- 1Department of Environmental, Biological and Pharmaceutical Sciences and Technologies, University of Campania “Luigi Vanvitelli”, Caserta, Italy
- 2Department of Agricultural Sciences, University of Naples Federico II, Portici, Italy
- 3Horticell Lab, Department of Plants and Crops, Ghent University, Ghent, Belgium
- 4Center for Microbial Ecology and Technology (CMET), Ghent University, Ghent, Belgium
Unlocking the potential of sustainable agriculture relies on innovative approaches such as recycling crop waste and exploiting wastewater. Herewith, human urine derivatives were integrated into the fertigation of soilless-grown lettuce. The effects were remarkable: not only did they impact primary metabolites such as amino acids, proteins, and sugars, but they also influenced secondary metabolites such as polyphenols and anthocyanins, along with antioxidant enzyme activity. K-struvite, a urine precipitate with CaO and NaOH, elicited responses comparable to those of the control NPK and correlated with high biometric values. Interestingly, urine precipitate–CaO and electrodialysis (ED) concentrate stimulated accumulation of leaf polyphenols and anthocyanins, while urine precipitate–CaO induced high ABTS antioxidant capacity, highlighting their potential to enhance antioxidant activity and nutritive quality. Moreover, hydrolyzed urine and aurin induced a significant boost of essential amino acid content, underlining their role in increasing the nutritive value of lettuce. However, they also correlated with higher levels of MDA, a marker of lipid peroxidation, and reduced APX activity, indicating the need for further optimization. These findings underscore the promising role of human urine as an organic component in soilless lettuce fertilization, paving the way for more sustainable and resource-efficient agricultural practices.
1 Introduction
In a context in which the United Nations has officially estimated that the world population surpassed 8 billion last November 2023,1 a reliable source like AsiaNews (an official press agency of the Roman Catholic Pontifical Institute for Foreign Missions) has suggested that it may have already crossed 9 billion (Fusco et al., 2022). Therefore, the 50% increase in agricultural production estimated to meet food demand by 2050 (FAO, 2019) may be needed in the next 10 years. Indeed, intensifying agricultural production seems to be the only useful strategy to achieve sustainable livelihoods. However, intensive farming requires a high use of land and cultural inputs with severe ecological outcomes such as environmental pollution and serious risks to human health (Scotti et al., 2015). In addition, changing lifestyles, including the culture of abundance, have led to an unprecedented demand for food, feed, and energy. This high food demand is putting significant pressure on natural resources (De Laurentiis et al., 2020), partly due to the need to use synthetic substances (among which fertilizers) obtained from non-renewable sources, the employment of which has substantially contributed to the increase in yield and, consequently, in world food production (Hoque et al., 2010).
Synthetic fertilizers mainly used in resource-intensive agriculture, in particular nitrogen (N), entail a high economic cost for farmers, and their prices are linked to energy costs and gas prices; moreover, they represent one of the major sources of global warming, pollution, and risk for humans (Aryal et al., 2021). A significant portion of the nitrogen used in agriculture derives from atmospheric nitrogen fixed by the Haber–Bosch process, which accounts for approximately 1–2% of the world’s current total energy consumption (Matassa et al., 2015). Lassaletta et al. (2014) analyzed 50-year data from 1961 to 2009 in the FAO database from 124 countries (www.faostat.fao.org) about total nitrogen inputs and crop productivity. The use of synthetic fertilizers increased by a factor of 9 over the 50 years, significantly boosting agricultural yields but also strongly decreasing nitrogen use efficiency (NUE) of crops. Therefore, the overuse of N fertilizers, and/or the imbalance between N and other nutrients, reduces the NUE of the plant associated with an increase in nitrogen losses. Approximately 55% of the N fertilizers are not absorbed by plants but lost from the farm fields, mostly by leaching, volatilization, and denitrification (Tamme et al., 2010), thus negatively impacting air and downstream water quality. Excessive application of N fertilizer contaminates groundwater, pollutes drinking water, generates the eutrophication of aquatic ecosystems, increases the formation of ground-level ozone, and worsens the global warming and climate changes related to greenhouse gasses (GHG) (UNEP, 2023). In addition, excessive application of nitrogen fertilizer results in nitrate accumulation in the edible parts of crops, above the maximum residue levels (MRLs), thus threatening human health (Liu et al., 2014). In addition, the gastrointestinal microflora can reduce nitrate to nitrite causing methemoglobinemia, not only in children (blue baby syndrome) but also in adults (van Velzen et al., 2008). The formed nitrite may be further transformed in N-nitroso compounds (NOCs) by nitrosation, a reaction of nitrite with amides and amines. NOCs have been classified as potential carcinogens for humans and correlated with esophagus, stomach, colon, and rectum cancers (Loh et al., 2011).
Moreover, potassium and phosphorus fertilizers also require the use of non-renewable resources. In fact, they derive from the mining industries of sedimentary (Ciceri et al., 2015) and phosphate rocks (Ashley et al., 2011), respectively, and are expected to be depleted in the coming decades (Jurgilevich et al., 2016; El-Nakhel et al., 2021). Thus, crop management needs to be optimized by improving resource use efficiency (RUE) while ensuring global food security and environmental friendliness. In this context, increasing agricultural production per unit area through fertilizers produced from residual biomass or wastewater while reducing synthetic chemicals use is probably the best strategy for the sustainable intensification of agricultural systems from a circular economy perspective (Pretty and Bharucha, 2014). Recycling waste to obtain organic raw materials and using them in agriculture is the first step toward reducing the cost of energy and materials in the food production process (Xu and Geelen, 2018). Separating human urine from the rest of sewage and recycling it as fertilizer could provide an almost cost-free and locally available source of nitrogen, phosphorus, potassium, and micronutrients. Moreover, collecting urine could avoid wasting large amounts of water to flush it and reduce pressure on outdated and overburdened sewer systems (Wald, 2022). Therefore, nutrient recovery from human urine is an attractive aspect because it can close the nutrient loop (Beal et al., 2008; El-Nakhel et al., 2021; Van Gerrewey et al., 2021) and provide a viable alternative to synthetic fertilizers while also reducing the impact of agrichemicals and human waste on the environment (Martin et al., 2022). In fact, each person produces approximately 500 L of urine per year, contributing, on average, 79% of N, 47% of phosphorus, and 60–70% of potassium to domestic wastewater (Friedler et al., 2013; Kundu et al., 2022). Hence, recycling all the nutrients emitted within urine could account for approximately 20% of inorganic N and approximately 10% of inorganic P input to agriculture (Trimmer et al., 2019). In addition, the recovery of N (in particular ammonia) represents a low-energy no industrial-scale alternative to the Haber–Bosch process (Martin et al., 2022), while the recovery of P is a sustainable alternative to mineral phosphorus fertilizers (El-Nakhel et al., 2021). Indeed, the composition of human urine varies according to food intake, age, gender, race, amount of water consumed, physical activity, and environmental factors (Karak and Bhattacharyya, 2011; Sarigul et al., 2019). However, compared to other organic fertilizers, its derivatives provide a distinct nutrient profile that is more balanced and readily available for plants. Therefore, unlike other innovative practices, such as biochar or green manure, urine-derived fertilizers offer prompt nutrient availability and require less energy to be produced. According to Ernsting et al. (2011), adding biochar does not consistently increase soil carbon compared to mineral fertilizers or organic materials such as sawdust or green manure due to the fast microbe-mediated carbon turning into CO2 and biochar erosion. Fine biochar particles like soot can also become airborne and contribute to warming as soot. The impact on crop yields is highly variable and sometimes negative, posing risks for small farmers. Biochar-making cook stoves promoted for rural families require more biomass fuel and produce low amounts of biochar compared to industrial trials. Therefore, large agribusinesses, not small farmers, would benefit most from biochar, making it not sustainable for poor communities (Ernsting et al., 2011). Conversely, green manure enhances soil organic matter but provides a slower nutrient release (Kim et al., 2023). With their high nutrient concentration, urine-derived fertilizers provide a quick nutrient boost to plants, similar to synthetic fertilizers, offering a unique advantage for fast crop growth. This immediate availability of nutrients is especially beneficial during critical growth stages, such as exponential growth, flowering, and seed filling. Several techniques for recovering nutrients from human urine [e.g., struvite precipitation (Nakao et al., 2017), electrodialysis (ED) (De Paepe et al., 2018), and spontaneous biological hydrolysis of urea (Udert et al., 2006)] have been developed.
El-Nakhel et al. (2021) evaluated the effects of using urine derivatives (UDs) on lettuce (Lactuca sativa L.) plants grown in soilless culture in terms of biometric parameters, Soil Plant Analysis Development (SPAD) index, minerals, and organic acid content. The results, compared with those obtained from the use of commercial fertilizer, underscored the potential of urine derivatives as a sustainable resource for lettuce fertilization in an off-soil system. In this study, we evaluated the application of the eight UDs used by El-Nakhel et al. (2021) on the metabolic profile and main antioxidant enzyme activities of lettuce plants, demonstrating how, even at the physiological level, plants can benefit from the sustainable use of mineral nutrients recovered from source-separated urine.
2 Methods
2.1 Plant material, urine derivatives, and greenhouse experiment
A greenhouse experiment was set up at the Flanders Research Institute for Agriculture, Fisheries and Food, Melle, Belgium, to grow Lactuca sativa cv. Grand Rapids TBR (West Coast Seeds, Delta, British Colombia, Canada) in a soilless system (2-L pots filled with Grodan® rockwool grow-cubes) with a density of 12.5 plants m−2, under different human UDs integrated into the nutrient solutions (NSs). The greenhouse temperature was 22 ± 2°C during the day and 16 ± 2°C at night, with relative humidity between 35 and 65%. Natural daylight was supplemented with metal halide lamps providing 100 μmoles s−1 m−2. The treatments were disposed of in a randomized complete block design (RCBD), with every treatment accounting for three replicates. All the biological replicates consisted of pooled plants to increase the data robustness. The used UDs for the fertigation of lettuce plants were as follows: stabilized urine–low pH, stabilized urine–high pH, hydrolyzed urine-high pH, electrodialysis (ED) concentrate, aurin, K-struvite, urine precipitate–NaOH, and urine precipitate–calcium oxide (CaO) compared to a control 20–10-20 + trace elements (TE). All the details about the preparation and dilution of UDs for further integration into the fertigation solutions are described in detail in the El-Nakhel et al. (2021) study, as well as the electrical conductivity and composition of NSs, together with all the growing conditions in the greenhouse. In brief, stabilized urine–low pH was kept at pH 2 after HCl addition (urea as the main N compound). Stabilized urine–high pH was kept at pH > 11 after NaOH and CaO addition (urea). Hydrolyzed urine was prepared through spontaneous urea hydrolysis (ammonia and ammonium). ED concentrate was prepared in three steps: precipitation, nitrification, and electrodialysis (nitrate). Aurin a commercial fertilizer prepared through partial nitrification and distillation (Ammonium nitrate). K-struvite was prepared by removing all NH4-N, adding Mg2+, and increasing pH to 10 (Ammonium). Urine precipitate–NaOH was obtained by precipitation at pH 12.5 via NaOH addition (Ammonium). Urine precipitate–CaO was obtained by precipitation at pH 12.6 via CaO addition (Ammonium). All the solid UDs (K-struvite, urine precipitate–NaOH, and urine precipitate–CaO) were dissolved by adding 2 M H2SO4 prior to mixing with the other components, where phosphorus was the limiting dilution factor. As for the liquid fertilizers, the limiting dilution factor was nitrogen. Then, to ensure consistent NPK concentrations in the UD nutrient solutions, supplementary fertilizers were added to all the UDs to meet the nutritional requirements of lettuce and normalize nitrogen, potassium, and phosphorus across the different NSs. Specifically, for solid UDs, potassium nitrate and ammonium nitrate were employed for K-struvite and urine precipitate–CaO and potassium sulfate and ammonium nitrate for urine precipitate–NaOH. For the liquid UDs, monopotassium sulfate and potassium sulfate were added to fulfill the P and K requirements. The specific concentrations of the different elements in the UDs after dilution are detailed in Table S2 of El-Nakhel et al. (2021). pH of all NSs was adjusted to reach a value of 6.0. Rainwater was used for the dilution of the different NSs; the electrical conductivity of the NSs: control, stabilized urine–low pH, stabilized urine–high pH, hydrolyzed urine, ED concentrate, aurin, K-struvite, urine precipitate–NaOH, and urine precipitate–CaO was 1.2, 1.1, 1.0, 2.1, 2.0, 2.1, 1.7, 2.7, and 2.2 mS cm−1, respectively.
2.2 Sampling, leaf fresh weight, specific leaf weight, and leaf succulence determination
Thirty-two days after transplant, lettuce plants were harvested and assessed for their leaf fresh weight (expressed in g plant−1). The leaf area was subsequently measured via ImageJ software version 1.50 (Wayne Rasband, National Institute of Health, Bethesda, MD, United States) and expressed in cm2 plant−1. Then, the leaves were placed for 3 days at 70°C to determine leaf dry weight and leaf dry matter percentage. Specific leaf weight was calculated as dry weight divided by leaf area and expressed in mg cm−2. On the other hand, leaf succulence was calculated as leaf fresh weight minus leaf dry weight, converted to milligrams, and then divided by leaf area and expressed as mg of fresh weight cm−2. As for the analysis and sampling, the leaves of five plants replicate−1 were pooled and shock-frozen in liquid nitrogen. Subsequently, the plant material was ground to a fine powder in liquid nitrogen and either used immediately for assays or stored at −80°C.
2.3 Analyses of metabolites
As our aim was to understand whether UDs could alter the synthesis and accumulation of metabolites, it was pivotal to measure them quantitatively. Therefore, targeted metabolic profiling methods were applied, making it possible to quantify specific known metabolites within samples. Instead, untargeted metabolomics provides an overall metabolic profile (called metabolites fingerprinting) of a sample in relation to known and unknown compounds without being able to precisely determine their amount. Changes in amino acid levels are usually analyzed by pre-column derivatization with ortho-phthalaldehyde (OPA) or 9-fluorenylmethyl chloroformate (FMOC), followed by reversed-phase HPLC and fluorescence detection. OPA derivatization does not resolve proline, while FMOC can cause overlapping of isoleucine and leucine. Though accurate and cost-effective, these methods can be time-consuming (up to 90 min). Despite high sensitivity and resolution, GC–MS requires laborious derivatization and fails to determine arginine. CE-MS and LC–MS analyze non-volatile polar amino acids without derivatization. LC–MS/MS is rapid but has overlapping peaks and interference issues. 1H NMR offers high-throughput analysis but detects only high-concentration metabolites. The enzymatic assay of proline by ELISA spectrophotometric method in plates with 96 wells allows us to quantitatively determine proline in 90 samples, also performing a calibration curve for each plate in a few hours. Therefore, we decided to combine HPLC with OPA derivatization and the colorimetric assay for proline to allow the determination of amino acids. Primary amino acids and proline were extracted from 20 mg of powdered pooled lettuce material, mixed with 1 mL of ethanol:water (2:3 v/v), and incubated overnight at 4°C. After centrifugation at 14,000 g for 10 min at 4°C, the supernatant was used for the analyses performed according to Woodrow et al. (2017).
Carbohydrates are challenging to analyze due to their high polarity, similar structures, and lack of chromophores or fluorophores, making ultraviolet and fluorescence detectors ineffective. Refractive index (RI) and evaporative light scattering (ELS) detectors used with ion-exchange (IE) or size-exclusion chromatography (SEC) columns suffer from low sensitivity, specificity, and drift and are optimized for single sugar classes. High-performance liquid chromatography (HILIC) suits polar compounds but requires derivatization and high organic content mobile phases, causing solubility issues. High-performance anion-exchange chromatography with pulsed amperometric detection (HPAEC-PAD) offers better sensitivity and selectivity at high pH but lacks commercial standards and is incompatible with MS due to high salt concentration in the mobile phase. Therefore, to determine key carbohydrates such as glucose, fructose, and sucrose, as well as starch, we choose to utilize an enzymatic method, coupled with the reduction in pyridine nucleotides. This process was carried out in 96-well microplates, making it highly suitable for high-throughput analysis. Similarly, other accredited spectrophotometric methods were adapted to microplate assays using ELISA readers, enabling the use of small quantities of samples and reagents. This approach is efficient, rapid, and cost-effective, allowing for the simultaneous processing of multiple samples with minimal expense and effort. Starch, soluble sugars, and total proteins were quantified following the methodologies described by Woodrow et al. (2017) with the modification for soluble sugars proposed by Salbitani et al. (2022). According to Rouphael et al. (2021), anthocyanins were determined with slight modifications. Frozen lettuce (40 mg) was extracted two times with 200 μL of ethanol 40% (v/v). Clear supernatants (150 μL) were mixed with either KCl pH 1.0 or sodium acetate pH 4.5 (75 μL), and concentration of anthocyanins was determined by recording the absorbance at 520 and 700 nm and expressed as μg cyanidin-3-glucoside (C3G) equivalents g−1 FW. The content of total polyphenols was determined as described by Singleton et al. (1999) with the modifications described in Carillo et al. (2019b).
2.4 Oxidative markers and antioxidant activity
Hydrogen peroxide (H2O2) was determined according to Dell’Aversana et al. (2021). Malondialdehyde (MDA) was extracted and assayed according to Havaux et al. (2005). For the determination of 2,2′-azino-bis(3-ethylbenzothiazolin-6-sulfonic acid) (ABTS) activity, the method described by Pellegrini et al. (1999) was modified as follows. Aliquots of 50 mg of frozen fresh lettuce were suspended in 500 μL of pure ethanol and cooled in ice for 2 h. After centrifugation at 14,000 g for 10 min at 4°C, 100 μL of clear supernatants was mixed with 900 μL of reaction mixture containing ABTS 7 mM and K2S2O8 2.45 mM freshly prepared; 200 μL was then disposed into a polypropylene microplate, and absorbance at 745 nm was determined by a microplate reader. The blank consisted of the same final mixture with 100 μL of pure ethanol, instead of extract. ABTS activity was determined using a Trolox calibration standard curve and expressed as Trolox equivalents (μmol Trolox g−1 FW) (Carillo et al., 2022). Ascorbate peroxidase (APX; EC 1.11.1.11), catalase (CAT; EC 1.11.1.6), and glutathione reductase (GR; EC 1.8.1.7) were assayed following the method described by Hediye Sekmen et al. (2007).
2.5 Statistical analysis
Three biological replicates were used, each consisting of five pooled plants. The choice of using pooled plants depended on the need to have enough homogenous plant material for each condition to perform all the different analyses. The main effect of the urine derivatives or control was analyzed using a univariate analysis of variance (one-way ANOVA) using the SPSS 20 software package (IBM, Armonk, NY, USA). Duncan’s multiple range test (DMRT) was performed for mean comparisons on each of the significant (p < 0.05) variables measured. The Pearson correlation analysis was performed by SIGMAPLOT 14.5 software (Systat Software Inc., Richmond, CA). Dendrogram clustering was carried out using R.v3.4.3. The loading plots and score plot of all analyzed parameters were determined after principal component analysis (PCA) using Minitab®18 statistical software (Minitab LLC, State College, PA, United States).
3 Results
3.1 ED concentrate, K-struvite, and urine precipitate–CaO did not affect leaf fresh weight and leaf succulence
The integration of urine derivatives in the nutrient solutions had a major effect on the growth of lettuce as reflected by the leaf fresh weight per plant (Table 1). ED concentrate, K-struvite, and urine precipitate–CaO engendered the same leaf fresh weight, just like the control. Whereas hydrolyzed urine and stabilized urine–high pH generated the lowest leaf fresh weight (4.4-fold lower on average) that was not significantly different from aurin and stabilized urine–low pH UDs. Leaf weight was positively correlated to proteins (r = 0.84; p < 0.05), while negatively correlated to total AA (r = −0.79; p < 0.05), glutamine (r = −0.85; p < 0.005), BCAAs (r = −0.72; p < 0.05), and tyrosine (r = −0.82; p < 0.01). As for specific leaf weight (SLW), the highest values were registered for aurin- and urine precipitate–NaOH-treated plants (Table 1), but these values were only significantly higher than those registered for ED concentrate and stabilized urine (both pH). SLW was positively correlated to CAT activity (r = 0.78; p < 0.05), MEA (r = 0.77; p < 0.05), GABA (r = 0.68; p < 0.05), and alanine (r = 0.87; p < 0.005), while it was negatively correlated to H2O2 (r = −0.77; p < 0.05). As for leaf succulence, control plants showed similar values such as ED concentrate, urine precipitate–CaO, and K-struvite the most performing UDs together with stabilized urine–low pH. Leaf succulence was positively correlated to proteins (r = 0.75; p < 0.05) while negatively correlated to glutamate (r = −0.76; p < 0.05), BCAAs (r = −0.69; p < 0.05), and tyrosine (r = −0.82; p < 0.01).
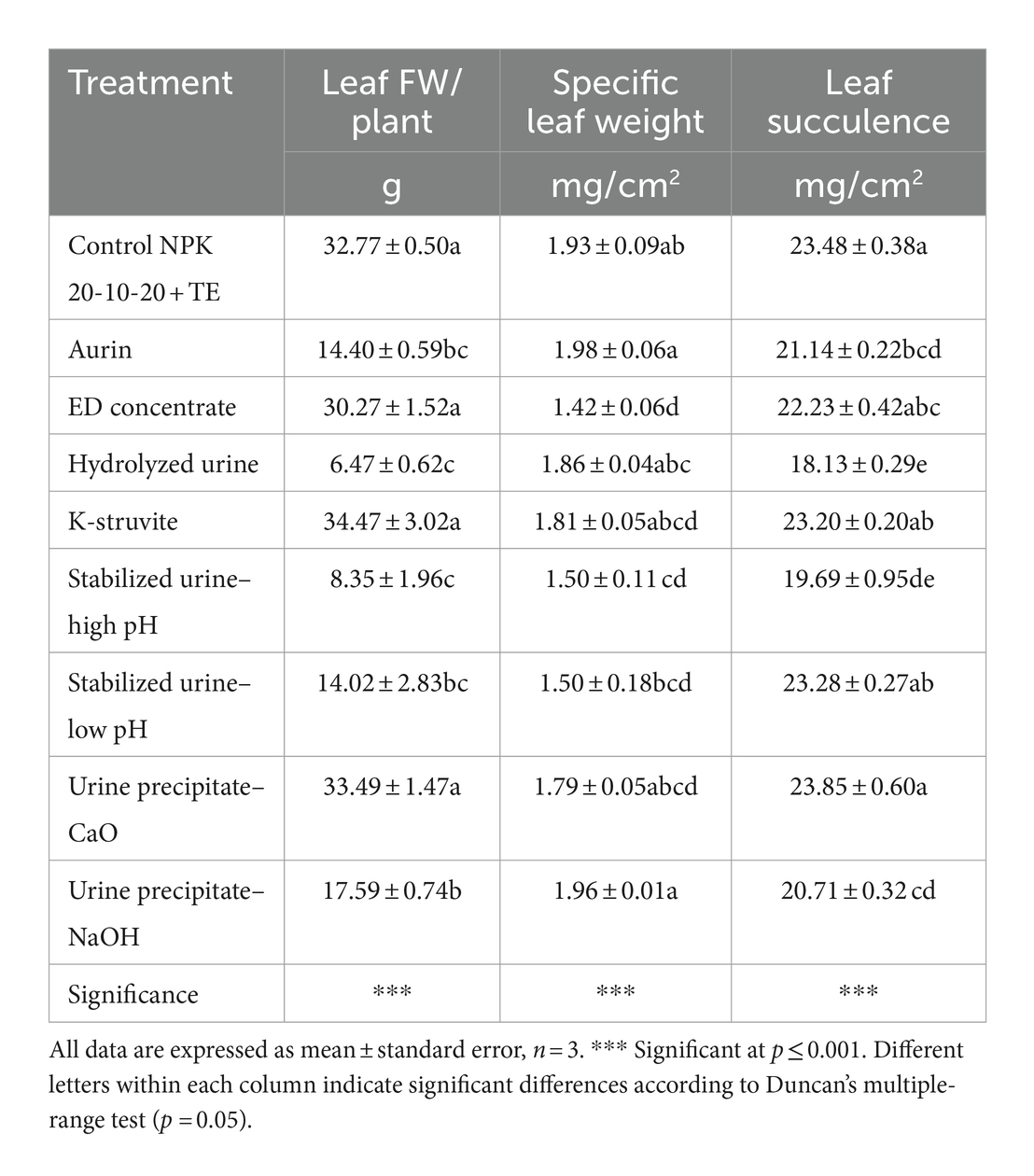
Table 1. Effects of treatments of urine derivatives on leaf fresh weight (g plant−1), specific leaf weight, and leaf succulence (mg/cm2) of lettuce plants.
3.2 Precipitates with CaO- and NaOH-induced high polyphenol and ABTS levels
As reported in Table 2, the two urine precipitates with CaO and NaOH determined the highest polyphenol content (0.408 and 0.369 μg gallic acid eq. g−1 FW, respectively), which decreased significantly in the lettuce fertilized with hydrolyzed urine (−51%) and aurin (−56%) compared to the best-performing UDs. Notwithstanding, urine precipitate–CaO significantly differed from the most of other UD treatments, except for urine precipitate–NaOH, which did not differ from NPK control treatment. In addition, none of the UD treatments induced statistically significant differences compared to the NPK control for polyphenols and anthocyanins, except for ED concentrate, which showed a significant increase in anthocyanin concentrations (+128%) compared to the NPK fertilizer. The content of anthocyanins was negatively correlated to GABA (r = −0.70; p < 0.05). ABTS levels increased significantly compared to the NPK control when treated with aurin and the two urine precipitates with CaO and NaOH (+94%, +172%, and + 130%, respectively). ABTS was negatively correlated to starch (r = −0.70; p < 0.05) and positively correlated to glutamate (r = 0.79; p < 0.01).
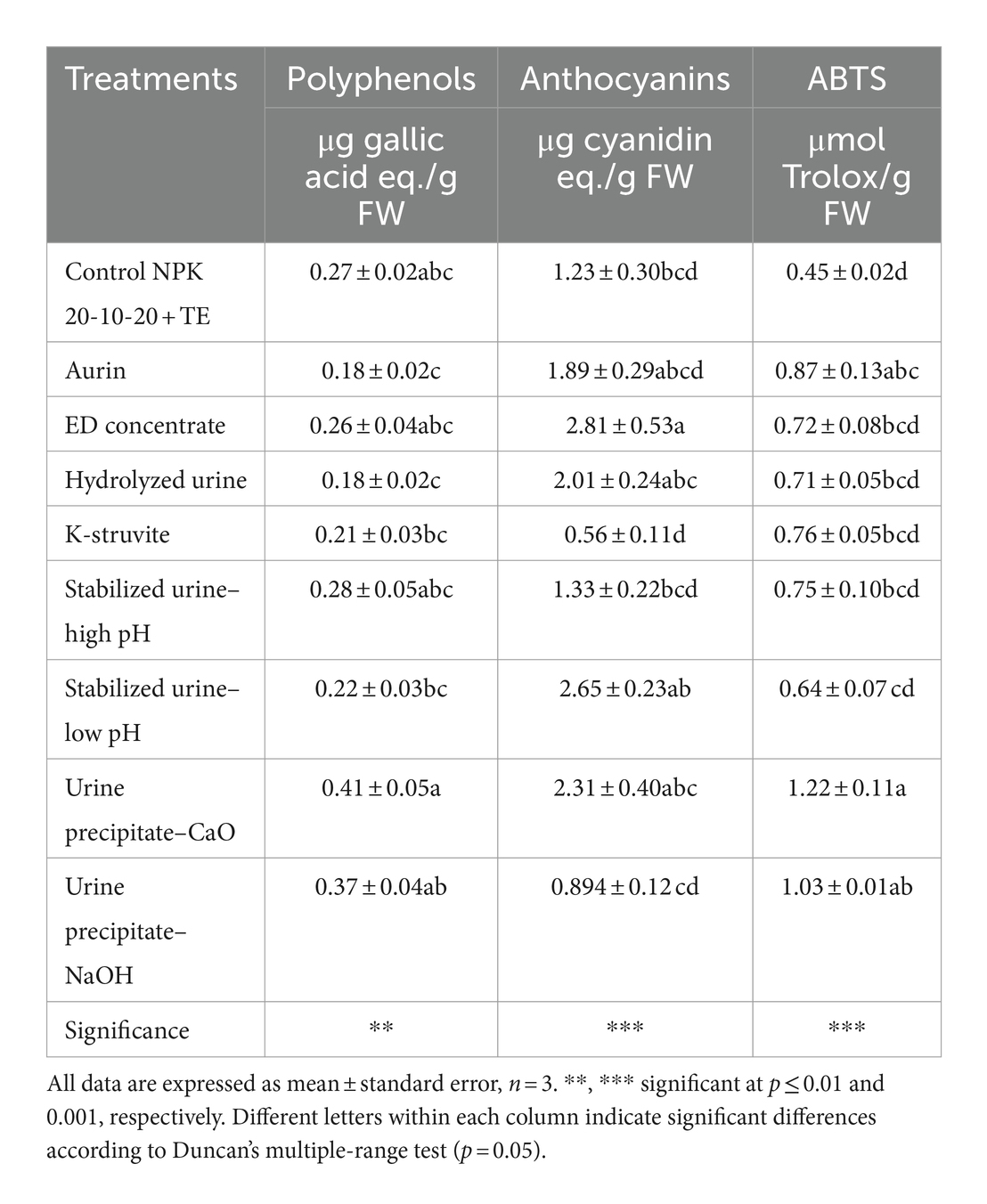
Table 2. Effects of treatments of urine derivatives on polyphenols (μg gallic acid eq./g FW), anthocyanins (μg cyanidin eq./g FW), and ABTS (μmol Trolox /g FW) content in lettuce plants.
3.3 Hydrolyzed urine and aurin increased essential amino acids but also correlated with higher levels of MDA and reduced APX activity
The starch content in lettuce grown with urine precipitate–CaO (60.34 μmol/g FW) was significantly lower than all other treatments (Table 3). The ED concentrate hydrolyzed urine and stabilized urine–low pH caused a significant decrease (on average, −50%) of glucose compared to the NPK control. ED concentrate and stabilized urine–low pH determined a decrease also in fructose content, although these values were not statistically different from those obtained using commercial NPK fertilizer. Sucrose content did not vary in the function of UD fertilizer. As shown in Table 4, the protein content was not influenced by the application of the different urine derivatives, while all amino acids were significantly affected by the UD treatments. ED concentrate, hydrolyzed urine, and stabilized urine–low pH induced a strong and significant increase or decrease in total amino acids contents (−79%, +65%, and +52%, respectively) compared to the NPK control. The hydrolyzed urine determined also significantly higher concentrations of essential amino acids (8.610 μmol/g FW) (the sum of histidine, isoleucine, leucine, lysine, methionine, phenylalanine, threonine, tryptophan, and valine) compared to NPK control, mostly due to the high levels of phenylalanine (data not shown). The essential amino acids value obtained in the lettuce treated with the hydrolyzed urine was like that of the plants fertilized with aurin (8.696 μmol/g FW), which picked up all other values. On the contrary, ED concentrate, and K-struvite showed essential amino acid values significantly lower than that of NPK fertilizer. GABA concentrations were negatively influenced using the different urine derivatives compared to the control, except for the urine precipitate–NaOH, the only one to not show a value statistically different from the NPK control. GABA was positively correlated to glucose (r = −0.88; p < 0.005), alanine (r = 0.67; p < 0.05), and MEA (r = −0.70; p < 0.05). In contrast, glycine levels increased significantly in almost all treatments compared to the control, except for aurin, ED concentrate, and K-struvite. Hydrolyzed urine and even more aurin led to a significant and strong 4.58- and 14.00-fold increase of proline compared to the control (1.040 μmol/g FW), respectively. According to Table 5, there were no statistically significant differences in the MDA content of plants fertilized with the different UDs compared to those treated with the NPK control. Aurin and hydrolyzed urine showed the highest MDA concentrations (0.589 and 0.536 nmol/mg, respectively), and these values were significantly different from those of K-struvite, which resulted in the lowest concentrations (0.171 nmol/mg) among all the derivatives. MDA was positively correlated to proline (r = 0.82; p < 0.01), BCAAs (r = 0.76; p < 0.05), and ornithine (r = 0.84; p < 0.001). Plants treated with commercial NPK fertilizer and urine precipitate–CaO were characterized by the lowest H2O2 accumulation (0.645 and 0.596 μmol/g FW, respectively) among all fertilizers. These values were significantly lower than those obtained from the use of stabilized urine–low pH (−84%) and ED concentrate (−79%). H2O2 content was negatively correlated to GABA (r = −0.75; p < 0.05), proteins (r = −0.70; p < 0.05), and MEA (r = −0.76; p < 0.05). The activity of the antioxidant enzyme GR decreased significantly in plants fertilized with hydrolyzed urine, ED concentrate, stabilized urine–high pH, and K-struvite compared to the control. The various derivatives did not induce statistically significant differences in CAT activity. Aurin, however, resulted in a significant increase in CAT activity compared to ED concentrate and stabilized urine–high pH (+158% and +122%, respectively). All UDs determined significant differences in APX activity, except for ED concentrate. In stabilized urine–high pH and urine precipitate–CaO treatments, the highest APX activities (1.204 and 1.480 μmol ASA ox/min/FW, respectively) were recorded, whereas hydrolyzed urine and aurin caused the lowest APX activities (0.111 and 0.137 μmol ASA ox/min/FW, respectively). CAT activity was positively correlated to MDA (r = 0.80; p < 0.01), alanine (r = 0.82; p < 0.01), BCAAs (r = 0.79; p < 0.05), essential AA (r = 0.71; p < 0.05), proline (r = 0.77; p < 0.05), ornithine (r = 0.79; p < 0.05), and serine (r = 0.89; p < 0.005). On the contrary, APX was negatively correlated to MDA (r = −0.75; p < 0.05), while positively correlated to polyphenols (r = 0.78; p < 0.05) and asparagine (r = 0.71; p < 0.05), whereas GR was positively correlated to serine (r = 0.68; p < 0.05), MEA (r = 0.84; p < 0.01), GABA (r = 0.71; p < 0.05), and alanine (r = 0.71; p < 0.05).
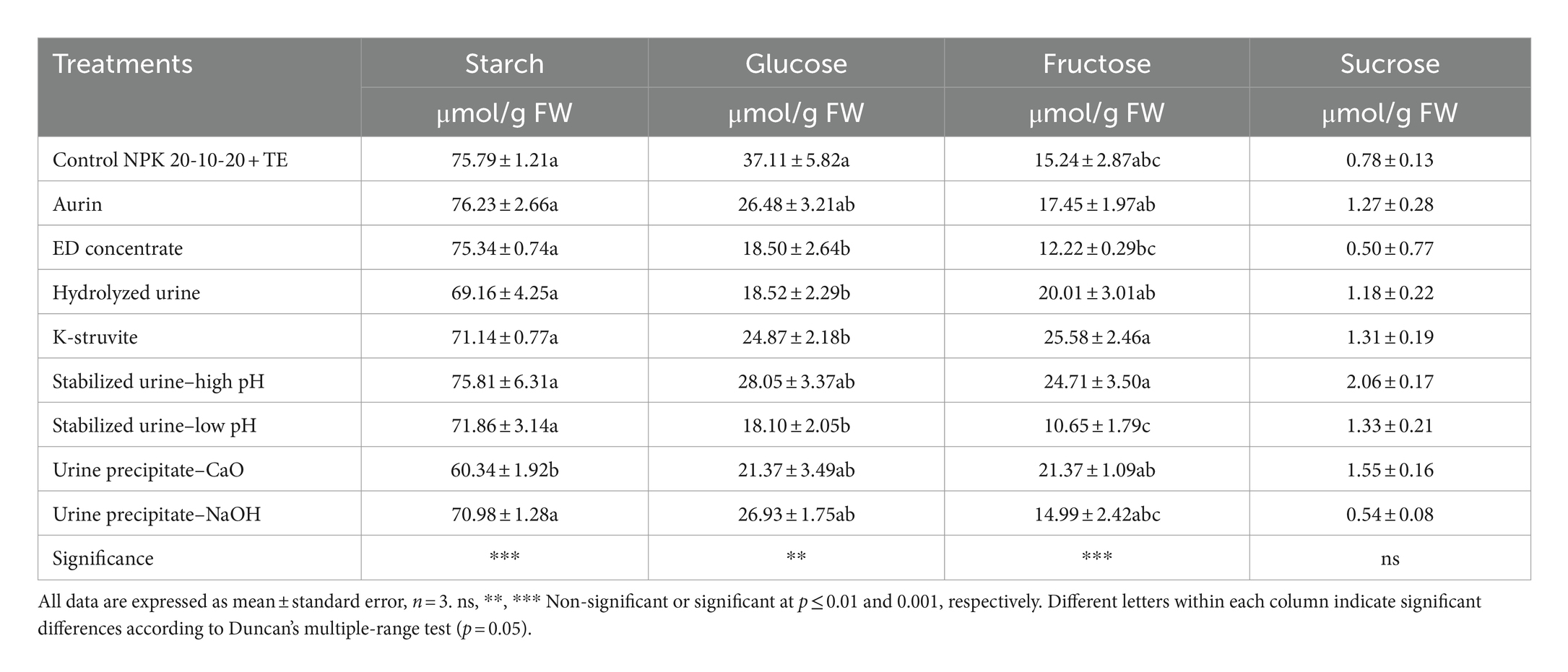
Table 3. Effects of urine derivatives treatments on starch and soluble sugars (glucose, fructose, and sucrose) (μmol/g FW) content in lettuce plants.
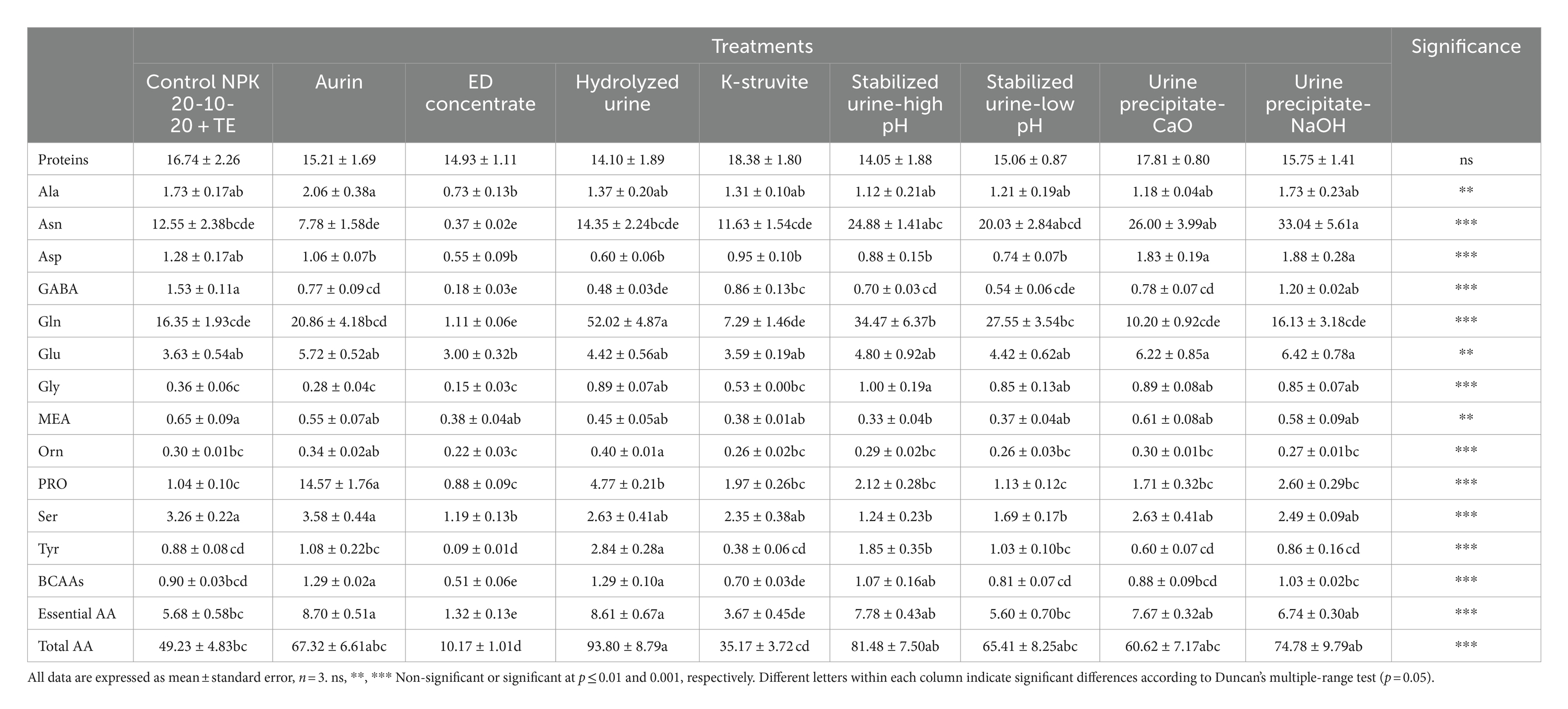
Table 4. Effects of urine derivatives treatments on content of soluble proteins (mg/g FW) and free amino acid profile (μmol/g FW) in lettuce plants.
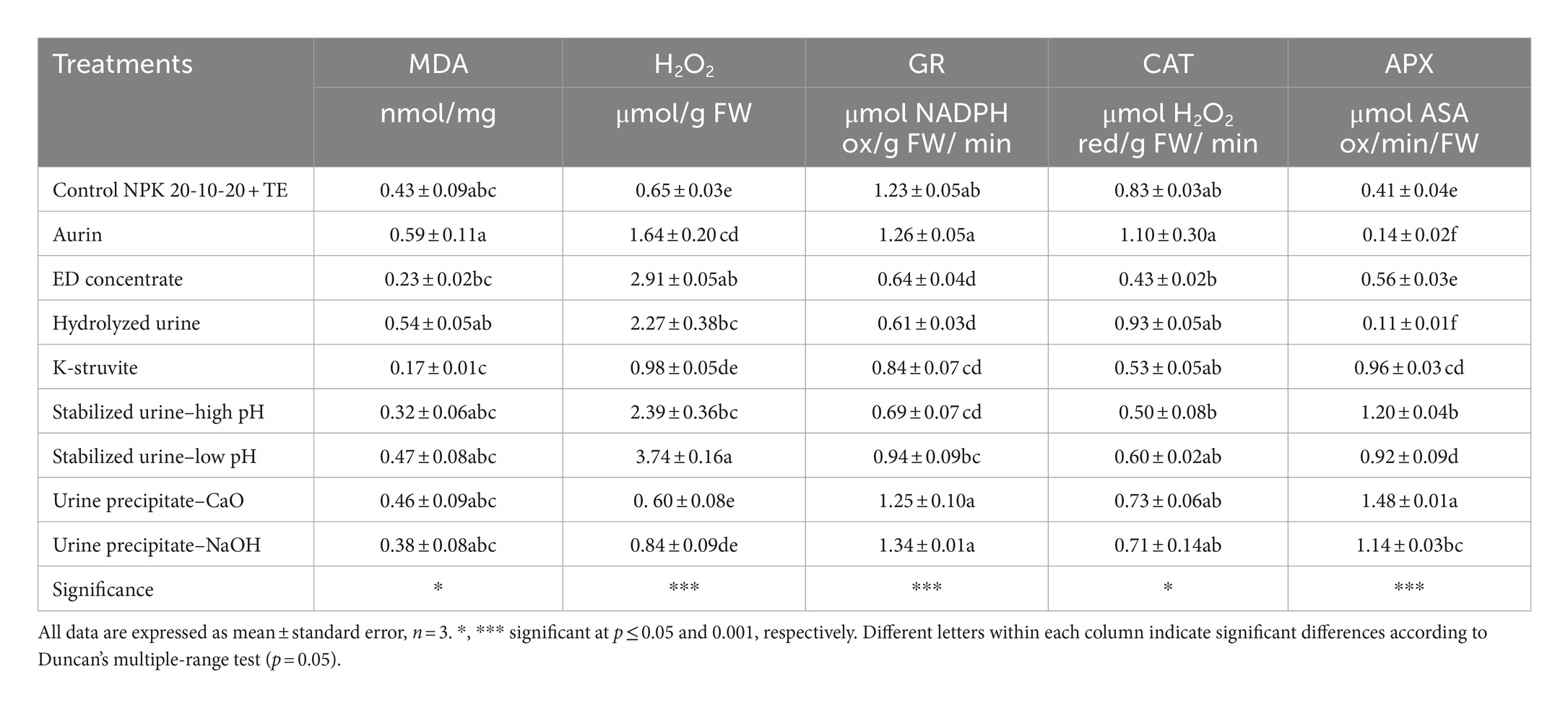
Table 5. Effects of urine derivatives treatments on malondialdehyde (MDA) (nmol/mg FW), hydrogen peroxide (H2O2) (μmol/g FW), glutathione reductase (GR) (μmol NADPH ox/g FW/min), catalase (CAT) (μmol H2O2 red/g FW/min), and ascorbate peroxidase (APX) (μmol ASA ox/min/FW) activity in lettuce plants.
3.4 PCA highlighted distinct biochemical and growth responses to various UDs
To have an overview of data, a principal component analysis was performed on all data about the different urine derivatives and the commercial fertilizer NPK, as reported in Supplementary Table 1. The first three principal components accounted for 75.5% of the total variance, with PC1, PC2, and PC3 corresponding to 32.1, 26.1, and 17.3%, respectively. PC1 was positively correlated to the content of total, essential, and branched-chain amino acids (BCAAs), CAT activity, ornithine, alanine, MDA, specific leaf weight, serine, glutamate, and proline. PC2, on the other hand, was positively correlated to hydrogen peroxide, glutamine and tyrosine, anthocyanins, starch, and sucrose, while it was negatively correlated to proteins, aspartate, leaf FW, GR activity, leaf succulence, MEA, polyphenols, GABA, APX activity, and glucose. PCA was effective in tracking the plant responses corresponding to treatments with the different urine derivatives. In particular, K-struvite and, even more, urine precipitate with CaO and NaOH showed responses comparable to those of the control NPK; in fact, they were located on the negative side of PC2 and correlated with higher biometric values (leaf succulence, fresh and specific fresh weight), proteins, polyphenols, GABA, MEA, aspartate and glucose accumulation, and higher activity of the antioxidant enzymes APX and GR. Stabilized urine at low and high pH was located in the upper left quadrant and characterized by a higher accumulation of starch, anthocyanins, and hydrogen peroxide. In the upper right quadrant, the hydrolyzed urine treatment was characterized by a higher accumulation of glutamine, and tyrosine, while aurin was clustered with total and essential amino acids, BCAAs, proline, MDA, and CAT activity (Figure 1).
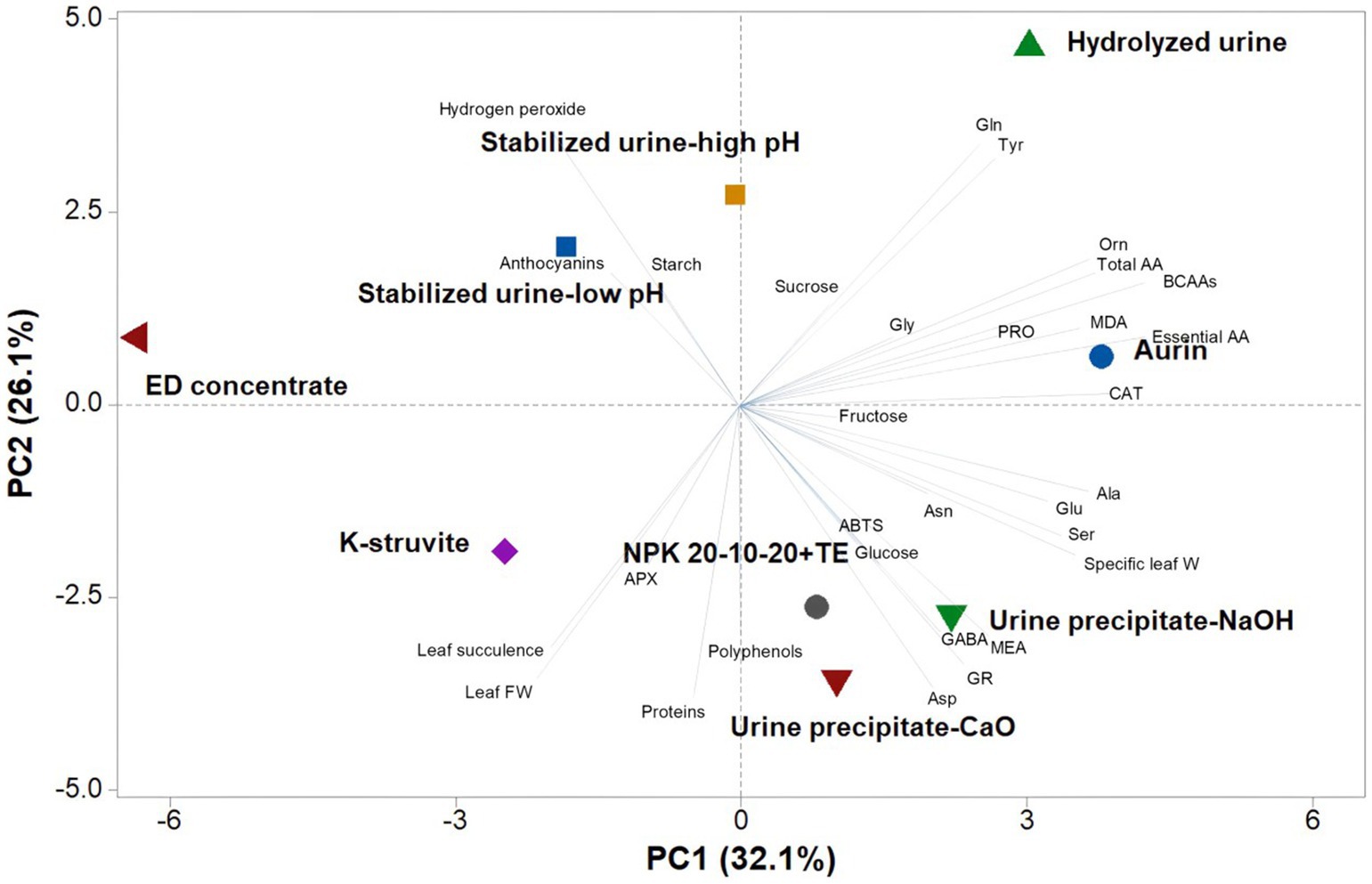
Figure 1. Principal component analysis (PCA) on the variation of the analyzed parameters in lettuce plants subjected to the treatments with urine derivatives and commercial fertilizer NPK 20-10-20 + TE.
4 Discussion
Urine derivatives have been successfully applied for lettuce cultivation in soilless systems (El-Nakhel et al., 2021). Here, we demonstrate how we investigated the metabolic benefits of the use of urine derivatives (UDs) as fertilizers, focusing on their impact on plant growth, nutrient uptake, and biochemical responses. We examined how these UDs influence key metabolic processes and compared their effectiveness with traditional NPK fertilizers. As already evidenced by El-Nakhel et al. (2021), lettuce fertilized with K-struvite, urine precipitate with CaO and NaOH, and ED concentrate showed comparable biometric values (leaf succulence and/or fresh leaf weight) as control NPK fertilizer plants, whereas those fertilized with stabilized urine at high and low pH, characterized by high concentrations of urea as the main N constituent, and hydrolyzed urine and aurin, having higher ammonium (NH4+) content (~45–50%) (Mauerer et al., 2023), highly affected plant growth and production. NH4+ can become toxic to plants if available as the sole N source, leading to growth inhibition (Britto and Kronzucker, 2002; Xiao et al., 2023). The toxicity of NH4+ has been attributed to futile secretion against high levels of ammonium outside, causing excessive energy consumption (futile NH4+ cycle) (Britto et al., 2001). The import of NH4+ itself does not need energy; however, when it is present at high concentration in the external medium, it enters the cytosol of root hair cells through ammonium transporters (AMTs), altering the membrane potential. Maintenance of the membrane potential requires proton pump activity at the expense of ATP, resulting in the acidification of the apoplasts and symplastic alkalinization (Ayling, 1993; Hao et al., 2020). High NH4+ concentrations may compete with/inhibit cations (K+, Mg2+, or Ca2+) uptake, perturbing plant ion balance (Britto and Kronzucker, 2002); the imbalance of ions can cause oxidative stress and interfere with photosynthesis, thus increasing Mehler reaction and photorespiration (Zhu et al., 2000). Accordingly, the imbalance between ammonium and nitrate present in aurin has been linked with a significant reduction of uptake and translocation of Ca2+ that increases the susceptibility of hydroponically grown tomato plants to blossom-end-rot (Halbert-Howard et al., 2021). Therefore, a high NH4+: NO3− ratio may alter calcium dynamics, rendering plants more prone to physiological disorders, hindering root development and nutrient absorption, and leading to stunted growth (Roosta and Schjoerring, 2007).
In plants treated with aurin and hydrolyzed urine compared to those fertilized with NPK control, the high content of ammonium may also cause toxicity altering intracellular pH, osmotic balance, nutrient uptake, and plant metabolism (Shilpha et al., 2023). In these conditions, amino acids such as amides (glutamine and asparagine) and proline strongly increase to re-assimilate ammonium, regulate osmotic balance, and prevent toxicity symptoms. However, the high consumption of carbon skeletons to synthesize these amino acids instead of promoting leaf expansion mechanisms resulted in lower leaf FW (Wang et al., 2022).
Urine precipitates CaO and NaOH, together with commercial fertilizer NPK, showed the highest content of polyphenols, which act as powerful antioxidants and activate plant defense networks against abiotic and biotic stresses (Carillo et al., 2019a). Polyphenols at high concentrations may also increase the post-harvest shelf-life representing an economic advantage for both producers and retailers (Patanè et al., 2021). Urine precipitates CaO and NaOH also exhibited the highest APX activity, which, in synergy with polyphenols, enhanced the plants’ ability to scavenge H₂O2 and protect plant cells and macromolecules against oxidative damage. However, these two treatments did not show the lowest MDA content. K-struvite, which promoted growth comparable to the control, exhibited the lowest MDA levels among treatments without significantly activating the antioxidant system, as APX activity remained similar to the control. Consequently, it appears clear that K-struvite did not elicit a stress response, aligning closely to the control in both growth and stress markers.
As mentioned above, proline showed a significant increase in aurin-treated plants, probably as a result of the stress caused by the high NH4+ concentrations in this derivative (Halbert-Howard et al., 2021). Instead, the highest levels of GABA were recorded in lettuce treated with the commercial fertilizer NPK, and under urine precipitate–NaOH treatment. GABA and proline play an important role in protecting cells under stress conditions as they can act as compatible osmolytes and ROS detoxifiers. Their degradation at the end of the stress state can also provide carbon, nitrogen, and energy to allow plants to recover and repair stress-induced damages (Carillo, 2018). GABA is also considered a bioactive and functional compound for human nutrition, so much to be approved by the FDA as a food ingredient or supplement (Food and Drug Administration, 2008). In fact, it has many beneficial effects, among which inducing hypotension, improving immunity, and preventing diabetes and cancer, in addition to controlling blood cholesterol levels. GABA is synthesized through decarboxylation of glutamate catalyzed by glutamate decarboxylase and since its synthesis is proton consuming, it also to prevent cytosol acidosis and stabilize pH (Fusco et al., 2023). Despite the strong increase in GABA, the treatment of the lettuce with the urine precipitate–NaOH did not decrease glutamate, which occurred in NPK control plants. In contrast, an increase in glutamate content occurred under urine precipitate–CaO treatment, which also led to an increase in aspartate, an important amino acid in plant metabolism that plays a role as a precursor of the essential amino acid isoleucine, lysine, methionine, and threonine (Galili, 2011). These amino acids proved to reduce oxidative stress-related damages when plants are under abiotic stress conditions (Woodrow et al., 2017) and had higher contents in lettuce plants fertilized with aurin and hydrolyzed urine. The use of these treatments also resulted in higher levels of branched-chain amino acids (BCAAs), which are used by plants as osmolytes to contrast the effects of water stress, antioxidants, and alternative electron donors for the mitochondrial electron transport chain (Woodrow et al., 2017). However, total amino acids decreased after fertilization with ED concentrate, probably because plants could use amides as compatible compounds for osmotic regulation, and not only as compounds for nitrogen storage and transport (Carillo et al., 2019a). An improvement in nitrogen metabolism (Miflin and Habash, 2002) compared to the control occurred in plants treated with hydrolyzed urine that showed high phenylalanine, ornithine, tyrosine, and glutamine content. The increase in phenylalanine, in particular, has been associated with enhanced plant defense mechanisms as it is the precursor of trans-cinnamic acid and, therefore, of a variety of important secondary metabolites, including polyphenols, flavonoids, and anthocyanins (Dixon and Paiva, 1995).
Alanine increased in lettuce plants treated with aurin. This amino acid is a precursor of CoA and is also involved in membrane phospholipid synthesis, fatty acid synthesis, and degradation and plays an active role in secondary metabolism and plant response to biotic and abiotic stresses (Wallace et al., 1984). The use of alanine, instead of glutamine and asparagine, as an N deposit is less expensive for plants when ATP is low (Diab and Limami, 2016), and in addition, its synthesis from the decarboxylation of malate to pyruvate, like that of GABA, consumes protons, preventing stress-induced cytoplasmic acidosis (Carillo, 2018).
An overproduction of radicals, which can cause oxidative damage to plant macromolecules and cellular structures, can be a cause of inhibition of plant development as found under aurin derivatives treatment and urine precipitate–NaOH (Hossain et al., 2015). In such cases, several antioxidant systems are involved in the scavenging of ROS (Hossain et al., 2015; Carillo, 2018), as could be seen from the increased activity of some antioxidant enzymes, in particular CAT in aurin and GR in urine precipitate–NaOH. Noctor et al. (2002) reported that CAT is involved in H2O2 scavenging only when this ROS is present at high cellular concentrations. It is relevant that CAT activity was positively correlated to MDA. This latter metabolite is the main end-product of polyunsaturated fatty acids (PUFAs) peroxidation by non-enzymatic and enzymatic processes upon oxidative stress via ROS and/or lipoxygenase (Ayala et al., 2014). Therefore, MDA content is used as a marker of lipid peroxidation and damage in plant membranes; however, this is only true when MDA levels remain consistently high, irreversibly damaging proteins and nucleic acids. In fact, the sudden and transient increase of MDA can interplay with ROS, amplifying the signal for the activation of stress regulatory genes (e.g., CAT) involved in plant defense and development, thus boosting the cellular defense systems, reducing ROS contents, and attenuating their damaging effects (Morales and Munné-Bosch, 2019). Accordingly, Tounekti et al. (2011) found that in rosemary plants under salinity, the transient increase of MDA in leaves was prodromic to the activation of an efficient ROS detoxification system that prevented oxidative damage. Therefore, the correct balance between MDA production, detoxification, and signaling is essential for redox control and plant tolerance or resistance to stress (Morales and Munné-Bosch, 2019). The increase in CAT activity occurred also in conjunction with the increase of ornithine, proline, essential AA, BCAAs, and serine. Ornithine is, like glutamate, a precursor of proline, that may be transaminated to P5C or Δ1-pyrroline-2-carboxylate, being both these intermediates reducible into proline (Roosens et al., 1998). Proline and catalase can exert an important function in stabilizing membranes and proteins, detoxifying ROS, and buffering redox potential in plant cells under oxidative stress (Carillo, 2018). Serine synthesis, through non-phosphorylated (glycerate) and phosphorylated (phosphohydroxypyruvate) pathways, and its subsequent metabolism may also play a key in biochemical adaptation to abiotic stresses by regulating intracellular redox status and pH (Igamberdiev and Kleczkowski, 2018), as previously seen also for alanine. GR that reduces the oxidized glutathione (GSSG) to reduced one (GSH), increased concomitantly to GABA, alanine, and serine, all playing a redox stabilizing and/or antioxidant role.
Our physiological study demonstrates that UDs such as K-struvite, urine precipitate with CaO and NaOH, and ED concentrate can be viable alternatives to conventional NPK fertilizers, offering comparable benefits in plant growth without affecting nutritional and nutraceutical attributes. Moreover, these UDs, particularly K-struvite, which behave like control, not inducing the antioxidant response, can reduce the nodes and node edges of root-associated bacterial community networks as NPK control fertilizer does (Van Gerrewey et al., 2021). This finding suggests that this UD supplies essential nutrients to plants and enhances their nutrient uptake and allocation efficiency. This reduces the need for plants to maintain extensive symbiotic relationships, thereby improving overall performance by directing more resources toward growth and productivity. Therefore, in general, the most of UDs show a composition that makes nutrients promptly available for plants, enhancing plant nutrient uptake efficiency while reducing nutrient runoff, thus mitigating water pollution and eutrophication in aquatic systems. Moreover, they may effectively be used for recycling nutrients and promoting a circular economy approach in agriculture. Therefore, using UDs can lower the carbon footprint of agricultural practices by recycling waste and reducing the need for energy-intensive production of synthetic fertilizers (Van Gerrewey et al., 2021). They can potentially promote sustainable agriculture over or with other emerging practices. In addition, Sutradhar et al. (2021) studied urine-enriched biochar-based fertilizers among smallholder farmers in rural Bangladesh, evidencing that it could significantly enhance garden productivity compared to traditional practices like cow manure. Partey et al. (2014) highlighted that combining biochar with green manure improved soil health and crop yields more effectively than using the amendment alone. Moussa et al. (2021) demonstrated in a trial in Niger that, either alone or combined with organic manure, sanitized urine could increase by approximately 30% yield pearl millet panicle yield.
5 Conclusion
Harnessing the power of urine derivatives in fertigation schemes for soilless lettuce cultivation triggers diverse morphological and metabolic responses due to their unique composition. Among these derivatives, those abundant in NH4+, such as aurin and hydrolyzed urine, triggered the most intriguing responses. Although they led to a reduction in leaf fresh weight due to NH4+ toxicity, they stimulated the accumulation of key amino acids such as amides (glutamine and asparagine) and proline, which play a key role in stabilizing membrane potential and protecting proteins from denaturation and oxidation processes. Other derivatives such as ED concentrate, urine precipitate–CaO, and in particular K-struvite, emerged as promising candidates for recovering nutrients from human urine. Urine precipitate–CaO not only enhanced plant growth, but it improved qualitative aspects of lettuce enhancing the content of polyphenols, anthocyanins, APX, and ABTS antioxidant activity, while also increasing glutamate and aspartate, proline, and essential amino acids precursors. This study underscores the potential of human urine as a rich source of nutrients, offering a sustainable solution for lettuce cultivation in soilless systems and agriculture in general. The integration of urine-derived fertilizers into agricultural practices not only supports circular economy principles but also has the potential to reduce dependency on synthetic fertilizers, thereby decreasing the environmental footprint of farming. Policymakers should consider promoting research and development in this area to facilitate the adoption of such sustainable practices on a larger scale. Adopting UDs can contribute to global food security by making farming practices more resilient and resource-efficient, ultimately supporting the growth of a more sustainable agricultural system. Future research should aim to optimize the formulation and application rates of UDs to prevent potential negative effects, such as NH4+ toxicity. Additionally, potential contamination with pharmaceuticals and pathogens should be addressed in future research to ensure the safety and efficacy of urine-derived fertilizers, and it is important to consider the variability in human urine composition due to factors such as diet, health status, and hydration levels. Investigations could examine the blending of various UDs to achieve a better nutrient balance and minimize toxicity risks. Furthermore, long-term field trials and multi-crop studies are essential to confirm the advantages of UDs in diverse agricultural environments and climatic conditions. Continuing research, including controlled environment agriculture studies and pilot programs incorporating UDs into commercial soilless systems, will help expand current findings and offer more detailed data on their effectiveness and safety.
Data availability statement
The raw data supporting the conclusions of this article will be made available by the authors, without undue reservation.
Author contributions
RN: Data curation, Investigation, Writing – original draft, Writing – review & editing. CE-N: Data curation, Writing – original draft, Writing – review & editing. DG: Conceptualization, Writing – review & editing. GMF: Data curation, Investigation, Methodology, Validation, Writing – original draft. SP: Writing – review & editing. YR: Writing – review & editing. PC: Methodology, Data curation, Funding acquisition, Supervision, Writing – review & editing.
Funding
The author(s) declare that financial support was received for the research, authorship, and/or publication of this article. This study was supported by the projects BBHORT (PRIN 2022 PNRR—grant P2022P52XK) and GREENHORT (PRIN 2022—grant 2022WHTNZT), funded by the Italian Ministry of University and Research.
Acknowledgments
The authors would like to thank Antonio Pannico for his scientific support during the greenhouse experiment, and Michele Ciriello and Luigi Formisano for statistics and table preparation. All authors have approved the final version of the manuscript.
Conflict of interest
The authors declare that the research was conducted in the absence of any commercial or financial relationships that could be construed as a potential conflict of interest.
Publisher’s note
All claims expressed in this article are solely those of the authors and do not necessarily represent those of their affiliated organizations, or those of the publisher, the editors and the reviewers. Any product that may be evaluated in this article, or claim that may be made by its manufacturer, is not guaranteed or endorsed by the publisher.
Supplementary material
The Supplementary material for this article can be found online at: https://www.frontiersin.org/articles/10.3389/fsufs.2024.1440014/full#supplementary-material
Footnotes
References
Aryal, J. P., Sapkota, T. B., Krupnik, T. J., Rahut, D. B., Jat, M. L., and Stirling, C. M. (2021). Factors affecting farmers’ use of organic and inorganic fertilizers in South Asia. Environ. Sci. Pollut. Res. Int. 28, 51480–51496. doi: 10.1007/s11356-021-13975-7
Ashley, K., Cordell, D., and Mavinic, D. (2011). A brief history of phosphorus: From the philosopher’s stone to nutrient recovery and reuse. Chemosphere 84, 737–746. doi: 10.1016/j.chemosphere.2011.03.001
Ayala, A., Muñoz, M. F., and Argüelles, S. (2014). Lipid peroxidation: production, metabolism, and signaling mechanisms of malondialdehyde and 4-hydroxy-2-nonenal. Oxidative Med. Cell. Longev. 2014:360438. doi: 10.1155/2014/360438
Ayling, S. M. (1993). The effect of ammonium ions on membrane potential and anion flux in roots of barley and tomato. Plant Cell Environ. 16, 297–303. doi: 10.1111/j.1365-3040.1993.tb00872.x
Beal, C., Gardner, T., Ahmed, W., Walton, C., and Hamlyn-Harris, D. (2008). Urine-separation and reuse trial. Water E-Journal 35, 66–69.
Britto, D. T., and Kronzucker, H. J. (2002). NH4+ toxicity in higher plants: a critical review. J. Plant Physiol. 159, 567–584. doi: 10.1078/0176-1617-0774
Britto, D. T., Siddiqi, M. Y., Glass, A. D., and Kronzucker, H. J. (2001). Futile transmembrane NH4(+) cycling: a cellular hypothesis to explain ammonium toxicity in plants. Proc. Natl. Acad. Sci. U S A. 98, 4255–4258. doi: 10.1073/pnas.061034698
Carillo, P. (2018). GABA shunt in durum wheat. Front. Plant Sci. 9:100. doi: 10.3389/fpls.2018.00100
Carillo, P., Colla, G., el-Nakhel, C., Bonini, P., D’Amelia, L., Dell’Aversana, E., et al. (2019a). Biostimulant application with a tropical plant extract enhances Corchorus olitorius adaptation to sub-optimal nutrient regimens by improving physiological parameters. Agronomy 9:249. doi: 10.3390/agronomy9050249
Carillo, P., Colla, G., Fusco, G. M., Dell’Aversana, E., El-Nakhel, C., Giordano, M., et al. (2019b). Morphological and physiological responses induced by protein hydrolysate-based biostimulant and nitrogen rates in greenhouse spinach. Agronomy 9:450. doi: 10.3390/agronomy9080450
Carillo, P., De Micco, V., Ciriello, M., Formisano, L., El-Nakhel, C, Giordano, M., et al. (2022). Morpho-anatomical, physiological, and mineral composition responses induced by a vegetal-based biostimulant at three rates of foliar application in greenhouse lettuce. Plants (Basel) 11:2030. doi: 10.3390/plants11152030
Ciceri, D., Manning, D. A. C., and Allanore, A. (2015). Historical and technical developments of potassium resources. Sci. Total Environ. 502, 590–601. doi: 10.1016/j.scitotenv.2014.09.013
De Laurentiis, V., Caldeira, C., and Sala, S. (2020). No time to waste: assessing the performance of food waste prevention actions. Resour. Conserv. Recycl. 161:104946. doi: 10.1016/j.resconrec.2020.104946
De Paepe, J., Lindeboom, R. E. F., Vanoppen, M., De Paepe, K., Demey, D., Coessens, W., et al. (2018). Refinery and concentration of nutrients from urine with electrodialysis enabled by upstream precipitation and nitrification. Water Res. 144, 76–86. doi: 10.1016/j.watres.2018.07.016
Dell’Aversana, E., Hessini, K., Ferchichi, S., Fusco, G. M., Woodrow, P., Ciarmiello, L. F., et al. (2021). Salinity duration differently modulates physiological parameters and metabolites profile in roots of two contrasting barley genotypes. Plants (Basel) 10:307. doi: 10.3390/plants10020307
Diab, H., and Limami, A. M. (2016). Reconfiguration of N metabolism upon hypoxia stress and recovery: roles of alanine aminotransferase (AlaAT) and glutamate dehydrogenase (GDH). Plan. Theory 5:25. doi: 10.3390/plants5020025
Dixon, R. A., and Paiva, N. L. (1995). Stress-induced phenylpropanoid metabolism. Plant Cell 7, 1085–1097. doi: 10.1105/tpc.7.7.1085
El-Nakhel, C., Geelen, D., De Paepe, J., Clauwaert, P., De Pascale, S., and Rouphael, Y. (2021). An Appraisal of urine derivatives integrated in the nitrogen and phosphorus inputs of a lettuce soilless cultivation system. Sustain. For. 13:4218. doi: 10.3390/su13084218
Ernsting, A., Smolker, R., and Paul, H. (2011). Biochar and carbon markets. Biofuels 2, 9–12. doi: 10.4155/bfs.10.78
FAO (2019). The State of Food and Agriculture—Moving forward on Food Loss and Waste Reduction. Rome: Food and Agriculture Organization of the United Nations. Available at: http://www.fao.org/3/ca6030en/ca6030en.pdf (Accessed June 17, 2023).
Food and Drug Administration (2008). Gamma-aminobutyric Acid GRAS Notice. Available at: https://www.fda.gov/files/food/published/GRAS-Notice-000595--Gamma-Aminobutyric-Acid-%28GABA%29.pdf (Accessed January 26, 2024).
Friedler, E., Butler, D., and Alfiya, Y. (2013). “Wastewater composition” in Source separation and decentralization for wastewater. eds. T. A. Larsen, K. M. Udert, and J. Lienert (London, UK: IWA Publishing), 241–257.
Fusco, G. M., Burato, A., Pentangelo, A., Cardarelli, M., Nicastro, R., Carillo, P., et al. (2023). Can microbial consortium applications affect yield and quality of conventionally managed processing tomato? Plan. Theory 12:14. doi: 10.3390/plants12010014
Fusco, G. M., Nicastro, R., Rouphael, Y., and Carillo, P. (2022). The effects of the microbial biostimulants approved by EU Regulation 2019/1009 on yield and quality of vegetable crops. Food Secur. 11:2656. doi: 10.3390/foods11172656
Galili, G. (2011). The aspartate-family pathway of plants. Plant Signal. Behav. 6, 192–195. doi: 10.4161/psb.6.2.14425
Halbert-Howard, A., Häfner, F., Karlowsky, S., Schwarz, D., and Krause, A. (2021). Evaluating recycling fertilizers for tomato cultivation in hydroponics, and their impact on greenhouse gas emissions. Environ. Sci. Pollut. Res. 28, 59284–59303. doi: 10.1007/s11356-020-10461-4
Hao, D.-L., Zhou, J.-Y., Yang, S.-Y., Qi, W., Yang, K.-J., and Su, Y.-H. (2020). Function and regulation of ammonium transporters in plants. Int. J. Mol. Sci. 21:3557. doi: 10.3390/ijms21103557
Havaux, M., Eymery, F., Porfirova, S., Rey, P., and Dörmann, P. (2005). Vitamin E protects against photoinhibition and photooxidative stress in Arabidopsis thaliana. Plant Cell 17, 3451–3469. doi: 10.1105/tpc.105.037036
Hediye Sekmen, A., Türkan, İ., and Takio, S. (2007). Differential responses of antioxidative enzymes and lipid peroxidation to salt stress in salt-tolerant Plantago maritima and salt-sensitive Plantago media. Physiol. Plant. 131, 399–411. doi: 10.1111/j.1399-3054.2007.00970.x
Hoque, M. M., Ajwa, H., Othman, M., Smith, R., and Cahn, M. (2010). Yield and postharvest quality of lettuce in response to nitrogen, phosphorus, and potassium fertilizers. HortScience Horts 45, 1539–1544. doi: 10.21273/HORTSCI.45.10.1539
Hossain, M. A., Bhattacharjee, S., Armin, S.-M., Qian, P., Xin, W., Li, H.-Y., et al. (2015). Hydrogen peroxide priming modulates abiotic oxidative stress tolerance: insights from ROS detoxification and scavenging. Front. Plant Sci. 6:420. doi: 10.3389/fpls.2015.00420
Igamberdiev, A. U., and Kleczkowski, L. A. (2018). The glycerate and phosphorylated pathways of serine synthesis in plants: the branches of plant glycolysis linking carbon and nitrogen metabolism. Front. Plant Sci. 9:318. doi: 10.3389/fpls.2018.00318
Jurgilevich, A., Birge, T., Kentala-Lehtonen, J., Korhonen-Kurki, K., Pietikäinen, J., Saikku, L., et al. (2016). Transition towards circular economy in the food system. Sustain. For. 8:69. doi: 10.3390/su8010069
Karak, T., and Bhattacharyya, P. (2011). Human urine as a source of alternative natural fertilizer in agriculture: A flight of fancy or an achievable reality. Resour. Conserv. Recycl. 55, 400–408. doi: 10.1016/j.resconrec.2010.12.008
Kim, R. H., Tagele, S. B., Jeong, M., Jung, D. R., Lee, D., Park, T., et al. (2023). Spinach (Spinacia oleracea) as green manure modifies the soil nutrients and microbiota structure for enhanced pepper productivity. Sci. Rep. 13:4140. doi: 10.1038/s41598-023-31204-8
Kundu, S., Pramanik, B. K., Halder, P., Patel, S., Ramezani, M., Khairul, M. A., et al. (2022). Source and central level recovery of nutrients from urine and wastewater: A state-of-art on nutrients mapping and potential technological solutions. J. Environ. Chem. Eng. 10:107146. doi: 10.1016/j.jece.2022.107146
Lassaletta, L., Billen, G., Grizzetti, B., Anglade, J., and Garnier, J. (2014). 50 year trends in nitrogen use efficiency of world cropping systems: the relationship between yield and nitrogen input to cropland. Environ. Res. Lett. 9:105011. doi: 10.1088/1748-9326/9/10/105011
Liu, C. W., Sung, Y., Chen, B. C., and Lai, H. Y. (2014). Effects of nitrogen fertilizers on the growth and nitrate content of lettuce (Lactuca sativa L.). Int. J. Environ. Res. Public Health 11, 4427–4440. doi: 10.3390/ijerph110404427
Loh, Y. H., Jakszyn, P., Luben, R. N., Mulligan, A. A., Mitrou, P. N., and Khaw, K.-T. (2011). N-nitroso compounds and cancer incidence: the European Prospective Investigation into Cancer and Nutrition (EPIC)–Norfolk Study. Am. J. Clin. Nutr. 93, 1053–1061. doi: 10.3945/ajcn.111.012377
Martin, T. M. P., Esculier, F., Levavasseur, F., and Houot, S. (2022). Human urine-based fertilizers: A review. Crit. Rev. Environ. Sci. Technol. 52, 890–936. doi: 10.1080/10643389.2020.1838214
Matassa, S., Batstone, D. J., Hülsen, T., Schnoor, J., and Verstraete, W. (2015). Can direct conversion of used nitrogen to new feed and protein help feed the world? Environ. Sci. Technol. 49, 5247–5254. doi: 10.1021/es505432w
Mauerer, M., Rocksch, T., Dannehl, D., Schuch, I., Mewis, I., Förster, N., et al. (2023). Replacing mineral fertilizer with nitrified human urine in hydroponic lettuce (Lactuca sativa L.) production. Sustain. For. 15:10684. doi: 10.3390/su151310684
Miflin, B. J., and Habash, D. Z. (2002). The role of glutamine synthetase and glutamate dehydrogenase in nitrogen assimilation and possibilities for improvement in the nitrogen utilization of crops. J. Exp. Bot. 53, 979–987. doi: 10.1093/jexbot/53.370.979
Morales, M., and Munné-Bosch, S. (2019). Malondialdehyde: facts and artifacts. Plant Physiol. 180, 1246–1250. doi: 10.1104/pp.19.00405
Moussa, H. O., Nwankwo, C. I., Aminou, A. M., Stern, D. A., Haussmann, B. I. G., and Herrmann, L. (2021). Sanitized human urine (OGA) as a fertilizer auto-innovation from women farmers in Niger. Agron. Sustain. Dev. 41:56. doi: 10.1007/s13593-021-00675-2
Nakao, S., Nishio, T., and Kanjo, Y. (2017). Simultaneous recovery of phosphorus and potassium as magnesium potassium phosphate from synthetic sewage sludge effluent. Environ. Technol. 38, 2416–2426. doi: 10.1080/09593330.2016.1264485
Noctor, G., Veljovic-Jovanovic, S., Driscoll, S., Novitskaya, L., and Foyer, C. H. (2002). Drought and oxidative load in the leaves of C3 plants: a predominant role for photorespiration? Ann. Bot. 89, 841–850. doi: 10.1093/aob/mcf096
Partey, S. T., Preziosi, R. F., and Robson, G. D. (2014). Short-term interactive effects of biochar, green manure, and inorganic fertilizer on soil properties and agronomic characteristics of maize. Agric. Res. 3, 128–136. doi: 10.1007/s40003-014-0102-1
Patanè, C., Siah, S., Pellegrino, A., Cosentino, S. L., and Siracusa, L. (2021). Fruit yield, polyphenols, and carotenoids in long shelf-life tomatoes in response to drought stress and rewatering. Agronomy 11:1943. doi: 10.3390/agronomy11101943
Pellegrini, N., Re, R., Yang, M., and Rice-Evans, C. (1999). Screening of dietary carotenoids and carotenoid-rich fruit extracts for antioxidant activities applying 2,2 0 -azinobis(3-ethylenebenzothiazoline-6-sulfonic acid radical cation decolorization assay). Methods Enzymol. 299, 379–384. doi: 10.1016/S0076-6879(99)99037-7
Pretty, J., and Bharucha, Z. P. (2014). Sustainable intensification in agricultural systems. Ann. Bot. 114, 1571–1596. doi: 10.1093/aob/mcu205
Roosens, N. H. C. J., Thu, T. T., Iskandar, H. M., and Jacobs, M. (1998). Isolation of the ornithine-δ-aminotransferase cDNA and effect of salt stress on its expression in Arabidopsis thaliana. Plant Physiol. 117, 263–271. doi: 10.1104/pp.117.1.263
Roosta, H. R., and Schjoerring, J. K. (2007). Effects of ammonium toxicity on nitrogen metabolism and elemental profile of cucumber plants. J. Plant Nutr. 30, 1933–1951. doi: 10.1080/01904160701629211
Rouphael, Y., Corrado, G., Colla, G., de Pascale, S., Dell’Aversana, E., D’Amelia, L. I., et al. (2021). Biostimulation as a means for optimizing fruit phytochemical content and functional quality of tomato landraces of the San Marzano area. Food Secur. 10:926. doi: 10.3390/foods10050926
Salbitani, G., Carillo, P., Di Martino, C., Bolinesi, F., Mangoni, O., Loreto, F., et al. (2022). Microalgae cross-fertilization: short-term effects of Galdieria phlegrea extract on growth, photosynthesis and enzyme activity of Chlorella sorokiniana cells. J. Appl. Phycol. 34, 1957–1966. doi: 10.1007/s10811-022-02769-0
Sarigul, N., Korkmaz, F., and Kurultak, İ. (2019). A new artificial urine protocol to better imitate human urine. Sci. Rep. 9:20159. doi: 10.1038/s41598-019-56693-4
Scotti, R., Bonanomi, G., Scelza, R., Zoina, A., and Rao, M. A. (2015). Organic amendments as sustainable tool to recovery fertility in intensive agricultural systems. J. Soil Sci. Plant Nutr. 15, 333–352. doi: 10.4067/S0718-95162015005000031
Shilpha, J., Song, J., and Jeong, B. R. (2023). Ammonium phytotoxicity and tolerance: an insight into ammonium nutrition to improve crop productivity. Agronomy 13:1487. doi: 10.3390/agronomy13061487
Singleton, V. L., Orthofer, R., and Lamuela-Raventós, R. M. (1999). [14] Analysis of total phenols and other oxidation substrates and antioxidants by means of folin-ciocalteu reagent. Methods Enzymol. 299, 152–178. doi: 10.1016/S0076-6879(99)99017-1
Sutradhar, I., Jackson-deGraffenried, M., Akter, S., McMahon, S. A., Waid, J. L., Schmidt, H. P., et al. (2021). Introducing urine-enriched biochar-based fertilizer for vegetable production: acceptability and results from rural Bangladesh. Environ. Dev. Sustain. 23, 12954–12975. doi: 10.1007/s10668-020-01194-y
Tamme, T., Reinik, M., and Roasto, M. (2010). “Chapter 21 - Nitrates and Nitrites in Vegetables: Occurrence and Health Risks” in Bioactive Foods in Promoting Health. eds. R. R. Watson and V. R. Preedy (San Diego: Academic Press), 307–321.
Tounekti, T., Vadel, A. M., Oñate, M., Khemira, H., and Munné-Bosch, S. (2011). Salt-induced oxidative stress in rosemary plants: Damage or protection? Environ. Exp. Bot. 71, 298–305. doi: 10.1016/j.envexpbot.2010.12.016
Trimmer, J. T., Margenot, A. J., Cusick, R. D., and Guest, J. S. (2019). Aligning product chemistry and soil context for agronomic reuse of human-derived resources. Environ. Sci. Technol. 53, 6501–6510. doi: 10.1021/acs.est.9b00504
Udert, K. M., Larsen, T. A., and Gujer, W. (2006). Fate of major compounds in source-separated urine. Water Sci. Technol. 54, 413–420. doi: 10.2166/wst.2006.921
UNEP (2023). UN environment program. Four reasons why the world needs to limit nitrogen pollution. Available at: https://www.unep.org/news-and-stories/story/four-reasons-why-world-needs-limit-nitrogen-pollution (Accessed June 17, 2023).
Van Gerrewey, T., El-Nakhel, C., De Pascale, S., De Paepe, J., Clauwaert, P., Kerckhof, F.-M., et al. (2021). Root-associated bacterial community shifts in hydroponic lettuce cultured with urine-derived fertilizer. Microorganisms 9:1326. doi: 10.3390/microorganisms9061326
van Velzen, A. G., Sips, A. J. A. M., Schothorst, R. C., Lambers, A. C., and Meulenbelt, J. (2008). The oral bioavailability of nitrate from nitrate-rich vegetables in humans. Toxicol. Lett. 181, 177–181. doi: 10.1016/j.toxlet.2008.07.019
Wald, C. (2022). The urine revolution: how recycling pee could help to save the world. Nature 602, 202–206. doi: 10.1038/d41586-022-00338-6
Wallace, W., Secor, J., and Schrader, L. E. (1984). Rapid accumulation of γ-aminobutyric acid and alanine in soybean leaves in response to an abrupt transfer to lower temperature, darkness, or mechanical manipulation 1. Plant Physiol. 75, 170–175. doi: 10.1104/pp.75.1.170
Wang, F., Wang, Q., Yu, Q., Ye, J., Gao, J., Liu, H., et al. (2022). Is the NH4+-induced growth inhibition caused by the NH4+ form of the nitrogen source or by soil acidification? Front. Plant Sci. 13:968707. doi: 10.3389/fpls.2022.968707
Woodrow, P., Ciarmiello, L. F., Annunziata, M. G., Pacifico, S., Iannuzzi, F., Mirto, A., et al. (2017). Durum wheat seedling responses to simultaneous high light and salinity involve a fine reconfiguration of amino acids and carbohydrate metabolism. Physiol. Plant. 159, 290–312. doi: 10.1111/ppl.12513
Xiao, C., Fang, Y., Wang, S., and He, K. (2023). The alleviation of ammonium toxicity in plants. J. Integr. Plant Biol. 65, 1362–1368. doi: 10.1111/jipb.13467
Xu, L., and Geelen, D. (2018). Developing biostimulants from agro-food and industrial by-products. Front. Plant Sci. 9:1567. doi: 10.3389/fpls.2018.01567
Keywords: recycling, wastewater, proline, catalase, MDA, H2O2, soilless cultivation, essential amino acids
Citation: Nicastro R, El-Nakhel C, Geelen D, Fusco GM, De Pascale S, Rouphael Y and Carillo P (2024) Exploring the potential of human urine derivatives in circular agriculture: a case study on lettuce. Front. Sustain. Food Syst. 8:1440014. doi: 10.3389/fsufs.2024.1440014
Edited by:
Jay Prakash Verma, Banaras Hindu University, IndiaReviewed by:
Peter R. Corridon, Khalifa University, United Arab EmiratesManoj Kumar Solanki, University of Silesia in Katowice, Poland
Mukesh Kumar Malviya, Sage University, India
Copyright © 2024 Nicastro, El-Nakhel, Geelen, Fusco, De Pascale, Rouphael and Carillo. This is an open-access article distributed under the terms of the Creative Commons Attribution License (CC BY). The use, distribution or reproduction in other forums is permitted, provided the original author(s) and the copyright owner(s) are credited and that the original publication in this journal is cited, in accordance with accepted academic practice. No use, distribution or reproduction is permitted which does not comply with these terms.
*Correspondence: Danny Geelen, ZGFubnkuZ2VlbGVuQHVnZW50LmJl; Petronia Carillo, cGV0cm9uaWEuY2FyaWxsb0B1bmljYW1wYW5pYS5pdA==
†These authors have contributed equally to this work