- 1Department of Humanities and Arts, The Technion-Israel Institute of Technology, Haifa, Israel
- 2Faculty of Civil and Environmental Engineering, The Technion-Israel Institute of Technology, Haifa, Israel
At the present state of industrialized agriculture and specialized economy, achieving nutritional self-sufficiency on a personal level is widely considered a naïve goal, unsuited to the present technological era. Furthermore, nutritional self-sufficiency is considered overly demanding in terms of training, land, labor intensity, and time requirements. This study contests these common notions. Drawing on a study of a small (approximately 0.075 ha) low-input self-sufficient farm in an industrialized country, we show that achieving nutritional self-sufficiency on this farm required modest initial agricultural training, a small parcel of land, and, perhaps most surprisingly, a single day of labor per month, on average. We offer these results as proof of concept that, when properly planned, nutritional self-sufficiency may be sustained over an extended period (more than a decade) and that doing so may be compatible with working full-time outside the farm, as well as engaging in regular leisure activities. We also propose that some upscaling is viable at the industrial, small-scale, and household levels.
1 Introduction
The philosophical ideal of small-scale self-sufficiency (i.e., providing for all one’s needs by oneself) has been promoted by thinkers of numerous traditions since antiquity. The reasons given in support of leading such a way of life span resilience (Gandhi and Jaju, 1951; Thoreau, 1971), cultivating personal character (Morris, 1888; Schopenhauer, 1890; Thoreau, 1971; Aristotle, 1999; Zhuangzi and Watson, 2013; Adamson, 2018, Chapter 29), and acting in a socially responsible manner (Gandhi and Jaju, 1951; Schumacher, 1973; Berry, 2018) and environmentally responsible manner (Schumacher, 1973; Næss and Rothenberg, 1990; Berry, 2018). With respect to self-sufficiency in food production specifically, the commonly mentioned benefits include improving one’s health, reducing negative impacts on the environment, acquiring independence, connecting with nature, realizing a wider range of human capacities, and not partaking in exploitative food-production industries (Jacob, 1997; Agnew, 2004; Belasco, 2007; Brown, 2011; Jehlička et al., 2020; Sandström, 2023; Suomalainen et al., 2023). However, it is widely believed that achieving nutritional self-sufficiency today is a naïve ideal, unfeasible, and unsuited to the current technological era. Self-sufficiency in food production is also judged to be inefficient and impractical, in that it is believed to be overly demanding in terms of its training, land use, labor intensity, and time requirements. Some charge that it may not even be environmentally desirable (Wilbur, 2013; Jehlička et al., 2019). In short, self-sufficiency is currently considered a romantic and unrealistic fantasy.
Here we wish to show that these assumptions are likely mistaken, by presenting evidence to the contrary. Surprisingly, nutritional self-sufficiency today may not require much investment of labor, time, land, or training. Moreover, when properly planned, it enables the individual to lead a normal lifestyle, including engaging in full-time work outside the farm and leisure activities, and it is environmentally superior to consuming food grown using industrial methods. Specifically, for the same cultivated area and locality, it generates far fewer environmental burdens, while supplying nearly the same nutritional yield as industrial agriculture. To assign values to the variables noted above, we use a single case study of a self-sufficient agroecological farm that one of the authors (Pelman) has been running for over a decade. These values include the amount of labor, plot size, and training required to live in a nutritionally self-sufficient manner, as well as the yields produced, and the resources used. To the best of our knowledge, studies that systematically evaluate fully self-sufficient farms in industrialzed societies are extremely scarce, most likely due to the small number of such farms.
2 Planning
2.1 Experiment framework
Pelman purchased a 0.2 ha plot of land in western Galilee, Israel, on which he has been living in nutritional self-sufficiency since May 2013. At the time, the plot was designated as agricultural land but was unplanted, with the exception of nine mature olive trees and one mature carob tree. Farming of the plot was designed to provide one person’s entire nutritional needs from a minimal land area while causing minimal negative environmental impact, and maintaining a normal modern lifestyle, involving retaining a full-time job and engaging in leisure activities.
2.2 Goal
In terms of nutrition, the goal was to meet the Recommended Daily Allowance (RDA) for a 70 kg male (Meyers et al., 2006), as well as the Acceptable Macronutrients Distribution Range (AMDR) guidelines (US Department of Agriculture and US Department of Health and Human Services, 2020). In terms of environmental impacts, the goal was to minimize the release of toxins resulting from the use of synthetic chemicals and the emission of pollutants such as greenhouse gases (GHG), and to minimize the use of resources, mainly water for irrigation and land. These considerations dictated crop selection and the farming methods adopted.
2.3 Training
Prior to purchasing the land, Pelman spent 1 year engaged in full-time farm work on a 1 ha commercial organic vegetable farm in southern Galilee. There, he acquired hands-on experience in all seasons during the days, and theoretical knowledge in the evenings through self-education. Based on these, he went on to plan the self-sufficient farm, as detailed below.
2.4 Crop selection
To minimize land use, water use and greenhouse gas emissions, it was decided to avoid livestock and adopt a plant-based diet. To minimize water use, rainfed crops were selected to provide most of the macronutrients. Given the strong seasonality of rainfall in this region (November to March), the durability of the harvested crops in year-round storage was another important consideration, and it was also preferable that they require little storage space. Consequently, the staple crops chosen were winter cereals (as a main source of carbohydrates), winter pulses (as a main source of protein), and olive oil (as a main source of fat). To avoid the use of synthetic chemicals, winter cereals and pulses that are indigenous to the area were selected, namely wheat and fava beans. Finally, to supply micronutrients and a varied diet, a small, irrigated vegetable garden was constructed and planted with 25 or 15 varieties of vegetables in the winter or summer, respectively. Vegetables were to be grown seasonally, thus avoiding the need for polytunnels, shading nets or other growing structures.
2.5 Farming practices
To reduce toxins, no synthetic chemicals are used, and compost is applied as fertilizer. To increase soil health and plant resilience, it was decided to practice a two-year rotation in the wheat and fava bean plot (fava bean is a nitrogen fixer) and a nine-year rotation in the vegetable garden. To reduce land use, grains are also grown beneath the olive canopies, all the way to the tree trunks. Such intercropping is a considerably more efficient use of land compared with industrial farming and is made possible by the farm’s small scale and the adoption of manual practices. The decision to use small power tools, and a tractor (the latter for merely 2 h per annum), was based on the understanding that, compared with their manual equivalents, they provide a considerable reduction in labor time for a relatively small additional annual environmental cost in terms of GHG emissions. To reduce GHG emissions and increase soil health, a reduced tilling (~10 cm depth) policy was adopted.
2.6 Crop quantity and plot sizes
Daily consumption of wheat, fava beans, olive oil, and assorted seasonal vegetables was calculated to provide a total of 2000 calories with a target distribution of carbohydrates (50%), fat (30%), and protein (20%) to meet the Acceptable Macronutrients Distribution Range (AMDR) guidelines (US Department of Agriculture and US Department of Health and Human Services, 2020) (see Table 1 for details). These daily required quantities were translated into the annual required yields for each crop, which in turn determined plot size per crop (Table 1). The whole operation required a total of 740 m2. The relatively small area needed for the trees—50 m2—is due to the intercropping of grains and olive trees (as described above).
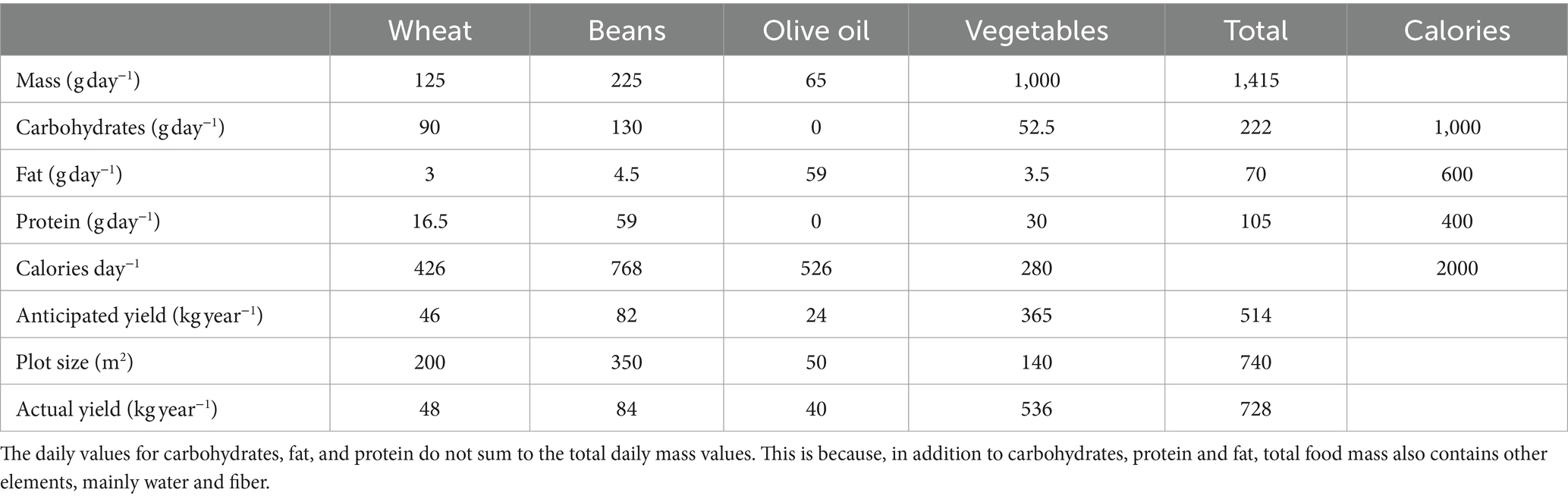
Table 1. Calculated yields and plot sizes required to achieve nutritional self-sufficiency from the selected crops.
3 Executing
The cultivated area of the farm (740 m2) consists of plots of wheat (200 m2) and fava bean (350 m2), a vegetable garden (140 m2), olive trees (45 m2), and one carob tree (5 m2). Over time, one beehive was added for honey supply and pollination. Data were collected with records maintained for harvest yield, labor time, and quantities of resources used. Out of approximately 40 varieties of vegetables, about one-third are grown from purchased seedlings, one-third from purchased seeds, and one-third from seeds collected onsite. The seeds of wheat and fava are normally a mix of remaining grains from last year and seeds purchased to make up the amount needed for the following season.
3.1 Inputs
Ongoing inputs invested in food production on the farm include labor time, water for irrigation, compost as fertilizer, and fuel to operate small power tools, as well as, once a year, a small tractor.
3.1.1 Fuel
The tools used on the farm are manual (e.g., sickle, hoe, pickaxe) and additionally include four small power tools: a string trimmer, a power tiller, an olive harvesting machine, and a grain threshing machine. In addition, a small tractor is used for 2 h once a year to till the soil before sowing the grain. The use of the four power tools and the small tractor is 21 h year−1, with 33 litres of fuel consumed. The electric olive harvester is powered by a car battery.
3.1.2 Labor
The wheat and fava beans require 0.5 days year−1 to till (using a small tractor), sow, and set up an electric wire against wild boar (in December), 2 days for manual harvesting and 1.5 days year−1 for threshing (using a small threshing machine) and storage (during June). Grains are then stored un-milled and are milled only before food preparation, if the recipe requires. Olive oil takes 1 day year−1 to harvest the nine trees (using a small electric harvester) and to press the oil at a nearby press, and 1 day year−1 to prune the trees and to apply compost around them (in early November). The oil is stored in a stainless still container. Vegetables require 0.5 days, eight times a year, to prepare a 35 m2 section of the garden (i.e., a quarter of the vegetable garden) and plant each time (using a power tiller and a string trimmer). Hive inspection takes 0.5 h eight times a year, and honey extraction takes 0.5 days once a year (in July). Annually, the hive produces approximately 25 kg of honey, of which only 10 kg is harvested, leaving 15 kg for the bees to feed on during the winter. Harvesting one carob tree and preparing syrup takes another 0.5 days year−1. In addition, approximately one more day year−1 is invested in general maintenance (e.g., fixing leaks in the drip irrigation system or repairing the seasonal electric wire). Thus, in total, growing food on the farm using traditional methods with limited aid from small power tools requires approximately 12.5 days year−1, which is one day month−1 on average, viz., 8.1 min (m2 year)−1. However, much of the work is concentrated in June, during grain harvesting and threshing. The low amount of labor presently invested is due to the farmer’s extensive experience accumulated over a decade of single-handedly operating the farm, in the specific method described.
3.1.3 Irrigation
Wheat, fava beans, olives and carob, which together constitute 80% (600 m2) of the area of the farm, rely solely on rainfall (700 mm annually). The remaining 20% of the area, which is occupied by the vegetable garden (140 m2), is irrigated in the dry months (March–October), requiring an estimated 50 m3 of blue water annually. The toilet used on the farm is a composting toilet, totally eliminating water wastage in flushing, thus saving 22 m3 of water (Israel Water Authority, 2021).
3.1.4 Compost input
Purchased compost is applied to the vegetable garden twice a year (10 L m−2, i.e., 1,400 L in winter and again in summer), for a total annual requirement of about 3 m3 of compost. Using compost produced on-site by the composting toilet, the olive trees are fertilized once a year before the rainy season (100 L of compost per tree, about 1 m3 for all nine trees). All synthetic chemicals, including pesticides, herbicides, synthetic fertilizers, plant growth regulators, and nanomaterials, are completely avoided.
Investing all the above inputs clearly indicates that the farm is not a closed system; as stated in the opening line in the ‘Introduction’ section above, by ‘nutritional self-sufficiency’, we only mean here the practice of providing for all one’s food by oneself.
3.2 Harvest
The bottom row in Table 1 summarizes annual crop production across the farm. Comparing the planned and actual yields, the wheat and fava beans yields were in line with expectations, whereas the actual yields from the olive trees and the vegetable garden significantly surpassed the expected yields.
A recent study undertaken on the farm (Pelman et al., 2024) calculated its nutritional output in relation to four macronutrients (carbohydrates, protein, fat, and calories) and four key micronutrients (zinc, iron, magnesium, and calcium). The study compared the farm’s nutritional output to the RDA for a 70 kg male adult and to the AMDR guidelines (Meyers et al., 2006). With the exception of calcium the farm supplies 80% of the RDA for calcium), the farm’s supply of all nutrients meets or exceeds the daily nutritional needs of an adult male – in some cases by large margins – and it also meets the AMDR guidelines (Pelman et al., 2024). The same study also compared the self-sufficient farm with average Israeli industrial farming, in terms of nutritional yield and environmental impacts [using life cycle assessment (LCA)]. Each of the two comparisons was normalized by mass and by land area. It was found that, per hectare, the self-sufficient farm provided nearly the same average amount of nutrients as average local industrial farming, while generating merely 20% of the negative environmental impacts (Pelman et al., 2024).
4 Discussion
The results of the above study show that an individual can cultivate all of their nutritional needs on as little as 740 m2 of land, compared with 2000 m2, which is the current average per capita Israeli cropland footprint (Fridman and Kissinger, 2019). Resource use (water and fuel) and GHG emissions are much lower compared with conventional agriculture (Pelman et al., 2024), requiring an average labor investment of merely one day a month. This is accomplished without the use of synthetic chemicals or heavy machinery. The relatively small land area needed for nutritional self-sufficiency is mainly due to consuming a plant-based diet and practising intercropping of grains and olive trees. The land area could be further reduced if grain production moved from relying solely on winter rains to incorporating summer irrigation, which would enable an additional crop, thus doubling production such that only half the area would be needed to obtain the same nutritional yield of grains. In such a scenario, the farm size used for self-sufficiency would drop to under 500 m2, although water usage would increase (and maybe also the chances of disease). The small labor investment required is mainly due to the small farmed area, 80% of which is occupied by low-maintenance grains and olive trees, and thanks to the use of some small power tools. Obtaining required theoretical and practical training to embark on this project took one year (see ‘planning’ section); nonetheless, we believe that with a purpose-built training program, the required ‘starter’ training could be acquired within a shorter period. The acquired knowledge would then continue to grow as more experience is accumulated through practice and learning over the years.
Overall, this study shows that common notions regarding the infeasibility of food self-sufficiency in the present era are misguided, at least with respect to countries where cereals, pulses and some oil plants can be easily grown, which applies to most countries (FAO, 2023). Such crops include cereals like rice, wheat, maize, oats or millet; pulses like a variety of beans, peas or lentils; and oil plants, which in many cases are the very same grains (e.g., corn oil or soybean oil) or other sources of vegetable oil like rapeseed, sunflower, nuts, sesame or olives. As our study shows, these three types of low-maintenance crops can supply about 85% of the calories within a balanced diet (Table 1). Some countries do not meet these criteria (e.g., countries with cold and long winters), and modifications to our proposition would be needed. However, in the countries that do meet the above prevalent conditions, when properly planned, achieving nutritional self-sufficiency may require modest agricultural training; a small parcel of land may be sufficient to provide the entire dietary needs of a single adult; and, perhaps most surprisingly, it may require on average a single day of labor per month. Although our study involves a single self-sufficient farm and thus provides deterministic rather than statistical conclusions, it clearly highlights the unrealized potential of practicing self-sufficiency, while adhering to nutritional best-practice guidelines and also providing considerable environmental benefits. Moreover, the study represents a proof of concept that such nutritional self-sufficiency may be sustained over an extended period (more than a decade) and that doing so is compatible with holding a full-time position outside the farm and engaging in regular leisure activities. Complete self-sufficiency with such a small annual time investment may take several years to achieve, depending on one’s skill and local conditions. But partial self-sufficiency (or, alternatively, full self-sufficiency with greater time investment) may be attained fairly early on.
Questions remain as to the likely general appeal of the diet and to the scalability of the food production approach. Concerning diet, the plant-based diet produced on the farm is quite distinct from typical Western diet (Cordain et al., 2005; Pingali, 2007; Clemente-Suárez et al., 2023) and, to a lesser extent, Mediterranean diet (Noah and Truswell, 2001; Russo et al., 2021), both of which contain animal products. With the exception of honey, the diet supplied by the self-sufficient farm is vegan, with a rich variety of 40 types of vegetables. Veganism is becoming increasingly popular—fully or partially—for its positive impact on health, animal welfare, and the environment, including its smaller demand for agricultural land (Godfray et al., 2018; Poore and Nemecek, 2018; Sexton et al., 2022). Also, should one wish to do so, it is possible to incorporate a small production of animal-based food on one’s self-sufficient farm, e.g., eggs, without dramatically changing the inputs, namely, land use, labor time, and training time.
The second question concerns upscaling the described method for food production. We suggest that this is achievable, to a degree, via three channels. Firstly, some of the agricultural practices described here could be extrapolated and adopted by large-scale industrial agriculture. For example, converting more farmland to seasonal crops, e.g., decreasing the cultivation of summer crops in the winter–like cucumbers, tomatoes or peppers–which compels farmers to use polytunnels and more synthetic chemicals for protection, would allow reducing the use of such chemicals (Macdiarmid, 2014; Vargas et al., 2021). Switching from imported summer grains that are accustomed to summer rains and hence require much summer irrigation (like corn or broad beans) to local winter grains (e.g., wheat, fava beans, chickpeas, and lentils) would reduce irrigation demand (Wani et al., 2007). Adopting intercropping of trees and grains and giving preference to crops with high nutritional yield would also reduce land use. Secondly, non-commercial small-scale farmers, like part-time farmers or homesteaders, can adopt the farm’s model, with the necessary adaptations to their specific geolocation, conditions, and farm size. Thirdly, at the urban household level, upscaling from a single independent working-age adult to more diverse households with dependent children or elders can be achieved to various extents, depending on dwelling type (Burgin, 2018; Hume et al., 2021). Families with access to a backyard or a rooftop can cultivate a small vegetable garden and plant some fruit trees, thus increasing their nutritional self-sufficiency. Apartment dwellers with access to a balcony can use the balcony to grow one or more olive trees in large pots and/or a pile of potatoes in a vertical device, to somewhat increase their nutritional self-sufficiency. Such implementations carry with them the various advantages of self-sufficiency, in proportion to the degree of application (Kortright and Wakefield, 2011).
Data availability statement
The original contributions presented in the study are included in the article/supplementary material, further inquiries can be directed to the corresponding author/s.
Ethics statement
Written informed consent was obtained from the individual(s) for the publication of any potentially identifiable images or data included in this article.
Author contributions
AP: Conceptualization, Data curation, Formal analysis, Investigation, Methodology, Project administration, Resources, Validation, Writing – original draft, Writing – review & editing. ON: Conceptualization, Methodology, Writing – original draft, Writing – review & editing. YC: Formal analysis, Investigation, Methodology, Supervision, Writing – review & editing.
Funding
The author(s) declare that no financial support was received for the research, authorship, and/or publication of this article.
Acknowledgments
We thank Alexandra Singer-Lavi for reading and commenting on an earlier draft.
Conflict of interest
The authors declare that the research was conducted in the absence of any commercial or financial relationships that could be construed as a potential conflict of interest.
Publisher’s note
All claims expressed in this article are solely those of the authors and do not necessarily represent those of their affiliated organizations, or those of the publisher, the editors and the reviewers. Any product that may be evaluated in this article, or claim that may be made by its manufacturer, is not guaranteed or endorsed by the publisher.
References
Agnew, E. (2004). Back from the land: How young Americans went to nature in the 1970s, and why they came back. Dee: Ivan R.
Belasco, W. J. (2007). Appetite for change: How the counterculture took on the food industry. Ithaca: Cornell University Press.
Brown, D. (2011). Back to the land: The enduring dream of self-sufficiency in modern America. Madison: University of Wisconsin Press, 290.
Burgin, S. (2018). ‘Back to the future’? Urban backyards and food self-sufficiency. Land Use Policy 78, 29–35. doi: 10.1016/j.landusepol.2018.06.012
Clemente-Suárez, V. J., Beltrán-Velasco, A. I., Redondo-Flórez, L., Martín-Rodríguez, A., and Tornero-Aguilera, J. F. (2023). Global impacts of Western diet and its effects on metabolism and health: a narrative review. Nutrients 15:2749. doi: 10.3390/nu15122749
Cordain, L., Eaton, S. B., Sebastian, A., Mann, N., Lindeberg, S., Watkins, B. A., et al. (2005). Origins and evolution of the Western diet: health implications for the 21st century. Am. J. Clin. Nutr. 81, 341–354. doi: 10.1093/ajcn.81.2.341
FAO. (2023). Production: Crops and livestock products. In: FAOSTAT. Rome. https://www.fao.org/faostat/en/#data/QCL based on UN Geospatial. 2020. Map geodata [shapefiles]. New York, USA, UN.
Fridman, D., and Kissinger, M. (2019). A multi-scale analysis of interregional sustainability: applied to Israel’s food supply. Sci. Total Environ. 676, 524–534. doi: 10.1016/j.scitotenv.2019.04.054
Gandhi, M., and Jaju, S. (1951). The ideology of the charkha: A collection of some of Gandhiji’s speeches and writings about khadi. (1st ed). All India Spinners’ Association.
Godfray, H. C. J., Aveyard, P., Garnett, T., Hall, J. W., Key, T. J., Lorimer, J., et al. (2018). Meat consumption, health, and the environment. Science 361:eaam5324. doi: 10.1126/science.aam5324
Hume, I., Summers, D., and Cavagnaro, T. (2021). Self-sufficiency through urban agriculture: Nice idea or plausible reality? Sustain. Cities Soc. 68:102770. doi: 10.1016/j.scs.2021.102770
Israel Water Authority. (2021). “Water saving—household.” Israel Water Authority. Available at: https://www.gov.il/he/departments/general/water-saving-household (Accessed April 20, 2024).
Jacob, J. (1997). New pioneers: The back-to-the-land movement and the search for a sustainable future. University Park: Penn State Press.
Jehlička, P., Daněk, P., and Vávra, J. (2019). Rethinking resilience: home gardening, food sharing and everyday resistance. Can. J. Dev. Stud. 40, 511–527. doi: 10.1080/02255189.2018.1498325
Jehlička, P., Grīviņš, M., Visser, O., and Balázs, B. (2020). Thinking food like an east European: a critical reflection on the framing of food systems. J. Rural. Stud. 76, 286–295. doi: 10.1016/j.jrurstud.2020.04.015
Kortright, R., and Wakefield, S. (2011). Edible backyards: a qualitative study of household food growing and its contributions to food security. Agric. Hum. Values 28, 39–53. doi: 10.1007/s10460-009-9254-1
Macdiarmid, J. I. (2014). Seasonality and dietary requirements: will eating seasonal food contribute to health and environmental sustainability? Proc. Nutr. Soc. 73, 368–375. doi: 10.1017/S0029665113003753
Meyers, L. D., Hellwig, J. P., and Otten, J. J. (2006). Dietary reference intakes: The essential guide to nutrient requirements. 1st ed. National Academies Press.
Næss, A., and Rothenberg, D. (1990). Ecology, community, and lifestyle: outline of an ecosophy. Ed. D. Rothenberg (1st pbk. ed.). Cambridge, New York: Cambridge University Press.
Noah, A., and Truswell, A. S. (2001). There are many Mediterranean diets. Asia Pac. J. Clin. Nutr. 10, 2–9. doi: 10.1046/j.1440-6047.2001.00198.x
Pelman, A., de Vries, J. W., Tepper, S., Eshel, G., Carmel, Y., and Shepon, A. (2024). A life-cycle approach highlights the nutritional and environmental superiority of agroecology over conventional farming: A case study of a Mediterranean farm. Plos Sustain. Transform. 3, 1–20. doi: 10.1371/journal.pstr.0000066
Pingali, P. (2007). Westernization of Asian diets and the transformation of food systems: implications for research and policy. Food Policy 32, 281–298. doi: 10.1016/j.foodpol.2006.08.001
Poore, J., and Nemecek, T. (2018). Reducing food’s environmental impacts through producers and consumers. Science 360, 987–992. doi: 10.1126/science.aaq0216
Russo, G. L., Siani, A., Fogliano, V., Geleijnse, J. M., Giacco, R., Giampaoli, S., et al. (2021). The Mediterranean diet from past to future: Key concepts from the second “Ancel keys” international seminar. Nutr. Metab. Cardiovasc. Dis. 31, 717–732. doi: 10.1016/j.numecd.2020.12.020
Sandström, E. (2023). Resurgent back-to-the-land and the cultivation of a renewed countryside. Sociol. Rural. 63, 544–563. doi: 10.1111/soru.12406
Schopenhauer, A. (1890). The wisdom of life and Counsels and maxims. ed. T. Bailey Saunders. Amherst, NY: Prometheus Books.
Schumacher, E. F. (1973). Small is beautiful: Economics as if people mattered. London: Blond & Briggs.
Sexton, A. E., Garnett, T., and Lorimer, J. (2022). Vegan food geographies and the rise of big veganism. Prog. Hum. Geogr. 46, 605–628. doi: 10.1177/03091325211051021
Suomalainen, M., Hohenthal, J., Pyysiäinen, J., Ruuska, T., Rinkinen, J., and Heikkurinen, P. (2023). Food self-provisioning: a review of health and climate implications. Glob. Sustain. 6:e7. doi: 10.1017/sus.2023.6
Thoreau, H. D. (1971) in Walden. 1854. ed. J. Lyndon Shanley (Princeton University Press: Princeton).
US Department of Agriculture and US Department of Health and Human Services. (2020). Dietary guidelines for Americans, 2020–2025. 9th ed. Washington, DC: US Government Publishing Office.
Vargas, A. M., de Moura, A. P., Deliza, R., and Cunha, L. M. (2021). The role of local seasonal foods in enhancing sustainable food consumption: a systematic literature review. Food Secur. 10:2206. doi: 10.3390/foods10092206
Wani, S. P., Sreedevi, T. K., Rockstrom, J., Wangkahart, T., Ramakrishna, Y. S., Dixin, Y., et al. (2007). Improved livelihoods and food security through unlocking the potential of rainfed agriculture. In: Food and water security. Balkema-proceedings and monographs in engineering, water, and earth sciences. (Abingdon, Oxford, UK: Taylor & Francis), 89–105.
Wilbur, A. (2013). Growing a radical ruralism: Back-to-the-land as practice and ideal. Geogr. Compass 7, 149–160. doi: 10.1111/gec3.12023
Keywords: self-provisioning, sustainable food systems, water-smart food production, food-security, sustainable diet, plant-based diet, dietary reference intake, agroecology
Citation: Pelman A, Nachtomy O and Carmel Y (2024) Individual nutritional self-sufficiency: a viable option in the present era. Front. Sustain. Food Syst. 8:1424879. doi: 10.3389/fsufs.2024.1424879
Edited by:
Stefanie Lemke, University of Natural Resources and Life Sciences Vienna, AustriaReviewed by:
Sabine Zikeli, University of Hohenheim, GermanyPetr Daněk, Masaryk University, Czechia
Copyright © 2024 Pelman, Nachtomy and Carmel. This is an open-access article distributed under the terms of the Creative Commons Attribution License (CC BY). The use, distribution or reproduction in other forums is permitted, provided the original author(s) and the copyright owner(s) are credited and that the original publication in this journal is cited, in accordance with accepted academic practice. No use, distribution or reproduction is permitted which does not comply with these terms.
*Correspondence: Alik Pelman, YWxpa3BlbG1hbkB0ZWNobmlvbi5hYy5pbA==