- 1Department of Nutrition and Food Processing, Al-Huson University College, Al-Balqa Applied University, Irbid, Jordan
- 2Department of Nutrition and Food Technology, School of Agriculture, The University of Jordan, Amman, Jordan
- 3Department of Food Science and Nutrition, Dankook University, Cheonan-si, Republic of Korea
Objective: Investigating the functional properties of treated flour composites derived from fruit by-products of avocado (Persea americana), kiwifruit (Actinidia deliciosa), pineapple (Ananas comosus), and pomegranate (Punica granatum) skins, peels, and/or seeds combined, aiming to understand their stability and potential applications in food products.
Methods: Fruit by-product flours were used to replace all-purpose wheat flour in ratios of 5, 10, 15, and 20%. The treated flours were evaluated for physicochemical characteristics and used in cookie preparation, with 100% all-purpose wheat flour as the control.
Results: The water holding capacity (WHC) of composite flours increased with higher substitution levels, regardless of the holding temperature (25, 35, 45, and 55°C), with the most significant increase observed in pomegranate treatments. Furthermore, freeze–thaw stability and viscoelasticity characteristics generally decreased for all composite flours compared to the control, likely due to gluten content dilution. The activation energy of the treated flours ranged from 11.1 to 62.0 kJ/mol. Therefore, gelatinization properties, formulation, and processing were affected by temperature variations due to viscosity dependence and activation energy requirements. Consumer testing indicated that cookies made with fruit by-product flours had an acceptable overall liking score of more than 6 out of 9.
Conclusion: Overall, the results suggest that utilizing fruit by-products for treated flours can offer valuable natural sources of nutrients with enhanced functional properties, highlighting potential benefits for various food products.
1 Introduction
There are increasing demands in fruit production due to the increasing markets for processed fruits, including juice, pulps, nectars, and jams (Pfaltzgraff et al., 2015). On the other hand, hundreds of millions of people are suffering from malnutrition at the moment, according to the FAO, as a result of a shortage of staple foods such as starchy cereals, tuber crops, and roots. This might be the reason behind the shift in better utilization of dietary habits and food processing strategies away from traditional plant-based diets and toward alternative foods, such as fruit and vegetable by-products and the by-products of the milling industry (Teshome et al., 2023; Al-Marazeeq, 2018; Al-Marazeeq et al., 2017). The use of food by-products is expected to enhance food security in light of the scarcity of food resources.
Several agricultural by-products from various sources are usually discarded by the food and pharmaceutical industry or used as animal feeds or fertilizers, regardless of their nutritional value (Ayala-Zavala et al., 2011). Fruit by-products, in particular, are considered one of the most promising biodegradable wastes that can be treated and used in various food applications. Cost reduction, nutritional additives, and fillers are representative advantages of such applications (Sancho et al., 2015). El-Baroty et al. (2014) reported remarkable antioxidant activity of fruit by-products, with mango kernel peel and pomegranates having an antioxidant activity comparable to that of butylated hydroxyanisol (BHA). An improvement in yogurt quality parameters including prebiotic contents was reported by Sah et al. (2016) when using pineapple waste powder.
Fruit by-products were reported to be a significant source of vitamins, fibers, phenolic compounds, minerals, and antioxidants (Saleh et al., 2021; Sancho et al., 2015). For example, avocado (Persea americana) seeds (i.e., representing 26% of the fruit) contain more than 70% of the fruit’s amino acids and have a substantial quantity of antioxidant activity (Hui, 2006). Raw pineapple wastes are usually high in crude fibers (16–25%), soluble sugars (40–75%), and pectin (Monspart-Senyi, 2006). The skins of pomegranate (Punica granatum L., Peel) and kiwifruit (Actinidia deliciosa) are also regarded as important sources of antioxidants (Hui, 2006). Moreover, pomegranate was reported to contain significant amounts of tannin, which has antibacterial and anti-inflammatory functions (Costantini et al., 2014).
The use of fruit by-products thus provides natural sources of valuable nutrients. For instance, El-Baroty et al. (2014) evaluated the total phenolic compounds and antioxidant activities in mango (Mangifera indica L.) seed pulp and kernel, pomegranate (Punica ranatum L.), and peanut (Arachis hypogaea L., Giza 6, shell). Pomegranates and mango kernel peel have been shown to exhibit radical-scavenging properties comparable to BHA. Sah et al. (2016), in the same manner, studied the effect of pineapple waste powder on probiotic growth, antioxidant, and anti-mutagenic activities in yogurt. The authors reported a 0.3–1.4 log cycle increase in probiotic populations as a result of pineapple supplementation and a significant increase in antioxidant activities. De Camargo et al. (2014) added peanut skins in ratios of 1.3, 1.8, and 2.5% to cookies, aiming to increase their polyphenol content. An addition of 2.5% resulted in a 52% increase in insoluble fiber contents and 30% polyphenols, and antioxidant activities increased with the increased peanut skin addition.
The use of fruit by-products in food could increase biological values and enhance functional properties, additionally reducing fruit waste. This work would provide a reference for understanding the physiochemical properties of fruit by-product composites. The information would also be useful for products’ functionality and quality control. Although the use of fruit by-products enhanced the bioactive compounds, no information in the literature was focused on the effect of fruit by-products on the processing functionality of these compounds. Therefore, the objectives of this work were to investigate the impacts of partially replacing wheat flour with flours made using selected fruit by-products on the functional characteristics of flour mixtures. The study also aims to investigate the temperature-viscosity dependence and activation energy of fruit by-products and the potential utilization of treated flour in the production of cookies.
2 Materials and methods
2.1 Materials
Avocado (Persea americana), kiwifruit (Actinidia deliciosa), pineapple (Ananas comosus), and pomegranate (Punica granatum) skins, peels, and/or seeds combined were purchased from a local market in Amman, Jordan, and kept frozen (−20°C) until use. Fruit by-products were blanched (90°C for 2 min) before cooling to room temperature (23.2°C). Blanching was performed to increase photochemicals availability according to Saleh et al. (2021). Blanched fruit by-products were dried separately in a conventional oven (PRECISION Winchester, VA, USA) at 50°C for 48 h. Dried samples were ground into flour and sieved to pass through a 100 μm sieve. Fruit by-product flours were then used to prepare treatments.
2.2 Design of the experiment
For the treatments formulation, fruit by-product flours were used to replace whole-purpose wheat flour in ratios of 5, 10, 15, and 20%. The treated flours were then thoroughly mixed using household mixer (ModelKitchenAid KSM150PSER Artisan, USA) at a speed of 6 for 15 min before physiochemical measurements and cookie preparation. Whole-purpose (100%) wheat flour was used as the control.
2.3 Proximate composition
Ash, protein, lipid, moisture, and fiber contents of avocado, kiwifruit, pineapple, and pomegranate by-products were determined according to AOAC (2011). Carbohydrate content was calculated by difference. The analyses were carried out in duplicate. Moisture content was determined by drying the samples of 5 g at 105°C in air dried oven (Memmert, Germany) to constant weight according to method number 925.09b AOAC (2011). For protein content, nitrogen content using Kjeldahl method with a factor of 6.25 was used to determine protein content following AOAC (2011) number 920.87. DK20 heating digester and UDK129 distillation unit (VELP Scientific, Italy) were used to determine the nitrogen content. Titration was carried out using 0.1 N HCl, and the protein content was calculated. Fat content was determined using soxlet (electrothermal, UK) method according to AOAC (2011) number 930.09. Fat content was extracted from 5 g sample using petroleum either. Then the solvent was separated by rotary evaporator (HEIDOLPH, LABORATA 4001, Germany) at 40–60°C, the bottom flask was weighed and fat content was calculated.
Ash was determined by incinerating samples in a muffle furnace (MF-120 Ash furnace Ankara, Turkey) at 550°C according to method 923.03 AOAC (2011). Total fiber content was determined using Megazyme Dietary Fiber Assay Kit (K-TDFR) according to AACC Method 32-45.01, AACC Method 32-50.01, AOAC Method 2009.01 and AOAC Method 2011.25.
2.4 Water holding capacity
A 5% of homogenized suspensions (×120, Igenieurbuero CAT, Stufen, Germany) of the treated flours were used to measure treatments WHC as described by Saleh et al. (2017). The treated flours were held separately at 25, 35, 45, and 55°C for 1 h before the WHC measurements. After 1 h of holding at the required temperature, the dispersed samples were centrifuged (Eppendorf, 5810R, Hamburg, Germany) at 3,800 rpm for 30 min. Sediments were weighted, and WHC (%) was calculated using Equation 1. Duplicate measurements were performed, and averages were reported.
2.5 Freeze–thaw stability
Aqueous dispersions of the treated flours (5% wt/vol) were prepared and used for freeze–thaw-stability measurements. In summary, treatments were gelatinized at 95°C with continuous shaking for 30 min. After cooling to 25°C, the gelatinized treatments were subjected to three consecutive freeze–thaw cycles. In summary, gelatinized treatments were frozen at −20°C for 24 h., thawed at 30°C for 2 h, and then centrifuged for 30 min at a speed of 3,800 rpm. The degree of syneresis per freezing cycle was expressed as the freeze–thaw stability of the treatments. Each freeze–thaw stability cycle was performed in triplicate, and the results were illustrated as the average for each cycle.
2.6 Rheological measurements
According to the procedure outlined by Saleh et al. (2016), rheological measurements were conducted. To summarize, slurry was prepared by combining 2.5 grams of each treatment with 40 ml of distilled water in polyethylene plastic tubes. Before the rheological measurements, the treatments were homogenized (×120, Ingenieurbüro CAT, Staufen, Germany) in the tubes. The rheological measurements were then performed after holding the samples for 1 h at temperatures of 25, 40, 60, or 70°C in a Haake viscometer 550 holding cell (Thermo Fisher Scientific, Waltham, MA, USA). Apparent viscosity of the treatments was measured during shear rates ranging from 1 to 200 s1. The flow behavior index and consistency coefficient of the treatments were also evaluated using the Herschel-Bulkley model (Equation 2).
Where τ is shear stress (mPa), τo is yield stress (mPa), m is the consistency coefficient (mPa.sn), γ˙ is the shear rate (s−1) and n is the flow behavior index (dimensionless).
2.7 Modeling viscosity and activation energy
Plots of ln η versus 1/T were determined, and slopes were calculated. The activation energy was calculated using Arrhenius-type equations (Pineappleul et al., 2003; Equation 3) with modifying the D value to be the viscosity of fruit by-product treated flour.
Where Ea = activation energy (kJ/mol), R = universal gas constant (8.3143 kJ/mol K), Ta = absolute temperature (°K) during viscosity measurements, A = pre-exponential factor (Pa.s) and η = viscosity (Pa.s).
2.8 Pasting measurements
A Rapid Visco Analyzer (RVA Model 4, Newport Scientific Inc., Warriewood, Australia) was used to measure the peak, trough, setback, breakdown, final viscosities, and pasting temperature of the treatments. The AACC approved method 76–21 was used as an RVA protocol (AACC, 2000), as described by Saleh et al. (2016). Data received from the RVA were recorded and processed using Thermocline version 1.2 software (Newport Scientific Inc., Warriewood, Australia). All samples were measured in duplicate.
2.9 Cookies preparation
For cookies making, sugar and butter were thoroughly mixed (i.e., 5 min) before adding the dry and wet ingredients. The formed dough was then mixed for an additional 12 min to form cohesive dough. Formed dough balls were then baked in a household oven at 180°C for 25 min. Ingredients and quantities (grams) used for cookie preparation are presented below. A full batch of 20 cookies was prepared. After baking, the cookies were cooled on racks at room temperature (~23.2°C) for 2 h before being stored in an airtight Ziploc bag (Table 1).
2.10 Consumer evaluation of cookies made using treated flour
Hedonic sensory perception of cookies made using wheat flour containing fractions of fruit by-products was assessed in a sensory evaluation laboratory, Department of Nutrition and Food Technology, School of Agriculture, The University of Jordan. Cookies were prepared 24 h before performing consumer-like testing. A total of 50 consumers participated in the consumer evaluation testing. According to Meilgaard et al. (1999), the three-digit identified samples were delivered to consumers at random in Styrofoam cups, and they were directed to complete a 9-point hedonic scale (1 being severely disliked and 9 being strongly loved). The test was sequential monadic and sample presentation orders were balanced at each serving position. Between each sample, consumers were asked to wash their palates. Overall liking, appearance, texture, flavor, aftertaste, color, and graininess of each sample were evaluated.
2.11 Statistical analysis
Using JMP version 10.0, analysis of variance (ANOVA) was performed on the data from physical treatments (SAS Institute, Cary, NC). At a 5% level of probability, the least significant differences (LSD) between treatments were identified.
3 Results and discussion
3.1 Chemical composition of flours
Table 2 shows the chemical composition of various fruit by-product flours, including avocado, kiwifruit, pineapple, and pomegranate. The protein content of fruit by-product flours varies considerably, ranging from 1.0% in some cases to 15.4% in others. This variation suggests that different fruit by-products can provide a diverse range of protein levels, potentially influencing their functional properties in food applications. For instance, higher protein content might contribute to improved nutritional value or altered texture in baked goods. Lipid content also shows significant variability, from 0.2 to 2.8%. While generally low, the lipid content in these flours could affect the fat content and overall flavor profile of products made with them. The relatively low lipid levels suggest that these by-products may not substantially contribute to the fat content of cookies or other baked goods but may still influence the sensory attributes to some extent (Krajewska and Dziki, 2023; Bhardwaj et al., 2022).
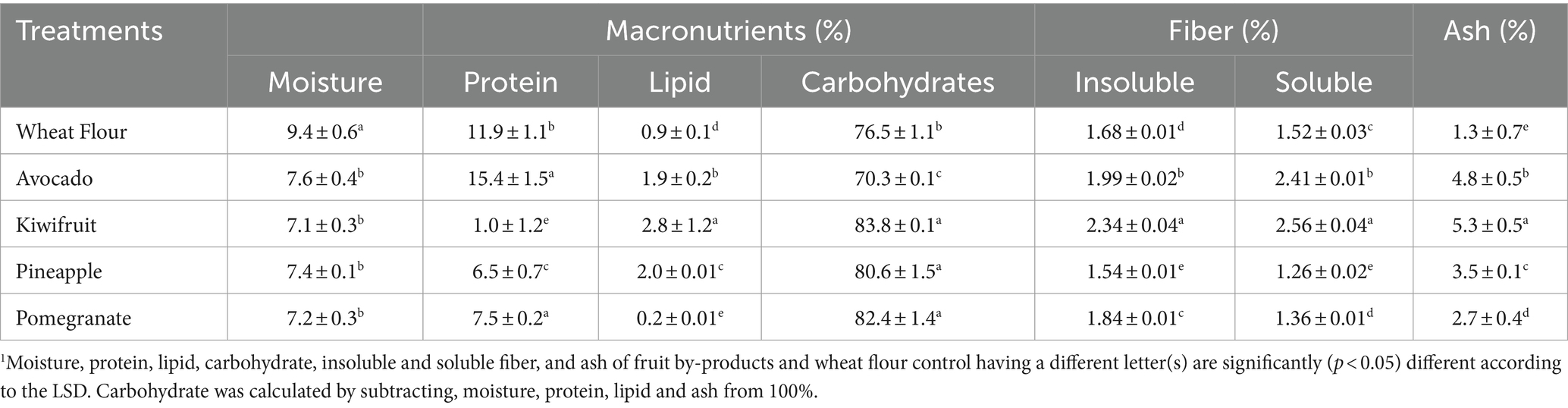
Table 2. Chemical composition (dry matter) of avocado, kiwifruit, pineapple, and pomegranate fruit by-product flours.1
Carbohydrate content is notably high across all fruit by-product flours, ranging from 70.3 to 83.8%. This high carbohydrate content aligns with the nature of fruit by-products, which are often rich in fiber and sugars. This could potentially impact the texture and sweetness of cookies, making them denser and sweeter compared to those made with traditional flour. The high carbohydrate levels may also affect the overall structure and moisture retention of the final baked products.
Ash content of the fruit by-product flours ranges from 1.3 to 5.3% and is significantly higher than that of the control. This increased ash content indicates a higher mineral content in these flours. Ask content might influence the nutritional profile of the cookies, potentially enhancing their mineral content, but could also impact the flavor and color of the final product (Krajewska and Dziki, 2023; Bhardwaj et al., 2022).
3.2 Water holding capacity of the treated flours
Table 3 provides a detailed examination of the Water Holding Capacity (WHC) of wheat flour when partially substituted with various percentages (0, 5, 10, 15, and 20%) of avocado, kiwifruit, pineapple, and pomegranate flours. These substitutions were analyzed over a range of temperatures (25°C, 35°C, 45°C, and 55°C) to understand how they influence the flour’s ability to retain water. The results showed that WHC varied significantly depending on the type of fruit flour and the level of substitution, with values ranging from 76.4% for the control (0% substitution) to an impressive 133.7% for the 20% pomegranate flour substitution. This indicates a clear trend: as the substitution level of fruit flour increases, the WHC of the flour also rises, irrespective of the holding temperature. This trend is critical because WHC is a key factor in determining the functional properties of flour in various food applications, influencing the texture, moisture retention, and overall quality of the final product.
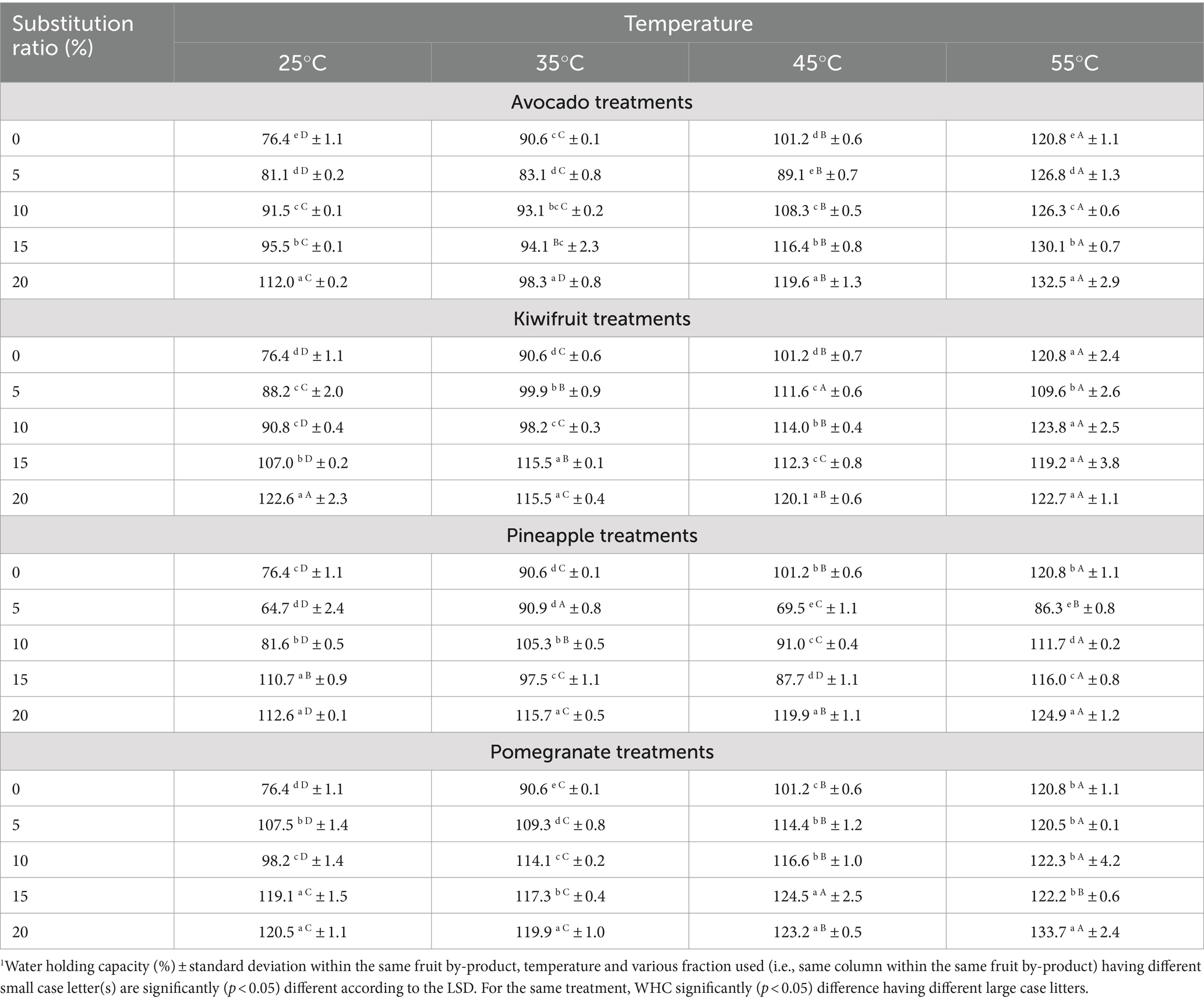
Table 3. Water holding capacity (WHC) of treatments containing 0, 5, 10, 15, or 20% of avocado, kiwifruit, pineapple and pomegranate by-product flours.1
For example, when wheat flour was substituted with 5, 10, 15, and 20% avocado flour and held at 25°C for 1 h, the WHC values increased from 81.1% at 5% substitution to 112.0% at 20% substitution. This suggests that even a modest substitution with avocado flour can enhance the water retention properties of wheat flour, which can be advantageous in baked goods and other products where moisture retention is crucial.
Similarly, the pomegranate flour substitution at 35°C demonstrated a consistent increase in WHC with higher substitution levels. Starting from 109.3% at 5% substitution, the WHC rose to 114.1, 117.3, and 119.9% for 10, 15, and 20% substitutions, respectively. This highlights the superior water-holding properties of pomegranate flour, making it a particularly effective ingredient for improving the hydration characteristics of flour-based products.
The study also revealed that holding temperature plays a significant role in enhancing WHC. As the temperature increased from 25 to 55°C, the WHC of the flour mixtures also increased. This is primarily due to the denaturation of proteins at higher temperatures, which alters their structure and enhances their ability to interact with water molecules. Denatured proteins unfold, exposing more sites for water binding, which results in higher WHC. Additionally, the interaction between proteins and polysaccharides under these conditions creates a more stable and cohesive network that effectively traps water, further boosting WHC (Smriti et al., 2023).
When comparing the WHC of different fruit flour treatments at the same substitution levels and temperatures, pomegranate flour consistently exhibited the highest WHC, with values ranging from 98.2 to 133.7%. This superior performance is attributed to the unique chemical composition of pomegranate by-products, which include higher levels of proteins and fibers such as cellulose, lignin, and both soluble and insoluble fibers. The presence of dietary fibers, particularly those rich in hydroxyl groups, facilitates water absorption in the flour dough through hydrogen bonding, leading to enhanced WHC (Kohajdova et al., 2011).
These findings are consistent with previous research. Hunter (2015) reported that increasing the fiber content in flax fiber significantly boosts water uptake, with substantial increases in WHC observed as the content of whole locust and insoluble locust bean gum increases. The study highlighted that components like cellulose, lignin, and pectin could enhance WHC by 32, 67, and 84%, respectively. This supports the current findings, suggesting that the introduction of high-fiber fruit flours into wheat flour not only improves water retention but also enhances the overall functionality of the flour in food applications.
Additionally, Ngoc et al. (2012) identified a strong positive correlation (r = 0.82) between soluble non-cellulosic polysaccharides and WHC. This correlation underscores the importance of soluble fibers in improving the hydration properties of flour mixtures. The interaction between these fibers and water molecules plays a critical role in stabilizing the dough matrix, which is essential for producing high-quality baked goods and other flour-based products.
3.3 Freeze–thaw stability of the treated flours
Table 4 provides a comprehensive analysis of the freeze–thaw stability of wheat flour when partially substituted with varying percentages (5, 10, 15, and 20%) of fruit by-products, specifically avocado, kiwifruit, pineapple, and pomegranate flours. The table presents syneresis percentages (%) across three freeze–thaw cycles for each fruit treatment, offering insights into the ability of these modified flours to retain water and maintain stability during freezing and thawing.
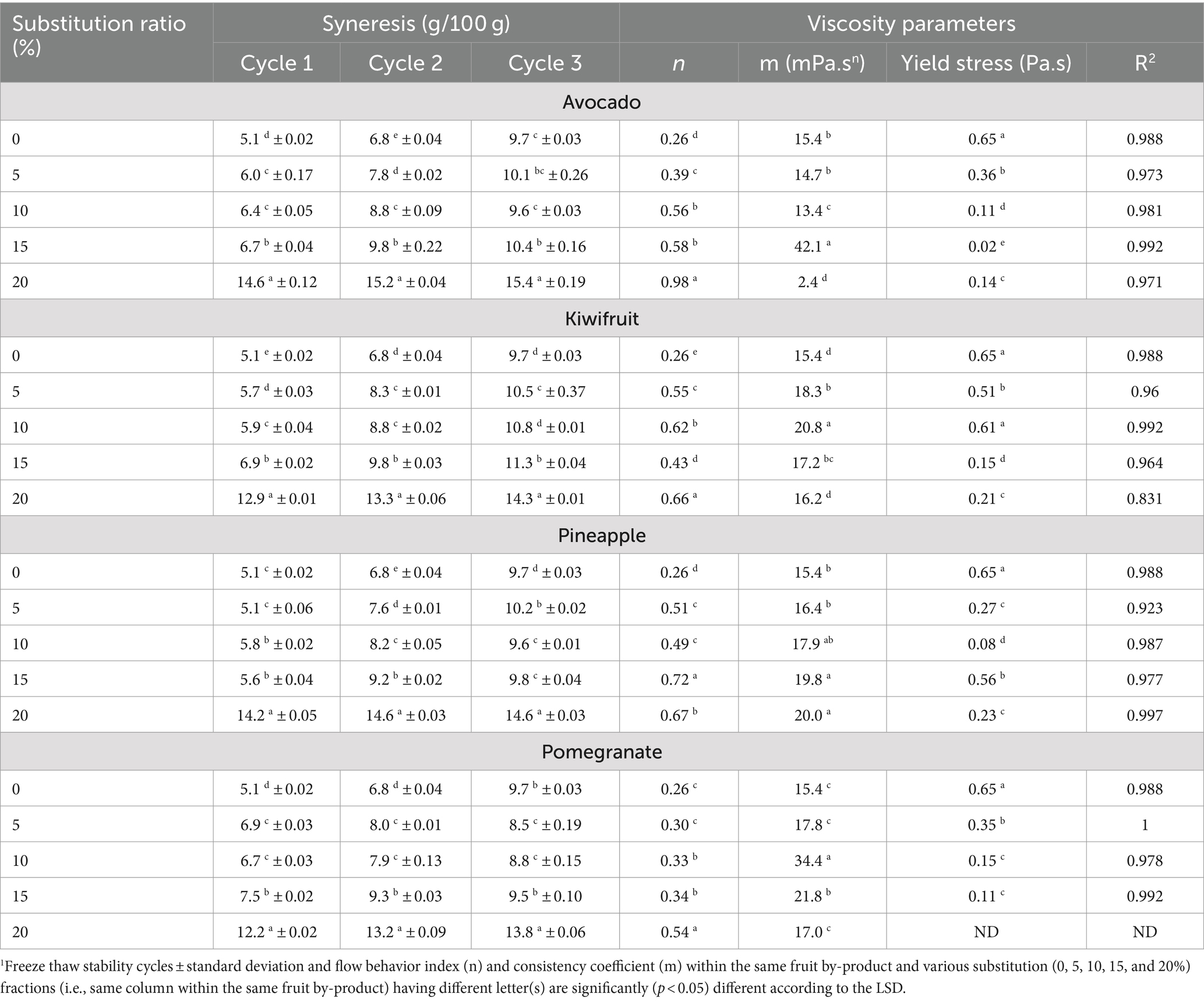
Table 4. Freeze–thaw stability, flow behavior index (n) and consistency coefficient (m) of treatments containing 0, 5, 10, 15, or 20% of avocado, kiwifruit, pineapple and pomegranate by-product flours.1
During the first freeze–thaw cycle, syneresis values ranged as follows: from 6.0 to 14.6% for avocado treatments, 5.7 to 12.9% for kiwifruit, 5.1 to 14.2% for pineapple, and 6.9 to 12.2% for pomegranate. These values indicate that the degree of water separation varied depending on both the type and level of fruit flour substitution. A similar pattern was observed in the second and third freeze–thaw cycles, where syneresis generally increased with higher substitution levels of fruit by-products, reflecting a decrease in the freeze–thaw stability of the wheat flour blends.
The increasing syneresis with higher substitution levels suggests that these treated flours have a limited ability to form a stable matrix network during the gelatinization process. This is critical because a well-formed matrix is essential for maintaining the structural integrity of dough during freeze–thaw cycles. The weakened stability observed in the treated flours is consistent with findings from other studies. For example, Kuchtová et al. (2018) reported a reduction in the stability of wheat dough when grape skins were incorporated, which was attributed to a decrease in gluten content. Gluten is crucial for forming the elastic network that traps moisture and gases, and its reduction can lead to increased syneresis and diminished freeze–thaw stability.
Although the incorporation of fruit by-products into wheat flour results in reduced freeze–thaw stability, there are potential strategies to mitigate this issue. One effective approach is the use of hydrocolloids, which are known for their ability to improve the water retention and structural stability of flour-based products. Several studies have demonstrated the positive impact of hydrocolloids on freeze–thaw stability. For instance, Saleh et al. (2016) found that the syneresis of acorn starch was significantly reduced when hydrocolloids such as carboxymethylcellulose, carrageenan, and xanthan gum were added. These hydrocolloids interact with water and starch molecules, forming a more stable network that minimizes water separation during freeze–thaw cycles.
3.4 Rheological properties of the treated flours
Table 4 also presents the flow behavior index (n) and consistency coefficient (m) of wheat flour substituted with 5, 10, 15, and 20% of avocado, kiwifruit, pineapple, and pomegranate by-product flours, respectively. Flow behavior index ranged from 0.30 to 0.98 regardless of fruit by-product and fractions used, compared to 0.26 for the control. Pomegranate treatments had the lowest flow behavior index value ranged from 0.3 to 0.54 compared to avocado (0.39–0.98), kiwifruit (0.55–0.66), and pineapple (0.51–0.67), but were greater than the control (0.26). Additionally, the results, generally, showed that the flow behavior index increased with a higher proportion of fruit by-product flour. The consistency coefficients for the treatments varied between 2.4 and 42.1, regardless of the fruit by-product fraction used. These findings suggest that the viscoelastic behavior of the treated flours was significantly influenced (p < 0.05) by changes in the chemical composition of the treatments (Arrieta-Durango et al., 2022). The presence of proteins and naturally occurring water-soluble gums likely contributed to the rise in the flow behavior index in the fruit by-product treatments. Pectin, which forms a substantial part of citrus fruit peels, is expected, along with certain monosaccharide’s and organic acids (primarily citric acid), to enhance the viscosity of the treatments (Hui, 2006).
The viscoelastic properties exhibited by wheat gluten play a crucial role in determining the distinctive attributes of various food products derived from wheat and in the application of wheat gluten proteins in diverse food formulations. Gluten aggregation induced by heat involves the formation of cross-links among different protein fractions present within gluten. Gluten elasticity is primarily governed by glutenin, whereas the viscosity of the network is influenced by gliadin. Subsequent to the application of heat, glutenin and gliadin undergo crosslinking interactions, resulting in the formation of an extensive protein network (Delcour et al., 2012); therefore, dilution of gluten protein by adding other flours affects the rheological properties of the wheat flour.
Al-Marazeeq et al. (2019) found that the water extract of sumac fruit affected the rheological properties of the wheat dough, such as increasing water uptake and strengthening the dough, which attributed to the extractable components such as acids that act as oxidizing agents, anthocyanin, tannins, and polyphenols that act as natural antioxidants altering the protein structure.
3.5 Viscosity and activation energy of the treated flours
Table 5 presented results demonstrating a linear relationship (i.e., with a slope of Ea/R) and a decrease in viscoelasticity across all fruit by-products, regardless of concentration, over the range of temperatures tested. Among the numerical empirical models that correlate the effect of temperature on the viscosity of materials, the Arrhenius equation is the most commonly proposed (Zhou et al., 2000). The observed results are likely due to variations in the chemical composition of the treatments. Mukprasirt et al. (2000) for example noted that differences in the size, shape, and gelatinization properties of rice flour significantly impact the water absorption rate and swelling power, and therefore, viscoelastic properties. Other researchers have also suggested that the temperature dependence of viscosity is influenced by molecule-to-molecule interactions at elevated temperatures (Maskan and Gogus, 2000).
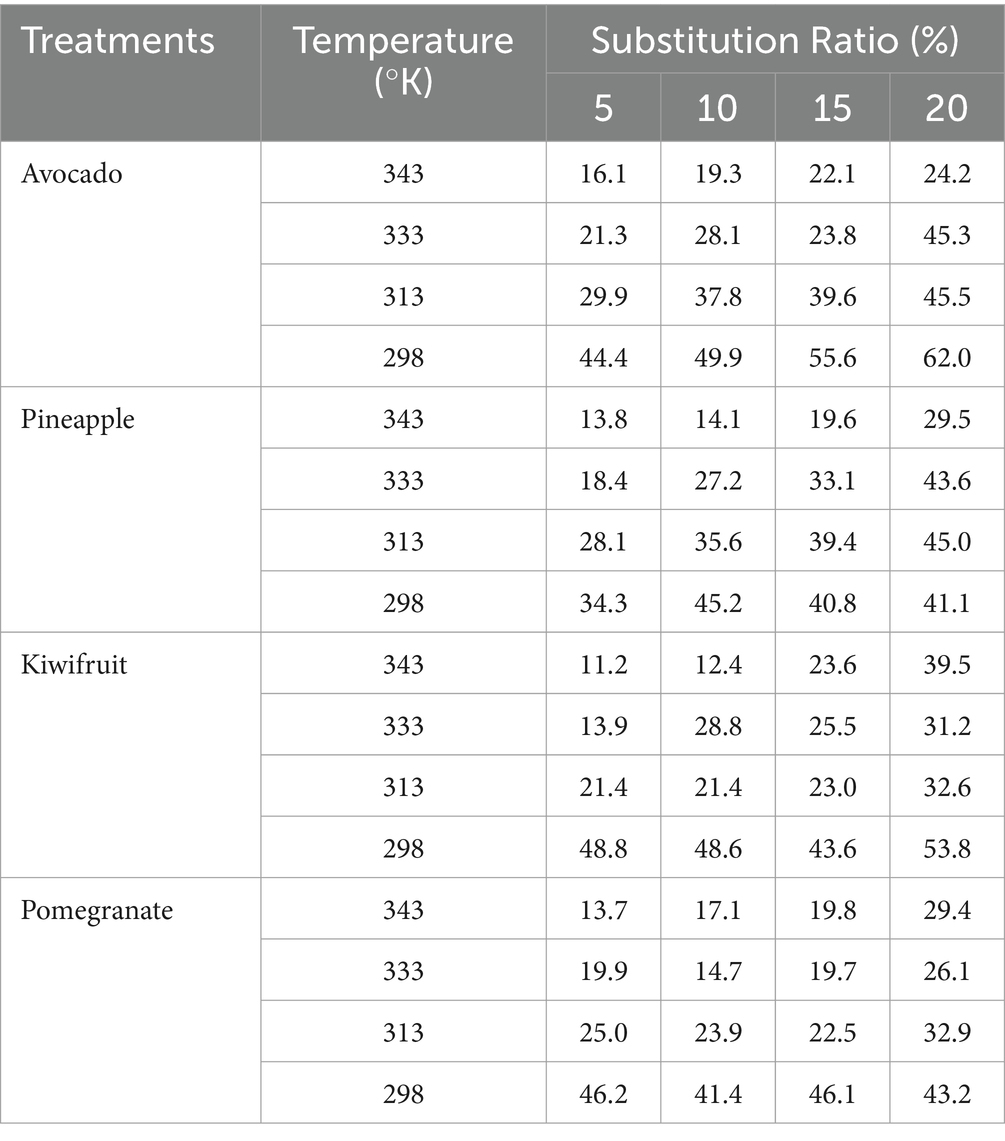
Table 5. Activation energy (kJ/mol) for different levels of treatments (i.e., flours) at various measuring temperatures.
The activation energy of the treatments at various temperatures is presented in Table 5. The activation energy was calculated using Arrhenius equations, where η is viscosity, Ta is temperature, A is a constant, Ea is the activation energy, and R is the universal gas constant. The activation energy Ea derived from the Arrhenius equation ranged from 11.1 to 62.0 kJ/mol. The activation energy increased with the decrease in temperature and with the increase in treatment concentration.
The activation energy of treatments reflected the sensitivity of viscosity measurements to temperature, hence altering the thermal stability of treatments during processing. Activation energy is considered the most essential parameter in determining flour gelatinization kinetics (Malecki et al., 1998). The highest Ea reported in this study was for the 20% avocado flour treatment, implying a less favorable gelatinization treatment (Alvarez et al., 2017). These results were supported by the fact that the lower carbohydrate and greater protein contents resulted in greater gelatinization temperature and lower pasting properties of avocado flour treatments compared to other treatments. Protein content was reported to significantly contribute to the changes in its viscoelasticity (Alvarez et al., 2017).
3.6 Pasting properties of the treated flours
The pasting viscosities of the treated flours, detailed in Table 6, provide a comprehensive understanding of how the inclusion of fruit by-products such as avocado, kiwifruit, pineapple, and pomegranate impacts the rheological behavior of wheat flour. These viscosities are crucial indicators of how the flour will behave during cooking and processing, directly influencing the texture, consistency, and quality of the final food product. Peak viscosities ranged from 1,527 to 2,902 cP across the various treatments, showing considerable variation depending on the type and proportion of fruit by-product used. The control sample, which contained no fruit by-products, exhibited a peak viscosity of 2,390 cP. The variation in peak viscosities between the treated and control flours suggests that the inclusion of fruit by-products significantly alters the flour’s ability to absorb water and swell. This alteration is closely linked to the flour’s physicochemical properties, particularly its Water Holding Capacity (WHC). Flours with higher WHC typically show higher peak viscosities because they can absorb and retain more water during gelatinization. However, the introduction of fruit by-products, which often increases the fiber content, can disrupt this process. Fibers, especially those present in fruit by-products, are bulky and insoluble, potentially hindering the full hydration of starch granules and leading to reduced peak viscosities.
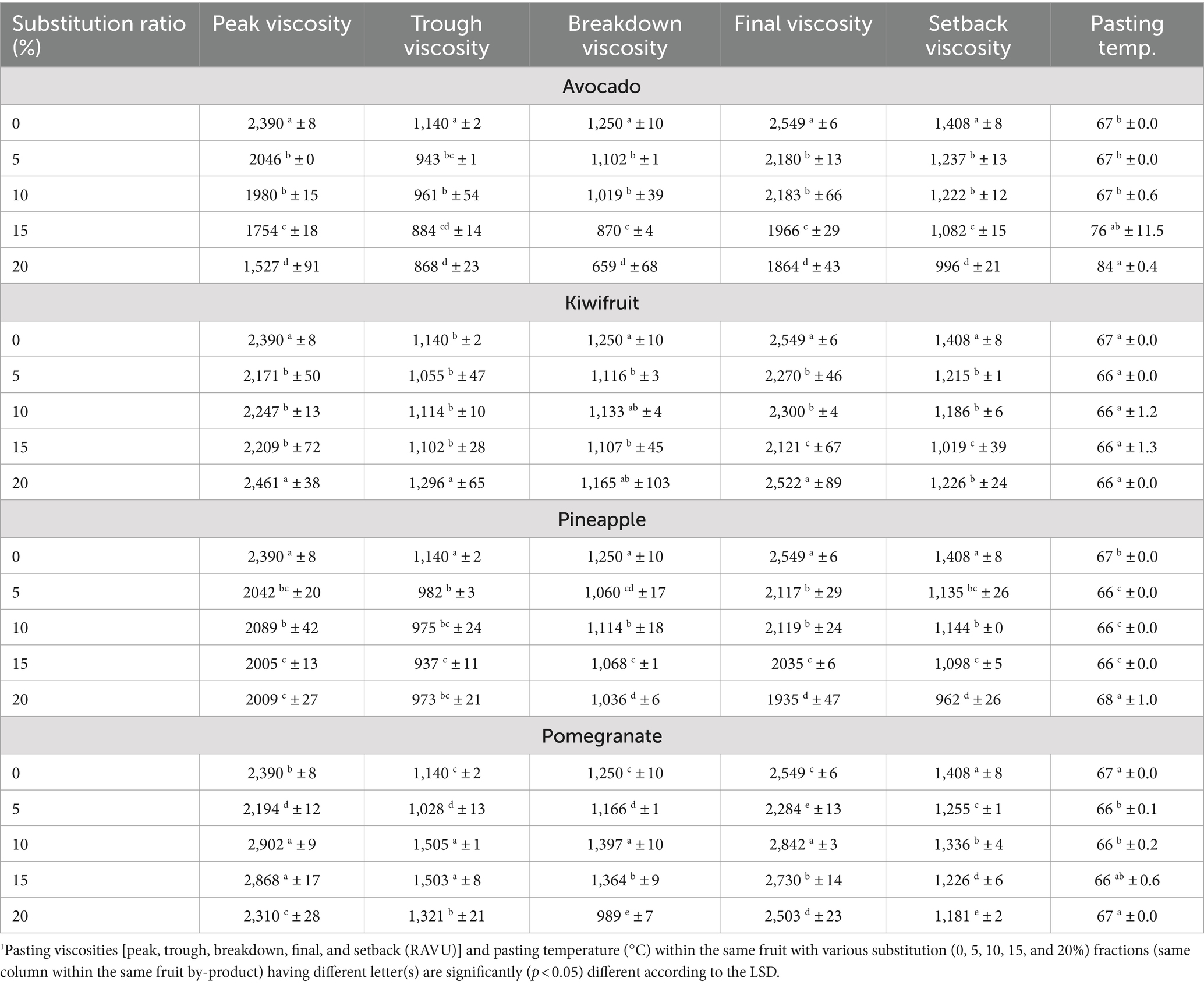
Table 6. Pasting viscosities [peak, trough, breakdown, final, and setback (cP)] and pasting temperature (°C) of flour treatments containing 0, 5, 10, 15, or 20% fractions of avocado, kiwifruit, pineapple and pomegranate by-product flours.1
Trough viscosities varied between 868 and 1,505 cP, compared to 1,140 cP in the control. The lower trough viscosities in some treatments suggest that the fruit by-products might weaken the starch network, making it less stable during continued heating. This reduction in stability is likely related to the flour’s syneresis, which refers to the separation of liquid from the gel matrix during storage or processing. Flours with higher syneresis are less able to retain water, leading to a less stable paste during heating and, consequently, lower trough viscosities.
Breakdown viscosity, the difference between peak and trough viscosities, reflects the starch granules’ stability during cooking. Breakdown viscosities ranged from 659 to 1,397 cP, with the control showing a value of 1,250 cP. Treatments that resulted in lower breakdown viscosities suggest that the starch granules in these flours were less stable and more prone to disintegration during cooking. This instability can be attributed to the altered protein-starch-lipid interactions in the presence of fruit by-products. Fibers from these by-products can disrupt the matrix that typically protects starch granules, making them more susceptible to breakdown under stress.
Final viscosities ranged from 1,864 to 2,842 cP, compared to 2,549 cP in the control. These values indicate that the treated flours have varying capacities to form stable gels upon cooling, which is crucial for the texture and shelf-life of the final product. The relationship between final viscosity and the flour’s physicochemical properties is evident; flours with higher WHC and lower syneresis are generally better at forming stable gels because they can retain more water and maintain a more cohesive structure.
Setback viscosities ranged from 962 cP in the 20% pineapple treatment to 1,336 cP in the 10% pomegranate treatment, compared to 1,408 cP in the control. Lower setback viscosities in some treatments suggest a reduced tendency for starch retrogradation, which can be advantageous for products that require a softer texture or extended shelf-life. However, the presence of fruit by-products can complicate this behavior. Increased fiber content can interfere with the retrogradation process by disrupting the starch matrix, resulting in lower setback viscosities and potentially altering the texture of the final product.
Results indicated a significant (p < 0.05) reduction in peak viscosity with the addition of fruit by-products, though some treatments deviated from this trend. This reduction is likely due to the higher fiber content introduced by the fruit by-products, which alters the chemical composition and structural integrity of the starch matrix. The relationship between pasting properties and physicochemical characteristics, such as WHC and syneresis, is crucial in understanding these changes. For example, a higher fiber content, which increases WHC, can also increase syneresis, leading to a less stable paste and reduced peak viscosity. The interaction between proteins, starches, and lipids plays a central role in forming the pasting profiles of flours. Fibers can disrupt these interactions, leading to reduced pasting viscosities (Saleh, 2017). The structure of proteins, rather than their quantity alone, is particularly important in determining pasting viscosities (Chiang et al., 2006). Even in treatments where avocado increased the protein content, the specific arrangement and interaction of these proteins within the starch matrix influence the stability of starch granules during heating and cooling, affecting the overall viscosity profile of the flour paste.
These findings underscore the complex interplay between the pasting properties and the physicochemical attributes of the treated flours. The inclusion of fruit by-products not only alters the chemical composition but also affects the structural interactions within the flour matrix, influencing key properties such as WHC, syneresis, and overall rheological behavior. This complexity highlights the importance of considering both compositional and structural factors when evaluating the functional properties of flours enhanced with fruit by-products. Understanding these relationships is critical for optimizing the use of such flours in various food applications, ensuring desired textures, stability, and shelf-life in the final products.
3.7 Consumer preference of cookies
Consumer attributes (i.e., overall, appearance, texture, flavor, aftertaste, color, and graininess liking) of cookies made using wheat flour substituted with fractions of avocado, kiwifruit, raw pineapple, and pomegranate by-product flours are presented in Table 7. Overall liking scores ranged from 6.3 for pomegranate (i.e., 15%) to 8.1 for the control. Although the addition of fruit by-products lowered overall liking scores of treatments compared to the control, results indicated that products were in the acceptable category in liking (>6.0). Cookies supplemented with the fractions of fruit by-products had a slight reduction in texture liking scores compared to the control, which was attributed to the increased fiber content of the treatments.
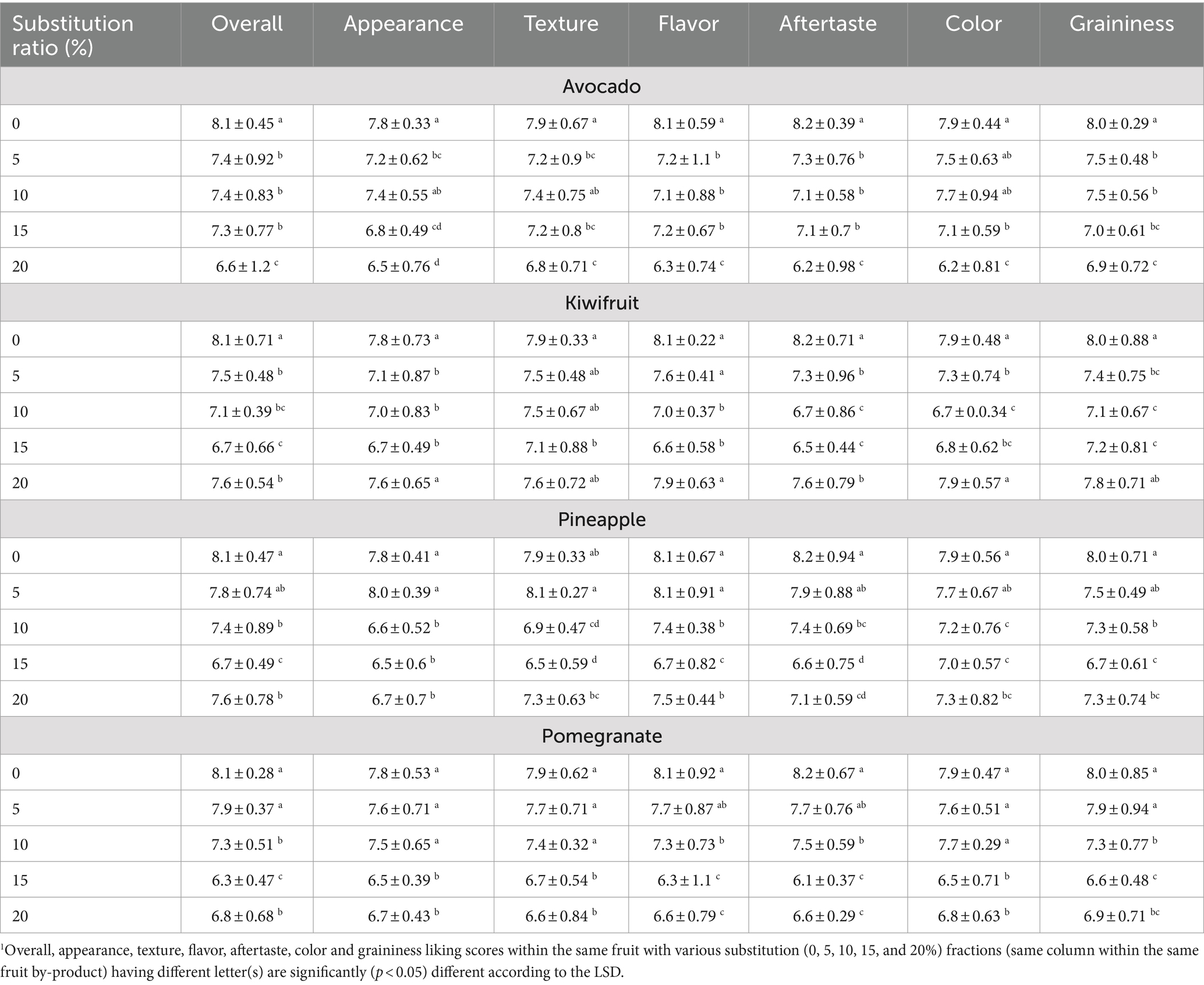
Table 7. Sensory properties of cookies made using wheat flour substituted with fractions of avocado, kiwifruit, pineapple, and pomegranate by-product flours.1
Appearance, flavor, aftertaste, color, and graininess liking scores varied (i.e., despite being lower than the control) but with no significant trends. For example, a treatment containing 5% pineapple was comparable to the control, which had hedonic scores ranging from 7.5 to 8.1 for overall, appearance, texture, flavor, aftertaste, color, and graininess liking scores that are very close to the classification “liked very much.”
The addition of fruit by-products with a dark brownish color negatively affected cookies’ color scores. Furthermore, the presence of a slight aftertaste of some fruit by-products, especially kiwifruit and pomegranate, may affect the cookies’ sensory characteristics. The greater the fruit by-product contribution in treatments, the lower the color liking score. For example, color liking scores significantly (p < 0.05) decreased from 7.5 to 6.2 and from 7.6 to 6.8 when avocado and pomegranate treatments increased from 5 to 20%. The dark brownish color of cookies made using various fractions of fruit by-products was attributed to the fruit by-product chemical composition. These results are similar to the findings by Turksoy and Ozkaya (2011), who reported a significant reduction in the color liking of cookies supplemented with pumpkin peel powder and carrot pomace powder rich in α-carotene.
In the same manner, the use of 15% kiwifruit and pomegranate treatment resulted in the lowest aftertaste liking score of 6.5 and 6.1, respectively, while the use of 20% avocado resulted in an aftertaste liking score of 6.2. This could be attributed to the phenolic, flavonoids, and tannin contents of these fruit by-products. Avocado peels were reported to have significant amounts of phenolic compounds (10,848.27 ± 162.34 mg GAE kg−1) and flavonoids (1,360.34 ± 188.65 mg EQ kg−1) that are believed to impart peel aftertaste (Rotta et al., 2016). The pomegranate content of tannins and alkaloids was also related to the lower aftertaste liking of produced cookies (Mohammad and Kashani, 2012).
4 Conclusion
The temperature dependence of fruit by-products was characterized to evaluate their suitability as a supplement to wheat flour in bakery products. Activation energy provided critical insight into the gelling mechanism during formulation and processing. The use of fruit by-products produced acceptable cookie characteristics, as expected to influence cookies’ nutritional aspects. Although the use of fruit by-products resulted in darker cookies’ colors, this had no impact on cookies’ sensorial attributes. Treatments with fruit by-product flours resulted in increased WHC, syneresis, and pasting viscosities; these results were attributed to the increased fiber content. Improvement in freeze–thaw stability may be achieved through the use of hydrocolloids that are known to provide stability in food applications. The nutritional value and anti-nutritional factors of fruit by-products are not studied and are believed to be a fruitful research area. Moreover, it would have been interesting to measure the antioxidant capacity of these cookies to get an idea of what happens with these types of compounds.
Data availability statement
The original contributions presented in the study are included in the article/supplementary material, further inquiries can be directed to the corresponding author.
Author contributions
KA-M: Conceptualization, Data curation, Formal analysis, Investigation, Methodology, Writing – original draft, Writing – review & editing. MS: Conceptualization, Data curation, Formal analysis, Methodology, Software, Validation, Writing – original draft, Writing – review & editing. MA: Data curation, Formal analysis, Visualization, Writing – review & editing. YL: Conceptualization, Software, Validation, Writing – review & editing.
Funding
The author(s) declare that no financial support was received for the research, authorship, and/or publication of this article.
Acknowledgments
The author expresses gratitude to all individuals involved in this research who made valuable contributions in various ways.
Conflict of interest
The authors declare that the research was conducted in the absence of any commercial or financial relationships that could be construed as a potential conflict of interest.
Publisher’s note
All claims expressed in this article are solely those of the authors and do not necessarily represent those of their affiliated organizations, or those of the publisher, the editors and the reviewers. Any product that may be evaluated in this article, or claim that may be made by its manufacturer, is not guaranteed or endorsed by the publisher.
References
AACC (2000). Approved methods of the American Association of Cereal Chemists. 10th Edn. St Paul: MN American Association of Cereal Chemists.
Al-Marazeeq, K. (2018). Evaluation of proximate composition and sensory attributes of dark chocolate fortified with wheat germ. Adv. J. Food Sci. Technol. 14, 103–107. doi: 10.19026/ajfst.14.5843
Al-Marazeeq, K., Abdulluh, M., and Angor, M. (2017). Characterization and sensory evaluation of pomegranate molasses fortified with wheat germ. Adv. J. Food Sci. Technol. 13, 178–181. doi: 10.19026/ajfst.13.4445
Al-Marazeeq, K., Al-Rousan, W., Al-obaidy, K., and Al-obaidy, M. (2019). The effect of using water sumac (Rhus coriaria L.) extract on wheat pan bread quality characteristics. Cereal Chem. 96, 847–855. doi: 10.1002/cche.10185
Alvarez, M. D., Cuesta, F. J., Herranz, B., and Canet, W. (2017). Rheometric non-isothermal gelatinization kinetics of chickpea flour-based gluten-free muffin batters with added biopolymers. Food Secur. 6, 2–18. doi: 10.3390/foods6010003
AOAC (2011). Official methods of analysis of the Association of Official Analytical Chemists. 18th Edn. Maryland, USA: Gaithersburg.
Arrieta-Durango, C., Henao-Rivas, L., and Andrade-Pizarro, R. (2022). Rheological behavior of passion fruit (Passiflora edulis) peel extract. Gels 8:566. doi: 10.3390/gels8090566
Ayala-Zavala, J. F., Vega-Vega, V., Rosas-Domínguez, C., Palafox-Carlos, H., Villa-Rodriguez, J. A., Siddiqui, M. W., et al. (2011). Agro-industrial potential of exotic fruit byproducts as a source of food additives. Food Res. Int. 44, 1866–1874. doi: 10.1016/j.foodres.2011.02.021
Bhardwaj, K., Najda, A., Sharma, R., Nurzyńska-Wierdak, R., Dhanjal, D. S., Sharma, R., et al. (2022). Fruit and vegetable Peel-enriched functional foods: potential avenues and health perspectives. Evid. Based Complement. Alternat. Med. 2022, 8543881–8543814. doi: 10.1155/2022/8543881
Chiang, S. H., Chen, C. S., and Chang, C. Y. (2006). Effect of wheat flour protein compositions on the quality of deep-fried gluten balls. J. Food Chem. 97, 666–673. doi: 10.1016/j.foodchem.2005.04.030
Costantini, S., Rusolo, F., De Vito, V., Moccia, S., Picariello, G., Capone, F., et al. (2014). Potential anti-inflammatory effects of the hydrophilic fraction of pomegranate (Punica granatum L.) seed oil on breast cancer cell line. Molecules 19, 8644–8660. doi: 10.3390/molecules19068644
De Camargo, A. C., Vidal, C. M. M., Canniatti-Brazaca, S. G., and Shahidi, F. (2014). Fortification of cookies with peanut skins: effect on the composition, polyphenols, antioxidant properties, and sensory quality. J. Agric. Food Chem. 62, 11228–11235. doi: 10.1021/jf503625p
Delcour, J., Joye, I., Pareyt, B., Wilderjans, E., Brijs, K., and Lagrain, B. (2012). Wheat gluten functionality as a quality determinant in cereal-based food products. Annu. Rev. Food Sci. Technol. 3, 469–492. doi: 10.1146/annurev-food-022811-101303
El-Baroty, G. S., Khalil, M. F., and Mostafa, S. H. A. (2014). Natural antioxidant ingredient from by-products of fruits. Am. J. Agric. Biol. Sci. 9, 311–320. doi: 10.3844/ajabssp.2014.311.320
Hui, Y. H. (2006). Handbook of fruits and fruit processing. Professional 2121 State Avenue, Ames, Iowa 50014, USA: Blackwell Publishing.
Hunter, U. (2015). Effect of water absorption on the mechanical properties of flax fiber reinforced epoxy composites. Adv. Sci. Technol. Res. J. 26, 1–6. doi: 10.12913/22998624/2357
Kohajdova, Z., Karovicova, J., Jurasova, M., and Kukurova, K. (2011). Application of citrus dietary fibre preparations in biscuit production. J. Food Nut. Res. 50, 182–190.
Krajewska, A., and Dziki, D. (2023). Enrichment of cookies with fruits and their by-products: chemical composition, antioxidant properties, and sensory changes. Molecules 28:4005. doi: 10.3390/molecules28104005
Kuchtová, V., Kohajdová, Z., Karovičová, J., and Michaela Lauková, M. (2018). Physical, textural and sensory properties of cookies incorporated with grape skin and seed preparations. Pol. J. Food Nut. Sci. 68, 309–317.
Malecki, A., Prochowska-Klisch, B., and Wojciechowski, K. T. (1998). Determination of the kinetics parameters of chemical reactions of the basis of non-isothermal measurement. J. Therm. Anal. Calorim. 54, 399–406. doi: 10.1023/A:1010143019676
Maskan, M., and Gogus, F. (2000). Effect of sugar on the rheological properties of sunflower oil-water emulsions. J. Food Eng. 43, 173–177. doi: 10.1016/S0260-8774(99)00147-8
Meilgaard, M., Civille, G. V., and Carr, B. T. (1999). Sensory Evaluation Techniques. 3rd Edn. CRC Press: Boca Raton, FL.
Mohammad, M. S., and Kashani, H. H. (2012). Chemical composition of the plant Punica granatum L. (pomegranate) and its effect on heart and cancer. J. Med. Plants Res. 6, 40, 5306–5310. doi: 10.5897/JMPR11.577
Monspart-Senyi, J. (2006). “Fruit processing waste management” in Handbook of fruits and fruit processing. ed. Y. H. Hui (Professional 2121 State Avenue Ames, Iowa 50014, USA: Blackwell Publishing).
Mukprasirt, A., Herald, T. J., and Flores, R. A. (2000). Rheological characterization of Rice flour-based batters. J. Food Sci. 65, 1194–1199. doi: 10.1111/j.1365-2621.2000.tb10264.x
Ngoc, T. T. B., Len, N. T., and Lindberg, J. E. (2012). Chemical characterization and water holding capacity of fibre-rich feedstuffs used for pigs in Vietnam. Asian-Aust. J. An. Sci. 25, 861–868. doi: 10.5713/ajas.2011.11294
Pfaltzgraff, L. A., Cooper, E. C., Budarin, V., and Clark, J. H. (2015). Food waste biomass: a resource for high-value chemicals. Agriculture 5, 10–29. doi: 10.1039/C2GC36978H
Pineappleul, M. N., Akpinar, E., Midilli, A., and Bicer, Y. (2003). Single layer drying behavior of potato slices in a convective cyclone and mathematical modeling. Energy Convers. Manag. 44, 1689–1705. doi: 10.1016/S0196-8904(02)00171-1
Rotta, E. M., de Morais, D. R. S., Biondo, P. B. F., dos Santos, V. J., Matsushita, M., and Visentainer, J. V. (2016). Use of avocado peel (Persea americana) in tea formulation: a functional product containing phenolic compounds with antioxidant activity. Acta Sci. Technol. 38, 23–29. doi: 10.4025/actascitechnol.v38i1.27397
Sah, B. N. P., Vasiljevic, T., McKechnie, S., and Donkor, O. N. (2016). Effect of pineapple waste powder on probiotic growth, antioxidant and antimutagenic activities of yogurt. J. Food Sci. Technol. 53, 1698–1708. doi: 10.1007/s13197-015-2100-0
Saleh, M. I. (2017). Protein-starch matrix microstructure during rice flour pastes formation. J. Cereal Sci. 74, 183–186. doi: 10.1016/j.jcs.2017.02.005
Saleh, M. I., Ajo, R., Ismail, K., and Ondier, G. (2016). Effects of hydrocolloids on acorn starch physical properties. Starch-Starke 68, 1169–1179. doi: 10.1002/star.201500304
Saleh, M. I., Amro, L., Barakat, H., Baker, R., Abu Reyash, A., Amro, R., et al. (2021). Fruit by-product processing and bioactive compounds. J. Food Qual. 2021, 1–9. doi: 10.1155/2021/5513358
Saleh, M. I., Ismail, K., and Ajo, R. (2017). Pasta quality as impacted by the type of flour and starch and the level of egg addition. J. Texture Stud. 48, 370–381. doi: 10.1111/jtxs.12238
Sancho, S. O., Silva, A. R. A., Dantas, A. N. S., Magalhaes, T. A., Lopes, G. S., Rodrigues, S., et al. (2015). Characterization of the industrial residues of seven fruits and prospection of their potential application as food supplements. J. Chem. 2015, 1–8. doi: 10.1155/2015/264284
Smriti, S., Vant Hag, L., Haritos, V., and Dhital, S. (2023). Rheological and textural properties of heat-induced gels from pulse protein isolates: lentil, mungbean and yellow pea. Food Hydrocoll. 143:108904. doi: 10.1016/j.foodhyd.2023.108904
Teshome, E., Teka, T. A., Nandasiri, R., Rout, J. R., Harouna, D. V., Astatkie, T., et al. (2023). Fruit by-products and their industrial applications for nutritional benefits and health promotion: a comprehensive review. Sustain. For. 15:7840. doi: 10.3390/su15107840
Turksoy, S., and Ozkaya, B. (2011). Pumpkin and carrot pomace powders as a source of dietary fiber and their effects on the mixing properties of wheat flour dough and cookie quality. Food Sci. Technol. Res. 17, 545–553. doi: 10.3136/fstr.17.545
Keywords: fruit by-products, composite flours, temperature-dependence viscosity, activation energy, rheological properties
Citation: Al-Marazeeq K, Saleh M, Angor M and Lee Y (2024) Cookie dough functional properties of partially replaced all-purpose wheat flour with powdered fruit skins and the hedonic perception of the resulting cookies. Front. Sustain. Food Syst. 8:1445206. doi: 10.3389/fsufs.2024.1445206
Edited by:
Kevser Karaman, Erciyes University, TürkiyeReviewed by:
Maria Simona Chis, University of Agricultural Sciences and Veterinary Medicine of Cluj-Napoca, RomaniaHatice Bekiroğlu, Yıldız Technical University, Türkiye
Copyright © 2024 Al-Marazeeq, Saleh, Angor and Lee. This is an open-access article distributed under the terms of the Creative Commons Attribution License (CC BY). The use, distribution or reproduction in other forums is permitted, provided the original author(s) and the copyright owner(s) are credited and that the original publication in this journal is cited, in accordance with accepted academic practice. No use, distribution or reproduction is permitted which does not comply with these terms.
*Correspondence: Khaled Al-Marazeeq, k.marazeq@bau.edu.jo