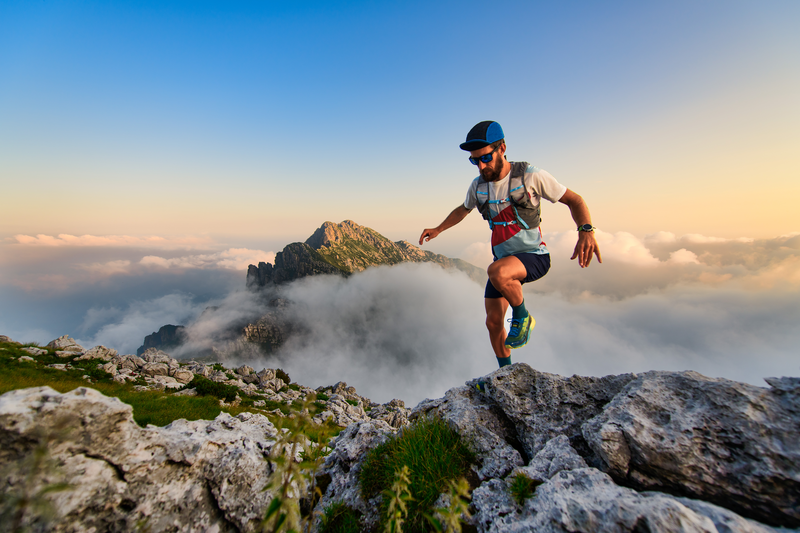
95% of researchers rate our articles as excellent or good
Learn more about the work of our research integrity team to safeguard the quality of each article we publish.
Find out more
ORIGINAL RESEARCH article
Front. Sustain. Food Syst. , 13 November 2024
Sec. Crop Biology and Sustainability
Volume 8 - 2024 | https://doi.org/10.3389/fsufs.2024.1446308
This article is part of the Research Topic Abiotic Stresses in Field Crops: Response, Impacts and Management under Climate Change Scenario View all 15 articles
Cypermethrin, a synthetic pyrethroid, is a widely employed pesticide in large-scale commercial agriculture and domestic settings to control pests and boost crop yields. Despite its effectiveness in controlling pests, concerns persist about the potential ecological and human health impacts associated with its use. In the present study, the impact of cypermethrin on different parameters, including growth indices, pollen morphology and pollen fertility, chlorophyll and carotenoid content, hydrogen peroxide (H2O2) scavenging, lipid peroxidation (MDA concentration), superoxide dismutase (SOD), and catalase (CAT) activities, was investigated in Cicer arietinum L. The results showed that plants exhibited decreasing trends in plant height, number of branches, pods, and seeds per plant as cypermethrin concentrations increased. These changes resulted in significant reductions in the overall yield of the plants. The percentage of wrinkled pollen significantly increased with higher concentrations of cypermethrin and longer exposure durations. Exposure to cypermethrin showed significant variations in pollen fertility of C. arietinum at different concentrations and time intervals in comparison to control, which had a baseline pollen fertility of 79.12% ± 4.59. Chlorophyll and carotenoid content of C. arietinum were considerably affected by cypermethrin, indicative of potential disturbances in essential photosynthetic processes crucial for plant health. Further, with increasing concentrations of cypermethrin ranging from 100 mg/L to 500 mg/L, noticeable effects were observed on H2O2− scavenging, MDA concentration, SOD and CAT activities of C. arietinum in dose-dependent manner. Further, it was found that the duration of exposure and concentration of cypermethrin played a crucial role in exacerbating these detrimental effects. The findings of the present study raise concerns regarding the harmful effects of agricultural pesticides like cypermethrin and highlight the need for more research on the nutritional value of products derived from plants and seeds exposed to these agents.
The world is grappling with the dual challenge of a rapidly growing population and diminishing food resources, prompting global concerns (Ridwan et al., 2022; Rajak et al., 2023). The intensified development of the global economy has resulted in a surge in pesticide production and usage. In agriculture, pesticides are common chemical substances that are used to eradicate weeds, insects, and other pests (Sharma et al., 2020; Kenko et al., 2023; Gautam et al., 2023). They are an essential component of modern agricultural practices and play a crucial role in safeguarding crops and enhancing yields, as approximately 45% of annual food production is lost due to pest invasions (Borowik et al., 2023a,b; Skubała et al., 2024). However, the widespread use of pesticides poses significant threats to ecosystems, human health, and other biotic elements of the environment (Khan et al., 2023; Liess and Gröning, 2024). Cypermethrin is a widely employed pesticide in large-scale commercial agriculture and domestic settings to control pests and boost crop yields (Nath et al., 2024). Classified as an extremely effective synthetic type II pyrethroid, cypermethrin is recognized as a valuable pesticide in agricultural, horticultural, and aquatic systems, having comparatively less noxiousness to non-target animals (Liu et al., 2009; Baruah et al., 2024). Cypermethrin has very low vapor pressure and solubility in water, but it is highly soluble in a wide range of organic solvents. It primarily targets sodium channels and adenosine triphosphatase in pests (Çavuşoğlu, 2011). Despite its effectiveness, concerns persist about the potential ecological and human health impacts associated with the use of cypermethrin and similar pesticides (Çavuşoğlu, 2011). Methods for the determination of residues of cypermethrin in foods and in the environment are well established. In most substrates, the practical limit of determination of is 0.01 mg/kg (World Health Organization, 1989).
Several crop plants, like Crepis capillaries, Vicia faba, Hordeum vulgare, Pisum sativum, Allium cepa, and Cicer arietinum, have been identified as bioindicators for evaluating the biological effects of chemicals from diverse sources (Sheikh et al., 2020; Hoseiny-Rad and Aivazi, 2020). Chickpea (Cicer arietinum L.) stands out as the second most cultivated legume globally, following soybeans (Pang et al., 2017). Although estimates vary, Nedumaran et al. (2015) reported cultivation at 11 million hectares with a production of 8 million tons, positioning chickpea as the third most crucial food legume ever grown. Beyond its role as a significant food source for both humans and animals, chickpea contributes substantially to soil fertility conservation, especially in dry, rain-fed regions, through symbiotic nitrogen fixation (Sharma, 2012; Angaji et al., 2013). However, chickpea faces challenges due to its limited competitiveness with weeds, attributed to its sluggish growth and constrained development of leaf area in the initial phases of growth (Hoseiny-Rad and Aivazi, 2020).
Previous research has explored the effects of various pesticides on plants, such as the accumulation of atrazine in rice plants, leading to toxic reactions through the over-formation of reactive oxygen species (ROS) and activation of plant safety mechanisms (Zhang et al., 2014). Yin et al. (2008) found that isoproturon delayed wheat plant growth and impacted various physiological processes, inducing oxidative stress. In our current investigation, we examined the impact of cypermethrin on different parameters in chickpea plants. This involved analyzing growth patterns, pollen fertility, and pollen morphology, as well as investigating potential alterations in the antioxidant structure and photosynthetic procedure in cypermethrin-induced stress. We specifically assessed hydrogen peroxide (H2O2) -scavenging, malondialdehyde (MDA) levels, catalase (CAT), and superoxide dismutase (SOD) activity, in addition to chlorophyll and carotenoid levels. Our primary objective was to develop a comprehensive understanding of how cypermethrin influences critical aspects of Cicer arietinum development and biochemistry.
Cypermethrin (CAS No. 52315-07-8) was purchased from Sigma Chemicals Ltd., USA. Cicer arietinum seeds were obtained from a certified dealer in Abha, Aseer Region, KSA.
The studies were carried out in the Department of Botany, Science College, Alfarra Campus, King Khalid University, Abha, Saudi Arabia. Abha is 2,270 meters above sea level and situated in the southern region of Saudi Arabia, near Aseer. Abha's high elevation gives it a semi-arid climate. Abha, Asir, Saudi Arabia is located at latitude 18.216797 and longitude 42.503765.
Fresh and vigorous seeds were surface sterilized using a 5% NaOCl solution for 10 min. Post-sterilization, the seeds were carefully rinsed multiple times with distilled water. Subsequently, the seeds were divided into distinct groups, each comprising 100 seeds. The experimental treatments involved subjecting the seeds to five different concentrations (100 mg/L, 200 mg/L, 300 mg/L, 400 mg/L and 500 mg/L) of a 250 ml cypermethrin solution, with exposure durations of 1 and 3 h. For control group (0) 100 seeds were treated with distilled water. Following treatment, the seeds were permitted to germinate on petri plates containing damp cotton, maintained at a controlled temperature in the dark for 24 h. The germination status of the seeds was then assessed, and the resulting sprouts were utilized for subsequent growth and yield investigations. Additionally, another set of seeds for each concentration was planted in earthen pots to facilitate biochemical studies. These seeds were grown in greenhouse conditions. The temperature and humidity in a greenhouse were 64 to 75°F and approximately 80%, respectively. In parallel, plants in the control group, devoid of any exposure, were cultivated to serve as a basis for comparative analysis. The entire experiment was replicated three times under consistent conditions to ensure the reliability and repeatability of the results.
Growth parameters were examined on 14-day-old seedlings. The studied growth parameters include Plant height, number of branches, number and length of pods, number of seeds per pod, along with their weights and total yield.
An examination of 100 pollens from each treatment was conducted to assess fertility, pollen morphology, and shape. The pollens were categorized based on their shape and dimensions in equatorial and polar views, following the classification method proposed by Erdtman (1966). For determining pollen viability, a total of 100 pollen grains from each group were observed under a light microscope. The viability level was assessed by a solution of 2, 3, 5-triphenyl tetrazolium chloride (TTC) (Norton, 1966).
To conduct this viability test, a drop of TTC solution was kept on a slide, and pollens were spread using a brush, followed by the placement of a cover slip. After the application of TTC, counting was done, and the pollens were categorized into three sets based on staining density. Pollen exhibiting a dark red stain were considered viable; those with a light red stain were categorized as semi-viable; and unstained pollens were classified as non-viable, following the criteria established by Eti (1991) and Stosser (1984).
The chlorophyll estimation was carried out using the Acetone method, following Arnon (1949). Chlorophyll extraction was performed in 80% acetone, and absorbance was measured at wavelengths of 663 nm and 645 nm. Chlorophyll and carotenoid content were calculated by Khan et al. (2019).
The assessment of hydrogen peroxide scavenging activity was conducted following the technique stated by Ruch et al. (1989), employing spectrophotometry at 230 nm. Leaf extracts in methanol were prepared at 100 μg/mL concentration and then mixed with a H2O2 solution (0.6 mL; 40 mM) in phosphate buffer (PB) having a pH of 7.4. The volume of the final solution was adjusted to 3 mL, and the mixture was kept in incubation for 10 min. PB solution with no H2O2 was kept as a reference. At 230 nm, absorbance was measured for all compounds. The degree of H2O2 scavenging by plant extracts was subsequently calculated by Khan et al. (2019).
Rao and Sresty (2000) were followed for the estimation of lipid peroxidation. 0.2 g of frozen leaf sections were homogenized in 2 mL of 0.1% trichloroacetic acid (TCA) and subjected to centrifugation at 10,000 rpm for 10 min to precipitate the remains. Supernatant aliquots were then added to 4 mL of 20% TCA with 0.5% thiobarbituric acid and 100 μL of butylated hydroxyl toluene in 4% ethanol. The mixture was incubated for 1 h at 90°C, later cooled in an ice bath and subsequently centrifuged at 10,000 rpm for 5 min. The supernatant and the unspecific turbidity of the sample were measured at 532 nm and 600 nm, respectively. This turbidity value was subtracted from the absorbance at 532 nm. A solution of 0.25% TBA in 10% TCA served as a blank reference. The content of TBA reactive substances was depicted as nmol/g fresh weight by means of an extinction coefficient of 155 mM−1 cm−1.
Total CAT activity was examined by quantifying the intake of H2O2 at 240 nm, following the technique described by Gallego et al. (1996). The enzymatic actions of both SOD and CAT were stated as units per milligram of protein (U mg−1 protein). The measurement of SOD activity was conducted following Khan et al. (2019) with slight modifications.
One way ANOVA using SPSS software (version 16.0, SPSS Inc., Chicago, IL, USA) was applied to find the significance of differences in variables. The changes were considered statistically significant at p < 0.05. All the outcomes are expressed as mean ± standard error.
Table 1, Figure 1 show the impact of diverse cypermethrin concentrations from 100 mg/L to 500 mg/L on several growth parameters of C. arietinum. In both 1 and 3 h exposures to increasing concentrations of cypermethrin, noticeable adverse effects were observed on various growth parameters of C. arietinum. Relative to the control group, the plants exhibited decreasing trends in plant height (21.04% in 1 h; 25.32% in 3 h), branches (31.48% in 1 h; 37.23% in 3 h), pods (13.87% in 1 h; 17.33% in 3 h), and seeds per plant (40.45% in 1 h; 44.87% in 3 h), as cypermethrin concentrations increased. These changes resulted in significant reductions in the overall yield of the plants (14.95% in 1 h; 40.53% in 3 h). Furthermore, negative consequences extended to individual seed quality, as evidenced by a decrease in pod length (72.54% in 1 h; 64.07% in 3 h) and the weight of seeds (14.61% in 1 h; 10.24% in 3 h).
Cypermethrin has a notable detrimental effect on the pollen morphology of C. arietinum, as evidenced by increased wrinkled pollen, a higher proportion of pollen without visible pores, and an elevated occurrence of abnormal pollen shapes. Table 2, Figure 2 illustrate the impact of various concentrations of cypermethrin (100 mg/L to 500 mg/L) and different exposure durations (1 h and 3 h) on the pollen morphology of C. arietinum. In comparison to control, the percentage of wrinkled pollen significantly increased with higher concentrations of cypermethrin (with 3.71 ± 1.47 in control group to 40.35 ± 4.58; p < 0.01 at 500 mg/L for 1 h) and longer exposure durations (with 2.77 ± 1.27 in control to 31.75 ± 5.37; p < 0.01 at 500 mg/L for 3 h). This is evident in all treated groups, indicating a dose-dependent and time-dependent effect on pollen morphology. Treatment with cypermethrin led to a significant rise in the percentage of pollen without visible pores. This effect was more pronounced with increased concentrations and exposure durations (with 2.54 ± 1.45 at control to 26.55 ± 3.39; at 500 mg/L for 1 h and, with 2.78 ± 0.91 at control to 51.37 ± 4.53; at 500 mg/L for 3 h) highlighting the adverse impact of cypermethrin on the structural integrity of pollen grains. Similarly, the percentage of pollen with abnormal shapes displayed a significant rise in relation to cypermethrin treatment in Table 2.
Figure 2. Impact on pollen morphology of C. arietinum treated with cypermethrin for 1 and or 3 h in PMCs. (A) Wrinkled pollen grain; (B, C) Pollen without visible pore; (D) Pollen with abnormal shape. Scale bars = 10 μM; bars = 10 μM.
Exposure to cypermethrin showed significant variations in pollen fertility of C. arietinum at different concentrations and time intervals in comparison to the control, which had a baseline pollen fertility of 79.12% ± 4.59. After a 1 h exposure, lower concentrations of cypermethrin slightly increased the pollen fertility of C. arietinum compared to the control, with concentrations of 100 mg/L and 200 mg/L resulting in increased pollen fertility to 84.54% ± 6.23 and 80.14% ± 5.73, respectively. However, higher concentrations exhibited a significant decline in the fertility of pollens, with 500 mg/L showing the maximum reduction in the fertility of pollens with a pollen fertility of 65.57% ± 4.32 (p < 0.01). In the 3 h exposure, a similar but non-significant pattern was observed. It was observed that the two-time intervals (1 h and 3 h) revealed distinct effects of cypermethrin on the pollen fertility of C. arietinum. For instance, after 1 h of exposure, a concentration of 300 mg/L demonstrated a significant reduction in pollen fertility to 73.12% ± 4.42, whereas at 3 h of exposure with the same concentration, a pollen fertility rate of 70.78% ± 6.12 was observed, and similar trends were observed for other concentrations as well (Figures 3, 4). The decrease in the percentage of fertile pollen with increasing dosage is likely attributable to the toxic effects of cypermethrin on pollen.
Figure 3. Effect of cypermethrin on pollen fertility C. arietinum for 1 and 3 h.cp < 0.05; bp < 0.01 compared to control group. Data are mean of three replicates ±SE, 0 = Control group.
Figure 4. Impact on pollen fertility of C. arietinum treated with cypermethrin for 1 and 3 h in PMCs. (A) Fertile pollen grain in PMCs; (B) Sterile pollen grain in PMCs of C. arietinum; bars =10 μM.
Chlorophyll (Figure 5A) and carotenoid content (Figure 5B) of C. arietinum were considerably affected by cypermethrin, indicative of potential disturbances in essential photosynthetic processes crucial for plant health. Upon exposure to cypermethrin for 1 h, a visible dose-dependent response was observed with a 12.5% decrease at 500 mg/L in comparison to the control. Overall, a statistically significant reduction in chlorophyll content was noted as the concentration of cypermethrin increased in Figure 5A. Concentrations equal to or exceeding 200 mg/L exhibited a noteworthy decline in chlorophyll content compared to the control group. Similarly, after a 3 h exposure, a dose-dependent decrease in chlorophyll content was evident, with all concentrations ranging from 100 mg/L to 500 mg/L displaying statistically significant reductions compared to the control, with an 18.75% reduction at 500 mg/L in Figure 5A. These findings emphasize the concentration-dependent and exposure duration-dependent impact of cypermethrin on the chlorophyll content of C. arietinum.
Figure 5. Effect of Cypermethrin on chlorophyll (A) and carotenoids (B) on C. arietinum for 1 and 3 h. cp < 0.05; bp < 0.01 compared to control group. Data are mean of three replicates ±SE, 0 = Control group.
Cypermethrin exposure also adversely affected the carotenoid content of C. arietinum indicating potential disruptions in essential pigment concentrations crucial for plant health. Following a 1 h exposure, a concentration dependent decrease in carotenoid content was observed, with a 58.9% decrease at 500 mg/L Similarly, after a 3 h exposure, a concentration-dependent decline in carotenoid content was evident across all concentrations compared to the control group, with a 54.1% decrease at 500 mg/L. The consistent and statistically significant differences highlight the concentration-dependent and exposure duration-dependent effects of cypermethrin on the carotenoid content of the plant in Figure 5B.
Figures 6A–D show the effect of different cypermethrin concentrations from 100 mg/L to 500 mg/L on various biochemical parameters such as H2O2 scavenging (Figure 6A), lipid peroxidation concentration (MDA; Figure 6B), superoxide dismutase (SOD) activity (Figure 6C) and catalase (CAT) activity (Figure 6D) on C. arietinum for 1 and 3 h. In both 1 and 3 h exposures to increasing concentrations of cypermethrin ranging from 100 mg/L to 500 mg/L, noticeable enhanced effects were observed on various parameters such as hydrogen peroxide scavenging, MDA, SOD and CAT activity of C. arietinum in a dose-dependent manner, which is very significantly increased (p < 0.01) when compared to control.
Figure 6. Effect of cypermethrin on Hydrogen peroxide scavenging (A), lipid peroxidation (B), SOD (C) and CAT (D) activities, on C. arietinum for 1 and 3 h. cp < 0.05; bp < 0.01 compared to control group. Data are mean of three replicates ± SE, 0 = Control group.
This implies that cypermethrin not only impacts the overall productivity of the plants but also affects the characteristics of the produced seeds. Interestingly, the duration of exposure played a crucial role in exacerbating these detrimental effects. The 3 h exposure to cypermethrin demonstrated a more pronounced negative influence on all measured growth parameters compared to the 1 h exposure. This suggests that prolonged exposure to cypermethrin intensifies its impact on the growth and reproductive aspects of chickpea plants. The findings align with results obtained by Obidola et al. (2019) in cowpea, indicating a common sensitivity of leguminous crops to cypermethrin. Ramzan et al. (2022) have also found that cypermethrin has the most toxic effects on growth parameters like root length, stem length, and dry weight in Helianthus annus and Brassica juncea. A very strong negative correlation was found between the concentration of cypermethrin and plant height in 1 h treatment (−0.88) and 3 h treatment (−0.95). Increased exposures to pesticides reduce the photosynthetic capacity of the plants by inhibiting PSII, which in turn reduces the overall growth of the plant (Ramzan et al., 2022; Borowik et al., 2023a; Khurshid et al., 2024).
Higher concentrations and longer durations were associated with a greater incidence of abnormal pollen shapes, suggesting a disruptive influence on the typical morphology of C. arietinum pollen. It has been found that pesticides have direct negative effects on the morphology and anatomy of pollens (Wrońska–Pilarek et al., 2023). It is well known that the generative reproduction organs of plants are more susceptible to stress than the vegetative portions. Because of this, pollen is frequently used to evaluate the harmful effects of xenobiotics (Zanelli et al., 2023).
This toxic impact becomes more pronounced at higher dosages, and the observed reduction in fertile pollens compared to the control may have negative implications for the long-term productivity and quality of chickpeas (Çali, 2009). Similar negative effects on pollen viability were reported in eggplants exposed to a combination of two organophosphate insecticides and a dinitro herbicide. Furthermore, the fungicide propiconazole was found to adversely affect pollen germination and pollen tube growth in Tradescantia virginiana. Regardless of the type or dosage of herbicide used, pesticide treatment reduces the viability of pollen grains in both laboratory testing and field observations (Wrońska–Pilarek et al., 2023), and thus the significance of adequate pollination and fertilization in economically important crops is emphasized in various studies.
In pigment investigations, when the concentration of cypermethrin increased, the chlorophyll content in C. ariteinum leaves reduced considerably. Reduced pigment content can be attributed to various factors, and several studies have revealed a decrease in chlorophyll content in responses to increased stress (Chen et al., 2024; Skubała et al., 2024; Somtrakoon et al., 2024).
The carotenoid contents in this study reduced when treated with cypermethrin, although at a slower rate than the contents of chlorophyll. Cypermethrin not only caused pigment degradation but also lowered the biosynthesis mechanism, as seen by the decrease in carotenoid concentration. It is well-established that carotenoids play a vital role in protecting cells against photooxidation by assisting in the dissipation of excessive excitation energy (Vuković et al., 2021; Gautam et al., 2023). Cypermethrin not only induces the dilapidation of pigments but hampers their biosynthesis process by inhibiting membrane bound enzymes (Stelzer and Gordon, 1988; Michelangeli et al., 1990; Çavuşoğlu, 2011; Somtrakoon et al., 2024; Ariola and Ramize, 2024).
In our study, we observed a gradual increase in both SOD and CAT activities in treated populations as the concentration of cypermethrin increased in comparison to the control group. The significant rise in SOD and CAT activity at maximum concentration suggests an augmented generation of ROS. This increase leads to the transformation of superoxide anion free radicals into H2O2, as indicated by the elevated hydrogen peroxide scavenging observed in our results. According to Ayhan et al. (2024), the presence of hazardous chemicals causes serious oxidative damage to various cell structures and biomolecules. Antioxidant enzymes like CAT and SOD can cause oxidative damage and SOD is an essential part of antioxidative mechanisms in cell metabolism.
To evaluate oxidative stress-induced harm to lipid membranes, we monitored the change in malondialdehyde (MDA) concentration. Higher levels of MDA indicate increased cellular harm and the presence of oxidative stress (Khan et al., 2019). Our findings revealed a concentration-dependent response to cypermethrin, with elevated MDA levels observed. Interestingly, we observed a trend where longer exposure durations tended to lower SOD and CAT activities (Figures 6C, D). This was evident in the comparison between the 1 and 3 h treatments, suggesting a potential adaptation or regulation mechanism in response to prolonged cypermethrin exposure. Several studies have consistently demonstrated that plants have developed intricate antioxidant defense mechanisms relying on enzymes like SOD and CAT to neutralize free radicals and peroxides (Sharma et al., 2024). The enzyme SOD serves as the primary defense against reactive oxygen species (ROS) generated during oxidative stress (Khan et al., 2019). The augmentation of stress tolerance in plants under various stressful conditions is closely linked to an elevation in the activity of antioxidant enzymes (Aioub et al., 2021; Khurshid et al., 2024).
The present study aimed to develop a comprehensive understanding of how cypermethrin influences critical aspects of development and biochemistry in C. arietinum. The results of this investigation showed that cypermethrin can alter and negatively affect the morphology, biochemistry, and physiological aspects of C. arietinum. The duration of exposure and concentration of cypermethrin played a crucial role in exacerbating these detrimental effects. Our concerns regarding the harmful effects of agricultural pesticides like cypermethrin should be raised by these results, which also highlight the need for more research on the nutritional value of products derived from plants and seeds exposed to these agents. In order to achieve high crop yields, traditional intensive cropping systems heavily utilize chemical fertilizers, plant growth regulators, and pesticides. However, increased use of chemical pesticides on agricultural crops has raised a number of economic, ecological and health concerns. Governments in developed nations are establishing goals to limit the use of pesticides as awareness of this issue grows, but the adoption and scope of organic farming are growing more slowly, despite the efforts of various organizations to support it. This low adoption rate can be explained by a variety of factors, including the information given to farmers and their perceptions of the production risk associated with the new technology. So, more robust efforts are needed in this direction.
The original contributions presented in the study are included in the article/supplementary material, further inquiries can be directed to the corresponding author.
SS: Conceptualization, Data curation, Formal analysis, Funding acquisition, Investigation, Methodology, Project administration, Resources, Software, Supervision, Validation, Visualization, Writing – original draft, Writing – review & editing.
The author(s) declare financial support was received for the research, authorship, and/or publication of this article. The author extends their appreciation to the Deanship of Research and Graduate Studies at King Khalid University for funding this work through a Large Group Project under grant number (RGP2/49/45).
The author declares that the research was conducted in the absence of any commercial or financial relationships that could be construed as a potential conflict of interest.
All claims expressed in this article are solely those of the authors and do not necessarily represent those of their affiliated organizations, or those of the publisher, the editors and the reviewers. Any product that may be evaluated in this article, or claim that may be made by its manufacturer, is not guaranteed or endorsed by the publisher.
Aioub, A. A., Zuo, Y., Aioub, A. A., and Hu, Z. (2021). Biochemical and phytoremediation of Plantago major L. to protect tomato plants from the contamination of cypermethrin pesticide. Environ. Sci. Pollut. Res. 28, 43992–44001. doi: 10.1007/s11356-021-13853-2
Angaji, S. A., Najafi, F., Khavarinejad, R. A., and Soltani, E. (2013). The analysis of gene expression to identify interaction of gibberellic acid and salinity using differential display-PCR in chickpea (Cicer arietinum L.). Adv. Environ. Biol. 7, 1058–1063.
Ariola, B., Ramize, H., and Kathelina, K. (2024). Different level of tolerance to herbicides is displayed by l. cultivars depending on herbicide category and mode of application. EuroBiotech J. 8, 44–54. doi: 10.2478/ebtj-2024-0005
Arnon, D. I. (1949). Copper enzymes in isolated chloroplasts. Polyphenoloxidase in Beta vulgaris. Plant Physiol. 24:1. doi: 10.1104/pp.24.1.1
Ayhan, B. S., Macar, T. K., Macar, O., Yalçin, E., Çavuşoglu, K., and Özkan, B. (2024). A comprehensive analysis of royal jelly protection against cypermethrin-induced toxicity in the model organism Allium cepa L., employing spectral shift and molecular docking approaches. Pest. Biochem. Physiol. 203:105997. doi: 10.1016/j.pestbp.2024.105997
Baruah, P., Srivastava, A., Mishra, Y., and Chaurasia, N. (2024). Modulation in growth, oxidative stress, photosynthesis, and morphology reveals higher toxicity of alpha-cypermethrin than chlorpyrifos towards a non-target green alga at high doses. Environm. Toxicol. Pharmacol. 2024:104376. doi: 10.1016/j.etap.2024.104376
Borowik, A., Wyszkowska, J., Zaborowska, M., and Kucharski, J. (2023a). Impact of cypermethrin (Arpon G) on soil health and zea mays growth: a microbiological and enzymatic study. Agriculture 13:2261. doi: 10.3390/agriculture13122261
Borowik, A., Wyszkowska, J., Zaborowska, M., and Kucharski, J. (2023b). The impact of permethrin and cypermethrin on plants, soil enzyme activity, and microbial communities. Int. J. Mol. Sci. 24:2892. doi: 10.3390/ijms24032892
Çali, I. Ö. (2009). Effects of a fungicide on pollen morphology and fertility of tomato (Lycopersicon esculentum Mill.). Bang. J. Botany 38, 7–11. doi: 10.3329/bjb.v38i1.5112
Çavuşoğlu, K. (2011). Investigation of toxic effects of the glyphosate on Allium cepa. J. Agric. Sci. 17, 131–142.
Chen, P., Niu, M., Qiu, Y., Zhang, Y., Xu, J., Wang, R., et al. (2024). Physiological effects of maize stressed by HPPD inhibitor herbicides via multi-spectral technology and two-dimensional correlation spectrum technology. Ecotoxicol. Environ. Saf. 272:116087. doi: 10.1016/j.ecoenv.2024.116087
Erdtman, G. (1966). Pollen Morphology and Plant Taxonomy Angiosperms. New York: Hafner Publishing Co., 553.
Eti, S. (1991). Bazi meyve tür çeşitlerinde degişik in vitro testler yardimiyla çiçek tozu canlilik ve çimlenme yeteneklerinin belirlenmesi. Çukurova Univ. Ziraat Fak. Der. 6, 69–81.
Gallego, S. M., Benavides, M. P., and Tomaro, M. L. (1996). Effect of heavy metal ion excess on sunflower leaves: evidence for involvement of oxidative stress. Plant Sci. 121, 151–159. doi: 10.1016/S0168-9452(96)04528-1
Gautam, H., Singh, S., Prashad, H., Kumar, A., Choudhary, A., Kaur, H., et al. (2023). “Pesticide toxicity and their impact on plant growth, active constituents and productivity,” in Plants and their Interaction to Environmental Pollution (London: Elsevier), 231–252.
Hoseiny-Rad, M., and Aivazi, A. A. (2020). Biochemical and cytogenetic effects of Imazethapyr on Cicer arietinum L. J. Appl. Biol. Biotechnol. 8, 73–77. doi: 10.7324/JABB.2020.80212
Kenko, D. B. N., Ngameni, N. T., Awo, M. E., Njikam, N. A., and Dzemo, W. D. (2023). Does pesticide use in agriculture present a risk to the terrestrial biota? Sci. Total Environm. 861:160715. doi: 10.1016/j.scitotenv.2022.160715
Khan, B. A., Nadeem, M. A., Nawaz, H., Amin, M. M., Abbasi, G. H., Nadeem, M., et al. (2023). “Pesticides: impacts on agriculture productivity, environment, and management strategies,” in Emerging Contaminants and Plants: Interactions, Adaptations and Remediation Technologies (Cham: Springer International Publishing), 109–134.
Khan, Z., Shahwar, D., Ansari, M. K. Y., and Chandel, R. (2019). Toxicity assessment of anatase (TiO2) nanoparticles: A pilot study on stress response alterations and DNA damage studies in Lens culinaris Medik. Heliyon 5:e02069. doi: 10.1016/j.heliyon.2019.e02069
Khurshid, A., Inayat, R., Basit, A., Mobarak, S. H., Gui, S. H., and Liu, T. X. (2024). Effects of thiamethoxam on physiological and molecular responses to potato plant (Solanum tuberosum), green peach aphid (Myzus persicae) and parasitoid (Aphidius gifuensis). Pest Managem. Sci. 80, 3000–3009. doi: 10.1002/ps.8006
Liess, M., and Gröning, J. (2024). Latent pesticide effects and their mechanisms. Sci. Total Environm. 909:168368. doi: 10.1016/j.scitotenv.2023.168368
Liu, T. F., Wang, T., Sun, C., and Wang, Y. M. (2009). Single and joint toxicity of cypermethrin and copper on Chinese cabbage (Pakchoi) seeds. J. Hazard. Mater. 163, 344–348. doi: 10.1016/j.jhazmat.2008.06.099
Michelangeli, F., Robson, M. J., East, J. M., and Lee, A. G. (1990). The conformation of pyrethroids bound to lipid bilayers. Biochimica et biophysica acta (bba)-biomembranes 1028, 49–57. doi: 10.1016/0005-2736(90)90264-O
Nath, U., Puzari, A., and Jamir, T. (2024). Toxicological assessment of synthetic pesticides on physiology of Phaseolus vulgaris L. and Pisum sativum L. along with their correlation to health hazards: a case study in south-west Nagaland, India. J. Saudi Soc. Agric. Sci. 23, 300–311. doi: 10.1016/j.jssas.2024.01.001
Nedumaran, S., Abinaya, P., Jyosthnaa, P., Shraavya, B., Parthasarathy, R., and Bantilan, C. (2015). “Grain legumes production, consumption and trade trends in developing countries,” in Working Paper Series No. 60. ICRISAT Research Program, Markets, Institutions and Policies (Patancheru: International Crops Research Institute for the Semi-Arid Tropics), 64.
Norton, J. D. (1966). Testing of plum pollen viability with Tetrazolium salts. Proc. Amer. Soc. Hort. Sci. 89:132–134.
Obidola, S. M., Ibrahim, I., Yaroson, A. Y., and Henry, U. I. (2019). Phytotoxicity of cypermethrin pesticide on seed germination, growth and yield parameters of cowpea (Vigna unguiculata). Asian J. Agricult. Horticult. Res. 3, 1–10. doi: 10.9734/ajahr/2019/v3i229995
Pang, J., Turner, N. C., Khan, T., Du, Y.-L., Xiong, J.-L., Colmer, T. D., et al. (2017). Response of chickpea (Cicer arietinum L.) to terminal drought: leaf stomatal conductance, pod abscisic acid concentration, and seed set. J. Exp. Bot. 68, 1973–1985. doi: 10.1093/jxb/erw153
Rajak, P., Roy, S., Ganguly, A., Mandi, M., Dutta, A., Das, K., et al. (2023). Agricultural pesticides–Friends or foes to biosphere? J. Hazardous Mater. Adv. 10:100264. doi: 10.1016/j.hazadv.2023.100264
Ramzan, M., Akram, M., Rahi, A. A., Mubashir, M., Ali, L., Fahad, S., et al. (2022). Physio-biochemical, anatomical and functional responses of Helianthus annuus L. and Brassica juncea (Linn) to cypermethrin pesticide exposure. J. King Saud Univers.-Sci. 34:102210. doi: 10.1016/j.jksus.2022.102210
Rao, K. M., and Sresty, T. V. S. (2000). Antioxidative parameters in the seedlings of pigeonpea (Cajanus cajan (L.) Millspaugh) in response to Zn and Ni stresses. Plant Sci. 157, 113–128. doi: 10.1016/S0168-9452(00)00273-9
Ridwan, Q., Anjum, N., Rashid, S., Akhter, F., and Hanief, M. (2022). “Plant-microbe interactions in agro-ecological perspectives and degradation of pesticides,” in Bioremediation and Phytoremediation Technologies in Sustainable Soil Management (Palm Bay, Florida: Apple Academic Press), 129–158.
Ruch, R. J., Cheng, S. J., and Klaunig, J. E. (1989). Prevention of cytotoxicity and inhibition of intercellular communication by antioxidant catechins isolated from Chinese green tea. Carcinogenesis 10, 1003–1008. doi: 10.1093/carcin/10.6.1003
Sharma, A., Sharma, S. K., Singh, N., Maurya, V., Kaur, S., Kumar, R., et al. (2024). “Pesticides-mediated ROS generation in plants,” in Pesticides in a Changing Environment (London: Elsevier), 179–202.
Sharma, A., Shukla, A., Attri, K., Kumar, M., Kumar, P., Suttee, A., et al. (2020). Global trends in pesticides: a looming threat and viable alternatives. Ecotoxicol. Environ. Saf. 201:110812. doi: 10.1016/j.ecoenv.2020.110812
Sharma, P. (2012). Influence of pesticide-treated seeds on survival of Mesorhizobium sp. Cicer, symbiotic efficiency and yield in chickpea. Plant Prot. Sci. 48, 37–43. doi: 10.17221/54/2010-PPS
Sheikh, N., Patowary, H., and Laskar, R. A. (2020). Screening of cytotoxic and genotoxic potency of two pesticides (malathion and cypermethrin) on Allium cepa L. Mol. Cellular Toxicol. 16, 291–299. doi: 10.1007/s13273-020-00077-7
Skubała, K., Styburski, J., and Chowaniec, K. (2024). Combined effect of fungicide, herbicide and plant elicitor used in apple orchards on non-target epiphytic moss Hypnum cupressiforme. Environm. Pollut. 342:123133. doi: 10.1016/j.envpol.2023.123133
Somtrakoon, K., Thala, C., Thinnok, C., Thumjan, C., and Chouychai, W. (2024). Using plant growth regulators to stimulate growth of napier grass under atrazine contamination. Soil Sedim. Contamin. 23, 1–17. doi: 10.1080/15320383.2023.2301042
Stelzer, K. J., and Gordon, M. A. (1988). Interactions of pyrethroids with gramicidin-containing liposomal membranes. Biochimica et Biophysica Acta (BBA)-Biomembranes 938, 114–120. doi: 10.1016/0005-2736(88)90128-9
Stosser, R. (1984). Untersuchungen uber die befruchtungsbiologie and pollen production innerhalb der gruppe Prunus domestica. Erwerbobstbau 26, 110–115.
Vuković, S., Žunić, A., Maksimović, I., Lazić, S., Šunjka, D., Žunić, V., et al. (2021). Insecticide-induced changes of photosynthetic pigments content in peach leaves. Pak. J. Agri. Sci. 58, 1705–1710. doi: 10.21162/PAKJAS/21.1066
World Health Organization (1989). International Programme on Chemical Safety & WHO Task Group Meeting on Environmental Health Criteria for Cypermethrin. Cypermethrin/published under the joint sponsorship of the United Nations Environment Programme, the International Labour Organisation, and the World Health Organization. Geneva: World Health Organization.
Wrońska–Pilarek, D., Maciejewska–Rutkowska, I., Lechowicz, K., Bocianowski, J., Hauke–Kowalska, M., Baranowska, M., et al. (2023). The effect of herbicides on morphological features of pollen grains in Prunus serotina Ehrh. in the context of elimination of this invasive species from European forests. Scient. Rep. 13:4657. doi: 10.1038/s41598-023-31010-2
Yin, X., Jiang, L., Song, N., and Yang, H. (2008). Toxic reactivity of wheat (Triticum aestivum) plants to herbicide isoproturon. J. Agric. Food. Chem. 56, 4825–4831. doi: 10.1021/jf800795v
Zanelli, D., Carniel, F. C., Fortuna, L., Pavoni, E., González, V. J., Vázquez, E., et al. (2023). Interactions of airborne graphene oxides with the sexual reproduction of a model plant: when production impurities matter. Chemosphere 312:137138. doi: 10.1016/j.chemosphere.2022.137138
Keywords: cypermethrin, growth indices, chlorophyll content, lipid peroxidation, Cicer arietinum L
Citation: Siddiqui S (2024) Effects of cypermethrin on morphological, physiological and biochemical attributes of Cicer arietinum (Fabales: Fabaceae). Front. Sustain. Food Syst. 8:1446308. doi: 10.3389/fsufs.2024.1446308
Received: 09 June 2024; Accepted: 28 October 2024;
Published: 13 November 2024.
Edited by:
Aliza Pradhan, National Institute of Abiotic Stress Management (ICAR), IndiaReviewed by:
Ahmed A. A. Aioub, Zagazig University, EgyptCopyright © 2024 Siddiqui. This is an open-access article distributed under the terms of the Creative Commons Attribution License (CC BY). The use, distribution or reproduction in other forums is permitted, provided the original author(s) and the copyright owner(s) are credited and that the original publication in this journal is cited, in accordance with accepted academic practice. No use, distribution or reproduction is permitted which does not comply with these terms.
*Correspondence: Sazada Siddiqui, c2FzZGVreUBra3UuZWR1LnNh
Disclaimer: All claims expressed in this article are solely those of the authors and do not necessarily represent those of their affiliated organizations, or those of the publisher, the editors and the reviewers. Any product that may be evaluated in this article or claim that may be made by its manufacturer is not guaranteed or endorsed by the publisher.
Research integrity at Frontiers
Learn more about the work of our research integrity team to safeguard the quality of each article we publish.