- 1Graduate School of Science and Technology, University of Tsukuba, Ibaraki, Japan
- 2International Service for the Acquisition of Agri-biotech Applications (ISAAA AfriCenter), Nairobi, Kenya
- 3Institute of Life and Environmental Sciences, University of Tsukuba, Ibaraki, Japan
- 4Tsukuba-Plant Innovation Research Center, University of Tsukuba, Ibaraki, Japan
The sustainability of global coffee supply is threatened by production challenges worsening with every decade since the turn of the 21st century. Coffee is among the most popular beverages in the world, and its production supports livelihoods of millions of people, mostly smallholder farmers. With a global market value exceeding US$130 billion, the coffee industry is a source of export revenue for producing countries, the majority of which are developing nations in the Global South. Climate change, pests, and diseases continue to hamper productivity, profitability, and countries’ competitiveness in the global market. At the same time, the global demand for coffee continues to rise. A crucial pillar to safeguarding the future of coffee is continuously developing improved varieties suitable for the changing environment, and that address the needs of farmers and the industry. Yet, most national breeding programs are grossly out of touch with climate change reality and market dynamics. Working in isolation, these programs are constrained by unstable funding, limited sources of genetic variability, and inaccessibility of modern technologies necessary to accelerate the breeding process. We review here key production challenges, status of genetic diversity, and breeding approaches. Additionally, we explore opportunities in leveraging a pre-competitive collaborative breeding approach encompassing sharing of germplasm, and cross-border multi-environment trials in developing next-generation cultivars. We argue that breeding efforts should be informed by the needs of all the stakeholders in the supply chain including researchers, smallholder farmers, small and medium-sized enterprises investing in coffee processing and roasting, and consumers. Using emerging and reviving producers in Southeast Asia and Eastern Africa as a model, we explore how a paradigm shift in coffee breeding from the historically nation-centric approach to a more forward-looking collaborative model could improve the efficiency of variety improvement in face of climate change and growing market demand.
1 Introduction
Coffee is an economically important agricultural commodity cultivated across more than 11 million hectares in about 80 countries (Krishnan, 2017). The genus Coffea L. comprises 130 species (Davis and Rakotonasolo, 2021), with Coffea arabica L. (Arabica) and Coffea canephora Pierre ex A. Froehner (Robusta/Conilon) accounting for 55.9 and 44.1% of the total global coffee production, respectively (International Coffee Organization, 2023). A gradual re-emergence of Coffea liberica W.Bull (Liberica or Liberian coffee) has been reported in Africa and Asia, likely as a response to the recent Arabica and Robusta supply chain disruptions (Davis et al., 2022). Over 90% of coffee cultivated in the world is localized in developing countries (Ponte, 2002). Production involves at least 25 million smallholder farmers who produce 70–80% of the total beans, with the industry estimated to support the livelihoods of 125 million people worldwide (Barreto Peixoto et al., 2023).
Coffee ranks as the world’s second most consumed beverage (Salvador et al., 2024), and the most extensively traded tropical commodity. In most producing countries, the coffee trade contributes significantly to the Gross Domestic Product (GDP) through export revenue. During the 2022–2023 period, global coffee production was 168.2 million 60-kg bags, with the top five producers being, i.e., Brazil, Vietnam, Indonesia, Colombia, and Ethiopia, accounting for 74.39% of the total production. India, Honduras, Uganda, Mexico and Peru completed the list of the top 10 coffee-producing countries (Figure 1; International Coffee Organization, 2023). In total, the top ten producing countries accounted for 89.37% of all the coffee produced in the 2022–2023 year (Table 1; International Coffee Organization, 2023). This dominant production share effectively channels the majority of coffee export revenue to a limited number of growers in the major producing countries.
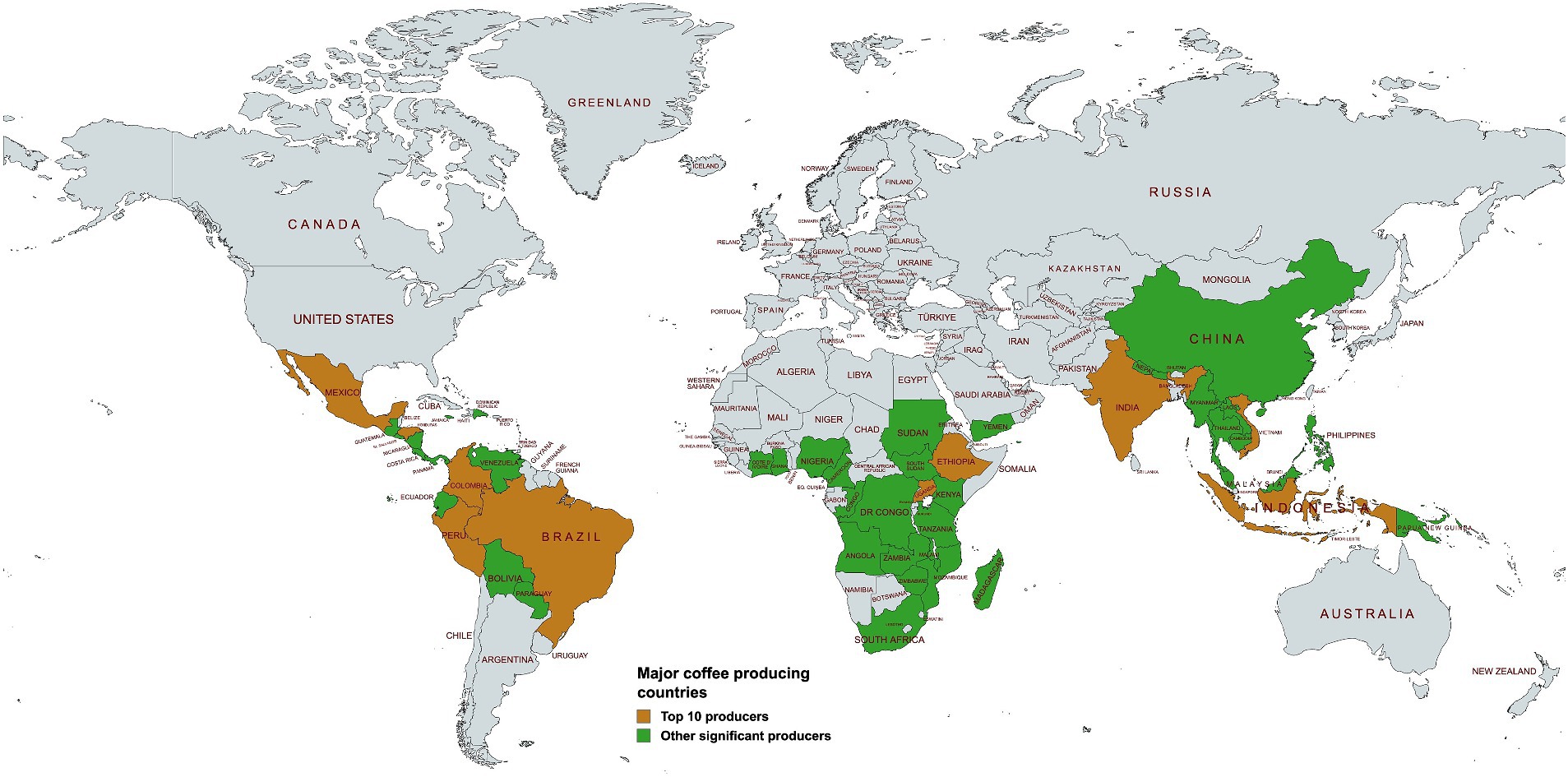
Figure 1. Major coffee-producing countries in the world. The top 10 producers as of 2022/2023 production year are shaded in brown, while other significant producers are shown in green (International Coffee Organization, 2023). Created with mapchart.net.
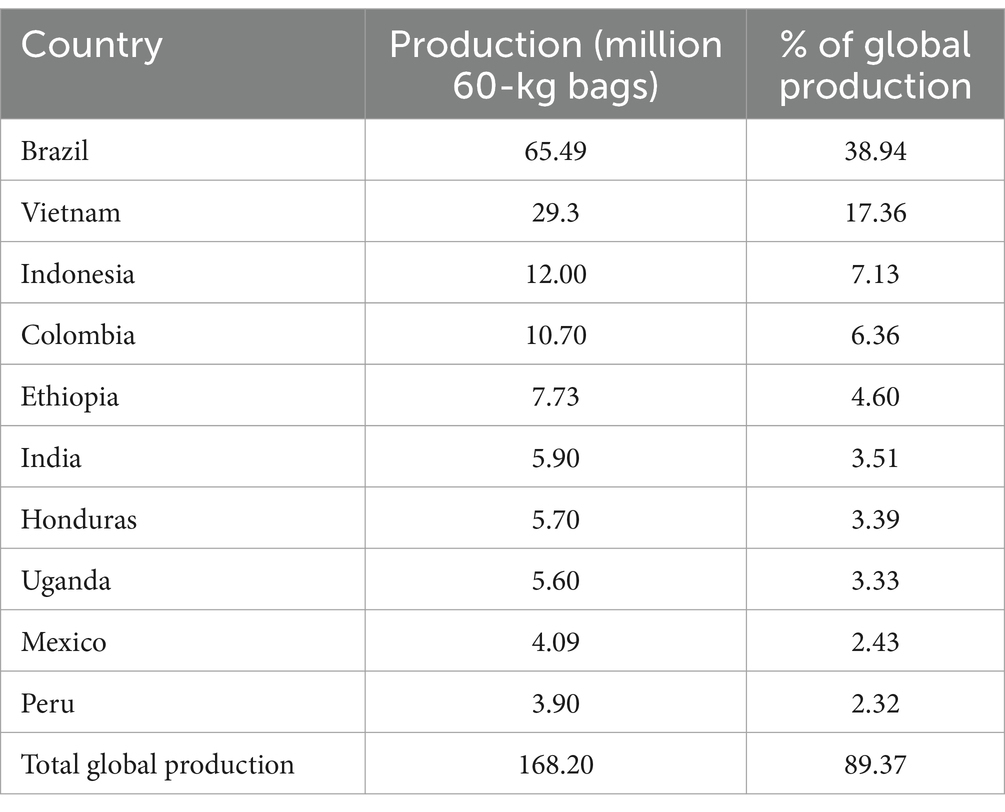
Table 1. Top 10 coffee producing countries and their contribution shares to global production during the 2022–2023 period (International Coffee Organization, 2023).
Despite the socioeconomic importance of coffee, the industry is increasingly facing challenges in every step along the product supply chain (Figure 2). To sustainably deliver quality coffee to global consumers, a complex web of actors and processes are involved in the value chain, from production, processing and distribution, to marketing. Presently, the production stage is hampered by several factors including climate change events, pests and diseases, labor shortages, rising cost of production, and the continued slow pace of improving varieties suitable for the changing environment and the global market (Mishra, 2019; Vegro and de Almeida, 2020). Post-production, the coffee product chain also faces sector-specific challenges majorly related to institutional framework constraints, volatility in global market prices, and fair-trade concerns (Ponte, 2002; Utting, 2009).
This review examines the major coffee production challenges, the status of genetic diversity, conventional and modern breeding approaches, and the role of collaborative breeding in future variety improvement. Particularly, we explore the potential of leveraging on a synergistic, pre-competitive breeding approach that encompasses stakeholder engagement, sharing of germplasm, and cross-border multi-location trials in addressing the dynamic and region-specific needs of actors in the coffee supply chain. To demonstrate the potential of this approach, we examine how selected emerging and reviving coffee producers in southeast Asia and East Africa stand to gain from the proposed paradigm shift in coffee breeding, considering the need for sustainability, profitability, and global market competitiveness in the face of climate change and the growing demand for high quality coffee.
1.1 Climate change, diseases, and pests
Climate variability in coffee production areas around the world continues to impact both the quantity and quality of beans. The main climatic factors affecting yield and bean quality are drought, extreme temperatures, excessive rainfall, and disease outbreaks (DaMatta and Ramalho, 2006; Läderach et al., 2017; Venancio et al., 2020; Bilen et al., 2022). For instance, during the austral winter of 2021, Brazil’s primary coffee-producing regions in the south and southeast endured heavy frost which led to a 30% fall in output. Coupled with other global market factors, this supply chain disruption in the world’s largest coffee producer and exporter contributed to a rise in global prices to above US$2/lb (Marengo et al., 2023).
Indirectly, climate variability affects coffee production through increased infestation, severity, and spread of diseases and pests including the notorious fungal pathogens Hemileia vastatrix Berkeley and Broome [coffee leaf rust (CLR)], Colletotrichum kahawae Bridge and Waller [coffee berry disease (CBD)], the destructive pests Hypothenemus hampei Ferrari [coffee berry borer], Leucoptera coffeela Guérin-Meneville [coffee leaf miner], Xylotrechus quadripes Chevlorat (Coleoptera: Cerambycidae) [coffee white stem borer], and Meloidogyne spp. [nematodes] (Ghini et al., 2008; Schroth et al., 2009; Bramel et al., 2017; Pham et al., 2019). For instance, a 30% increase in CLR disease incidence and severity was recorded in Colombia between 2008 and 2011 due to excess rainfall (Cristancho et al., 2012). We review in detail (section 2), the impacts of climate change, major diseases, and pests on global coffee production. In a business-as-usual scenario, these impacts are expected to worsen in the next decades, further threatening the sustainability of global coffee supply.
1.2 Labor shortages
In addition to difficulties brought on by climate change, diseases, and pests, producing coffee requires intensive labor, including experienced personnel to implement specialized operations along the commodity chain. A successful coffee commodity chain entails growing and propagating seedlings, managing nurseries, planting, pruning, controlling pests and diseases, harvesting, processing, sorting, grading, and roasting. Over the past decades, the industry has increasingly experienced labor shortages in several production regions at certain times of the year. In the affected regions, primarily in Latin America and Asia, the situation is further aggravated by changes in international border regulations, political dynamics, national immigration policies, aging farm workers, and labor force competition by other industries (Roseberry et al., 1995; Winkels, 2008; Griffith et al., 2017; Rhiney et al., 2021; Santos et al., 2022). Migrant labor has been particularly crucial in many coffee-producing countries, especially during the harvest seasons (Rhiney et al., 2021). Recently, a period of labor shortage was experienced in several producing countries due to travel and movement restrictions caused by the COVID-19 pandemic (Guido et al., 2020; Rhiney et al., 2021), further demonstrating vulnerability of the coffee sector to external shocks.
1.3 Rising cost of production
A fast-growing challenge facing the coffee sector in recent years is the increasing cost of production. At the farm level, this is majorly attributed to high input prices (fertilizers, pesticides, transportation et cetera.), and the rising cost of hired labor (Krishnan, 2017). This situation is worsened by unpredictable rainfall patterns caused by climate variability, as well as the frequent outbreaks of coffee pests and diseases in growing areas, necessitating additional expenses for irrigation and pesticides. In most cases, coffee farmers are compelled to make a price trade-off based on the prevailing selling prices. Usually, the global market price of coffee is influenced by several factors including supply and demand dynamics, weather conditions, commodity chain logistics, economic conditions, as well as political and social events in key market shareholder regions (Ponte, 2002; Lewin et al., 2004). The prevailing market volatility presents a complex price trade-off issue to growers from around the world, especially resource-limited smallholder farmers who lack bargaining power and access to alternative markets. When the market price disregards the true cost of production, and in the absence of structured fair-trade considerations, farmers are unable to earn a sustainable income from producing coffee, oftentimes forcing a switch to alternative crops with higher returns on farm investment (Gemech and Struthers, 2007; Millard, 2017; Guido et al., 2020).
1.4 Slow pace of breeding improved varieties
Perhaps the most urgent challenge in securing the future of coffee is the slow pace of developing improved varieties to match the effects of climate variability and the increasing demand for quality coffee. Presently, it is not uncommon to find farmers from around the world cultivating varieties selected several decades ago. For C. arabica, these are mainly selections of Typica and Bourbon ancestral lines and introgressed varieties related to Catimor and Sarchimor, while C. canephora varieties mostly being cultivated include groups selected from Uganda, Congo, Guinea, Guinea x Congo, and Guinea x Coffea congensis A. Froehner (World Coffee Research, 2024a).
The past three decades have demonstrated that the challenges of coffee production are ever evolving, and so are the buyer and consumer preferences. In the next three to five decades, the coffee sector is expected to experience significant changes in both the production environment and market demands. It takes at least 25 years to develop and release a new pure-line variety to farmers (McCook and Montero-Mora, 2024), and even longer to achieve widespread adoption. Hence, there is an urgent need to continually develop and introduce improved varieties that effectively meet the needs of both the farmers and the industry.
1.5 Limited sources of genetic variability
Coffea arabica is characterized by an extremely low level of genetic diversity. This is majorly attributed to its origin, a single hybridization event between the diploid progenitor parents C. canephora and Coffea eugenoides S. Moore (Scalabrin et al., 2020), and the self-pollinating reproductive nature of this allopolyploid species (Anthony et al., 2002). This is aggravated by the fact that most commercial varieties being cultivated around the world originate from a few cultivars, resulting in a significantly narrow genetic base (Silvestrini et al., 2007; da Silva et al., 2019). Limited access to sources of agronomic and genetic variability is a key challenge that continues to impede breeding efforts aimed at developing durable resilience against biotic and abiotic stresses, while keeping up with the growing consumer demand for high quality coffee.
In a business and usual scenario, this challenge is expected to impact the future productivity of Arabica coffee more than that of Robusta coffee, owing to C. arabica’s higher vulnerability to pests, diseases and climate variability as compared to that of the more resilient C. canephora. As such, there is an urgent need for breeding programs to exploit novel sources of agronomic and genetic variability for use in C. arabica variety improvement, including sourcing from the two diploid progenitors and wild species (Van der Vossen, 2018; Scalabrin et al., 2020).
1.6 A systemic bottleneck in national coffee breeding programs
In most producing countries, coffee breeding programs are anchored within national research institutes mandated by the state to conduct research and provide farmers with solutions to country-specific challenges. Ideally, this should entail continuous variety improvement for climate resilience and adaptation, productivity, profitability, and keeping pace with market demands including strategic alignment with the industry’s quality needs. However, most in-country programs face systemic hurdles such as inadequate funding, lack of specialized facilities, expertise, and field trial sites across multiple locations, limited access to diverse genetic resources and modern breeding technologies. Moreover, lack of cross-border collaboration with other regional and international coffee industry stakeholders means that most national breeding programs operate in isolation, with little or no sharing of knowledge, resources, and genetic materials. This creates a bottleneck in the breeding programs, leaving farmers with limited access to improved varieties suited for the changing production environment and market needs.
1.7 The need for a paradigm shift in breeding future varieties
Most national breeding programs are grossly out of touch with the urgency to continuously improve varieties that meet the needs of farmers and industry. Considering the need for industry sustainability in the face of a rapidly changing climate and consumer preferences, coffee breeding programs could be revamped to encompass a strategic, pre-competitive collaborative approach that allows for co-development of future cultivars by incorporating inputs of the stakeholders along the value chain. That way, the sector reduces supply chain disruptions brought about by climate change events, diseases, and pests, while meeting the needs of farmers, industry players and consumers alike.
A vital starting point would be ensuring that coffee improvement programs constitute clear need-based breeding objectives, diverse germplasm collection, effective breeding tools, collaborative cross-border multi-environment trials, seedling production, dissemination, and intellectual property management. After highlighting the major production challenges, status of genetic diversity, and breeding approaches, this review explores key opportunities in leveraging shared genetic resources and pre-competitive collaborative breeding aimed at continuous variety improvement and its contribution in securing the future of global coffee supply.
2 Key coffee production challenges
2.1 Climate change
Climate change has a significant impact on the production of both C. arabica and C. canephora (Bunn et al., 2015a). This is primarily as a result of the associated climatic stresses including recurring drought events, extreme temperatures, erratic precipitation patterns, and hurricanes in coffee-growing areas (Krishnan, 2022). The effects of these shocks on coffee production range from reduction in yield and bean quality, increased severity and spread of pests and diseases, to loss of land suitable for coffee cultivation (Schroth et al., 2009; Laderach et al., 2011; Bramel et al., 2017; Moreira et al., 2021).
Extreme temperatures and inadequate water retard coffee plant growth, flowering, and berry development, resulting in suboptimal quantity and quality of yield (Schroth et al., 2009; Zullo et al., 2011). This negatively impacts the productivity and profitability of coffee farm ventures. For instance, during dry years, some marginalized areas have recorded coffee yield reductions of up to 80% in the absence of irrigation (DaMatta and Ramalho, 2006). In recent years, the impacts of climate change on coffee growers across the world have worsened, especially on smallholder farmers who depend on the crop for income and household livelihood.
In Kenya, for instance, the year 2022 recorded long spells of drought conditions resulting in drying up of trees in smallholder farms that have no access to irrigation. In the following year, most farmers were not only forced to invest in irrigation, but also to replace the dried-up trees that left empty gaps in their farms. Without proper mitigation and adaptive measures in place, the increasingly worsening effects of climate change spell a bleak future for such smallholders around the world who cultivate 70–80% of the coffee produced globally (Barreto Peixoto et al., 2023).
Climate change is also expected to impact both geographical distribution and the amount of land suitable for growing coffee in intertropical regions. Under the representative concentration pathways (RCP) adopted in the Fifth Assessment Report (AR5) of the Intergovernmental Panel on Climate Change (IPCC), future projections models predict a geographical upward shift of the lower altitude limit, and up to 50% reduction in areas suitable for C. arabica cultivation by 2050 (Bunn et al., 2015b; Ovalle-Rivera et al., 2015). Under similar climatic projections, however, the area suitable for cultivating the hardier, more climate-resilient C. canephora species is expected to increase. There are concerns that the upward shift in lower altitude limit and shrinking of land area suitable for C. arabica cultivation will fundamentally trigger forest clearance to match the growing global demand for Arabica coffee, as this presents a threat to forest biodiversity in producing countries (Magrach and Ghazoul, 2015).
2.2 Diseases
Coffee is vulnerable to several diseases that impact both crop productivity and bean quality. These include the fungal pathogens H. vastatrix, C. kahawae, Fusarium xylarioides R. Heim and Saccas [coffee wilt disease (CWD)], Mycena citricolor (Berkeley and Curtis) Saccardo [American leaf spot disease (ALSD)], Fusarium sp. [root rot disease], Cercospora coffeicola Berkeley and Cooke [brown eye spot (BES)], and the bacterial pathogens Pseudomonas syringae pv. garcae [bacterial halo blight (BHB)], Pseudomonas syringae pv. tabaci [bacterial leaf spot (BLS)], Pseudomonas chicorii [bacterial leaf blight (BLB)], and Xylella fastidiosa subsp. pauca [coffee leaf scorch (CLS)] (Table 2; Waller et al., 2007; Van der Vossen et al., 2015; Krishnan, 2017, 2022; Avelino et al., 2018; Badel and Zambolim, 2019).
2.2.1 Coffee leaf rust—Hemileia vastatrix
Coffee leaf rust, caused by the fungal pathogen H. vastatrix, has been the most devastating biotic stress to C. arabica around the world. The effect of CLR on the world’s production of C. arabica has been projected to result in annual crop losses of 20–25%, or US$ 1–2 billion (Van der Vossen, 2001). Without proper control measures, crop losses of up to 70% have been reported in heavy CLR infestation of susceptible C. arabica cultivars (Prakash et al., 2004).
The worst recorded case of CLR infestation occurred in Central America between 2012 and 2013 when an outbreak swept through coffee farms across Guatemala, Nicaragua, El Salvador, Honduras, and Costa Rica (International Coffee Organization, 2013). Other affected countries included Panama, Jamaica, and the Dominican Republic. A total of 593,037 ha representing 55% of the total coffee growing area in Central America was severely affected. This infestation resulted in a yield loss of 2,706, 454 (60-kg bags) worth USD 499.4 million, with serious economic implications in the affected areas. Furthermore, the outbreak is estimated to have resulted in a loss of 374,000 jobs (International Coffee Organization, 2013), further exacerbating the negative socioeconomic implications across the region.
In Kenya, coffee farmers majorly cultivating CLR-susceptible C. arabica varieties such as SL28 and SL34 are required to apply copper-based fungicides regularly to control the disease. When left untreated, the fungal pathogen multiplies rapidly on the underside of infected leaves through the characteristic orange lesions (Figure 3A). Each lesion can produce up to 400,000 spores in 3–5 months, which are easily spread by wind across and between farms (Kawabata and Nakamoto, 2021). Starting from lower and spreading upwards toward the higher leaves (Figure 3B), the fungus causes extensive defoliation of the infected trees (Figure 3C), and ultimately severe yield loss.
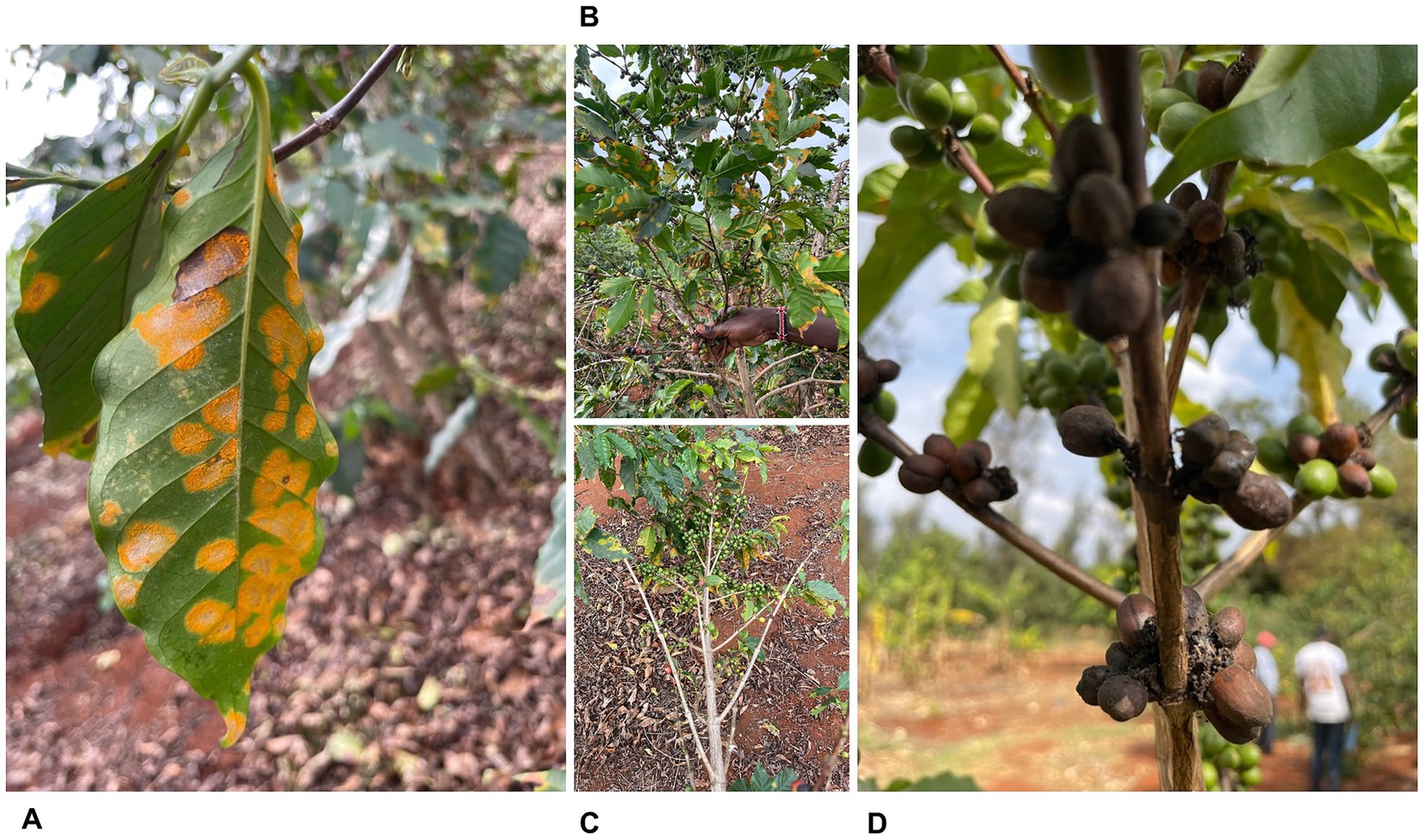
Figure 3. Coffee leaf rust H. vastatrix and Coffee berry disease C. kahawae infecting C. arabica SL28 in Kenya. (A) CLR orange lesions on the underside of infected leaves. (B) CLR infestation spreading from the lower to upper leaves of an infected tree. (C) Extensive defoliation of heavily CLR-infected trees. (D) CBD infection on developing coffee berries. (Photograph credit: Godfrey Ngure).
2.2.2 Coffee berry disease—Colletotrichum kahawae
Coffee berry disease, caused by the hemibiotrophic fungus C. kahawae, is an economically significant disease affecting coffee production. Unlike CLR, CBD is only found in African countries that grow C. arabica, having originated from Kenya and later spreading to other East, Central, and South African coffee-growing regions in the period between 1922 and 1985 (Waller et al., 1993; Hindorf and Omondi, 2011).
Primarily affecting developing green berries, CBD starts off as small water-soaked lesions that quickly sunken, turns dark brown to black, and expands engulfing the whole berry (Figure 3D). Infected berries eventually dry out and fall off the branch prematurely. Without proper control measures, CBD infestation can cause yield losses of up to 80% (Silva et al., 2006; Van der Vossen and Walyaro, 2009). This is exacerbated by the pathogen’s ability to spread rapidly under favorable conditions and persist in fields for years, making control and eradication challenging.
It is estimated that crop loss and the cost of chemical control of CBD have a US$300–500 million annual economic impact on C. arabica production in Africa (Van der Vossen and Walyaro, 2009). As such, an integrated disease management strategy comprising good cultural practices, chemical application, and use of CBD-resistant varieties is recommended to keep the pathogen under control.
2.2.3 Coffee wilt disease—Fusarium xylarioides
Coffee wilt disease is a vascular wilt disease caused by the fungal pathogen F. xylarioides (anamorph: Gibberella xylarioides R. Heim and Saccas), endemic to East and Central Africa (Rutherford, 2006; Hindorf and Omondi, 2011; Zhang et al., 2023; Peck and Boa, 2024). It was first observed infecting Coffea excelsa A. Chev (now C. liberica) in 1927 in the present-day Central African Republic (CAR) (Figueres, 1940). From CAR, the pathogen subsequently spread to C. liberica and C. canephora plantations in the neighboring countries including the Democratic Republic of the Congo (DRC, formerly Zaire), Cameroon, Côte d’Ivoire (formerly Ivory Coast) and Guinea (Peck and Boa, 2024).
All developmental phases of wild and cultivated Coffea species are susceptible to CWD, which manifests as wilting, defoliation, necrosis in the wood beneath the bark, and eventually death of infected trees (Flood, 2021). Infestation can result in production losses of up to 80%, with its localized infection of the host’s vascular system making it difficult to employ chemical control (Hakiza et al., 2010; Alemu, 2012; Flood, 2021).
The first major outbreak of CWD in 1940s and 1950s in Central and West Africa had such a devastating effect on coffee farming in the region, that it essentially decimated C. liberica from commercial production (Rutherford, 2006; Peck and Boa, 2024). A separate outbreak infecting C. arabica was recorded in Ethiopia in 1957 (Kranz and Mogk, 1973). In DRC, Guinea, and Côte d’Ivoire, losses of up to 50% were incurred from the outbreak (Rutherford, 2006; Peck and Boa, 2024). To manage the disease, the affected countries adopted phytosanitary practices of disinfecting farm tools, systematic uprooting and burning of infected trees, providing farmers with clean planting materials, relocating production to new areas, and exploring long-term breeding for host resistance (Rutherford, 2006; Peck and Boa, 2024).
The widescale phytosanitary response effectively controlled the outbreak, leading to a gradual recovery of C. canephora production in the affected regions. However, the pathogen continued to spread silently in abandoned plantations in the DRC, and in the 1970s, the disease resurfaced in a second major outbreak (Flood, 2021). By 1995, the disease had spread to the neighboring countries Uganda and Tanzania (Phiri and Baker, 2009). In Uganda, the outbreak destroyed 14.5 million C. canephora trees across the country, with yield losses of up to 77% being reported in some affected Robusta-growing districts (Hakiza et al., 2010; Flood, 2021).
Presently, the pathogen exists in two host-specific populations, one targeting C. arabica in Ethiopia, and the other targeting C. canephora in Uganda, Tanzania, and the DRC (Rutherford, 2006; Alemu, 2012; Olal et al., 2018). Since there are no CWD-resistant varieties available to coffee farmers, control is limited to phyto-sanitation and pathogen containment to prevent introduction to new areas. This necessitates concerted breeding efforts to identify novel sources of resistance for introgression in farmer-preferred varieties as a safeguard against future disease outbreaks.
2.3 Pests
Coffee is a host to several insect pests with varying degrees of significance based on the severity of the damage, ease of control, persistence in farms, and overall impact on the health of trees, crop yield, and bean quality. Whereas minor coffee pest species are usually confined in their respective endemic regions, major pests are widespread across larger growing regions comprising multiple countries. The latter include H. hampei, L. coffeela, X. quadripes, Pseodococcidae spp. [coffee root mealybugs], Meloidogyne spp. and Antestiopsis thunbergii Gmelin (Hemiptera: Pentatomidae) [antestia bug] (Table 3; Barrera, 2008; Vega et al., 2015; Ahmed et al., 2016; Krishnan, 2017; Carvalho et al., 2019).
The coffee berry borer continues to be the pest with the greatest economic impact worldwide (Jaramillo et al., 2006; Vega et al., 2015). Endemic to Africa, the pest was inadvertently distributed around the world through infested seeds and has now been reported in all coffee-producing countries except Nepal and Papua New Guinea (Vega et al., 2015). Without proper control measures, crop losses from heavy coffee berry borer infestation can be significant, ranging from 50 to 100% of the affected berries. Moreover, chemical control is challenging owing to the pest’s characteristic life cycle inside the infested berries—where it spends most of its life (Le Pelley, 1968). None of the commercially cultivated C. arabica and C. canephora varieties exhibit resistance to coffee berry borer. As such, integrated pest management comprising both cultural measures and chemical control is practiced by most farmers to reduce infestation (Duque and Baker, 2003; Crowe, 2009). Attempts to use natural enemies as biological control have only attained modest, unsustainable gains (Jaramillo et al., 2006), necessitating the exploration of novel sources of durable resistance to the pest.
Coffee leaf miner is a moth of African origin, now considered the second most significant insect pest of coffee. Its larvae feed on the parenchyma tissues of coffee leaves, creating meandering tunnels or “mines” as they consume the leaf tissue. Infested leaves fall prematurely, and resultant crop losses ranging between 50 and 80% have been reported in African and Neotropical countries (Crowe, 2009; Carvalho et al., 2019). While pesticide use has proven to be successful in managing the coffee leaf miner, it has an implication on the cost of production due to the additional expenses of procuring and applying chemicals, and potential environmental repercussions. Again, this calls for further breeding research to provide farmers with high-yielding varieties resistant to the pest.
Other significant pests of coffee have been reported to cause considerable crop losses with socio-economic impacts in different parts of the world (Table 3). For instance, the coffee white stem borer is the most serious pest of coffee in Nepal, responsible for up to 70% of crop loss (Pandey et al., 2022). In East Africa, yield losses of up to 45% due to severe antestia bug infestation have been reported (Ahmed et al., 2016; Bigirimana et al., 2019). In Espírito Santo, Brazil, root-knot nematodes are increasingly becoming a serious concern for C. canephora growers (Barros et al., 2014), while in Colombia, root mealybugs are regarded as the second most significant pest of coffee after coffee berry borer (Gil et al., 2016; Acevedo et al., 2019).
3 Coffee genetic diversity
About 350,000–610,000 years ago, two diploid coffee species C. canephora and C. eugenoides underwent a single polyploidization event, giving rise to the allotetraploid C. arabica (Salojärvi et al., 2024). This event occurred spontaneously in the highlands of Southwestern Ethiopia, the primary center of origin and diversity of C. arabica where the species was discovered in AD 850 (Smith, 1985; Anthony et al., 2002; Herrera and Lambot, 2017; Scalabrin et al., 2020). The rise of C. arabica species from a single individual post-polyploidization resulted in a weak population structure, and an extremely low level of genetic diversity in the present cultivated germplasm (Lashermes et al., 2014; Scalabrin et al., 2020). This is further perpetuated by the species’ self-fertile reproductive biology (i.e., autogamy) and the fact that most commercial varieties originated from a few cultivars, majorly from Typica and Bourbon genetic base (Lashermes et al., 2000). Moreover, allelic variation between cultivated C. arabica vis-à-vis the diploid progenitor parents further supports the hypothesis that present diversity arose post-polyploidization. This has consistently been confirmed by various studies employing different molecular markers to assess genetic variability within and between commercially cultivated C. arabica varieties, the progenitor diploid parents, and wild accessions from the primary center of origin (Lashermes et al., 1996; Anthony et al., 2002; Steiger et al., 2002; Maluf et al., 2005; Silvestrini et al., 2007; da Silva et al., 2019).
Genomic analyses of C. arabica continue to yield further insights into its evolutionary history, genetic diversity, population structure, and breeding potential, enhancing our understanding of this economically important crop species. Using chromosome-level assemblies, Salojärvi et al. (2024) reported largely conserved genome structures between the progenitor diploid parents and descendant subgenomes, with no clear dominance of one subgenome over the other. Importantly, the study further demonstrated that genetic variation among C. arabica wild accessions was already significantly low due to multiple pre-domestication bottlenecks. By resequencing 90 accessions from Choche germplasm bank in Ethiopia, Mekbib et al. (2022) identified 9.74 million single nucleotide polymorphisms (SNPs) and 1.34 million insertions/deletions (InDels). Phylogenetic analysis using the SNPs revealed distinct genetic groups with a strong geographical pattern, indicating population differentiation. Updating and expanding such genomic information is particularly crucial for breeding programs focused on germplasm conservation and genomics-based breeding of improved varieties with desirable traits. Crop wild relatives typically exhibit higher genetic variability and are important reservoirs of genes for adaptation to changing environments and resistance to pests and diseases (Tobón-Niedfeldt et al., 2022). Evidence indicate that this is more so true for coffee. For instance, compared to C. arabica, the wild relative Coffea racemosa Lour in Mozambique displayed higher levels of genetic diversity and heterozygosity, suggesting a greater capacity for adaptation and resilience. Moreover, SNP analysis of functional pathways indicated that stress signaling in C. racemosa is more robust, further underscoring its potential as a more resilient species against environmental challenges (Tapaça et al., 2023).
To date, many C. arabica coffee farmers around the world still cultivate varieties selected and released over three decades ago. For instance, farmers in Kenya, Uganda, Malawi, and Zimbabwe still cultivate the bourbon derived SL28 developed in Kenya by the Scott Agricultural Laboratories in the 1930s. Although SL28 is famous for its high yield, exceptional cup quality, and drought tolerance, it is highly susceptible to CLR, CBD, and nematodes. In Latin America, Caturra, Catuai, and Mundo Novo first selected and distributed to farmers in 1937, 1949, and 1952, respectively, are still popular with farmers due to good yield potential and cup quality. Given the worsening effects of climate change and its consequential impact on the increased severity and prevalence of diseases and pests, alongside the growing worldwide demand for high-quality coffee, there is an ongoing need for constant improvement in C. arabica coffee varieties. Most importantly, coordinated regional collaborations based on the mutual sharing of knowledge and resources are required for the effective conservation of germplasm. This is a crucial pillar in strategic breeding efforts aimed at tackling location-specific production challenges in various C. arabica coffee-growing regions.
On the other hand, while the extent of variability in C. canephora is still being explored, it is widely acknowledged that genetic diversity within this species is substantially greater as compared to C. arabica (Montagnon et al., 1992). The first cultivation of C. canephora has been traced back to the Congo basin in 1870, and its center of origin was mapped in the lowland forests of tropical central and western Africa (Smith, 1985; Charrier and Berthaud, 1990; Gomez et al., 2009; Herrera and Lambot, 2017). Today, Robusta coffee makes up 44.1% of the global coffee production, with the top five producers Vietnam, Brazil, Indonesia, Uganda, and India accounting for 89% of the total Robusta production (International Coffee Organization, 2023).
Since 1986, genetic diversity studies utilizing different molecular markers have successfully categorized C. canephora into three main diversity groups comprising Congolese (originating from the Democratic Republic of Congo), Guinean (originating from Guinea and Côte d’Ivoire—formerly Ivory Coast), and Ugandan genetic groups, with the Congolese-Guinean group further separated into five sub-groups (Montagnon et al., 1992; Gomez et al., 2009; Musoli et al., 2009; Akpertey et al., 2021). Whole genome sequencing of C. canephora revealed extensive expansion of key species-specific gene families including flavonoids and alkaloids enzymes, defense-related genes, and key N-methyltransferases involved in caffeine biosynthesis (Denoeud et al., 2014). This, coupled with the demonstrated genetic variability within the species makes C. canephora a valuable source of desired traits including disease resistance, resilience to climate variability, and ability to grow and produce in lower altitude environments (Carvalho, 1988; Lashermes et al., 2000; Herrera and Lambot, 2017). As such, in addition to in situ conservation measures, prioritizing collaborative efforts for the collection, and ex situ conservation of these valuable genetic resources within sustainable germplasm banks is crucial. This will ensure their availability for utilization in coffee breeding programs for continuous variety improvement of both C. arabica and C. canephora.
4 Coffee breeding
4.1 Conventional breeding approaches
Since the early 1920s, C. arabica has been routinely bred using conventional techniques in different countries (Mishra, 2019). Before the 1990s, the objectives of most breeding programs were majorly geared toward increasing crop productivity and building durable tolerance to coffee leaf rust. Gradually, the focus of these programs expanded to incorporate regional/country-specific goals including cup quality, plant vigor, resistance to insect pests (e.g., coffee berry borer, white stem borer, leaf miner, and nematodes et cetera), and coffee berry disease, drought tolerance, compact growth, and uniform ripening (Mishra, 2019; Krishnan, 2022).
To date, conventionally bred varieties released from the early breeding programs are still cultivated in many countries. An example of this is Ruiru 11, a dwarf, composite hybrid variety released in Kenya in 1985 by the Coffee Research Foundation (now Kenya Agricultural and Livestock Research Organization—Coffee Research Institute, KALRO–CRI). It is known for its high yield, compact growth, tolerance to CLR, and resistance to CBD (Figure 4). Despite being released several decades ago, Ruiru 11 is still cultivated by farmers in Kenya and Tanzania. Batian, a fifth filial (F5) generation of Ruiru 11 released in Kenya in 2010, has recently gained popularity in both countries.
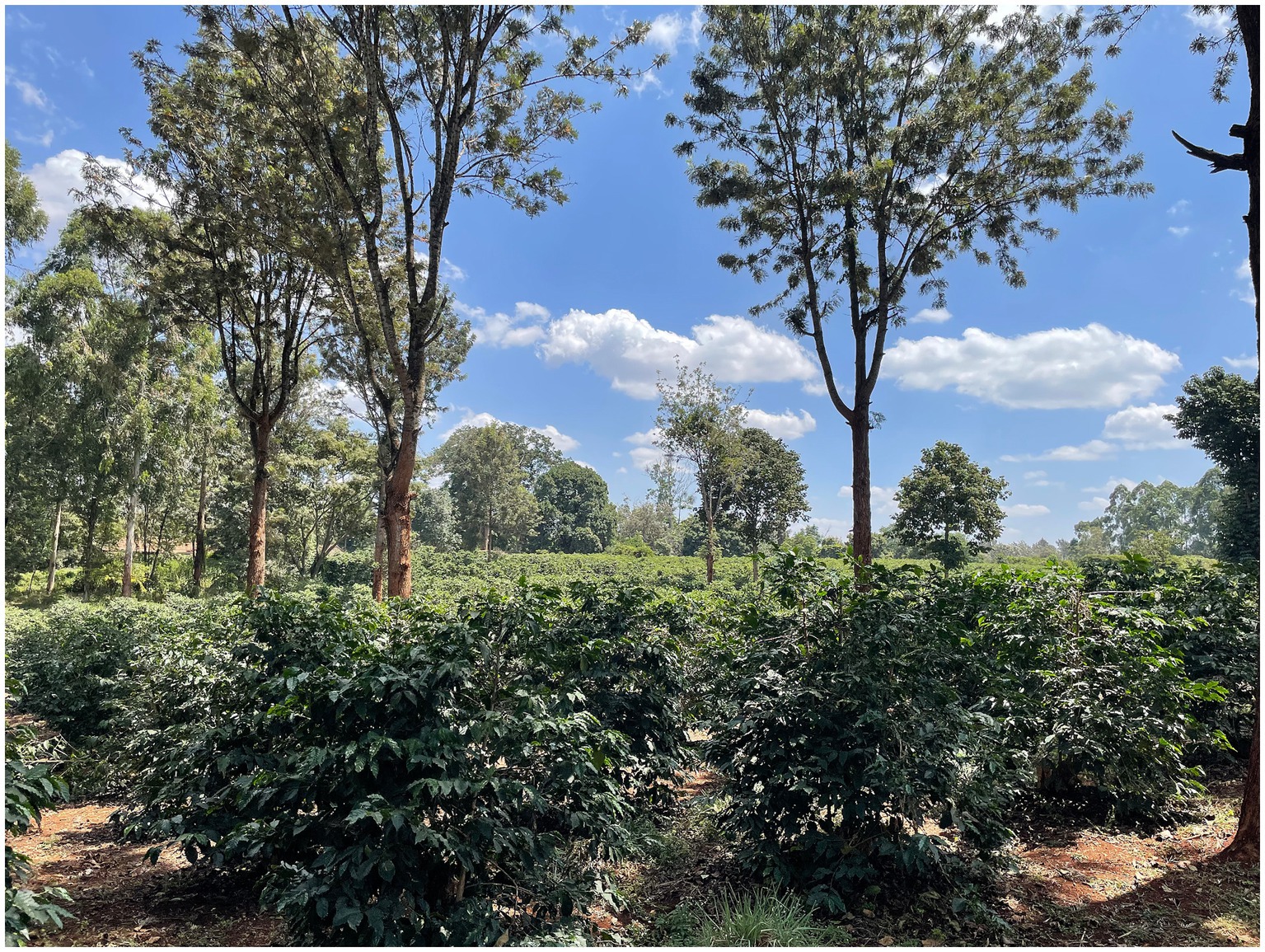
Figure 4. Coffea arabica Ruiru 11 at the Kenya Agricultural and Livestock Research Organization—Coffee Research Institute (KALRO-CRI) in Ruiru, Kenya. The dwarf, composite F1 hybrid variety is popular with Kenyan farmers due to its compact growth that allows for easy harvesting, high yields, good cup quality, tolerance to CLR, and resistance to CBD. (Photograph credit: Godfrey Ngure).
Various researchers have extensively reviewed the conventional breeding strategies and techniques that have been employed in the development of new and improved coffee varieties since the early 1920s (Carvalho, 1985; Charrier and Berthaud, 1985; Wrigley, 1988; Eskes and Leroy, 2004; Van der Vossen, 2018; Mishra, 2019). These include direct selection of superior plants from existing varieties, intra-and inter-varietal crossing followed by selection of F1 hybrids or pedigree selection, and inter-specific hybridization followed by backcrossing and pedigree selection as summarized in Table 4 (Van der Vossen, 2018; Mishra, 2019; Krishnan, 2022). Selected representative examples of C. arabica varieties released from these approaches in different countries and their distinctive traits are also provided in Table 4.
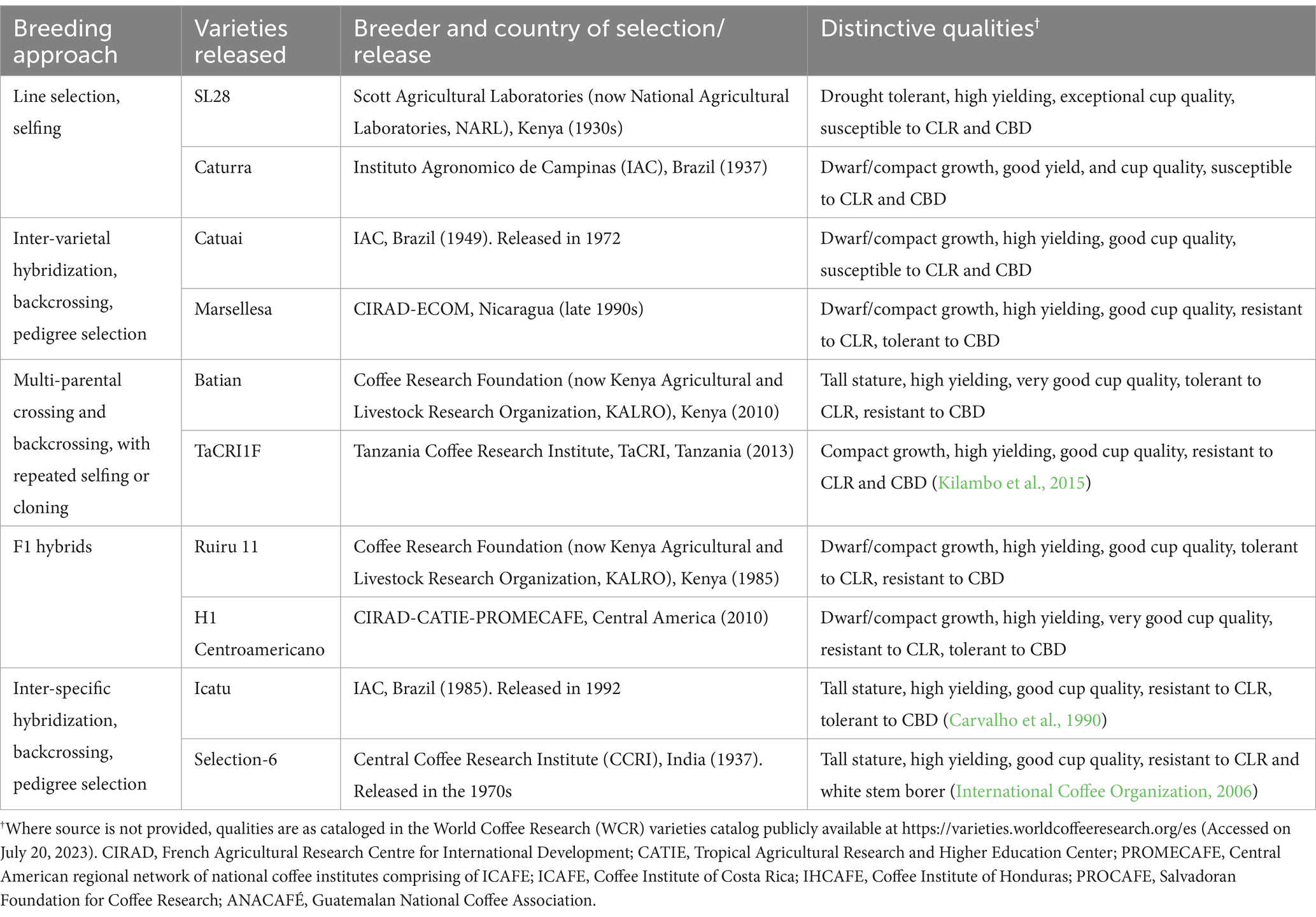
Table 4. Coffea arabica conventional breeding strategies, examples of resultant materials and their distinctive traits.
4.2 Modern breeding approaches
Over the past century, conventional breeding has achieved success in developing improved coffee varieties with desirable traits in various countries. However, this process is rather costly, labor-intensive, and time-consuming owing to the crop’s lengthy breeding cycle. Moreover, hybridization-based approaches are limited by linkage drag, and the long time spent back-crossing filial generations to restore the genetic background of the recipient cultivar (Lashermes et al., 2000).
Typically, it takes between 25 and 35 years to develop and release a novel pure-line coffee variety, and even longer for it to be fully adopted by growers. This is further complicated by the imprecision of phenotypic selection, and the extremely limited genetic variability within the currently available coffee gene pool (Scalabrin et al., 2020). The latter limits the introduction of novel traits required to maintain productivity under the 21st century emerging threats including climate change, pests, and diseases. This is in addition to the fast-rising global demand for premium coffee by consumers. Under these circumstances, there is an urgent need for coffee breeding programs to integrate modern breeding approaches that bypass the bottlenecks of conventional methods. This will enable efficient development and timely delivery of improved varieties, meeting the demands of both farmers and consumers.
The turn of the 20th century came with accelerated advancements in plant breeding, with novel innovative technologies now transforming key crop production systems. Particularly, the integration of biotechnology, genomic research, and the utilization of DNA-based genetic markers ushered in a new era in molecular plant breeding, which is now considered the fundamental basis for crop improvement in the 21st century (Moose and Mumm, 2008). This presents crop breeders with an additional set of innovative tools that improve the ease, speed, precision, cost, and generation time of novel varieties with desired traits. Although significant progress has been made in deciphering the coffee genome, and development of molecular breeding tools, coffee breeding programs are yet to fully incorporate these outputs in routine crop improvement strategies.
Whole genome sequences of both C. canephora (Denoeud et al., 2014) and C. arabica (Scalabrin et al., 2020, 2024; Mekbib et al., 2022) have been published, providing molecular breeders with a resource for studying allelic variation in these two commercially relevant species. Moreso, the SNPs markers identified during C. arabica sequencing, and re-sequencing provide useful insights into genetic variation and population divergence within the species and in comparison, with its diploid progenitor parents (Scalabrin et al., 2020; Mekbib et al., 2022). Before this, first-generation DNA-based genetic markers with less resolving power, including Restriction Fragment Length Polymorphism (RFLP), Amplified Fragment Length Polymorphism (AFLP), and Random Amplified Polymorphic DNA (RAPD) had been utilized in coffee genotyping (Orozco-Castillo et al., 1994; Lashermes et al., 1996, 2000; Paillard et al., 1996). With advancements in genotyping technology, a second generation of more informative high throughput markers was later developed and used to evaluate coffee genetic diversity and population structure. These include Simple Sequence Repeats (SSR), Inter-SSR (ISSR), Diversity Arrays Technology (DArT), and genotyping by sequencing (GBS) for large-scale SNP identification (Aga et al., 2005; Missio et al., 2009; Garavito et al., 2016; Spinoso-Castillo et al., 2020). Molecular markers like SSR have a resolving power to estimate genetic distances between plants or clones, measure genetic variability, and identify divergent plants for use as parents in breeding programs. For instance, Sousa et al. (2022) used 21 SSR markers to evaluate the genetic variability in a population of 44 C. canephora and 1 C. arabica genotypes, ultimately recommending specific plants for future crossing to preserve the population’s genetic diversity.
Today, the availability of high throughput genetic markers and whole genome sequences presents an opportunity to transition coffee improvement from the cumbersome conventional approaches to more focused molecular breeding, especially utilizing Marker-Assisted Selection (MAS) and Genomic Selection (GS). Wide adoption of these approaches in coffee breeding will improve the efficiency of identification and selection of both monogenic and polygenic desirable traits, thereby accelerating the breeding process to deliver superior varieties that meet the needs of farmers and the industry alike.
An additional valuable biotechnological tool for enhancing coffee breeding and research is in vitro plant tissue culture, presently being used for the rapid multiplication of elite genotypes, germplasm preservation, and mass propagation of disease-free plants for distribution to farmers. The first case of direct somatic embryogenesis (SE) in Coffea spp. was reported by (Staritsky, 1970) using stem pieces of orthotropic shoots obtained from C. arabica, C. canephora, and C. liberica. Thereafter, Söndahl and Sharp (1977) reported high-frequency somatic embryogenesis (HFSE) and plantlet regeneration from in vitro cultures using leaf explants obtained from plagiotropic shoots of C. arabica. Since then, different SE protocols utilizing different phytohormones and culture conditions have been proposed for in vitro regeneration of C. canephora and C. arabica (Kumar et al., 2006; Santana-Buzzy et al., 2007). Since the early 1990s, SE has been scaled up for commercial propagation of elite C. arabica F1 hybrids in Central America, and multiplication of selected C. arabica and C. canephora genotypes in Asia and Africa (Etienne et al., 2018; Aguilar et al., 2022).
The C. canephora subgenome contributes 21,254 gene models in the C. arabica allotetraploid, with the other 22,888 provided by the C. eugenoides progenitor parent (Scalabrin et al., 2020). Presently, C. canephora remains a crucial source of important traits not available in the C. arabica gene pool. These include resistance to CLR, CBD, and root-knot nematodes (Lashermes et al., 2000). Additionally, Híbrido de Timor (HdT), a natural interspecific hybrid between C. arabica and C. canephora has also been utilized as a source of resistance to CLR, CBD, nematodes, and BHB (Pereira et al., 2005; Silva et al., 2018). As coffee continues to face more production challenges in the 21st century, HdT and diploid species (both cultivated and wild accessions) will play a critical role as genetic sources of agronomic traits required to build resilience in commercially cultivated varieties.
Over the past decade, there has been increasing interest in the synthetic polyploidization of Coffea spp. to develop new germplasms. Polyploids have been effectively produced using chemically-induced polyploidization using anti-tubulin agents, followed by in vitro plant recovery via indirect SE (Sanglard et al., 2017, 2019; Venial et al., 2020). This area of research shows potential in advancing the development and conservation of novel coffee germplasm, potentially expanding sources of genetic variability for use in future breeding initiatives.
5 Coffee variety improvement through collaborative breeding
Since the Green Revolution in the mid-20th century, collaborative research and breeding efforts have proven to be highly effective in advancing agricultural productivity, sustainability, and resilience in the face of increasing production challenges. The success of some major crops including wheat, rice, maize, and potato can majorly be attributed to collaborative breeding efforts between international agricultural research centers (IARCs), national agricultural research systems (NARS), scientists, and local farmers (Evenson and Gollin, 2003). Through collaborative breeding, breeders can synergize efforts with researchers and value chain stakeholders from different regions, to address specific challenges informed by farmers, consumers, and the actors in between. This facilitates sharing of knowledge, expertise, best practices, and resources toward achieving a common overarching goal of sustainable production. Given the production challenges highlighted in this review, a global shortage is projected within the next decade as demand exceeds supply (Krishnan, 2022), and considering the changing dynamics in consumer demand for premium and specialty coffee, breeding programs stand to benefit more from collaborative research aimed at strategic variety improvement. Going forward, sustainability of the coffee industry will be intrinsically linked to how efficiently we can develop and disseminate superior, climate-resilient varieties that meet the demands of farmers in different agroecological regions, processors, roasters, and consumers around the world.
5.1 Collaborative research for coffee variety improvement
Many commercially cultivated C. arabica varieties were selected and released through breeding efforts led by country-based private autonomous institutions, public-private partnerships, and national breeding programs. For instance, the high-yielding, CLR-resistant, and drought-tolerant Anacafe 14 variety was released in 2014 by the National Coffee Association of Guatemala (ANACAFE), a union institution established under the country’s Coffee Law in 1960. Cuscatleco and Parainema varieties resistant to CLR and some Meloidogyne spp. nematodes were selected by the national breeding programs PROCAFE and IHCAFE, in El Salvador and Honduras, respectively.
In Brazil, Instituto Agronomico de Campinas (IAC), the country’s premier agricultural research institution established in 1887, has been instrumental in the selection and development of key Brazilian C. arabica commercial varieties. Some representative examples include Mundo Novo, Caturra, Catuai, Icatu, Tupi, Obata, IAPAR-59, and their derivative cultivars registered individually by the National Register of Cultivars (RNC). Similarly in Kenya, Coffee Research Foundation (now Kenya Agricultural and Livestock Research Organization— Coffee Research Institute, KALRO–CRI) developed and released the commonly cultivated compact (Ruiru 11) and tall (Batian) varieties in 1985 and 2010, respectively. These high-yielding varieties not only produce good cup quality but also exhibit resistance to CBD and tolerance to CLR. Particularly, the development of Ruiru 11 in Kenya was a reaction to a CBD epidemic in 1968, which resulted in a 50% loss in coffee production, threatening to cripple the country’s coffee sector. In India, the Central Coffee Research Institute (CCRI) has released 13 C. arabica selections (Selection-1–13), in targeted breeding efforts to build durable resistance to CLR, anthracnose, and other diseases (International Coffee Organization, 2006).
A few commercial C. arabica varieties have been released through collaborative breeding efforts. For instance, Casiopea, a high-yielding F1 hybrid resulting from a cross between Caturra and an Ethiopian wild accession (ET41) was developed by a consortium comprising of CIRAD, CATIE, and PROMECAFE (ICAFE-IHCAFE-PROCAFE-ANACAFE). H1 Centroamericano, another high-yielding F1 hybrid with resistance to CLR, was also made available to Central American coffee farmers in 2010 by the same consortium (Turreira-García, 2022). Other notable F1 hybrids developed through this consortium include H3 and Milenio. Additionally, CIRAD has collaborated with ECOM Agroindustrial Corp. Ltd., with the public-private partnership resulting in the development of the introgressed variety Marsellesa, and F1 hybrids Mundo Maya, Nayarita, and Starmaya (World Coffee Research, 2024a).
There is consensus that the nature of the coffee crop makes it impractical to centrally develop and globally distribute improved varieties. This essentially necessitates the strengthening of national and regional programs aimed at the breeding and multiplication of novel varieties adapted to local conditions (Krishnan, 2022). However, the success of these programs lies in the availability, access, and utilization of diverse coffee genetic resources, currently harbored in isolated field genebanks around the world, forests, farmers’ fields, and a few protected areas at the origin center of diversity. In a proposed global strategy for the conservation of coffee genetic resources (Bramel et al., 2017) examined the status of existing ex-situ collections and outlined priority actions toward establishing a secure, cost-effective, and sustainable system based on collective responsibility and collaboration. These include securing stable funding, upgrading facilities and capacity of collections, adopting favorable access and benefit sharing (ABS) policies, fostering global collaboration, safety duplication of conserved accessions, and enhancing complementarity of ex-situ and in situ conservation of coffee genetic resources (Bramel et al., 2017).
Over the past decade, there has been a growing interest among coffee value chain stakeholders to synergize efforts in accelerating agricultural innovation for sustainable production. A notable example is the coffee research and development work being led by World Coffee Research (WCR)—an industry-driven organization founded in 2012 by the global coffee industry. With a current membership of over 170 companies representing 29 coffee-growing countries, WCR embodies the partnerships required to safeguard the future of coffee. In its current operational model, WCR has identified 11 focus countries in East Africa (Kenya, Uganda, and Ethiopia), Asia (India, Indonesia, and Papua New Guinea), and in Latin America (Peru, Nicaragua, Honduras, Guatemala, and Mexico) where primary support to improve competitiveness in the global market is being targeted (World Coffee Research, 2024b).
Arguably, the WCR collaboration model is poised for success mostly because it breaks the barriers of isolated country-centric efforts that have dominated coffee breeding in the past, achieving only modest gains. Working together, collaborating coffee-producing countries will have access to a wider pool of breeding populations and modern breeding tools, thereby accelerating the pace of coffee research and development, and delivering to farmers and consumers varieties suited to their needs. By fostering a global network for advancing innovation in coffee, the WCR model demonstrates the importance of collaboration in the conservation of coffee origin diversity and strategic utilization of genetic resources to develop improved varieties that exhibit high yield, exceptional cup quality, adaptability to the changing climate, and durable resistance to pests and diseases.
5.2 International multi-location coffee variety testing
The agronomic performance of coffee is affected not only by genotype, but also by the environmental factors prevailing in the cultivation region, and genotype by environment interaction (GxE). By evaluating 17 C. arabica genotypes in 12 environments across three major coffee-growing agroecological areas in south Ethiopia, Gebreselassie et al. (2023) reported significant variations in yield attributed to the effect of environment and GxE. Similarly, Akpertey et al. (2023) reported the presence of significant effects of environment and GxE on growth and yield traits in 39 C. canephora hybrids evaluated in two agro-ecological zones in Ghana.
Employing a Bayesian approach to model the interaction of coffee genotypes with the environment using a bisegmented regression, Partelli et al. (2022) identified stable and adapted C. canephora genotypes from a pool of 43 genotypes mainly pre-selected by coffee farmers in the state of Espírito Santo, Brazil. From the same genotype pool, Covre et al. (2022) used the Bayesian approach to recommend five most productive genotypes evaluated in two environments in Brazil—south of Bahia and north of Espírito Santo—between the year 2016 and 2019. Such findings underscore the importance of selecting coffee genotypes with broad adaptability, good stability, and high productivity to mitigate the influences of GxE interactions and enhance coffee production in diverse cultivation environments that characterizes the coffee belt. Overall, the Bayesian approach facilitated a more comprehensive understanding of the interaction between coffee genotypes and the environment, leading to valuable insights for coffee breeding and production (Covre et al., 2022; Partelli et al., 2022).
The effect of environment and GxE on trait stability across different agro-ecologies had also previously been reported in C. arabica (Wamatu et al., 2003; Belete et al., 2014; Marie et al., 2020) and C. canephora (Montagnon et al., 2000; Anim-Kwapong et al., 2011). This necessitates multi-environment trials (MET) to determine the extent of GxE on the performance of newly developed materials, genotype stability, and adaptability in diverse agro-ecologies before varieties can be released for commercial cultivation in target locations. This is more so important considering the current and projected reality of climate variability in the major coffee-growing regions (DaMatta et al., 2018).
Establishing and maintaining MET is a costly yet essential step in coffee breeding. Identifying high-performing cultivars adaptable to diverse agro-ecologies is paramount. Farmers need to be provided with varieties tested and proven to perform optimally in their area. Unfortunately, most national breeding programs are not adequately equipped with the facilities and stable funds required to conduct MET on novel genotypes for several years before they can be released. Therefore, there is an urgent need for strengthening MET in coffee breeding programs, primarily through mobilization for stable, long-term resources from governments and research and development partners including the private sector.
In 2015, the WCR initiated an International Multi-location Variety Trial (IMLVT) program to evaluate the performance of selected varieties in various climates across the world. To date, this pioneering initiative has collected 31 high-performing coffee varieties from 11 suppliers worldwide and distributed them for long-term evaluation across 29 research sites in 18 coffee-growing countries (World Coffee Research, 2022). Initial aggregated global performance data on yield, disease resistance, and cup quality has been shared with country partners to identify their top-performing varieties and inform possible new variety introduction. Upon completion, data from the IMLVT is expected to provide crucial insights on GxE effects on variety performance, laying a foundation for future coffee research and breeding for climate resilience (World Coffee Research, 2022). Given the limited capacity of individual national breeding programs working in isolation to generate comprehensive MET data, access to such information and collaboration with similar initiatives to evaluate the performance of both local and global varieties will facilitate the process of delivering to farmers varieties suited to country-specific local conditions.
6 Gains for emerging and reviving coffee-producing regions
Although coffee is cultivated in more than 80 countries, the top five producers contribute 74.39% of the overall global output (International Coffee Organization, 2023). This not only concentrates major benefits of coffee export revenue within a few countries but also presents a threat to the sustainability of global supply. If production and export are hampered in one of the major producers with a large market share, the ripple effects are likely to cascade throughout the entire global market. Furthermore, few sources of globally supplied coffee limit the diversity of unique flavors and profiles available to consumers. There is a need to close this gap by enhancing the competitiveness of more producers across the bean belt. This will not only mitigate risks in the global supply chain but also boost farmer profitability and export revenues for the countries. Emerging and reviving producers in Southeast Asia and Eastern Africa are among the potential primary beneficiaries of accelerated, continuous variety improvement through pre-competitive collaborative breeding.
6.1 Southeast Asia
Southeast Asia has emerged as a significant player in the world coffee market, contributing to both regional economies and the global coffee supply. Output from Southeast Asia contributed a large portion of the Asia and Oceania regional share of world coffee production that closed at 29.6% in the 2022/23 coffee year. Vietnam is the world’s second-largest coffee producer after Brazil, and the largest producer in Asia and Oceania. Moreover, it is the leading global producer of Robusta coffee, contributing 37.5% of the total Robusta production globally during the 2022/23 period. In the same year, Vietnam produced a total of 29.2 million bags, a 9.8% decrease from the previous year, mainly attributed to prolonged rains in the major coffee-producing provinces, limited fertilizer usage and farmers shifting to cultivating more profitable crops. Nonetheless, with favorable climatic conditions, better selling prices and robust government support, Vietnam is expected to maintain and advance its position as a global coffee powerhouse. Coming second in the region is Indonesia which boasts a well-established coffee tradition, and world-renowned unique profiles and flavors from regions such as Sumatra and Java. In the 2022/23 coffee year, Indonesia produced 12.0 million bags, a 2.4% increase from the previous year owing to expansion of area under coffee by net 71,000 ha between 2018 and 2022 (International Coffee Organization, 2023).
Other significant producers in southeast Asia include Malaysia, Laos, Thailand, and the Philippines. During the period between the 2019/20 and 2022/23 coffee years, Malaysia and Thailand averaged a steady output of 1.98 and 0.66 million bags, respectively (United States Department of Agriculture, 2024). Over the last two decades, Laos has recorded a surge in coffee production. The period between 2000/01 and 2018/19 saw the country triple its production, growing at an average of 11.4% per annum, and consequently overtaking Thailand and the Philippines. During the 2022/23 production year, Laos produced 0.8 million bags of coffee, recording an increase from the 0.7 million bags produced in the previous year (International Coffee Organization, 2023). In the Philippines, coffee has been a cultural heritage crop since the 1800s. In the early 1880s, the country was the world’s fourth-largest coffee exporter. However, in 1892, the industry was severely hit by a CLR outbreak sweeping across the Indian Ocean basin and has since struggled to bounce back to its former glory (McCook, 2019). In the period between the 2019/20 and 2022/23 coffee years, the Philippines averaged an output of 0.45 million bags per annum (United States Department of Agriculture, 2024).
Some of the main challenges facing coffee growers in Southeast Asia include the worsening effects of climate change including erratic rainfall and fluctuating temperatures, pests, and diseases, rising cost of production owing to high prices of farm inputs and hired labor, and volatility in market prices. Future projection models based on representative concentration pathways (RCP) predict significant changes in the suitability of important coffee production regions in Southeast Asia. For instance, under RCP 6.0 scenario, the dominant Vietnamese C. canephora production regions lose suitability by the year 2050 owing to the negative impacts of climate change (Bunn et al., 2015a). This calls for expedited development and adoption of climate-resilient varieties to secure the future of region competitiveness, farmer profitability, and commodity supply to the global market. Particularly, there is a need to incorporate strategic stakeholder engagement and needs assessment in breeding programs. This way, farmers, processors, roasters, and consumers have an opportunity to co-develop future varieties that respond to their unique needs.
Coffee-growing countries in Southeast Asia, including up-and-coming (e.g., Thailand and Laos) reviving (e.g., Philippines), and progressive (e.g., Vietnam and Indonesia) producers have an opportunity to boost their productivity and competitiveness in the face of climate variability and the changing consumer preferences. By embracing (a) pre-competitive collaborative breeding for variety improvement—based on the mutual sharing of germplasm, expertise, and modern technologies, (b) user-driven variety development that responds to the needs of both the farmers and the industry’s downstream (researchers, processors, roasters, consumers), and (c) sustainable farming practices, the region could see a continued increase in productivity, farmer profitability, share of the global market, and contribution of coffee export revenue to the regional economies.
6.2 Eastern Africa
Eastern Africa is an important coffee production region and will continue playing a vital role in responding to the current and future dynamics in the global coffee industry. In addition to being host to a rich coffee genetic diversity, the region comprises expansive agroecological zones suitable for the cultivation of both C. arabica and C. canephora. Coffee production in Eastern Africa not only plays a significant role in the preservation of cultural heritage but also supports millions of livelihoods and contributes to economic growth through foreign exchange earnings. Nevertheless, the region’s coffee sector grapples with persistent challenges relating to the impacts of climate change, pests and diseases, aging coffee trees, lack of improved varieties, rising cost of production, land fragmentation, limited infrastructure, and market fluctuations. These challenges are increasingly impacting crop productivity, farmer profitability, and the region’s ability to maintain competitiveness in the global market.
During the 2022/23 coffee year, only two Eastern African countries—Ethiopia and Uganda—made the top 10 global coffee producers, producing 7.73 and 5.6 million bags, respectively. Ethiopia recorded a 1.0% increase in coffee output, reflecting a consistent upward trend in production in recent years. This growth was largely attributed to the expansion of coffee cultivation areas in the country, and increased adoption of good agronomic practices. Conversely, Uganda experienced a 6.8% drop in output, a second consecutive annual decrease in production attributed to drought conditions affecting key coffee-growing areas (International Coffee Organization, 2023). This has a direct socio-economic implication given that coffee is the biggest export commodity in Uganda, cultivated by an estimated one million smallholder farmers (Wang et al., 2015). Trailing farther behind in the region are Tanzania and Kenya, with an output of 1.20 and 0.75 million bags in 2022/23, respectively (United States Department of Agriculture, 2024).
While each of these countries encounters several distinct setbacks unique to their respective coffee sectors, a common overarching challenge is apparent: the slow pace of development and adoption of improved coffee varieties suited for the changing climate and the rising global demand for higher quality coffee. This situation is closely related to weakly supported national coffee breeding programs usually characterized by limited capacity and unstable funding. In the past, limited collaboration, and lack of ABS policies to govern multilateral germplasm sharing have resulted in the programs having little to no access to modern breeding technologies developed elsewhere, and diverse germplasm for use as novel sources of farmer/industry desired traits. Yet, there is a growing common understanding that any efforts to build sustainability of the coffee industry in the region must incorporate efficient development and widespread adoption of improved varieties that address the needs of all the coffee value chain stakeholders.
For instance, Ayele et al. (2021) recommended the use of improved varieties and good agronomic practices, as opposed to increasing the area under coffee to increase and sustain coffee production in Ethiopia. In Uganda, a recent survey of wild coffee species identified three indigenous species, presenting useful genetic resources for utilization in variety improvement for climate adaptation, pest, and disease resistance, better agronomic performance, and market differentiation (Davis et al., 2023). Such wild genetic resources are scattered in forests across Eastern Africa, some of which remain uncharacterized. Their conservation and utilization in breeding efforts hold potential in safeguarding the future of coffee in the region and beyond.
One potential model for achieving sustainability of coffee in Eastern Africa is through adopting pre-competitive collaborative breeding for variety improvement that encompasses mutually beneficial multilateral sharing of germplasm, information on accessions, breeding technologies, and data from multi-location trials. In such a model, participating countries would have access to a pool of diverse genetic sources of traits required to boost productivity in the respective growing environments. Working together, the countries could significantly cut the time required to continually develop and disseminate improved varieties tailored to the unique needs of their farmers and markets. With proper ABS mechanisms, collaborating countries can acquire what they need to enhance their regional and global market competitiveness, while ensuring that benefits from the utilization of imported genetic resources are shared fairly and equitably.
7 Conclusion and future outlook
The sustainability of future global coffee supply is under threat. In the last decade, both production and consumption have been on the rise, with increasingly more years recording annual global deficit than surplus (International Coffee Organization, 2023; United States Department of Agriculture, 2024). Under a business-as-usual scenario, this deficit is expected to grow significantly within the decade as global demand increases while production continues to grapple with recurrent intractable challenges (Krishnan, 2022). As the effects of climate change worsen, output in major production regions will be affected more frequently in the future than we have witnessed in the past. Recurrent droughts, extreme temperatures, and excessive rainfall will continue to affect both yield stability and bean quality. Furthermore, climatic change will trigger increased spread, infestation, and severity of coffee pests and diseases. To aggravate this scenario, coffee farmers are increasingly faced with rising costs of production owing to high prices of fertilizer, pesticides, and hired labor. Additionally, labor shortages present an emerging challenge due to aging farmers, international border regulations, labor force competition by other industries, and unforeseen shocks such as the recent COVID-19 pandemic (Rhiney et al., 2021).
The current breeding strategies in most producing countries are grossly out of touch with the urgency to develop and disseminate improved “climate-change ready” varieties suitable for the changing environment and the global market demand for higher quality. The sustainability of the coffee industry is, now more than ever, intrinsically linked to our ability to anticipate and respond to the changing climate, supply chain disruptions, and market dynamics, effectively addressing the needs of farmers, processors, roasters, and consumers alike. Since coffee has a long breeding cycle, the foundation for breeding-dependent solutions to future challenges must be strategically laid now. Going forward, we believe that coffee-producing countries will significantly benefit from:
I. Embracing pre-competitive collaborative breeding strategy for variety improvement based on mutually beneficial sharing of germplasm sources of variability and novel traits, knowledge, expertise, and breeding tools (Figure 5).
II. Wide adoption of genomics-assisted breeding to accelerate the process of delivering superior varieties that meets the needs of farmers and the post-harvest coffee industry players alike.
III. Participation in cross-border multi-environment trials for local and global varieties, sharing performance data to inform potential introduction of varieties suited to country-specific local conditions (Figure 5).
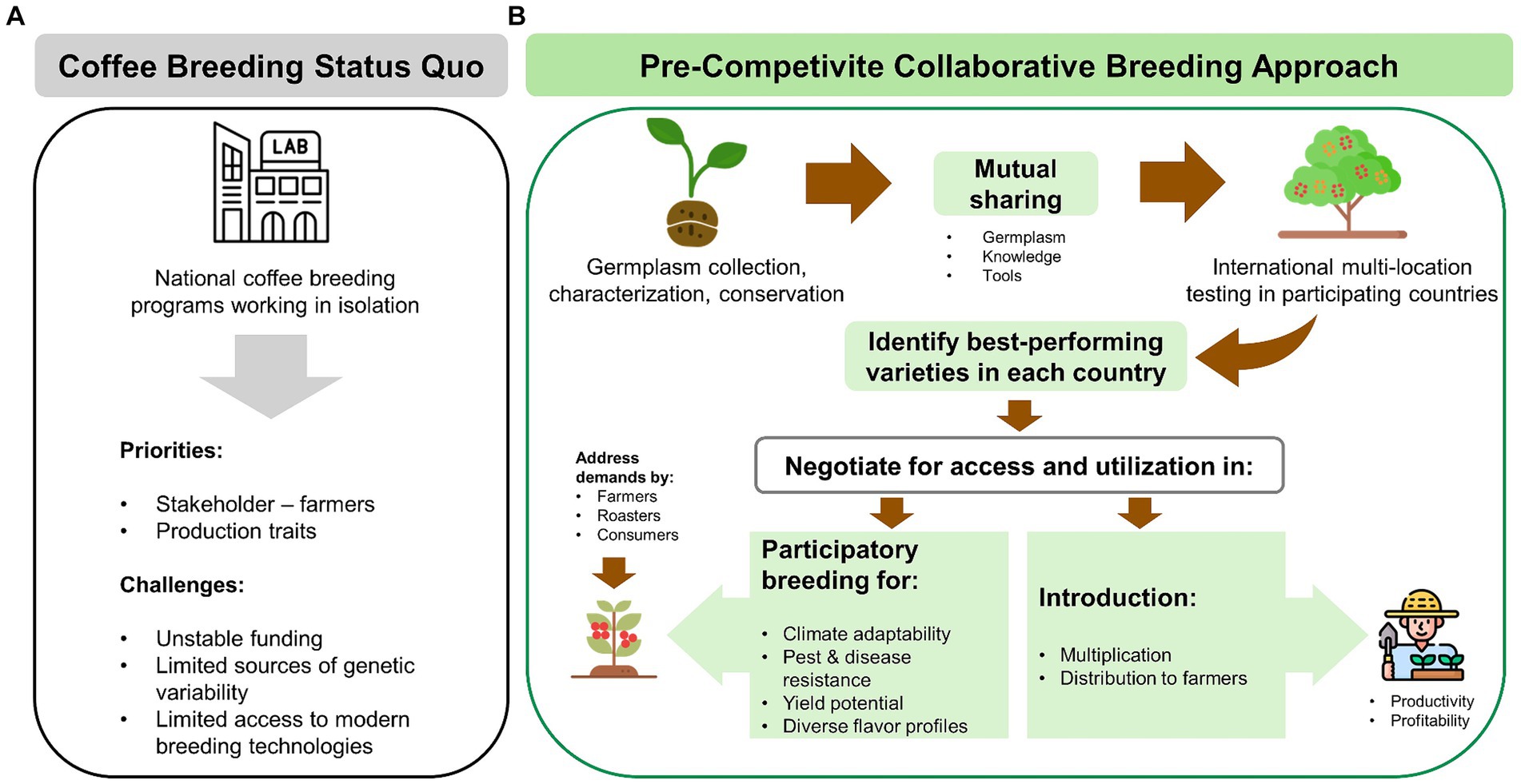
Figure 5. (A) Status quo of most national coffee breeding programs and their major challenges. (B) A pre-competitive, collaborative breeding model based on mutual sharing of germplasm, multi-location variety testing, and incorporation of priorities by farmers, roasters, and consumers in the development of future coffee varieties (this figure was designed using icons from flaticon.com).
Emerging and reviving coffee-producing countries in Southeast Asia and Eastern Africa are among the potential primary beneficiaries of a shift in breeding approach from the historically isolated country-centric systems to a more forward-looking collaborative approach. While the former has struggled with limited germplasm and tools only available in the country, the latter promises to break these barriers by availing a wider pool of genetic variability and access to modern breeding tools. The resultant accelerated genetic gains from a more efficient collaborative breeding system will not only enhance productivity and farmer profitability but also increase countries’ foreign exchange earnings and competitiveness in the global market.
Author contributions
GMN: Visualization, Writing – original draft, Writing – review & editing. KNW: Conceptualization, Supervision, Writing – review & editing.
Funding
The author(s) declare that financial support was received for the research, authorship, and/or publication of this article. The authors acknowledged the Plant Transgenic Design Initiative (PTraD) of the Tsukuba-Plant Innovation Research Center (T-PIRC), University of Tsukuba, Ibaraki, Japan, for providing facility resources to conduct this work.
Acknowledgments
We acknowledge Adchariya Jeatrakul, from Lanna Roastery, Nonthaburi, Thailand, and Kyi Sein, from Advanced Agricultural Engineering, Yangon, Myanmar, for sharing insights on local coffee cultivation and post-harvest processing in southeast Asia.
Conflict of interest
The authors declare that the research was conducted in the absence of any commercial or financial relationships that could be construed as a potential conflict of interest.
Publisher’s note
All claims expressed in this article are solely those of the authors and do not necessarily represent those of their affiliated organizations, or those of the publisher, the editors and the reviewers. Any product that may be evaluated in this article, or claim that may be made by its manufacturer, is not guaranteed or endorsed by the publisher.
References
Acevedo, F. E., Jiménez, M., Pimentel, J. P., and Benavides, P. (2019). Spatial distribution of mealybugs (Hemiptera: Coccomorpha: Coccoidea) in the root system of pruned and non-pruned Coffea arabica trees. J. Econ. Entomol. 113, 172–184. doi: 10.1093/jee/toz242
Aga, E., Bekele, E., and Bryngelsson, T. (2005). Inter-simple sequence repeat (ISSR) variation in forest coffee trees (Coffea arabica L.) populations from Ethiopia. Genetica 124, 213–221. doi: 10.1007/s10709-005-1484-6
Aguilar, M. E., Wang, X., Escalona, M., Yan, L., and Huang, L. (2022). Somatic embryogenesis of Arabica coffee in temporary immersion culture: advances, limitations, and perspectives for mass propagation of selected genotypes. Front. Plant Sci. 13:994578. doi: 10.3389/fpls.2022.994578
Ahmed, A. G., Murungi, L. K., and Babin, R. (2016). Developmental biology and demographic parameters of antestia bug Antestiopsis thunbergii (Hemiptera: Pentatomidae), on Coffea arabica (Rubiaceae) at different constant temperatures. Int. J. Trop. Insect Sci. 36, 119–127. doi: 10.1017/S1742758416000072
Akpertey, A., Anim-Kwapong, E., Adu-Gyamfi, P. K. K., Dadzie, A. M., Nyadanu, D., and Ofori, A. (2023). Exploring genotype × environment interaction in Robusta coffee for growth and yield stability under tropical environments. J. Crop. Sci. Biotechnol. 26, 179–197. doi: 10.1007/s12892-022-00171-3
Akpertey, A., Padi, F. K., Meinhardt, L., and Zhang, D. (2021). Effectiveness of single nucleotide polymorphism markers in genotyping germplasm collections of Coffea canephora using KASP assay. Front. Plant Sci. 11:612593. doi: 10.3389/fpls.2020.612593
Alemu, T. (2012). A review of coffee wilt disease, GIBBERELLA XYLARIOIDES (FUSARIUM XYLARIOIDES) in Africa with special reference to Ethiopia. Ethiop. J. Biol. Sci 11, 65–103.
Anim-Kwapong, E., Anim-Kwapong, G., and Adomako, B. (2011). Variation and association among characters genetically related to yield and yield stability in Coffea canephora genotypes. J. Plant Breed. Crop. Sci 3, 311–320. doi: 10.5897/JPBCS11.054
Anthony, F., Combes, M., Astorga, C., Bertrand, B., Graziosi, G., and Lashermes, P. (2002). The origin of cultivated Coffea arabica L. varieties revealed by AFLP and SSR markers. Theor. Appl. Genet. 104, 894–900. doi: 10.1007/s00122-001-0798-8
Avelino, J., Allinne, C., Cerda, R., Willocquet, L., and Savary, S. (2018). Multiple-disease system in coffee: from crop loss assessment to sustainable management. Annu. Rev. Phytopathol. 56, 611–635. doi: 10.1146/annurev-phyto-080417-050117
Ayele, A., Worku, M., and Bekele, Y. (2021). Trend, instability and decomposition analysis of coffee production in Ethiopia (1993–2019). Heliyon 7:e08022. doi: 10.1016/j.heliyon.2021.e08022
Azevedo De Paula, P. V. A., Pozza, E. A., Santos, L. A., Chaves, E., Maciel, M. P., and Paula, J. C. A. (2016). Diagrammatic scales for assessing Brown eye spot (Cercospora coffeicola) in red and yellow coffee cherries. J. Phytopathol. 164, 791–800. doi: 10.1111/jph.12499
Badel, J. L., and Zambolim, L. (2019). Coffee bacterial diseases: a plethora of scientific opportunities. Plant Pathol. 68, 411–425. doi: 10.1111/ppa.12966
Barrera, J. F. (2008). “Coffee pests and their management” in Encyclopedia of entomology. ed. J. L. Capinera (Dordrecht: Springer Netherlands), 961–998.
Barreto Peixoto, J. A., Silva, J. F., Oliveira, M. B. P. P., and Alves, R. C. (2023). Sustainability issues along the coffee chain: from the field to the cup. Comp. Rev. Food Sci. Food Safe 22, 287–332. doi: 10.1111/1541-4337.13069
Barros, A. F., Oliveira, R. D. L., Lima, I. M., Coutinho, R. R., Ferreira, A. O., and Costa, A. (2014). Root-knot nematodes, a growing problem for Conilon coffee in Espírito Santo state, Brazil. Crop Prot. 55, 74–79. doi: 10.1016/j.cropro.2013.10.004
Belete, Y., Belachew, B., and Fininsa, C. (2014). Performance evaluation of indigenous Arabica coffee genotypes across different environments. J. Plant Breed. Crop Sci. 6, 171–178. doi: 10.5897/JPBCS2014.0447
Bertrand, B., Anthony, F., and Lashermes, P. (2001). Breeding for resistance to Meloidogyne exigua in Coffea arabica by introgression of resistance genes of Coffea canephora. Plant Pathol. 50, 637–643. doi: 10.1046/j.1365-3059.2001.00597.x
Bigirimana, J., Uzayisenga, B., and Gut, L. J. (2019). Population distribution and density of Antestiopsis thunbergii (Hemiptera: Pentatomidae) in the coffee growing regions of Rwanda in relation to climatic variables. Crop Prot. 122, 136–141. doi: 10.1016/j.cropro.2019.04.029
Bilen, C., El Chami, D., Mereu, V., Trabucco, A., Marras, S., and Spano, D. (2022). A systematic review on the impacts of climate change on coffee agrosystems. Plan. Theory 12:102. doi: 10.3390/plants12010102
Bramel, P., Krishnan, S., Horna, D., Lainoff, B., and Montagnon, C. (2017). Global conservation strategy for coffee genetic resources. Bonn, Germany: The Crop Trust and World Coffee Research.
Bunn, C., Läderach, P., Ovalle Rivera, O., and Kirschke, D. (2015a). A bitter cup: climate change profile of global production of Arabica and Robusta coffee. Clim. Chang. 129, 89–101. doi: 10.1007/s10584-014-1306-x
Bunn, C., Läderach, P., Pérez Jimenez, J. G., Montagnon, C., and Schilling, T. (2015b). Multiclass classification of agro-ecological zones for Arabica coffee: an improved understanding of the impacts of climate change. PLoS One 10:e0140490. doi: 10.1371/journal.pone.0140490
Campos, V., Sivapalan, P., and Gnanapragasam, N. (1990). Nematode parasites of coffee, cocoa and tea. In: Plant parasitic nematodes in subtropical and tropical agriculture. eds. M. Luc, R. A. Sikora, and J. Bridge. Wallingford, UK: CAB International, 387–430.
Campos, V. P., and Villain, L. (2005). “Nematode parasites of coffee and cocoa” in Plant parasitic nematodes in subtropical and tropical agriculture (Wallingford: CABI Publishing), 529–579.
Carvalho (1985). “Principles and pratice of coffee plant breeding for productivity and quality factors” in Coffee-botany, biochemistry and production of beans and beverage. eds. M. N. Clinffort and K. C. Willson (London & Sidney: Croom Helm), 48–96.
Carvalho, A. (1988). Principles and practice of coffee plant breeding for productivity and quality factors: Coffea arabica. Coffee 4, 129–165.
Carvalho, C. F., Carvalho, S. M., and Souza, B. (2019). “Coffee” in Natural enemies of insect pests in neotropical agroecosystems: biological control and functional biodiversity. eds. B. Souza, L. L. Vázquez, and R. C. Marucci (Cham: Springer International Publishing), 277–291.
Carvalho, A., Teixeira, A. A., Fazuoli, L. C., and Guerreiro Filho, O. (1990). Qualidade da bebida em espécies e populações derivadas de híbridos interespecíficos de Coffea. Bragantia 49, 281–290. doi: 10.1590/S0006-87051990000200009
Charrier, A., and Berthaud, J. (1985). “Botanical classification of coffee” in Coffee: botany, biochemistry and production of beans and beverage. Eds. M. N. Clinffort and K. C. Willson (Boston, MA: Springer), 13–47.
Charrier, A., and Berthaud, J. (1990). Use and value of genetic resources of Coffea for breeding and their long-term conservation. Mitt. Inst. Allg. Bot. Hamburg, 23a, 53–64.
Covre, A. M., Da Silva, F. A., Oliosi, G., Correa, C. C. G., Viana, A. P., and Partelli, F. L. (2022). Multi-environment and multi-year Bayesian analysis approach in coffee canephora. Plan. Theory 11:3274. doi: 10.3390/plants11233274
Cristancho, M. A., Rozo, Y., Escobar, C., Rivillas, C. A., and Gaitán, A. L. (2012). Outbreak of coffee leaf rust Hemileia vastatrix in Colombia. New Dis. Rep. 25:19. doi: 10.5197/j.2044-0588.2012.025.019
Crowe, T. (2009). Coffee pests in Africa: coffee: growing, processing, sustainable production: a guidebook for growers, processors, traders and researchers. 2nd Ed. Ed. J. N. Wintgens. (KGaA, Weinheim: Wiley-VCH Verlag GmbH & Co), 425–462.
Da Rocha, J. G., Zambolim, L., Maciel-Zambolim, E., and Do Vale, F. X. R. (2010). Temporal and spatial dynamics of coffee leaf scorch caused by Xylella fastidiosa. Austr. Plant Pathol. 39:234. doi: 10.1071/AP09088
da Silva, B. S. R., Sant’Ana, G. C., Chaves, C. L., Godoy Androcioli, L., Ferreira, R. V., Sera, G. H., et al. (2019). Population structure and genetic relationships between Ethiopian and Brazilian Coffea arabica genotypes revealed by SSR markers. Genetica 147, 205–216. doi: 10.1007/s10709-019-00064-4
DaMatta, F. M., Avila, R. T., Cardoso, A. A., Martins, S. C. V., and Ramalho, J. C. (2018). Physiological and agronomic performance of the coffee crop in the context of climate change and global warming: a review. J. Agric. Food Chem. 66, 5264–5274. doi: 10.1021/acs.jafc.7b04537
DaMatta, F. M., and Ramalho, J. D. C. (2006). Impacts of drought and temperature stress on coffee physiology and production: a review. Braz. J. Plant Physiol. 18, 55–81. doi: 10.1590/S1677-04202006000100006
Davis, A. P., Kiwuka, C., Faruk, A., Mulumba, J., and Kalema, J. (2023). A review of the indigenous coffee resources of Uganda and their potential for coffee sector sustainability and development. Front. Plant Sci. 13:1057317. doi: 10.3389/fpls.2022.1057317
Davis, A. P., Kiwuka, C., Faruk, A., Walubiri, M. J., and Kalema, J. (2022). The re-emergence of Liberica coffee as a major crop plant. Nat. Plants 8, 1322–1328. doi: 10.1038/s41477-022-01309-5
Davis, A. P., and Rakotonasolo, F. (2021). Six new species of coffee (Coffea) from northern Madagascar. Kew Bull. 76, 497–511. doi: 10.1007/s12225-021-09952-5
de Lima, R. F., de Oliveira Aparecido, L. E., Torsoni, G. B., Chiquitto, A. G., Moraes, J. R., and de Souza Rolim, G. (2022). Agroclimatic zoning for the incidence of brown eye spot on coffee under climate change scenarios. Theor. Appl. Climatol. 149, 1471–1496. doi: 10.1007/s00704-022-04123-3
De Lima, J. E. O., Miranda, V. S., Hartung, J. S., Brlansky, R. H., Coutinho, A., Roberto, S. R., et al. (1998). Coffee leaf scorch bacterium: axenic culture, pathogenicity, and comparison with Xylella fastidiosa of Citrus. Plant Dis. 82, 94–97. doi: 10.1094/PDIS.1998.82.1.94
Denoeud, F., Carretero-Paulet, L., Dereeper, A., Droc, G., Guyot, R., Pietrella, M., et al. (2014). The coffee genome provides insight into the convergent evolution of caffeine biosynthesis. Science 345, 1181–1184. doi: 10.1126/science.1255274
Duque, H., and Baker, P. S. (2003). Devouring profit the socio-economics of coffee berry borer IPM. Available at: http://hdl.handle.net/10778/4331
Eskes, A. B., and Leroy, T. (2004). “Coffee selection and breeding” in Coffee: growing, processing, sustainable production: A guidebook for growers, processors, traders, and researchers. Ed. J. N. Wintgens. (Weinheim: WILEY-VCH Verlag GmbH & Co. KGaA), 57–86.
Etienne, H., Breton, D., Breitler, J.-C., Bertrand, B., Déchamp, E., Awada, R., et al. (2018). Coffee somatic embryogenesis: how did research, experience gained and innovations promote the commercial propagation of elite clones from the two cultivated species? Front. Plant Sci. 9:1630. doi: 10.3389/fpls.2018.01630
Evenson, R. E., and Gollin, D. (2003). Assessing the impact of the green revolution, 1960 to 2000. Science 300, 758–762. doi: 10.1126/science.1078710
Figueres, R. (1940). Sur une maladie très grave du caféier en Oubangui. Ministère des Colonies, Paris, France: Rapport.
Flood, J. (2021). “Coffee wilt disease” in Burleigh Dodds series in agricultural science, eds. CATIE and R. Muschler (Costa Rica: Burleigh Dodds Science Publishing).
Garavito, A., Montagnon, C., Guyot, R., and Bertrand, B. (2016). Identification by the DArTseq method of the genetic origin of the Coffea canephora cultivated in Vietnam and Mexico. BMC Plant Biol. 16:242. doi: 10.1186/s12870-016-0933-y
Gebreselassie, H., Tesfaye, B., Gedebo, A., and Tolessa, K. (2023). Genotype by environment interaction and stability analysis using AMMI and GGE-biplot models for yield of Arabica coffee genotypes in South Ethiopia. J. Crop. Sci. Biotechnol. 27, 65–77. doi: 10.1007/s12892-023-00213-4
Gemech, F., and Struthers, J. (2007). Coffee price volatility in Ethiopia: effects of market reform programmes. J. Int. Dev. 19, 1131–1142. doi: 10.1002/jid.1389
Ghini, R., Hamada, E., Pedro Júnior, M. J., Marengo, J. A., and Gonçalves, R. R. V. (2008). Risk analysis of climate change on coffee nematodes and leaf miner in Brazil. Pesq. Agropec. Bras. 43, 187–194. doi: 10.1590/S0100-204X2008000200005
Giddegowda Venkatesha, M., and Dinesh, A. S. (2012). The coffee white stemborer Xylotrechus quadripes (Coleoptera: Cerambycidae): bioecology, status and management. Int. J. Trop. Insect Sci. 32, 177–188. doi: 10.1017/S1742758412000331
Gil, Z., Benavides, P., and Constantino, L. (2016). Hemiptera: Coccoidea de las raíces del café y sus parasitoides en el Valle del Cauca. Available at: http://hdl.handle.net/10778/680
Gomez, C., Dussert, S., Hamon, P., Hamon, S., De Kochko, A., and Poncet, V. (2009). Current genetic differentiation of Coffea canephorapierre ex a. Froehn in the Guineo-Congolian African zone: cumulative impact of ancient climatic changes and recent human activities. BMC Evol. Biol. 9:167. doi: 10.1186/1471-2148-9-167
Góngora, C. E., Gil, Z. N., Constantino, L. M., and Benavides, P. (2023). Sustainable strategies for the control of pests in coffee crops. Agronomy 13:2940. doi: 10.3390/agronomy13122940
Granados-Montero, M. D. M., Avelino, J., Arauz-Cavallini, F., Castro-Tanzi, S., and Ureña, N. (2020). Hojarasca e inóculo de Mycena citricolor sobre la epidemia de ojo de gallo. Agron. Mesoam., 31, 77–94. doi: 10.15517/am.v31i1.36614
Griffith, D., Zamudio Grave, P., Cortés Viveros, R., and Cabrera Cabrera, J. (2017). Losing labor: coffee, migration, and economic change in Veracruz, Mexico. Cult. Agric. Food Environ. 39, 35–42. doi: 10.1111/cuag.12086
Guerreiro Filho, O. (2006). Coffee leaf miner resistance. Braz. J. Plant Physiol. 18, 109–117. doi: 10.1590/S1677-04202006000100009
Guido, Z., Knudson, C., and Rhiney, K. (2020). Will COVID-19 be one shock too many for smallholder coffee livelihoods? World Dev. 136:105172. doi: 10.1016/j.worlddev.2020.105172
Hakiza, G., Kyetere, D., Musoli, P., Wetala, P., Njuki, J., Kucel, P., et al. (2010). “Coffee wilt disease in Uganda” in Coffee wilt disease (Wallingford: CABI), 28–49.
Hall, D. R., Cork, A., Phythian, S. J., Chittamuru, S., Jayarama, B. K., Venkatesha, M. G., et al. (2006). Identification of components of male-produced pheromone of coffee white Stemborer, Xylotrechus quadripes. J. Chem. Ecol. 32, 195–219. doi: 10.1007/s10886-006-9360-0
Herrera, J. C., and Lambot, C. (2017). “The coffee tree—genetic diversity and origin” in The craft and science of coffee. Ed. B. Folmer (New York and London: Elsevier), 1–16.
Hindorf, H., and Omondi, C. O. (2011). A review of three major fungal diseases of Coffea arabica L. in the rainforests of Ethiopia and progress in breeding for resistance in Kenya. J. Adv. Res. 2, 109–120. doi: 10.1016/j.jare.2010.08.006
International Coffee Organization (2006). Breeding coffee plants with durable resistance to coffee leaf rust, anthracnose and other diseases. London. Available at: https://www.ico.org/documents/wpboard979r1e.pdf (Accessed May 10, 2024).
International Coffee Organization (2013). Report on the outbreak of coffee leaf rust in Central America and action plan to combat the pest. International coffee organization. Available at: https://www.ico.org/documents/cy2012-13/ed-2157e-report-clr.pdf (Accessed May 10, 2024).
International Coffee Organization (2023). Coffee report and outlook (CRO). Available at: https://icocoffee.org/documents/cy2023-24/Coffee_Report_and_Outlook_December_2023_ICO.pdf (Accessed May 7, 2024).
Jaramillo, J., Borgemeister, C., and Baker, P. (2006). Coffee berry borer Hypothenemus hampei (Coleoptera: Curculionidae): searching for sustainable control strategies. Bull. Entomol. Res. 96, 223–233. doi: 10.1079/BER2006434
Kawabata, A. M., and Nakamoto, S. T. (2021). Spraying to suppress coffee leaf rust (Hemileia vastatrix) in Hawaiʻi. Plant Dis. PD–118, College of Tropical Agriculture and Human Resources, University of Hawai‘i at Mānoa, Honolulu, HI, USA.
Kilambo, D., Mtenga, D., Nghoma, N., Ngomuo, R., Teri, J., and Mlwilo, B. (2015). A decade of contributing to a profitable and sustainable coffee industry in Tanzania: the Arabica and Robusta improvement Programmes. Am. J. Res. Commun. 3, 31–35.
Kranz, J., and Mogk, M. (1973). Gibberella xylarioides Heim et Saccas on arabica coffee in Ethiopia. Phytopathol. Z. 78, 365–366. doi: 10.1111/j.1439-0434.1973.tb04186.x
Krishnan, S. (2017). “Sustainable coffee production” in Oxford Research Encyclopedia of Environmental Science (New York, USA: Oxford University Press), 1–29.
Krishnan, S. (2022). “Coffee: genetic diversity, Erosion, conservation, and utilization” in Cash crops: genetic diversity, erosion, conservation and utilization. eds. P. M. Priyadarshan and S. M. Jain (Cham: Springer International Publishing), 55–80.
Kumar, V., Madhava Naidu, M., and Ravishankar, G. A. (2006). Developments in coffee biotechnology—in vitro plant propagation and crop improvement. Plant Cell Tissue Organ Cult. 87, 49–65. doi: 10.1007/s11240-006-9134-y
Laderach, P., Lundy, M., Jarvis, A., Ramirez, J., Portilla, E. P., Schepp, K., et al. (2011). “Predicted impact of climate change on coffee supply chains” in The economic, social and political elements of climate change. ed. W. L. Filho (Berlin, Heidelberg: Springer Berlin Heidelberg), 703–723.
Läderach, P., Ramirez-Villegas, J., Navarro-Racines, C., Zelaya, C., Martinez-Valle, A., and Jarvis, A. (2017). Climate change adaptation of coffee production in space and time. Clim. Chang. 141, 47–62. doi: 10.1007/s10584-016-1788-9
Lashermes, P., Combes, M.-C., Hueber, Y., Severac, D., and Dereeper, A. (2014). Genome rearrangements derived from homoeologous recombination following allopolyploidy speciation in coffee. Plant J. 78, 674–685. doi: 10.1111/tpj.12505
Lashermes, P., Combes, M. C., Topart, P., Graziosi, G., Bertrand, B., and Anthony, F. (2000). “Molecular breeding in coffee (Coffea Arabica L.)” in Coffee biotechnology and quality. eds. T. Sera, C. R. Soccol, A. Pandey, and S. Roussos (Dordrecht: Springer Netherlands), 101–112.
Lashermes, P., Trouslot, P., Anthony, F., Combes, M. C., and Charrier, A. (1996). Genetic diversity for RAPD markers between cultivated and wild accessions of Coffea arabica. Euphytica 87, 59–64. doi: 10.1007/BF00022965
Lewin, B., Giovannucci, D., and Varangis, P. (2004). Coffee markets: new paradigms in global supply and demand. Agriculture and Rural Development Discussion Paper No. 3, (Washington, DC: The World Bank), 1-133.
Magrach, A., and Ghazoul, J. (2015). Climate and Pest-driven geographic shifts in global coffee production: implications for forest cover, biodiversity and carbon storage. PLoS ONE 10:e0133071. doi: 10.1371/journal.pone.0133071
Maluf, M. P., Silvestrini, M., Ruggiero, L. M. D. C., Guerreiro Filho, O., and Colombo, C. A. (2005). Genetic diversity of cultivated Coffea arabica inbred lines assessed by RAPD, AFLP and SSR marker systems. Sci. Agric. 62, 366–373. doi: 10.1590/S0103-90162005000400010
Marengo, J., Espinoza, J. C., Bettolli, L., Cunha, A. P., Molina-Carpio, J., Skansi, M., et al. (2023). A cold wave of winter 2021 in Central South America: characteristics and impacts. Clim. Dyn. 61, 2599–2621. doi: 10.1007/s00382-023-06701-1
Marie, L., Abdallah, C., Campa, C., Courtel, P., Bordeaux, M., Navarini, L., et al. (2020). G × E interactions on yield and quality in Coffea arabica: new F1 hybrids outperform American cultivars. Euphytica 216:78. doi: 10.1007/s10681-020-02608-8
McCook, S. (2019). Coffee is not forever: a global history of the coffee leaf rust. Athens, OH: Ohio University Press.
McCook, S., and Montero-Mora, A. (2024). Coffee breeding in a time of crisis: F1 hybrids in Central America since 1990. Plants People Planet. 1–10. doi: 10.1002/ppp3.10480
McNutt, D. (1979). Control of Antestiopsis spp. on coffee in Uganda. Pans 25, 5–15. doi: 10.1080/09670877909411653
Mekbib, Y., Tesfaye, K., Dong, X., Saina, J. K., Hu, G.-W., and Wang, Q.-F. (2022). Whole-genome resequencing of Coffea arabica L. (Rubiaceae) genotypes identify SNP and unravels distinct groups showing a strong geographical pattern. BMC Plant Biol. 22:69. doi: 10.1186/s12870-022-03449-4
Millard, E. (2017). Still brewing: fostering sustainable coffee production. World Dev. Perspect. 7-8, 32–42. doi: 10.1016/j.wdp.2017.11.004
Mishra, M. K. (2019). “Genetic resources and breeding of coffee (Coffea spp.)” in Advances in plant breeding strategies: nut and beverage crops. eds. J. M. Al-Khayri, S. M. Jain, and D. V. Johnson (Cham: Springer International Publishing), 475–515.
Missio, R. F., Caixeta, E. T., Zambolim, E. M., Zambolim, L., and Sakiyama, N. S. (2009). Development and validation of SSR markers for Coffea arabica L. CBAB 9, 361–371. doi: 10.12702/1984-7033.v09n04a11
Montagnon, C., Cilas, C., Leroy, T., Yapo, A., and Charmetant, P. (2000). Genotype-location interactions for Coffea canephora yield in the Ivory Coast. Agronomie 20, 101–109. doi: 10.1051/agro:2000110
Montagnon, C., Leroy, T., and Yapo, A. (1992). Genotypic and phenotypic diversity of some coffee groups (Coffea canephora) in the collections. Consequences on their use in breeding. Café Cacao Thé (France). 36, 187–198.
Moose, S. P., and Mumm, R. H. (2008). Molecular plant breeding as the foundation for 21st century crop improvement. Plant Physiol. 147, 969–977. doi: 10.1104/pp.108.118232
Moreira, T. R., da Silva, S. F., da Silva, N. B., dos Santos, G. M. A. D. A., and dos Santos, A. R. (2021). “Global warming and the effects of climate change on coffee production” in Quality determinants in coffee production. eds. L. Louzada Pereira and T. Rizzo Moreira (Cham, Switzerland: Food Engineering Series. Springer 65–100.
Morris, J. R., and Perfecto, I. (2016). Testing the potential for ant predation of immature coffee berry borer (Hypothenemus hampei) life stages. Agric. Ecosyst. Environ. 233, 224–228. doi: 10.1016/j.agee.2016.09.018
Musoli, P., Cubry, P., Aluka, P., Billot, C., Dufour, M., De Bellis, F., et al. (2009). Genetic differentiation of wild and cultivated populations: diversity of Coffea canephora Pierre in Uganda. Genome 52, 634–646. doi: 10.1139/G09-037
Noir, S., Anthony, F., Bertrand, B., Combes, M.-C., and Lashermes, P. (2003). Identification of a major gene (Mex-1) from Coffea canephora conferring resistance to Meloidogyne exigua in Coffea arabica. Plant Pathol. 52, 97–103. doi: 10.1046/j.1365-3059.2003.00795.x
Olal, S., Olango, N., Kiggundu, A., Ochwo, S., Adriko, J., Nanteza, A., et al. (2018). Using translation elongation factor gene to specifically detect and diagnose Fusarium xylaroides, a causative agent of coffee wilt disease in Ethiopia, east and Central Africa. J Plant Pathol Microbiol 9:2. doi: 10.4172/2157-7471.1000440
Orozco-Castillo, C., Chalmers, K. J., Waugh, R., and Powell, W. (1994). Detection of genetic diversity and selective gene introgression in coffee using RAPD markers. Theoret. Appl. Genetics 87, 934–940. doi: 10.1007/BF00225787
Ovalle-Rivera, O., Läderach, P., Bunn, C., Obersteiner, M., and Schroth, G. (2015). Projected shifts in Coffea arabica suitability among major global producing regions due to climate change. PLoS One 10:e0124155. doi: 10.1371/journal.pone.0124155
Paillard, M., Lashermes, P., and Pétiard, V. (1996). Construction of a molecular linkage map in coffee. Theor. Appl. Genet. 93-93, 41–47. doi: 10.1007/BF00225725
Pandey, M., Kayastha, P., Khanal, S., Shrestha, S., Thakur, G., Adhikari, K., et al. (2022). An overview on possible management strategies for coffee white stem borer Xylotrechus quadripes Chevrolat (Coleoptera: Cerambycidae) in Nepal. Heliyon 8:e10445. doi: 10.1016/j.heliyon.2022.e10445
Paradela Filho, O., Sugimori, M., Ribeiro, I., Garcia, A. Jr., Beretta, M., Harakawa, R., et al. (1997). Constatação de Xylella fastidiosa em cafeeiro no Brasil. Summa Phytopathol. 23, 46–49.
Partelli, F. L., Da Silva, F. A., Covre, A. M., Oliosi, G., Correa, C. C. G., and Viana, A. P. (2022). Adaptability and stability of Coffea canephora to dynamic environments using the Bayesian approach. Sci. Rep. 12:11608. doi: 10.1038/s41598-022-15190-x
Peck, L. D., and Boa, E. (2024). Coffee wilt disease: the forgotten threat to coffee. Plant Pathol. 73, 506–521. doi: 10.1111/ppa.13833
Pereira, A., Sakiyama, N., Zambolim, L., Moura, W., Zambolim, E., and Caixeta, E. (2005). “Identification and use of sources of durable resistance to coffee leaf rust in the UFV/EPAMIG breeding program” in Durable resistance to coffee leaf rust. Eds. L. Zambolim, E. Maciel-Zambolim and V. M. P. Várzea (Viçosa: UFV), 215–232.
Pham, Y., Reardon-Smith, K., Mushtaq, S., and Cockfield, G. (2019). The impact of climate change and variability on coffee production: a systematic review. Clim. Chang. 156, 609–630. doi: 10.1007/s10584-019-02538-y
Phiri, N., and Baker, P. (2009). Coffee wilt in Africa: final technical report of the regional coffee wilt programme (2000–2007). Wallingford: CABI Publishing.
Ponte, S. (2002). The ‘latte revolution’? Regulation, markets and consumption in the global coffee chain. World Dev. 30, 1099–1122. doi: 10.1016/S0305-750X(02)00032-3
Prakash, N. S., Marques, D. V., Varzea, V. M. P., Silva, M. C., Combes, M. C., and Lashermes, P. (2004). Introgression molecular analysis of a leaf rust resistance gene from Coffea liberica into C. arabica L. Theor. Appl. Genet. 109, 1311–1317. doi: 10.1007/s00122-004-1748-z
Rhiney, K., Guido, Z., Knudson, C., Avelino, J., Bacon, C. M., Leclerc, G., et al. (2021). Epidemics and the future of coffee production. Proc. Natl. Acad. Sci. USA 118:e2023212118. doi: 10.1073/pnas.2023212118
Robbs, C., Kimura, O., Ribeiro, R., and Oyadomari, L. (1974). Bacterial leaf blight: a new disease of coffee (Coffea arabica L.) caused by Pseudomonas cichorii (Swingle) Stapp. Arquiv. Univ. Federal Rural do Rio de Janeiro 4, 1–5.
Rodrigues, L. M. R., Almeida, I. M. G., Patrício, F. R. A., Beriam, L. O. S., Maciel, K. W., Braghini, M. T., et al. (2017). Aggressiveness of strains and inoculation methods for resistance assessment to bacterial halo blight on coffee seedlings. J. Phytopathol. 165, 105–114. doi: 10.1111/jph.12543
Rodrigues Neto, J., Silva, C., Beriam, L., Patrício, F., Rodrigues, L., and Thomazielo, R. (2006). Mancha bacteriana do cafeeiro causada por Pseudomonas syringae pv. tabaci. Summa Phytopathol. 32:85.
Rodriguez, J. G., Campbfll, J. M., and Eveleens, K. G. (1966). Effect of some oil-insecticide combinations on coffee leaf Miner12. J. Econ. Entomol. 59, 773–779. doi: 10.1093/jee/59.4.773
Roseberry, W., Gudmundson, L., and Kutschbach, M. S. (1995). Coffee, society, and power in Latin America. Baltimore, MD: Johns Hopkins University Press.
Rutherford, M. A. (2006). Current knowledge of coffee wilt disease, a major constraint to coffee production in Africa. Phytopathology 96, 663–666. doi: 10.1094/PHYTO-96-0663
Salojärvi, J., Rambani, A., Yu, Z., Guyot, R., Strickler, S., Lepelley, M., et al. (2024). The genome and population genomics of allopolyploid Coffea arabica reveal the diversification history of modern coffee cultivars. Nat. Genet. 56, 721–731. doi: 10.1038/s41588-024-01695-w
Salvador, H. P., Berilli, A. P. C. G., Rodrigues, W. P., Mazzafera, P., and Partelli, F. L. (2024). “A climate change perspective on the selection, development, and management of Coffea canephora genotypes” in Advances in botanical research. Eds. V. Kuete and J. P. Jacquot (Amsterdam, The Netherlands: Elsevier). In press.
Sánchez, L., Zapata, M., Rodríguez, R. P., and Beaver, J. S. (2003). Distribución y patogenicidad de Pseudomonas cichorii (Swingle) Stapp en el cultivo del café en Puerto Rico. JAUPR 87, 123–135. doi: 10.46429/jaupr.v87i3-4.1104
Sanglard, N. A., Amaral-Silva, P. M., Sattler, M. C., de Oliveira, S. C., Cesário, L. M., Ferreira, A., et al. (2019). Indirect somatic embryogenesis in Coffea with different ploidy levels: a revisiting and updating study. Plant Cell Tissue Organ Cult. 136, 255–267. doi: 10.1007/s11240-018-1511-9
Sanglard, N. A., Amaral-Silva, P. M., Sattler, M. C., de Oliveira, S. C., Nunes, A. C. P., Soares, T. C. B., et al. (2017). From chromosome doubling to DNA sequence changes: outcomes of an improved in vitro procedure developed for allotriploid “Híbrido de Timor” (Coffea arabica L. × Coffea canephora Pierre ex a. Froehner). Plant Cell Tissue Organ Cult. 131, 223–231. doi: 10.1007/s11240-017-1278-4
Santana-Buzzy, N., Rojas-Herrera, R., Galaz-Ávalos, R. M., Ku-Cauich, J. R., Mijangos-Cortés, J., Gutiérrez-Pacheco, L. C., et al. (2007). Advances in coffee tissue culture and its practical applications. In Vitro Cell. Dev. Biol.-Plant 43, 507–520. doi: 10.1007/s11627-007-9074-1
Santos, V. P., Ribeiro, P. C. C., and Rodrigues, L. B. (2022). Sustainability assessment of coffee production in Brazil. Environ. Sci. Pollut. Res. 30, 11099–11118. doi: 10.1007/s11356-022-22922-z
Scalabrin, S., Magris, G., Liva, M., Vitulo, N., Vidotto, M., Scaglione, D., et al. (2024). A chromosome-scale assembly reveals chromosomal aberrations and exchanges generating genetic diversity in Coffea arabica germplasm. Nat. Commun. 15:463. doi: 10.1038/s41467-023-44449-8
Scalabrin, S., Toniutti, L., Di Gaspero, G., Scaglione, D., Magris, G., Vidotto, M., et al. (2020). A single polyploidization event at the origin of the tetraploid genome of Coffea arabica is responsible for the extremely low genetic variation in wild and cultivated germplasm. Sci. Rep. 10:4642. doi: 10.1038/s41598-020-61216-7
Schroth, G., Laderach, P., Dempewolf, J., Philpott, S., Haggar, J., Eakin, H., et al. (2009). Towards a climate change adaptation strategy for coffee communities and ecosystems in the Sierra Madre de Chiapas, Mexico. Mitig Adapt Strateg Glob Change 14, 605–625. doi: 10.1007/s11027-009-9186-5
Silva, M. D. C., Várzea, V., Guerra-Guimarães, L., Azinheira, H. G., Fernandez, D., Petitot, A.-S., et al. (2006). Coffee resistance to the main diseases: leaf rust and coffee berry disease. Braz. J. Plant Physiol. 18, 119–147. doi: 10.1590/S1677-04202006000100010
Silva, R. A., Zambolim, L., Castro, I. S. L., Rodrigues, H. S., Cruz, C. D., and Caixeta, E. T. (2018). The Híbrido de Timor germplasm: identification of molecular diversity and resistance sources to coffee berry disease and leaf rust. Euphytica 214:153. doi: 10.1007/s10681-018-2231-2
Silvestrini, M., Junqueira, M. G., Favarin, A. C., Guerreiro-Filho, O., Maluf, M. P., Silvarolla, M. B., et al. (2007). Genetic diversity and structure of Ethiopian, Yemen and Brazilian Coffea arabica L. accessions using microsatellites markers. Genet. Resour. Crop. Evol. 54, 1367–1379. doi: 10.1007/s10722-006-9122-4
Smith, R. F. (1985). “A history of coffee” in Coffee. eds. M. N. Clifford and K. C. Willson (Boston, MA: Springer US), 1–12.
Söndahl, M., and Sharp, W. (1977). High frequency induction of somatic embryos in cultured leaf expiants of Coffea arabica L. Z. Pflanzenphysiol. 81, 395–408. doi: 10.1016/S0044-328X(77)80175-X
Sousa, P., Vieira, H., Santos, E., Viana, A., Boaechat, M., and Partelli, F. (2022). Coffea canephora: heterotic crosses indicated by molecular approach. Plan. Theory 11:3023. doi: 10.3390/plants11223023
Spinoso-Castillo, J. L., Escamilla-Prado, E., Aguilar-Rincón, V. H., Morales Ramos, V., De Los Santos, G. G., Pérez-Rodríguez, P., et al. (2020). Genetic diversity of coffee (Coffea spp.) in Mexico evaluated by using DArTseq and SNP markers. Genet. Resour. Crop. Evol. 67, 1795–1806. doi: 10.1007/s10722-020-00940-5
Staritsky, G. (1970). EMBRYOID formation in callus tissues of coffee. Acta Bot. Neerlandica 19, 509–514. doi: 10.1111/j.1438-8677.1970.tb00677.x
Steiger, D., Nagai, C., Moore, P., Morden, C., Osgood, R., and Ming, R. (2002). AFLP analysis of genetic diversity within and among Coffea arabica cultivars. Theor. Appl. Genet. 105, 209–215. doi: 10.1007/s00122-002-0939-8
Tapaça, I. D. P. E., Mavuque, L., Corti, R., Pedrazzani, S., Maquia, I. S. A., Tongai, C., et al. (2023). Genomic evaluation of Coffea arabica and its wild relative Coffea racemosa in Mozambique: settling resilience keys for the coffee crop in the context of climate change. Plan. Theory 12:2044. doi: 10.3390/plants12102044
Tobón-Niedfeldt, W., Mastretta-Yanes, A., Urquiza-Haas, T., Goettsch, B., Cuervo-Robayo, A. P., Urquiza-Haas, E., et al. (2022). Incorporating evolutionary and threat processes into crop wild relatives conservation. Nat. Commun. 13:6254. doi: 10.1038/s41467-022-33703-0
Turreira-García, N. (2022). Farmers’ perceptions and adoption of Coffea arabica F1 hybrids in Central America. World Dev. Sustain. 1:100007. doi: 10.1016/j.wds.2022.100007
United States Department of Agriculture (2024). Coffee: world markets and trade. Foreign Agricultural Service/USDA. Available at: https://fas.usda.gov/data/coffee-world-markets-and-trade-06202024 (Accessed July 16, 2024).
Utting, K. (2009). Assessing the impact of fair trade coffee: towards an integrative framework. J. Bus. Ethics 86, 127–149. doi: 10.1007/s10551-008-9761-9
Van der Vossen, H. (2001). “Agronomy I: coffee breeding practices” in Coffee: recent developments, eds. R. J. Clarke and O. G. Vitzthum (London, UK: Blackwell Science) 184–201.
Van der Vossen, H. (2018). “Developing varieties of Arabica coffee” in Achieving sustainable cultivation of coffee. Breeding and quality traits, vol 1, ed. P. Lashermes (Cambridge, UK: Burleigh Dodds Science Publishing), 83–113.
Van der Vossen, H., Bertrand, B., and Charrier, A. (2015). Next generation variety development for sustainable production of arabica coffee (Coffea arabica L.): a review. Euphytica 204, 243–256. doi: 10.1007/s10681-015-1398-z
Van der Vossen, H., and Walyaro, D. J. (2009). Additional evidence for oligogenic inheritance of durable host resistance to coffee berry disease (Colletotrichum kahawae) in arabica coffee (Coffea arabica L.). Euphytica 165:105. doi: 10.1007/s10681-008-9769-3
Vega, F. E., Infante, F., and Johnson, A. J. (2015). “The genus Hypothenemus, with emphasis on H. Hampei, the coffee berry borer” in Bark beetles. eds. F. E. Vega and R. W. Hofstetter (London, UK: Elsevier/Academic Press), 427–494.
Vegro, C. L. R., and de Almeida, L. F. (2020). “Global coffee market: socio-economic and cultural dynamics” in Coffee consumption and industry strategies in Brazil. eds. L. F. de Almedida and E. E. Spers (Sawston, UK: Elsevier Woodhead Publishing), 3–19.
Venancio, L. P., Filgueiras, R., Mantovani, E. C., do Amaral, C. H., da Cunha, F. F., dos Santos Silva, F. C., et al. (2020). Impact of drought associated with high temperatures on Coffea canephora plantations: a case study in Espírito Santo state, Brazil. Sci Rep 10:19719. doi: 10.1038/s41598-020-76713-y
Venial, L. R., Mendonça, M. A. C., Amaral-Silva, P. M., Canal, G. B., Passos, A. B. R., de Ferreira, A., et al. (2020). Autotetraploid Coffea canephora and auto-Alloctaploid Coffea arabica from in vitro chromosome set doubling: new germplasms for Coffea. Front. Plant Sci. 11:154. doi: 10.3389/fpls.2020.00154
Waller, J. M., Bigger, M., and Hillocks, R. J. (2007). Coffee pests, diseases and their management. Cambridge, MA: CABI.
Waller, J. M., Bridge, P. D., Black, R., and Hakiza, G. (1993). Characterization of the coffee berry disease pathogen, Colletotrichum kahawae sp. nov. Mycol. Res. 97, 989–994. doi: 10.1016/S0953-7562(09)80867-8
Wamatu, J. N., Thomas, E., and Piepho, H. P. (2003). Responses of different arabica coffee (Coffea arabica L.) clones to varied environmental conditions. Euphytica 129, 175–182. doi: 10.1023/A:1021927302410
Wang, N., Jassogne, L., Van Asten, P. J. A., Mukasa, D., Wanyama, I., Kagezi, G., et al. (2015). Evaluating coffee yield gaps and important biotic, abiotic, and management factors limiting coffee production in Uganda. Eur. J. Agron. 63, 1–11. doi: 10.1016/j.eja.2014.11.003
Winkels, A. (2008). Rural in-migration and global trade: managing the risks of coffee farming in the central highlands of Vietnam. Mt. Res. Dev. 28, 32–40. doi: 10.1659/mrd.0841
World Coffee Research (2022). International multi-location variety trial produces initial global results. Available at: https://worldcoffeeresearch.org/news/2022/imlvt-global-results (Accessed August 7, 2023).
World Coffee Research (2024a). Coffee variety catalog: a global catalog of Arabica and Robusta coffee varieties from around the world. World Coffee Research. Available at: https://varieties.worldcoffeeresearch.org/ (Accessed March 19, 2024).
World Coffee Research (2024b). Innovea global coffee breeding network. Transforming coffee agriculture for climate-resilience through collaborative global breeding. Available at: https://worldcoffeeresearch.org/programs/global-breeding-network (Accessed May 12, 2024).
Zhang, X., Peck, L. D., Flood, J., Ryan, M. J., and Barraclough, T. G. (2023). Temperature contributes to host specialization of coffee wilt disease (Fusarium xylarioides) on arabica and robusta coffee crops. Sci. Rep. 13:9327. doi: 10.1038/s41598-023-36474-w
Zoccoli, D. M., Takatsu, A., and Uesugi, C. H. (2011). Ocorrência de mancha aureolada em cafeeiros na Região do Triângulo Mineiro e Alto Paranaíba. Bragantia 70, 843–849. doi: 10.1590/S0006-87052011000400017
Keywords: coffee, production challenges, variety improvement, collaborative breeding, sustainability
Citation: Ngure GM and Watanabe KN (2024) Coffee sustainability: leveraging collaborative breeding for variety improvement. Front. Sustain. Food Syst. 8:1431849. doi: 10.3389/fsufs.2024.1431849
Edited by:
Willian Dos Santos Gomes, Federal University of Espirito Santo, BrazilReviewed by:
Ana I. F. Ribeiro-Barros, University of Lisbon, PortugalFábio Luiz Partelli, Federal University of Espirito Santo, Brazil
Copyright © 2024 Ngure and Watanabe. This is an open-access article distributed under the terms of the Creative Commons Attribution License (CC BY). The use, distribution or reproduction in other forums is permitted, provided the original author(s) and the copyright owner(s) are credited and that the original publication in this journal is cited, in accordance with accepted academic practice. No use, distribution or reproduction is permitted which does not comply with these terms.
*Correspondence: Godfrey M. Ngure, s2236024@u.tsukuba.ac.jp