- 1College of Food and Biological Engineering, Chengdu University, Chengdu, China
- 2School of Public Health, Chengdu University of Traditional Chinese Medicine, Chengdu, China
- 3College of Children’s Development and Health Management, Chongqing Vocational and Technical University of Mechatronics, Chongqing, China
Lactobacillus plantarum is a functional probiotic and could be used in yogurt fermentation to improve the function and flavor. However, L. plantarum has relatively poor acid resistance, and cell viability gradually decreases as pH decreases during yogurt fermentation. Therefore, exploring suitable strategies to promote the fermentation of L. plantarum for yogurt processing is important. In the present study, the effects of quinoa protein hydrolysates (QPHs) on the qualities and volatiles of yogurt fermented by L. plantarum were investigated. QPH addition significantly promoted bacterial growth and organic acids production, subsequently improved the water-holding capacity and viscosity of yogurt. QPH addition also increased the overall antioxidant capacity of yogurt, but the DPPH radical-scavenging ability of 1% QPH-supplemented yogurt was significantly greater than that of 2% QPH-supplemented yogurt. Additionally, QPHs promoted the metabolism of L. plantarum and further promoted the production of volatile flavor compounds. Fifty-two volatile compounds (mainly acids, esters, and ketones) were detected in 2% QPH-supplemented yogurt, which was 1.86-fold greater than the number detected in the control samples. Some aroma components, such as nonanoic acid and maltol, were significantly increased, but undesirable volatiles, such as decanal, were decreased. QPH composition analysis indicated that 60.79% of the peptides contained hydrophobic amino acids at the N-terminus or the C-terminus, which may explain QPHs’ strong antioxidant properties.
1 Introduction
Yogurt is a common fermented dairy product that plays an important role in the human diet due to its unique flavor, high nutritional value, and functional properties (Dan et al., 2022). Streptococcus thermophilus and Lactobacillus bulgaricus, which improve the quality of yogurt, are often used for yogurt fermentation (Dan et al., 2019). However, most S. thermophilus and L. bulgaricus strains may lead to galactose accumulation in yogurt, which may result in a health burden for humans (especially for patients with galactosemia) (Zhang S. S. et al., 2020). Lactobacillus plantarum is a functional probiotic that has been widely studied in recent years, and L. plantarum has also been used in yogurt fermentation to promote the production of amino acids and fatty acids (Liu et al., 2022). Studies have shown that the consumption of fermented milk containing L. plantarum can improve health via regulation of the intestinal flora, immunomodulatory effects, and reduced gestational hypertension (Zhang et al., 2017; Choi et al., 2023; Yi et al., 2023). However, L. plantarum has relatively poor acid resistance, and cell viability gradually decreases as pH gradually decreases during yogurt fermentation. Therefore, exploring suitable strategies to promote the growth and fermentation of L. plantarum for yogurt processing is important.
Plant protein hydrolysates mainly provide abundant nitrogen sources, such as free amino acids and peptides (Ashaolu, 2020), which are absorbed by microorganisms to balance nitrogen sources or to promote the biosynthesis of intracellular protectants (Li et al., 2021), thereby promoting the fermentation and environmental tolerance of microorganisms. In recent years, several studies have shown that protein hydrolysates have biological activities, including antioxidant (Torres-Fuentes et al., 2015), antidiabetic (Al-Bukhaiti et al., 2023), and pro-fermentation (Li et al., 2021) activities. Furthermore, the addition of plant protein hydrolysates has also been demonstrated to enhance the quality and flavor of fermented foods (Ghelich et al., 2022). Wongsa et al. (2022) reported that the addition of rice protein hydrolysate improved the flavor and texture of yogurt and improved its functional properties.
Quinoa (Chenopodium quinoa Willd.) is a low-fat, low-calorie healthy food with comprehensive nutritional value, and is considered by the Food and Agriculture Organization (FAO) of the United Nations to be a perfect food that meets all the requirements of the human body (Vazquez-Luna et al., 2019). Quinoa is rich in protein, with a protein content as high as 23% (Abbasi et al., 2022), which is higher than that of traditional cereals (Huang et al., 2022). The essential amino acid content in quinoa protein is greater than those in other cereals and beans (López et al., 2018). Recently, researchers have identified antioxidant peptides, antihypertensive peptides, and antidiabetic peptides from quinoa protein hydrolysates (Abbasi et al., 2022), and our previous studies demonstrated that quinoa protein hydrolysates (QPHs) could significantly improve the antioxidant properties and flavor of quinoa beverages (Meng et al., 2022b). Based on these studies, QPHs have certain application prospects for promoting the fermentation efficiency of lactic acid bacteria (LAB) and the function and qualities of yogurt. However, no reports on the effect of QPH on the properties and flavor of yogurt are available. Therefore, this study investigated the effects of QPH addition on milk fermentation to provide a reference for the subsequent development of L. plantarum-fermented functional yogurt. In addition, quinoa peptides were isolated and purified to provide a reference for the application of functional quinoa peptides.
2 Materials and methods
2.1 Materials and chemicals
Quinoa (Chenopodium quinoa Willd.) was provided by the Key Laboratory of Coarse Cereal Processing, Ministry of Agriculture and Rural Affairs of China (Chengdu, China). Lactobacillus plantarum LZBY2-2 was isolated from Tibetan highland barley liquor koji and stored at −80°C before use (Meng et al., 2022a). Pure milk was purchased from a local supermarket. Papain, trypsin, and organic acid standards were purchased from Shanghai Yuanye Biotechnology Co., Ltd. (Shanghai, China). MRS culture medium was purchased from Beijing Aoboxing Biotechnology Co., Ltd. (Beijing, China). 2,2-Diphenyl-1-pyridylhydrazide (DPPH) was purchased from Sigma Aldrich Chemical Company (St. Louis, MO, United States). 2,2′-Azinobis (3-ethylbenzothiazole-6-sulfonic acid ammonium salt) (ABTS) was purchased from Shanghai Hualan Chemical Technology Co., Ltd. (Shanghai, China). All other chemicals were of analytical reagent grade.
2.2 Preparation of quinoa protein hydrolysate
Crushed quinoa powder was sifted through a 100-mesh sieve, and anhydrous ethanol was added at a ratio of 1:5 (w:v) for degreasing. The reaction was conducted at room temperature for 10 h. After samples were oven dried, an appropriate amount of degreased quinoa powder was weighed, ultrapure water was added at a ratio of 1:10 (w:v), the pH was adjusted to 10.0 with 1 M NaOH, and the samples were then reacted in a water bath at 45°C for 2 h. The samples were subsequently centrifuged at 5000 × g for 20 min, the supernatant was obtained, and the pH was adjusted to 4.5 with 1 M HCl. After standing for 30 min, the sample was centrifuged again. The precipitate was collected and lyophilized.
QPHs were prepared according to a previous study (Zhou et al., 2023) with slight modifications: 5% (w/v) quinoa protein solution was prepared with ultrapure water, the pH was adjusted to 7.5, and 1% (w/w) papain and 1% (w/w) trypsin were added. After reacting at 50°C for 5 h, the enzyme reaction mixture was inactivated by boiling for 15 min and then cooled to room temperature. After centrifugation, the supernatant was collected and lyophilized. The QPH powder was stored in a refrigerator at −18°C for subsequent experiments.
2.3 Preparation of fermented yogurt
Pure milk was mixed with 1 and 2% QPs (w/v) and 1 and 2% QPHs (w/v), and then the mixture was pasteurized at 75°C for 20 min and quickly cooled to 40°C. The activated L. plantarum was inoculated separately according to a total inoculated amount of 4 × 107, fermented at 37°C for 12 h, and then stored at 4°C for 12 h. Pasteurized milk treated under the same L. plantarum inoculation but without QPs or QPHs addition was used as the control.
2.4 Viable LAB counts
Viable cells were counted using the MRS plate counting method. One milliliter of each yogurt sample was diluted with 9 mL of sterile saline solution. After 2 to 3 consecutive suitable concentrations were diluted, 100 μL of solution was pipetted to evenly coat the MRS plates, which were subsequently incubated at 37°C for 36 h under anaerobic conditions.
2.5 Titratable acidity and organic acid analysis
Ten grams of yogurt sample was weighed and mixed with 20 mL of ultrapure water. The mixture was titrated with 0.1 M NaOH solution using phenolphthalein as an indicator. The titratable acidity (TA) was calculated based on the consumption of NaOH (Chen et al., 2024).
A total of 2.5 g of the sample was accurately weighed and centrifuged at 10,000 × g for 10 min, and then the supernatant was mixed with acetone at a 1:1 (v/v) ratio, subjected to vibration for 10 min, and centrifuged again. The supernatant was filtered through a 0.22 μm membrane. Organic acids were analyzed by a 1260 high-performance liquid chromatography (HPLC) system (Agilent Technology Co., Ltd., Santa Clara, CA, United States) equipped with a Hypersil GOLD column (250 mm × 4.6 mm, 5 μm). The mobile phases were as follows: A, 95% KH2PO4; B, acetonitrile; and C, 5% water. The flow rate was 0.8 mL/min, and the UV measurement wavelength was 210 nm. The column temperature was 35°C, and the injection sample volume was 20 μL.
2.6 Physical property analysis
Water-holding capacity (WHC) was determined according to the method of Rao et al. (2022): a certain amount of quinoa yogurt was weighed, which was recorded as m1, and centrifuged at 4,000 × g for 20 min. The supernatant was removed, and the weight of the sediment was recorded as m2. WHC was calculated as in Eq. (1):
The viscosity of the yogurt samples was measured using an NDJ-5S rotary viscometer (Shanghai Pingxuan Scientific Instrument Co., Ltd., Shanghai, China).
2.7 Sensory evaluation
Sensory evaluation was performed according to Abdeldaiem et al. (2023) with slight modifications. Ten students majoring in food science and engineering who had studied the “food sensory evaluation” were randomly selected for sensory evaluation of the yogurt from four aspects: color (25), texture (25), taste (25), and odor (25). The total score was 100. The experimental process was reviewed and authorized by the commission of the College of Food Science and Biological Engineering of Chengdu University.
2.8 Antioxidant activity determination
2.8.1 DPPH radical-scavenging ability
Five grams of sample was accurately weighed, and 1:9 (w/v) anhydrous ethanol solution was added. After mixing evenly, the mixture was centrifuged at 5,000 × g for 20 min, and the supernatant was obtained. One hundred microliters of the sample solution and 100 μL of DPPH (100 μmol/L) were mixed well in a 96-well plate and incubated in the dark at room temperature for 30 min. The absorbance was measured at 517 nm, and DPPH radical-scavenging activity was calculated according to Luo et al. (2023).
2.8.2 ABTS radical-scavenging ability
ABTS solution (7 mmol/L) and K2S2O8 (2.45 mmol/L) were mixed and incubated in the dark for 14 h. The mixture was diluted with phosphate buffer (0.2 mol/L, pH 7.4) until the absorbance at 734 nm reached 0.70 ± 0.02. Then, 100 μL of sample solution and 100 μL of ABTS solution were mixed well in a 96-well plate and incubated in the dark for 10 min at room temperature. The absorbance was recorded at 734 nm, and ABTS radical-scavenging activity was calculated according to Meng et al. (2022b).
2.9 Volatile compound analysis
The volatiles in yogurt were analyzed by solid-phase microextraction-gas chromatography-mass spectrometry (SPME-GC-MS) according to Chen et al. (2024) with slight modifications: 5.0 g of yogurt was accurately weighed into a 20-mL headspace injection vial, 20 μL of 2-methyl-3 heptanone (72 μg/mL) was added as the internal standard, and then the vial was sealed with a cap. After equilibrating in a water bath at 40°C for 15 min, a pretreated (250°C, 20 min) SPME fiber (DVB/CAR/PDMS) was inserted into the headspace vial to adsorb the volatiles in the sample headspace for 30 min. Desorption was conducted at 250°C for 5 min.
The volatiles were analyzed by a GCMS-QP2010 SE (Shimadzu, Kyoto, Japan). A stabilwax capillary column (30 m × 0.25 mm × 0.25 μm, Restek, Bellefonte, PA, United States) was used. The carrier gas was helium at a flow rate of 1.0 mL/min, the sample was manually split, and the temperature of the GC-MS injection port was 250°C. The heating program was as follows: an initial temperature of 30°C (held for 3 min), which was then increased to 90°C at a rate of 3°C/min (held for 1 min), increased to 120°C at a rate of 4°C/min (held for 1 min), and finally increased to 240°C at a rate of 6°C/min (held for 6 min). The MS instrument was operated in electron impact mode with an electron impact energy of 70 eV and an ion source temperature of 220°C. The mass spectrometer scan range was 35–500 m/z. Qualitative analysis of the volatile compounds were identified by matching the instrument’s NIST14.L spectral library to select substances with a match higher than 80%. The volatile compounds were semi-quantified analysis by determining the ratio of the peak area of a specific component to the peak area of an internal standard at a known concentration.
2.10 QPH separation and analysis
2.10.1 Ultrafiltration separation
Ultrafiltration separation of QPHs was performed according to the method of Wen et al. (2020) with slight modifications. Ultrafiltration membranes with molecular weights of 300 kDa, 10 kDa, and 1 kDa (Merck Millipore, Billerica, MA, United States) were used to filter hydrolyzed quinoa protein products. Finally, four ultrafiltration components with molecular weights of >300 kDa, 10–300 kDa, 1 kDa–10 kDa, and <1 kDa were prepared. The ultrafiltration components were lyophilized. The free radical-scavenging activities of DPPH and ABTS were measured to select the target components according to the process described in section 2.6. The components with the highest antioxidant activity were used for subsequent separation and purification.
2.10.2 Purification by gel filtration chromatography
The ultrafiltration fraction containing the highest antioxidant activity was further separated by a SephadexG-25 gel filtration column (12 mm × 80 cm). It was eluted with deionized water at a flow rate of 0.5 mL/min and monitored at 280 nm. The fractions of each peak were collected and lyophilized, and the free radical-scavenging activities of DPPH and ABTS were measured to select the target components according to the process described in section 2.6.
2.10.3 Identification of peptides by LC-MS/MS
The gel filtration fractions with the highest antioxidant activity were selected for LC-MS/MS separation and identification, which was performed on an EASY nLC-1200 system (Thermo, Waltham, MA, United States) coupled with a Q Exactive HF-X quadrupole orbitrap mass spectrometer (Thermo). Briefly, a C18 reversed-phase column (75 μm × 25 cm, Thermo) was equilibrated with solvent A (2% CAN with 0.1% formic acid) and solvent B (80% CAN with 0.1% formic acid). The peptides were eluted using the following gradient: 0–34 min, 5–23% B; 34–39 min, 23–29% B; 39–41 min, 29–38% B; 41–42 min, 38–48% B; 42–43 min, 48–100% B; and 43–60 min, 100–100% B. The flow rate of the sample was 300 nL/min. The Q Exactive HF-X instrument was operated in data-dependent acquisition mode (DDA) to automatically switch between full-scan MS and MS/MS acquisition.
Peptides were scored according to the method of Sheng et al. (2019) with slight modifications. Specifically, the score is based on four factors: the abundance of the MS/MS spectrum, sequencing confidence, deviation of peptide mass from theoretical values, and specific amino acid content. The total score of the selected sequence is based on the Eq. (2):
where A is the min–max linear normalized value of abundance in the MS/MS spectrum, which was mapped to 60–100; B is the min–max linear normalized value of sequencing confidence, which was mapped to 60–100; C is the min–max linear normalized value of the deviation from the theoretical value of the peptide mass, which was mapped to 60–100; and D is the min–max linear normalized value of the content of a particular amino acid (E, R, D, and G), which was also mapped to 80–100. The top 6 peptides with the highest scores were selected as the final identified antioxidant peptides.
2.11 Statistical analysis
All tests except peptide identification were conducted in triplicate, and the results were expressed as the mean ± standard deviation. Excel 2021 and one-way ANOVA with IBM SPSS 22.0 software (Armonk, NY, United States) were used for statistical analysis. Principal component analysis (PCA) and heatmaps were generated by Origin 2022 (OriginLab, Northampton, MA, United States).
3 Results and discussion
3.1 Effect of QPH addition on viable bacteria counts
The number of viable LAB in yogurt is an important index for evaluating the quality of yogurt (Feng et al., 2019). As shown in Figure 1A, compared with the control group, the addition of QPs and QPHs significantly promoted L. plantarum growth during yogurt fermentation. Although 1% QPH addition did not significantly increase the viable bacteria count compared to 2% QP addition, the viable bacteria count in the 2% QPH group increased from 4.2 × 107 CFU/mL to 1.21 × 109 CFU/mL, which was significantly greater than that in the 2% QP group. This finding suggests that QPH addition promotes the growth of L. plantarum, which is in line with the findings of Zhao et al. (2022), who reported that the addition of dairy bioactive peptides promotes the growth of probiotics by increasing free amino acids and small peptides.
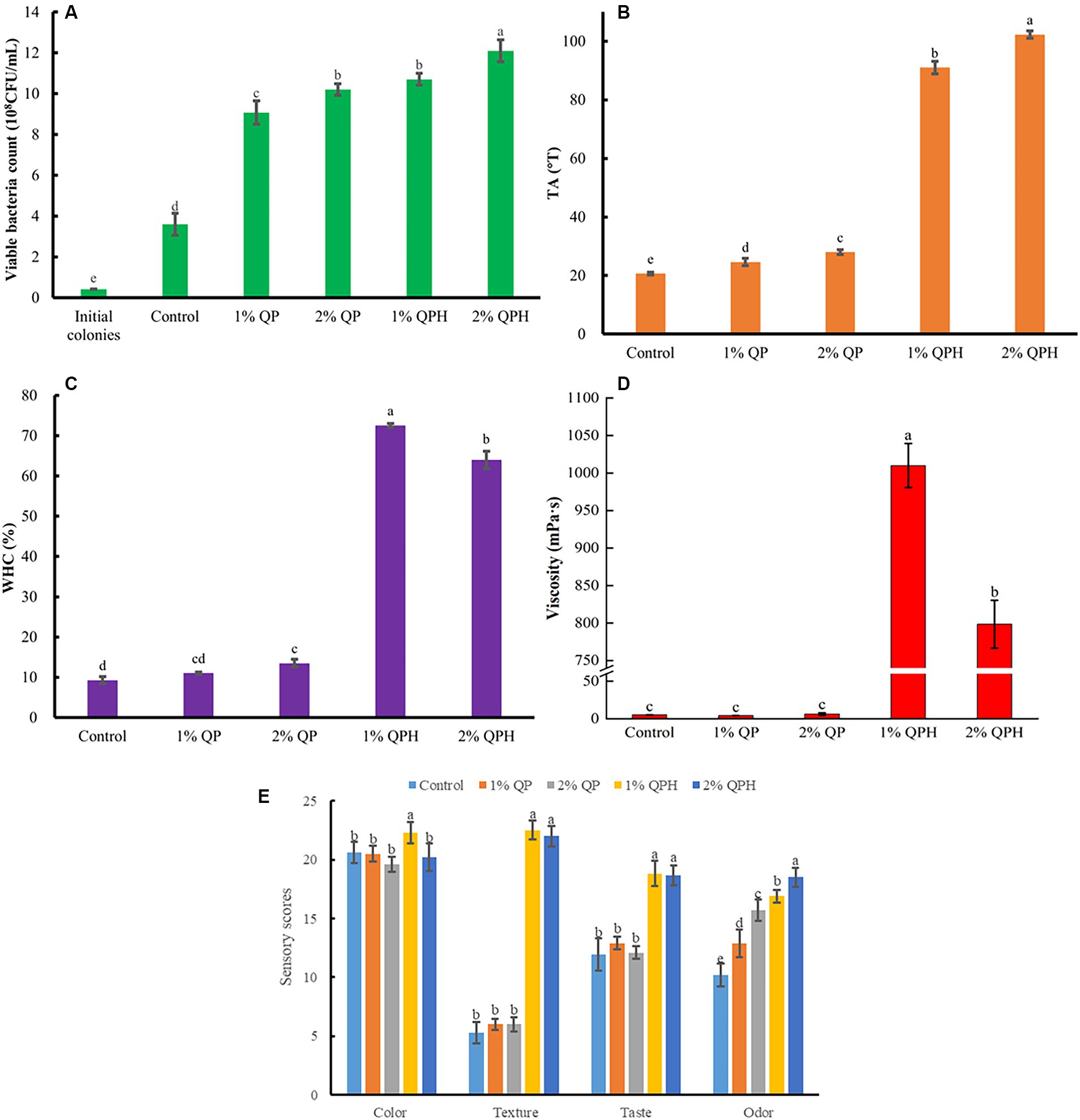
Figure 1. Effects of QP and QPH addition on the (A) cell viability, (B) titratable acidity (TA), (C) water-holding capacity (WHC), (D) viscosity, and (E) sensory scores of yogurts. The data are reported as the means ± standard deviations of three replicates. The different letters above the bars indicate significant differences (p < 0.05).
3.2 Effect of QPH addition on the titratable acidity of yogurt
TA is the main index used to judge the quality of yogurt, which is mainly related to the degree of fermentation of probiotics and sugars in the product (Medina et al., 2023). With increasing yogurt fermentation time, organic acids such as lactic acid produced by LAB further accumulated, reflecting an increase in product acidity. As shown in Figure 1B, the QP group had greater TA values than the control groups (p < 0.05). The TA in 1% QPH and 2% QPH group reached 91.00 and 102.33, respectively, which is 4.40- and 4.95-fold higher than that of control group. The change trend of TA was consistent with that of the viable bacteria count, possibly because QPHs provide more nitrogen sources (amino acids and peptides) for L. plantarum, which promotes the growth and metabolism of L. plantarum, thereby increasing the TA of yogurt. Similarly, Gheshlaghi et al. (2021) also reported that sturgeon skin gelatin hydrolysate contains a high percentage of small peptides, which promote the growth of LAB, ultimately increasing the TA.
3.3 Effect of QPH addition on the organic acids of yogurt
Organic acids are extremely important components of yogurt quality, aroma, and safety. As shown in Table 1, oxalic acid and citric acid were detected in the control sample and the 1% QPH sample. The addition of 2% QPs promoted only malic acid production. QPH addition obviously increased organic acid production regardless of the type or content of organic acids. Lactic acid and citric acid are the main organic acids in QPH-supplemented yogurt, and lactic acid production is important for flavor development in dairy products (Ndhlala et al., 2022). Citric acid is an organic acid found at high levels in fresh milk and is one of the reasons for the refreshing taste of fresh milk (Garavand et al., 2023); it has been reported to be the main precursor for the production of diacetyl and acetoin, which are used by LAB during milk fermentation to impart the desired flavor to yogurt (Güzel-Seydim et al., 2000). The above results suggest that QPH addition effectively enhances the metabolism of L. plantarum, which results in the formation of large amounts of lactic acid and citric acid and small amounts of malic, acetic, and formic acids, thus giving yogurt its unique flavor and aroma.
3.4 Effect of QPH addition on the physical properties of yogurt
The WHC of yogurt refers to the ability of yogurt to retain all or part of its own water, which reflects the density of the gel network and the texture of the yogurt (Qu et al., 2021). As shown in Figure 1C, compared with that of the control group, the WHCs of the groups supplemented with 1% QPHs and 2% QPHs significantly increased, and the WHC of the 1% QPH group was greater than that of the 2% QPH group. Previously, Wang et al. (2017) reported that excessive addition of silkworm pupae peptides beyond a certain concentration decreased the WHC of yogurt, which may be related to extensive rearrangement of the gel network.
The viscosity of yogurt is an important indicator of its quality. As shown in Figure 1D, compared with that in the control group, QP addition did not significantly improve the viscosity of yogurt. However, the addition of 1% QPHs and 2% QPHs significantly increased yogurt viscosity by 190.9- and 160.0-fold, respectively, compared to that of the control sample. Varedesara et al. (2021) also showed that the addition of grapeseed protein hydrolysate could increase yogurt viscosity. In addition, the viscosity of yogurt with 1% QPHs was greater than that of yogurt with 2% QPHs. This result may be due to the greater number of viable bacteria in the 2% QPH group, which ultimately led to a decrease in total solid content, weakened protein interactions, and a decrease in yogurt viscosity (Hu et al., 2020), which is consistent with the WHC trend.
3.5 Sensory evaluation
The sensory scores of the yogurts are shown in Figure 1E. Overall, the addition of QPs and QPHs had no effect on the color of the yogurts. However, the yogurt with QPH addition scored the highest on texture because it has higher solidification characteristics, which is consistent with the WHC and viscosity results. In addition, the taste and odor scores of yogurts with QPH addition (especially 2% QPH group) were higher than that of the other yogurts, indicating that the addition of QPH could improve the sensory quality of yogurt and makes it more appealing to consumers. Similarly, Chi et al. (2019) reported that the addition of papain egg white hydrolysate to yak milk positively affected the sensory properties of the resulting yogurt, and the addition of moderate amounts of papain egg white hydrolysate even improved the sensory properties of yak milk yogurt.
3.6 Effect of QPH addition on the antioxidant activities of yogurt
Compared with the control group, the QPH group had a greater ability to scavenge DPPH and ABTS radicals (Figure 2), which may be due to the strong radical-scavenging ability of various bioactive components contained in QPHs. Many previous studies have shown that adding substances with bioactive ingredients can improve the antioxidant activity of yogurt. For example, Li et al. (2023) reported that highland barley hydrolysate could improve the antioxidant activities of soy-based yogurt. However, the DPPH radical-scavenging ability of the 1% QPH-supplemented yogurt was significantly greater than that of the 2% QPH-supplemented yogurt (Figure 2A), which may be attributed to the changes in the TA of the yogurts after fermentation, and the amino acid content of the 2% QPH-supplemented yogurt may have changed, which may have affected the cohesiveness of the yogurt, resulting in lower antioxidant activity (Mashayekh et al., 2023).
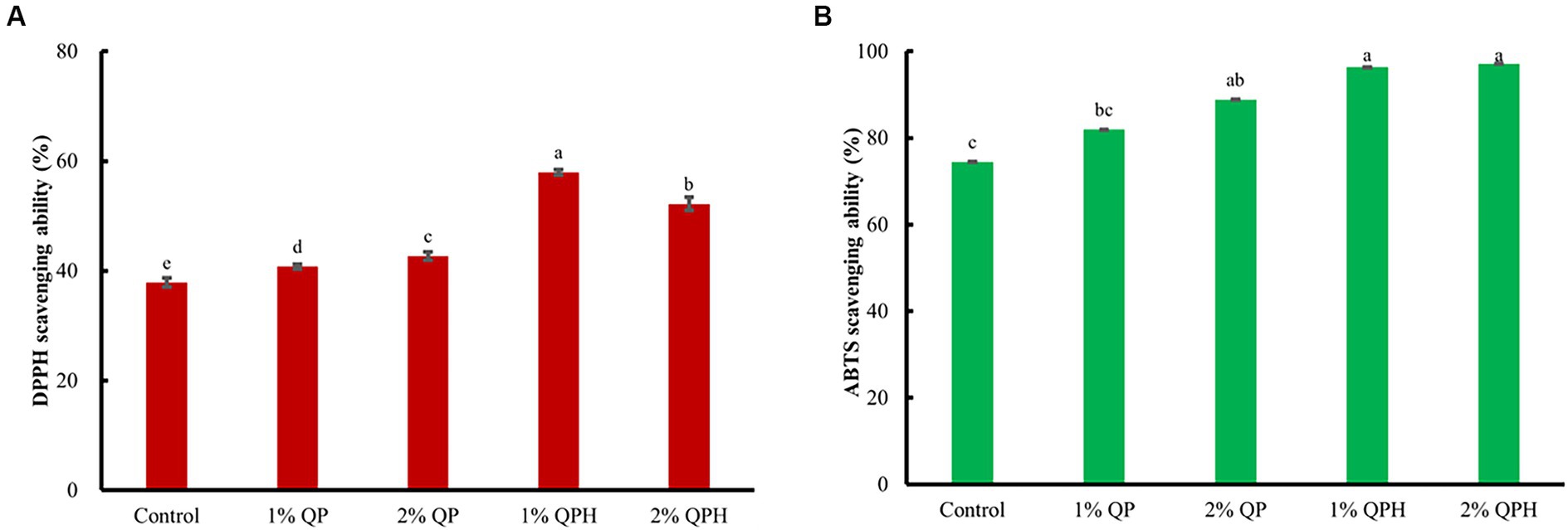
Figure 2. Effects of QP and QPH addition on the (A) DPPH-scavenging ability and (B) ABTS-scavenging ability of yogurts. The data are reported as the means ± standard deviations of three replicates. The different letters above the bars indicate significant differences (p < 0.05).
3.7 Effect of QPH addition on volatile compounds in yogurt
According to Figure 3 and Table 2, 83 volatile compounds were identified, including 15 acids, 13 alcohols, 5 aldehydes, 15 esters, 21 ketones, 1 phenol, and 13 hydrocarbons (Figure 3A). PCA was applied to assess differences in volatile compounds in different samples, and the results are shown in Figure 3B. From the score plots, the constructed PCA plots explained 77.8% of the total variance, 49.9% of which came from the first principal component (PC1), while 27.9% came from the second principal component (PC2). All samples occupied relatively independent regions in the PCA distribution space, and the yogurt of the 2% QP and 2% QPH groups was far from the yogurt of the control group, indicating that the added substances caused significant changes in volatile substances (Wang et al., 2023). In addition, the distance between the yogurts of the 1% QP and 1% QPH groups was shorter than that of the control group, indicating a small difference in volatile compounds between the samples. In addition, the heatmap (Figure 3C) shows the differences in volatile components between the experimental groups, with the yogurts in the 2% QP and 2% QPH groups clearly showing more volatile compounds.
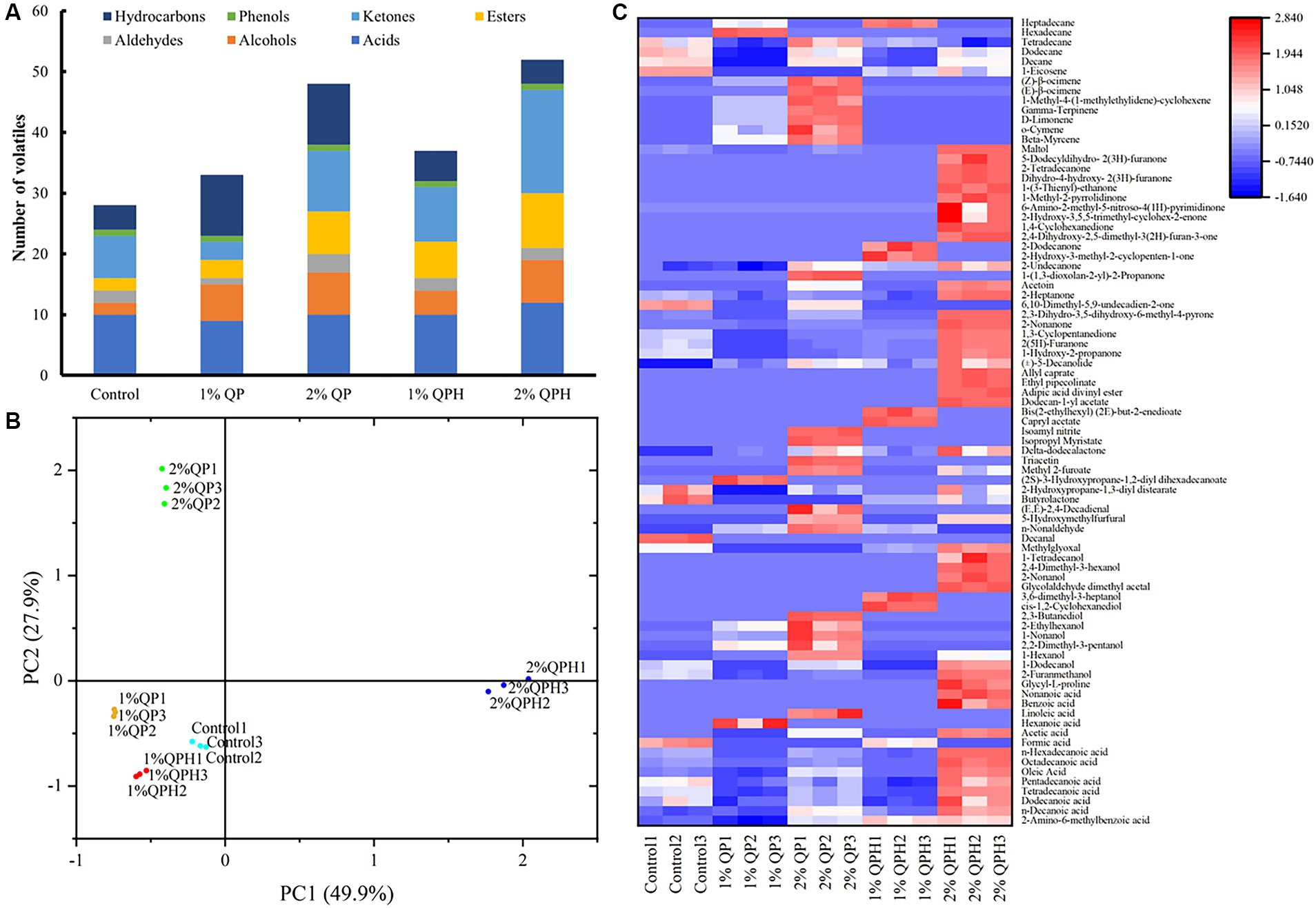
Figure 3. Effects of QP and QPH addition on the volatile components of yogurt. (A) The number of volatiles, (B) principal component analysis (PCA), and (C) heatmap analysis of the volatile components.
Acids are key volatile substances in yogurt. Hexanoic acid is the main source of flavor and function in fermented yogurt (Dan et al., 2017). Hexanoic acid was detected in the 1% QP group, which contributed spicy, rancid, and flowery flavors to the yogurt (Liu et al., 2022). Benzoic acid and nonanoic acid, which have a urine flavor and a fruity flavor (Fan et al., 2022), respectively, were detected in the 2% QPH group (Table 2). The types of alcohols, such as 1-hexanol, increased in the QP and QPH groups with increasing QP and QPH concentrations, which gave the yogurt a floral green flavor (Wang et al., 2023). In addition, 1-nonanol, 2-ethylhexanol and 2,3-butanediol were detected in the 1% QP and 2% QP groups, which had citrus flavors, rose and green flavors, and buttery flavors, respectively (Chen et al., 2017; Xu et al., 2022); these substances provided good flavors to yogurt. Aldehydes are mainly formed by the oxidation of unsaturated fatty acids. n-Nonaldehyde was detected in the QP and QPH groups but not in the control group (Table 2), which imparted fat, citrus, and green flavors to the yogurts. Interestingly, we detected only decanal, which has fatty flavors (Chen et al., 2017), in the control group, suggesting that the addition of QPs or QPHs may reduce some undesirable odors in yogurt. This may be one of the reasons for the high yogurt odor scores with added QPHs.
Esters are important flavor substances in yogurt. Two typical flavor substances of the yogurt, delta-dodecalactone and (±)-5-decanolide, were detected in the QP- and QPH-supplemented yogurts, providing the yogurts with pleasant fruit, sweet, and coconut flavors (Wang et al., 2021). In addition, capryl acetate, dodecan-1-yl acetate and bis(2-ethylhexyl) (2E)-but-2-enedioate were detected in yogurt supplemented with QPHs (Table 2), which enhanced the taste and flavor of the yogurt. Ketones often play an important role in the formation of fermented yogurt flavors. Acetoin is an important flavor substance in yogurt that give yogurt the desirable flavor (Chen et al., 2017). Acetoin provides a creamy butter flavor in yogurt (Huang et al., 2020) and was detected in yogurt supplemented with 2% QPs and 2% QPHs, with the acetoin content in the 2% QPH group showing the highest acetoin content (27.65 ± 0.81 μg/mL) among all samples. In addition, the content of 2-nonanone, which contributes to the floral, fruity, and peach flavor of dairy products (Cheng, 2010), in the 2% QPH-supplemented yogurt was 242.37 ± 4.26 μg/mL, which was 5.53-, 8.20-, 4.71-, and 5.80-fold greater than those in the control, 1% QP-supplemented, 2% QP-supplemented, and 1% QPH-supplemented yogurts, respectively (Table 2), which is the main reasons for the high sensory score of the 2% QPH yogurt. Studies have shown that the odor of 2-heptanone in dairy products is musty, sweet, moldy, and similar to varnish (Dan et al., 2022), and the 2-heptanone contents in the QP-supplemented and 1% QPH-supplemented yogurts were significantly decreased. However, the 2-heptanone content was significantly increased in the 2% QPH-supplemented yogurt. In addition, the yogurts supplemented with 2% QPs and 2% QPHs showed significant increases in 2-undecanone compared to the control group yogurt (Table 2), indicating that the yogurts had a waxy and fruity flavor (Sfakianakis and Tzia, 2017). In addition, maltol was detected in all the samples, and the 2% QPH-supplemented yogurt had the highest maltol content, which imparted a caramel flavor to the yogurt.
3.8 QPH classification
The above results revealed that QPHs significantly promoted L. plantarum growth and fermentation, improved antioxidant properties and flavor of the L. plantarum fermented yogurt. Previous studies have indicated that the higher the antioxidant activity of protein hydrolysates is, the greater their ability to promote the fermentation of probiotics (Noor et al., 2022; Meng et al., 2022b). Therefore, using antioxidant performance as an indicator, this study further separated and analyzed the composition of QPHs. After ultrafiltration, QPHs were divided into four components with different molecular weights: QPH-I (<1 kDa), QPH-II (1–10 kDa), QPH-III (10–300 kDa), and QPH-IV (>300 kDa). QPH-I, QPH-II, and QPH-IV had the highest ABTS radical-scavenging abilities at 97.08 ± 1.18, 98.65 ± 0.58, 98.48 ± 0.98%, respectively, but QPH-II showed the highest DPPH radical-scavenging ability at 68.49% ± 2.36% (Figure 4A). Some studies have shown that the antioxidant activity of peptides is related to their molecular weight, and low-molecular-weight peptides may have greater antioxidant activity (Wen et al., 2019; Ren et al., 2023). Therefore, the ultrafiltration fraction of the QPH-II group was selected for further separation and purification.
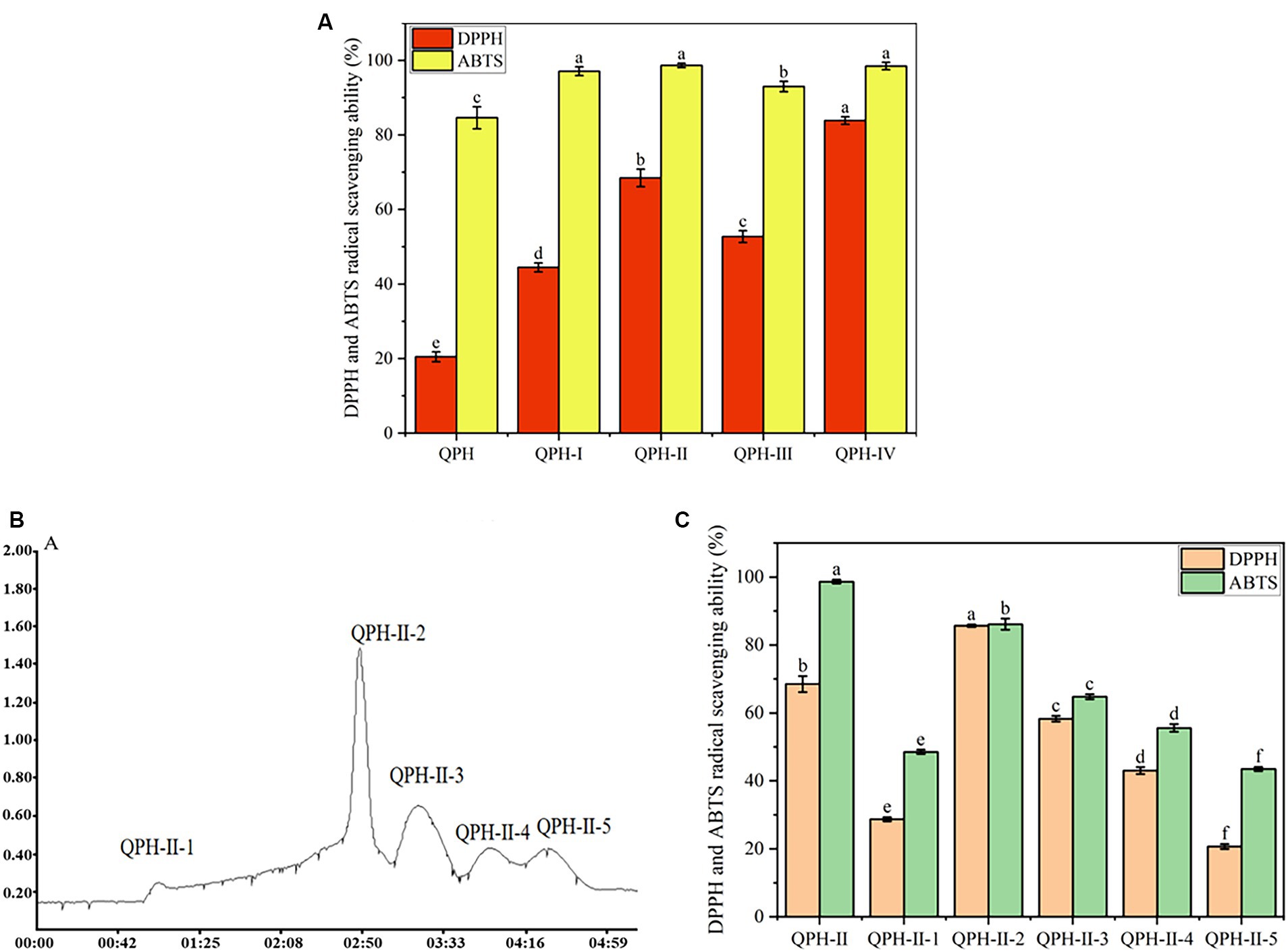
Figure 4. Antioxidant activities of the QPH fractions. (A) The DPPH and ABTS radical-scavenging abilities of the QPH ultrafiltration fractions, (B) Sephadex G-25 gel filtration chromatography for the QPH-II fraction, and (C) the DPPH and ABTS radical-scavenging abilities of the QPH components separated by gel chromatography. The data are reported as the means ± standard deviations of three replicates. The different letters above the bars indicate significant differences (p < 0.05).
After isolation and purification by gel chromatography, 5 subcomponents were obtained, which were named QPH-II-1 to QPH-II-5. As shown in Figure 4B, the peak of the QPH-II-2 fraction is the highest, and the span of the peak is the narrowest, indicating that QPH-II-2 has the highest component content and the most concentrated molecular weight. The ABTS radical-scavenging ability significantly decreased after gel chromatography separation, but in the QPH-II-2 fraction, the DPPH radical-scavenging ability significantly increased (Figure 4C); therefore, this fraction was selected for LC–MS/MS analysis.
3.9 Identification of QPH-II-2 fractions
The QPH-II-2 fractions were identified by LC-MS/MS. A total of 1,538 peptides with molecular weights ranging from 698.354 to 3681.886 were detected (Supplementary Table S1). The peptides were selected based on the abundance of MS/MS spectra of the peptides, peptide confidence, peptide mass deviation from theoretical values, and specific amino acid content using Eq. 2. Antioxidant peptides have been reported to usually be small molecules containing 3–30 amino acids (Tyagi et al., 2023), and the amino acid compositions of peptides can affect their antioxidant properties. In particular, peptides containing hydrophobic amino acids or aromatic amino acids may have greater antioxidant properties, and a high proportion of hydrophobic amino acids may have a positive effect on their antioxidant activity (Guan et al., 2018; Liu et al., 2023). As shown in Supplementary Table S1, almost all peptides contained hydrophobic amino acids (Tyr, Phe, Val, Leu, Ile, Ala, Pro, and Met), and the proportion of hydrophobic amino acids in single peptides was greater than 50% among the 647 peptides. Hydrophobic amino acid sequences may be able to prevent oxidation by making protons available to free radicals (Najafian and Babji, 2018). In addition to amino acid composition, the N-terminal and C-terminal sequence properties of peptide sequences, especially those containing hydrophobic amino acid residues, are related to antioxidant peptide activity (Zhang J. et al., 2020; Zhang S. S. et al., 2020). In this study, 60.79% of the peptides contained hydrophobic amino acids at the N-terminus or the C-terminus (Supplementary Table S1), which may increase the antioxidant activity of the peptides. However, this study also has limitations; that is, too many peptides were present in the QPH-II-2 fraction. To further study the antioxidant and fermentation-promoting properties of the peptides, further separation, such as reversed-phase HPLC, of the QPH-II-2 fraction is necessary.
4 Conclusion
In this study, the effect of quinoa protein hydrolysates (QPHs) on the quality of yogurt fermented by Lactobacillus plantarum was evaluated. The addition of QPHs could significantly promote bacterial growth and the production of organic acids such as lactic acid and citric acid. Subsequently, coagulation was promoted, and the water-holding capacity and viscosity of the yogurt were thus improved, which were approximately 6- and 150-fold of the control group. QPH addition also increased the antioxidant capacity, but the DPPH radical-scavenging ability of the 1% QPH-supplemented yogurt was slightly greater than that of the 2% QPH-supplemented yogurt. Both the types and quantities of volatile flavor compounds in QPH-supplemented yogurt were increased from 28 in the control group to 37 and 52 in 1% QPH-supplemented and 2% QPH-supplemented group, respectively, and the levels of some aroma components, such as nonanoic acid and maltol, were significantly increased, but the levels of some undesirable volatiles in yogurt, such as decanal, were decreased. In addition, there were 1,538 peptides were identified in QPHs, and further analysis indicated that almost all peptides contained hydrophobic amino acids, and 60.79% of the peptides contained hydrophobic amino acids at the N-terminus or the C-terminus, which is a possible reason why QPHs have strong antioxidant properties.
Data availability statement
The original contributions presented in the study are included in the article/Supplementary material, further inquiries can be directed to the corresponding authors.
Author contributions
A-XC: Data curation, Validation, Writing – original draft. F-BM: Conceptualization, Methodology, Writing – original draft. J-JL: Formal analysis, Resources, Writing – original draft. X-CC: Investigation, Project administration, Writing – original draft. Y-CL: Funding acquisition, Supervision, Writing – review & editing. L-SJ: Software, Writing – review & editing.
Funding
The author(s) declare that financial support was received for the research, authorship, and/or publication of this article. This work was supported by Sichuan Science and Technology Program (2022YFS0514 and 2023YFSY0050).
Conflict of interest
The authors declare that the research was conducted in the absence of any commercial or financial relationships that could be construed as a potential conflict of interest.
Publisher’s note
All claims expressed in this article are solely those of the authors and do not necessarily represent those of their affiliated organizations, or those of the publisher, the editors and the reviewers. Any product that may be evaluated in this article, or claim that may be made by its manufacturer, is not guaranteed or endorsed by the publisher.
Supplementary material
The Supplementary material for this article can be found online at: https://www.frontiersin.org/articles/10.3389/fsufs.2024.1432631/full#supplementary-material
References
Abbasi, S., Moslehishad, M., and Salami, M. (2022). Antioxidant and alpha-glucosidase enzyme inhibitory properties of hydrolyzed protein and bioactive peptides of quinoa. Int. J. Biol. Macromol. 213, 602–609. doi: 10.1016/j.ijbiomac.2022.05.189
Abdeldaiem, A. M., Ali, A. H., Shah, N., Ayyash, M., and Mousa, A. H. (2023). Physicochemical analysis, rheological properties, and sensory evaluation of yogurt drink supplemented with roasted barley powder. LWT 173:114319. doi: 10.1016/j.lwt.2022.114319
Al-Bukhaiti, W. Q., Al-Dalali, S., Li, H. X., Yao, L. Y., Abed, S. M., Zhao, L. Y., et al. (2023). Identification and in vitro characterization of novel antidiabetic peptides released enzymatically from peanut protein. Plant Foods Hum. Nutr. 79, 66–72. doi: 10.1007/s11130-023-01118-x
Ashaolu, T. J. (2020). Health applications of soy protein hydrolysates. Int. J. Pept. Res. Ther. 26, 2333–2343. doi: 10.1007/s10989-020-10018-6
Chen, X.-C., Meng, F.-B., Tian, H.-L., Chen, A.-X., Wang, X.-B., and Li, Y.-C. (2024). Dynamic changes in metabolomics and flavoromics during industrial scale fermentation of Chinese traditional soy sauce. Food Biosci. 59:103940. doi: 10.1016/j.fbio.2024.103940
Chen, C., Zhao, S. S., Hao, G. F., Yu, H. Y., Tian, H. X., and Zhao, G. Z. (2017). Role of lactic acid bacteria on the yogurt flavour: a review. Int. J. Food Prop. 20, S316–S330. doi: 10.1080/10942912.2017.1295988
Cheng, H. (2010). Volatile flavor compounds in yogurt: a review. Crit. Rev. Food Sci. Nutr. 50, 938–950. doi: 10.1080/10408390903044081
Chi, F., Liu, T., Liu, L., Tan, Z., Gu, X., Yang, L., et al. (2019). Optimization of antioxidant hydrolysate produced from Tibetan egg white with papain and its application in yak milk yogurt. Molecules 25:109. doi: 10.3390/molecules25010109
Choi, H. S., Seong, H., Kim, S.-A., Song, Y., Sim, E. Y., Kang, H., et al. (2023). Lysine-fortified rice germ yogurt fermented with Lactiplantibacillus plantarum JSA 22 and its beneficial health effects. J. Funct. Foods 109:105787. doi: 10.1016/j.jff.2023.105787
Dan, T., Hu, H., Li, T., Dai, A., He, B., and Wang, Y. (2022). Screening of mixed-species starter cultures for increasing flavour during fermentation of milk. Int. Dairy J. 135:105473. doi: 10.1016/j.idairyj.2022.105473
Dan, T., Ren, W., Liu, Y., Tian, J., Chen, H., Li, T., et al. (2019). Volatile flavor compounds profile and fermentation characteristics of milk fermented by Lactobacillus delbrueckii subsp. bulgaricus. Front. Microbiol. 10:2183. doi: 10.3389/fmicb.2019.02183
Dan, T., Wang, D., Jin, R. L., Zhang, H. P., Zhou, T. T., and Sun, T. S. (2017). Characterization of volatile compounds in fermented milk using solid-phase microextraction methods coupled with gas chromatography-mass spectrometry. J. Dairy Sci. 100, 2488–2500. doi: 10.3168/jds.2016-11528
Fan, X., Li, X., Zhang, T., Guo, Y., Shi, Z., Wu, Z., et al. (2022). Novel millet-based flavored yogurt enriched with superoxide dismutase. Front. Nutr. 8:791886. doi: 10.3389/fnut.2021.791886
Feng, C., Wang, B., Zhao, A., Wei, L., Shao, Y., Wang, Y., et al. (2019). Quality characteristics and antioxidant activities of goat milk yogurt with added jujube pulp. Food Chem. 277, 238–245. doi: 10.1016/j.foodchem.2018.10.104
Garavand, F., Daly, D. F. M., and Gómez-Mascaraque, L. G. (2023). The consequence of supplementing with synbiotic systems on free amino acids, free fatty acids, organic acids, and some stability indexes of fermented milk. Int. Dairy J. 137:105477. doi: 10.1016/j.idairyj.2022.105477
Ghelich, S., Ariaii, P., and Ahmadi, M. (2022). Evaluation of functional properties of wheat germ protein hydrolysates and its effect on physicochemical properties of frozen yogurt. Int. J. Pept. Res. Ther. 28:69. doi: 10.1007/s10989-022-10378-1
Gheshlaghi, S. P., Khaledabad, M. A., Nikoo, M., Regenstein, J. M., and Gavlighi, H. A. (2021). Impact of sturgeon gelatin hydrolysates (SGH) on physicochemical and microbiological properties of fat-free set-type yogurt. LWT 148:111665. doi: 10.1016/j.lwt.2021.111665
Guan, H., Diao, X., Jiang, F., Han, J., and Kong, B. (2018). The enzymatic hydrolysis of soy protein isolate by Corolase PP under high hydrostatic pressure and its effect on bioactivity and characteristics of hydrolysates. Food Chem. 245, 89–96. doi: 10.1016/j.foodchem.2017.08.081
Güzel-Seydim, Z. B., Seydim, A. C., Greene, A. K., and Bodine, A. B. (2000). Determination of organic acids and volatile flavor substances in kefir during fermentation. J. Food Compos. Anal. 13, 35–43. doi: 10.1006/jfca.1999.0842
Hu, Y., Li, Y., and Liu, X. Q. (2020). Soybean peptides promote yoghurt fermentation and quality. Biotechnol. Lett. 42, 1927–1937. doi: 10.1007/s10529-020-02912-2
Huang, K., Liu, Y., Zhang, Y., Cao, H., Luo, D.-K., Yi, C., et al. (2022). Formulation of plant-based yoghurt from soybean and quinoa and evaluation of physicochemical, rheological, sensory and functional properties. Food Biosci. 49:101831. doi: 10.1016/j.fbio.2022.101831
Huang, Y.-Y., Yu, J.-J., Zhou, Q.-Y., Sun, L.-N., Liu, D.-M., and Liang, M.-H. (2020). Preparation of yogurt-flavored bases by mixed lactic acid bacteria with the addition of lipase. LWT 131:109577. doi: 10.1016/j.lwt.2020.109577
Li, Y. C., Du, W., Meng, F. B., Rao, J. W., Liu, D. Y., and Peng, L. X. (2021). Tartary buckwheat protein hydrolysates enhance the salt tolerance of the soy sauce fermentation yeast Zygosaccharomyces rouxii. Food Chem. 342:128382. doi: 10.1016/j.foodchem.2020.128382
Li, K., Duan, Z., Zhang, J., and Cui, H. (2023). Growth kinetics, metabolomics changes, and antioxidant activity of probiotics in fermented highland barley-based yogurt. LWT 173:114239. doi: 10.1016/j.lwt.2022.114239
Liu, W., Pu, X., Sun, J., Shi, X., Cheng, W., and Wang, B. (2022). Effect of Lactobacillus plantarum on functional characteristics and flavor profile of fermented walnut milk. LWT 160:113254. doi: 10.1016/j.lwt.2022.113254
Liu, H., Sun, H.-N., Zhang, M., Mu, T.-H., and Khan, N. M. (2023). Production, identification and characterization of antioxidant peptides from potato protein by energy-divergent and gathered ultrasound assisted enzymatic hydrolysis. Food Chem. 405:134873. doi: 10.1016/j.foodchem.2022.134873
López, D. N., Galante, M., Robson, M., Boeris, V., and Spelzini, D. (2018). Amaranth, quinoa and chia protein isolates: physicochemical and structural properties. Int. J. Biol. Macromol. 109, 152–159. doi: 10.1016/j.ijbiomac.2017.12.080
Luo, Y., Li, Y.-C., Wang, M., Zhou, L., Meng, F.-B., and Jiang, L.-S. (2023). Effects of grafting methods and raw materials on the physicochemical properties and biological activities of phenolic acids grafted oat β-glucan. Food Res. Int. 173:113250. doi: 10.1016/j.foodres.2023.113250
Mashayekh, F., Pourahmad, R., Eshaghi, M. R., and Akbari-Adergani, B. (2023). Improving effect of soy whey-derived peptide on the quality characteristics of functional yogurt. Food Sci. Nutr. 11, 3287–3296. doi: 10.1002/fsn3.3312
Medina, L., Aleman, R. S., Cedillos, R., Aryana, K., Olson, D. W., Marcia, J., et al. (2023). Effects of carao (Cassia grandis L.) on physico-chemical, microbiological and rheological characteristics of yogurt. LWT 183:114891. doi: 10.1016/j.lwt.2023.114891
Meng, F.-B., Lei, Y.-T., Li, Q.-Z., Li, Y.-C., Deng, Y., and Liu, D.-Y. (2022a). Effect of Lactobacillus plantarum and Lactobacillus acidophilus fermentation on antioxidant activity and metabolomic profiles of loquat juice. LWT 171:114104. doi: 10.1016/j.lwt.2022.114104
Meng, F.-B., Zhou, L., Li, J.-J., Li, Y.-C., Wang, M., Zou, L.-H., et al. (2022b). The combined effect of protein hydrolysis and Lactobacillus plantarum fermentation on antioxidant activity and metabolomic profiles of quinoa beverage. Food Res. Int. 157:111416. doi: 10.1016/j.foodres.2022.111416
Najafian, L., and Babji, A. S. (2018). Fractionation and identification of novel antioxidant peptides from fermented fish (pekasam). J. Food Meas. Charact. 12, 2174–2183. doi: 10.1007/s11694-018-9833-1
Ndhlala, A. R., Kavaz Yüksel, A., and Yüksel, M. (2022). Nutritional supplementation of yogurt with Jerusalem artichoke tubers: organic acid profiles and quality parameters. Plants 11:3086. doi: 10.3390/plants11223086
Noor, H. S. M., Ariff, R. M., Chang, L. S., Chai, X. Y., Tan, H. Y., Daud, N. A., et al. (2022). Enzymatic recovery of glycopeptides from different industrial grades edible bird’s nest and its by-products: nutrient, probiotic and antioxidant activities, and physicochemical characteristics. Food Sci. Human Wellness 11, 1555–1564. doi: 10.1016/j.fshw.2022.06.013
Qu, X., Nazarenko, Y., Yang, W., Nie, Y., Zhang, Y., and Li, B. (2021). Effect of oat β-glucan on the rheological characteristics and microstructure of set-type yogurt. Molecules 26:4752. doi: 10.3390/molecules26164752
Rao, J.-W., Meng, F.-B., Li, Y.-C., Chen, W.-J., Liu, D.-Y., and Zhang, J.-M. (2022). Effect of cooking methods on the edible, nutritive qualities and volatile flavor compounds of rabbit meat. J. Sci. Food Agric. 102, 4218–4228. doi: 10.1002/jsfa.11773
Ren, L.-K., Fan, J., Yang, Y., Liu, X.-F., Wang, B., Bian, X., et al. (2023). Identification, in silico selection, and mechanism study of novel antioxidant peptides derived from the rice bran protein hydrolysates. Food Chem. 408:135230. doi: 10.1016/j.foodchem.2022.135230
Sfakianakis, P., and Tzia, C. (2017). Flavour profiling by gas chromatography-mass spectrometry and sensory analysis of yoghurt derived from ultrasonicated and homogenised milk. Int. Dairy J. 75, 120–128. doi: 10.1016/j.idairyj.2017.08.003
Sheng, J., Yang, X., Chen, J., Peng, T., Yin, X., Liu, W., et al. (2019). Antioxidative effects and mechanism study of bioactive peptides from defatted walnut (Juglans regia L.) meal hydrolysate. J. Agric. Food Chem. 67, 3305–3312. doi: 10.1021/acs.jafc.8b05722
Torres-Fuentes, C., Contreras, M., Recio, I., Alaiz, M., and Vioque, J. (2015). Identification and characterization of antioxidant peptides from chickpea protein hydrolysates. Food Chem. 180, 194–202. doi: 10.1016/j.foodchem.2015.02.046
Tyagi, A., Chelliah, R., Banan-Mwine Daliri, E., Sultan, G., Madar, I. H., Kim, N.-H., et al. (2023). Antioxidant activities of novel peptides from Limosilactobacillus reuteri fermented brown rice: a combined in vitro and in silico study. Food Chem. 404:134747. doi: 10.1016/j.foodchem.2022.134747
Varedesara, M. S., Ariaii, P., and Hesari, J. (2021). The effect of grape seed protein hydrolysate on the properties of stirred yogurt and viability of Lactobacillus casei in it. Food Sci. Nutr. 9, 2180–2190. doi: 10.1002/fsn3.2188
Vazquez-Luna, A., Cortés, V. P., Carmona, F. F., and Díaz-Sobac, R. (2019). Quinoa leaf as a nutritional alternative. Cienc. Investig. Agrar. 46, 137–143. doi: 10.7764/rcia.v46i2.2098
Wang, X., Kong, X., Zhang, C., Hua, Y., Chen, Y., and Li, X. (2023). Comparison of physicochemical properties and volatile flavor compounds of plant-based yoghurt and dairy yoghurt. Food Res. Int. 164:112375. doi: 10.1016/j.foodres.2022.112375
Wang, W., Wang, N., Liu, C., and Jin, J. (2017). Effect of silkworm pupae peptide on the fermentation and quality of yogurt. J. Food Process. Preserv. 41:e12893. doi: 10.1111/jfpp.12893
Wang, J., Yang, Z. J., Wang, Y. D., Cao, Y. P., Wang, B., and Liu, Y. (2021). The key aroma compounds and sensory characteristics of commercial Cheddar cheeses. J. Dairy Sci. 104, 7555–7571. doi: 10.3168/jds.2020-19992
Wen, C., Zhang, J., Feng, Y., Duan, Y., Ma, H., and Zhang, H. (2020). Purification and identification of novel antioxidant peptides from watermelon seed protein hydrolysates and their cytoprotective effects on H2O2-induced oxidative stress. Food Chem. 327:127059. doi: 10.1016/j.foodchem.2020.127059
Wen, C., Zhang, J., Zhang, H., Duan, Y., and Ma, H. (2019). Effects of divergent ultrasound pretreatment on the structure of watermelon seed protein and the antioxidant activity of its hydrolysates. Food Chem. 299:125165. doi: 10.1016/j.foodchem.2019.125165
Wongsa, P., Yuenyongrattanakorn, K., Pongvachirint, W., and Auntalarok, A. (2022). Improving anti-hypertensive properties of plant-based alternatives to yogurt fortified with rice protein hydrolysate. Heliyon 8:e11087. doi: 10.1016/j.heliyon.2022.e11087
Xu, J., Xu, X., Yuan, Z., Hua, D., Yan, Y., Bai, M., et al. (2022). Effect of hemp protein on the physicochemical properties and flavor components of plant-based yogurt. LWT 172:114145. doi: 10.1016/j.lwt.2022.114145
Yi, L., Min, J. T., Jun, C. L., Long, H. X., Khoo, H. E., Ying, Z. J., et al. (2023). Buffalo yogurt fermented with commercial starter and Lactobacillus plantarum originating from breast milk lowered blood pressure in pregnant hypertensive rats. J. Dairy Sci. 107, 62–73. doi: 10.3168/jds.2023-23566
Zhang, J., Du, H., Zhang, G., Kong, F., Hu, Y., Xiong, S., et al. (2020). Identification and characterization of novel antioxidant peptides from crucian carp (Carassius auratus) cooking juice released in simulated gastrointestinal digestion by UPLC-MS/MS and in silico analysis. J. Chromatogr. B 1136:121893. doi: 10.1016/j.jchromb.2019.121893
Zhang, S. S., Xu, Z. S., Qin, L. H., and Kong, J. (2020). Low-sugar yogurt making by the co-cultivation of Lactobacillus plantarum WCFS1 with yogurt starter cultures. J. Dairy Sci. 103, 3045–3054. doi: 10.3168/jds.2019-17347
Zhang, J., Zhao, X., Jiang, Y., Zhao, W., Guo, T., Cao, Y., et al. (2017). Antioxidant status and gut microbiota change in an aging mouse model as influenced by exopolysaccharide produced by Lactobacillus plantarum YW11 isolated from Tibetan kefir. J. Dairy Sci. 100, 6025–6041. doi: 10.3168/jds.2016-12480
Zhao, X., Cheng, M., Wang, C., Jiang, H., and Zhang, X. (2022). Effects of dairy bioactive peptides and lotus seeds/lily bulb powder on flavor and quality characteristics of goat milk yogurt. Food Biosci. 47:101510. doi: 10.1016/j.fbio.2021.101510
Keywords: quinoa protein hydrolysates, antioxidant activity, Lactobacillus plantarum, yogurt, quality promotion
Citation: Chen A-X, Meng F-B, Li J-J, Chen X-C, Li Y-C and Jiang L-S (2024) Quinoa protein hydrolysates improved the qualities and volatiles of yogurt fermented by Lactobacillus plantarum. Front. Sustain. Food Syst. 8:1432631. doi: 10.3389/fsufs.2024.1432631
Edited by:
Pedro Ferreira Santos, University of Minho, PortugalReviewed by:
Nutsuda Sumonsiri, Teesside University, United KingdomYu Xiao, Hunan Agricultural University, China
Copyright © 2024 Chen, Meng, Li, Chen, Li and Jiang. This is an open-access article distributed under the terms of the Creative Commons Attribution License (CC BY). The use, distribution or reproduction in other forums is permitted, provided the original author(s) and the copyright owner(s) are credited and that the original publication in this journal is cited, in accordance with accepted academic practice. No use, distribution or reproduction is permitted which does not comply with these terms.
*Correspondence: Yun-Cheng Li, liyunchengs@126.com; Li-Shi Jiang, Jlishi@cdutcm.edu.cn
†These authors have contributed equally to this work