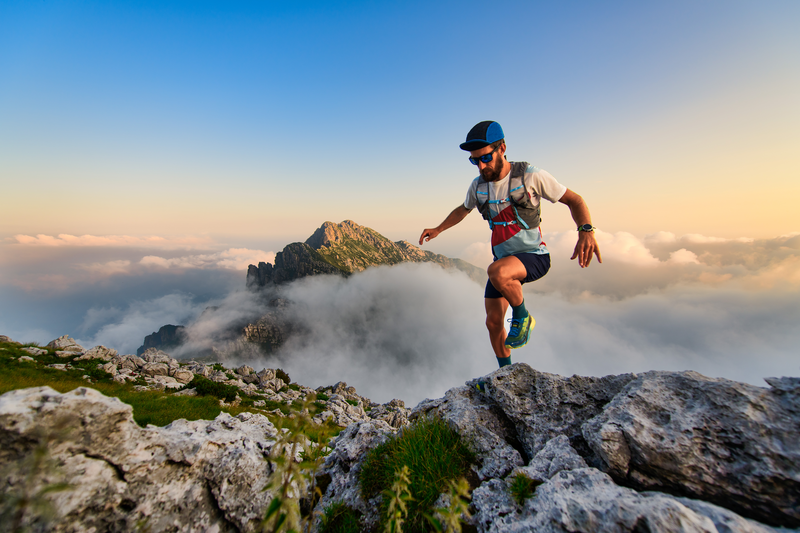
94% of researchers rate our articles as excellent or good
Learn more about the work of our research integrity team to safeguard the quality of each article we publish.
Find out more
ORIGINAL RESEARCH article
Front. Sustain. Food Syst. , 24 September 2024
Sec. Agricultural and Food Economics
Volume 8 - 2024 | https://doi.org/10.3389/fsufs.2024.1417469
Introduction: Warming caused by greenhouse gas (GHG) emissions has become a global environmental issue of widespread concern, and China, as a responsible power, has the pressing task of reducing carbon emissions. China is one of the world’s major beef producers and consumers, and at the same time, beef cattle, as a large livestock, is the largest source of GHG emissions in the livestock industry.
Methods: This study considered the panel data of 31 provinces in China from 2008 to 2022. The kernel density estimation and Dagum Gini coefficient were used to analyze the spatiotemporal dynamic evolution patterns and influencing factors of carbon emissions from China’s beef cattle farming industry.
Results: (1) The carbon emission trajectory of beef cattle production follows a distinctive “ascend-descend-ascend” three-phase pattern. By 2022, the sector’s cumulative carbon emissions had burgeoned by 37.62% relative to 2008, reflecting an average annual escalation of 2.31%. Despite the overall upward trend in carbon emissions, significant regional differences were observed. The Central Plains region has witnessed a consistent decline, in stark contrast to the Southwest and Northeast regions, which have emerged as hotspots for heightened carbon emissions and intensified emission densities within China’s beef cattle production landscape, underscoring the intensifying significance of carbon mitigation measures. (2) The kernel density curve shows an overall rightward shift with a specific gradient effect on carbon emissions. In addition, the range of the right drag of the curve in 2022 was significantly reduced, which laterally reflects the narrowing of the difference between the provinces with the highest and lowest carbon emissions from beef cattle farming. The principal source of variance in the overall carbon emissions from beef cattle production is the disparities between regions, which accounts for an average annual contribution rate of 52.52%. Conversely, the within-region contribution rates have remained relatively stable, while those for the intensity of transvariation have witnessed a substantial rise, with annual averages of 18.31 and 28.96%, respectively. (3) Regarding the factors influencing carbon emissions reduction, environmental regulations and production efficiency significantly drive carbon emissions reduction in beef cattle farming.
Discussion: Relevant government departments should actively guide farmers toward green production, establish perfect policies and regulations for low-carbon beef cattle farming, and promote low-carbon beef cattle farming models based on local conditions.
The low-carbon economy has gradually become a new development mode globally, and it is a significant topic for future development in seeking the balance between industry development and low-carbon emissions. The beef cattle breeding industry is one of the pillars of the Chinese animal husbandry industry. At the same time, the accelerated development of the beef cattle breeding industry has brought about a large amount of greenhouse gas emissions, extremely affecting ecological and environmental safety and the health of residents. Green and low-carbon practices are the primary means to achieve a carbon peak and neutrality in the beef cattle farming industry, as well as an intrinsic requirement for promoting high-quality development and transitioning to new growth drivers within this sector. The dispersed and extensive nature of beef cattle farming, along with the low production rate in the processing industries, constrains the green and low-carbon development of the beef cattle industry.
Currently, the proportion of large-scale beef cattle farmers in China is <5% and the yield per unit of meat is <40% of that in developed countries. However, the carbon emission level per beef unit is close to 1.5–2.0 times that of developed countries (Zhang and Yan, 2022; He et al., 2014). China’s per capita beef consumption is still at a relatively low level. Suppose future per capita consumption reaches 10 kilograms per year, then, China’s beef supply must increase by at least one-third, indicating the importance of advancing green and low-carbon development in the beef cattle industry. Beef cattle farming produces greenhouse gasses such as methane and nitrous oxide (Smith and Martino, 2007; Johnson and Ward, 2013; Gerber et al., 2013), leading to environmental pollution (Opio, 2011; Pelletier, 2010; Williams, 2012), posing risks to human health (Watts et al., 2015) and threatening the ecological environment (Hergoualc, 2019; Mar et al., 2022), which severely impacts the low-carbon and sustainable development of the beef cattle farming industry.
Scholars have previously delved into the realm of carbon emissions emanating from agriculture and livestock (Bell et al., 2014; Bellarby et al., 2013; Crosson et al., 2011), shedding light on various facets such as carbon emission quantification (Li G. et al., 2023), time series characteristics (Deng et al., 2023), geographical disparities (Li G. et al., 2023), and underpinning factors (Yin et al., 2023). A perusal of the existing literature reveals that most studies exploring regional disparities in carbon emissions within China’s beef cattle farming sector remain limited to basic descriptions or generalizations. Only some studies have employed relevant measurement indicators to conduct quantitative analyses of these regional carbon emission differences, and even fewer endeavors have been made to structurally decompose such variances. To date, the most recent contributions include the study by Jia et al. (2023), which examined regional discrepancies in CO2 emissions across various sectors in China. They applied the extended logarithmic mean divisia index to attribute the changes of CO2 emissions from multi-sectors and multi-stages. Furthermore, numerous scholars have studied the Spatiotemporal Dynamics and Sustainable Policy of Direct Carbon Emissions from CO2 emissions (Sun et al., 2022; Liu et al., 2023). The existing literature offers a cornerstone of theory for current research. However, it unveils vistas for further exploration. First, beef cattle farming is a pivotal contributor to carbon emissions within the livestock sector. There exists inadequate research on the complete long-term analysis of China’s carbon emissions from the beef cattle farming industry. Therefore, it is necessary to perform a complete analysis. To accomplish a complete analysis, we selected the study period beginning from the inception and subsequent ascendancy of China’s beef cattle industry as the preeminent sector within the nation’s livestock farming industry. Therefore, we considered the period from 2008 selected as it was of great significance owing to China’s formulation of the “National Beef Cattle Advantageous Regional Planning (2008–2015)” in China. The extant research has predominantly focused on carbon peaking hypotheses and the efficiency of carbon emissions, with a lack of scholarly endeavors into its regional impacts and determinants. Second, to unravel the factors influencing carbon emissions, this study employs generalized linear models, coupled with an attentive selection of variables and meticulous regularization, which is the primary objective of this study. The other objectives include a detailed analysis of the current characteristics and changes in the mechanism of carbon emissions in the beef cattle farming sector and the main drivers behind these changes. Generally speaking, carbon dioxide emissions are influenced by a confluence of factors, each exerting varying impacts on emission levels. Consequently, this study closely aligns with the specific constraints on China’s beef cattle farming industry. It considers various factors that influence the CO2 emissions from this industry. Furthermore, the nation is divided into five distinct production regions: Northeast, Central Plains, Northwest, Southwest, and remaining areas (excluding Beijing, Shanghai, Hainan, Hong Kong, Macao, and Taiwan), to facilitate a detailed analysis of the regional characteristics, temporal fluctuations, and determinants of carbon emissions within China’s beef cattle farming industry.
As China’s beef cattle industry advanced, the nation’s beef output steadily increased from 6.177 million tonnes in 2008 to 7.183 million tonnes in 2022, reflecting a growth of 16.29%. In tandem with this growth, there has been a significant evolution in the geographical layout of the leading beef production regions. Notably, the Northeast prime region has witnessed a consistent expansion in beef production figures, escalating from 1.534 million tonnes in 2008 to 2.012 million tonnes in 2022. Thereby, leading to an increase in its contribution to the national beef output from 24.83% in 2008 to 28.01% in 2022, an enhancement of 3.17 percentage points. Recently, with the consolidation of the Northeast as a pivotal hub for national grain production, the region has witnessed an augmentation in its corn cultivation area. Catalyzed by straw-fed cattle projects and similar initiatives in certain locations, the Northeast’s beef production has maintained a trajectory of stable growth. Beef production in the Central Plains advantageous region has notably shrunk. The region’s beef output plunged from 2.287 million tonnes in 2008 to 1.669 million tonnes in 2022, with its contribution to the national total decreasing from 37.02% in 2008 to 23.24% in 2022, representing a substantial decline of 13.79 percentage points. This downturn can be attributed to the rapid pace of agricultural mechanization and the abundant non-agricultural job opportunities near Shanghai, Jiangsu, and Zhejiang. Furthermore, the Central Plains, except for certain areas, commonly confront high costs associated with breeding cattle, exacerbating the reduction in beef production within this region. Beef production in the Northwest advantageous region has experienced growth, increasing from 0.616 million tonnes in 2008 to 0.980 million tonnes in 2022. The proportion of national beef output also increased, from 9.97% in 2008 to 13.64% in 2022, an increase of 3.67 percentage points. This expansion can be partly attributed to the implementation of the state’s initiative to convert grazing land back to forest and grass, which led to an increase in the forage planting area in certain parts of the Northwest. Forage cultivation has facilitated favorable conditions for the advancement of beef cattle farming in this region. Meanwhile, the Southwest advantageous region has witnessed a modest growth in beef production, increasing from 0.827 million tonnes in 2008 to 1.279 million tonnes by 2022. Its contribution to national beef output also increased from 13.39% in 2008 to 17.81% in 2022, representing a rise of 4.42 percentage points. This growth can be partly attributed to the abundant forage resources found on the southern grassy hills and slopes. Recently, certain areas within this region have prioritized beef cattle breeding as a pivotal industry for development at the county and village levels. Furthermore, some southern locales are renowned for their superior-quality beef cattle breeds, which often fetch higher market prices than their northern counterparts. These factors have led to a gradual expansion in beef production in the Southwest region. However, variations in regional layouts, disparities in beef cattle production efficiency, and differences in environmental regulations across regions have resulted in noticeable differences in carbon emissions from the beef cattle farming industry among these areas (Table 1).
Therefore, this study analyzes the relationship between beef industry benefits and carbon emissions by measuring the carbon emissions of primary factors of production in the process of beef cattle production in China and studying the beef production process of carbon emission levels from concrete data. It not only has specific reference significance in the beef industry policy formulation and implementation of agricultural policy but is also beneficial to ensure the sustainable development of the beef cattle breeding industry.
The pursuit of low-carbon development in the beef cattle farming industry hinges on reducing carbon emissions across all production phases, from pre-to post-production. This study, informed by Cheng and Yao (2024), quantifies the entire lifecycle of carbon emissions from beef cattle farming. Embracing the life-cycle approach, we segment the process into six pivotal stages: cultivation of feed grains, transportation of feed grains, intestinal fermentation, manure management, rearing of beef cattle, and slaughtering and processing. The emissions generated at each stage are meticulously measured and aggregated to derive a standardized total carbon emissions figure for beef cattle farming (Pigot et al., 2023).
As articulated by the United Nations Intergovernmental Panel on Climate Change (IPCC), the IPCC coefficient method is the gold standard for quantifying the carbon emissions from livestock during intestinal fermentation and manure management. It is the most extensively applicable and prevalent carbon accounting protocol and is tailored for comprehensive assessments at national or provincial levels (Jahan et al., 2023; Bayer et al., 2023; Li J. et al., 2023). Combining the life cycle approach with the IPCC coefficient method, the formula for calculating carbon emissions from the beef cattle farming industry is as follows:
where Y denotes the carbon emissions attributable to the beef cattle farming industry. X represents the volume of beef production, Q denotes the number of beef cattle, and T denotes the total beef cattle stock maintained. The coefficients ef1, ef2……ef7 correspond to the emission factors for the respective stages: feed grain cultivation, feed transportation, intestinal fermentation, methane (CH4) generation from manure, nitrous oxide (N2O) generation from manure, general cattle breeding practices, and slaughtering and processing. GWPCH4 and GWPN2O are the greenhouse warming potential weights assigned to CH4 and N2O, respectively.
We use the Kernel Density Estimation (KDE) method to elucidate the dynamic distribution of carbon emissions from China’s beef cattle farming industry between 2008 and 2022. The positioning of the KDE curve provides insights into the levels of carbon emissions, whereas its spread and tail characteristics portray disparities in emission levels between provinces with the highest and lowest carbon footprints. Additionally, the number of peaks within the curve indicates the extent of polarization of carbon emissions across various regions within the beef cattle sector (Ruehr et al., 2023; Liu et al., 2022). The corresponding functional expression is as follows:
where Y denotes the carbon emission levels of the beef cattle farming industry across various provinces. The term represents the kernel density function, h is the bandwidth parameter, and N is the number of observations and represents the mean carbon emissions from the beef cattle farming industry in each province.
By applying the aforementioned analytical techniques, this study further utilizes the Dagum Gini coefficient to examine regional disparities in carbon emissions. This method allows for more accurate identification of the underlying causes of regional variances in carbon emission levels. The formula used for this calculation is as follows:
where GI represents the Gini coefficient, r denotes the number of beef cattle production regions, t signifies the number of provinces, tk and tj are the quantities of provinces within regions k and j respectively, Yks (Yju) indicates the carbon emissions from the beef cattle farming industry in province s(u) within region k(j), and Ya is the mean carbon emissions from the beef cattle farming industry.
The factors influencing carbon emissions from beef cattle farming are intricately linked and complex. To effectively eliminate irrelevant explanatory variables or to minimize omitting critical information, thereby enhancing the model’s predictive accuracy during the modeling process, this study utilizes the Lasso regression method (Li L. et al., 2023; Ahrens et al., 2020). This technique is a form of compressed estimation aimed at reducing high-dimensional data and involves variable selection and complexity regulation while fitting generalized linear models. This is achieved by shrinking the coefficients of the non-essential features to zero, which indirectly aids in extracting the salient characteristics from the influential factors, ultimately pinpointing the critical driving forces. This method strategically incorporates variables into the model to improve performance metrics by meticulously selecting variables and diminishing model complexity. This circumvents the limitations of least squares estimation and stepwise regression local optimum estimation and addresses multicollinearity among the variables.
As conventional Lasso regression imposes a uniform penalty across all variables, it yields biased estimators. To address this issue, Tran et al. (2023) introduced the adaptive Lasso method, which endows each coefficient with an individual weight, thus mitigating estimation bias. The adaptive Lasso model is formulated as follows:
where denotes the weight function, defined as , where . The weight is an inverse function of , signifying that it assigns a lesser penalty to larger magnitudes of , thereby diminishing the bias and potentially yielding unbiased estimators. Conversely, a more significant penalty is conferred for smaller magnitudes of , resulting in a more refined estimation model. Cross-validation is utilized to employ the adaptive Lasso model to select influential factors, and the optimal regression parameters are determined using the extended Bayesian information criterion (Wu et al., 2021).
Numerous carbon emission-related factors influence the Chinese beef cattle farming industry. Considering data availability, this study selected the following factors as explanatory variables, with descriptions provided for each: (1) Environmental regulation: it is often employed as a principal strategy for curbing environmental pollution, serves as an indicator of carbon emission levels and can elucidate the shifts in carbon emissions within China’s beef industry (Ali et al., 2023; Pei et al., 2019; Rodríguez et al., 2017; Singbo et al., 2020; Neves et al., 2020). Recognizing that provinces with robust economic growth typically enforce stricter environmental standards, exert stronger regulatory measures, and possess specific capabilities for ecological oversight, it follows that smaller jurisdictions are likely to maintain higher-quality environmental regulations. Consequently, this study gages the environmental regulation variable by integrating regional economic development with provincial area dimensions. (2) Arable land area per livestock unit: To a certain extent, this metric reflects the endowment of resources. It correlates positively with the number of beef cattle raised, represented as the ratio converting beef cattle numbers into an equivalent number of pigs, by the “Technical Guide for Assessing the Land Carrying Capacity of Livestock and Poultry Waste” which standardizes the conversion of beef cattle into pig equivalents. (3) The operational scale of beef cattle producers: This is indicated by the proportion of beef cattle producers at various scales relative to the total number of producers. (4) Efficiency of production: This metric encapsulates the influence of resource allocation, labor dynamics, and cultivation techniques on cattle rearing, as measured by the carbon emission burden associated with the cattle farming industry per unit of beef produced per cow and (5) the composition of livestock products: The share of beef within the meat category. (6) Degree of mechanization (Peláez-Samaniego et al., 2008; Amin, 2022). An increased level of mechanization enhances the utilization efficiency of resources such as coal and electricity, minimizes waste, and, in turn, diminishes carbon emissions from the beef cattle sector. This indicator is calculated as the ratio of total agricultural machinery power to total area sown. (7) The local veterinary workforce: By providing essential low-carbon technology support and disease prevention inspections, local veterinary services enable carbon emission reduction goals within the beef cattle industry. (8) Disposable income per capita of rural residents: The financial status of beef cattle producers affects their motivation and the extent of cattle rearing, which subsequently indirectly affects carbon emissions within the beef industry. (9) Urbanization rate: As urbanization increases, reducing the rural population percentage creates a conducive environment for the industrialized growth of beef cattle farming, thereby aiding in carbon emissions reduction (Prastiyo et al., 2020; Martínez-Zarzoso and Maruotti, 2011; Barla et al., 2011). (10) Transportation infrastructure (Hendricks et al., 2018): This is expressed as the railway and roadway mileage ratio of the regional area (Table 2).
The calculation indicators for carbon emissions from the beef cattle farming industry and the corresponding influencing factors, including environmental regulations, land area per livestock unit, scale of breeding operations, and production efficiency, are derived from authoritative sources such as the “China Statistical Yearbook,” “China Rural Statistical Yearbook,” “China Animal Husbandry and Veterinary Yearbook,” and various provincial statistical yearbooks.
The results indicate that carbon emissions from China’s beef cattle farming industry have increased substantially in recent years. By 2022, the total carbon footprint of the sector had risen to 1040.75 million metric tonnes, representing a 37.62% growth since 2008. Examining the time series characteristics of carbon emissions from China’s beef cattle farming industry, there has been an increasing trend in total emissions from 2008 to 2022, with an average annual growth rate of 2.31%. The evolution of carbon emissions from China’s beef cattle farming sector has transpired in three varied phases: “substantial increase, followed by a rapid decline, and then sustained growth,” with the detailed progression described as follows:
The first phase (2008–2016) was marked by a significant increase in carbon emissions from the beef cattle farming sector. Notwithstanding the minor setback in emissions in 2011, the overall trajectory continued to increase. By 2016, there had been a 29.58% surge in carbon emissions compared to 2008, maintaining an average annual growth rate of 3.29%. This significant increase can be explained primarily by the robust expansion and growing scale of China’s beef cattle industry during this timeframe, and a steady increase in energy consumption for livestock production.
The second phase (2016–2017) was defined as a rapid decrease in carbon emissions from the beef cattle farming sector. There was a significant drop of 12.60% in emissions in 2017 compared with the previous year. This notable reduction was primarily owing to the influence of low-priced imported beef products, which disrupted the domestic market and led to fluctuations in the scale and yield of China’s beef cattle industry, impacting carbon emissions figures.
The third and final phase (2017–2022) was marked by a persistent upward trend in carbon emissions within the beef cattle farming sector, with a 21.51% increase by 2022 over the baseline year of 2017. This surge had its roots in dual influential factors: first, the containment of illicit imports of beef products led to improved profitability in the domestic beef cattle industry, which encouraged breeders to capitalize on this boom and enlarge their breeding operations; second, the African swine fever outbreak resulted in soaring pork prices, enhancing the substitution effect of beef owing to robust demand. The economic viability of cattle farming has significantly improved, fostering an expansion of the industry and, consequently, an escalation in carbon emissions.
An analysis of carbon emissions from the beef cattle farming industry across China’s premier production regions reveals significant disparities. Throughout the observation period, the Central Plains region notably achieved “negative growth” in carbon emissions, with the 2022 figure standing at 143.84 million metric tonnes, a substantial decrease of 32.80% since 2008, representing an average annual decline of 2.80%. The refinement of the market economy structure in the Central Plains region has been instrumental in fostering carbon emission reductions in the beef cattle farming sector. Conversely, beef cattle farming sectors in Northeast, Northwest, and Southwest China experienced a progressive annual increase in carbon emissions. This uptrend is primarily attributed to the shift in China’s primary beef cattle production areas from the Central Plains toward the Northeast, Northwest, and Southwest regions.
Between 2008 and 2022, carbon emission levels from the beef cattle farming industry in the Northeast region surged by 40.36%. The Northeast region, recognized as a critical grain-producing region within China, leverages its wealth of forage resources to foster a cost-effective environment for cultivating the beef cattle industry. Furthermore, the region intends to advance integrated agro-pastoral systems and has strongly emphasized the selective breeding and genetic enhancement of beef cattle. This concerted effort, underpinned by the region’s natural resource endowments and complemented by enabling policy frameworks, has culminated in the prosperous development of the beef cattle sector and an inevitable increase in carbon emissions. During the observation period, carbon emissions from beef cattle farming in the Northwest and Southwest regions rose from 58.15 million metric tonnes and 169.52 million metric tonnes in 2008 to 157.30 million metric tonnes and 252.20 million metric tonnes in 2022, respectively. Notably, the carbon emission levels in the Northwest region witnessed a significant rise, approaching 1.7 times the baseline figure.
In contrast to the Central Plains and Northeast regions, the Northwest and Southwest regions are geographically closer to the southern market, where the per capita beef consumption is significantly higher. To meet robust beef demand, these western regions have been compelled to utilize their advantages in beef cattle farming, which has consequently triggered a significant surge in carbon emissions. The other areas witnessed an approximate 70.8% increase in carbon emissions from the beef cattle industry during the same period. Encompassing a broader array of provinces, the primary impetus behind the escalation in carbon emissions in these areas was an increase in the number of cattle farmed over time within their respective provinces (Figure 1).
Figure 2 shows a visual representation of the dynamic progression of carbon emissions from China’s beef cattle farming industry during the observation period. With the carbon emission levels of the beef cattle industry in 2008 serving as the reference point, a rightward shift is evident in the kernel density curve, signifying a consistent upward trend in emissions throughout the sampled timeframe. In 2008 and 2022, the kernel density curves exhibit a pronounced bimodal distribution. In 2008, the principal peak occurred at lower carbon emissions and was accompanied by a secondary peak in the higher emission range. By 2022, the curve’s primary peak shifted toward the higher emission band, with the secondary peak receding to the lower emission quadrant. This shift in peaks’ prominence suggests that a handful of provinces were initially responsible for a significant share of emissions, while the majority emitted less. However, by 2022, the situation had flipped, with the bulk of the provinces contributing more significantly to carbon emissions and fewer provinces emitting less. The probable cause for this shift is the change in the primary beef cattle-producing regions; the Central Plains region has experienced a decline in its dominant position concerning beef cattle capacity, while the Northeast, Northwest, and Southwest regions have gained prominence in China’s beef cattle industry. The emergence of a new peak signifies a bifurcation in carbon emissions from China’s beef cattle farming sector during a specified timeframe. The 2008 curve displays a prominent right skewness which diminishes by 2022, indicating that the disparity between the provinces with the highest and lowest carbon emissions from beef cattle farming is narrowing. This is owing to the varying levels of economic development across different regions, which influences the feasibility of achieving carbon emission reductions. The country has encouraged areas with higher economic status and better technological infrastructure to lead carbon reduction efforts, reducing the differences among the provinces.
Figures 2B–F sequentially illustrate the dynamic evolution of carbon emissions from the beef cattle farming industry in the Northeast, Central Plains, Northwest, Southwest and other regions, regions during the observation period. In the Northeast region, the carbon emission kernel density curve has uniformly shifted to the right, with a reduced peak height and an increased curve width, signaling a progressive increment in carbon emissions from the beef cattle industry. This shift also highlights substantial variances in carbon emission levels among the provinces within the region. The emergence of both leftward and rightward tails on the kernel density curve points to distinct gradient differences in carbon emissions across the beef cattle sector in the Northeast. The underlying cause is the swift expansion of the beef cattle industry in Inner Mongolia and Heilongjiang provinces, which have significantly surpassed the other two provinces in scale and number of cattle, widening the developmental gap in the beef cattle industry among the four provinces. The Central Plains region’s carbon emission kernel density curve has predominantly shifted to the left, exhibiting an elevated peak and a reduced width. This shift signifies a diminishing disparity in carbon emissions within the beef cattle industry across the provinces in the region, indicating a trend toward convergence in carbon emission variance. The curve exhibited tailing in 2008, which significantly converged in the later periods, suggesting that early on, there were gradient differences in carbon emissions within the Central Plains region that dissipated over time. A likely interpretation is that Henan province initially outpaced other areas in developing its beef cattle industry. However, as economic restructuring took place over the later periods and the growth of the industry decelerated, the development of the beef cattle industry across the four provinces in the region gradually converged to a similar level, thereby indirectly lessening the regional differences in carbon emissions from this sector. The carbon emission kernel density curves for the Northwest, Southwest, and other regions have all shifted rightward, characterized by a lower peak and a broader span. This trend signifies a rise in carbon emissions from the beef cattle industry within these areas, accompanied by significant variations in emissions across the provinces. The emergence of trailing edges in the kernel density curves for these three major regions implies the persistence of gradient differences in carbon emissions from the beef cattle industry across the regions.
An examination of the overall Gini coefficient for carbon emissions within China’s beef cattle farming sector reveals a notably high coefficient, with an average value of 0.3868. The coefficient exhibits a pattern of fluctuation with an overall downward trajectory, suggesting that although there are considerable regional discrepancies in carbon emissions across the industry, these disparities progressively narrowed over time. Considering the Gini coefficient for carbon emissions within various regions of China’s beef cattle farming industry, it is evident that the most substantial differences in emission levels are found in other areas, with a coefficient value of 0.4781, surpassing the national average. Owing to the inclusion of a more significant number of provinces than the four major production regions and a more extensive geographical spread, these regions exhibit diverse industrial statuses for beef cattle farming across their provincial areas. This diversity, coupled with a lack of uniformity in the progress of carbon emissions reduction initiatives, has resulted in pronounced imbalances in industrial development and significant disparities in regional carbon emissions. In the Southwest and Northwest regions, the average Gini coefficient values are 0.3389 and 0.3094, respectively, reflecting internal disparities within the beef cattle farming industry across the nine western provinces. During the observation period, the Central Plains region showed a fluctuating yet consistent downward trend in its Gini coefficient, with a reduction of 39.43%. This decline was mainly owing to economic restructuring in Henan Province, which decreased the number of beef cattle farms. Consequently, the development trajectories of the beef cattle farming industry in the four provinces of the Central Plains began to converge, mitigating the regional differences in carbon emissions from this sector. The Northeast region boasts the lowest Gini coefficient, with an average value of 0.0958, suggesting a relatively uniform distribution of carbon emissions from the beef cattle farming industry across the area. Nevertheless, a marked increase in the sample values post-2016 has led to a broader divergence in regional disparities. This shift aligns with the polarization trends identified in the evolving patterns of carbon emissions within the beef cattle farming sector in the Northeast region (Figure 3).
Figure 3. Regional variations in carbon emissions within the beef cattle farming sector across the country and the five key production areas.
Analysis of the inter-regional Gini coefficient for carbon emissions in China’s beef cattle farming industry revealed that the most pronounced differences were between other regions and the Southwest, with a coefficient value of 0.4857, highlighting the substantial disparities in carbon emissions from beef cattle farming across these areas. Conversely, the least variation in carbon emissions was observed between the Northeast and Central Plains, where the coefficient value was 0.2340. Significant inter-regional disparities in carbon emissions from the beef cattle farming industry were evident among various regions, including the Northeast versus other regions, the Central Plains versus other regions, the Central Plains versus the Northwest, other regions versus the Northwest, other regions versus the Southwest, and the Northwest versus the Southwest, with Gini coefficient values surpassing 0.4000. A comparative analysis of these coefficients reveals that the most substantial divergence is between other regions and the Southwest, which emerges as a critical factor influencing inter-regional carbon emission variance within China’s beef cattle sector. An examination of the evolution of the Gini coefficient reveals a notable increasing trend between the Northeast and the Southwest, with an increase of 10.54%. This points to a considerable gap in beef cattle farming practices and industrial foundations between these two regions, leading to an overly wide interregional divergence. Meanwhile, the Gini coefficients for other inter-regional combinations declined. This suggests that despite pronounced spatial and temporal variations in carbon emission disparities across the five major beef cattle production areas, there is a general trend toward diminishing differences in inter-regional carbon emissions (Figure 4).
An analysis of the sources and contribution rates of regional differences in carbon emissions from China’s beef cattle farming industry between 2008 and 2022 reveals that while the intra-regional contribution rate remained relatively steady, the inter-regional and ultra-high-density contribution rates underwent notable changes. The mean annual intra-regional contribution rate was 18.31%, the mean annual ultra-high-density contribution rate was 28.96%, and the mean annual net inter-regional contribution rate was 52.52%. The inter-regional contribution rate, being the most substantial of the three, clearly demonstrates that the principal driver of regional disparities in carbon emissions within China’s beef cattle farming sector is the variance between regions. The trends in the three types of contribution rates vary considerably; there has been a notable decrease of 33.36% in the interregional contribution rate. In contrast, the intra-regional and ultra-high-density contribution rates showed increasing tendencies, with respective rises of 67.37 and 25.58%, respectively. Narrowing the gaps between regions could help advance carbon emission reductions in the beef cattle farming sector in China. Nevertheless, it is equally crucial to monitor the ascending trends in both intra-regional contributions and ultra-high density (Figure 5).
To discern the pivotal factors affecting carbon emissions in beef production, this study devised a comprehensive panel data model that mirrors the factors that influence carbon emissions within the beef cattle sector. The model is subsequently estimated using an adaptive Lasso approach with national provincial-level panel data, with the findings presented in Table 1. To control for the variance in model disturbances, autocorrelation, and the possible effects of outliers, this study used a cross-validation method to estimate the control function. We also compared these findings with the results obtained from the fixed effects model and random effects model regressions to confirm the robustness and efficacy of the adaptive Lasso model estimates. The empirical outcomes, underscored by the significant Wald statistics for each variable, are all statistically significant at the 1% level, suggesting a perfect fit for the model.
Considering the factors that influence carbon emissions mitigation, the estimation results revealed that environmental regulations significantly propel carbon reduction in beef cattle farming, with an impact coefficient of 0.230, which is statistically significant at the 1% level. This underscores the considerable role that environmental regulations play in fostering decreased carbon emissions within the sector. Fortifying the impact of environmental regulations on diminishing carbon emissions in beef cattle farming and synergistically reducing greenhouse gas discharge through source control and recycling are pivotal for realizing a low-consumption, low-emission, and high-efficiency beef production model. Such measures are of substantial importance for mitigating carbon emissions within the sector. Historically, pollution from livestock has not been a central concern of governmental policies; nevertheless, with the growing stringency of environmental regulations, it has become imperative for farms to incur certain costs associated with using ecological resources (Copeland and Taylor, 1993). The impact of environmental regulations on carbon emission reduction in the beef cattle sector is derived from two main aspects: first, the “regulatory-induced emission reduction effect” which spurs producers to pursue technological innovations and improve efficiency, thereby accomplishing the dual objectives of reducing carbon emissions and enhancing productivity (Piot-Lepetit and Moing, 2007); second, the “pollution haven” effect, which drives the migration of carbon-intensive industries from areas with strict environmental regulations to those with more relaxed standards (Casson et al., 2023).
Production efficiency exerts a notably adverse effect on carbon emissions from beef cattle farming, corroborating the significant pro-carbon reduction effect of augmented production efficiency (Valin et al., 2013). Assessing the influence coefficients, the mitigating effect of production efficiency on emissions transcends that of other factors, underscoring that enhancing the production efficiency of beef cattle constitutes a productive pathway for carbon emission reduction in China’s beef cattle industry. Boosting production efficiency can substantially improve irrational methods of agricultural production, mitigate the squandering of resources such as water and agricultural inputs, and curtail the growth trend of carbon emissions from beef cattle farming, both directly and indirectly, during the pre-production and production stages. Hence, enhancing production efficiency is pivotal for reducing carbon emissions in the beef cattle industry. The veterinary workforce in rural townships contributes significantly to emissions reductions in the beef cattle sector. During the beef cattle breeding process, veterinarians can swiftly execute disease inspections and surveillance, elevate the standards of beef cattle farming management, counsel breeders on the most suitable farming practices, curtail feed consumption, and effectively mitigate carbon emissions during the production cycle. Furthermore, these veterinary experts can promote low-carbon breeding techniques among producers, substantially reducing the reliance on chemical fertilizers and pesticides, advocating clean energy adoption, and enhancing the energy efficiency imperative for beef cattle rearing. This generates economic value for beef cattle, safeguards the environment, and substantially reduces carbon emissions (Table 3).
The arable land area per livestock unit, composition of livestock products, disposable income per capita of rural residents, and state of transportation infrastructure all significantly influence the increase in carbon emissions from the beef cattle sector, aligning with expectations. In particular, the composition of livestock products is such that the growing share of beef in meat consumption inevitably increases carbon emissions. This underscores the inescapable challenge in developing China’s beef cattle industry: reconciling the tension between supply and demand while reducing carbon emissions. Hence, it is essential to refine the structure of livestock products to satisfy consumer needs while simultaneously reducing carbon emissions from the beef cattle sector. The ratio of cultivated land per livestock unit significantly catalyzes carbon emissions within the beef cattle sector, stemming from the principle of “land-livestock balance, where land dictates livestock capacity.” An expansion in arable land area permits an increase in beef cattle, which correspondingly escalates emission levels. It is crucial to highlight that while judiciously diminishing land area could curb the number of livestock and thus reduce emissions, it might concurrently lead to a decline in beef production. To offset this reduction, additional land must be cultivated for cattle rearing, which could potentially lead to an indirect increase in carbon emissions. The average disposable income of rural residents plays a significant role in driving carbon emissions from the beef cattle sector. An increase in income bolsters producers’ enthusiasm, and they tend to widen their breeding operations for enhanced economic returns. This results in a heightened input of production elements such as feed and a concomitant increase in energy use for farming activities, inevitably leading to increased carbon emissions.
Furthermore, if manure is not managed efficiently and promptly, it can harm carbon emission mitigation efforts in the beef cattle sector. Additionally, transportation conditions are conducive to the carbon footprint of the industry. Favorite transport links reduce the distance between producers and markets, thereby facilitating the transport of beef products. As transportation is an integral part of the life cycle of beef cattle, an escalation in carbon emissions during this phase could engender a comprehensive impact on lifecycle carbon emissions associated with beef cattle.
Other key control variables, including the scale of farming households, the level of mechanization, and urbanization, have also passed significance tests. The Chinese beef cattle farming industry is characterized by small-scale and large groups, with the current industrial structure still primarily composed of small-scale free-range farmers. However, as the degree of specialization and standardization within the industry escalates, there is a marked enhancement in the scaling of beef cattle operations, from 25.58% in 2008 to 29.45% in 2022, manifesting a gradual yet significant influence on the sector. Furthermore, while mechanization can facilitate carbon reduction in beef cattle farming to a certain extent, the predominantly small-scale and free-range nature of China’s beef cattle farming means that the level of mechanization remains relatively subdued. Nevertheless, it contributes positively to carbon reduction efforts and is poised to be a key strategy for carbon mitigation in China’s beef cattle farming in the foreseeable future. Urbanization exerts its influence primarily through the continual migration of the rural population to cities, which reduces the rural labor force and thereby promotes the scaling of beef cattle farming as a carbon reduction measure. However, as urbanization accelerates, the heightened demand for beef cattle spurred by urbanization fuels the swift expansion of the beef cattle farming industry. Concurrently, the mass exodus of farmers from the beef cattle sector due to urbanization fosters larger-scale farming practices, also impacting the carbon footprint of the beef cattle farming industry.
Within the broader objectives of peaking carbon emissions, achieving carbon neutrality, embracing new development philosophies, shaping a novel development paradigm, and fostering high-quality growth, the push toward low-carbon development in the beef cattle farming sector is not only in harmony with China’s green growth aspirations but also echoes the principle that “Clear waters and green mountains are as good as mountains of gold and silver.” This approach can mitigate greenhouse gas emissions and safeguard the ecological balance, ensuring sustainable prosperity of the environment. This study considered data on China’s beef cattle industry from 2008 to 2022 to estimate carbon emissions. Subsequently, this study adopted kernel density estimation and the Dagum Gini coefficient as analytical tools to examine the sector’s dynamic progression and regional disparities in carbon emissions. The conclusions of this study are as follows:
First, between 2008 and 2022, China’s beef cattle farming industry witnessed an incremental growth in carbon emissions, culminating in a peak in 2022. The carbon emissions trajectory within this period was characterized by diverse phases: an initial rapid increase, a substantial decline, and a continuous rise. Among the various key producing areas, the Central Plains region uniquely experienced a “negative growth” in carbon emissions from beef cattle farming. Conversely, the Northeast, Northwest, Southwest, and other areas exhibited progressive increases in carbon emissions during the examined timeframe. As China’s economic development and urbanization progress to higher echelons in the future, there is an anticipated surge in the demand for beef among Chinese consumers. Consequently, to achieve the carbon reduction targets, it becomes imperative to promote the adoption of development paradigms that are both green and low-carbon. Hence, there should be a concerted effort to propagate a sustainable, green, and low-carbon development approach within the beef cattle farming industry. This involves overhauling the conventional extensive operational models and rationally redistributing modern, eco-friendly, and low-carbon resources to catalyze a green and low-carbon metamorphosis, particularly in regions with elevated carbon emissions. It is imperative to hasten the formulation of a coherent and productive policy framework that harnesses beef cattle manure as a resource, encourages its multifaceted application, and establishes an advanced circular economic paradigm for livestock breeding. Furthermore, there is a need to build an efficient and clean energy ecosystem, embrace green livestock management practices, and introduce large-scale, standardized husbandry techniques to diminish the environmental footprint of pollutants.
Second, when examining dynamic evolutionary patterns, it is evident that the overall national trends and those in the Northeast, Northwest, Southwest, and other regions demonstrate an increasing trajectory. Conversely, the Central Plain region exhibited a decreasing trend. The overall national carbon emissions from the beef cattle farming industry exhibited a polarization phenomenon, with the Northeast, Northwest, Southwest, and other regions displaying gradient differences in carbon emissions. Considering regional disparities, the carbon emission variance within China’s beef cattle farming industry is substantial; however, there is a general trend toward convergence. The most significant regional differences in carbon emissions are found in the other regions, with the Southwest, Northwest, and Central Plains regions following suit and the Northeast exhibiting the slightest variation. The inter-regional difference is most pronounced between the other areas and the Southwest, whereas it is least notable between the Northeast and the Central Plains. Inter-regional differences are identified as the main driver of the disparities in carbon emissions across various regions in China’s beef cattle farming industry. In light of the regional characteristics of China’s beef cattle farming and the regional traits of carbon emissions, it is imperative to establish a collaborative governance scheme for carbon reduction in the beef cattle farming industry. Therefore, developing a synergistic governance plan for carbon emission reduction within the beef cattle farming sector is essential. The regional disparities in carbon emissions within China’s beef cattle farming industry are significant, and an increasing degree of imbalance between regions needs to be conducive to collaborative regional governance. By adhering to the tenets of minimization, efficiency, and harmlessness, there is a need to focus on cultivating a sustainable cycle of “low consumption - zero pollution - high output - recycling - increased profitability” to mitigate carbon pollution. This strategy will encourage the moderate scaling and intensification of beef cattle farming, ultimately realizing green and low-carbon emission reduction goals within the industry.
Third, environmental regulations, production efficiency, and the township veterinary workforce are considered to have a critical negative influence on carbon emissions from beef cattle farming in China. Production efficiency is a critical factor in suppressing carbon emissions across industries. Conversely, the arable land area per livestock unit, composition of livestock products, average disposable income of rural residents, and transportation infrastructure significantly increased carbon emissions, with the structure of livestock products being the predominant contributor to the industry’s carbon footprint. Other key control variables, i.e., the scale of producer, degree of mechanization, and urbanization rate exhibit statistically significant impacts as well. Based on the research conclusions presented above, fortifying and refining the policy support framework for low-carbon beef cattle farming is imperative. This involves instituting incentives and compensation schemes that encourage low-carbon agricultural practices, complementing stringent governmental environmental regulations. Efforts should be made to reinforce the linkages between beef cattle producers and market dynamics, easing the financial risks of adopting low-carbon breeding techniques through subsidization and investment support. Additionally, there is a need to bolster the financial infrastructure conducive to low-carbon production, devise favorable credit policies for low-carbon farming endeavors, and safeguard the breeders’ economic welfare and compliance with environmental statutes. Furthermore, rigorous monitoring of the enforcement of low-carbon farming legislation is essential, along with the cultivation of an expert and highly trained law enforcement workforce to ensure strict adherence to these statutes.
The datasets presented in this study can be found in online repositories. The names of the repository/repositories and accession number(s) can be found in the article/supplementary material.
JW: Conceptualization, Writing – original draft, Writing – review & editing. YL: Investigation, Methodology, Supervision, Writing – review & editing. JC: Project administration, Validation, Visualization, Writing – review & editing.
The author(s) declare that financial support was received for the research, authorship, and/or publication of this article. This research was funded by the Ministry of Agriculture Modern Industrial Technology System Project, “Economic Research on China’s Beef and Yak Industries” (CARS-37); National Natural Science Foundation of China, “Study on the Effective Conversion Paths and Incentive Policies for Non-Material Agricultural Technologies” (72173054); Agriculture Modern Industrial Technology System Project of The Tibet Autonomous Region, “Economic and Policy Research on Yak Industries”(XZARS-MN-2024).
The authors wish to acknowledge Jilin Agricultural University and Bohai University for Research, Innovation, and Discovery for making the publication of this article in an open access journal possible. We also thank the reviewers for their helpful and extensive comments that contributed to a greatly improved manuscript.
The authors declare that the research was conducted in the absence of any commercial or financial relationships that could be construed as a potential conflict of interest.
All claims expressed in this article are solely those of the authors and do not necessarily represent those of their affiliated organizations, or those of the publisher, the editors and the reviewers. Any product that may be evaluated in this article, or claim that may be made by its manufacturer, is not guaranteed or endorsed by the publisher.
Ahrens, A., Hansen, C. B., and Schaffer, M. E. (2020). Lassopack: model selection and prediction with regularized regression in Stata. Stata J. 20, 176–235. doi: 10.1177/1536867X20909697
Ali, A., Radulescu, M., and Balsalobre-Lorente, D. (2023). A dynamic relationship between renewable energy consumption, nonrenewable energy consumption, economic growth, and carbon dioxide emissions: evidence from Asian emerging economies. Energ. Environ. 34, 3529–3552. doi: 10.1177/0958305X231151684
Amin, S. (2022). Long-term and short-term effects of agricultural modernization on carbon emissions: evidence from Nepal, Bangladesh, Pakistan, and India. J. Clean. Prod. 31, 1–12.
Barla, P., Miranda-Moreno, L. F., and Lee-Gosselin, M. (2011). Urban travel CO2 emissions and land use: a case study for Quebec City. Transp. Res. Part D: Transp. Environ. 16, 423–428. doi: 10.1016/j.trd.2011.03.005
Bayer, A. D., Lautenbach, S., and Arneth, A. (2023). Benefits and trade-offs of optimizing global land use for food, water, and carbon. Proc. Natl. Acad. Sci. 120:e2220371120. doi: 10.1073/pnas.2220371120
Bell, M. J., Cloy, J. M., and Rees, R. M. (2014). The true extent of agriculture's contribution to national greenhouse gas emissions. Environ. Sci. Pol. 39, 1–12. doi: 10.1016/j.envsci.2014.02.001
Bellarby, J., Tirado, R., Leip, A., Weiss, F., Lesschen, J. P., and Smith, P. (2013). Livestock greenhouse gas emissions and mitigation potential in Europe. Glob. Chang. Biol. 19, 3–18. doi: 10.1111/j.1365-2486.2012.02786.x
Casson, A., Zambelli, M., Giovenzana, V., Tugnolo, A., Pampuri, A., Vignati, S., et al. (2023). Simplified environmental impact tools for Agri-food system: a systematic review on trends and future prospective. Environ. Impact Assess. Rev. 102:107175. doi: 10.1016/j.eiar.2023.107175
Cheng, M., and Yao, W. (2024). Trend prediction of carbon peak in China’s animal husbandry based on the empirical analysis of 31 provinces in China. Environ. Dev. Sustain. 26, 2017–2034. doi: 10.1007/s10668-022-02794-6
Copeland, B. R., and Taylor, M. S. (1993). North-south trade and the environment. Q. J. Econ., 1994 109 7, 755–787.
Crosson, P., Shalloo, L., O’Brien, D., Lanigan, G. J., Foley, P. A., Boland, T. M., et al. (2011). A review of whole farm systems models of greenhouse gas emissions from beef and dairy cattle production systems. Anim. Feed Sci. Technol. 167, 29–45. doi: 10.1016/j.anifeedsci.2011.04.001
Deng, L., Yuan, S., Bai, P., and Li, H. (2023). Evaluation of agricultural carbon emissions in Xinjiang and analysis of driving factors based on machine learning algorithms. Chin. J. Eco-Agric. 31, 265–279.
Gerber, P. J., Steinfeld, H., Henderson, B., Mottet, A., Opio, C., Dijkman, J., et al. (2013). Tackling climate change through livestock: a global assessment of emissions and mitigation opportunities. Rome: Food and agriculture Organization of the United Nations (FAO).
He, Z. W., Liu, F., Bai, L. Z., and Zhao, H. Y. (2014). Characteristics and reference of foreign beef cattle and mutton subsidy policy. World Agric. 420, 95–98.
Hendricks, J., Righi, M., Dahlmann, K., Gottschaldt, K. D., Grewe, V., Ponater, M., et al. (2018). Quantifying the climate impact of emissions from land-based transport in Germany. Transp. Res. Part D: Transp. Environ. 65, 825–845. doi: 10.1016/j.trd.2017.06.003
Hergoualc, C. (2019). The climate impact of methane and nitrous oxide emissions from agriculture. Agric. Syst. 177, 57–66.
Jahan, M., Reis, J., and Shortridge, J. (2023). Assessing climate service products with evaluation metrics: an application to decision support tools for climate change adaptation in the USA. Clim. Chang. 176:113. doi: 10.1007/s10584-023-03595-0
Jia, J., Xin, L., Lu, C., Wu, B., and Zhong, Y. (2023). China's CO2 emissions: a systematical decomposition concurrently from multi-sectors and multi-stages since 1980 by an extended logarithmic mean Divisia index. Energ. Strat. Rev. 49:101141. doi: 10.1016/j.esr.2023.101141
Johnson, D. W., and Ward, R. L. (2013). Methane emissions from livestock and manure management. Anim. Feed Sci. Technol. 185, 169–179.
Li, G., Chen, X., and You, X. Y. (2023). System dynamics prediction and development path optimization of regional carbon emissions: a case study of Tianjin. Renew. Sust. Energ. Rev. 184:113579. doi: 10.1016/j.rser.2023.113579
Li, J., Gao, M., Luo, E., Wang, J., and Zhang, X. (2023). Does rural energy poverty alleviation really reduce agricultural carbon emissions? The case of China. Energ. Econ. 119:106576. doi: 10.1016/j.eneco.2023.106576
Li, L., Liu, H., Gong, P., Lin, E., Bai, Z., Li, P., et al. (2023). Multi-objective optimization of winter irrigation for cotton fields in salinized freeze-thaw areas. Eur. J. Agron. 143:126715. doi: 10.1016/j.eja.2022.126715
Liu, Z., Deng, Z., Davis, S. J., Giron, C., and Ciais, P. (2022). Monitoring global carbon emissions in2022. Nat. Rev. Earth Environ. 3, 217–219. doi: 10.1038/s43017-022-00285-w
Liu, S., Jia, J., Huang, H., Chen, D., Zhong, Y., and Zhou, Y. (2023). China’s CO2 emissions: a thorough analysis of spatiotemporal characteristics and sustainable policy from the agricultural land-use perspective during 1995–2020. Land 12:1220. doi: 10.3390/land12061220
Mar, K. A., Unger, C., Walderdorff, L., and Butler, T. (2022). Beyond CO2 equivalence: the impacts of methane on climate, ecosystems, and health. Environ. Sci. Pol. 134, 127–136. doi: 10.1016/j.envsci.2022.03.027
Martínez-Zarzoso, I., and Maruotti, A. (2011). The impact of urbanization on CO2 emissions: evidence from developing countries. Ecol. Econ. 70, 1344–1353. doi: 10.1016/j.ecolecon.2011.02.009
Neves, S. A., Marques, A. C., and Patrício, M. (2020). Determinants of CO2 emissions in European Union countries: does environmental regulation reduce environmental pollution? Econ. Anal. Policy 68, 114–125. doi: 10.1016/j.eap.2020.09.005
Opio, C. (2011). Environmental impact of livestock production: the importance of environmental improvement strategies. Livest. Sci. 129, 1–8.
Pei, Y., Zhu, Y., Liu, S., Wang, X., and Cao, J. (2019). Environmental regulation and carbon emission: the mediation effect of technical efficiency. J. Clean. Prod. 236:117599. doi: 10.1016/j.jclepro.2019.07.074
Peláez-Samaniego, M. R., Garcia-Perez, M., Cortez, L. B., Rosillo-Calle, F., and Mesa, J. (2008). Improvements of Brazilian carbonization industry as part of the creation of a global biomass economy. Renew. Sust. Energ. Rev. 12, 1063–1086. doi: 10.1016/j.rser.2006.10.018
Pelletier, N. (2010). Canadian greenhouse gas emissions from livestock production: a life cycle analysis. Can. J. Anim. Sci. 90, 377–389.
Pigot, A. L., Merow, C., Wilson, A., and Trisos, C. H. (2023). Abrupt expansion of climate change risks for species globally. Nat. Ecol. Evol. 7, 1060–1071. doi: 10.1038/s41559-023-02070-4
Piot-Lepetit, I., and Moing, M. L. (2007). Productivity and environmental regulation: the effect of the nitrates directive in the French pig sector. Environ. Resour. Econ. 38, 433–446. doi: 10.1007/s10640-007-9086-7
Prastiyo, S. E., Hardyastuti, S., and Jamhari, F. (2020). How agriculture, manufacture, and urbanization induced carbon emission? The case of Indonesia. Environ. Sci. Pollut. Res. 27, 42092–42103. doi: 10.1007/s11356-020-10148-w
Rodríguez, V., Olarte-Pascual, C., and Saco, M. (2017). Application of geographical information systems for the optimal location of a commercial network. Eur. J. Manag. Bus. Econ. 26, 220–237. doi: 10.1108/EJMBE-07-2017-013
Ruehr, S., Keenan, T. F., Williams, C., Zhou, Y., Lu, X., Bastos, A., et al. (2023). Evidence and attribution of the enhanced land carbon sink. Nat. Rev. Earth Environ. 4, 518–534. doi: 10.1038/s43017-023-00456-3
Singbo, A., Larue, B., and Tamini, L. D. (2020). Total factor productivity change in hog production and Quebec's revenue insurance program. Can. J. Agric. Econ. 68, 21–46. doi: 10.1111/cjag.12220
Smith, P., and Martino, D. (2007). Agriculture. In climate change 2007: Mitigation. Contribution of working group iii to the fourth assessment report of the intergovernmental panel on climate change. Cambridge: Cambridge University Press.
Sun, Y., Jia, J., Ju, M., and Chen, C. (2022). Spatiotemporal dynamics of direct carbon emission and policy implication of energy transition for China’s residential consumption sector by the methods of social network analysis and geographically weighted regression. Land 11:1039. doi: 10.3390/land11071039
Tran, L., Li, G., Luo, L., and Jiang, H. (2023). A fast solution to the lasso problem with equality constraints. J. Comput. Graph. Stat., 1–10.
Valin, H., Havlík, P., Mosnier, A., Herrero, M., Schmid, E., and Obersteiner, M. (2013). Agricultural productivity and greenhouse gas emissions: trade-offs or synergies between mitigation and food security? Environ. Res. Lett. 8:035019. doi: 10.1088/1748-9326/8/3/035019
Watts, N., Adger, W., Agnolucci, P., Blackstock, J., Byass, P., Cai, W., et al. (2015). Health and climate change: policy responses to protect public health. Lancet 386, 1861–1914. doi: 10.1016/S0140-6736(15)60854-6
Williams, K. (2012). Mitigating and adapting to climate change by reducing greenhouse gas emissions from the irish agricultural sector. Sci. Total Environ. 408, 1780–1791.
Wu, L., Sun, L., Qi, P., Ren, X., and Sun, X. (2021). Energy endowment, industrial structure upgrading, and CO2 emissions in China: revisiting resource curse in the context of carbon emissions. Res. Policy 74:102329. doi: 10.1016/j.resourpol.2021.102329
Yin, M., Song, P., and Yan, W. (2023). How does network infrastructure construction affect livestock carbon emissions? Agriculture 13:2244. doi: 10.3390/agriculture13122244
Keywords: beef cattle farming industry, carbon emission measurement, regional effects, influencing factors, Dagum Gini coefficient
Citation: Wang J, Liang Y and Cao J (2024) Carbon emission measurement and regional decomposition analysis of China’s beef cattle farming industry. Front. Sustain. Food Syst. 8:1417469. doi: 10.3389/fsufs.2024.1417469
Received: 15 April 2024; Accepted: 12 September 2024;
Published: 24 September 2024.
Edited by:
Chenxi Li, Xi’an University of Architecture and Technology, ChinaReviewed by:
Junsong Jia, Jiangxi Normal University, ChinaCopyright © 2024 Wang, Liang and Cao. This is an open-access article distributed under the terms of the Creative Commons Attribution License (CC BY). The use, distribution or reproduction in other forums is permitted, provided the original author(s) and the copyright owner(s) are credited and that the original publication in this journal is cited, in accordance with accepted academic practice. No use, distribution or reproduction is permitted which does not comply with these terms.
*Correspondence: Jianmin Cao, bm1nY2Fvam1AMTI2LmNvbQ==
Disclaimer: All claims expressed in this article are solely those of the authors and do not necessarily represent those of their affiliated organizations, or those of the publisher, the editors and the reviewers. Any product that may be evaluated in this article or claim that may be made by its manufacturer is not guaranteed or endorsed by the publisher.
Research integrity at Frontiers
Learn more about the work of our research integrity team to safeguard the quality of each article we publish.