less common route compared to mosquito-borne transmission. Furthermore, despite the detection of JEV in swine semen (Ogasa et al., 1977; Teng et al., 2013; Wu et al., 2017; Xiao et al., 2018), neither experimental nor observational evaluation of JEV transmission through artificial insemination was described in any of the reports identified in this review.
Once infected, domestic and feral swine can serve as amplifying hosts, typically experiencing viral titers of 104 infectious units per mL, viremia levels described as sufficient for transmission to mosquito vectors (Scherer et al., 1959; Sasaki et al., 1982; Takashima et al., 1988; Imoto et al., 2010; cited by Ladreyt et al., 2019). The viremia period for JEV in pigs has been reported to start as soon as 1 day post infection (Ricklin et al., 2016a), and the duration of the viremia window varies depending on the route of infection, dose of inoculum, and the viral strain. In subcutaneously challenged pigs, viremia has been reported to last anywhere from 1 to 8 days (Sazawa et al., 1969a; Yoshida et al., 1981; Ricklin et al., 2016a; García-Nicolás et al., 2017) and from 2 to 4 days following natural infection (Williams et al., 2001). Evidence of differences in the length of viremia among different genotypes, which could provide a fitness advantage for transmission, was not directly evaluated during this review. An evaluation of the onset, duration, and magnitude of viral titers enabling a comparison among genotypes warrants a comprehensive meta-analysis.
Researchers have reported that JEV can remain detectable in the tonsils of swine for over 21 days post infection (Ricklin et al., 2016a, 2016b; Park et al., 2018; Redant et al., 2022), a period 5x longer than the average 4-day viremia period. However, the implications of this persistence in tonsils and the subsequent impact on transmission are poorly understood. Although JEV latency and reactivation has been demonstrated in mice (Mathur et al., 1986), this process has yet to be described regarding the persistent JEV in swine tonsils.
4.2 Pathological lesions, viral organotropism, and clinical signs
Japanese encephalitis virus infection can be clinically identified in swine herds when reproductive disorders are present in adults, including fetal abnormalities (mummified and stillborn fetuses) and abortions in sows, or by observing neurological signs in young animals. The similarity of JEV clinical manifestations to other endemic viruses, such as the porcine circovirus type 2 (PCV-2) or porcine reproductive and respiratory syndrome virus (PRRSV), presents a potential challenge for early disease detection in the event of a JEV incursion in the United States.
Despite reproductive clinical signs being well reported for sows, a lack of reports describing clinical signs in mature boars constitutes a knowledge gap in this area. Notably, JEV detection in the semen and “testicular fluid” of boars manifesting swollen testicles was reported in Teng et al. (2013) and Nie et al. (2022), respectively. Both studies used convenience samples based on abnormal clinical signs, and thus were not included in the clinical sign synthesis table of this review. However, these potential clinical signs warrant further investigation.
Our review added new evidence to the topic of macro- and microscopic lesions to what it has been previously summarized (Ladreyt et al., 2019) and compared differences in pathological lesions and viral organotropism within systems or organs, between JEV genotypes and study subject demographics; however, available published information was limited to genotypes I and III. Pathological lesions and organotropism were predominantly observed in the central nervous, lymphatic, and reproductive systems, with multiple other systems also affected. An especially intriguing aspect is the involvement of the olfactory bulb as a site for JEV infection. The potential for the olfactory bulb or other structures implicated in organotropic findings to serve as primary entry points into the central nervous system during non-vector transmission remains unclear, but it raises important questions about their role in altering the progression of the infection.
Although we did not identify any reports describing pathological lesions and organotropism of JEV in feral swine populations at the time of our search, a recent study conducted in Sinclair miniature pigs with a feral phenotype observed pathological outcomes consistent with those found in domestic swine after intradermal inoculation with genotype Ib (Park et al., 2023).
4.3 Diagnostic tests
Our review presents a detailed compilation of diagnostic tests evaluated or used for the detection of JEV in swine up to August 2022. Based on our findings, molecular tests, including RT-PCR assays, varied substantially in their diagnostic sensitivity and specificity estimates when detecting JEV in swine-derived samples. However, these assays exhibited high analytical sensitivity and specificity compared to alternatives such as viral antigen capture, or agglutination assays. When Williams et al. (2001) investigated the effect of prior exposure to Murray Valley encephalitis and Kunjin viruses on levels of neutralization and monoclonal antibodies against JEV, via microtiter serum neutralization test and a series of blocking enzyme-linked immunosorbent assay (B-ELISA) tests, serologic cross-reaction of swine antibodies was observed, leading the authors to conclude that these diagnostic tests were inadequate for JEV surveillance in areas where other related flaviviruses are present. More recently, Chan et al. (2022) recognized that the broad antigenic cross-reactivity of antibodies to flaviviruses, specifically dengue viruses, Zika virus, West Nile virus, and JEV, poses a challenge in interpreting serological results in regions where multiple flaviviruses are endemic. Despite these limitations among serological assays, including neutralization tests, enzyme-linked immunosorbent assay, hemagglutination-inhibition test, Western blot, and immunofluorescence test, neutralization tests are still considered the gold standard to differentiate these flaviviruses (Chan et al., 2022; World Organisation for Animal Health, 2023).
Currently, the proposed draft of the U.S. Disease Response Strategy: Japanese Encephalitis (United States Department of Agriculture - Animal and Plant Health Inspection Service (APHIS), 2023) references the intention to implement no-cost diagnostic testing for clinically affected animals as an initial outbreak response measure. The National Veterinary Services Laboratories, Foreign Animal Disease Diagnostic Laboratory, plans to use a reverse transcription-quantitative polymerase chain reaction (RT-qPCR) assay capable of detecting JEV genotypes I-IV, as well as sequencing methods for identification and confirmation of JEV in suspect samples. As the United States advances its strategies for disease response and preparedness concerning JEV, including surveillance and diagnostic testing infrastructure, it is imperative to address issues of cross-reactivity and test capacity. Furthermore, additional information regarding testing throughput and cost estimates would significantly benefit decision-makers as they develop response strategies and evaluate diagnostic alternatives. Portable, rapid diagnostic tests are becoming essential tools for quick, cost-effective, on-site diagnosis, particularly in remote or resource-limited settings (Mohsin et al., 2022). As diagnostic technologies advance, these portable assays can offer significant improvements in JEV surveillance. For instance, Roberts et al. (2022) developed a portable sandwich-type lateral flow assay for detecting JEV in pig serum on-site, whereas You et al. (2024) introduced a portable one-pot RPA-EsCas13d detection system for rapid field detection of JEV. These tools are especially valuable in swine farms, where early detection is crucial to prevent virus spread. To keep recommendations relevant and accurate, future reviews incorporating more recent advancements, particularly those published post-2022, are necessary to provide up-to-date guidance for JEV detection in both endemic and at-risk areas.
4.4 Seroprevalence
Serosurveys from endemic countries provide valuable insights into annual disease epidemic patterns. Ladreyt et al. (2019) reported a thorough overview of epidemiological patterns of JEV seroprevalence in swine, encompassing both domestic and feral populations, from 16 of the 25 countries where JEV has been identified. Although these data are specific to the climate and geographical regions of collection, their patterns can be studied and compared to those observed for other closely related flaviviruses present in the United States, such as WNV (Johnson et al., 2015; Kretschmer et al., 2023), to predict JEV behavior and prepare for an incursion. However, predictions based on data from past climate patterns may be unreliable in the face of ongoing climate changes and its effects on disease dynamics. To support reliable prediction, an up-to-date repository for surveillance data on these epidemiologically complex diseases would not only support research endeavors in monitoring endemic flaviviruses in the United States, but also facilitate contemporary understanding of mosquito population dynamics. This, in turn, would provide a foundation for the creation of efficient preparedness and intervention plans in the event of a JEV introduction to the United States.
4.5 Risk factors
4.5.1 Swine demographic risk factors
There was no evidence of a significant association between breed or sex with JEV seroprevalence. However, older pigs were consistently reported to have greater odds of being seropositive for JEV compared to younger animals. Based on seroprevalence alone, it is not possible to conclude whether this is due to a higher likelihood of exposure over their longer lifespan or an actual greater susceptibility to infection. This ambiguity is a result of utilizing JEV seroprevalence as the outcome measure, which describes a static state of seropositivity in a specific population but lacks the temporal aspect of disease onset. Seroprevalence was the primary outcome utilized in reports evaluating demographic risk factors in both domestic and feral swine. Incidence rate, often preferred for evaluating risk factors in epidemiological studies because it provides a time-specific measure of new cases in the population at risk, was not described in any of the reports included in this review regarding demographic risk factors (i.e., sex, breed, and age).
4.5.2 Swine operation risk factors
When investigating environmental risk factors for JEV seropositivity in swine, our review summarized available literature from regions where JEV is present. The small number of reports evaluating environmental risk factors limits our understanding of those risk factors to the specific swine production settings represented in these studies. Furthermore, the applicability or extrapolation of findings to the United States swine production system is limited due to the complex interaction of environmental factors impacting JEV infection risk for a particular geographic location.
Reports describing studies specifically designed to evaluate farm risk factors associated with JEV infection in swine herds were limited. Additionally, incomplete or inconsistent reporting of results, including statistical significance, and unclear reporting of comparison groups or study design hindered our ability to draw meaningful conclusions about these risk factors. Lee et al. (2020), Lindahl et al. (2013), and Di Francesco et al. (2018) reported outcome values descriptively rather than analytically. Zhou et al. (2019) focused on validating a new diagnostic test for detection of JEV in swine-derived samples, and although samples were collected from swine groups at farms with different production stages, results were not reported or compared among these groups. Yamanaka et al. (2010) aimed to evaluate the association of pig density on two islands with JEV seroprevalence in swine farms, however, sampling was performed during the rainy season on one island and the dry season on the other, biasing their estimations.
Furthermore, reports in which seroprevalence or related outcomes in swine populations located in areas with varying levels of JEV risk were evaluated often did not explicitly discuss or describe these intrinsic characteristics and differences among study groups; therefore, comparative outcomes could not be extracted. Consequently, these reports were not included in the synthesis process.
Lastly, of the swine operation risk factors included in our synthesis, most were evaluated in only one study, except for swine housing type, which was evaluated by two different studies. The four reports summarized in this knowledge synthesis used a proportion of pigs that were JEV seropositive out of the total number of pigs at risk of JEV exposure as the outcome measure, which, as previously discussed, only depicts a static state of disease in a particular study population. Additional studies investigating the association between environmental risk factors and JEV infection in swine, via seroprevalence or other outcome measures, would not only strengthen the body of evidence supporting previously identified farm-level risk factors, but also help identify additional risk factors relevant to other production systems.
4.6 Surveillance
Given the recent expansion of JE’s geographic range throughout the Australian mainland, the World Health Organization (WHO) has recognized the need for strengthened surveillance efforts to assess the disease burden (World Organisation for Animal Health, 2022). Effective surveillance systems are crucial for timely detection of diseases, maximizing the efficiency of response efforts in resource-constrained environments. However, no reports evaluating the effectiveness of surveillance strategies in relation to swine, were identified in this review. Because swine species are considered amplifying hosts for JEV, their use as sentinels is not ideal for indicating the presence of JEV activity. High cost, ambiguous serology caused by cross-reacting antibodies to other flaviviruses, occupational risk associated with swine blood sampling, and increased public health risk of JEV transmission when sentinel pigs become viremic were highlighted as major disadvantages for the use of sentinel pigs (Ritchie et al., 2007). Testing feral swine during the hunting season has been also proposed as a monitoring strategy for JEV endemic areas, though the effectiveness of this approach has not been demonstrated (Nidaira et al., 2014).
Reports evaluating strategies in other species, and their potential effectiveness, although not covered in this review, are available. Mackenzie et al. (2022) described the JEV surveillance strategies in mainland Australia, prior to JEV GIV emergence and spread, which ultimately failed to provide early evidence of JEV activity. These surveillance measures included testing of sentinel chickens and field-caught mosquitoes, combined with predictive modeling of climatic conditions (Mackenzie et al., 2022). Furthermore, although Mackenzie et al. (2022) did not address the effectiveness of surveillance systems, previous literature was contrasted to present a comprehensive discussion of the pros and cons regarding the effectiveness and costs of swine-alternative species—such as chickens, cattle, goats, dogs, and field-caught mosquitoes—for use in animal sentinel systems. Monath (2023) also discussed the recent JEV outbreak in Australia and speculated that JEV had likely spread southwards undetected in birds, feral pigs, and potentially bat populations for months before being detected in commercial pig farms located in southern Australia. A similar scenario could occur in other countries suitable for an incursion and lacking an active JEV surveillance system. Experts suggest that should a JEV epidemic occur in the United States, as it did in mainland Australia, abortion storms in swine could serve as the initial indicator of the disease. However, due to the clinical resemblance to other endemic swine diseases, recognition of a foreign animal disease may be delayed. Diagnostic challenges for a similar flaviviral incursion occurred during the emergence of West Nile virus (WNV) in the United States, resulting in delayed diagnosis and subsequent nationwide spread. Crucially, the introduction of WNV underscored the need for having robust surveillance and response systems in place to effectively manage emerging mosquito-vectored flaviviral diseases.
Currently, there is no active surveillance program for Japanese Encephalitis (JE) in the United States Environmental surveillance strategies exist for West Nile Virus (WNV) and include mosquito trapping and testing, live bird serology, and testing of horses and other vertebrate hosts. Specifically, the national arboviral surveillance system (ArboNET), managed by the Centers for Disease Control and Prevention [Center for Disease Control and Prevention (CDC), 2022], detects seasonal epidemics of WNV. State health departments primarily report human cases, but ArboNET also collects data on arboviral infections from mosquitoes, dead birds, and sentinel animals. Despite its utility, ArboNET has significant limitations due to passive reporting and inconsistent surveillance investment among states, resulting in incomplete data, delayed reports, and underreporting. Since JEV and WNV are transmitted by similar mosquito species and infect comparable hosts, integrating JEV surveillance into existing WNV activities could be feasible but would face the same challenges if reliant on ArboNET.
4.7 Vaccine efficacy
Vaccines exist locally and are used in swine vaccination programs in JEV-endemic countries, including Japan, Korea, Nepal, Taiwan, and China, to protect sows from reproductive disorders. Most vaccines, inactivated and live-attenuated, are derived from cell cultures and are based on GIII, raising concerns about efficacy against other genotypes, such as IV and V. Vaccinating the breeding stock before the mosquito season starts can control viral amplification and reproductive disease in swine. The high turnover of market pig populations, maternal antibody interference, short time window for administration, genotype shifts, and logistic and budgetary constraints, may explain the low vaccination uptake and the slow progress in the development of JEV veterinary vaccines (Williams et al., 2019). Furthermore, pig immunization, along with mosquito mitigation, and human immunization and treatment have been evaluated using mathematical compartmental models. While each control measure contributed toward the reduction of JEV transmission, in most models, vaccination alone (of humans or pigs) was sub-optimally effective in reducing the number of infected pigs and thereby decreasing transmission (De et al., 2016; Kharismawati et al., 2019; Goswami, 2022).
While our review offers a comprehensive summary of the available information regarding efficacy of swine vaccines, a significant gap exists regarding the accessibility and licensure status of these vaccines for swine. There are currently no approved JEV vaccines for use in swine in the United States (United States Department of Agriculture - Animal and Plant Health Inspection Service (APHIS), 2023). Therefore, it seems imperative for the United States to have a plan for the rapid approval of swine vaccines in case of a JEV incursion. In its current draft form (United States Department of Agriculture - Animal and Plant Health Inspection Service (APHIS), 2023), the disease response strategy for JE states “if JE was identified in the U.S., APHIS would investigate availability of acceptable vaccines, potentially involving emergency approval of vaccines being used in other countries.” Identifying effective vaccines that can prevent JEV infection or impede transmission among swine, adopting specific emergency approval procedures, and securing emergency supply contracts, are critical for the responsible authorities to establish a rapid response in the event of a JEV outbreak.
4.8 Basic reproduction ratio
A key epidemiological metric used to describe transmissibility of infectious diseases, the basic reproduction ratio (R0), represents the average number of secondary infections produced by a single infected individual in a completely susceptible population. Values for R0 greater than one can lead to an epidemic as on average each infected host will infect more than one other host, whereas with R0 less than one, the disease will decline and die out in the population. Most studies reported R0 estimates for vector-borne transmission greater than one but ranged between −1.00 and 2.90. When pig-to-pig transmission was considered, R0 was less than one, ranging from 0.001 to 0.83, indicating that direct transmission alone is insufficient to cause an epidemic. In Diallo et al. (2018), the direct transmission among swine populations accounted for up to 11.9% of the overall R0. While it may contribute to the epidemic’s magnitude, it is unlikely to sustain transmission among pigs as effectively as the vector-borne route (Zhao et al., 2018). Thus far, these findings support the conclusion that mosquito-mediated transmission remains the most efficient method for disease spread.
Previously overlooked in prior reviews, this parameter provides crucial information for modeling the transmission and spread of JEV in the United States. However, R0 is not a fixed value and is dependent on factors modeled, such as host availability, mosquito abundance and survival, biting rates, and the incubation period of the virus within the mosquito vector. Given the limited number of models and their geographical/management settings (i.e., Bangladesh, Cambodia, India, Indonesia, and Hong Kong), more work needs to be done in this area to expand the usability of these models to other areas.
4.9 Biosecurity
In addition to vaccination, the implementation of biosecurity measures can contribute to the prevention of viral exposure and infection. Only one report evaluating biosecurity measures, specifically the use of insecticide-treated mosquito nets in pig pens, was identified and included in this review (Dutta et al., 2011). The scarcity of information regarding the evaluation of biosecurity interventions may be attributed to the swine-specific search terms used in this review. Consequently, unless the records explicitly referenced swine, they were not considered, potentially overlooking strategies only targeting mosquito vectors. This lack of information hinders the formulation of evidence-based recommendations on key biosecurity measures, including but not limited to vector control, quarantine, the safe introduction of replacement animals, and low-risk movements of people, animals, and vehicles within swine production systems. Other conventional biosecurity strategies typically employed in commercial swine production systems, such as air filtration, cleaning and disinfection of barns, vehicles, and fomites, as well as perimeter fencing, remain to be evaluated in the context of JEV transmission.
Current biosecurity recommendations for United States swine farms prioritize the prevention of endemic, and some foreign animal diseases like African Swine Fever, Classical Swine Fever, and Foot and Mouth Disease (Secure Pork Supply, 2017). These practices address the primary transmission pathways for those diseases, which include direct contact, mechanical vectors (fomites), aerosols, and contaminated feed. While these programs encompass a range of strategies, including environmental management, the establishment of physical barriers, and the application of chemical and biological controls, to mitigate the risk of disease transmission via insect vectors and wildlife, the recommendations may not be sufficiently robust to prevent diseases where mosquito vectors are the primary route of transmission (Secure Pork Supply, 2017; Alarcón et al., 2021).
4.10 Pork products and other disease impacts
To date, no import or export restrictions have been imposed on JEV positive herds or countries. Recently, messaging from Australia has emphasized that JE is not a food safety concern (Business Queensland, 2023). This messaging is also included in the preliminary draft of the United States Disease Response Strategy for JEV (United States Department of Agriculture - Animal and Plant Health Inspection Service (APHIS), 2023). However, our search yielded no reports pertaining to the safety of processed pork and pork by-products to provide definitive support for these statements. The poor environmental stability described for JEV and other related flaviviruses, such as Zika virus, which are sensitive to UV and gamma radiation, heat, extreme pH, and various chemical disinfectants (Müller et al., 2016; Williams et al., 2019; World Organisation for Animal Health, 2019) suggests that JEV is unlikely to survive in pork products. However, publishing literature addressing the safety of pork products originating from JEV-endemic regions is pivotal for informing public health and food safety policies and recommendations.
Although not initially among our primary research questions, economic impact was identified by experts and stakeholders as a critical outcome of interest. However, given its indirect relationship to JEV transmission, our overarching theme, we deemed it to be a secondary topic of interest. While production losses associated with abortion in sows and infertility in boars, and additional costs related to control and prevention for JEV are expected, studies quantifying the economic impact of JEV introduction into swine production systems are scarce. Although, no peer-reviewed reports evaluating the economic impact of JEV within the context of swine production systems were identified in this review, a recently published economic assessment exploring the potential impacts of a JEV incursion in the United States reported that an estimated 32% of the total United States breeding herd (2,135,940 sows) would be impacted, resulting in a 1 to 2% reduction in United States pork production output, and an estimated economic loss to the United States pork industry ranging between USD 306 million and USD 612 million (Hayes et al., 2024).
4.11 Study design and limitations
Systematic reviews, which are designed to minimize bias, employ explicit methods for conducting a comprehensive literature search, assessing eligibility, and critically appraising study quality. Scoping reviews map the literature by extent, time range, and nature of existing research on a particular topic. This review combined traits from both systematic and scoping reviews to comprehensively explore overall themes pertaining to the role of swine in the transmission of JEV and its effects on swine production systems, particularly focusing on the U.S. From our synthesis, we identified knowledge gaps in the understanding of the role of swine in JEV transmission. These knowledge gaps can inform United States research initiatives needed to improve our understanding of mechanisms of JEV introduction, transmission, and disease impacts, and aid in the development of preparedness efforts for a potential JEV incursion in the United States.
By design, rapid systematic reviews are a modified version of traditional systematic reviews. They incorporate methods to expedite the review process without compromising its systematicity, reproducibility, and transparency. By creating a protocol a priori and thoroughly documenting our methods using the PRISMA-P 2015 (Moher et al., 2015) and PRISMA 2020 (Page et al., 2021) checklists, we ensured a reproducible and transparent methodology. However, the necessary streamlining of a rapid process due to logistical and time constraints may result in some relevant studies being excluded. For example, as we only captured articles written in English, relevant literature, considering the Asian origin and distribution of this disease, was likely excluded. This limitation may have led to a biased selection of literature included for this review. Acknowledging this risk, the benefits of a systematic review completed in a shorter timeframe are more valuable to policymakers and stakeholders needing to make evidence-based decisions.
5 Conclusion
Pigs have a central role in the transmission cycle of JEV. They not only sustain transmission and facilitate spillover to other hosts by producing sufficient viremia to infect mosquito vectors, but they also can display clinical signs of reproductive and neurological disease. This review provides insights into the disease process dynamics, detection methods, control and preventive options, and potential impacts in the swine production system.
Most of the evidence suggests that domestic pigs may be pivotal in the transmission of JEV, while the relative importance of feral swine remains unclear. Research in feral swine is imperative for informing policymaking and the development of strategic initiatives aimed at mitigating JEV spread and surveilling viral activity. The estimated 6 million feral swine present in 35 U.S. states could serve as efficient hosts of JEV, should it be introduced. Improved knowledge regarding the dynamics of feral swine populations in the United States and their potential role in the spread and establishment of JEV, would be invaluable for assessing the risk posed to commercial swine operations located in various regions across the country.
Given the importance of the swine industry to the United States economy, a JEV incursion would cause a significant impact to this sector, considering the susceptible immune status of the United States pig population. Previous exposure to other flaviviruses (e.g., WNV) may confer some level of cross-protection to United States swine herds, however, the extent of this protection and its impact on JEV’s basic reproduction ratio is not known. In the event of a JEV incursion in the United States, mathematical models will likely be used to inform policy decisions regarding its control, highlighting the importance of understanding dynamics of disease transmission and spread.
Having access to information for making evidence-based decisions is crucial for minimizing significant potential impacts on animal health and the economy within the swine sector. Once information is gathered, providing education to and encouraging communication between government officials, veterinarians, academics, and swine producers becomes critical. The consideration of existing evidence aids in the identification of research priorities by highlighting important areas where missing or inadequate information limits the ability of a researcher to reach a conclusion and make an evidence-based decision. This is particularly crucial in the event of an outbreak in the United States, where preemptive measures can help minimize the spread of the virus, safeguard both human and animal populations, and ensure the long-term sustainability of the swine production sector.
Data availability statement
The original contributions presented in the study are included in the article/Supplementary material, further inquiries can be directed to the corresponding author.
Author contributions
VH: Writing – review & editing, Writing – original draft, Visualization, Validation, Methodology, Investigation, Formal analysis, Data curation, Project administration, Conceptualization. CH: Writing – review & editing, Writing – original draft, Visualization, Validation, Methodology, Investigation, Formal analysis, Data curation, Conceptualization. AD: Writing – review & editing, Writing – original draft, Visualization, Validation, Methodology, Investigation, Formal analysis, Data curation, Conceptualization. SE: Writing – review & editing, Investigation. AT: Writing – review & editing, Investigation. DM: Writing – review & editing, Methodology, Investigation, Funding acquisition. LN: Writing – review & editing, Methodology, Investigation, Funding acquisition. LC: Writing – review & editing, Funding acquisition. VB: Writing – review & editing, Methodology, Resources. CM: Writing – review & editing, Funding acquisition. NC: Writing – review & editing, Writing – original draft, Visualization, Validation, Methodology, Investigation, Formal analysis, Project administration, Supervision, Funding acquisition, Conceptualization.
Funding
The author(s) declare that financial support was received for the research, authorship, and/or publication of this article. Financial support was provided by the Swine Health Information Center (SHIC Project ID 22-070), the Center for Outcomes Research and Epidemiology, and the College of Veterinary Medicine at Kansas State University.
Acknowledgments
We would like to express our gratitude to the following individuals for their contributions to this research including Madison Evje (ME), Taylor McAtee (TM), Shakirat Adetunji, John Ekakoro, and David Edache.
Conflict of interest
The authors declare that the research was conducted in the absence of any commercial or financial relationships that could be construed as a potential conflict of interest.
Publisher’s note
All claims expressed in this article are solely those of the authors and do not necessarily represent those of their affiliated organizations, or those of the publisher, the editors and the reviewers. Any product that may be evaluated in this article, or claim that may be made by its manufacturer, is not guaranteed or endorsed by the publisher.
Supplementary material
The Supplementary material for this article can be found online at: https://www.frontiersin.org/articles/10.3389/fsufs.2024.1455455/full#supplementary-material
References
Alarcón, L. V., Allepuz, A., and Mateu, E. (2021). Biosecurity in pig farms: a review. Porci. Health Manag. 7:5. doi: 10.1186/s40813-020-00181-z
Baniya, V., and Keval, R. (2020). The influence of vaccination on the control of JE with a standard incidence rate of mosquitoes, pigs and humans. J. Appl. Math. Comput. 64, 519–550. doi: 10.1007/s12190
Bhattacharya, S., and Basu, P. (2014). Japanese encephalitis virus (JEV) infection in different vertebrates and its epidemiological significance: a review. Int. J. Fauna Biol. Stud. 1, 32–37.
Brown, V. R., Miller, R. S., Pepin, K. M., Carlisle, K. M., Cook, M. A., Vanicek, C. F., et al. (2024). African swine fever at the wildlife-livestock interface: challenges for management and outbreak response within invasive wild pigs in the United States. Front. Vet. Sci. 11:1348123. doi: 10.3389/fvets.2024.1348123
Burke, D. S., Tingpalapong, M., Ward, G. S., Andre, R., and Leake, C. J. (1985). Intense transmission of Japanese encephalitis virus to pigs in a region free of epidemic encephalitis. The Southeast Asian J. Trop. Med. Public Health. 16, 199–206.
Burns, K. F. (1950). Congenital Japanese B encephalitis infection of swine. Proc. Soc. Exp. Biol. Med. 75, 621–625. doi: 10.3181/00379727-75-18285
Business Queensland (2023). Japanese encephalitis. Available online at: https://www.business.qld.gov.au/industries/farms-fishing-forestry/agriculture/biosecurity/animals/diseases/guide/japanese-encephalitis (Accessed March 12, 2024).
Campbell, G. L., Hills, S. L., Fischer, M., Jacobson, J. A., Hoke, C. H., Hombach, J. M., et al. (2011). Estimated global incidence of Japanese encephalitis: a systematic review. Bull. World Health Organ. 89, 766–774. doi: 10.2471/BLT.10.085233
Cao, Q. S., Li, X. M., Zhu, Q. Y., Wang, D. D., Chen, H. C., and Qian, P. (2011). Isolation and molecular characterization of genotype I Japanese encephalitis virus, SX09S-01, from pigs in China. Virol. J. 8, 1–9. doi: 10.1186/1743-422X-8-472
Cappelle, J., Duong, V., Pring, L., Kong, L., Yakovleff, M., Prasetyo, D. B., et al. (2016). Intensive circulation of Japanese encephalitis virus in peri-urban sentinel pigs near Phnom Penh, Cambodia. PLoS Negl. Trop. Dis. 10:e0005149. doi: 10.1371/journal.pntd.0005149
Center for Disease Control and Prevention (CDC) (2022). Environmental Surveillance. Available online at: https://www.cdc.gov/mosquitoes/guidelines/west-nile/surveillance/environmental-surveillance.html (Accessed March 18, 2024).
Cha, G. W., Lee, E. J., Lim, E. J., Sin, K. S., Park, W. W., Jeon, D. Y., et al. (2015). A novel immunochromatographic test applied to a serological survey of Japanese encephalitis virus on pig farms in Korea. Plos one. 10. doi: 10.1371/journal.pone.0127313
Chan, K. R., Ismail, A. A., Thergarajan, G., Raju, C. S., Yam, H. C., Rishya, M., et al. (2022). Serological cross-reactivity among common flaviviruses. Front. Cell. Infect. Microbiol. 12:975398. doi: 10.3389/fcimb.2022.975398
Chang, H. C., Takashima, I., Arikawa, J., and Hashimoto, N. (1984a). Biotin-labeled antigen sandwich enzyme-linked immunosorbent assay (BLA-S-ELISA) for the detection of Japanese encephalitis antibody in human and a variety of animal sera. J. Immunol. Methods. 72, 401–409. doi: 10.1016/0022-1759(84)90008-5
Chang, H. C., Takashima, I., Arikawa, J., and Hashimoto, N. (1984b). Biotin-labeled protein-A enzyme-linked immunosorbent assay for the detection of Japanese encephalitis antibody in sera from humans, swine and several animal species. J. Virol. Methods. 9, 143–151. doi: 10.1016/0166-0934(84)90006-5
Chauhan, J., Dhanze, H., Kumar, H. B., Kumar, M. S., and Bhilegaonkar, K. N. (2020). Development of dipstick enzyme linked immunosorbent assay for on-site sero-diagnosis of Japanese encephalitis in swine. J. Virol. Methods. 280:113876. doi: 10.1016/j.jviromet.2020.113876
Chen, H. Y., Wei, Z. Y., Zhang, H. Y., Lü, X. L., Zheng, L. L., Cui, B. A., et al. (2010). Use of a multiplex RT-PCR assay for simultaneous detection of the North American genotype porcine reproductive and respiratory syndrome virus, swine influenza virus and Japanese encephalitis virus. Agric. Sci. China. 9, 1050–1057. doi: 10.1016/S1671-2927(09)60189-9
Conlan, J.V., Vongxay, K., and Jarman, R.G., Gibbons, R.V, Lunt, R.A., Fenwick, S., Thompson, R.A., and Blacksell, S.D. (2012). Serologic study of pig-associated viral zoonoses in Laos. Am. J. Trop. Med. Hyg. 86, 1077–1084. doi: 10.4269/ajtmh.2012.11-0195
Di Francesco, J., Choeung, R., Peng, B., Pring, L., Pang, S., Duboz, R., et al. (2018). Comparison of the dynamics of Japanese encephalitis virus circulation in sentinel pigs between a rural and a peri-urban setting in Cambodia. PLoS Negl. Trop. Dis. 12:e0006644. doi: 10.1371/journal.pntd.0006644
De, A., Maity, K., Jana, S., and Maiti, M. (2016). Application of various control strategies to Japanese encephalitic: a mathematical study with human, pig and mosquito. Math. Biosci. 282, 46–60. doi: 10.1016/j.mbs.2016.09.015
De Wispelaere, M., Ricklin, M., Souque, P., Frenkiel, M.-P., Paulous, S., Garcìa-Nicolàs, O., et al. (2015). A lentiviral vector expressing Japanese encephalitis virus-like particles elicits broad neutralizing antibody response in pigs. PLoS Negl. Trop. Dis. 9:e0004081. doi: 10.1371/journal.pntd.0004081
Desingu, P. A., Ray, P. K., Patel, B. H. M. M., Singh, R., Singh, R. K., and Saikumar, G. (2016). Pathogenic and genotypic characterization of a Japanese encephalitis virus isolate associated with reproductive failure in an Indian pig herd. PLoS One 11:e0147611. doi: 10.1371/journal.pone.0147611
Dhanze, H., Bhilegaonkar, K. N., Kumar, C., Kumar, M. S., Singh, P., and Kumar, A. (2020a). Development and evaluation of lateral flow assay for sero-diagnosis of Japanese encephalitis in swine. Anim. Biotechnol. 31, 350–356. doi: 10.1080/10495398.2019.1602539
Dhanze, H., Bhilegaonkar, K. N., Ravi Kumar, G. V. P. P. S., Thomas, P., Chethan Kumar, H. B., Suman Kumar, M., et al. (2015). Comparative evaluation of nucleic acid-based assays for detection of Japanese encephalitis virus in swine blood samples. Arch. Virol. 160, 1259–1266. doi: 10.1007/s00705-015-2385-3
Dhanze, H., Bhilegaonkar, K. N., Rawat, S., Chethan Kumar, H. B., Kumar, A., and Gulati, B. R. (2019). Development of recombinant nonstructural 1 protein based indirect enzyme linked immunosorbent assay for sero-surveillance of Japanese encephalitis in swine. J. Virol. Methods. 272:113705. doi: 10.1016/j.jviromet.2019.113705
Dhanze, H., Kumar, M. S., Singh, V., Gupta, M., Bhilegaonkar, K. N., Kumar, A., et al. (2020b). Detection of recent infection of Japanese encephalitis virus in swine population using IgM ELISA: a suitable sentinel to predict infection in humans. J. Immunol. Methods 486:112848. doi: 10.1016/j.jim.2020.112848
Diallo, A. O. I. I., Chevalier, V., Cappelle, J., Duong, V., Fontenille, D., and Duboz, R. (2018). How much does direct transmission between pigs contribute to Japanese encephalitis virus circulation? A modelling approach in Cambodia. PLoS One 13:e0201209. doi: 10.1371/journal.pone.0201209
Duong, V., Sorn, S., Holl, D., Rani, M., Deubel, V., and Buchy, P. (2011). Evidence of Japanese encephalitis virus infections in swine populations in 8 provinces of Cambodia: implications for national Japanese encephalitis vaccination policy. Acta tropica, 120, 146–150. doi: 10.1016/j.actatropica.2011.07.0
Dutta, P., Khan, S. A., Khan, A. M., Borah, J., Sarmah, C. K., and Mahanta, J. (2011). The effect of insecticide-treated mosquito nets (ITMNs) on Japanese encephalitis virus seroconversion in pigs and humans. Am. J. Trop. Med. Hyg. 84, 466–472. doi: 10.4269/ajtmh.2011.10-0270
Fan, Y.-C., Chen, J.-M., Chen, Y.-Y., Lin, J.-W., and Chiou, S.-S. (2013). Reduced neutralizing antibody titer against genotype I virus in swine immunized with a live-attenuated genotype III Japanese encephalitis virus vaccine. Vet. Microbiol. 163, 248–256. doi: 10.1016/j.vetmic.2013.01.017
Fan, Y.-C., Chen, J.-M., Lin, J.-W., Chen, Y.-Y., Wu, G.-H., Su, K.-H., et al. (2018). Genotype I of Japanese encephalitis virus virus-like particles elicit sterilizing immunity against genotype I and III viral challenge in swine. Sci. Rep. 8:7481. doi: 10.1038/s41598-018-25596-1
Fan, Y.-C., Liang, J.-J., Chen, J.-M., Lin, J.-W., Chen, Y.-Y., Su, K.-H., et al. (2019). NS2B/NS3 mutations enhance the infectivity of genotype I Japanese encephalitis virus in amplifying hosts. PLoS Pathog. 15:e1007992. doi: 10.1371/journal.ppat.1007992
Fan, J.-M., Luo, J., Chen, L., Teng, M., Bu, D., Wang, F.-Y., et al. (2010a). Genetic analysis of strains of Japanese encephalitis virus isolated from swine in Central China. Virus Genes 40, 357–361. doi: 10.1007/s11262-010-0464-9
Fan, Y.-C., Chen, Y.-Y., Chen, J.-M., Huang, C., Huang, M., and Chiou, S.-S. (2022). Effectiveness of live-attenuated genotype III Japanese encephalitis viral vaccine against circulating genotype I viruses in swine. Viruses, 14:114. doi: 10.3390/v14010114
Fei-fei, G., Jian, W., Feng, X., Li-ping, S., Quan-yun, S., Jin-ping, Z., et al. (2008). Japanese encephalitis protein vaccine candidates expressing neutralizing epitope and M.T hsp70 induce virus-specific memory B cells and long-lasting antibodies in swine. Vaccine 26, 5590–5594. doi: 10.1016/j.vaccine.2008.07.104
Fujisaki, Y., Sugimori, T., Morimoto, T., Miura, Y., and Kawakami, Y. (1975). Immunization of pigs with the attenuated S-strain of Japanese encephalitis virus. Natl. Inst. Anim. Health Q. 15, 55–60.
García-Nicolás, O., Ricklin, M., Liniger, M., Vielle, N., Python, S., Souque, P., et al. (2017). A Japanese encephalitis virus vaccine inducing antibodies strongly enhancing in vitro infection is protective in pigs. Viruses 9:124. doi: 10.3390/v9050124
Garritty, C., Gartlehner, G., Nussbaumer-Streit, B., King, V. J., Hamel, C., Kamel, C., et al. (2021). Cochrane rapid reviews methods group offers evidence-informed guidance to conduct rapid reviews. J. Clin. Epidemiol. 130, 13–22. doi: 10.1016/j.jclinepi.2020.10.007
Goswami, N. K. (2022). Sensitivity and optimal control analysis of Japanese encephalitis disease: a mathematical model. Adv. Syst. Sci. Appl. 22, 148–166. doi: 10.25728/assa.2022.22.1.1180
Grace, M. R., Chauhan, J., Suman Kumar, M., Kumar, A., Bhilegaonkar, K. N., Singh, M., et al. (2019b). Evaluation of carboxyl beads based latex agglutination test for rapid sero-diagnosis of Japanese encephalitis. Biologicals, 62, 72–76. doi: 10.1016/j.biologicals.2019.09.003
Grace, M. R., Dhanze, H., Pantwane, P., Sivakumar, M., Gulati, B., and Kumar, A. (2019a). Latex agglutination test for rapid on-site serodiagnosis of Japanese encephalitis in pigs using recombinant NS1 antigen. J. Vector Borne Dis. 56, 105–110. doi: 10.4103/0972-9062.263717
Guo, H.-C., Ren, Z.-W., Ding, M.-M., Xiao, W.-J., Peng, P., He, B., et al. (2019). Serologic and molecular survey for major viral pathogens in grazing hybrid wild boars in Northeast China. J. Integr. Agric. 18, 2133–2140. doi: 10.1016/S2095-3119(19)62650-X
Hayes, C.H., Myer, D., Weaver, M., and Wagstrom, L. (2024). Potential impacts of introduction and establishment of Japanese encephalitis virus in the United States swine herd. Available online at: https://www.swinehealth.org/march-2024-shic-enewsletter/#three (Accessed March 19, 2024).
Henriksson, E., Söderberg, R., Ström Hallenberg, G., Kroesna, K., Ly, S., Sear, B., et al. (2021). Japanese encephalitis in small-scale pig farming in rural Cambodia: pig seroprevalence and farmer awareness. Pathogens 10:578. doi: 10.3390/pathogens10050578
Higgins, D. A. (1970). A serological survey of pigs in Hong Kong for antibodies to Japanese encephalitis virus. Trop. Anim. Health Prod. 2, 23–27. doi: 10.1007/BF02359325
Imoto, J.-I., Ishikawa, T., Yamanaka, A., Konishi, M., Murakami, K., Shibahara, T., et al. (2010). Needle-free jet injection of small doses of Japanese encephalitis DNA and inactivated vaccine mixture induces neutralizing antibodies in miniature pigs and protects against fetal death and mummification in pregnant sows. Vaccine 28, 7373–7380. doi: 10.1016/j.vaccine.2010.09.008
Jayatilleke, K. (2020). Challenges in implementing surveillance tools of high-income countries (HICs) in low middle income countries (LMICs). Curr. Treat. Options Infect. Dis. 12, 191–201. doi: 10.1007/s40506-020-00229-2
Johnson, M. G., Adams, J., McDonald-Hamm, C., Wendelboe, A., and Bradley, K. K. (2015). Seasonality and survival associated with three outbreak seasons of West Nile virus disease in Oklahoma—2003, 2007, and 2012. J. Med. Virol. 87, 1633–1640. doi: 10.1002/jmv.24235
Khan, S. U., Salje, H., Hannan, A., Islam, M. A., Bhuyan, A. A. M., Rahman, M. Z., et al. (2014). Dynamics of Japanese encephalitis virus transmission among pigs in Northwest Bangladesh and the potential impact of pig vaccination. PLoS Negl. Trop. Dis. 8:e3166. doi: 10.1371/journal.pntd.0003166
Kharismawati, H., Fatmawati,, and Windarto,. (2019). Optimal control of a mathematical model for Japanese encephalitis transmission. J. Phys. 1306:012034. doi: 10.1088/1742-6596/1306/1/012034
Kliegman, R. M., Stanton, B. F., Geme, J. S., and Schor, N. F. (2015). Nelson Textbook of Pediatrics, 2-Volume. 20th edition. Philadelphia (PA): Elsevier.
Kodama, K., Sasaki, N., and Inoue, Y. K. (1968). Studies of live attenuated Japanese encephalitis vaccine in swine. J. Immunol. 100, 194–200. doi: 10.4049/jimmunol.100.1.194
Kolhe, R. P., Bhilegaonkar, K. N., Dubbal, Z. B., Kaur, S., Das, S., and Agarwal, R. K. (2015). Indirect ELISA for serosurveillance of Japanese encephalitis in pigs. Indian J. Anim. Res. 49, 343–349. doi: 10.5958/0976-0555.2015.00065.5
Konishi, E., Pincus, S., Paoletti, E., Laegreid, W. W., Shope, R. E., and Mason, P. W. (1992). A highly attenuated host range-restricted vaccinia virus strain, NYVAC, encoding the prM, E, and NS1 genes of Japanese encephalitis virus prevents JEV viremia in swine. Virology 190, 454–458. doi: 10.1016/0042-6822(92)91233-K
Konishi, E., and Yamaoka, M. (1982). Enzyme-linked immunosorbent assay for detection of antibodies to Japanese encephalitis virus in swine sera. Kobe J. Med. Sci. 28, 7–17.
Konishi, E., and Yamaoka, M. (1983). Rapid enzyme-linked immunosorbent assay of whole blood for detection of antibodies to Japanese encephalitis virus. J. Virol. Methods 7, 21–28. doi: 10.1016/0166-0934(83)90019-8
Konishi, E., Yamaoka, M., Kurane, I., and Mason, P. W. (2000). Japanese encephalitis DNA vaccine candidates expressing premembrane and envelope genes induce virus-specific memory B cells and long-lasting antibodies in swine. Virology 268, 49–55. doi: 10.1006/viro.1999.0142
Kretschmer, M., Ruberto, I., Townsend, J., Zabel, K., Will, J., Maldonado, K., et al. (2023). Unprecedented outbreak of West Nile virus—Maricopa County, Arizona, 2021. MMWR Morb. Mortal Wkly. Rep. 72, 452–457. doi: 10.15585/mmwr.mm7217a1
Kumar, K., Arshad, S. S., Selvarajah, G. T., Abu, J., Toung, O. P., Abba, Y., et al. (2018). Prevalence and risk factors of Japanese encephalitis virus (JEV) in livestock and companion animal in high-risk areas in Malaysia. Trop. Anim. Health Prod. 50, 741–752. doi: 10.1007/s11250-017-1490-6
Kumar, H. B. C., Dhanze, H., Bhilegaonkar, K. N., Chakurkar, E. B., Kumar, A., and Yathish, H. M. (2020a). Reply to commentary on serological evidence of Japanese encephalitis virus infection in pigs in a low human incidence state, Goa, India. Prev. Vet. Med. 177:104945. doi: 10.1016/j.prevetmed.2020.104945
Kumar, R., Mathur, A., Kumar, A., Sharma, S., Chakraborty, S., and Chaturvedi, U. C. (1990). Clinical features & prognostic indicators of Japanese encephalitis in children in Lucknow (India). Indian J. Med. Res. 91, 321–327
Ladreyt, H., Auerswald, H., Tum, S., Ken, S., Heng, L., In, S., et al. (2020). Comparison of Japanese encephalitis force of infection in pigs, poultry and dogs in Cambodian villages. Pathogens 9:719. doi: 10.3390/pathogens9090719
Ladreyt, H., Chevalier, V., and Durand, B. (2022). Modelling Japanese encephalitis virus transmission dynamics and human exposure in a Cambodian rural multi-host system. PLoS Negl. Trop. Dis. 16:e0010572. doi: 10.1371/journal.pntd.0010572
Ladreyt, H., Durand, B., Dussart, P., and Chevalier, V. (2019). How central is the domestic pig in the epidemiological cycle of Japanese encephalitis virus? A review of scientific evidence and implications for disease control. Viruses 11:949. doi: 10.3390/v11100949
Le Flohic, G., Porphyre, V., Barbazan, P., and Gonzalez, J. P. (2013). Review of climate, landscape, and viral genetics as drivers of the Japanese encephalitis virus ecology. PLoS Negl. Trop. Dis. 7:e2208. doi: 10.1371/journal.pntd.0002208
Lee, H. S., Bui, V. N., Nguyen, H. X., Bui, A. N., Hoang, T. D., Nguyen-Viet, H., et al. (2020). Seroprevalences of multi-pathogen and description of farm movement in pigs in two provinces in Vietnam. BMC Vet. Res. 16:15. doi: 10.1186/s12917-020-2236-7
Lee, G. C.-Y., Huang, Y.-T., and Chang, L.-C. (1975). Preliminary study of attenuated Japanese encephalitis virus. J. Formos. Med. Assoc. 74, 606–612.
Lee, H. S., Thanh, T. L., Ly, N. K., Nguyen-Viet, H., Thakur, K. K., and Grace, D. (2019). Seroprevalence of leptospirosis and Japanese encephalitis in swine in ten provinces of Vietnam. PLoS One 14:e0214701. doi: 10.1371/journal.pone.0214701
Li, Y., Hou, L., Ye, J., Liu, X., Dan, H., Jin, M., et al. (2010). Development of a convenient immunochromatographic strip for the diagnosis of infection with Japanese encephalitis virus in swine. J. Virol. Methods 168, 51–56. doi: 10.1016/j.jviromet.2010.04.015
Li, P., Zheng, Q. S., Wang, Q., Li, Y., Wang, E. X., Liu, J. J., et al. (2008). Immune responses of recombinant adenoviruses expressing immunodominant epitopes against Japanese encephalitis virus. Vaccine 26, 5802–5807. doi: 10.1016/j.vaccine.2008.08.035
Lindahl, J., Boqvist, S., Ståhl, K., Thu, H. T. V., and Magnusson, U. (2012). Reproductive performance in sows in relation to Japanese encephalitis virus seropositivity in an endemic area. Trop. Anim. Health Prod. 44, 239–245. doi: 10.1007/s11250-011-0005-0
Lindahl, J. F., Ståhl, K., Chirico, J., Boqvist, S., Thu, H. T. V., and Magnusson, U. (2013). Circulation of Japanese encephalitis virus in pigs and mosquito vectors within Can Tho city, Vietnam. PLoS Negl. Trop. Dis. 7:e2153. doi: 10.1371/journal.pntd.0002153
Liu, H., Lu, H. J., Liu, Z. J., Jing, J., Ren, J. Q., Liu, Y. Y., et al. (2012). Reverse transcription loop-mediated isothermal amplification for rapid detection of Japanese encephalitis virus in swine and mosquitoes. Vector Borne Zoo. Dis. 12, 3861–3869. doi: 10.3923/javaa.2012.3861.3869
Lopez, A. L., Aldaba, J. G., Roque, V. G. Jr., Tandoc, A. O. III, Sy, A. K., Espino, F. E., et al. (2015). Epidemiology of Japanese encephalitis in the Philippines: a systematic review. PLoS Negl. Trop. Dis. 9:e0003630. doi: 10.1371/journal.pntd.0003630
Mackenzie, J. S., Williams, D. T., Van Den Hurk, A. F., Smith, D. W., and Currie, B. J. (2022). Japanese encephalitis virus: the emergence of genotype IV in Australia and its potential endemicity. Viruses 14:2480. doi: 10.3390/v14112480
Mathur, A., Arora, K. L., Rawat, S., and Chaturvedi, U. C. (1986). Persistence, latency and reactivation of Japanese encephalitis virus infection in mice. J. Gen. Virol. 67, 381–385. doi: 10.1099/0022-1317-67-2-381
Meiklejohn, G., Simpson, T. W., and Stacy, I. B. (1947). Experimental infection of domestic animals with Japanese B encephalitis virus. Proc. Soc. Exp. Biol. Med. 65, 359–364. doi: 10.3181/00379727-65-15959
Moher, D., Shamseer, L., Clarke, M., Ghersi, D., Liberati, A., Petticrew, M., et al. (2015). Preferred reporting items for systematic review and meta-analysis protocols (PRISMA-P) 2015 statement. Syst. Rev. 4, 1–9. doi: 10.1186/2046-4053-4-1
Mohsin, F., Suleman, S., Anzar, N., Narang, J., and Wadhwa, S. (2022). A review on Japanese encephalitis virus emergence, pathogenesis and detection: from conventional diagnostics to emerging rapid detection techniques. Int. J. Biol. Macromol. 217, 435–448. doi: 10.1016/j.ijbiomac.2022.07.027
Monath, T. P. (2023). Japanese encephalitis: risk of emergence in the United States and the resulting impact. Viruses 16:54. doi: 10.3390/v16010054
Müller, J. A., Harms, M., Schubert, A., Jansen, S., Michel, D., Mertens, T., et al. (2016). Inactivation and environmental stability of Zika virus. Emerg. Infect. Dis. 22, 1685–1687. doi: 10.3201/eid2209.160664
Nam, J.-H., Chae, S.-L., and Cho, H.-W. (2002). Immunogenicity of a recombinant MVA and a DNA vaccine for Japanese encephalitis virus in swine. Microbiol. Immunol. 46, 23–28. doi: 10.1111/j.1348-0421.2002.tb02672.x
Nidaira, M., Kyan, H., Taira, K., Okano, S., Oshiro, T., Kato, T., et al. (2014). Survey of Japanese encephalitis virus in pigs and wild boars on Ishigaki and Iriomote islands in Okinawa, Japan. Epidemiol. Infect. 142, 856–860. doi: 10.1017/S0950268813001611
Nie, M., Zhou, Y., Li, F., Deng, H., Zhao, M., Huang, Y., et al. (2022). Epidemiological investigation of swine Japanese encephalitis virus based on RT-RAA detection method. Sci. Rep. 12:9392. doi: 10.1038/s41598-022-13604-4
Ogasa, A., Yokoki, Y., Fujisaki, Y., and Habu, A. (1977). Reproductive disorders in boars infected experimentally with Japanese encephalitis virus. Jpn. J. Anim. Reprod. 23, 171–175.
Ogata, M., Nagao, Y., Jitsunari, F., Kikui, R., and Kitamura, N. (1970). Vaccination with complete adjuvant-added inactivated virus vaccine of Japanese encephalitis to swine for preventing viremia (with specific reference to the effect of vaccination on viremia; epidemiological study on Japanese encephalitis. 35). Acta Med. Okayama 24, 579–587.
Ogata, M., Nagao, Y., Jitsunari, F., and Kitamura, N. (1969). Vaccination with complete adjuvant-added inactivated virus vaccine of Japanese encephalitis to swine, rabbits and chicks for preventing viremia (epidemiological study on Japanese encephalitis 25). Acta Med. Okayama 23, 541–551
Ogata, M., Nagao, Y., Kikui, R., Kitamura, N., and Ariyasu, H. (1971). Effect of complete adjuvant on vaccination of live attenuated Japanese encephalitis vaccine to swine for preventing viremia. 39. Epidemiological studies of JE. Acta Med. Okayama 25, 71–75
Ohkubo, Y., Takashima, I., Hashimoto, N., and Fujita, I. (1984). Enzyme-linked immunosorbent assay (ELISA) for detection of IgM and IgG antibodies to Japanese encephalitis virus in sera from experimentally infected swine. Jpn. J. Vet. Sci. 46, 57–64. doi: 10.1292/jvms1939.46.57
Ohno, Y., Sato, H., Suzuki, K., Yokoyama, M., Uni, S., Shibasaki, T., et al. (2009). Detection of antibodies against Japanese encephalitis virus in raccoons, raccoon dogs and wild boars in Japan. J. Vet. Med. Sci. 71, 1035–1039. doi: 10.1292/jvms.71.1035
Oliveira, A. R. S., Cohnstaedt, L. W., Strathe, E., Etcheverry, L., McVey, D. S., Piaggio, J., et al. (2018a). Meta-analyses of Japanese encephalitis virus infection, dissemination, and transmission rates in vectors. Am. J. Trop. Med. Hygiene 98, 883–890. doi: 10.4269/ajtmh.17-0622
Oliveira, A. R. S., Cohnstaedt, L. W., Strathe, E., Hernández, L. E., McVey, D. S., Piaggio, J., et al. (2017). Meta-analyses of the proportion of Japanese encephalitis virus infection in vectors and vertebrate hosts. Parasit. Vectors 10, 418–415. doi: 10.1186/s13071-017-2354-7
Oliveira, A. R. S., Strathe, E., Etcheverry, L., Cohnstaedt, L. W., McVey, D. S., Piaggio, J., et al. (2018b). Assessment of data on vector and host competence for Japanese encephalitis virus: a systematic review of the literature. Prev. Vet. Med. 154, 71–89. doi: 10.1016/j.prevetmed.2018.03.018
Page, M. J., Moher, D., Bossuyt, P. M., Boutron, I., Hoffmann, T. C., Mulrow, C. D., et al. (2021). The PRISMA 2020 statement: an updated guideline for reporting systematic reviews. BMJ 372. doi: 10.1136/bmj.n71
Pantawane, P. B., Dhanze, H., Ravi Kumar, G. V. P. P. S., Grace, M. R., Dudhe, N. C., and Bhilegaonkar, K. N. (2019). TaqMan real-time RT-PCR assay for detecting Japanese encephalitis virus in swine blood samples and mosquitoes. Anim. Biotechnol. 30, 267–272. doi: 10.1080/10495398.2018.1481417
Park, S. L., Huang, Y. J. S., Lyons, A. C., Ayers, V. B., Hettenbach, S. M., McVey, D. S., et al. (2018). North American domestic pigs are susceptible to experimental infection with Japanese encephalitis virus. Sci. Rep. 8:7951. doi: 10.1038/s41598-018-26208-8
Park, S. L., Huang, Y. S., Lyons, A. C., Ayers, V. B., Hettenbach, S. M., McVey, D. S., et al. (2023). Infection of feral phenotype swine with Japanese encephalitis virus. Vector Borne Zoo. Dis. 23, 645–652. doi: 10.1089/vbz.2023.0030
Park, S. L., Huang, Y. S., and Vanlandingham, D. L. (2022). Re-examining the importance of pigs in the transmission of Japanese encephalitis virus. Pathogens 11:575. doi: 10.3390/pathogens11050575
Quan, T. M., Thao, T. T. N., Duy, N. M., Nhat, T. M., and Clapham, H. (2020). Estimates of the global burden of Japanese encephalitis and the impact of vaccination from 2000-2015. eLife 9:e51027. doi: 10.7554/eLife.51027
Rao, P., Wu, H., Jiang, Y., Opriessnig, T., Zheng, X., Mo, Y., et al. (2014). Development of an EvaGreen-based multiplex real-time PCR assay with melting curve analysis for simultaneous detection and differentiation of six viral pathogens of porcine reproductive and respiratory disorder. J. Virol. Methods 208, 56–62. doi: 10.1016/j.jviromet.2014.06.027
Redant, V., Favoreel, H. W., Dallmeier, K., Van Campe, W., and De Regge, N. (2020). Efficient control of Japanese encephalitis virus in the central nervous system of infected pigs occurs in the absence of a pronounced inflammatory immune response. J. Neuroinflammation 17:315. doi: 10.1186/s12974-020-01974-3
Redant, V., Favoreel, H. W., Dallmeier, K., Van Campe, W., and De Regge, N. (2022). Japanese encephalitis virus persistence in porcine tonsils is associated with a weak induction of the innate immune response, an absence of IFNγ mRNA expression, and a decreased frequency of CD4+CD8+ double-positive T cells. Front. Cell. Infect. Microbiol. 12:834888. doi: 10.3389/fcimb.2022.834888
Riad, M. H., Scoglio, C. M., McVey, D. S., and Cohnstaedt, L. W. (2017). An individual-level network model for a hypothetical outbreak of Japanese encephalitis in the USA. Stoch. Env. Res. Risk A. 31, 353–367. doi: 10.1007/s00477-016-1353-0
Ricklin, M. E., García-Nicolás, O., Brechbühl, D., Python, S., Zumkehr, B., Nougairede, A., et al. (2016a). Vector-free transmission and persistence of Japanese encephalitis virus in pigs. Nat. Commun. 7:10832. doi: 10.1038/ncomms10832
Ricklin, M. E., Garcìa-Nicolàs, O., Brechbühl, D., Python, S., Zumkehr, B., Posthaus, H., et al. (2016b). Japanese encephalitis virus tropism in experimentally infected pigs. Vet. Res. 47:34. doi: 10.1186/s13567-016-0319-z
Ritchie, S. A., Van Den Hurk, A. F., Zborowski, P., Kerlin, T. J., Banks, D., Walker, J. A., et al. (2007). Operational trials of remote mosquito trap systems for Japanese encephalitis virus surveillance in the Torres Strait, Australia. Vector Borne Zoo. Dis. 7, 497–506. doi: 10.1089/vbz.2006.0643
Roberts, A., Prakashan, D., Dhanze, H., Gandham, R. K., Gandhi, S., and Sharma, G. T. (2022). Immuno-chromatic probe based lateral flow assay for point-of-care detection of Japanese encephalitis virus NS1 protein biomarker in clinical samples using a smartphone-based approach. Nanoscale Adv. 4, 3966–3977. doi: 10.1039/d2na00463a
Robinson, K.A., Akinyede, O., Dutta, T., Sawin, V.I., Li, T., Spencer, M.R., et al. (2013). Framework for determining research gaps during systematic review: evaluation. Agency for Healthcare Research and Quality (US), Rockville (MD).
Sasaki, O., Karoji, Y., Kuroda, A., Karaki, T., Takenokuma, K., and Maeda, O. (1982). Protection of pigs against mosquito-borne Japanese encephalitis-virus by immunization with a live attenuated vaccine. Antivir. Res. 2, 355–360. doi: 10.1016/0166-3542(82)90005-5
Sazawa, H., Sugimori, T., Morimoto, T., and Miura, Y. (1969b). Antibody response in piglets by simultaneous inoculation with attenuated viruses of Japanese encephalitis and hog cholera. Natl. Inst. Anim. Health Q. 9, 112–113
Sazawa, H., Sugimori, T., Morimoto, T., Miura, Y., and Watanabe, M. (1969a). Response of swine to an attenuated strain of Japanese encephalitis virus obtained by passage in bovine kidney cell cultures. Natl. Inst. Anim. Health Q. 9, 74–82
Scherer, W. F., Moyer, J. T., and Izumi, T. (1959). Immunologic studies of Japanese encephalitis virus in Japan. V. Maternal antibodies, antibody responses and viremia following infection of swine. J. Immunol. 83, 620–626. doi: 10.4049/jimmunol.83.6.620
Secure Pork Supply (2017). Biosecurity manuals. Information manual for enhanced biosecurity for pork producers: Animals raised indoors. Section 9. Available online at: www.securepork.org/Resources/SPS_Biosecurity_Information_Manual_IndoorAnimals.pdf (Accessed March 26, 2024).
Sheng, Z., Gao, N., Cui, X., Fan, D., Chen, H., Wu, N., et al. (2016). Electroporation enhances protective immune response of a DNA vaccine against Japanese encephalitis in mice and pigs. Vaccine 34, 5751–5757. doi: 10.1016/j.vaccine.2016.10.001
Shimizu, T., Kawakami, Y., Fukuhara, S., and Matumoto, M. (1954). Experimental stillbirth in pregnant swine infected with Japanese encephalitis virus. Jpn. J. Exp. Med. 24, 363–375.
Sugiyama, I., Shimizu, E., Nogami, S., Suzuki, K., Miura, Y., and Sentsui, H. (2009). Serological survey of arthropod-borne viruses among wild boars in Japan. J. Vet. Med. Sci. 71, 1059–1061. doi: 10.1292/jvms.71.1059
Suresh, K. P., Nayak, A., Dhanze, H., Bhavya, A. P., Shivamallu, C., Achar, R. R., et al. (2022). Prevalence of Japanese encephalitis (JE) virus in mosquitoes and animals of the Asian continent: a systematic review and meta-analysis. J. Infect. Public Health 15, 942–949. doi: 10.1016/j.jiph.2022.07.010
Takashima, I., Watanabe, T., Ouchi, N., and Hashimoto, N. (1988). Ecological studies of Japanese encephalitis virus in Hokkaido: interepidemic outbreaks of swine abortion and evidence for the virus to overwinter locally. Am. J. Trop. Med. Hyg. 38, 420–427. doi: 10.4269/ajtmh.1988.38.420
Teng, M., Luo, J., Fan, J. M., Chen, L., Wang, X. T., Yao, W., et al. (2013). Molecular characterization of Japanese encephalitis viruses circulating in pigs and mosquitoes on pig farms in the Chinese province of Henan. Virus Genes 46, 170–174. doi: 10.1007/s11262-012-0813-y
Thakur, K. K., Pant, G. R., Wang, L., Hill, C. A., Pogranichniy, R. M., Manandhar, S., et al. (2012). Seroprevalence of Japanese encephalitis virus and risk factors associated with seropositivity in pigs in four mountain districts in Nepal. Zoonoses Public Health 59, 393–400. doi: 10.1111/j.1863-2378.2012.01456.x
Tian, C. J., Lin, Z. X., He, X. M., Luo, Q., Luo, C. B., Yu, H. Q., et al. (2012). Development of a fluorescent-intercalating-dye-based reverse transcription loop-mediated isothermal amplification assay for rapid detection of seasonal Japanese B encephalitis outbreaks in pigs. Arch. Virol. 157, 1481–1488. doi: 10.1007/s00705-012-1330-y
Ueba, N., Kimura, T., Nakajima, S., Kurimura, T., and Kitaura, T. (1978). Field experiments on live attenuated Japanese encephalitis virus vaccine for swine. Biken J. 21, 95–103.
Ueba, N., Maeda, A., Otsu, K., Mitsuda, B., Kimoto, T., Fujito, S., et al. (1972). Natural infections of swine by Japanese encephalitis-virus and its modification by vaccination. Biken J. 15, 67–79
United States Department of Agriculture - Animal and Plant Health Inspection Service (APHIS). (2023). Disease response strategy: Japanese encephalitis. Available online at: https://www.aphis.usda.gov/animal_health/downloads/draft-disease-strategy.pdf (Accessed March 12, 2024)
United States Department of Agriculture—Economic Research Service (ERS) (2024). Hogs & Pork. Available online at: https://www.ers.usda.gov/topics/animal-products/hogs-pork/ (Accessed February 15, 2024).
United States Department of Agriculture. (n.d.). Feral swine bomb. USDA Climate Hubs. [online]. Available at: https://www.climatehubs.usda.gov/hubs/southwest/topic/feral-swine-bomb [Accessed 18 November 2024].
van den Hurk, A. F., Ritchie, S. A., Johansen, C. A., MacKenzie, J. S., and Smith, G. A. (2008). Domestic pigs and Japanese encephalitis virus infection, Australia. Emerg. Infect. Dis. 14, 1736–1738. doi: 10.3201/eid1411.071368
Veloso, V.D.A., Hanthorn, C.J., Dixon, A.L., and Cernicchiaro, N. (2023). Revisiting the role of swine on the risk of Japanese encephalitis virus (JEV) transmission in the United States: A rapid systematic review of the literature.
Wang, X., Guo, S., Hameed, M., Zhang, J., Pang, L., Li, B., et al. (2020). Rapid differential detection of genotype I and III Japanese encephalitis virus from clinical samples by a novel duplex TaqMan probe-based RT-qPCR assay. J. Virol. Methods 279:113841. doi: 10.1016/j.jviromet.2020.113841
Wildlife Health Australia. (2022). WHA fact sheet on Japanese encephalitis. Available at: https://wildlifehealthaustralia.com.au/Incidents/Incident-Information/wildlifehealthaustralia.com.au/Portals/0/ResourceCentre/FactSheets/PublicHealth/Japanese_Encephalitis.pdf [Accessed August 18, 2022].
Williams, D. T., Daniels, P. W., Lunt, R. A., Wang, L. F., Newberry, M. K., and Mackenzie, J. S. (2001). Experimental infections of pigs with Japanese encephalitis virus and closely related Australian flaviviruses. Am. J. Trop. Med. Hyg. 65, 379–387. doi: 10.4269/ajtmh.2001.65.379
Williams, D. T., MacKenzie, J. S., and Bingham, J. (2019). Flaviviruses. Dis. Swine, 530–543. doi: 10.1002/9781119350927.ch33
World Health Organization. (2006). Japanese encephalitis vaccines. Weekly Epidemiological Record, 81, 331–340.
World Organisation for Animal Health (2019). Japanese Encephalitis Technical Disease Card. Available online at: https://www.woah.org/app/uploads/2021/03/japanese-encephalitis.pdf (Accessed April 09, 2024).
World Organisation for Animal Health. (2022) Disease outbreak news; Japanese Encephalitis—Australia. Available online at: https://www.who.int/emergencies/disease-outbreak-news/item/2022-DON365 (Accessed May 18, 2024).
World Organisation for Animal Health (2023). “Japanese encephalitis” in Manual of Diagnostic Test and Vaccines for Terrestrial Animals. 12th ed.
Wu, X., Lin, H., Chen, S., Xiao, L., Yang, M., An, W., et al. (2017). Development and application of a reverse transcriptase droplet digital PCR (RT-ddPCR) for sensitive and rapid detection of Japanese encephalitis virus. J. Virol. Methods 248, 166–171. doi: 10.1016/j.jviromet.2017.06.015
Wu, H., Rao, P., Jiang, Y., Opriessnig, T., and Yang, Z. (2014). A sensitive multiplex real-time PCR panel for rapid diagnosis of viruses associated with porcine respiratory and reproductive disorders. Mol. Cell. Probes 28, 264–270. doi: 10.1016/j.mcp.2014.07.001
Wu, R., Wang, Q., Liu, H., Chai, C., He, B., Huang, X., et al. (2016). Phylogenetic analysis reveals that Japanese encephalitis virus genotype III is still prevalent in swine herds in Sichuan province in China. Arch. Virol. 161, 1719–1722. doi: 10.1007/s00705-016-2814-y
Xiao, L., Wang, Y., Kang, R., Wu, X., Lin, H., Ye, Y., et al. (2018). Development and application of a novel bio–Plex suspension array system for high–throughput multiplexed nucleic acid detection of seven respiratory and reproductive pathogens in swine. J. Virol. Methods 261, 104–111. doi: 10.1016/j.jviromet.2018.08.017
Xie, S., Pan, J., Zhang, Q., Guan, R., Yang, X., Yang, X., et al. (2022). Japanese encephalitis virus (JEV) NS1’ enhances the viral infection of dendritic cells (DCs) and macrophages in pig tonsils. Microbiol. Spectr. 10, e01147–e01122. doi: 10.1128/spectrum.01147-22
Xinglin, J., Huanchun, C., Qigai, H., Xiang, W., Bin, W., Dexin, Q., et al. (2002). The development and application of the latex agglutination test to detect serum antibodies against Japanese encephalitis virus. Vet. Res. Commun. 26, 495–503. doi: 10.1023/A:1020546626694
Xinglin, J., Huanchun, C., Xiang, W., and Changming, Q. (2005). Quantitative and qualitative study of enzyme-linked immunosorbent assay to detect IgG against Japanese encephalitis virus in swine sera. Vet. Res. Commun. 29, 159–169. doi: 10.1023/B:VERC.0000047485.85866.6e
Xu, X.-G., Wang, Z.-S., Zhang, Q., Li, Z.-C., Zhao, H.-N., Li, W., et al. (2011). Baculovirus surface display of E envelope glycoprotein of Japanese encephalitis virus and its immunogenicity of the displayed proteins in mouse and swine models. Vaccine 29, 636–643. doi: 10.1016/j.vaccine.2010.11.045
Xu, G., Xu, X., Li, Z., He, Q., Wu, B., Sun, S., et al. (2004). Construction of recombinant pseudorabies virus expressing NS1 protein of Japanese encephalitis (SA14-14-2) virus and its safety and immunogenicity. Vaccine 22, 1846–1853. doi: 10.1016/j.vaccine.2003.09.015
Yamada, M., Nakamura, K., Yoshii, M., and Kaku, Y. (2004). Nonsuppurative encephalitis in piglets after experimental inoculation of Japanese encephalitis flavivirus isolated from pigs. Vet. Pathol. 41, 62–67. doi: 10.1354/vp.41-1-62
Yamada, M., Nakamura, K., Yoshii, M., Kaku, Y., and Narita, M. (2009). Brain lesions induced by experimental intranasal infection of Japanese encephalitis virus in piglets. J. Comp. Pathol. 141, 156–162. doi: 10.1016/j.jcpa.2009.04.006
Yamanaka, A., Mulyatno, K. C., Susilowati, H., Hendrianto, E., Utsumi, T., Amin, M., et al. (2010). Prevalence of antibodies to Japanese encephalitis virus among pigs in Bali and East Java, Indonesia, 2008. Jpn. J. Infect. Dis. 63, 58–60. doi: 10.7883/yoken.63.58
Yamaoka, M. (1983). Enzyme-linked immunosorbent assay for detection of IgG and IgM class antibodies to Japanese encephalitis virus. Kobe J. Med. Sci. 29, 197–226.
Yamaoka, M., Konishi, E., and Matsumura, T. (1982). Use of enzyme-linked immunosorbent assay for detection of IgM antibodies to Japanese encephalitis virus in swine sera. Kobe J. Med. Sci. 28, 143–153.
Yang, D. K., Kim, H. H., Jo, H. Y., Lee, S. H., Jang, S. H., Lee, S. O., et al. (2017). Improvement of indirect enzyme-linked immunosorbent assay for detection of Japanese encephalitis virus antibodies in swine sera. Kor. J. Vet. Res. 57, 31–36. doi: 10.14405/kjvr.2017.57.1.31
Yang, D. K., Kim, B. H., Kweon, C. H., Kwon, J. H., Lim, S. I., and Han, H. R. (2004a). Biophysical characterization of Japanese encephalitis virus (KV1899) isolated from pigs in Korea. J. Vet. Sci. 5, 125–130. doi: 10.4142/jvs.2004.5.2.125
Yang, D. K., Kim, B. H., Lim, S. I., Kwon, J. H., Lee, K. W., Choi, C. U., et al. (2006). Development and evaluation of indirect ELISA for the detection of antibodies against Japanese encephalitis virus in swine. J. Vet. Sci. 7, 271–275. doi: 10.4142/jvs.2006.7.3.271
Yang, Z., Wu, R., Li, R. W., Li, L., Xiong, Z., Zhao, H., et al. (2012). Chimeric classical swine fever (CSF)-Japanese encephalitis (JE) viral replicon as a non-transmissible vaccine candidate against CSF and JE infections. Virus Res. 165, 61–70. doi: 10.1016/j.virusres.2012.01.007
Yang, L., Xiao, A., Wang, H., Zhang, X., Zhang, Y., Li, Y., et al. (2022). A VLP-based vaccine candidate protects mice against Japanese encephalitis virus infection. Vaccine 10:197. doi: 10.3390/vaccines10020197
Yonemitsu, K., Minami, S., Noguchi, K., Kuwata, R., Shimoda, H., and Maeda, K. (2019). Detection of anti-viral antibodies from meat juice of wild boars. J. Vet. Med. Sci. 81, 155–159. doi: 10.1292/jvms.18-0576
Yoshida, I., Takagi, M., Inokuma, E., Goda, H., Ono, K., Takaku, K., et al. (1981). Establishment of an attenuated ML-17 strain of Japanese encephalitis virus. Biken J. 24, 47–67.
You, D., Xu, T., Huang, B. Z., Zhu, L., Wu, F., Deng, L. S., et al. (2024). Rapid, sensitive, and visual detection of swine Japanese encephalitis virus with a one-pot RPA-CRISPR/EsCas13d-based dual readout portable platform. Int. J. Biol. Macromol. 277:134151. doi: 10.1016/j.ijbiomac.2024.134151
Young, C. L., Lyons, A. C., Hsu, W.-W., Vanlandingham, D. L., Park, S. L., Bilyeu, A. N., et al. (2020). Protection of swine by potent neutralizing anti-Japanese encephalitis virus monoclonal antibodies derived from vaccination. Antivir. Res. 174:104675. doi: 10.1016/j.antiviral.2019.104675
Zhang, H., Rehman, M. U., Li, K., Luo, H., Lan, Y., Nabi, F., et al. (2017b). Epidemiologic survey of Japanese encephalitis virus infection, Tibet, China, 2015. Emerg. Infect. Dis. 23, 1023–1024. doi: 10.3201/eid2306.152115
Zhang, M., Xie, Z., Xie, L., Deng, X., Luo, S., Liu, J., et al. (2015). Simultaneous detection of eight swine reproductive and respiratory pathogens using a novel GeXP analyser-based multiplex PCR assay. J. Virol. Methods 224, 9–15. doi: 10.1016/j.jviromet.2015.08.001
Zhao, S., Lou, Y., Chiu, A. P. Y., and He, D. (2018). Modelling the skip-and-resurgence of Japanese encephalitis epidemics in Hong Kong. J. Theor. Biol. 454, 1–10. doi: 10.1016/j.jtbi.2018.05.017
Zheng, B., Wang, X., Liu, Y., Li, Y., Long, S., Gu, C., et al. (2019). Japanese encephalitis virus infection induces inflammation of swine testis through RIG-I—NF-ĸB signaling pathway. Vet. Microbiol. 238:108430. doi: 10.1016/j.vetmic.2019.108430
Zhou, Y., Chen, L., Zhang, L., Shao, C., Sun, J., Jiang, S., et al. (2020). Simultaneous identification of 6 pathogens causing porcine reproductive failure by using multiplex ligation-dependent probe amplification. Transbound. Emerg. Dis. 67, 2467–2474. doi: 10.1111/tbed.13585
Keywords: Japanese encephalitis virus, rapid systematic review, swine, transmission, knowledge synthesis
Citation: Horton V, Hanthorn CJ, Dixon AL, Edache SE, Thackrah A, Mitzel D, Noronha L, Cohnstaedt LW, Brown VR, Mire C and Cernicchiaro N (2024) Synthesizing evidence and identifying knowledge gaps in the role of swine in Japanese encephalitis virus transmission: a rapid systematic review of the literature. Front. Sustain. Food Syst. 8:1455455. doi: 10.3389/fsufs.2024.1455455
Edited by:
Ruth Stewart, University College London, United KingdomReviewed by:
Edward D. Walker, Michigan State University, United StatesAkanksha Roberts, Helmholtz Institute for RNA-based Infection Research (HIRI), Germany
Copyright © 2024 Horton, Hanthorn, Dixon, Edache, Thackrah, Mitzel, Noronha, Cohnstaedt, Brown, Mire and Cernicchiaro. This is an open-access article distributed under the terms of the Creative Commons Attribution License (CC BY). The use, distribution or reproduction in other forums is permitted, provided the original author(s) and the copyright owner(s) are credited and that the original publication in this journal is cited, in accordance with accepted academic practice. No use, distribution or reproduction is permitted which does not comply with these terms.
*Correspondence: N. Cernicchiaro, bmNlcm5pY0B2ZXQuay1zdGF0ZS5lZHU=