- 1Laboratory of Molecular Biology, Epidemiology, and Monitoring of Bacteria and Virus Transmitted by Food (LaBESTA), University Joseph KI-ZERBO, Ouagadougou, Burkina Faso
- 2Laboratory of Virology and Plant Biotechnology, Institute of Environment and Agricultural Research (INERA), Ouagadougou, Burkina Faso
- 3Department of Natural Resources Management and Production Systems, Institute of Environment and Agricultural Research (INERA), Ouagadougou, Burkina Faso
- 4Division of Crop, Livestock, and Environment, Japan International Research Center for Agricultural Sciences, Tsukuba, Japan
Soil degradation and limited access to chemical fertilizers partly explain the persistently low crop yields in Sub-Saharan Africa. To provide local farmers with alternative fertilizer options, this study examined the impact of locally sourced fertilizers on sorghum productivity and soil health. Treatments were set up in a completely randomized block design with five replications each. These treatments included Burkina phosphate rock (BPR), calcined BPR (Cal-BPR), triple super phosphate (TSP), four types of phosphocomposts, and an unfertilized phosphate control (Control). The phospho-composts, prepared during 4 months in advance, were as follows: Comp-1 (sorghum straw + BPR + sorghum rhizosphere soil and roots), Comp-2 (sorghum straw + Cal-BPR + sorghum rhizosphere soil and roots), Comp-3 (sorghum straw + BPR + chicken manure), and Comp-4 (sorghum straw + Cal-BPR + chicken manure). They were applied at a rate of 2.5 t ha−1 at sowing, alongside BPR, Cal-BPR, and TSP that provided 23 kg P2O5 ha−1. Urea and potassium chloride were added 15 days after sowing (DAS) to all treatments to standardize nitrogen and potassium levels to 37 kg N ha−1 and 14 kg K2O ha−1. Soil properties were evaluated using the samples collected at 42, 70, and 120 DAS. Rhizosphere soil showed higher bacterial and fungal abundance than bulk soil, particularly in compost treatments. Comp-4 notably improved soil properties, including increased phosphorus-cycling microbial genes, available phosphorus, cation exchange capacity, and pH, and it produced the highest sorghum grain yield. However, Comp-3 offered superior economic benefits, with higher net returns from grain sales. Overall, phosphocomposts improve soil health and boost sorghum yield in Sub-Saharan Africa. Calcined BPR-chicken manure-enriched compost may be used to increase crop yields. However, the study shows that BPR-chicken manure-enriched compost provides a more cost-effective solution for soil revitalization and sustainable food production in infertile soils in the region.
1 Introduction
Sub-Saharan Africa (SSA) grapples with severe food insecurity owing to low crop production (Giller, 2020). The region is projected to experience a threefold increase in population by 2,100 (Ezeh et al., 2020; Vollset et al., 2020). This demographic shift necessitates a significant boost in agricultural output to meet the escalating food requirements, potentially requiring a 335% surge in cereal production by the year 2050 (van Ittersum et al., 2016). It necessitates intensifying agricultural practices or expanding croplands (van Ittersum et al., 2016; Vanlauwe et al., 2023). However, over 65% of SSA land is degraded and unsuitable for agriculture (Zingore et al., 2015). SSA soils suffer from deficiencies in nitrogen and available phosphorus, which are essential for plant growth and productivity (Stewart et al., 2020). Phosphorus deficiency is widespread and significantly impairs crop yield, as reported by Rakotoson et al. (2022), with substantial grain losses in sorghum (Sagnon et al., 2022) and lowland rice (Saito et al., 2019) systems. Additionally, low organic matter, limited cation exchange capacity, poor water holding capacity, and increased acidity of soils (Zingore et al., 2015) further complicate the agricultural productivity in the region.
Despite the efficacy of chemical fertilizers in addressing soil nutrient deficiencies and enhancing agricultural productivity (Shen et al., 2018; Alori et al., 2017), SSA faces challenges owing to cost and accessibility issues. In 2006, African Union member states convened in Nigeria and endorsed the “Abuja Declaration on Fertilizer for the African Green Revolution” due to the low fertilizer application rate of 8 kg per hectare. They committed to raising this to 50 kg/ha by 2015, which is still significantly below the global average of 150 kg/ha. Unfortunately, there has been minimal advancement, as evidenced by fertilizer utilization in Sub-Saharan Africa (SSA), which has remained relatively unchanged at 13–20 kg/ha since then (Sheahan and Barrett, 2017). This fertilizer application rate falls below the estimated annual nutrient loss of 40 kg ha−1 NPK (Vanlauwe et al., 2023). Additionally, the limited use of chemical fertilizers may serve as a precaution against environmental harm, as excessive application can result in soil and water pollution, contribute to global warming (Alori et al., 2017; Dimkpa et al., 2023), and disrupt soil health. Achieving food security in SSA while mitigating environmental impacts necessitates a strategy for soil fertilization that balances agricultural productivity with environmental sustainability.
In this context, organic fertilization has been well documented and traditionally utilized by smallholder farmers in SSA to restore soil fertility. Farmyard manures provide soils with rich organic matter, nitrogen, phosphorus, potassium, and micronutrients (Liu et al., 2020). They also enhance soil structure, increase water retention capacity, and promote microbial activity (Cui et al., 2018). Biochar application increases cation exchange capacity (CEC), modifies soil pH that influences the availability of certain nutrients in the soil, provides a microbial habitat, and reduces nitrogen loss (Lehmann et al., 2011; Bolan et al., 2024; Xie et al., 2013). Composts also improve nutrient availability, soil structure, and water retention (Kumar et al., 2024). In SSA, crop residues are often used as organic material during composting. However, this material is not always available due to other domestic uses. This limitation is compounded by most of these composts only replenish the soil with the nutrients plants previously took up. Therefore, supplementing composts with other essential elements becomes necessary.
Although soil total phosphorus concentrations typically range from 500 to 800 mg/kg of dry soil (Mengel et al., 2001), only a small fraction (0.1%) is readily available for plant uptake due to the low solubility of Pi, slow diffusion, and high soil reactivity (Menezes-Blackburn et al., 2018). Many soils in SSA have low available phosphorus levels (1 μmol l−1), significantly below the plant requirement of approximately 30 μmol l−1 for maximum productivity (Sashidhar and Podile, 2010). Soil available phosphorus may be improved using phosphate rock-enhanced compost (Qureshi et al., 2024; Ditta et al., 2018). Sarr et al. (2020) supplemented the phosphate rock-enriched compost with soil collected near plant roots, as a source of beneficial microbes, and further increased the compost nutrients and microbiome, including P-solubilizing microbes. These include bacteria, fungi, and actinomycetes that release organic acids and enzymes into the rhizosphere (Zuluaga et al., 2021; Alori et al., 2017; Liu et al., 2018), which promote phosphorus solubilization and mineralization. Glucose dehydrogenase (GCD) is pivotal in inorganic phosphorus solubilization, facilitated by its encoding gene (gcd) and cofactors (pqqFABCDEG), as reported by (An and Moe, 2016). Moreover, microbes harbor genes responsible for phosphorus transportation and absorption (Liang et al., 2020), enabling phosphorus transfer from the soil solution into microbial cells. Harnessing the capabilities of these soil microorganisms to enhance soil nutrient accessibility is a fundamental component of integrated soil fertility management approaches. Such strategies endorse the coordinated utilization of chemical fertilizers and organic amendments to rejuvenate soil health and fertility (Vanlauwe et al., 2023).
From this perspective, Sagnon et al. (2022) demonstrated the effectiveness of the phosphate rock-soil-compost mixture, comparable to that of chemical fertilizer NPK, for sorghum yield and soil microbiome. However, although the studies of Sarr et al. (2020) and Sagnon et al. (2022) demonstrated positive implications for soil fertility improvement and crop yields, only phosphate rock and rhizosphere soil were used as supplemental composting materials. We think providing farmers with various composting options is crucial, depending on resource availability. Phosphate-solubilizing microbes are in high numbers in rhizosphere soils (Elhaissoufi et al., 2022) and in plant roots as endophytes. We hypothesized that the mixture of soil and roots could also be an option for providing P-solubilizing microbes during composting. These P-solubilizing microbes are also present in organic wastes, such as livestock manure. Therefore, manures such as chicken manure could be another alternative microbial source during phospho-composting. Finally, calcined phosphate rock, with a higher water-soluble P than row phosphate rock, may be considered as P source during composting.
This study aims to propose cost-effective methods for enhancing soil fertility in SSA, specifically focusing on improving soil phosphorus availability. We expect composted phosphate rock enriched with a rhizosphere soil and roots mixture, or chicken manure would significantly improve soil chemical and microbiological properties. Consequently, this improvement would enhance crop production and economic profitability compared with chemical fertilizers and no-input controls, thereby contributing to sustainable agricultural practices and soil health in Sub-Saharan Africa. To address this, we compared the growth of sorghum [Sorghum bicolor (L.) Moench, Var. Sariasso 11] under different treatments: chemical fertilizer, locally made organo-mineral inputs (composted phosphate rock), and a control group with no phosphorus supply or organic amendment. Three main objectives were pursued: (1) evaluating the effect of treatments on sorghum production and economic profitability; (2) assessing changes in soil microbiological properties during plant growth, particularly focusing on soil phosphorus-cycling microbial genes, total bacteria, and total fungi; and (3) examining the impact of fertilization and cropping on soil nutrient availability.
2 Materials and methods
2.1 Experimental site
The field experiment was conducted at the Saria station (12°16’N, 2°09’W, 300-m altitude) at the Institute of Environment and Agricultural Research (INERA) in Burkina Faso’s northern Sudanian climate zone, featuring a ferric Lixisol soil type with a 1.5% slope (Zougmoré et al., 2004). Before the experiment, the site remained uncultivated for 1 year. Soil analysis at a 0–10 cm depth revealed low carbon (4.44 g kg−1), nitrogen (470 mg kg−1), total phosphorus (121.69 mg kg−1), and available phosphorus (4.01 mg kg−1) levels with a pH of 5.2. The soil exhibited a C/N ratio of 9.57, 15.6% soil saturation, exchangeable bases (1.25 meq/100 g), cation exchange capacity (7.96 meg/100 g), low water holding capacity (80–100 mm/m), and low water infiltration (Zougmoré, 2003). The nitrogen and available phosphorus levels in the soil were below those reported by Zougmoré et al. (2004), which were 700 mg kg−1 of nitrogen and 15 mg kg−1 of available phosphorus, respectively, indicating a declining soil fertility. The region typically encounters an annual average temperature of 28°C and rainfall between 600 and 900 mm (Zampaligré et al., 2022). However, during the study period, it received a heavy rainfall of 1,166 mm over 75 days (Supplementary Figure S1), the highest recorded in the past 5 years.
2.2 Phospho-composting
Four distinct compost types were prepared during the dry season (February–June 2022) before the field experiment (July–November 2022). Sorghum straw was utilized as the organic material, and the composting process was enhanced by adding beneficial microbes sourced from chicken manure and a mixture of rhizosphere soil and sorghum roots collected during the previous vegetative growth season. These materials were stored at room temperature until composting, to maintain the presence of microbes essential for organic matter decomposition and phosphorus solubilization.
Each compost type was designed with a specific goal to provide a unique set of benefits. Compost 1 (Comp-1), for instance, was composed of 80% sorghum straw (SS), 10% BPR (phosphorus source), and 10% mixture of rhizosphere soil and roots (RSR). The addition of BPR and the rhizosphere soil + roots was intended to supply phosphorus and beneficial microbes for decomposition and phosphorus solubilization. Compost 2 (Comp-2) took a different approach, replacing BPR with calcined BPR. The calcination of phosphate rock is known to enhance phosphorus solubility and reactivity, potentially enhancing the quality of the compost fertilizer. Compost 3 (Comp-3) maintained the BPR used in Comp-1 but substituted the soil and root mixture with chicken manure, a rich source of organic matter and beneficial microbes. Compost 4 (Comp-4) was made by mixing calcined BPR and chicken manure. This allowed for the assessment of whether using calcined BPR could further improve phosphorus solubilization and enhance the quality of compost fertilizer. The composting process adhered to the methodology of Sarr et al. (2020). Briefly, compost piles measuring 1.5 m x 1.15 m × 1 m were set up on tight plastic sheets. Each pile consisted of four successive layers of the composting materials listed above for each compost type (e.g., 20 kg SS, 2.5 kg BPR, 2.5 kg RSR). An appropriate amount of urea was added to each layer to reduce the initial C/N ratio of the SS from 55:1 to 25:1. Water was added to maintain a moisture content of 65%. The piles were then covered to initiate decomposition, and they were regularly uncovered to monitor temperature changes and mix and adjust moisture levels (every 2 weeks) as needed until maturity. The temperature of the piles reached 57–62°C on the 7th day after initiation and dropped to 35°C at 120 days. At the end of composting (120 days), the piles were uncovered, air-dried, and mixed thoroughly and later used for field applications. As Nakamura et al. (2015) described, the calcination of phosphate rock using carbonates enhances phosphorus solubility, chemical properties, reactivity, and alkaline characteristics, which can help to correct soil acidity. It is anticipated that the improved phosphorus solubility of calcined BPR could result in higher nutrient content and availability in the compost, making it a higher-quality fertilizer. However, without specific data or results from this study, it is impossible to provide a definitive assessment of the effect of replacing BPR with calcined BPR. This study provides further details on the nutrient content, solubility, and other relevant parameters to evaluate the specific influence of this replacement on compost quality. Table 1 provides information on the total carbon, total nitrogen, C/N ratio, total phosphorus, total potassium, and dry compost quantity at maturity.
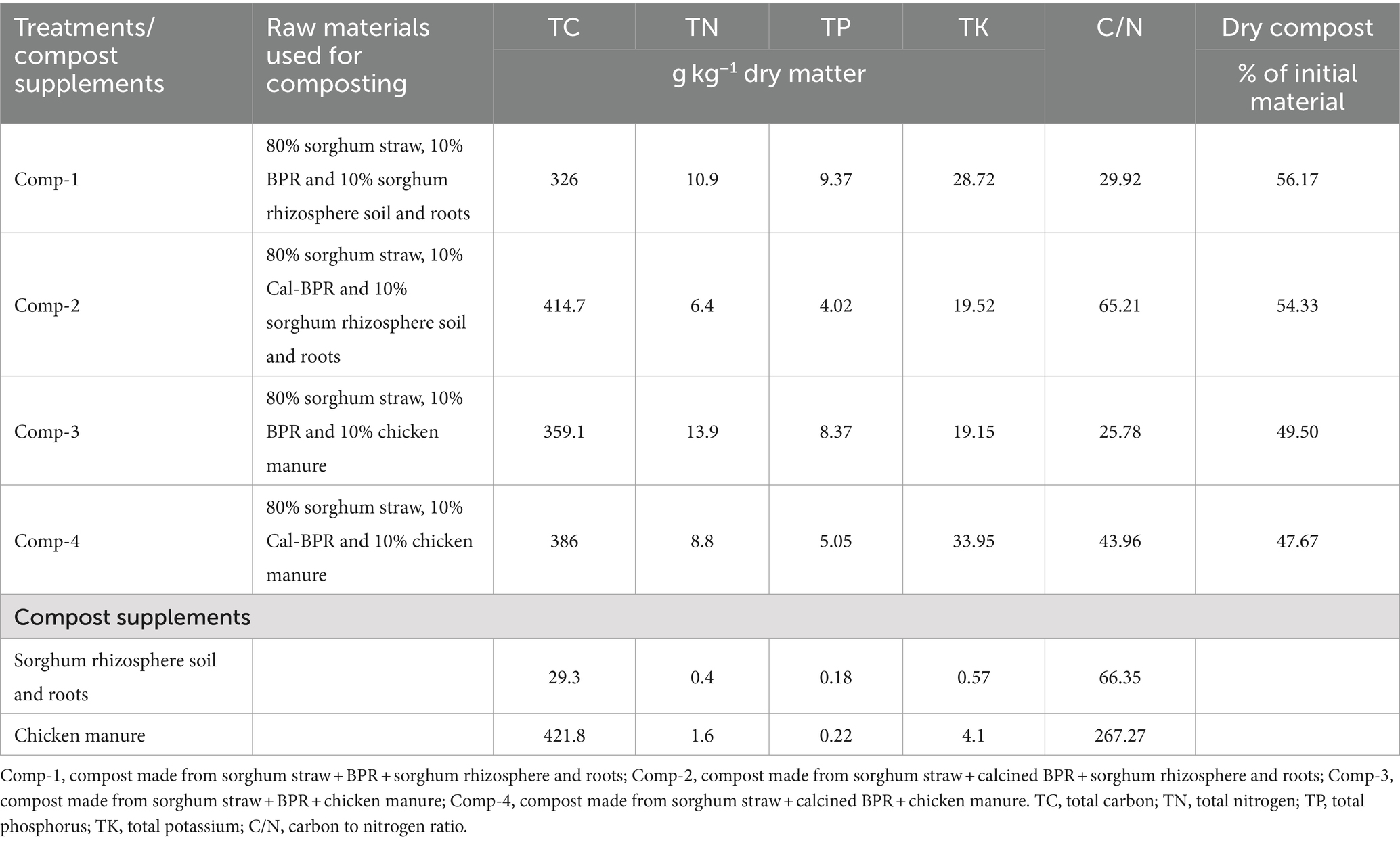
Table 1. Chemical characteristics of the composts and compost supplements (sorghum rhizosphere soil and roots, chicken manure).
2.3 Treatments and field experimental design
The treatments included the four compost types—Comp-1, Comp-2, Comp-3, and Comp-4—prepared from February to June, along with BPR, calcined BPR (Cal-BPR), triple super phosphate (TSP), and a control with no phosphorus input (Control). The experiment was arranged in a completely randomized block design with five replications each. The composts were applied at 2.5 t ha−1 during sowing. Based on their nutrient contents (Table 1), Comp-1, Comp-2, Comp-3, and Comp-4 supplied 27, 16, 35, and 22 kg N ha−1; 54, 23, 48, and 29 kg P2O5 ha−1; and 87, 59, 58, and 103 kg K2O ha−1, respectively. Since the region’s nutrient requirements are 37 kg N ha−1, 23 kg P2O5 ha−1, and 14 kg K2O ha−1 (AGRA, 2018), additional phosphorus and potassium application was unnecessary. Urea was added to the compost treatments to standardize nitrogen to 37 kg N ha−1. The quantities for the BPR, Cal-BPR, and TSP treatments were calculated to provide 23 kg P2O5 ha−1, with urea and KCl added to meet nitrogen and potassium requirements. The Control received urea and KCl for nitrogen and potassium, but no phosphorus. The experimental site was prepared by plowing to a depth of approximately 15 cm using an animal-drawn plow. Harrowing was done before arranging the eight treatments in the experimental layout, with each plot measuring 6 m × 4 m and 1 m spacing. All amendments were applied at sowing, except for nitrogen and potassium, which were applied 15 days after sowing (DAS). The compost was broadcast and lightly incorporated into the soil with a hoe. The effects of these treatments on the growth of sorghum [Sorghum bicolor (L.) Moench], a staple food crop in Burkina Faso, were evaluated using the Sariasso 11 variety, commonly grown in the region, planted at a density of 0.4 m × 0.8 m (31,250 ha−1). Planting was carried out on July 18, 2022, following a 22.5 mm rainfall.
2.4 Soil sampling during field trial
Weeds were consistently removed during the experiment. Composite samples of the sorghum rhizosphere and bulk soil were collected at 42, 70, and 120 DAS. Rhizosphere soil was collected by randomly selecting three sorghum plants from the plot borders, uprooting them, and carefully retrieving the soil adhering to roots with a paintbrush after shaking to remove loose soil. Special care was taken to avoid root damage and prevent the possible influence of root exudates on soil microbial abundance and diversity (Zhao et al., 2021). The collected rhizosphere soil was thoroughly mixed and a portion of approximately 15 g was kept at −20°C until DNA extraction and subsequent molecular analysis. The remaining rhizosphere soil was dried in an oven and used for chemical analysis. Similarly, the bulk soil obtained from three sub-samples collected from the center of the plots at 0–10 cm depth underwent the same processing. The bulk soil was used exclusively to determine microbial abundance.
2.5 Evaluation of sorghum biological yields
Sorghum was harvested at 120 DAS by cutting plants from the central area of the plots, which potentially contained 27 plant hills. The panicles and stems were air-dried for 4 weeks, and their dry weights were measured. The panicles were then dehulled, and the grain dry weights were determined. The thousand-grain weight was obtained by counting the grains in triplicate using a seed counter (NUMIGRAL GTEP, France) and measuring their weight. Biological yield, representing the combined biomass and grains of the entire plant, was evaluated along with the harvest index, which indicates the ratio of grain yield to biological yield (Poker et al., 2020). Assessing parameters such as grain yield, individual grain weight, overall biomass, and resource allocation provides insights into the productivity and efficiency of sorghum, encompassing grain production and plant growth.
2.6 Soil and plant chemical analysis
Rhizosphere soil samples and sorghum grains were processed and analyzed at the National Soil Office in Burkina Faso (BUNASOL). Soil samples were ground and then sieved through a 2-mm mesh and a 1-mm mesh to prepare them for various chemical analyses, including organic carbon, total nitrogen, total phosphorus, available phosphorus, pH (using the 1-mm sieved soil), exchangeable bases, and cation exchange capacity (CEC) using the 2-mm sieved soil. Similarly, sorghum grains were ground and powdered before the total phosphorus content was quantified.
The total phosphorus and nitrogen contents of soil and grain samples were assessed through the Kjeldahl method, involving the hot mineralization of 1 g Kjeldahl (1883), followed by colorimetric quantification (Houba et al., 1988). Available soil phosphorus was extracted using the Bray I method (Bray and Kurtz, 1945) and measured at 720 nm using the molybdate-blue method with a spectrophotometer (UV-6300PC Double Beam Spectrophotometer, China). Soil organic carbon was extracted following Walkley and Black (1934) and quantified at 650 nm using the same spectrophotometer. Exchangeable bases were extracted using a solution of 0.01 M Ag(H2NCSNH)22+ as described by Pleysier and Juo (1980), and the concentrations of magnesium (Mg2+) and calcium (Ca2+), along with cation exchange capacity (CEC), were measured using an Atomic Absorption Spectrometer (PerkinElmer, France). The concentrations of sodium (Na+) and potassium (K+) were assessed utilizing a flame photometer (pfp7 flame photometer, UK), and soil pH was measured using a compact pH meter (LAQUAtwinpH-22, Horiba Scientific, Japan) after creating a soil-water slurry with a 1:2 ratio.
2.7 Soil DNA extraction and quantification of microbial genes
Soil genomic DNA was extracted using the DNeasy PowerSoil Pro Kit (QIAGEN, Germany) at INERA in Burkina Faso following standard protocols. Soil moisture content was simultaneously determined during the DNA extraction after oven-drying about 1 g of soil at 70°C for 72 h. Molecular analysis of the extracted DNA was performed in Japan after obtaining a material transfer agreement and approvals from plant quarantine offices in Burkina Faso and Japan, ensuring compliance with regulations for the safe transfer of biological materials.
Extracted soil DNA was used to quantify the abundance of various microbial groups and genes by real-time PCR (qPCR). Total bacteria were assessed using the 16S rRNA gene (Weisburg et al., 1991), total fungi using the ITS region (Schoch et al., 2012), and phosphorus turnover microbial genes, including glucose dehydrogenase (gcd) (An and Moe, 2016) and alkaline phosphatase (phoD) (Ragot et al., 2015). The primer sequences, qPCR procedure, and conditions for the 16S rRNA, ITS, and gcd genes have been previously described by Sarr et al. (2019, 2020). However, the quantification of phoD differed according to primer sequences and annealing temperatures. Specifically, phoD was amplified using phoD-F733 (5`-TGGGAYGATCAYGARGT-3′) and phoD-R1083 (5’-CTGSGCSAKSACRTTCCA-3′) primers (Ragot et al., 2015), at an annealing temperature of 59°C. The amplification efficiencies and r2 values for the different genes varied within the following ranges: 16S rRNA [79.5–90.6; 0.997–1.000], ITS [72.8–79.7; 0.997–1.000], gcd [77.3–84.5; 0.997–0.999], and phoD [80.0–85.6; 0.996–0.998]. These values provide insight into the performance and reliability of qPCR assays for each target gene.
The abundance of microbial genes per gram of dry soil was then calculated using the formula below:
gcn: gene copy number (copy number g−1 dry soil−1).
sq.: starting quantity value following qPCR analysis.
f: dilution factor of the extracted DNA used for the qPCR (we diluted 10 times to reduce the presence of qPCR inhibitors).
v: volume of eluted DNA (μl) during the DNA extraction.
c: soil moisture content (%).
s: weight (g) of fresh soil used for DNA extraction (0.25 g of soil is used during this study).
2.8 Cost–benefit analysis of treatments
A cost–benefit analysis was conducted considering the expenses of fertilizers obtained through market prices and the production cost of compost based on the materials used. BPR was priced at 5,000 FCFA/50 kg, whereas TSP, urea, and KCl cost 60,000 FCFA/50 kg, 30,000 FCFA/50 kg, and 50,000 FCFA/50 kg, respectively. Chicken manure, a component of compost, was acquired at 2,500 FCFA/50 kg. The acquisition of sorghum straw and rhizosphere soil was presumed to be cost-free because of their availability to the farmers. The selling price of sorghum grains was assessed using weekly price data from the National Office for the Management of Food Security Stock (SONAGESS) in Burkina Faso, with a price of 324 FCFA/kg observed from June 5 to 11, 2023, at the Sankaryare market. Farmers are estimated to sell sorghum dry stems at a rate of 40 FCFA/kg. Total income includes earnings from both grains and stems. The acceptability index (AI) of each treatment was calculated based on its benefit relative to the control, with AI ≥2 indicating potential for quick adoption, 1.5 ≤ AI <2 indicating reluctant adoption, and AI <1.5 indicating rejection (Laminou et al., 2020). It is, therefore, the ratio of the benefits of the two treatments.
2.9 Statistical analysis
Two-way analysis of variance (ANOVA) assessed the interaction between treatments and sampling periods for soil chemical and microbial abundance data using the agricolae package (de Mendiburu, 2021) of R version 4.2.2 (R Core Team, 2022). Significant interactions (p ≤ 0.05) following two-way ANOVA prompted further one-way ANOVA for the individual sampling periods. Mean comparisons were performed using Duncan’s multiple range test (DMRT) or Fisher’s least significant difference (LSD). The Bonferroni correction was applied to mitigate type I errors (Lee and Lee, 2018). Sorghum yield parameters were first subjected to one-way ANOVA to determine the effect of the treatments on sorghum productivity. The outputs of Fisher’s LSD text were expressed as boxplots using multcompView (Graves et al., 2024) and ggplot2 (Wickham, 2016) packages. Pearson’s correlation matrices were generated using the metan package (Olivoto and Lúcio, 2020) to explore the relationships among microbial gene abundance, soil properties, and sorghum yield components at different growth stages.
3 Results
3.1 Sorghum yields and economic profitability of treatments
Significant variations were observed among treatments for phosphorus content in sorghum grains and all yield parameters except for the harvest index (Figure 1). The four composts and TSP had statistically similar yields for dry stems (Figure 1a), panicles (Figure 1b), grains (Figure 1c), biomass (Figure 1d), weight of 1,000 g (Figure 1e), P concentration in grains (Figure 1f). Comp-4 resulted in significantly higher values of these parameters than Cal-BPR, BPR, or the control. The Comp-3 group also yielded significantly higher than the control group. TSP yields were not significantly different from those of the composts, with Comp-4 exhibiting the highest grain yield.
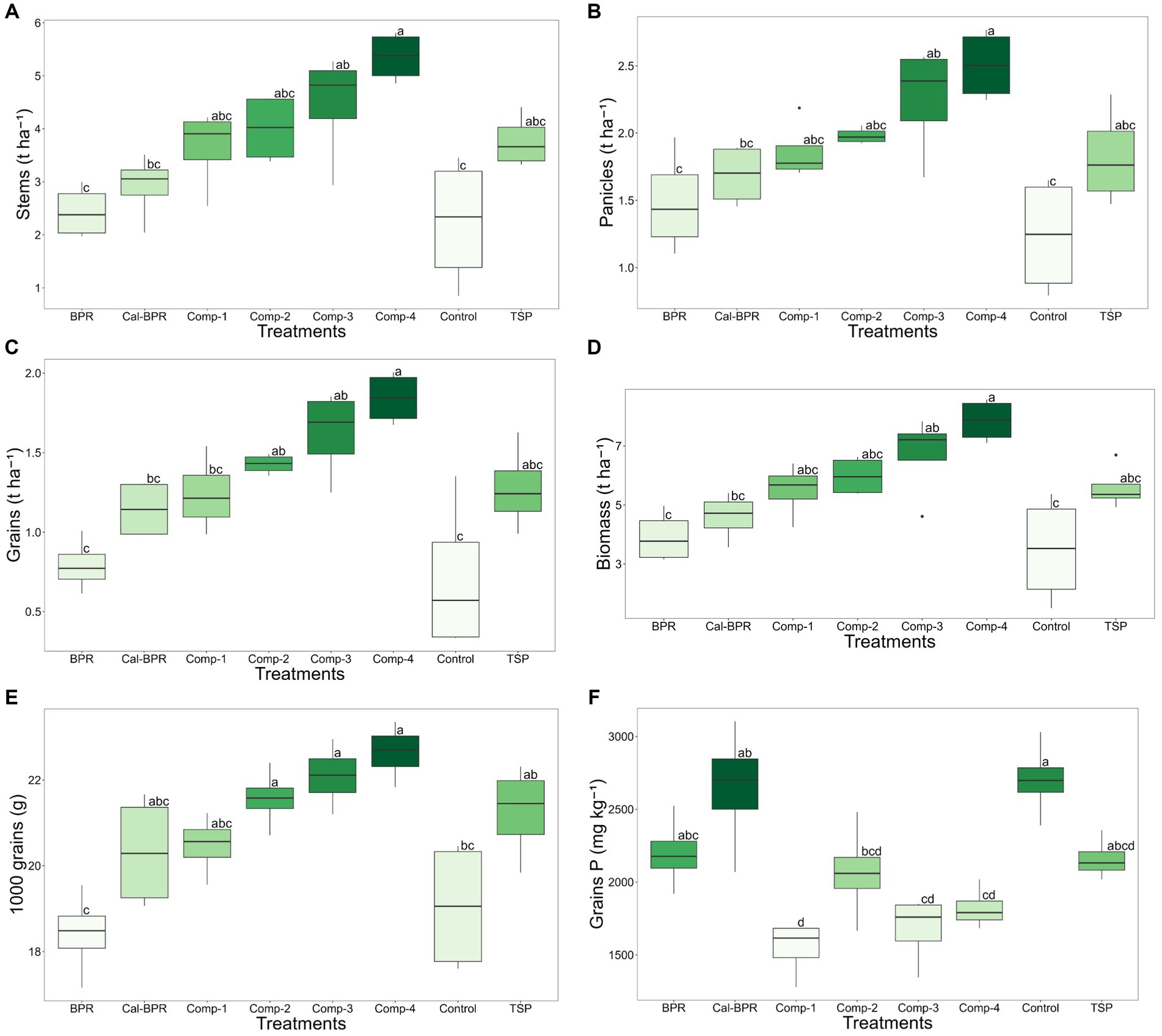
Figure 1. (A) Effect of treatments on the production of sorghum dry stems, (B) Effect of treatments on the production of sorghum dry panicles, (C) Effect of treatments on sorghum dry grains yield, (D) Effect of treatments on sorghum total dry biomass, (E) Effect of treatments on the weight of 1000 grains of sorghum, (F) Effect of treatments of the concentration of phosphorus (P) in the sorghum grains. Sorghum yield components were analyzed following one-way ANOVA. Comp-4: compost made from sorghum straw + calcined BPR + chicken manure, Comp-3: compost made from sorghum straw + BPR + chicken manure, Comp-2: compost made from sorghum straw + calcined BPR + sorghum rhizosphere and roots, Comp-1: compost made from sorghum straw + BPR + sorghum rhizosphere and roots, TSP: Triple superphosphate, Cal-BPR: calcined BPR, BPR: Burkina phosphate rock, Control: without phosphate or compost addition. t ha-1: tons per hectare, mg kg-1: milligrams per kilogram, g: gram, grains P: grains phosphorus, Parameter values assigned by different letters differ significantly at p < 0.05.
Comp-2 showed a significantly higher grain yield than that of the control, which did not differ from that of Comp-1, TSP, and BPR. Comp-4 and Comp-3 significantly increased the weight of 1,000 grains compared to BPR and the control. However, the control had the highest phosphorus content in the sorghum grains. The four composts showed the lowest grain total phosphorus content, which was similar to that of TSP.
Table 2 summarizes the cost of inputs, income, benefits, and the acceptability index of the treatments in terms of cost–benefit analysis, Comp-3 yielded the highest benefit and the best acceptability index (AI = 2.25), whereas BPR had the lowest income and acceptability index.
3.2 Changes in soil chemical properties
Soil available phosphorus significantly increased (p < 0.001) from the control to TSP treatments (Table 3). Comp-4 and Comp-3 showed notable increases in available phosphorus following TSP treatment, although not statistically different from the Comp-1, Comp-2, Cal-BPR, and BPR treatments. Generally, available phosphorus in the rhizosphere was higher at 42 DAS. Soil Ca2+, soil saturation, and available P were significantly higher at 42 DAS, whereas soil organic carbon, organic matter, and the C/N ratio were higher at 120 DAS.
Two-way ANOVA showed a significant (p ≤ 0.05) interaction between treatments and sampling periods for Mg2+, K+, Na+, exchangeable bases, CEC, total N, total P, and pH. Consequently, one-way ANOVA was conducted for each sampling period. At 42 DAS, Comp-3 and Comp-2 significantly increased soil Mg2+ compared to TSP, BPR, and the control, whereas all composts and Cal-BPR led to similar soil Mg2+ levels (Supplementary Table S1). At 120 DAS, only Comp-4-amended soil differed significantly from Cal-BPR in terms of Mg2+ content. Similarly, Comp-2 significantly increased K+ at 42 DAS compared to TSP, BPR, and the control, with no difference from the other composts and Cal-BPR. At 120 DAS, K+ decreased in all treatments except for the control, with BPR and Cal-BPR showing the lowest K+ levels. No significant difference was observed for Na+ among treatments, but the levels increased toward the end of the cropping season. These results detail the treatment-specific effects on Mg2+, K+, Na+, and other parameters across different sampling periods, thereby elucidating the element dynamics throughout the crop growth cycle.
Significant differences among treatments were mainly observed at 42 DAS, with generally higher values for exchangeable bases, CEC, total nitrogen, and total phosphorus than at 120 DAS. Comp-3 significantly increased exchangeable bases compared to BPR and the control, whereas Comp-2 showed higher CEC than all treatments except Comp-4. Soil nitrogen levels were similar between Comp-4 and the control (0.036 and 0.035%, respectively) but higher than TSP at 42 DAS. Total phosphorus was similar across treatments except for BPR, which had higher levels than the control. The soil pH increased from 42 to 120 DAS, ranging from slightly acidic to neutral (Supplementary Table S1; Supplementary Figure S2). The four composts and Cal-BPR resulted in a higher pH than BPR and the control during both sampling periods.
3.3 Microbial genes abundance in rhizosphere and bulk soils
The interaction between sampling periods and treatments was insignificant (p > 0.05) for the abundance of 16S rRNA, ITS, gcd, and phoD genes in the bulk soil and gcd in the rhizosphere soil. The average abundance data for the three sampling periods are summarized in Table 4. Treatments significantly differed among them only for the abundance of phoD in bulk soil. Additionally, the Comp-3 treatment resulted in a significantly higher abundance of the phoD in the bulk soil than in the control and in the Cal-BPR and BPR treatments. Comp-4 exhibited a significantly higher abundance of phoD in the bulk soil than in the Cal-BPR and BPR treatments. Changes in microbial gene abundance over time were also observed. Bacterial abundance (16S rRNA) in bulk soil significantly increased at 120 DAS compared to earlier periods, whereas fungal abundance (ITS) remained consistent at 42 and 72 DAS but notably increased at 120 DAS.
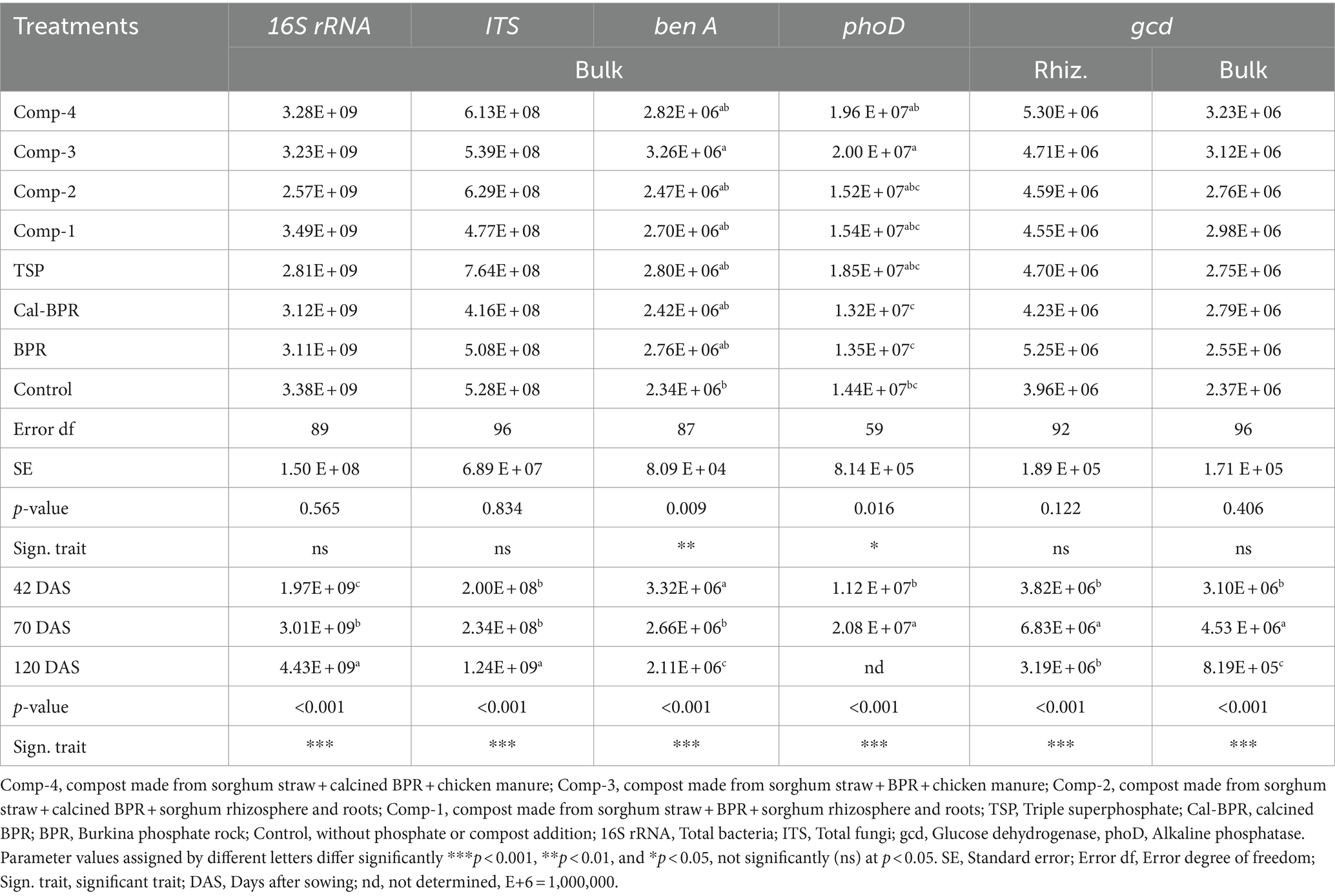
Table 4. Bulk and rhizosphere soils microbial gene abundance (copy number g−1 dry soil) following two-way ANOVA.
The total abundance of bacteria and fungi in the bulk soil increased by 1.25-fold and 5.26-fold, respectively, at the third sampling compared to the first sampling (Supplementary Figure S3). However, the abundance of gcd was significantly higher at 70 DAS in both bulk and rhizosphere soils than at 42 and 120 DAS. The presence of gcd was higher in rhizosphere soil than in bulk soil. The phoD gene was only assessed at 42 and 70 DAS and was significantly higher in the bulk soil at 70 DAS. Two-way ANOVA indicated a positive interaction between treatments and sampling periods for microbial genes (16S rRNA, ITS, and phoD) in rhizosphere soil. Subsequently, one-way ANOVA was conducted for each sampling period. The results (Supplementary Table S2) show a gradual increase in rhizosphere soil bacterial abundance over time, which was notably higher at 120 DAS. Significant differences in 16S rRNA among treatments were observed at 70 and 120 DAS. At 70 DAS, only Comp-4 exhibited a significant increase in bacterial abundance compared with the control. Similarly, Comp-4, Comp-3, and Comp-2 significantly increased rhizosphere bacterial abundance at 120 DAS compared to the control and BPR treatments. Fungal abundance differed significantly among the treatments only at 120 DAS, with Comp-4 showing the highest fungal abundance. The phoD gene abundance in the rhizosphere soil was higher at 70 DAS, influenced by treatments only at this time point, with Comp-4 exhibiting a notably higher abundance than the control. The increase in Comp-4 soil compared to the control soil was 0.74- and 0.87-fold for 16S rRNA and phoD genes, respectively (Supplementary Figure S4).
3.4 Relationship between soil chemical and microbial properties, and sorghum yields
Two Pearson correlations were performed using the soil chemical and microbial data at 42 DAS (Supplementary Table S3), and soil properties at 70 DAS and 120 DAS associated with sorghum yield parameters (Supplementary Table S4). The results revealed positive and negative interactions between the soil properties and sorghum yield parameters. At 42 DAS (Supplementary Table S3), phoD positively correlated with 16S rRNA and gcd. The pH exhibited a positive correlation with the sum of exchangeable bases but a negative correlation with total fungi (ITS). However, no correlation was found between the soil-available phosphorus (P) and soil microbial gene abundance at this stage.
Our findings at 70 DAS and 120 DAS (Supplementary Table S4) revealed a complex web of correlations between soil microbial properties at 70 DAS, soil chemical properties, and yield parameters at harvest. The abundance of gcd, for instance, was found to be positively correlated with fungi (ITS), phoD, and bacteria (16S rRNA) at 70 DAS but negatively correlated with the sum of exchangeable bases (SEB) at 120 DAS. Total fungi showed a positive correlation with biological yield at 120 DAS and total bacteria at 70 DAS, but a negative correlation with phosphorus concentration in sorghum grains. Additionally, soil total phosphorus negatively correlated with grain phosphorus concentration.
This study found strong correlations between various soil parameters and yield. Biological yields were positively correlated with soil pH at 120 DAS and the abundance of phoD and 16S rRNA genes at 70 DAS, while they were negatively correlated with grain phosphorus concentration. Grain yields were positively correlated with the weight of 1,000 grains, soil pH at 120 DAS, and the abundance of phoD and 16S rRNA genes at 70 DAS. However, grain yields were negatively correlated with grain phosphorus concentration. The weight of 1,000 grains showed a positive correlation with soil pH, phoD, and 16S rRNA. Additionally, the soil pH at 120 DAS was positively correlated with the abundance of phoD and 16S rRNA genes at 70 DAS. The data revealed a strong positive correlation between phoD expression and 16S rRNA gene abundance at 70 DAS. Other observed negative correlations included those between 16S rRNA and grain phosphorus concentration, as well as between CEC and the sum of exchangeable bases. The radar chart (Figure 2) summarizes the effects of the treatments on the soil properties and sorghum yield parameters, with Comp-4 showing the highest yields and soil microbial abundance (16S rRNA and ITS). Comp-2 significantly increased the soil pH at 120 DAS, TSP treatment provided abundant available phosphorus, and Comp-3 offered notable benefits.
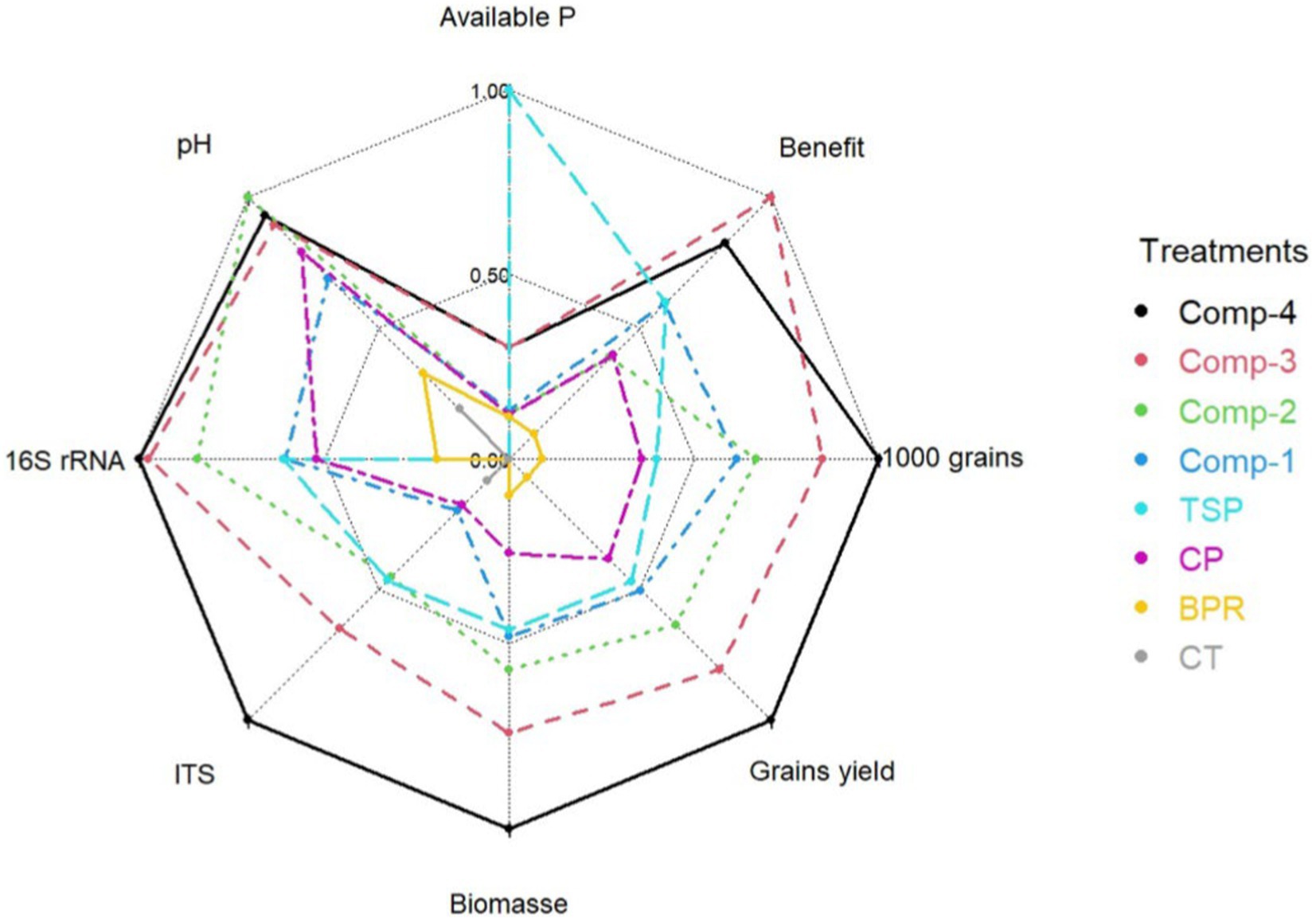
Figure 2. Radar chart showing treatment effect on significant soil properties and sorghum yield components Available P: available phosphorus, Comp-4: compost made from sorghum straw + calcined BPR + chicken manure, Comp-3: compost made from sorghum straw + BPR + chicken manure, Comp-2: compost made from sorghum straw + calcined BPR + sorghum rhizosphere and roots, Comp-1: compost made from sorghum straw + BPR + sorghum rhizosphere and roots, TSP, Triple superphosphate; Cal-BPR, calcined BPR; BPR, Burkina phosphate rock; Control: without phosphate or compost addition, 16S rRNA: total bacteria, ITS: total fungi, Biological yield: sorghum biomass yield (t ha−1), Grains yield: sorghum grains yield (t ha−1), 1,000 grains: 1000 grains weight (g), Benefit: economic profit from grains selling.
4 Discussion
This study investigated the impact of various compost types on sorghum growth and soil properties to provide affordable fertilizer options for smallholder farmers in SSA. Composts containing different phosphorus sources and organic materials were also compared. According to Nakamura et al. (2015), calcined Burkina phosphate rock contains 18% P2O5, whereas used Burkina phosphate rock contains 27% P2O5. This explains why Comp-4 and Comp-2, which received calcined phosphate rock, had lower total phosphorus contents than Comp-1 and Comp-3. Despite variations in phosphorus content, Comp-4 achieved higher sorghum yields, possibly because of nutrient provision (CEC, exchangeable bases, available phosphorus, and pH) and beneficial soil microbes. Previous research by Sarr et al. (2020) reported that phosphate rock-enriched composts are rich in essential plant nutrients and host beneficial microbes, including P-solubilizing and P-mineralizing bacteria and fungi. Zhou et al. (2022) also reported that organic fertilizer incorporation improves soil fauna feeding activity, in opposition to the excessive application of inorganic nitrogen fertilizer. This may be attributed to the ability of organic fertilizers to provide essential elements and create a suitable environment for soil fauna communities. Organic fertilizers are known to enhance soil organic matter content, cation exchange capacity (CEC), and contain a wide diversity of nutrients (Vanlauwe et al., 2023). Organic matters like composts have also been shown to improve soil water-holding capacity, resulting to increased crop productivity (Jain and Kalamdhad, 2020; Kebede et al., 2023). Additionally, they stimulate soil microbial biomass and enzymatic activities (Zhen et al., 2014), which are critical factors in increasing available phosphorus in the soil (Liu et al., 2018).
Although fungal involvement in organic phosphate mineralization was minor in this study, the contribution of other microbial genes may have been prominent. Correlations between microbial gene abundance and soil properties such as pH were observed, indicating the importance of maintaining appropriate pH levels and promoting beneficial microbial populations to improve crop yields. In soil, pH governs the availability and uptake of many nutrients, such as phosphorus, which is more available at pH values between 6 and 7.5 (Alori et al., 2017). It is also reported that pH is a key factor influencing bacterial community structure and abundance in agricultural soils (Wang et al., 2019). Bacteria were positively correlated with the phosphate-solubilizing microbial genes gcd and phoD, which are vital for releasing bound phosphorus and making it accessible to plants. This correlation implies that a higher abundance of bacteria can enhance various soil functions, including organic matter decomposition, nutrient cycling, and disease suppression, thereby supporting overall soil health.
This study found that compost amendments increased soil elements, such as exchangeable cations, which is consistent with the findings of Kebede et al. (2023) and Vanlauwe et al. (2023). Applying organic matter improves nutrient retention, soil buffering capacity, and water-holding capacity and can sustainably improve soil health over time (Brar et al., 2015; Zhou et al., 2022). However, the lack of correlation between soil phosphorus levels and sorghum yields suggests that elevated soil phosphorus might not directly enhance crop uptake, requiring solubilization/mineralization by related microbes. Following TSP treatment, Comp-4 and Comp-3 similarly increased the available phosphorus levels, possibly because of the enhanced P solubilization of Cal-BPR during composting or plant cultivation. The BPR and Cal-BPR used for the fabrication of Comp-3 and Comp-4 differed in their water-soluble P content of 2.4 and 0.2%, respectively, as reported by Fukuda et al. (2021). The observed relationships between different composts and soil phosphorus levels confirmed that adding chicken manure during composting activates phosphorus solubilization by P-solubilizing microbes, thereby affecting soil phosphorus levels even after application.
Indeed, the rhizosphere hosts numerous beneficial microbes, including phosphate-solubilizers (Elhaissoufi et al., 2022; Alori et al., 2017), which are nourished by organic compounds released through root exudates, thus supporting their metabolic activity (Shen et al., 2018). Rhizosphere microbial diversity and abundance are affected by root exudates, plant growth stages, and soil chemistry (Xu et al., 2021). In the Comp-4 treatment, increased total bacteria and fungi at specific growth stages likely facilitated the conversion of organic and mineral phosphorus into accessible forms for plants, outperforming other treatments. The abundance of gcd, linked to inorganic P-solubilizing bacteria, showed no difference between the treatments in the rhizosphere and bulk soils. However, it was higher in the rhizosphere, indicating the significance of the P-solubilizing bacteria in this area.
The striking increase in the abundance of the phoD gene in the sorghum rhizosphere of Comp-4 treatment at 70 DAS suggests a significant mineralization process of the organic phosphorus. The phoD gene encodes the alkaline phosphatase enzyme, which activity has been reported as strongly correlated to organic phosphate bioconversion in previous studies (Liang et al., 2020; Ragot et al., 2015, 2017). The increase of the phoD gene in this study was directly linked to the addition of organic matter and the subsequent increase in soil pH following the Comp-4 amendment. The simultaneous increase in phoD and total bacteria suggests that bacterial clusters predominantly carry phoD. Understanding the positive correlations among soil properties, microbial gene abundance, and crop yields can be a valuable tool for farmers in optimizing soil fertility. It underscores the importance of maintaining appropriate pH levels and promoting beneficial microbial populations, with the phoD gene playing a pivotal role in the Comp-4 treatment.
This study found a lack of correlation between phoD and total fungi, suggesting a minor role of fungi in organic phosphate mineralization, although microbes carrying the phoD gene normally include bacterial, archaeal, and fungal phyla (Ragot et al., 2017). Other microbial genes not examined in this study are also responsible for mineralizing organic P compounds such as phytate, phosphonate, and phosphoester in the soil (Liu et al., 2018; Liang et al., 2020).
5 Conclusion
The limited availability of commercial fertilizers poses a challenge in Sub-Saharan Africa and hampers agricultural production. Our study demonstrates the efficacy and economic viability of composts as fertilizers for smallholder farmers in Sub-Saharan Africa. Composts, particularly those made with sorghum residues, calcined phosphate rock, and chicken manure, outperform chemical fertilizers in terms of sorghum yield and cost-effectiveness. These composts also improve soil health by promoting increased levels of exchangeable cations, pH, and beneficial microbial abundance. Promoting the abundance of beneficial microorganisms through organic amendments and cover cropping can enhance nutrient availability and plant health, thereby offering promising solutions for sustainable agriculture in this region.
Data availability statement
The raw data supporting the conclusions of this article will be made available by the authors, without undue reservation.
Ethics statement
This study was conducted in collaboration with the Japan International Research Center for Agricultural Sciences (JIRCAS) and the Environmental Institute for Agricultural Research (INERA) of Burkina Faso. It involved the collection of soil samples from a field in Burkina Faso and the DNA extraction of the collected samples. Soil DNA was transferred from INERA (Burkina Faso) to JIRCAS (Japan) for biological analysis. The transfer of samples was performed in accordance with the Joint Research Contract (JRC) and Material Transfer Agreement (MTA) between the two institutions. All laboratory analyses adhered to the relevant institutional, national, and international guidelines and regulations.
Author contributions
AS: Writing – review & editing, Writing – original draft, Software, Methodology, Investigation, Formal analysis, Data curation, Conceptualization. MT: Writing – review & editing, Validation, Supervision, Conceptualization. ET: Writing – review & editing, Visualization, Validation, Supervision, Methodology, Investigation, Formal analysis, Data curation, Conceptualization. SZ: Writing – review & editing, Validation, Investigation, Formal analysis. IB: Writing – review & editing, Visualization, Validation, Supervision. SN: Writing – review & editing, Visualization, Validation, Funding acquisition. NB: Writing – review & editing, Visualization, Validation, Supervision. FT: Writing – review & editing, Visualization, Validation, Supervision, Methodology. PS: Writing – review & editing, Writing – original draft, Validation, Methodology, Funding acquisition, Formal analysis, Data curation, Conceptualization.
Funding
The author(s) declare that financial support was received for the research, authorship, and/or publication of this article. This research was funded by the Japan Science and Technology Agency (JST) and the Japan International Cooperation Agency (JICA) through the Burkina SATREPS (Science and Technology Research Partnerships) project no. JPMJSA1609.
Acknowledgments
We express our sincere gratitude to JICA and JST for funding this research through the SATREPS-Burkina Project. We are very thankful to Dr. Fujio Nagumo of JIRCAS, the leader of the SATREPS project, for his comments and guidance during this study. We thank the staff of INERA and BUNASOL (National Office of Soil in Burkina Faso) for their assistance during the fieldwork and laboratory analyses in Burkina Faso.
Conflict of interest
The authors declare that the research was conducted in the absence of any commercial or financial relationships that could be construed as a potential conflict of interest.
Publisher’s note
All claims expressed in this article are solely those of the authors and do not necessarily represent those of their affiliated organizations, or those of the publisher, the editors and the reviewers. Any product that may be evaluated in this article, or claim that may be made by its manufacturer, is not guaranteed or endorsed by the publisher.
Supplementary material
The Supplementary material for this article can be found online at: https://www.frontiersin.org/articles/10.3389/fsufs.2024.1445683/full#supplementary-material
Supplementary Figure S1 | Rainfall distribution in Saria station from 2018 to 2022.
Supplementary Figure S2 | pH dynamics in sorghum rhizosphere during sorghum cropping. Comp-4, compost made from sorghum straw + calcined BPR + chicken manure; Comp-3, compost made from sorghum straw + BPR + chicken manure; Comp-2, compost made from sorghum straw + calcined BPR + sorghum rhizosphere and roots; Comp-1, compost made from sorghum straw + BPR + sorghum rhizosphere and roots; TSP, Triple super phosphate; Cal-BPR, calcined BPR; BPR, Burkina phosphate rock; Control, without phosphate or compost addition; DAS, Days after sowing.
Supplementary Figure S3 | Distribution of microbial genes in the bulk soil. 16S rRNA: total bacteria, ITS: total fungi, gcd: glucose dehydrogenase, phoD: alkaline phosphatase, DAS: Days after sowing.
Supplementary Figure S4 | Distribution of microbial genes in the rhizosphere soil. Comp-4, compost made from sorghum straw + calcined BPR + chicken manure; Comp-3, compost made from sorghum straw + BPR + chicken manure; Comp-2, compost made from sorghum straw + calcined BPR + sorghum rhizosphere and roots; Comp-1, compost made from sorghum straw + BPR + sorghum rhizosphere and roots; TSP, Triple super phosphate; Cal-BPR, calcined BPR; BPR, Burkina phosphate rock; Control, without phosphate or compost addition; 16S rRNA, total bacteria; ITS, total fungi; phoD, alkaline phosphatase; DAS, days after sowing.
References
AGRA (2018). Assessment of fertilizer distribution systems and opportunities for developing fertilizer blends in Burkina Faso. Available at: https://agra.org/wp-content/uploads/2020/08/Burkina-Report_Assessment-of-Fertilizer-Distribution-Systems-and-Opportunities-for-Developing-Fertilizer-Blends.pdf (accessed March 25, 2024)
Alori, E. T., Glick, B. R., and Babalola, O. O. (2017). Microbial phosphorus solubilization and its potential for use in sustainable agriculture. Front. Microbiol. 8:971. doi: 10.3389/fmicb.2017.00971
An, R., and Moe, L. A. (2016). Regulation of pyrroloquinoline quinone-dependent glucose dehydrogenase activity in the model rhizosphere-dwelling bacterium Pseudomonas putida KT2440. Appl. Environ. Microbiol. 82, 4955–4964. doi: 10.1128/AEM.00813-16
Bolan, S., Sharma, S., Mukherjee, S., Kumar, M., Rao, C. S., Nataraj, K. C., et al. (2024). Biochar modulating soil biological health: a review. Sci. Total Environ. 914:169585. doi: 10.1016/j.scitotenv.2023.169585
Brar, B. S., Singh, J., Singh, G., and Kaur, G. (2015). Effects of long-term application of inorganic and organic fertilizers on soil organic carbon and physical properties in maize-wheat rotation. Agron. 5, 220–238. doi: 10.3390/agronomy5020220
Bray, R. H., and Kurtz, L. T. (1945). Determination of total, organic, and available forms of phosphorus in soils. Soil Sci. 59, 39–46. doi: 10.1097/00010694-194501000-00006
Cui, S., Liang, S., Zhang, X., Li, Y., Liang, W., Sun, L., et al. (2018). Long-term fertilization management affects the C utilization from crop residues by the soil micro-food web. Plant Soil 429, 335–348. doi: 10.1007/s11104-018-3688-4
de Mendiburu, F. (2021). Agricolae: Statistical procedures for agricultural research. R package version 1.3–5. Available at: https://CRAN.R-project.org/package=agricolae (accessed May 15, 2024)
Dimkpa, C., Adzawla, W., Pandey, R., Atakora, W. K., Kouame, A. K., Jemo, M., et al. (2023). Fertilizers for food and nutrition security in sub-Saharan Africa: an overview of soil health implications. Front. Soil Sci. 3:1123931. doi: 10.3389/fsoil.2023.1123931
Ditta, A., Muhammad, J., Imtiaz, M., Mehmood, S., Qian, Z., and Tu, S. (2018). Application of rock phosphate enriched composts increases nodulation, growth and yield of chickpea. Int. J. Rec. Org. Waste Agric. 7, 33–40. doi: 10.1007/s40093-017-0187-1
Elhaissoufi, W., Ghoulam, C., Barakat, A., Zeroual, Y., and Bargaz, A. (2022). Phosphate bacterial solubilization: a key rhizosphere driving force enabling higher P use efficiency and crop productivity. J. Adv. Res. 38, 13–28. doi: 10.1016/j.jare.2021.08.014
Ezeh, A., Kissling, F., and Singer, P. (2020). Why sub-Saharan Africa might exceed its projected population size by 2100. Lancet 396, 1131–1133. doi: 10.1016/S0140-6736(20)31522-1
Fukuda, M., Soma, D. M., Iwasaki, S., Nakamura, S., Kanda, T., Ouattara, K., et al. (2021). Site-specific responses of lowland rice to acidulated and calcined phosphate rock fertilizers in the center-west region of Burkina Faso. PLoS One 16:e0250240. doi: 10.1371/journal.pone.0250240
Giller, K. E. (2020). The food security conundrum of sub-Saharan Africa. Glob. Food Sec. 26:100431. doi: 10.1016/j.gfs.2020.100431
Graves, S., Piepho, H., and Selzer, L. (2024). Multcompview: visualizations of paired comparisons. R package version 0. Vienna: R Foundation for Statistical Computing, 1–10.
Houba, J., van der Lee, J., Novozamsky, I., and Walinga, I. (1988). Soil and plant analysis. Part 5. Wageningen: Wageningen Agricultural University.
Jain, M. S., and Kalamdhad, A. S. (2020). Soil revitalization via waste utilization: compost effects on soil organic properties, nutritional, sorption and physical properties. Environ. Technol. Innov. 18:100668. doi: 10.1016/j.eti.2020.100668
Kebede, T., Diriba, D., and Boki, A. (2023). The effect of organic solid waste compost on soil properties, growth, and yield of Swiss chard crop (Beta vulgaris L.). Sci. World J. 2023:6175746. doi: 10.1155/2023/6175746
Kjeldahl, J. (1883). A new method for the determination of nitrogen in organic matter. Zeits. Anal. Chem. 22, 366–382. doi: 10.1007/BF01338151
Kumar, V., Pathania, N., Sharma, S., and Sharma, R. (2024). Dynamics of plant nutrient signaling through compost. Microbe 2:100047. doi: 10.1016/j.microb.2024.100047
Laminou, R. H. M., Ndiaye, S., Diallo, D., Dieye, A. B., Diallo, M. D., and Guisse, A. (2020). Mineral fertilizer microdosing alone or combined with urea on maize and according to the soil chemical elements variation (Thies, Senegal). Am. J. Agric. Fores. 8, 69–76. doi: 10.11648/j.ajaf.20200803.13
Lee, S., and Lee, D. K. (2018). What is the proper way to apply the multiple comparison test? Kor. J. Anesthesiol. 71, 353–360. doi: 10.4097/kja.d.18.00242
Lehmann, J., Rillig, M. C., Thies, J., Masiello, C. A., Hockaday, W. C., and Crowley, D. (2011). Biochar effects on soil biota–a review. Soil Biol. Biochem. 43, 1812–1836. doi: 10.1016/j.soilbio.2011.04.022
Liang, J. L., Liu, J., Jia, P., Yang, T., Zeng, Q., Zhang, S., et al. (2020). Novel phosphate-solubilizing bacteria enhance soil phosphorus cycling following ecological restoration of land degraded by mining. ISME J. 14, 1600–1613. doi: 10.1038/s41396-020-0632-4
Liu, J., Cade-Menun, B. J., Yang, J., Hu, Y., Liu, C. W., Tremblay, J., et al. (2018). Long-term land use affects phosphorus speciation and the composition of phosphorus cycling genes in agricultural soils. Front. Microbiol. 9:1643. doi: 10.3389/fmicb.2018.01643
Liu, S., Wang, J., Pu, S., Blagodatskaya, E., Kuzyakov, Y., and Razavi, B. S. (2020). Impact of manure on soil biochemical properties: a global synthesis. Sci. Total Environ. 745:141003. doi: 10.1016/j.scitotenv.2020.141003
Menezes-Blackburn, D., Giles, C., Darch, T., George, T. S., Blackwell, M., Stutter, M., et al. (2018). Opportunities for mobilizing recalcitrant phosphorus from agricultural soils: a review. Plant Soil 427, 5–16. doi: 10.1007/s11104-017-3362-2
Mengel, K., Kirkby, E. A., Kosegarten, H., and Appel, T. (2001). “Phosphorus” in Principles of plant nutrition. eds. K. Mengel, E. A. Kirkby, H. Kosegarten, and T. Appel (Dordrecht: Springer), 453–479.
Nakamura, S., Imai, T., Toriyama, K., Tobita, S., Matsunaga, R., Fukuda, M., et al. (2015). Solubilization of Burkina Faso phosphate rock through calcination method. Jpn. J. Soil Sci. Plant Nutr. 86, 534–538. doi: 10.20710/dojo.86.6_534
Olivoto, T., and Lúcio, A. D. (2020). Metan: an R package for multi-environment trial analysis. Methods Ecol. Evol. 11, 783–789. doi: 10.1111/2041-210X.13384
Pleysier, J. L., and Juo, A. S. R. (1980). A single-extraction method using silver-thiourea for measuring exchangeable cations and effective CEC in soils with variable charges. Soil Sci. 129, 205–211. doi: 10.1097/00010694-198004000-00002
Poker, K., Straight, M., and Hunt, J. R. (2020). Evaluation of G × E × M interactions to increase harvest index and yield of early sown wheat. Front. Plant Sci. 11:994. doi: 10.3389/fpls.2020.00994
Qureshi, S. A., Rajput, A., Memon, M., and Solangi, M. A. (2024). Nutrient composition of rock phosphate enriched compost from various organic wastes. J. Sci. Res. 2, 47–51. Available at: http://www.e3journals.org
R Core Team (2022). A language and environment for statistical computing. Vienna: R Foundation for Statistical Computing.
Ragot, S. A., Kertesz, M. A., and Bünemann, E. K. (2015). phoD alkaline phosphatase gene diversity in soil. Appl. Environ. Microbiol. 81, 7281–7289. doi: 10.1128/AEM.01823-15
Ragot, S. A., Kertesz, M. A., Mészáros, É., Frossard, E., and Bünemann, E. K. (2017). Soil phoD and phoX alkaline phosphatase gene diversity responds to multiple environmental factors. FEMS Microbiol. Ecol. 93:fiw212. doi: 10.1093/femsec/fiw212
Rakotoson, T., Tsujimoto, Y., and Nishigaki, T. (2022). Phosphorus management strategies to increase lowland rice yields in sub-Saharan Africa: a review. Field Crop Res. 275:108370. doi: 10.1016/j.fcr.2021.108370
Sagnon, A., Iwasaki, S., Tibiri, E. B., Zongo, N. A., Compaore, E., Bonkoungou, I. J. O., et al. (2022). Amendment with Burkina Faso phosphate rock-enriched composts alters soil chemical properties and microbial structure, and enhances sorghum agronomic performance. Sci. Rep. 12:13945. doi: 10.1038/s41598-022-18318-1
Saito, K., Vandamme, E., Johnson, J. M., Tanaka, A., Senthilkumar, K., Dieng, I., et al. (2019). Yield-limiting macronutrients for rice in sub-Saharan Africa. Geoderma 338, 546–554. doi: 10.1016/j.geoderma.2018.11.036
Sarr, P. S., Ando, Y., Nakamura, S., Deshpande, S., and Subbarao, G. V. (2019). Sorgoleone release from sorghum roots shapes the composition of nitrifying populations, total bacteria, and archaea and determines the level of nitrification. Biol. Fert. Soils 56, 145–166. doi: 10.1007/s00374-019-01405-3
Sarr, P. S., Tibiri, E. B., Fukuda, M., Zongo, A. N., Compaore, E., and Nakamura, S. (2020). Phosphate-solubilizing fungi and alkaline phosphatase trigger the P solubilization during the co-composting of sorghum straw residues with Burkina Faso phosphate rock. Front. Environ. Sci. 8:559195. doi: 10.3389/fenvs.2020.559195
Sashidhar, B., and Podile, A. (2010). Mineral phosphate solubilization by rhizosphere bacteria and scope for manipulation of the direct oxidation pathway involving glucose dehydrogenase. J. Appl. Microbiol. 109, 1–12. doi: 10.1111/j.1365-2672.2009.04654.x
Schoch, C. L., Seifert, K. A., Huhndorf, S., Robert, V., Spouge, J., Levesque, C. A., et al. (2012). Nuclear ribosomal internal transcribed spacer (ITS) region as a universal DNA barcode marker for Fungi. Proc. Natl. Acad. Sci. 109, 6241–6246. doi: 10.1073/pnas.1117018109
Sheahan, M., and Barrett, C. (2017). Ten striking facts about agricultural input use in sub-Saharan Africa. Food Pol. 67, 12–25. doi: 10.1016/j.foodpol.2016.09.010
Shen, S., Yang, M., Ba, C., Yu, S., Jiang, Y., Zou, H., et al. (2018). Preparation and characterization of slow-release fertilizer encapsulated by biochar-based waterborne copolymers. Sci. Total Environ. 615, 431–437. doi: 10.1016/j.scitotenv.2017.09.209
Stewart, Z. P., Pierzynski, G. M., Middendorf, B. J., and Prasad, P. V. V. (2020). Approaches to improve soil fertility in sub-Saharan Africa. J. Exp. Bot. 71, 632–641. doi: 10.1093/jxb/erz446
van Ittersum, M. K., van Bussel, L. G. J., Wolf, J., Grassini, P., van Wart, J., Guilpart, N., et al. (2016). Can sub-Saharan Africa feed itself? Proc. Nat. Acad. Sci. 113, 14964–14969. doi: 10.1073/pnas.1610359113
Vanlauwe, B., Amede, T., Bationo, A., Bindraban, P., Breman, H., Cardinael, R., et al. (2023). Fertilizer and soil health in Africa: The role of fertilizer in building soil health to sustain farming and address climate change. Muscle Shoals, AL: International fertilizer development center (IFDC).
Vollset, S. E., Goren, E., Yuan, C. W., Ca, J., Smith, A. E., Hsiao, T., et al. (2020). Fertility, mortality, migration, and population scenarios for 195 countries and territories from 2017 to 2100: a forecasting analysis for the global burden of disease study. Lancet 396, 1285–1306. doi: 10.1016/S0140-6736(20)30677-2
Walkley, A., and Black, I. A. (1934). An examination method of the Degtjareff and a proposed modification of the chromic acid titration method. Soil Sci. 37, 29–38. doi: 10.1097/00010694-193401000-00003
Wang, C., Zhou, X., Guo, D., Zhao, J., Yan, L., Feng, G., et al. (2019). Soil pH is the primary factor driving the distribution and function of microorganisms in farmland soils in northeastern China. An. Microbiol. 69, 1461–1473. doi: 10.1007/s13213-019-01529-9
Weisburg, W. G., Barns, S. M., Pelletier, D. A., and Lane, D. J. (1991). 16S ribosomal DNA amplification for phylogenetic study. J. Bacteriol. 173, 697–703. doi: 10.1128/jb.173.2.697-703.1991
Xie, Z., Xu, Y., Liu, G., Liu, Q., Zhu, J., Tu, C., et al. (2013). Impact of biochar application on nitrogen nutrition of rice, greenhouse-gas emissions and soil organic carbon dynamics in two paddy soils of China. Plant Soil 370, 527–540. doi: 10.1007/s11104-013-1636-x
Xu, H., Du, H., Zeng, F., Song, T., and Peng, W. (2021). Diminished rhizosphere and bulk soil microbial abundance and diversity across succession stages in karst area, Southwest China. Appl. Soil Ecol. 158:103799. doi: 10.1016/j.apsoil.2020.103799
Zampaligré, N., Yoda, G., Delma, J., Sanfo, A., Balehegn, M., Rios, E., et al. (2022). Fodder biomass, nutritive value, and grain yield of dual-purpose improved cereal crops in Burkina Faso. Agron. J. 114, 115–125. doi: 10.1002/agj2.20860
Zhao, M., Zhao, J., Yuan, J., Hale, L., Wen, T., Huang, Q., et al. (2021). Root exudates drive soil-microbes-nutrient feedbacks in response to plant growth. Plant Cell Environ. 44, 613–628. doi: 10.1111/pce.13928
Zhen, Z., Liu, H., Wang, N., Guo, L., Meng, J., Ding, N., et al. (2014). Effects of manure compost application on soil microbial community diversity and soil microenvironments in a temperate cropland in China. PLoS One 9:e108555. doi: 10.1371/journal.pone.0108555
Zhou, Z., Zhang, S., Jiang, N., Xiu, W., Zhao, J., and Yang, D. (2022). Effects of organic fertilizer incorporation practices on crops yield, soil quality, and soil fauna feeding activity in the wheat-maize rotation system. Front. Environ. Sci. 10:1058071. doi: 10.3389/fenvs.2022.1058071
Zingore, S., Mutegi, J., Agesa, B., Tamene, L., and Kihara, J. (2015). Soil degradation in sub-Saharan Africa and crop production options for soil rehabilitation. Better Crops Plant Food 99, 24–26. Available at: https://hdl.handle.net/10568/68702
Zougmoré, R. B. (2003). Integrated water and nutrient management for sorghum production in semi-arid Burkina Faso (doctoral dissertation). Wageningen University and Research Centre: Wageningen, 205.
Zougmoré, R., Mando, A., and Stroosnijder, L. (2004). Effect of soil and water conservation and nutrient management on the soil–plant water balance in semi-arid Burkina Faso. Agri. Water Manag. 65, 103–120. doi: 10.1016/j.agwat.2003.07.001
Zuluaga, M. Y. A., de Oliveira, A. L. M., Valentinuzzi, F., Tiziani, R., Pii, Y., Mimmo, T., et al. (2021). Can inoculation with the bacterial biostimulant Enterobacter sp. strain 15S be an approach for the smarter P fertilization of maize and cucumber plants? Front. Plant Sci. 12:719873. doi: 10.3389/fpls.2021.719873
Keywords: Burkina phosphate rock, calcined phosphate, phospho-compost, rhizosphere soil, chicken manure, sorghum
Citation: Sagnon A, Traore M, Tibiri EB, Zongo S, Bonkoungou IJO, Nakamura S, Barro N, Tiendrebeogo F and Sarr PS (2024) Enhancing the use of phosphate rock through microbially-mediated compost transformation to improve agronomic and economic profitability in Sub-Saharan Africa. Front. Sustain. Food Syst. 8:1445683. doi: 10.3389/fsufs.2024.1445683
Edited by:
Anne Tremier, UR Opaale - INRAE, FranceReviewed by:
Xiaomeng Chen, Northeast Agricultural University, ChinaMohd Huzairi Mohd Zainudin, University of Putra Malaysia, Malaysia
Copyright © 2024 Sagnon, Traore, Tibiri, Zongo, Bonkoungou, Nakamura, Barro, Tiendrebeogo and Sarr. This is an open-access article distributed under the terms of the Creative Commons Attribution License (CC BY). The use, distribution or reproduction in other forums is permitted, provided the original author(s) and the copyright owner(s) are credited and that the original publication in this journal is cited, in accordance with accepted academic practice. No use, distribution or reproduction is permitted which does not comply with these terms.
*Correspondence: Papa Saliou Sarr, c2FsaW91QGFmZnJjLmdvLmpw
c2FycnAwMjk1QGppcmNhcy5nby5qcA==