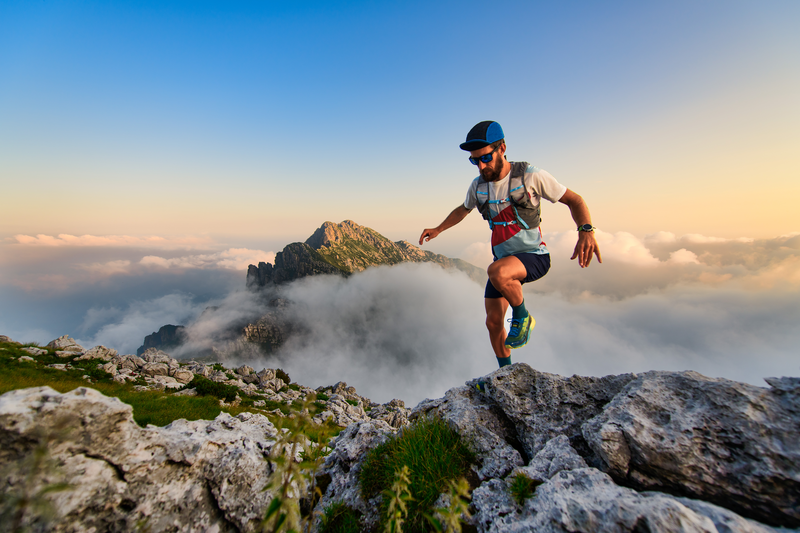
94% of researchers rate our articles as excellent or good
Learn more about the work of our research integrity team to safeguard the quality of each article we publish.
Find out more
ORIGINAL RESEARCH article
Front. Sustain. Food Syst. , 11 September 2024
Sec. Sustainable Food Processing
Volume 8 - 2024 | https://doi.org/10.3389/fsufs.2024.1436542
This article is part of the Research Topic Metabolomics to Uncover the Dynamic Changes and Formation Mechanism of Flavor in Fermented Food During Processing View all articles
Rolling plays an important role in shaping the characteristic quality of black tea. However, the dynamic alterations in volatile compounds during the rolling process remain unclear, which hampers the quality control of black tea. In this study, the dynamic changes of volatile compounds in Congou black tea throughout the rolling process were explored by a combination of GC-E-Nose and GC–MS analysis. The results of PCA and PLS-DA by GC-E-Nose revealed significant alterations in the volatile profiles, particularly at the 15-min rolling stage. A total of 82 volatile compounds including eight categories were identified in Congou black tea during the rolling process. The most abundant volatiles were aldehydes, alcohols, and esters. Through the integration of variable importance in the projection >1 and p < 0.05, 21 volatile compounds were pinpointed as pivotal volatiles responsible for distinguishing diverse rolling processes. According to their pathways, glycoside-derived volatiles (GDVs) (such as linalool and geraniol) and fatty acid-derived volatiles (FADVs) (such as (Z)-2-hexen-1-ol and hexanal) highlighted the pivotal role in the formation of the aroma of black tea during rolling process. GDVs, characterized by floral, honeyed, and fruity aromas, reached the lowest point at 35-min rolling and subsequently rebounded. FADVs exhibited an ascending trajectory during the initial 15-min rolling, followed by a downward trend. These findings provide invaluable insights into the aroma evolution during the rolling stage, offering strategies for enhancing the aroma quality of Congou black tea.
• Notable alterations in the volatile profiles occurred at 15-min rolling.
• A total of 82 volatiles were successfully identified during rolling process.
• Twenty-one volatiles played a key role in differentiating different rolling stages.
• GDVs and FADVs play an important role in aroma formation during rolling process.
Tea, acknowledged as one of the world’s most beloved beverages, is categorized into six major categories (green tea, white tea, yellow tea, oolong tea, black tea, and dark tea) in China based on the differences in processing techniques (Fang et al., 2023). Among these, Congou black tea, known as a fully fermented tea, has exceeded the others in global popularity due to its rich aroma, distinctive flavor, exquisite color, and perceived health benefits (Zheng et al., 2023). The post-harvest processing of Congou black tea typically involves withering, rolling, fermentation, and drying (Tu, 2015). As a crucial step, rolling involves curling tea leaves into fixed shapes either manually or mechanically, aiding in shaping their appearance and forming their internal qualities. Luo et al. (2016) have discovered that black tea with different modeling technology, could enhance the taste quality. In addition, the rolling process ensures the uniform mixing of tea leaves, facilitating various enzyme-catalyzed reactions and oxidation reactions during the rolling stage (Ozdemir et al., 2017). At present, relevant studies mainly focus on the effects of withering (Ye et al., 2020), fermentation (Chen et al., 2022a), and drying (Wang et al., 2023) on the aroma quality of black tea. However, the dynamic changes in volatile compounds during the rolling process are still unclear, which hampers the quality control of black tea, to some extent.
The comprehensive aroma profile of black tea is determined by an extensive array of volatile compounds with varying concentrations. The typical aromas of black tea include sweet, fruity, and floral fragrances (Li et al., 2021a). Traditional aroma quality assessment mainly relies on sensory evaluation, which is subjective and prone to variation. To overcome the limitations of human sensory perception, electronic nose (E-nose) technology, simulating the olfactory system of mammals, has been developed to provide more objective assessments (Peng et al., 2023). Gas chromatography electronic nose (GC-E-Nose), as a cutting-edge olfactory technology, offers advantages such as speediness, non-destructiveness, stability, and high sensitivity, and has been extensively employed in the tea field (Chen et al., 2022b; Wang et al., 2024a). GC-E-Nose surpasses traditional electronic nose in detecting a broader scope of volatile compounds. It is equipped with two chromatographic columns (a weak-polarity column of MXT-5 and a medium-polarity column of MXT-1701), effectively separating volatiles with diverse polarities (Liu et al., 2022). On the other hand, gas chromatography–mass spectrometry (GC–MS), renowned for its strong qualitative and quantitative capabilities, has emerged as the most prevalent and critical technology in tea aroma analysis (Zhang et al., 2023a). For instance, various storage periods of Pu-erh teas have been successfully distinguished by utilizing GC-E-Nose combined with GC–MS (Rong et al., 2023). Similarly, the profiles of volatile compounds in sweet and floral aroma black tea have been effectively characterized by using a comprehensive analysis strategy of GC-E-Nose and GC–MS (Yang et al., 2024a).
The precursors of tea aroma include lipids, glycosides, amino acids, and carotenoids (Chen et al., 2022a; El Hadi et al., 2013; Ho et al., 2015). According to the pathways, volatile compounds can be categorized into fatty acid-derived volatiles (FADVs), glycoside-derived volatiles (GDVs), amino acid-derived volatiles (AADVs), and carotenoid-derived volatiles (CDVs) (Xie et al., 2023). Considering the pivotal role of processing techniques in shaping the aroma, some scholars have endeavored to explore the mechanisms underlying aroma formation of black tea. However, these studies have predominantly focused on exploring the aroma formation throughout the full-scope manufacturing process of black tea, overlooking the unique aspects of the rolling stage. The purpose of this study is to systematically investigate the dynamic changes of volatile compounds in Congou black tea during the rolling stage via GC-E-Nose combined with GC–MS. In addition, the potential formation mechanisms for key volatile compounds were also explored. The results will provide theoretical support for quality control and strategic processing of black tea.
The 20-mL headspace vials with 18 mm magnetic PTFE/silicone caps were procured from Agilent Technologies Inc. (Palo Alto, California, United States). Ethyl decanoate was purchased from Shanghai Aladdin Biochemical Technology Co., Ltd. (Shanghai, China). Purified water was obtained from Hangzhou Wahaha Group Co., Ltd. (Hangzhou, China).
The freshly plucked leaves of “Jiukeng” cultivar from one bud one leaf to one bud two leaves were harvested in September.
bud two leaves were harvested in September, 2023 from Shengzhou City, Zhejiang Province, China. The processing protocol encompassed the stages of withering and rolling. The parameters of the rolling process were based on previous studies with some minor adjustments (Yang et al., 2024b; Zhang et al., 2023b). The specific processing flow is depicted in Supplementary Figure S1. Tea samples were collected during the rolling process at different intervals (0 min, 15 min, 35 min, 55 min, and 75 min), and subsequently subjected to freeze-drying for further analysis.
The tea samples were analyzed using GC-E-Nose (Alpha M.O.S., Toulouse, France), adhering to the method parameters detailed in a previous study (Xie et al., 2024). Initially, 0.5 g of tea samples were weighed and transferred into a 20-mL hermetically sealed headspace vial. Subsequently, the headspace vial was placed in an incubator and subjected to incubation at 60°C for 20 min. Next, 5,000 μL of headspace gas was injected at a temperature of 200°C and a pressure of 10 kPa. The programmed temperature was as follows: initially maintained at 50°C for 5 s, then increased from 50°C to 80°C at a rate of 0.1°C/s, and finally ramped from 80°C to 250°C at a rate of 0.4°C/s (holding for 100 s). The two flame ionization detectors (FIDs) were maintained at a temperature of 260°C. The volatile profiles underwent detailed analysis via the built-in AlphaSoft software. Subsequently, a qualitative assessment was performed by employing a comparative analysis of the calculated retention indices (RIs) from C6-C16 against those within the AroChemBase database (Xie et al., 2023).
The volatile compounds were analyzed using an Agilent 7890B gas chromatograph coupled to a 7000C mass spectrometer (Agilent Technologies, Palo Alto, CA, USA), following the method parameters described by Wang et al. (2024b). For each analysis, 0.5 g of tea samples, 2 μL of ethyl decanoate (internal standard, 50 mg/L), and 5 mL of pure water were introduced into a 20-mL headspace vial. Subsequently, the samples were extracted at 60°C for 60 min employing a divinylbenzene/carboxen/polydimethylsiloxane fiber (DVB/CAR/PDMS, 50/30 μm, 2 cm). After extraction, the DVB/CAR/PDMS fiber was thermally desorbed for 5 min in the GC–MS injector. Volatile compounds were separated utilizing a DB-5MS capillary column (Agilent Technologies, Palo Alto, CA, USA). The heating program of the oven temperature was as follows: initially maintained at 40°C for 5 min, then increased to 160°C at a rate of 4°C/min (maintaining for 2 min), and finally increased to 270°C at a rate of 10°C/min (maintaining for 2 min). Mass spectrometry analysis was performed in electron ionization mode at 70 eV, with a mass scan range of 40–450 m/z. The temperatures of the inlet, transfer line, and ion source were set to be 250°C, 270°C, and 230°C, respectively.
The qualitative analysis of volatile compounds was conducted using the Agilent MassHunter workstation software, via searching against the NIST 11 library. The RIs were determined using the homologous series of normal alkanes (C7 ∼ C40) and compared with data obtained from scientific literatures (https://webbook.nist.gov/chemistry/ and https://www.flavornet.org/flavornet.html). The volatile compounds were quantitatively determined using the internal standard (ethyl decanoate) method, with the following calculation formula:
whereas Ci represents the concentration of the targeted volatile component in μg/L, Ai denotes the peak area of the targeted volatile component, Cis represents the concentration of ethyl decanoate (internal standard) in μg/L, and Ais denotes the peak area of ethyl decanoate.
All the experiments were conducted in triplicate, and the results were presented as mean values ± standard deviations. Principal component analysis (PCA) and partial least squares-discriminant analysis (PLS-DA) were performed using the SIMCA-P 14.1 software (Umetrics, Umea, Sweden). The significance analysis was conducted using the SPSS Statistics 20.0 software (SPSS Inc., Chicago, IL, United States). Heat maps, bar charts, sunburst charts and change trajectories were generated using the Origin 10.1 software (OriginLab Corporation, Northampton, MA).
In this study, GC-E-Nose was employed to analyze the volatile profiles of black tea during the rolling stage. The volatile compounds were qualitatively analyzed by comparing the retention index calculated by C6-C16 with those in the AroChemBase database, and the detailed information were listed in Supplementary Table S1. The parallel radar graphs for MXT-5 and MXT-1701 were illustrated in Supplementary Figure S2. Each peak represented a specific volatile compound, with the peak area indicating its content. Notably, the alterations in peak intensity with extended rolling time demonstrated the distinct variations in volatile compounds during the rolling stage. Initially, unsupervised PCA was conducted for analysis (Fu et al., 2024). As depicted in Supplementary Figure S3, the first two principal components explained 63.3% of the total variance of R2X, with the contribution rate of PC1 achieving 44.1%, and that of PC2 reaching 19.2%. Notable spatial distribution was observed, and the scatter plots of the 15-min rolling samples were distributed far away from the scatters of other groups, indicating that volatile compounds exhibited substantial discrepancies during the rolling process, particularly at the 15-min rolling stage. Subsequently, PLS-DA was conducted on the volatile profiles obtained from GC-E-Nose. As a supervised statistical analysis technique, PLS-DA integrates partial least squares regression and linear discriminant analysis to minimize intra-group disparities and maximize inter-group differences, assisting in the classification of distinct categories or groups. The R2Y and Q2 parameters are crucial indicators for evaluating the model performance. R2Y represents the proportion of variance in the observed variables (Y variables), and Q2 represents the model’s predictive capability. The closer the values of R2Y and Q2 are to 1, the stronger the model’s explanatory and predictive power (Li et al., 2023). As illustrated in Figure 1A, a robust model with favorable parameters (R2Y = 0.910, Q2 = 0.745) was obtained, indicating its considerable explanatory and predictive power. The score plots intuitively revealed that the distances between 0-min and 15-min rolling, as well as 55-min and 75-min rolling, were larger compared to other rolling periods, suggesting that notable alterations in the volatile profiles occurred at 15-min and 75-min rolling. To further validate the model’s robustness, 200 permutation tests were performed, yielding R2 = 0.373 and Q2 = −0.469 (Figure 1B). In summary, our results demonstrate that GC-E-Nose is a promising technology capable of quickly and efficiently distinguishing the volatile profiles across various rolling stages of black tea.
Figure 1. PLS-DA results by GC-E-Nose during the rolling process. (A) Score plots (R2Y = 0.910, Q2 = 0.745); (B) Cross-validation with 200 times permutation test (R2 = 0.373, Q2 = −0.469).
The volatile compounds were analyzed by GC–MS, and the total ion chromatograms were shown in Supplementary Figure S4. A total of 82 volatile compounds were successfully identified during the rolling stage. These volatiles could be categorized into eight major classes, encompassing 15 aldehydes, 14 alcohols, 14 esters, 11 ketones, 10 alkanes, 9 aromatic compounds, 6 alkenes, and 3 heterocyclic compounds. The detailed information were listed in Supplementary Table S2. Notably, aldehydes, alcohols, and esters occupied the predominant proportions, accounting for 18.3%, 17.1%, and 17.1%, respectively (Figure 2A).
Figure 2. Volatile compounds identified by GC–MS during the rolling process. (A) Proportions of different categories; (B) Dynamic changes of different categories during the rolling process.
Additionally, obvious alterations in volatile compounds were observed during the rolling stage (Figure 2B). Aldehydes exhibited a noticeable increasing trend, whereas esters, alkanes, aromatic compounds, and heterocyclic compounds displayed distinct decreasing trends. Interestingly, alkanes (1.40 μg/L ~ 5.80 μg/L) and heterocyclic compounds (1.50 μg/L ~ 4.95 μg/L) not only demonstrated uniform changing trends during the rolling stage but also possessed comparable concentration levels. Alcohols, alkenes, and ketones displayed minor fluctuations throughout the rolling process.
To further investigate the alterations in volatile compounds during the rolling stage, PLS-DA was conducted on the acquired volatile compounds. Favorable model was achieved with parameters of R2Y = 0.892 and Q2 = 0.613, indicating the robust explanatory and predictive capabilities. As depicted in the Figure 3A, considerable changes occurred during the rolling stage, particularly at the 15-min rolling. In addition, 200 permutation tests were implemented, yielding parameters of R2 = 0.530, Q2 = −0.539. All Q2 points were lower than the rightmost point, confirming the validity of the model (Figure 3B). Moreover, utilizing the criteria of variable importance in the projection (VIP) > 1 and p < 0.05, 21 key volatile compounds were pinpointed, such as 1,2-dimethyl-4-vinylbenzene (VIP = 1.38), o-cymene (VIP = 1.37), β-myrcene (VIP = 1.18), linalool (VIP = 1.11), and (+)-4-carene (VIP = 1.03), which functioned as pivotal differentiators among diverse rolling stages (Figure 3C).
Figure 3. Multivariate statistical analysis on the volatile compounds obtained from GC–MS. (A) Score plots of PLS-DA (R2Y = 0.892, Q2 = 0.613); (B) Cross-validation with 200 times permutation test (R2 = 0.530, Q2 = −0.539); (C) A total of 21 key compounds with VIP > 1.
Based on the pathways, the aforementioned 21 key differentiating volatile compounds were classified into five categories: 8 GDVs, 6 FADVs, 3 AADVs, 1 CDV, and 3 others (Figure 4A). This categorization highlighted the significance of GDVs and FADVs in the aroma formation of black tea during the rolling stage. As shown in Fig. S5, the level of GDVs exhibited a descending from the initial 0-min rolling (107.90 μg/L) to the 35-min rolling (75.36 μg/L), subsequently exhibiting an ascending trend. As far as FADVs is concerned, the concentration of FADVs increased from the 0-min rolling (11.97 μg/L) to the 15-min rolling (31.24 μg/L), followed by a downward trend. As for the AADVs, the concentration progressively escalated throughout the rolling process (from 20.14 μg/L to 38.09 μg/L). The concentration of CDV was several orders of magnitude inferior compared to other three categories. However, owing to the low threshold characteristics of CDV, its influence could not be overlooked (Xu et al., 2023).
Figure 4. A total of 21 key differential volatile compounds. (A) Categories of 21 volatile compounds; (B) Formation pathways. LOX: lipoxygenase; HPL: hydroperoxide lyase; ADH: alcohol dehydrogenase; GPP, geranyl pyrophosphate; CCD, carotenoid cleavage dioxygenase.
FADVs are prevalent and essential volatile compounds in tea (Li et al., 2021b). In this study, characteristic FADVs included hexanal, (Z)-2-hexen-1-ol, 2-butylfuran, (E)-4-heptenal, (E, E)-2,4-heptadienal and (E)-2-nonenal. FADVs were mainly derived from lipid oxidation, and there existed two primary pathways for generating FADVs (Ho et al., 2015). The first pathway involved free radical-induced auto-oxidation reaction, with (E, E)-2,4-heptadienal generated from the auto-oxidation of α-linolenic acid (Figure 4B) (El Hadi et al., 2013). (E, E)-2,4-Heptadienal could enhance the freshness of the aroma (Yang et al., 2024b; Zhang et al., 2023c). It was evident that the concentration of (E, E)-2,4-heptadienal was consistently increased during the rolling stage (Figure 5A). On the other hand, the applied force could potentially cause the breakage of tea leaves, leading to the release of cell sap that accelerated the auto-oxidation of α-linolenic acid and resulted in a higher concentration of (E, E)-2,4-heptadienal.
Figure 5. Dynamics changes of key differential volatile compounds during rolling process. (A) Fatty acid-derived volatiles; (B) Glycoside-derived volatiles; (C) Amino acid-derived volatiles; (D) Carotenoid-derived volatiles; (E) Other types.
The second pathway was the lipoxygenase-mediated lipid oxidation pathway, which was the main pathway for tea aroma generation (Ho et al., 2015). Of the six FADVs identified in this study, five FADVs such as hexanal, (E)-4-heptenal, and (E)-2-nonenal were produced through this pathway (Figure 4B). Specifically, hexanal stood as one of the key aroma compounds in sweet-floral aroma black tea, contributing to the unique green, leafy, and grassy aromas (Yang et al., 2022a). In this study, hexanal exhibited a progressively increasing pattern throughout the rolling process (Figure 5A). It was produced from the oxidation of linoleic acid by lipoxygenase (LOX), which was subsequently further cleaved by hydroperoxide lyases (HPL). Similarly, (E)-4-heptenal and (E)-2-nonenal were the oxidation products of palmitoleic acid and α-linolenic acid, respectively (Caipo et al., 2021). (E)-4-Heptenal was recognized as a crucial aroma compound in Dianhong Congou tea, and it exhibited a continuous ascend during the rolling stage (Chen et al., 2022b). Additionally, (E)-2-nonenal was reported to exhibit a strong and intense aroma in Assam black tea, and it attained the lowest concentration at 35-min rolling (Kang et al., 2019). It is worth mentioning that these aldehydes could be transformed into the corresponding alcohols under the action of alcohol dehydrogenases (ADH) or undergo isomerization reactions. For instance, (Z)-2-hexen-1-ol was predominantly formed through the oxidation of α-linolenic acid to 13S-hydroperoxylinolenic acid, which subsequently gave rise to (Z)-2-hexenal under the action of HPL and further underwent isomerization (Chen et al., 2022a). (Z)-2-Hexen-1-ol was generally associated with a banana-like fruity aroma, and it achieved the maximum concentration at 15-min rolling (Ríos-Reina et al., 2023). As for 2-butylfuran, the formation primarily involved linoleic acid via LOX and ADH reactions to yield (E)-2-octenal, which was further transformed under the catalysts of amino acids (Adams et al., 2011). As an important N,O-heterocyclic compound in tea, 2-butylfuran exhibited a decreased trend from 0-min rolling to 35-min rolling, subsequently increasing progressively (Yang et al., 2022b).
GDVs in fresh tea leaves generally exist in glycosidic forms, and are easily hydrolyzed by endogenous glycosidases during processing, which generally exhibit floral, honey, and fruity aromas (Yang et al., 2024a; Wang et al., 2022). In this study, representative GDVs such as benzyl alcohol, β-myrcene, (+)-4-carene, o-cymene, linalool, 1,3,8-p-menthatriene, geraniol, and β-citral were identified.
Geraniol and linalool were formed from geranyl pyrophosphate (geranyl-PP) precursor, liberated by geraniol synthase and linalool synthase, respectively. β-Citral and β-myrcene were the derivatives of geraniol and linalool, respectively (Figure 4B) (Ho et al., 2015; Yang et al., 2024a). These compounds were typical glycosidically bound terpene alcohols, primarily exhibiting floral, sweet, and fruity aromas (Liu et al., 2023; Ouyang et al., 2022). Rolling process influences the levels and compositions of GDVs in tea by causing cellular damage, which releases various enzymes and substrates that interact to form or alter GDVs. For example, increased interaction between enzyme and substrate during rolling can accelerate β-glucosidase reactions, promoting the release of GDVs. β-Citral exhibited an upward trend during the rolling process, and was recognized as an essential aroma-active compound in sweet aroma black tea (Yang et al., 2024a). Geraniol, offering a rose-like aroma, was one of the primary aroma components in green tea, black tea, and oolong tea, and exhibited an increasing trend during the rolling process (Yu et al., 2021; Zhang et al., 2020). Linalool with citrus and floral notes, exhibited a descending trend during rolling (Figure 5B) (Chen et al., 2022b; Yang et al., 2022b). β-Myrcene, possessing geranium-like, carrot-like, and hop-like aromas, was a pivotal volatile component in sweet floral black tea. It attained the maximize level at 15-min rolling (Chen et al., 2022c; Yang et al., 2024a).
O-cymene was reported to play an essential role in the floral aroma of Wuyi rock tea (Peng et al., 2023). It demonstrated similar trend to β-myrcene during rolling, reaching the highest level at 15-min rolling prior to stabilization. Benzyl alcohol, a typical GDV and aromatic alcohol derived from glycosidic substances, contributed to the sweetness and roasty notes (Ho et al., 2015; Ouyang et al., 2022; Xie et al., 2024). It displayed a progressive upward trend during the rolling stage, which corroborated with the finding of Chen et al. (2022a). (+)-4-Carene was identified as one of the key aroma distinctions between shaken black tea and traditional black tea (Wang et al., 2023). It declined from 0-min rolling to 35-min rolling, and then remained stable unaltered. 1,3,8-p-Menthatriene attained its lowest concentration at 35-min rolling (Figure 5B).
Amino acids perform a dual role in tea, serving not merely as crucial taste factors but also as the precursors of volatiles (Yang et al., 2024a). In this study, the representative AADVs including styrene, 1,2-dimethyl-4-vinylbenzene, and benzeneacetaldehyde are highlighted. The primary pathway of styrene in plants was from linolenic acid, which was derived from phenylalanine (Schwab et al., 2008; Warhurst and Fewson, 1994). Benzeneacetaldehyde is derived from phenylalanine via Strecker degradation (Figure 4B). The generally mechanism of Strecker degradation of amino acids involves the nucleophilic addition of an amine group to a carbonyl group, generating an unstable aldimine, which subsequently eliminates a molecule of water to form a Schiff base. Subsequent decarboxylation yields an imine polymer, which then undergoes the addition of a molecule of water to form an unstable amino acid. Ultimately, this amino acid decomposes into a ketoamine and Strecker aldehyde, resulting in the formation of the Strecker aldehyde possessing one less carbon atom than its precursor amino acid (Ho et al., 2015). As depicted in Figure 5C, styrene exhibited a “V” pattern throughout the rolling stage, with the lowest concentration occurring at 35-min rolling. Styrene was characterized by sweet and floral aromas, exhibiting notable manifestation during the fermentation phase of black tea (Xiao et al., 2022). 1,2-Dimethyl-4-vinylbenzene exhibited an inverted “V” trend throughout the rolling stage, with the highest concentration occurring at 15-min rolling. Benzeneacetaldehyde exhibited a progressive upward trajectory during the rolling stage, corresponding with previous findings (Chen et al., 2022a). It is noteworthy that benzeneacetaldehyde possessed rose-like and sweet aromas, and was the dominant aromatic compound in Dajiling black tea (Xie et al., 2024).
Carotenoids, a category of tetraterpenoid pigments, are commonly stored in the plastids of plant leaves, flowers, and fruits. They play an indispensable and crucial role as accessory pigments and colorants in photosynthesis. Additionally, carotenoids act as vital precursors influencing the aroma quality of tea (Schwab et al., 2008). Typically, CDVs are generated through the action of carotenoid cleavage dioxygenase (CCD), resulting in the formation of carotenoids and their degradation products (El Hadi et al., 2013). In this study, typical CDVs such as geranyl acetone was identified in the rolling stage of black tea. Geranyl acetone possessed a low threshold, contributing to the unique sweet and floral aromas of black tea (Xu et al., 2023). It was derived from phytoene via CCD catalysis (Figure 4B). The concentration of geranyl acetone exhibited a distinctive “V” trend during the rolling stage. Specifically, it commenced from the highest concentration (0.31 μg/L) at 0-min rolling, gradually declined to 0.15 μg/L at 35-min rolling, and subsequently ascended to 0.29 μg/L at 75-min rolling (Figure 5D).
We also identified three other types of volatile compounds such as cis-3-hexenyl benzoate, decanoic acid, methyl ester, and m-xylene that markedly influenced the aroma during the rolling stage. Cis-3-hexenyl benzoate reached its peak at 0-min rolling, with the lowest point at 35-min rolling (Figure 5E). Decanoic acid, methyl ester was reported to play an important role in the formation of fruity and floral aromas (Tchouakeu Betnga et al., 2020). It reached the lowest concentrations at 35-min rolling. m-Xylene was reported as a predominant volatile component in aloe tea, exhibiting floral and sweet aromas (Zhang et al., 2024). In this study, m-xylene reached its peak concentration at 15-min rolling, followed by a primarily decreasing trend, reaching its lowest concentration at 75-min rolling.
This study employed a combination of GC-E-Nose and GC–MS analysis for exploring the dynamic alterations in volatile compounds throughout the rolling process of black tea. The PLS-DA results of GC-E-Nose and GC-MS demonstrated the capability to rapidly distinguish black teas at different rolling stages. Notable alterations in the volatile profiles occurred at 15-min rolling. A total of 82 volatile compounds were identified by GC–MS. Through integration of VIP > 1 and p < 0.05, 21 volatile compounds such as β-myrcene, (+)-4-carene, o-cymene, 1,2-dimethyl-4-vinylbenzene, and linalool were pinpointed as pivotal volatiles responsible for distinguishing diverse stages of the rolling process. Notably, AADVs such as benzeneacetaldehyde, primarily exhibiting sweet and floral fragrances, exhibited an upward trend during the rolling stage. GDVs with floral, honeyed, and fruity notes, attained their lowest contents at 35-min rolling and subsequently rebounded. In conclusion, this study provides valuable insights into the aroma evolution during the rolling stage, offering strategies for enhancing the aroma quality of black tea. Despite the promising results, this study does possess certain limitations. Although the key differential volatile compounds were classified based on their formation pathways, the investigation did not quantify the precursors of these volatiles or the specific environmental conditions influencing their formation. As future innovations permit, we will monitor the rolling temperature to better understand the impact of temperature on the formation and/or degradation of volatile compounds during the rolling process. In addition, we intend to explore the changes of precursor substances during the rolling process, and trace the specific formation pathway of volatile substances by using stable isotope tracer technology.
The original contributions presented in the study are included in the article/Supplementary material, further inquiries can be directed to the corresponding authors.
QW: Data curation, Formal analysis, Investigation, Methodology, Resources, Software, Validation, Visualization, Writing – original draft. DS: Conceptualization, Resources, Software, Writing – original draft. JH: Formal analysis, Software, Writing – original draft. JT: Formal analysis, Software, Writing – original draft. XZ: Software, Validation, Writing – original draft. LW: Resources, Software, Writing – original draft. JX: Formal analysis, Resources, Writing – original draft. YJ: Funding acquisition, Project administration, Writing – review & editing. HY: Funding acquisition, Project administration, Writing – review & editing. YY: Conceptualization, Investigation, Writing – review & editing.
The author(s) declare that financial support was received for the research, authorship, and/or publication of this article. This work was supported by the Science and Technology Innovation Project of the Chinese Academy of Agricultural Sciences (CAAS-ASTIP-TRICAAS) and China Agriculture Research System of MOF and MARA (CARS-19).
The authors declare that the research was conducted in the absence of any commercial or financial relationships that could be construed as a potential conflict of interest.
All claims expressed in this article are solely those of the authors and do not necessarily represent those of their affiliated organizations, or those of the publisher, the editors and the reviewers. Any product that may be evaluated in this article, or claim that may be made by its manufacturer, is not guaranteed or endorsed by the publisher.
The Supplementary material for this article can be found online at: https://www.frontiersin.org/articles/10.3389/fsufs.2024.1436542/full#supplementary-material
Adams, A., Bouckaert, C., Van Lancker, F., De Meulenaer, B., and De Kimpe, N. (2011). Amino acid catalysis of 2-Alkylfuran formation from lipid oxidation-derived α,β-unsaturated aldehydes. J. Agric. Food Chem. 59, 11058–11062. doi: 10.1021/jf202448v
Caipo, L., Sandoval, A., Sepúlveda, B., Fuentes, E., Valenzuela, R., Metherel, A. H., et al. (2021). Effect of storage conditions on the quality of Arbequina extra virgin olive oil and the impact on the composition of flavor-related compounds (phenols and volatiles). Food Secur. 10:2161. doi: 10.3390/foods10092161
Chen, J., Yang, Y., Deng, Y., Liu, Z., Shen, S., Zhu, J., et al. (2022c). Characterization of the key differential volatile components in different grades of Dianhong Congou tea infusions by the combination of sensory evaluation, comprehensive two-dimensional gas chromatography-time-of-flight mass spectrometry, and odor activity value. LWT 165:113755. doi: 10.1016/j.lwt.2022.113755
Chen, J., Yang, Y., Deng, Y., Liu, Z., Xie, J., Shen, S., et al. (2022b). Aroma quality evaluation of Dianhong black tea infusions by the combination of rapid gas phase electronic nose and multivariate statistical analysis. LWT 153:112496. doi: 10.1016/j.lwt.2021.112496
Chen, Q., Zhu, Y., Liu, Y., Liu, Y., Dong, C., Lin, Z., et al. (2022a). Black tea aroma formation during the fermentation period. Food Chem. 374:131640. doi: 10.1016/j.foodchem.2021.131640
El Hadi, M., Zhang, F.-J., Wu, F.-F., Zhou, C.-H., and Tao, J. (2013). Advances in fruit aroma volatile research. Molecules 18, 8200–8229. doi: 10.3390/molecules18078200
Fang, X., Liu, Y., Xiao, J., Ma, C., and Huang, Y. (2023). GC–MS and LC-MS/MS metabolomics revealed dynamic changes of volatile and non-volatile compounds during withering process of black tea. Food Chem. 410:135396. doi: 10.1016/j.foodchem.2023.135396
Fu, Z., Hao, S., Zhou, J., Feng, W., Zhu, M., Wu, J., et al. (2024). Profiling volatile compounds in fresh leaves of 22 major oolong tea germplasm cultivated in Fujian of China. Sci. Hortic. 327:112849. doi: 10.1016/j.scienta.2024.112849
Ho, C.-T., Zheng, X., and Li, S. (2015). Tea aroma formation. Food Sci. Human Wellness 4, 9–27. doi: 10.1016/j.fshw.2015.04.001
Kang, S., Yan, H., Zhu, Y., Liu, X., Lv, H.-P., Zhang, Y., et al. (2019). Identification and quantification of key odorants in the world’s four most famous black teas. Food Res. Int. 121, 73–83. doi: 10.1016/j.foodres.2019.03.009
Li, J., Hua, J., Yuan, H., Deng, Y., Zhou, Q., Yang, Y., et al. (2021b). Investigation on green tea lipids and their metabolic variations during manufacturing by nontargeted lipidomics. Food Chem. 339:128114. doi: 10.1016/j.foodchem.2020.128114
Li, C., Yue, C.-N., Yang, P.-X., Cao, H.-H., Zhu, Y.-H., Lin, S.-H., et al. (2021a). Research progress on characteristic aroma of Congou black tea. J. Food Saf. Qual. 12, 8834–8842. doi: 10.19812/j.cnki.jfsq11-5956/ts.2021.22.028
Li, Q., Zhang, C., Wang, H., Chen, S., Liu, W., Li, Y., et al. (2023). Machine learning technique combined with data fusion strategies: a tea grade discrimination platform. Ind. Crop. Prod. 203:117127. doi: 10.1016/j.indcrop.2023.117127
Liu, Y., Chen, Q., Liu, D., Yang, L., Hu, W., Kuang, L., et al. (2023). Multi-omics and enzyme activity analysis of flavour substances formation: major metabolic pathways alteration during Congou black tea processing. Food Chem. 403:134263. doi: 10.1016/j.foodchem.2022.134263
Liu, H.-Y., Liu, Y., Li, M.-Y., Mai, Y.-H., Guo, H., Wadood, S. A., et al. (2022). The chemical, sensory, and volatile characteristics of instant sweet tea (Lithocarpus litseifolius [Hance] Chun) using electronic nose and GC-MS-based metabolomics analysis. LWT 163:113518. doi: 10.1016/j.lwt.2022.113518
Luo, X., Li, L., Zou, Y., Jing, T., and Zhao, X. (2016). Effect of different modeling technology on quality of granular black tea. Guizhou Agricultural Sciences 44, 152–155. doi: 10.3969/j.issn.1001-3601.2016.02.039
Ouyang, W., Yu, Y., Wang, H., Jiang, Y., Hua, J., Ning, J., et al. (2022). Analysis of volatile metabolite variations in strip green tea during processing and effect of rubbing degree using untargeted and targeted metabolomics. Food Res. Int. 162:112099. doi: 10.1016/j.foodres.2022.112099
Ozdemir, F., Tontul, I., Balci-Torun, F., and Topuz, A. (2017). Effect of rolling methods and storage on volatile constituents of Turkish black tea. Flavour Fragr. J. 32, 362–375. doi: 10.1002/ffj.3385
Peng, Q., Li, S., Zheng, H., Meng, K., Jiang, X., Shen, R., et al. (2023). Characterization of different grades of Jiuqu hongmei tea based on flavor profiles using HS-SPME-GC-MS combined with E-nose and E-tongue. Food Res. Int. 172:113198. doi: 10.1016/j.foodres.2023.113198
Ríos-Reina, R., Aparicio-Ruiz, R., Morales, M. T., and García-González, D. L. (2023). Contribution of specific volatile markers to green and ripe fruity attributes in extra virgin olive oils studied with three analytical methods. Food Chem. 399:133942. doi: 10.1016/j.foodchem.2022.133942
Rong, Y., Xie, J., Yuan, H., Wang, L., Liu, F., Deng, Y., et al. (2023). Characterization of volatile metabolites in Pu-erh teas with different storage years by combining GC-E-Nose, GC–MS, and GC-IMS. Food Chemistry: X 18. doi: 10.1016/j.fochx.2023.100693
Schwab, W., Davidovich-Rikanati, R., and Lewinsohn, E. (2008). Biosynthesis of plant-derived flavor compounds. Plant J. 54, 712–732. doi: 10.1111/j.1365-313X.2008.03446.x
Tchouakeu Betnga, P. F., Dupas de Matos, A., Longo, E., and Boselli, E. (2020). Impact of closure material on the chemical and sensory profiles of grappa during storage in bottle. LWT 133:110014. doi: 10.1016/j.lwt.2020.110014
Tu, Y.-Y. (2015). The effects of processing technology on the quality of black tea. J. Tea Cul. Industry Stud. 30, 309–330. doi: 10.21483/qwoaud.30.201512.309
Wang, L., Xie, J., Deng, Y., Jiang, Y., Tong, H., Yuan, H., et al. (2023). Volatile profile characterization during the drying process of black tea by integrated volatolomics analysis. LWT 184:115039. doi: 10.1016/j.lwt.2023.115039
Wang, Q., Xie, J., Wang, L., Jiang, Y., Deng, Y., Zhu, J., et al. (2024b). Comprehensive investigation on the dynamic changes of volatile metabolites in fresh scent green tea during processing by GC-E-nose, GC–MS, and GC × GC-TOFMS. Food Res. Int. 187:114330. doi: 10.1016/j.foodres.2024.114330
Wang, L., Yang, Y., Xie, J., Miao, Y., Wang, Q., Jiang, Y., et al. (2024a). Aroma quality evaluation of high-quality and quality-deficient black tea by electronic nose coupled with gas chromatography-mass spectrometry. Food Sci. 45, 274–282. doi: 10.7506/spkx1002-6630-20230417-163
Wang, H., Yu, Y., Ouyang, W., Jiang, Y., Wang, J., Hua, J., et al. (2022). Assessment of variations in round green tea volatile metabolites during manufacturing and effect of second-drying temperature via nontargeted Metabolomic analysis. Front. Nutr. 9:877132. doi: 10.3389/fnut.2022.877132
Warhurst, A. M., and Fewson, C. A. (1994). Microbial metabolism and biotransformations of styrene. J. Appl. Bacteriol. 77, 597–606. doi: 10.1111/j.1365-2672.1994.tb02807.x
Xiao, Y., Huang, Y., Chen, Y., Zhu, M., He, C., Li, Z., et al. (2022). Characteristic fingerprints and change of volatile organic compounds of dark teas during solid-state fermentation with Eurotium cristatum by using HS-GC-IMS, HS-SPME-GC-MS, E-Nose and sensory evaluation. LWT 169:113925. doi: 10.1016/j.lwt.2022.113925
Xie, J., Wang, Q., Cui, H., Wang, L., Deng, Y., Yuan, H., et al. (2024). Characterization of Gardenia tea based on aroma profiles using GC-E-nose, GC-O-MS and GC × GC-TOFMS combined with chemometrics. Beverage Plant Res. 4:e001. doi: 10.48130/bpr-0023-0034
Xie, J., Wang, L., Deng, Y., Yuan, H., Zhu, J., Jiang, Y., et al. (2023). Characterization of the key odorants in floral aroma green tea based on GC-E-nose, GC-IMS, GC-MS and aroma recombination and investigation of the dynamic changes and aroma formation during processing. Food Chem. 427:136641. doi: 10.1016/j.foodchem.2023.136641
Xu, Y., Liu, Y., Yang, J., Wang, H., Zhou, H., and Lei, P. (2023). Manufacturing process differences give Keemun black teas their distinctive aromas. Food Chem. 19:100865. doi: 10.1016/j.fochx.2023.100865
Yang, Y., Xie, J., Chen, J., Deng, Y., Shen, S., Hua, J., et al. (2022b). Characterization of N,O-heterocycles in green tea during the drying process and unraveling the formation mechanism. Food Control 139:109079. doi: 10.1016/j.foodcont.2022.109079
Yang, Y., Xie, J., Wang, Q., Deng, Y., Zhu, L., Zhu, J., et al. (2024a). Understanding the dynamic changes of volatile and non-volatile metabolites in black tea during processing by integrated volatolomics and UHPLC-HRMS analysis. Food Chem. 432:137124. doi: 10.1016/j.foodchem.2023.137124
Yang, Y., Xie, J., Wang, Q., Wang, L., Shang, Y., Jiang, Y., et al. (2024b). Volatolomics-assisted characterization of the key odorants in green off-flavor black tea and their dynamic changes during processing. Food Chem. 22:101432. doi: 10.1016/j.fochx.2024.101432
Yang, Y., Zhu, H., Chen, J., Xie, J., Shen, S., Deng, Y., et al. (2022a). Characterization of the key aroma compounds in black teas with different aroma types by using gas chromatography electronic nose, gas chromatography-ion mobility spectrometry, and odor activity value analysis. LWT 163:113492. doi: 10.1016/j.lwt.2022.113492
Ye, Y., Dong, C., Luo, F., Cui, J., Liao, X., Lu, A., et al. (2020). Effects of withering on the main physical properties of withered tea leaves and the sensory quality of Congou black tea. J. Texture Stud. 51, 542–553. doi: 10.1111/jtxs.12498
Yu, J., Liu, Y., Zhang, S., Luo, L., and Zeng, L. (2021). Effect of brewing conditions on phytochemicals and sensory profiles of black tea infusions: a primary study on the effects of geraniol and β-ionone on taste perception of black tea infusions. Food Chem. 354:129504. doi: 10.1016/j.foodchem.2021.129504
Zhang, S., Sun, L., Wen, S., Chen, R., Sun, S., Lai, X., et al. (2023a). Analysis of aroma quality changes of large-leaf black tea in different storage years based on HS-SPME and GC–MS. Food Chem. 20:100991. doi: 10.1016/j.fochx.2023.100991
Zhang, H., Wang, J., Wang, H., Cheng, C., Zhang, X., Xue, J., et al. (2024). Comparative analysis of asparagus tea processing and flavor component analysis. LWT 194:115795. doi: 10.1016/j.lwt.2024.115795
Zhang, S., Wu, S., Yu, Q., Shan, X., Chen, L., Deng, Y., et al. (2023b). The influence of rolling pressure on the changes in non-volatile compounds and sensory quality of Congou black tea: the combination of metabolomics, E-tongue, and chromatic differences analyses. Food Chem. 20:100989. doi: 10.1016/j.fochx.2023.100989
Zhang, J., Xia, D., Li, T., Wei, Y., Feng, W., Xiong, Z., et al. (2023c). Effects of different over-fired drying methods on the aroma of Lu’an Guapian tea. Food Res. Int. 173:113224. doi: 10.1016/j.foodres.2023.113224
Zhang, M., Yang, Y., Yuan, H., Hua, J., Deng, Y., Jiang, Y., et al. (2020). Contribution of addition theanine/sucrose on the formation of chestnut-like aroma of green tea. LWT 129:109512. doi: 10.1016/j.lwt.2020.109512
Keywords: rolling, black tea, volatile compounds, GC-E-nose, GC–MS.
Citation: Wang Q, Shi D, Hu J, Tang J, Zhou X, Wang L, Xie J, Jiang Y, Yuan H and Yang Y (2024) Unraveling the dynamic changes of volatile compounds during the rolling process of Congou black tea via GC-E-nose and GC–MS. Front. Sustain. Food Syst. 8:1436542. doi: 10.3389/fsufs.2024.1436542
Received: 22 May 2024; Accepted: 26 August 2024;
Published: 11 September 2024.
Edited by:
Bruna Laratta, Institute of Bioscience and Bioresources, National Research Council (CNR), ItalyReviewed by:
Qingli Dong, University of Shanghai for Science and Technology, ChinaCopyright © 2024 Wang, Shi, Hu, Tanga, Zhou, Wang, Xie, Jiang, Yuan and Yang. This is an open-access article distributed under the terms of the Creative Commons Attribution License (CC BY). The use, distribution or reproduction in other forums is permitted, provided the original author(s) and the copyright owner(s) are credited and that the original publication in this journal is cited, in accordance with accepted academic practice. No use, distribution or reproduction is permitted which does not comply with these terms.
*Correspondence: Haibo Yuan, MTkyMTY4MDkyQHRyaWNhYXMuY29t; Yanqin Yang, eWFuZ3lxQHRyaWNhYXMuY29t
†These authors have contributed equally to this work
Disclaimer: All claims expressed in this article are solely those of the authors and do not necessarily represent those of their affiliated organizations, or those of the publisher, the editors and the reviewers. Any product that may be evaluated in this article or claim that may be made by its manufacturer is not guaranteed or endorsed by the publisher.
Research integrity at Frontiers
Learn more about the work of our research integrity team to safeguard the quality of each article we publish.