- 1Clinical Department for Farm Animals and Food Safety Systems, Centre for Veterinary Systems Transformation and Sustainability, University of Veterinary Medicine, Vienna, Austria
- 2Clinical Department for Farm Animals and Food Safety Systems, Centre for Animal Nutrition and Welfare, University of Veterinary Medicine Vienna, Vienna, Austria
- 3Institute of Food Research and Product Development, University of Kasetsart, Bangkok, Thailand
- 4Clinical Department for Farm Animals and Food System Science, Clinical Centre for Population Medicine in Fish, Pig and Poultry, University of Veterinary Medicine Vienna, Vienna, Austria
The re-integration of former foodstuffs, such as bakery products (BP), into pig feed reduces the feed-food competition and increases the sustainability of pig production. Nevertheless, there are concerns about the altered fatty acid profile in BP compared to standard diets and their effects on meat quality and the intestinal microbiome. The present study investigated the effects of replacing 30% cereal grains with BP in the diet on performance, microbiome composition and metabolites in feces of fattening pigs, economic aspects and meat quality characteristics. A total of 132 pigs (Large White × Piétrain; 11 weeks of age) were fed two diets containing 0 or 30% BP until reaching slaughter weight in three replicate batches. Fecal samples were collected for 16S rRNA amplicon sequencing after 6 weeks, whereas body fat samples were collected after 9 weeks on the diet. The results showed that BP can replace cereals in pig diets by up to 30% without impairing animal performance, while improving feed efficiency and reducing feed costs by 9% compared to the control. Additionally, pigs fed the BP diet had a 50%-higher n-3 fatty acid content in the neck and abdominal fat compared to the control. The improved feed efficiency in BP-fed pigs may be related to positive effects on the fecal microbiome, such as a higher alpha diversity and a reduction of fungi and yeasts. Our study provides valuable insights into the benefits of including BP in diets of fattening pigs, which should be investigated in future studies on other BP types.
1 Introduction
Animal-based foods contribute to 16% of the global food supply and represent an important protein source in human diets. However, their production uses a disproportionately large fraction of land and water resources (Govoni et al., 2023). In intensive pig production systems, cereals represent about 60% of the composition (Govoni et al., 2022). As (pseudo-)cereal grains are human-edible ingredients, pigs are in direct competition with food production. Due to the increasing world population (Godfray et al., 2010) and occurrence of extreme weather conditions (i.e., drought, flooding, and hail) due to climate change, which may reduce crop yields, enhance the competitive situation between farm animals and humans (Makkar, 2018). A global assessment of the land and water resource demand for pork supply in 2022 estimated that pig production needs more than 40 Mtons of cereal grains in the European Union and about 200 Mtons worldwide (Govoni et al., 2022). One option to decrease the competition between pig feed production and human food production is the utilization of by-products. Another option is the re-utilization of food products as it does not only help decreasing the competition with humans and avoiding food waste but concurrently it reduces the environmental impact of food animal production (Pinotti et al., 2023). About one-third of the worldwide produced food is lost or wasted per year (Cederberg et al., 2011). The 2030 Agenda for Sustainable Development promotes the recycling of food losses including their use as ingredients in animal feed (FAO - Food and Agriculture Organization of the United Nations, 2019). Recycling energy and nutrients from former food products, such as bakery products (BP), into animal feed may replace conventional starchy feed ingredients (Kaltenegger et al., 2021). However, recycling energy and nutrients from food products, such as BP, into diets for pigs has not been attractive due to the high variability in the BP product and lack of data on the digestibility of energy and nutrients (Fung et al., 2019). Bakery products comprise a wide range of products from bakeries and supermarkets that were unsold, were due to expire or were taken from the shelves due to consumer preferences for freshness [i.e., (sliced) bread, croissants, biscuits, cakes, and dough; França et al., 2012]. Nutritionally, the nutrient composition can vary greatly depending on the type of BP (Arosemena et al., 1995; Humer et al., 2018). Typically, they are higher in ether extract (EE) (e.g., between 4 and 12% EE in DM), sugars and salt compared to cereal grains (Humer et al., 2018). The high variability in the chemical composition renders precise feed formulation difficult, which is necessary to keep the environmental impact of pig production low. Consequently, dietary inclusion levels remain low in practice, showing the need to evaluate higher inclusion levels in pig diets. Nevertheless, the few studies that were already conducted used different types of food products including BP. These studies showed that bakery and confectionary food products are sustainable ingredients and no adverse effects on growth performance were observed in weaned pigs and dairy cows when included in the diet at 30% and 15 or 30%, respectively (Kaltenegger et al., 2021; Luciano et al., 2021; Tretola et al., 2022). However, concerns remain as the differences in the dietary fatty acid (FA) profile may affect the animal product, especially at higher dietary inclusion levels. This aspect is of importance for sausage production as well as consumer health because of the putative health-related effects of different FA. Moreover, the source of fat in BP such as cakes and cookies are often coconut and palm oil which comprise higher amounts of medium-chain fatty acids (MCFA) that can affect the gut microbiota composition. For instance, lauric and myristic acid have certain antimicrobial properties (e.g., López-Colom et al., 2019). By contrast, the higher sugar and lower fiber content of BP compared to cereal grains may also affect the structure and biodiversity of the gut microbiome (Tretola et al., 2022). Due to the few studies that were only conducted in pigs so far (Tretola et al., 2022), there is a need to understand more how the replacement of cereals by BP influence the microbial homeostatic conditions in the gut.
The present study aimed to evaluate the null hypothesis that a replacement of dietary cereals by BP (mixture of different bakery types such as bread, rolls and sweet pastries) at a level of 30% would not affect growth performance, feed efficiency, total tract digestibility, fecal microbiome composition, structure and activity, and carcass (fat) composition in fattening pigs. We also assessed economical aspects. For these, we hypothesized lower feed costs by the replacement of cereals by BP.
2 Materials and methods
The experimental protocol was in compliance with the Good Scientific Practice guidelines of the institutional ethics committee of the University of Veterinary Medicine Vienna and national legislation (Protocol no. ETK-123/08/2021).
2.1 Animals and housing
A total of 132 pigs [Large White × Piétrain; 66 gilts and 66 barrows] at 11 weeks of age [average initial body weight (BW) 35.2 ± 4.83 kg] were used in three consecutive replicate batches (36, 48 and 48 pigs in batch 1, 2 and 3, respectively) at the pig facility (Vetfarm) of the University of Veterinary Medicine Vienna, which is a commercial pig farm. Pigs were housed in the fattening unit which comprised 6 pens (3.50 m × 5.48 m per pen) with slatted flooring and a laying area. In replicate batches 1, 2, and 3, pigs were penned in groups of 6, 8, and 8 per pen, respectively; each pen comprised equal numbers of gilts and barrows. Each pen was equipped with two nipple-drinkers and one transponder-based feeder (Feed Intake Recording Equipment (FIRE) feeder, SCHAUER Agrotonic GmbH, Prambachkirchen, Austria). Each pig received an individual radio-frequency identification ear tag (RFDI; CI Tiris AF-Ohrchip 25.5 mm, SCHAUER Agrotonic GmbH, Prambachkirchen, Austria) when they were moved to the fattening unit. Pigs were acclimatized to the housing and feeding stations for 1 week before the start of the experiment. Each replicate batch lasted 11 weeks. Water was freely available. The health status of the pigs was monitored daily by visual inspection and examining the feed intake records.
2.2 Diets and feeding
Two isonitrogenous and isoenergetic diets (Table 1) were formulated to meet or exceed the current recommendations for nutrient requirements (National Research Council, 2012): (1) control diet (CON); and (2) bakery product (BP) diet. The diets were formulated to correspond to rations that were fed on Austrian pig farms at the time of the experiment. This includes the BP diet. Diets were produced at a local feed mill (Königshofer GmbH, Ebergassing, Austria) that collected the BP from Viennese bakeries and supermarkets and regularly includes BP in their compound feed. Therefore, the BP used contained a mixture of different bakery product types such as bread, rolls and sweet pastries, which were dried, ground and pelletized and then mixed together with the other ingredients. In the BP diet, BP replaced 30% of the cereals (corn and wheat). To achieve equal energy concentrations, the BP diet contained more wheat bran. Pens were randomly allocated to the two diets; in each replicate batch three pens received one of the two diets, resulting in a total of nine pens per diet across all three replicate batches (n = 66 pigs/diet). Diet samples were collected in each replicate batch. The pigs had free access to pelleted feed via the transponder-based feeders. One pig at a time got access to the feeder by means of the RFID ear tag. Every visit of the pig (time) and amount of feed was registered by the FIRE feeder by weighing the trough before and after the pig got access to it. The data for each pig were summed up and used to calculate average daily feed intake (ADFI).
2.3 Body weight measurement and calculation of feed efficiency and feed costs
Pigs were weighed when transferred to the fattening barn, at the beginning of the experiment and every 2 weeks thereafter using a transportable animal scale (Agreto Einzeltierwaage, Agreto electronics GmbH, Raabs, Austria). The average daily gain (ADG) and ADFI were calculated for the two-week-intervals from week 2 to 10. Moreover, ADG and ADFI were used to calculate the feed conversion ratio (FCR) and the residual feed intake (RFI) as feed efficiency metrics. The RFI partitions the feed intake (FI) into maintenance and production components. Linear regression is used to compute the expected FI based on mid-test BW0.75, growth, and the difference between actual and expected FI is defined as RFI (Bottje and Carstens, 2009). Accordingly, the RFI was calculated as the residuals from a least squares regression model of ADFI on ADG, metabolic mid-weight (corresponds to the average metabolic weight of the pig while on test), sex and all relevant two-way interactions, using the PROC REG procedure in SAS (version 9.4; SAS Inst. Inc., Cary, NC, United States). Data on the feed intake were used to calculate the feed costs per animal. In order to do so, the feed costs per kg feed were multiplied with the feed intake of each individual pig in each of the three replicate batches.
2.4 Fecal sampling
Fecal samples for dry matter (DM) content, total tract digestibility, short-chain fatty acid (SCFA), and microbiome analysis were aseptically collected from all pigs via rectal stimulation in the morning on day 42 (week 6) of the experimental period. During the collection, samples were kept on ice. Thereafter, fecal samples were quickly homogenized using a spatula, then aliquoted and transferred to −20°C until analysis.
2.5 Proximate nutrient and fatty acid analysis of diets
Pooled dietary samples were sent for proximate nutrient analysis to a commercial feed laboratory (Feed Laboratory Rosenau, Haag, Austria; Supplementary Table S1). Dry matter, crude ash, crude protein, ether extract, neutral detergent fiber, acid detergent fiber, acid detergent lignin, and total starch were measured according to the methods described by Naumann and Bassler (2012). Briefly, to determine the DM content (method 3.1), diet samples were oven-dried at 103°C for 4 h, whereas crude ash (method 3.5) was determined by incinerating at 580°C for 4 h (Naumann and Bassler, 2012). Ether extract (method 5.1.1) was analyzed by solvent extraction with petroleum ether. The content of crude protein was determined according to DUMAS (method 4.1.2). The adapted methods of van Soest et al. (1991) were used for the analyses of neutral and acid detergent fiber, as well as acid detergent lignin (methods 6.5.1, 6.5.2, and 6.5.3). The nitrogen-free extract fraction was calculated by subtracting crude ash, crude protein, crude fiber, and ether extract from the DM (Naumann and Bassler, 2012). Total starch was determined using polarimetric method (method 7.2.1). Dietary fatty acids (Supplementary Table S2) were analyzed according to the description in Metzler-Zebeli et al. (2021). Fecal samples from all replicate batches were freeze-dried before they were ground to pass a 1-mm sieve and analyzed for DM, crude ash and crude protein as described above. In addition, fecal and diet samples were analyzed for acid-insoluble ash (AIA; method 8.2; Naumann and Bassler, 2012) as indigestible marker. The apparent total tract digestibility (ATTD) of DM, crude ash and protein were calculated using the equation:
2.6 Short-chain fatty acid analysis in feces
Concentrations of SCFA (acetate, propionate, butyrate, iso-butyrate, valerate, iso-valerate, and caproate) were determined in feces from all replicate batches using gas chromatography (GC) after extraction with 25% phosphoric acid and addition of 4-methylvaleric acid as internal standard (Sigma-Aldrich, St. Louis, MO, United States). Homogenized sample solutions were centrifuged (20,000 × g for 20 min) and the clear supernatant was used to measure SCFA on the GC-2010 Plus Capillary GC (Shimadzu Corp., Kyoto, Japan) using a 30 m × 0.53 mm × 0.5 μm capillary column (Trace TR Wax, Thermo Fisher Scientific, Waltham, MA, United States) and helium as carrier gas. The gas chromatograph was equipped with an autosampler and injector (AOC-20s Auto Sampler; AOC-20i Auto-Injector, Shimadzu Corp., Kyoto, Japan) and a flame-ionization detector (FID-2010 Plus, Shimadzu Corp., Kyoto, Japan).
2.7 Carcass composition and cost calculation of lean meat production
In replicate batch 2, pigs received an additional tattoo which allowed following the individual pigs throughout the processing at a commercial slaughter plant. Parameters obtained from the slaughter plant were gross and net weight, lean percentage and pH of the carcass. The feed costs to produce 1 kg of lean meat were additionally calculated in replicate batch 2.
2.8 Fatty acid composition of neck, kidney and subcutaneous fat
The composition of the FA in neck, kidney and subcutaneous fat was determined in eight pigs (four males and four females) per dietary group on days 62 and 63 (week 9) of the experimental period in replicate batch 3. Gilt and barrow pairs were selected per pen based on the average body weight per dietary group. From one pen per dietary group, two gilt and barrow pairs were sampled, divided on the two sampling days. Pigs were bold stunned and bled before fat tissue pieces were cut from the mid neck, from the mid abdomen and from around the kidneys. The fat tissue pieces were cut in small pieces, placed in 8-mL tubes (Sarstedt, Nümbrecht, Germany) and kept in crushed ice before storing them at −20°C until analysis.
Prior to analysis, the frozen tissue samples were treated with liquid nitrogen and homogenized by use of mortar and pestle. After grinding, the method of Folch et al. (1957) with slight modifications was applied for extraction of tissue lipids. In order to do so, approx. 100 mg of the samples were mixed with 7.5 mL of a mixture of chloroform and methanol (2/1, v/v), sealed and then gently shaken by hand occasionally for 2.5 h at room temperature. The obtained extract was placed in a new glass tube and diluted with 1.8 mL of an aqueous KCl solution (0.88%, w/v) before being mixed to homogeneity. Subsequently, the mixture was centrifuged at 1,811 × g for 10 min at room temperature. After discarding the aqueous supernatant, the lower organic phase was collected in a pre-weighed glass tube and evaporated under N2 current. Then, the extracted lipids were weighed and dissolved in hexane to achieve a final concentration of approx. 20 mg/mL.
Each fraction was extracted and then exposed to acid-catalyzed transesterification by diluting in 0.5 mL of hexane and then in 2 mL of a 10% methanolic HCl solution in a sealed glass and heated at 80°C for 10 min. Heptadecanoic acid (17:0) was used as an internal standard and therefore added to the liquid fraction. Following cooling, 5 mL of hexane and then 2 mL of an aqueous solution of K2CO3 (6%, w/v) were added under vigorous stirring. This was followed by a centrifugation step at 1,600 × g at room temperature for 5 min. After the upper phase was collected in a new glass tube, it was evaporated under a stream of N2. Before the resulting FA methyl esters (FAMEs) were stored in sealed glass vials at −20°C until analysis, they were dissolved in 200 μL hexane. All chemicals and solvents used were of analytical grade and were obtained from Sigma-Aldrich and Merck. FAMEs were analyzed and quantified using a GC instrument (Shimadzu GC Plus with FID detector) that was equipped with a Zebron ZB-WAXplus fused silica capillary column (30 m × 0.25 mm i.d. × 0.25 μm) (Phenomenex, Aschaffenburg, Germany). The temperatures of injector and detector were 250°C and 260°C, respectively and helium was used as carrier gas. The peak identification of FAME was carried out by comparison with an external standard using a FAME mixture (Supelco 37 Component FAME Mix, Supelco, Bellefonte, PA, United States). The quantification of FA was carried out on the basis of both internal and external standards according to the AOAC method no. 2012.13 (Golay and Moulin, 2016).
2.9 DNA extraction, bacterial quantification and sequencing
Total DNA were isolated from fecal samples from replicate batch 1 using the PowerSoil DNA isolation kit (Qiagen, Hilden, Germany) using previously described modifications (Metzler-Zebeli et al., 2019). Samples were homogenized for three times 60 s with 60 s on ice in between (6.5 m/s) using the FastPrep-24 instrument (MP Biomedicals, Heidelberg, Germany). The Qubit 4 fluorometer (Thermo Fisher Scientific Inc., Waltham, MA, United States) with the Qubit DNA assay kit were used to quantify the total DNA concentration.
The extracted DNA was quantified using quantitative PCR with primers and amplification conditions described in Lerch et al. (2022, 2023) (Supplementary Table S3). Cytochrome B was also determined as a marker for cell turnover in the large intestine. Amplicon sequencing of the V3-V4 region of the 16S rRNA gene was performed on an Illumina MiSeq sequencing v2 platform using a paired-end protocol by a commercial provider (Microsynth, Balgach, Switzerland). This included the 16S rRNA PCRs, library preparation (Nextera XT DNA Sample Preparation Kit, Illumina, San Diego, CA, United States), and sequencing. The primers 341F-ill (5′-CCTACGGGNGGCWGCAG-3′) and 802R-ill (5′-GACTACHVGGGTATCTAATCC-3′) were used, which generate an amplicon of approximately 460 bp. The 16S rRNA PCRs were performed using the KAPA HiFi HotStart PCR Kit (Roche, Baden, Switzerland). Subsequently, reads were demultiplexed and adapter sequences were removed using cutadapt.1
Raw sequencing reads (Fastq files) of the 16S rRNA amplicon were processed, aligned and categorized using the Divisive Amplicon Denoising Algorithm 2 (DADA2; version 1.26.0) in R studio (version 1.4.1106). The DADA2 protocol was similar to that described in Lerch et al. (2022, 2023). By using the “file.path” function, sequences were pre-filtered and reads with ambiguous bases were removed. The first 10 nucleotides were trimmed and the total length of forward and reverse reads were truncated to 220 nucleotides to account for the decrease in quality score of the following nucleotides using the “filterAndTrim” function. In addition, reads exceeding the probabilistic estimated error of two nucleotides were removed in the same step (“filterAndTrim” function). After de-replication of the filtered data and estimation of error rates, amplicon sequence variants were inferred. Thereafter, the function “mergepairs” was used to merge the denoised pairs of forward and reverse reads. Pairs were rejected that did not sufficiently overlap, or which contained too many mismatches in the overlap region. Chimera were removed using the “removeBimeraDenovo()” function. Taxonomy was assigned using the function “assignTaxonomy,” which implements the RDP Naive Bayesian Classifier algorithm and reference databases—SILVA 138.1 ribosomal RNA (rRNA) database for bacteria (Quast et al., 2013). Taxonomic tables, taxonomic assignment and corresponding metadata were combined to create phyloseq objects in the R phyloseq (version 1.42.0) (McMurdie and Holmes, 2013).
2.10 Statistical analysis
The residuals of data for feed intake, growth, feed efficiency, total tract digestibility, bacterial abundances and diversity, SCFA, FA composition in body fat tissues, carcass, and costs were tested for normal distribution by the Shapiro–Wilk test with the UNIVARIATE procedure in SAS (Version 9.4, SAS Inst. Inc., Cary, NC, United States). If not normally distributed, log-transformed data were used for subsequent statistical analysis in SAS. Thereafter, data were subjected to ANOVA using the MIXED procedure in SAS. Several random models were tested. The final random model included the fixed effects of replicate batch, pen, sex, diet, and the two-way interaction sex × diet. For data that were collected in all three replicate batches (feed intake, growth, feed efficiency, total tract digestibility, SCFA, and feed costs), the random effect was replicate batch; pig nested within pen represented the experimental unit. For data that were collected only in one replicate batch (data for bacterial microbiome, carcass and FA composition of fat tissues), the fixed effects were pen, sex, diet, and the two-way interaction sex × diet, the random effect was pen and the experimental unit pig. Relative abundances of individual bacterial genera were analyzed by ANOVA only if their relative abundance was above 0.5%. Body weight at the beginning of the experiment was used as covariate for the analysis of growth and feed intake data. The degrees of freedom were approximated by the Kenward-Rogers method (ddfm = kr). The means were reported as least-squares means ± standard error of the mean (SEM). Comparisons between least squares means were performed using the pdiff option in SAS. Differences were considered significant if p ≤ 0.05 and as trend if p ≤ 0.10.
Non-metric multidimensional scaling (NMDS) followed by permutational analysis of variance (PERMANOVA) was applied to statistically assess dissimilarity matrices (Bray-Curtis) derived from the microbiome data for sex, diet and the interaction of sex × diet “community-type” using the “metaNMDS” and “adonis2” functions, respectively, in the vegan R package (version 2.6.4; Oksanen et al., 2022). Specifically, the two-dimensional NMDS ordination plots based on the Bray–Curtis dissimilarity matrix (“metaMDS” function) were employed to determine microbiome clustering by feed samples and were visualized using the ggplot2 package (Wickham, 2016). Statistical significance was calculated after 999 random permutations. Alpha-diversity indices and fatty acid composition of neck, kidney and subcutaneous fat were visualized with the ggplot2 package (Wickham, 2011).
3 Results
3.1 Feed intake and growth performance
Supplementary Table S4 displays the BW, ADG and total body weight gain (TBWG) of the pigs. During the whole experimental period, there were no interactions between sex and diet on BW, ADG, or TBWG. Sex affected BW, ADG, and TBWG. Male pigs had higher BW, ADG and TBWG in male pigs throughout the entire experimental period (p ≤ 0.05), except for BW in week 1 (p > 0.05), compared to female pigs. Concerning the diet effect, pigs fed the BP had a lower BW (p = 0.045), TBWG (p = 0.026) and ADG (p = 0.022) in week 9 and week 7–9, respectively, compared to the pigs fed the CON diet.
The feed intake (FI) and ADFI of pigs are shown in Supplementary Table S5. There were no interactions between sex and diet on FI or ADFI during the whole experiment. However, from the beginning to the end of the experiment, male animals had a higher FI (p ≤ 0.04) and ADFI (p ≤ 0.04) than females. Moreover, pigs fed the BP diet had a lower FI in week 11 compared to pigs fed the CON diet (p = 0.032).
3.2 Feed efficiency and costs
Table 2 illustrates the feed efficiency and cost calculation of the different diets. No sex × diet interaction was found for any of the measured parameters (p > 0.05). However, sex affected the mid-test body weight (p = 0.002), the metabolic mid-test body weight (p = 0.003), FCR (p < 0.001), and the feed costs per pig (p < 0.001). Barrows had a higher mid-test body weight and metabolic mid-test body weight compared to females, whereas females had a better FCR and lower feed costs per pig. Moreover, there was a trend for an interaction between sex and diet on RFI (p = 0.076). The BP diet improved the RFI (p = 0.038) and lowered the feed costs per pig by 9% compared to the CON diet (p < 0.001).
3.3 Apparent total tract digestibility
The results of the ATTD are given in Table 3. There was no interaction between sex and diet (p > 0.10). In addition, there was no main effect of sex (p > 0.10) or diet (p > 0.10) on the ATTD.
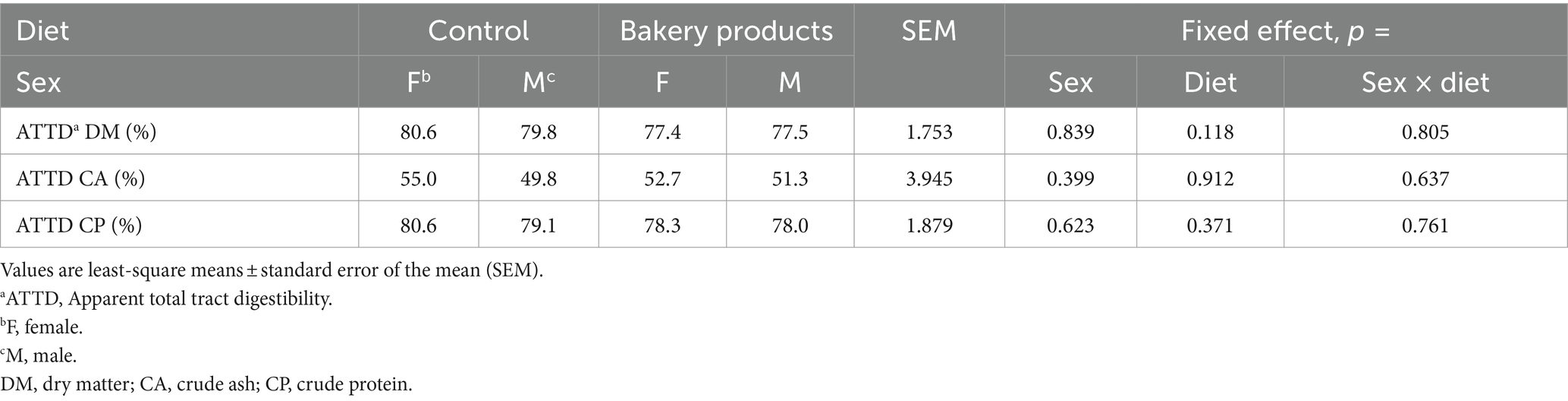
Table 3. Apparent total tract digestibility of pigs fed a control diet or a diet containing bakery products.
3.4 Short-chain fatty acids in feces
The concentration of SCFA in fecal samples of the pigs are illustrated in Table 4. Again, there was no interaction between sex and diet on the concentration of SCFA (p > 0.10). However, the BP diet increased the molar proportion of isobutyric acid compared to the CON diet (2.27 and 2.46% of total SCFA for CON and BP diet, respectively, p = 0.022).
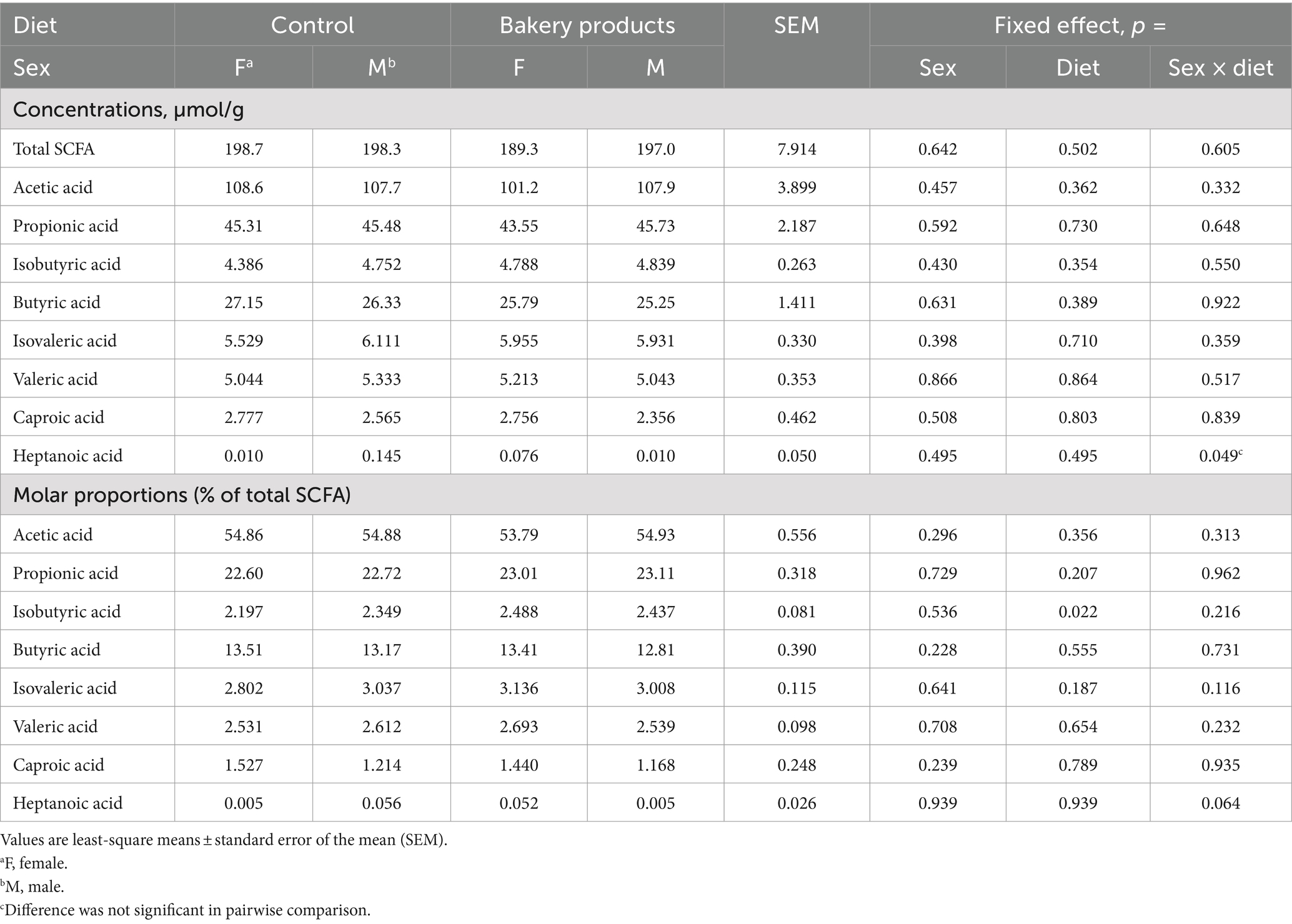
Table 4. Concentration of short-chain fatty acids in feces of pigs fed the control diet or the diet containing bakery products.
3.5 Carcass parameters
Table 5 displays the results of the carcass parameters determined at slaughter. There was no interaction between sex and diet on any of the analyzed carcass parameters (p > 0.10). However, sex affected the lean meat content. Females had a 4%-higher lean meat content than barrows (p < 0.001). By contrast, barrows hat a lower meat pH being lower in barrows than females (6.2 and 6.3 in barrows and females, respectively, p = 0.009). The BP diet reduced the feed costs per kg of lean meat by 18% compared to the CON group (p < 0.001).
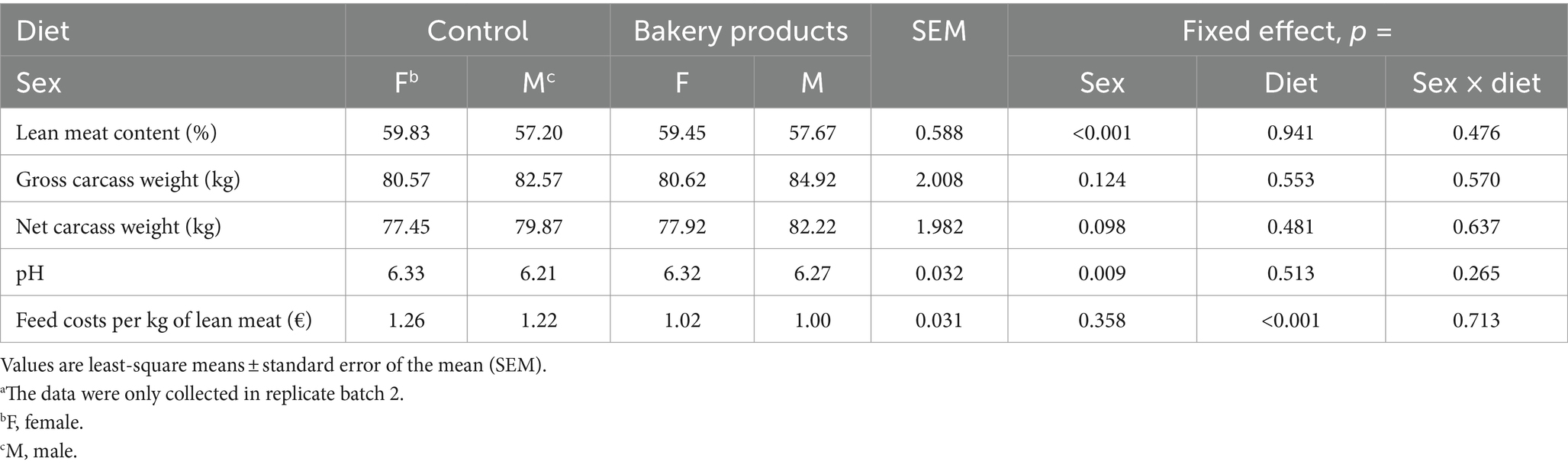
Table 5. Carcass parameters of pigs fed the control diet or the diet containing bakery products.a
3.6 Fatty acid composition in the kidney, neck and subcutaneous fat
Neither sex nor diet affected the FA composition in the kidney fat (p > 0.10; Supplementary Table S6). A sex × diet interaction existed for the neck fat. The concentration of C22:4 n-6 in the neck fat (p = 0.029) of barrows consuming the BP diet was 78% lower than in barrows fed with CON but similar to the females in both dietary groups (Supplementary Table S7). Another interaction was observed for C22:4 n-6 (p = 0.029): the concentration of C22:4 n-6 was higher in barrows and below the detection limit in females fed CON. Barrows had a higher concentration of C22:5 n-3 (docosapentaenoic acid) (p = 0.013), while it was under the detection limit in females. Moreover, pigs receiving the BP diet had a 51%-higher concentration of C18:3 n-3 than pigs receiving the CON diet (p = 0.033; Figure 1A) and also tended to have a higher sum of n-3 polyunsaturated FA (PUFA; p = 0.063; Supplementary Table S7). Concerning the FA profile in the subcutaneous abdominal fat, diet affected the concentrations of C18:3 n-3 (p = 0.036) and C20:3 n-3 (p = 0.039) was observed (Supplementary Table S8). Pigs fed the BP diet had 35 and 24%-higher concentrations of both FA compared to pigs fed the CON diet, respectively (Figures 1B,C). Additionally, the sum of n-3 PUFA was 50%-higher in the subcutaneous abdominal fat of pigs fed the BP diet compared to pigs fed the CON diet (p = 0.037; Figure 1D).
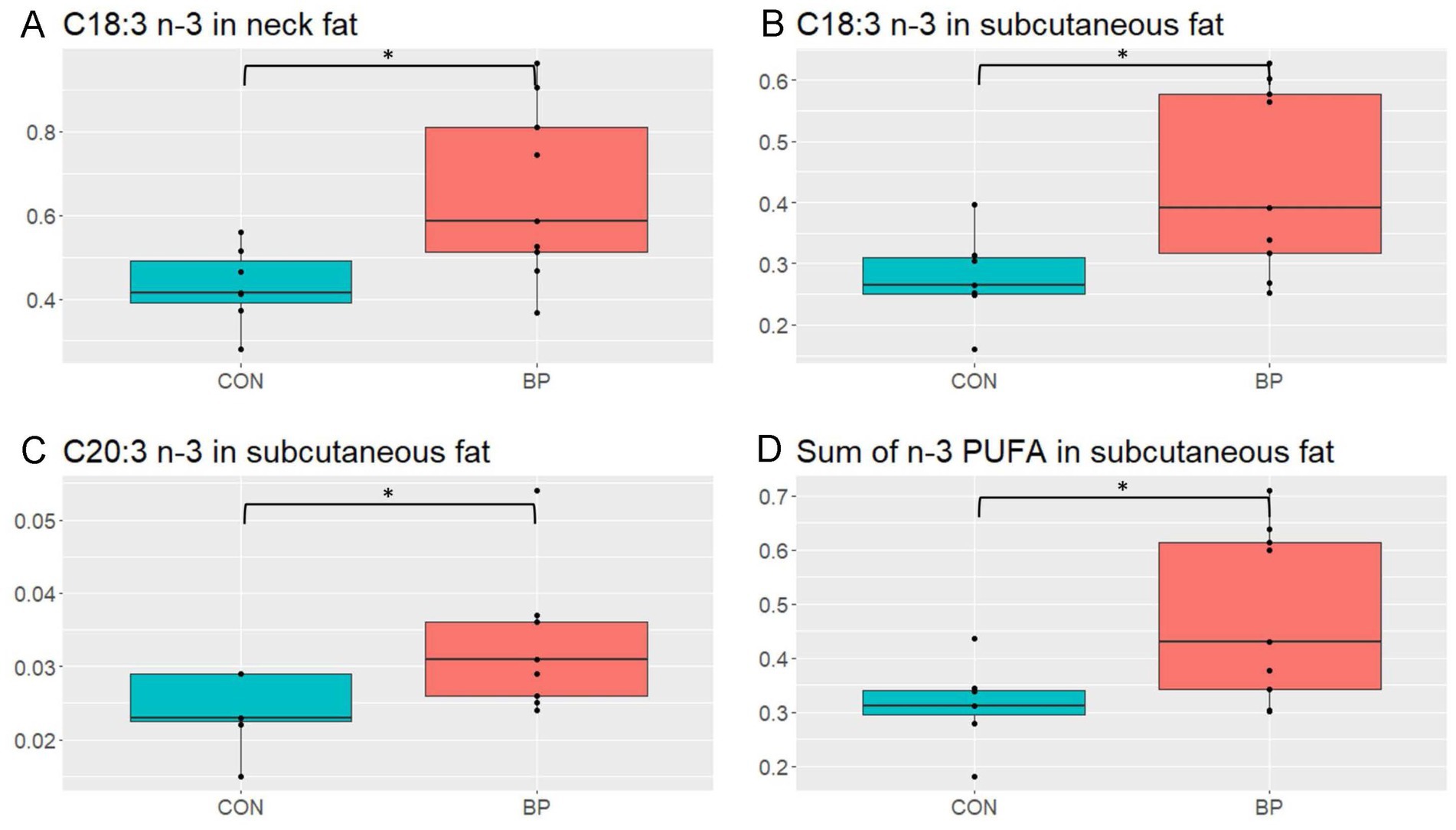
Figure 1. Concentrations of different fatty acids (g fatty acid/100g fresh sample) in the neck and subcutaneous abdominal fat of pigs fed either the control diet (CON) or the diet containing bakery products (BP). (A) Comparison of the concentration of C18:3 n-3 in the neck fat of CON or BP fed pigs (p = 0.033); (B) comparison of the concentration of C18:3 n-3 in the subcutaneous abdominal fat of CON or BP fed pigs (p = 0.036); (C) comparison of the concentration of C20:3 n-3 in the subcutaneous abdominal fat of CON or BP fed pigs (p = 0.040); and (D) comparison of the concentration of the sum of n-3 PUFA in the subcutaneous fat of CON or BP fed pigs (p = 0.037). Data were only collected in replicate batch 3.
3.7 Bacterial composition in feces
Table 6 displays the absolute abundance of microbes and cytochrome B in the feces of the pigs. Sex did not affect the absolute abundances (p > 0.10). However, the diet affected the absolute abundance of total bacteria (p = 0.035) and total fungi and yeast (p = 0.019) in the feces of the pigs. For both, feeding BP resulted in lower absolute abundance of the respective trait compared to CON.
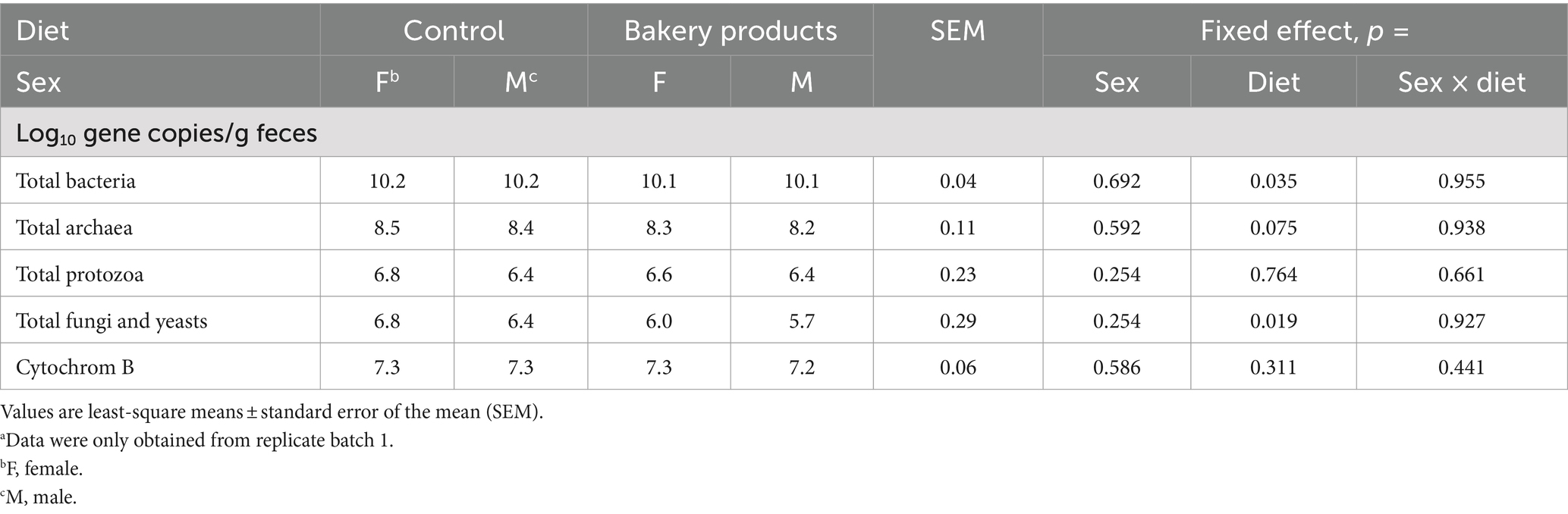
Table 6. Effect of bakery products on the absolute abundance of microbes and cytochrome B as a marker for intestinal cell renewal in the feces of pigs after 7 weeks of feeding.a
Comparison of species richness and α-diversity indices showed that pigs fed the CON diet had a lower (p < 0.05) alpha-diversity compared to pigs fed the BP diet as indicated by Shannon and Simpson indices (4.73 and 0.97 in CON compared to 4.99 and 0.98 in BP for Shannon and Simpson indices, respectively; Figure 2). Regarding the beta-diversity of bacterial communities in the feces of pigs, the PERMANOVA (Bray-Curtis) revealed no interaction between sex and diet, but the main effects of sex (p = 0.016) and diet (p = 0.032) influenced the bacterial community structure (Supplementary Table S10; Figure 3). The NMDS (Bray-Curtis distance) displayed mainly overlapping clusters between fecal microbiomes of pigs fed the BP or CON diet. However, samples from pigs fed the BP diet clustered closer together than samples from pigs fed the CON diet (Figure 3A). The separation of the CON and BP groups by sex showed that the cluster of male pigs fed the CON diet overlayed almost the entire clusters of the female pigs fed the CON diet and male and female pigs fed the BP diet (Figure 3B).
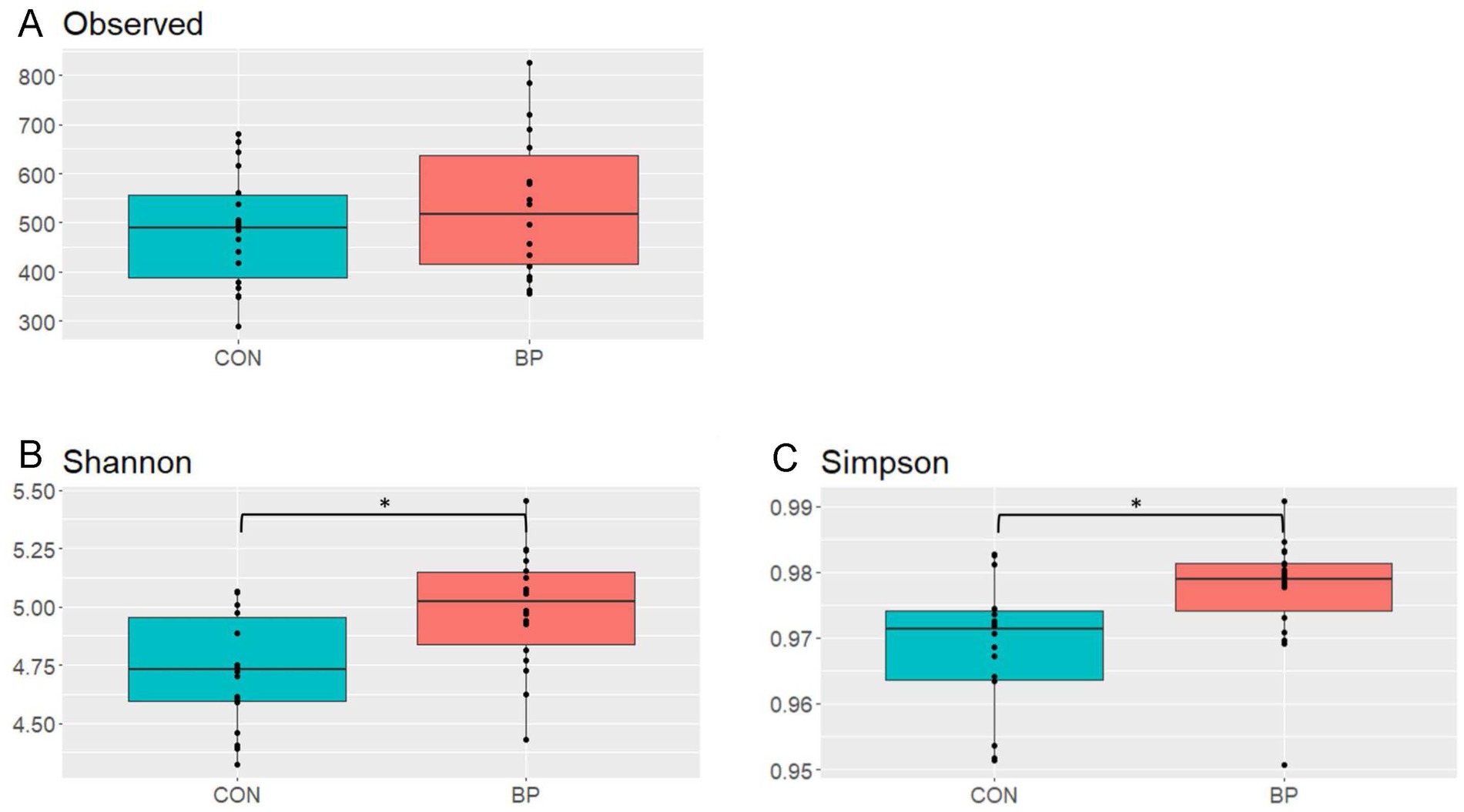
Figure 2. Comparison of species richness [Observed (A) and (B)] and α-diversity indices [Shannon (C) and Simpson (D)] in feces of pigs fed either the control diet (CON) or the diet containing bakery products (BP). Shannon and Simpson diversity indices differed significantly between CON and BP fed pigs (p = 0.004 and p = 0.013, respectively). Data were only collected in replicate batch 1.
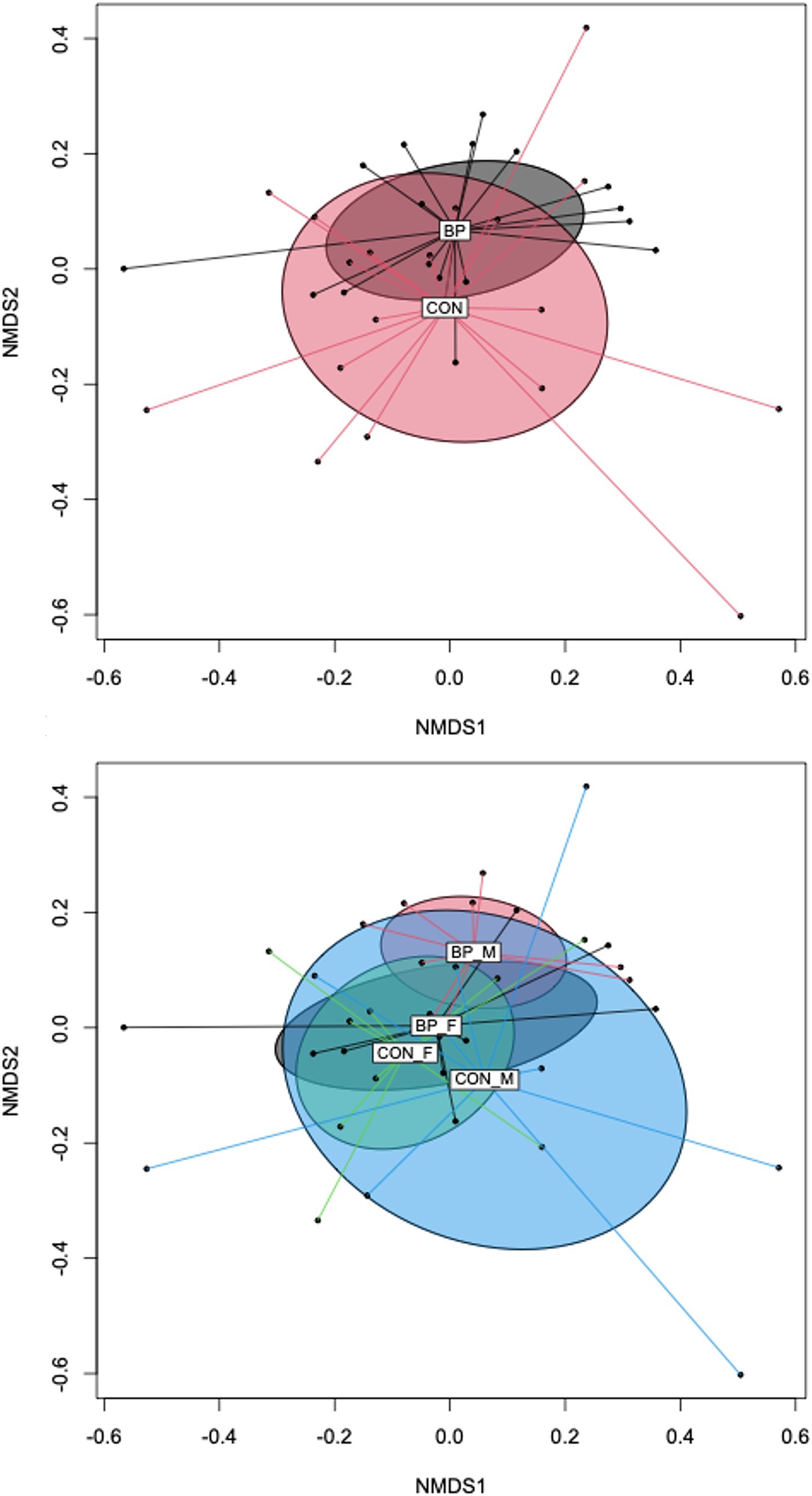
Figure 3. Beta-diversity of bacterial communities in feces of pigs. Non-metric multidimensional scaling (NMDS) calculated by Bray-Curtis distance. (A) Comparison of the bacterial community in pigs fed either the control diet (CON) or the diet containing bakery products (BP); and (B) comparison of the bacterial community in pigs fed either CON or BP subdivided into female (F) and male (M) pigs. Data were only collected in replicate batch 1.
Differences in the relative abundance of the most abundant genera in the feces of pigs are given in Table 7 and significant differences were found for 16 genera. There was an interaction effect between sex and diet for the relative abundance of UCG-002 (p = 0.043) and Marvinbryantia (p = 0.012). For UCG-002, female pigs had a 1.3 percentage points higher relative abundance but only when fed the BP diet and not when fed the CON diet. Concerning Marvinbryantia, male pigs had a one percentage point higher relative abundance when fed the BP diet compared to males fed the CON diet (p < 0.001). Moreover, male pigs fed the BP diet had a 0.7 percentage points higher relative abundance of Marvinbryantia compared to the female pigs. Sex affected the relative abundance of certain genera with males having higher abundances of Blautia (1.9 percentage points, p = 0.001), Agathobacter (1.14 percentage points, p = 0.019), Solobacterium (0.64 percentage points, p = 0.011) and Ruminococcus gauvreauii group (0.27 percentage points, p = 0.038) than females. By contrast, females had higher abundances of Clostridium sensu stricto 1 (p = 0.034), Terrisporobacter (p = 0.037), HT002 (1.98 percentage points p = 0.012) and Turicibacter (0.56 percentage points, p = 0.050) than males. Pigs fed the BP diet had higher relative abundances of Blautia (1.38 percentage points, p = 0.012), Dorea (0.46 percentage points, p = 0.037), Collinsella (0.34 percentage points, p = 0.047), Eubacterium ruminantium group (0.5 percentage points, p = 0.049), Oribacterium (0.24 percentage points, p = 0.008) and Lachnospiraceae ND3007 group (0.44 percentage points, p < 0.001) compared to pigs fed the CON diet, whereas pigs fed the BP diet had a lower relative abundance of Streptococcus (2.54 percentage points, p = 0.001) and Roseburia (0.53 percentage points, p = 0.011) in their feces compared to pigs fed the CON diet.
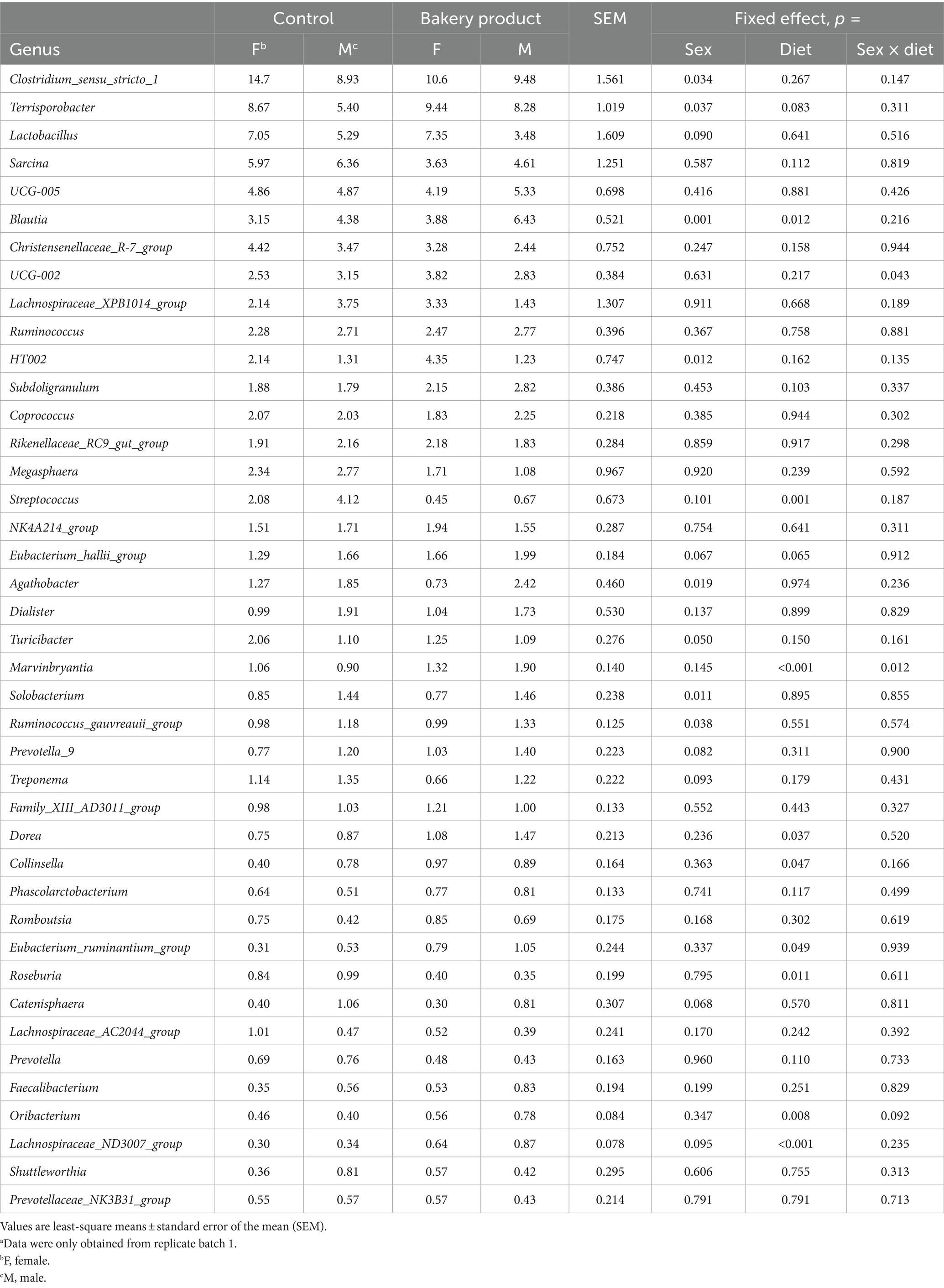
Table 7. Differences in the relative abundance (%) of most abundant genera in feces of pigs fed either the control diet or the diet containing bakery products.a
4 Discussion
Recycling of food leftovers, such as BP, for use in animal feed offers the opportunity to increase the sustainability of pig production by decreasing the feed-food-competition. One limiting factor for the utilization of BP in pig diets is the variability in the composition of the BP, which fluctuates depending on the daily returns from bakeries and supermarkets. In the present study, we used a BP diet composition that was already fed to fattening pigs on Austrian farms to simulate the commercial situation. Moreover, we opted for a high inclusion level to investigate whether BP can replace a reasonable proportion of cereals in pig diets. The dietary inclusion level is an important aspect, as only higher inclusion levels of BP result in a reduction of resource use, which is a key component when evaluation potential sustainable solutions to reduce the food-feed competition (Govoni et al., 2022). The present findings confirm our hypothesis that BP can replace cereals in pig diets by up to 30% without impairing animal performance, and even enhancing feed efficiency, animal product quality (i.e., n-3 FA concentration in neck and subcutaneous abdominal fat) and reducing feed costs. Moreover, our study showed that the present type of BP did not negatively impact the microbiota in the distal large intestine. In contrast, the BP inclusion increased the bacterial diversity, a proxy for gut homeostasis.
In accordance with our hypothesis, the overall growth performance of the fattening pigs was not affected by replacing 30% of the cereals in the diet with BP. This finding is in line with previous studies showing that feeding up to either 30% salty (bakery) or 30% sugary (confectionary) BP has no detrimental effects on performance of fattening pigs (Mazzoleni et al., 2023), as well as in other rearing phases, e.g., weaning (Tretola et al., 2019; Luciano et al., 2021; Luciano et al., 2022). Moreover, our findings demonstrate that including BP in the diet has the potential to enhance the feed efficiency (i.e., RFI), whereby the effect was more pronounced in male pigs. This finding matches with previous research indicating an improved FCR in piglets when fed 30% former foodstuffs, which comprised BP based on pasta, confectionary products but also wheat by-products and wheat flour (Tretola et al., 2019). The driver for improved feed efficiencies with BP feeding can be a higher digestibility of BP compared to cereals due to processing-related changes such as starch gelatinization and protein denaturation (Pinotti et al., 2021). In the present study, the ATTD of all key nutrients was not affected by the inclusion of BP. As the feed efficiency was improved, it may be assumed, although not measured, that the ileal digestibility of nutrients in the BP diet was higher compared to the CON diet. In anyway, higher feed efficiencies are advantageous both from an economic and an ecological point of view. First, the profitability of the individual farms increases when less feed is consumed, as feed accounts for the majority (50–85%) of the variable cost in pork production (McGlone and Pond, 2003; Mullan et al., 2011). Furthermore, a higher feed efficiency means less demand on global feed (and food) resources (Patience et al., 2015; Govoni et al., 2022). Therefore, in addition to avoiding competition between food and animal feed, a higher feed efficiency increases the sustainability of the use of BP. Regarding feed costs, replacement of cereals with BP also lowered feed costs per pig and the feed costs per kg of lean meat in the present study, which is mainly due to lower cost for BP compared to cereals. Summarizing, feeding BP in substitution of cereals did not impair animal performance but provided improvements in feed efficiency and economic aspects.
In addition to animal performance, the quality of the generated product, i.e., the pork, should not be affected negatively when using alternative feedstuffs such as BP. The analysis of FA in neck and subcutaneous abdominal fat showed that the content of n-3 fatty acids increased with BP in the diet. Next to C18:3 n-3 (alpha-linolenic acid) also the abundance of C20:3 n-3 (eicosatrienoic acid) and the sum of PUFA increased in the subcutaneous abdominal fat of the BP group. This finding contrasts the results of Tretola et al. (2024), who found less C18:3 n-3 and overall n-3 fatty acids in the backfat of pigs fed with the same inclusion level (30%) of sugary former food products. This difference was probably due to the fact that, despite the same BP inclusion level, the mixture of ingredients in the used BP (e.g., selected confectionary products vs. mixture of different bakery types such as bread, rolls and sweet pastries) and hence their chemical composition, i.e., the n-3 fatty acid content, was different. This shows the need for a better categorization of types of food products before mixing and using them in animal feed to allow predicting their effect on the animal product. In this respect, the higher concentration of C20:3 n-3 in the present study is valuable from a nutritional and health point of view for humans, as it exerts anti-inflammatory and fibrinolytic effects (Oltman et al., 1998; Spector et al., 2004; Yang et al., 2019). Additionally, alpha-linolenic acid represents an essential FA that serves as a precursor for the longer chain n-3 PUFA (Sinclair et al., 2002). With regard to health, the ratio of the intake of the equally essential n-6 FA to n-3 FA is particularly important. A high ratio of n-6/n-3 FA is considered a risk factor for the development of cardiovascular, inflammatory and autoimmune diseases, while a low ratio has an inhibitory effect (Simopoulos, 2008). As this ratio is very high in modern Western society due to its typical dietary conditions (Connor, 2000; Simopoulos, 2008; Calder, 2012), an increase in the proportion of n-3 FA in the particularly popular meat cuts neck and abdominal fat (i.e., bacon) is desirable as it would shift the n-6/n-3 FA ratio toward a lower and thus healthier ratio of FA for the consumer.
Contrasting to our hypothesis that the substitution of cereals with BP would not affect the microbiome composition and structure, alterations in the microbiome were observed due to BP feeding, which could be linked to feed efficiency (McCormack et al., 2017; Gardiner et al., 2020). First, along with the improved feed efficiency in BP-fed pigs, we observed an increase in the genera Dorea and Blautia in the feces. A positive correlation has recently been reported between both genera and feed efficiency, i.e., RFI and FCR, in pigs (Bergamaschi et al., 2020), but due to its magnitude (Spearman correlation coefficient 0.15) this relationship was rather weak (Chan, 2003; Akoglu, 2018). Second, alpha-diversity in the feces was higher in the BP group. A more diverse microbiome has previously been also connected to higher feed efficiency (Quan et al., 2018), but there is no general consensus but rather conflicting reports on the presence or absence of a correlation between bacterial diversity and feed efficiency (Gardiner et al., 2020). Nevertheless, a higher bacterial diversity in the gastrointestinal tract, as seen in our data, is generally considered beneficial (Gardiner et al., 2020) as it indicates a well-balanced microbial structure and hence confers more resilience to invasion on a microbial community (Levine and D’Antonio, 1999; Lozupone et al., 2012; Mancin et al., 2024), which may contribute to an improved feed efficiency. The beta-diversity in the feces also confirmed certain differences in the bacterial communities in the feces of CON and BP fed pigs, as well as between male and females. Whether the BP-related changes in the gut microbiome also influenced the n-3 FA concentrations in the neck and abdominal fat may have been possible either via modification of bile acids, synthesis of lipids in the small and large intestines, or by modifying the hepatic metabolism (Metzler-Zebeli, 2021). The fecal microbiome composition and the FA composition of the body fat were investigated in two different replicate batches. Therefore, it is difficult to deduce associations in the present study and should be investigated in future research.
Of note, although the butyrate concentration in the feces remained the same, we observed a shift within some butyrate-producing genera, i.e., Roseburia and Eubacterium ruminatium group, in pigs’ feces. The relative abundance of Roseburia declined, coincidently the abundance of Eubacterium ruminantium group increased. As we investigated the bacterial composition in feces, the change in Roseburia and Eubacterium may indicate changes in bacterial cross-feeding relationships (Dehority, 1991; de Vuyst and Leroy, 2011; Fu et al., 2019) and hence changes in the carbohydrate availability during the passage of digesta in the large intestine. Another butyrate-producing genus that was more present in the BP feeding was Oribacterium, which may also have been engaged in substrate exchange between the different (hemi-)cellulytic and saccharolytic producing genera to break down intermediary metabolites. The decrease in the relative abundance of Streptococcus can be interpreted as beneficial, as this genus harbors not only species that are considered physiological, but also pathogenic species (Krzyściak et al., 2013).
Another interesting finding of our study was the decrease in the absolute abundance of total fungi and yeasts in the BP fed pigs. This could possibly be attributed to the altered FA profiles in the BP diet, which unlike CON, contained the MCFA caprylic, lauric and myristic acid. These MCFA possess antimicrobial properties (López-Colom et al., 2019; Jackman et al., 2020), including a proven inhibitory action against fungi (Jia et al., 2020). The presence of these FA could therefore have caused – or at least contributed to – a reduction of fungi and yeasts in the feces of the pigs fed the BP diet in the present study. Although numerically inferior, fungi, alongside bacteria, are essential members and contributors to the gastrointestinal microbiome and both interact with each other in a complex interplay (Arfken et al., 2019; Summers and Arfken, 2022). The data on their absolute abundances may provide an indication about potentially beneficial effects by BP inclusion in pig diets. However, to substantiate the present findings, data on the fungal community composition should be generated in future studies to understand which taxa were suppressed. Overall, there were some interesting and beneficial effects of feeding BP on the fecal microbiome, and it can be speculated whether the changes within the microbiome (microbial structure and composition), e.g., triggered by a higher alpha-diversity or the reduction of fungi and yeasts may have contributed to the improved feed efficiency. It should be noted that the analysis of the absolute microbial abundances was only conducted in one replicate batch, which is why these measurements should be reproduced in future studies.
By analyzing the profile of microbial metabolites in feces, valuable information about the metabolic response of the intestinal microbiota toward nutritional influences can be derived (Rehman et al., 2007). Regarding this, an interesting finding of our study was a higher molar proportion of isobutyric acid in the feces of BP fed pigs. Two factors may have contributed to this finding. First, due to the potentially higher starch digestion, fewer carbohydrates may have reached the large intestine, resulting in a shift toward protein fermentation. Next to dietary carbohydrates, gastrointestinal microbiota also metabolize endogenous proteins, such as the glycoproteins from mucus (Macfarlane et al., 1992; Macfarlane and Macfarlane, 1995; Rist et al., 2013), from which branched-chain fatty acids such as isobutyrate and isovalerate are formed (Macfarlane and Macfarlane, 1995). Second, a higher molar proportion of isobutyric acid in the feces of BP fed pigs may indicate a lower enzymatic protein digestibility of the BP diet (Macfarlane et al., 1992), resulting that more dietary protein residues reach the large intestine (Tuohy et al., 2006). Causative for the lower protein digestibility could be Maillard reactions that are typically occurring in (thermally) processed foods, including BP (Starowicz and Zieliński, 2019), which are poorly digestible for the host animal. Considering the improved RFI in pigs fed the BP diet, the latter assumption probably only played a minor role. Lastly, it should be noted that measured concentrations of fecal SCFA are net sums which are influenced by digestion, production, absorption, and passage rate, it is difficult to make a clear statement about changes in their concentration. Analyzing ileal digestibility instead of ATTD in future studies could be helpful to shed more light on carbohydrate and protein digestibility of the BP.
In conclusion, the present study showed that cereals in the diets of pigs can be replaced by up to 30% by BP, without compromising growth performance and nutrient digestibility. Moreover, our findings demonstrated an improved feed efficiency (i.e., RFI) and meat quality in terms of the n-3 FA content of the neck and abdominal fat, respectively. The analysis of the intestinal microbiome pointed toward beneficial effects of BP feeding on the fecal microbiome, higher alpha-diversity and reduction of fungi and yeasts, which may have contributed to the improved feed efficiency in BP fed pigs. Taken together, the study improved the understanding on the use of BP as a sustainable substitute for cereals in pig feeding. Still, the chemical composition of BP and in consequence the obtained effects on animal performance and health can vary considerably, which is why further research on this topic is needed.
Data availability statement
The datasets presented in this study can be found in online repositories. The names of the repository/repositories and accession number(s) can be found at: https://www.ncbi.nlm.nih.gov/, PRJNA1122992.
Ethics statement
The experimental protocol was in compliance with the Good Scientific Practice guidelines of the institutional ethics committee of the University of Veterinary Medicine Vienna and national legislation (Protocol no. ETK-123/08/2021). The study was conducted in accordance with the local legislation and institutional requirements.
Author contributions
KH: Data curation, Formal analysis, Writing – original draft. JV: Investigation, Writing – review & editing. SK: Investigation, Methodology, Writing – review & editing. JK: Investigation, Writing – review & editing. SS: Data curation, Methodology, Writing – review & editing. DV: Investigation, Writing – review & editing. BM-Z: Conceptualization, Data curation, Formal analysis, Funding acquisition, Investigation, Writing – review & editing.
Funding
The authors received funding from the open access fund of the University of Veterinary Medicine Vienna.
Acknowledgments
The authors thank A. Geier and R. Retsch from Königshofer Futtermittel GmbH (Ebergassing, Austria) for providing the experimental diets including the BP. We also thank S. Posseth, T. Strini (VetFarm, Pig facility “Medau,” Pottenstein, Austria), E. Draxler (VetFarm, Dairy Farm “Kremesberg,” Pottenstein, Austria) and T. Schwarcz-Enzinger (Centre for Animal Nutrition and Welfare) for their support during the experiment. We are grateful for the assistance of C. Knecht, L. Schwarz (Clinical Centre for Population Medicine in Fish, Pig and Poultry) and F. Lerch (Centre for Veterinary Systems Transformation and Sustainability) during the invasive sampling in replicate batch 3.
Conflict of interest
The authors declare that the research was conducted in the absence of any commercial or financial relationships that could be construed as a potential conflict of interest.
Publisher’s note
All claims expressed in this article are solely those of the authors and do not necessarily represent those of their affiliated organizations, or those of the publisher, the editors and the reviewers. Any product that may be evaluated in this article, or claim that may be made by its manufacturer, is not guaranteed or endorsed by the publisher.
Supplementary material
The Supplementary material for this article can be found online at: https://www.frontiersin.org/articles/10.3389/fsufs.2024.1448608/full#supplementary-material
Footnotes
References
Akoglu, H. (2018). User’s guide to correlation coefficients. Turk. J. Emerg. Med. 18, 91–93. doi: 10.1016/j.tjem.2018.08.001
Arfken, A. M., Frey, J. F., Ramsay, T. G., and Summers, K. L. (2019). Yeasts of burden: exploring the Mycobiome-Bacteriome of the piglet GI tract. Front. Microbiol. 10:2286. doi: 10.3389/fmicb.2019.02286
Arosemena, A., DePeters, E. J., and Fadel, J. G. (1995). Extent of variability in nutrient composition within selected by-product feedstuffs. Anim. Feed Sci. Technol. 54, 103–120. doi: 10.1016/0377-8401(95)00766-G
Bergamaschi, M., Tiezzi, F., Howard, J., Huang, Y. J., Gray, K. A., Schillebeeckx, C., et al. (2020). Gut microbiome composition differences among breeds impact feed efficiency in swine. Microbiome 8:110. doi: 10.1186/s40168-020-00888-9
Bottje, W. G., and Carstens, G. E. (2009). Association of mitochondrial function and feed efficiency in poultry and livestock species. J. Anim. Sci. 87, E48–E63. doi: 10.2527/jas.2008-1379
Calder, P. C. (2012). Mechanisms of action of (n-3) fatty acids. J. Nutr. 142, 592S–599S. doi: 10.3945/jn.111.155259
Cederberg, C., Gustavsson, J., Meybeck, A., Sonesson, U., and van Otterdijik, R. (2011). Global food losses and food waste: Extent, causes and prevention. Rome: Food and Agriculture Organization of the United Nations.
Connor, W. E. (2000). Importance of n-3 fatty acids in health and disease. Am. J. Clin. Nutr. 71, 171S–175S. doi: 10.1093/ajcn/71.1.171S
de Vuyst, L., and Leroy, F. (2011). Cross-feeding between bifidobacteria and butyrate-producing colon bacteria explains bifdobacterial competitiveness, butyrate production, and gas production. Int. J. Food Microbiol. 149, 73–80. doi: 10.1016/j.ijfoodmicro.2011.03.003
Dehority, B. A. (1991). Effects of microbial synergism on fibre digestion in the rumen. Proc. Nutr. Soc. 50, 149–159. doi: 10.1079/PNS19910026
FAO - Food and Agriculture Organization of the United Nations (2019). State of food and agriculture 2019: Moving forward on food loss and waste reduction. Rome: Food & Agriculutre ORG.
Folch, J., Lees, M., and Stanley, G. S. (1957). A simple method for the isolation and purification of total lipides from animal tissues. J. Biol. Chem. 226, 497–509. doi: 10.1016/s0021-9258(18)64849-5
França, A. B., Morenz, M. J. F., Lopes, F. C. F., Madeiro, A. S., Morenz, D. A., De Faria, B. M., et al. (2012). Bakery waste in sheep diets: intake, digestibility, nitrogen balance and ruminal parameters. R. Bras. Zootec. 41, 147–153. doi: 10.1590/S1516-35982012000100022
Fu, X., Liu, Z., Zhu, C., Mou, H., and Kong, Q. (2019). Nondigestible carbohydrates, butyrate, and butyrate-producing bacteria. Crit. Rev. Food Sci. Nutr. 59, S130–S152. doi: 10.1080/10408398.2018.1542587
Fung, L., Urriola, P. E., Baker, L., and Shurson, G. C. (2019). Estimated energy and nutrient composition of different sources of food waste and their potential for use in sustainable swine feeding programs. Trans. Anim. Sci. 3, 359–368. doi: 10.1093/tas/txy099
Gardiner, G. E., Metzler-Zebeli, B. U., and Lawlor, P. G. (2020). Impact of intestinal microbiota on growth and feed efficiency in pigs: a review. Microorganisms 8:1886. doi: 10.3390/microorganisms8121886
Godfray, H. C. J., Beddington, J. R., Crute, I. R., Haddad, L., Lawrence, D., Muir, J. F., et al. (2010). Food security: the challenge of feeding 9 billion people. Science 327, 812–818. doi: 10.1126/science.1185383
Golay, P.-A., and Moulin, J. (2016). Determination of labeled fatty acids content in Milk products, infant formula, and adult/pediatric nutritional formula by capillary gas chromatography: collaborative study, final action 2012.13. J. AOAC Int. 99, 210–222. doi: 10.5740/jaoacint.15-0140
Govoni, C., Chiarelli, D. D., Luciano, A., Pinotti, L., and Rulli, M. C. (2022). Global assessment of land and water resource demand for pork supply. Environ. Res. Lett. 17, 074003–074015. doi: 10.1088/1748-9326/ac74d7
Govoni, C., D'Odorico, P., Pinotti, L., and Rulli, M. C. (2023). Preserving global land and water resources through the replacement of livestock feed crops with agricultural by-products. Nat. Food 4, 1047–1057. doi: 10.1038/s43016-023-00884-w
Humer, E., Aditya, S., Kaltenegger, A., Klevenhusen, F., Petri, R. M., and Zebeli, Q. (2018). Graded substitution of grains with bakery by-products modulates ruminal fermentation, nutrient degradation, and microbial community composition in vitro. J. Dairy Sci. 101, 3085–3098. doi: 10.3168/jds.2017-14051
Jackman, J. A., Boyd, R. D., and Elrod, C. C. (2020). Medium-chain fatty acids and monoglycerides as feed additives for pig production: towards gut health improvement and feed pathogen mitigation. J. Anim. Sci. Biotechnol. 11:44. doi: 10.1186/s40104-020-00446-1
Jia, M., Zhang, Y., Gao, Y., and Ma, X. (2020). Effects of medium chain fatty acids on intestinal health of Monogastric animals. Curr. Protein Pept. Sci. 21, 777–784. doi: 10.2174/1389203721666191231145901
Kaltenegger, A., Humer, E., Pacífico, C., and Zebeli, Q. (2021). Feeding dairy cows bakery by-products enhanced nutrient digestibility, but affected fecal microbial composition and pH in a dose-dependent manner. J. Dairy Sci. 104, 7781–7793. doi: 10.3168/jds.2020-19998
Krzyściak, W., Pluskwa, K. K., Jurczak, A., and Kościelniak, D. (2013). The pathogenicity of the Streptococcus genus. Eur. J. Clin. Microbiol. Infect. Dis. 32, 1361–1376. doi: 10.1007/s10096-013-1914-9
Lerch, F., Vötterl, J. C., Schwartz-Zimmermann, H. E., Sassu, E. L., Schwarz, L., Renzhammer, R., et al. (2022). Exposure to plant-oriented microbiome altered jejunal and colonic innate immune response and barrier function more strongly in suckling than in weaned piglets. J. Anim. Sci. 100:skac310. doi: 10.1093/jas/skac310
Lerch, F., Yosi, F., Vötterl, J. C., Koger, S., Ehmig, J., Sharma, S., et al. (2023). An insight into the temporal dynamics in the gut microbiome, metabolite signaling, immune response, and barrier function in suckling and weaned piglets under production conditions. Front. Vet. Sci. 10:1184277. doi: 10.3389/fvets.2023.1184277
Levine, J. M., and D’Antonio, C. M. (1999). Elton revisited: a review of evidence linking diversity and Invasibility. Oikos 87:15. doi: 10.2307/3546992
López-Colom, P., Castillejos, L., Rodríguez-Sorrento, A., Puyalto, M., Mallo, J. J., and Martín-Orúe, S. M. (2019). Efficacy of medium-chain fatty acid salts distilled from coconut oil against two enteric pathogen challenges in weanling piglets. J. Anim. Sci. Biotechnol. 10:89. doi: 10.1186/s40104-019-0393-y
Lozupone, C. A., Stombaugh, J. I., Gordon, J. I., Jansson, J. K., and Knight, R. (2012). Diversity, stability and resilience of the human gut microbiota. Nature 489, 220–230. doi: 10.1038/nature11550
Luciano, A., Espinosa, C. D., Pinotti, L., and Stein, H. H. (2022). Standardized total tract digestibility of phosphorus in bakery meal fed to pigs and effects of bakery meal on growth performance of weanling pigs. Anim. Feed Sci. Technol. 284:115148. doi: 10.1016/j.anifeedsci.2021.115148
Luciano, A., Tretola, M., Mazzoleni, S., Rovere, N., Fumagalli, F., Ferrari, L., et al. (2021). Sweet vs. salty former food products in post-weaning piglets: effects on growth, apparent Total tract digestibility and blood metabolites. Animals 11:3315. doi: 10.3390/ani11113315
Macfarlane, G. T., Gibson, G. R., Beatty, E., and Cummings, J. H. (1992). Estimation of short-chain fatty acid production from protein by human intestinal bacteria based on branched-chain fatty acid measurements. FEMS Microbiol. Lett. 101, 81–88. doi: 10.1111/j.1574-6968.1992.tb05764.x
Macfarlane, S., and Macfarlane, G. T. (1995). “Proteolysis and amino acid fermentation” in Human colonic bacteria: Role in nutrition, physiology, and pathology. eds. G. R. Gibson and G. T. Macfarlane (Boca Raton, Florida: CRC Press), 75–100.
Makkar, H. P. S. (2018). Review: feed demand landscape and implications of food-not feed strategy for food security and climate change. Animal 12, 1744–1754. doi: 10.1017/S175173111700324X
Mancin, E., Maltecca, C., Huang, Y. J., Mantovani, R., and Tiezzi, F. (2024). A first characterization of the microbiota-resilience link in swine. Microbiome 12:53. doi: 10.1186/s40168-024-01771-7
Mazzoleni, S., Tretola, M., Luciano, A., Lin, P., Pinotti, L., and Bee, G. (2023). Sugary and salty former food products in pig diets affect energy and nutrient digestibility, feeding behaviour but not the growth performance and carcass composition. Animal 17:101019. doi: 10.1016/j.animal.2023.101019
McCormack, U. M., Curião, T., Buzoianu, S. G., Prieto, M. L., Ryan, T., Varley, P., et al. (2017). Exploring a possible link between the intestinal microbiota and feed efficiency in pigs. Appl. Environ. Microbiol. 83:e00380-17. doi: 10.1128/AEM.00380-17
McGlone, J., and Pond, W. G. (2003). Pig production: Biological principles and applications. Australia, Clifton Park, NY: Cengage Learning.
McMurdie, P. J., and Holmes, S. (2013). Phyloseq: an R package for reproducible interactive analysis and graphics of microbiome census data. PLoS One 8:e61217. doi: 10.1371/journal.pone.0061217
Metzler-Zebeli, B. U. (2021). The role of dietary and microbial fatty acids in the control of inflammation in neonatal piglets. Animals (Basel) 11:2781. doi: 10.3390/ani11102781
Metzler-Zebeli, B. U., Klinsoda, J., Vötterl, J., Sharma, S., Koger, S., and Sener-Aydemir, A. (2021). Short-, medium-, and long-chain fatty acid profiles and signaling is responsive to dietary phytase and lactic acid treatment of cereals along the gastrointestinal tract of growing pigs. J. Anim. Sci. 99:skab117. doi: 10.1093/jas/skab117
Metzler-Zebeli, B. U., Newman, M. A., Ladinig, A., Kandler, W., Grüll, D., and Zebeli, Q. (2019). Transglycosylated starch accelerated intestinal transit and enhanced bacterial fermentation in the large intestine using a pig model. Br. J. Nutr. 122, 1–13. doi: 10.1017/S0007114519000849
Mullan, B. P., Moore, K. L., Payne, H. G., Trezona-Murray, M., Pluske, J. R., and Kim, J. C. (2011). Feed efficiency in growing pigs–what’s possible? Recent Adv. Anim. Nutr. 18, 17–22.
National Research Council (2012). Nutrient requirements of swine. 10th Edn. Washington, DC: National Academy Press.
Naumann, C., and Bassler, R. (2012). Methodenbuch, Band III: Die chemische Untersuchung von Futtermitteln. Darmstadt: VDLUFA-Verlag.
Oksanen, J., Blanchet, F. G., Friendly, M., Kindt, R., Legendre, P., McGlinn, D., et al. (2022). Vegan: Community ecology package R package.
Oltman, C. L., Weintraub, N. L., VanRollins, M., and Dellsperger, K. C. (1998). Epoxyeicosatrienoic acids and dihydroxyeicosatrienoic acids are potent vasodilators in the canine coronary microcirculation. Circ. Res. 83, 932–939. doi: 10.1161/01.res.83.9.932
Patience, J. F., Rossoni-Serão, M. C., and Gutiérrez, N. A. (2015). A review of feed efficiency in swine: biology and application. J. Anim. Sci. Biotechnol. 6:33. doi: 10.1186/s40104-015-0031-2
Pinotti, L., Ferrari, L., Fumagalli, F., Luciano, A., Manoni, M., Mazzoleni, S., et al. (2023). Review: pig-based bioconversion: the use of former food products to keep nutrients in the food chain. Animal 17:100918. doi: 10.1016/j.animal.2023.100918
Pinotti, L., Luciano, A., Ottoboni, M., Manoni, M., Ferrari, L., Marchis, D., et al. (2021). Recycling food leftovers in feed as opportunity to increase the sustainability of livestock production. J. Clean. Prod. 294:126290. doi: 10.1016/j.jclepro.2021.126290
Quan, J., Cai, G., Ye, J., Yang, M., Ding, R., Wang, X., et al. (2018). A global comparison of the microbiome compositions of three gut locations in commercial pigs with extreme feed conversion ratios. Sci. Rep. 8:4536. doi: 10.1038/s41598-018-22692-0
Quast, C., Pruesse, E., Yilmaz, P., Gerken, J., Schweer, T., Yarza, P., et al. (2013). The SILVA ribosomal RNA gene database project: improved data processing and web-based tools. Nucleic Acids Res. 41, D590–D596. doi: 10.1093/nar/gks1219
Rehman, H. U., Vahjen, W., Awad, W. A., and Zentek, J. (2007). Indigenous bacteria and bacterial metabolic products in the gastrointestinal tract of broiler chickens. Arch. Anim. Nutr. 61, 319–335. doi: 10.1080/17450390701556817
Rist, V. T. S., Weiss, E., Eklund, M., and Mosenthin, R. (2013). Impact of dietary protein on microbiota composition and activity in the gastrointestinal tract of piglets in relation to gut health: a review. Animal 7, 1067–1078. doi: 10.1017/S1751731113000062
Simopoulos, A. P. (2008). The importance of the omega-6/omega-3 fatty acid ratio in cardiovascular disease and other chronic diseases. Exp. Biol. Med. (Maywood) 233, 674–688. doi: 10.3181/0711-MR-311
Sinclair, A. J., Attar-Bashi, N. M., and Li, D. (2002). What is the role of alpha-linolenic acid for mammals? Lipids 37, 1113–1123. doi: 10.1007/s11745-002-1008-x
Spector, A. A., Fang, X., Snyder, G. D., and Weintraub, N. L. (2004). Epoxyeicosatrienoic acids (EETs): metabolism and biochemical function. Prog. Lipid Res. 43, 55–90. doi: 10.1016/S0163-7827(03)00049-3
Starowicz, M., and Zieliński, H. (2019). How Maillard reaction influences sensorial properties (color, flavor and texture) of food products? Food Rev. Int. 35, 707–725. doi: 10.1080/87559129.2019.1600538
Summers, K. L., and Arfken, A. M. (2022). “The gut Mycobiome and animal health” in Gut microbiota, immunity, and health in production animals. eds. M. H. Kogut and G. Zhang (Cham: Springer International Publishing), 85–125.
Tretola, M., Ferrari, L., Luciano, A., Mazzoleni, S., Rovere, N., Fumagalli, F., et al. (2022). Sugary vs salty food industry leftovers in postweaning piglets: effects on gut microbiota and intestinal volatile fatty acid production. Animal 16:100584. doi: 10.1016/j.animal.2022.100584
Tretola, M., Mazzoleni, S., Silacci, P., Dubois, S., Proserpio, C., Pagliarini, E., et al. (2024). Sustainable pig diets: partial grain replacement with former food products and its impact on meat quality. J. Anim. Sci. 102:skae070. doi: 10.1093/jas/skae070
Tretola, M., Ottoboni, M., Luciano, A., Rossi, L., Baldi, A., and Pinotti, L. (2019). Former food products have no detrimental effects on diet digestibility, growth performance and selected plasma variables in post-weaning piglets. Ital. J. Anim. Sci. 18, 987–996. doi: 10.1080/1828051X.2019.1607784
Tuohy, K. M., Hinton, D. J. S., Davies, S. J., Crabbe, M. J. C., Gibson, G. R., and Ames, J. M. (2006). Metabolism of Maillard reaction products by the human gut microbiota—implications for health. Mol. Nutr. Food Res. 50, 847–857. doi: 10.1002/mnfr.200500126
van Soest, P. J., Robertson, J. B., and Lewis, B. A. (1991). Methods for dietary fiber, neutral detergent fiber, and nonstarch polysaccharides in relation to animal nutrition. J. Dairy Sci. 74, 3583–3597. doi: 10.3168/jds.S0022-0302(91)78551-2
Keywords: pigs, bakery products, microbiome, animal nutrition, feed efficiency
Citation: Hartinger K, Vötterl JC, Koger S, Klinsoda J, Sharma S, Verhovsek D and Metzler-Zebeli BU (2024) Feeding of bakery products as replacement of cereal grains alter fecal microbiome and improve feed efficiency in fattening pigs. Front. Sustain. Food Syst. 8:1448608. doi: 10.3389/fsufs.2024.1448608
Edited by:
Muhammad Akbar Shahid, Bahauddin Zakariya University, PakistanReviewed by:
Claudia Terezia Socol, University of Oradea, RomaniaDoriana Eurosia Angela Tedesco, University of Milan, Italy
Copyright © 2024 Hartinger, Vötterl, Koger, Klinsoda, Sharma, Verhovsek and Metzler-Zebeli. This is an open-access article distributed under the terms of the Creative Commons Attribution License (CC BY). The use, distribution or reproduction in other forums is permitted, provided the original author(s) and the copyright owner(s) are credited and that the original publication in this journal is cited, in accordance with accepted academic practice. No use, distribution or reproduction is permitted which does not comply with these terms.
*Correspondence: Barbara U. Metzler-Zebeli, barbara.metzler@vetmeduni.ac.at