- 1Division of Convergence Interdisciplinary Education of Maritime and Ocean Contents, Korea Maritime & Ocean University, Busan, Republic of Korea
- 2Department of Aquaculture, Bangabandhu Sheikh Mujibur Rahman Agricultural University, Gazipur, Bangladesh
- 3Division of Convergence on Marine Science, Korea Maritime & Ocean University, Busan, Republic of Korea
- 4Department of Marine Production Management, Chonnam National University, Yeosu, Republic of Korea
Inclusion effect of different amount of jack mackerel meal (JMM) in the diets substituting 50% fish meal (FM) with corn gluten meal (CGM) on growth, feed availability, biochemical composition, plasma, and serum chemistry of olive flounder (Paralichthys olivaceus) was elucidated. Seven experimental diets were formulated. The control (Con) diet contained 60% FM. Fifty percent of FM in the Con diet was substituted with CGM, and then 5, 10, 20, 30, 40, and 50% JMM were added at the expense of FM to formulate the CJ5, CJ10, CJ20, CJ30, CJ40, and CJ50 diets, respectively. All formulated feeds were assigned to triplicate groups of fish. A total of 420 juvenile fish averaging 18 ± 0.01 g (mean ± SEM) was uniformly divided into 21, 50-L tanks, with 20 fish per tank. Fish were carefully hand-fed to satiation for 8 weeks. The weight gain of olive flounder fed the Con diet was significantly (p < 0.001) higher than that of fish fed the CJ5, CJ10, and CJ20 diets, but not significantly (p > 0.05) different from that of fish fed the CJ30, CJ40, and CJ50 diets. Olive flounder fed the Con diet achieved significantly (p < 0.001 and p < 0.04) greater specific growth rates (SGR) and feed consumption than those of fish fed the CJ5 and CJ10 diets, but not significantly (p > 0.05) different from those of fish fed the CJ20, CJ30, CJ40, and CJ50 diets. However, there were no significant (p > 0.05) differences in feed utilization, proximate composition, amino acid profiles, and plasma and serum parameters of olive flounder. In conclusion, incorporation of 30, and 20% JMM are the most desirable treatments in the olive flounder diets replacing 50% FM with CGM based on weight gain, and SGR and feed consumption, respectively. This study will be very helpful to develop low-FM diets for sustainable olive flounder culture.
1 Introduction
Olive flounder (P. olivaceus) is a carnivorous demersal flatfish species (Hamidoghli et al., 2020). The Eastern Asian nations including Korea, China, and Japan are the major commercial producers of olive flounder because of its short grow-out time, good flavor, low susceptibility to disease, and high market value and demand (Kim et al., 2019; Stieglitz et al., 2021). Olive flounder has become the top species produced from aquaculture in Korea, with a production of 45,801 metric tons (MT) (50.6% of total marine aquaculture fin fish production) of fish in 2022 (KOSIS, 2023). Being a carnivorous fish species, in particular, olive flounder requires a high-protein (up to 60%) diet where FM is utilized as the primary protein source (Hamidoghli et al., 2020). However, the production of FM has reduced by 26.5%, and the price of FM has increased by 3.5 times in the last two decades due to increasing production costs and decreasing global marine production (Jannathulla et al., 2019). The increasing cost and unstable supply of FM will lower the inclusion level of FM in aquaculture diets in the long term (Tacon and Metian, 2008). Therefore, searching for a replacer for FM that is both inexpensive and supply-stable is a high priority for sustainable aquaculture production of fish.
Plant protein sources as a replacer for FM have been emphasized by fish nutritionists. Some plant protein sources including microalgae (Rahimnejad et al., 2017), macroalgae (Zeynali et al., 2020), rice distillers dried grain (Bae et al., 2015), and soybean meal and cotton seed meal (Pham et al., 2007; Lim and Lee, 2008) have been examined as the replacers to FM in the olive flounder diets because of their sufficient protein content and low market price. However, higher fiber content, amino acid (AA) imbalance, and the presence of anti-nutritional factors (ANF) limit the extensive use of plant proteins, which may lower the palatability and growth of fish (Jannathulla et al., 2019; Li et al., 2022). Corn gluten meal (CGM) have a high potential to be used as a replacer to FM in fish feeds due to its low fiber level, high protein content (60–70% of dry matter), and almost devoid of ANF (Peter et al., 2000; Oliva-Teles et al., 2015). CGM could replace FM up to 40.4% in turbot (Psetta maxima) (Regost et al., 1999) and 60% in both spotted rose snapper (Lutjanus guttatus) (Hernández et al., 2021) and gilthead sea bream (Sparus aurata) (Pereira and Oliva-Teles, 2003) feeds, respectively. In particular, Kikuchi (1999a) showed that CGM could replace up to 40% FM in olive flounder diets without having negative impacts on growth and feed utilization when juvenile olive flounder were fed with a 75% FM-based feed or one of the feeds replacing 20, 40, and 60% FM with CGM supplemented with limiting amino acid (AA) (arginine, lysine, and tryptophan) for 8 weeks. In his study, however, a feed substituting 40% FM with CGM without supplementation of limiting AA produced inferior growth and feed utilization compared to fish fed a 75% FM-basal feed or a feed substituting 40% FM with CGM supplemented with limiting AA.
Furthermore, CGM has been tested in combination with other alternatives, such as animal by-products (Ye et al., 2011), soybean meal (Sevgili et al., 2015), soy protein isolates (Gamboa-Delgado et al., 2013), and pea protein isolates (Wang et al., 2020) for FM in different aquaculture species. Nevertheless, the imbalanced AA profiles (deficiency in lysine and arginine) and the presence of non-soluble carbohydrates limit the application of CGM in fish diets because of its poor feed consumption (palatability) and feed utilization (Peter et al., 2000; Hernández et al., 2021). Therefore, inclusion of protein rich feed ingredient having feed attractants and stimulants effect into low-FM diets is one of the best methods to resolve those undesirable problems.
Feed attractants and/or stimulants are compounds that stimulate the feeding response and feed consumption of fish (Kubitza and Lovshin, 1999). A formulated diet must contain modest quantities of chemostimulants to be chemically appealing. Along with the proper nutritional balance, palatability is also considered an essential factor in the development of artificial diets (Takakuwa et al., 2019). The addition of attractants and/or stimulants to the diet allows fish to quickly access the feed, improve the palatability, and acceptability of the diet, in other words, minimize the leaching-out ratio of nutrients and deterioration of water quality (Kim et al., 2019; Hancz, 2020; Jeong et al., 2022). The effectiveness of the synthetic chemicals stimulants in practical feeding is still controversial (Morais, 2017). However, natural feed stimulants were found to increase feed consumption with a significant improvement in growth of fish (Kader et al., 2010; Nagel et al., 2014; Khosravi et al., 2018).
Jack mackerel (Trachurus japonicus) meal (JMM) was found to be an effective protein source having feed attractants and stimulants effect to olive flounder (Jeong et al., 2020, 2022; Jeong and Cho, 2023), rockfish (Sebastes schlegeli) (Kim and Cho, 2019; Baek et al., 2021), and yellowtail (Seriola quinqueradiata) (Hosokawa et al., 2001). Especially, Kim et al. (2019) and Jeong et al. (2020) emphasized that both rockfish and olive flounder exhibited the maximum attraction behavior (movement) to JMM among 15–16 various feed ingredients. Furthermore, Ikeda et al. (2012) proved that AA in the muscle extracts of jack mackerel, especially histidine showed outstanding feeding stimulant activity for olive flounder. The muscle extracts of jack mackerel are rich in AA and nucleotides, particularly inosine monophosphate (IMP) exhibiting a strong feed stimulatory effect on greater amberjack (Seriola dumerili) (Takakuwa et al., 2019).
Therefore, we evaluated the inclusion effect of various levels of JMM in low-FM diets substituting 50% FM by CGM on growth, feed availability, biochemical composition, and plasma and serum parameters of olive flounder in the current study.
2 Materials and methods
2.1 Experimental diet preparation
Seven experimental diets were formulated (Table 1). The main protein sources in the control (Con) diet were 60% FM and 15% fermented soybean meal. In the Con diet, 18.3% wheat flour, and 2.1% each of fish oil and soybean oil were included as the carbohydrate and lipid sources, respectively. Fifty percent of FM in the Con diet were replaced by CGM, and then various levels (5, 10, 20, 30, 40, and 50%) of JMM at the expense of FM were included to formulate CJ5, CJ10, CJ20, CJ30, CJ40, and CJ50 diets, respectively. However, a diet replacing 50% FM with CGM without JMM inclusion was not included in this experiment because the substitutability of FM with CGM was estimated to be 40% in the olive flounder feeds without AA supplementation (Kikuchi, 1999a). After properly combining the ingredients, a 3:1 ratio of water was added to the mixture to create a dough. Depending on the size of the fish’s mouth, the dough was then pelletized by running it through a laboratory-style pellet extruder (4–6 mm in diameter) (Dongsung Mechanics, Korea). Finally, after being dried at 25°C in a forced air oven (SI-2400, Sin II Drying Machine Co. Ltd., Korea) for 48 h, all sinking type pellets were kept at −20°C until further use.
2.2 Experimental condition and feeding trial
Healthy juvenile olive flounder were purchased from a commercial fish farm (Taean-gun, Chungcheongnam-do, Korea) and transported to the laboratory. Prior to initiation of the feeding experiment, fish were acclimatized to the rearing environments for 2 weeks by feeding with a commercial extruded pellet (55% crude protein and 8% crude lipid) twice a day at a ratio of 2–3% biomass of fish. A number of 420 juvenile fish averaging 18.0 ± 0.01 g (mean ± SEM) was uniformly assigned into twenty-one 50-L rectangular flow-through tanks (20 fish/tank). Each tank received sand-filtered seawater with a flow rate of 4.2 L/min. A multifunctional water quality meter (AZ-8603, AZ Instrument, Taichung, Taiwan) was used daily to monitor the water quality. Temperature, salinity, dissolved oxygen, and pH ranged 17.7–24.0°C (21.3°C ± 2.02°C; mean ± SD), 31.6–33.6 g/L (32.6 ± 0.47 g/L), 7.0–7.9 mg/L (7.3 ± 0.13 mg/L), and 7.1–7.5 (7.3 ± 0.12), respectively.
All experimental diets were assigned to triplicate groups of fish. Throughout the 8-week feeding experiment, fish were carefully hand-fed to apparent satiation twice a day (08:00 and 17:00). However, unfed feeds were not collected. Daily siphoning was used to clean the fish tanks’ bottom, and the photoperiod followed the natural cycle (14 L: 10 D, h of light: dark cycle).
2.3 Determination of the biological indices of fish
At the termination of the 8-week experiment, all live fish were anesthetized with MS-222 at a concentration of 100 ppm after the 24-h starvation. The number of live fish from each tank was counted for calculating the survival and collectively weighed to evaluate weight gain. Ten anesthetized fish randomly taken from each tank were dissected to collect liver and viscera. Growth, feed utilization, and biological indices of fish were calculated by using the following formula: specific growth rate (SGR, %/day) = (Ln final weight of fish – Ln initial weight of fish) × 100/days of feeding (56 days), feed efficiency (FE) = weight gain of fish/feed consumption, protein efficiency ratio (PER) = weight gain of fish/protein consumption, protein retention (PR, %) = protein gain of fish × 100/protein consumption, condition factor (K, g/cm3) = body weight of fish (g) × 100/total length of fish (cm)3, viscerosomatic index (VSI, %) = viscera weight of fish × 100/body weight of fish, and hepatosomatic index (HSI, %) = liver weight of fish × 100/body weight of fish.
2.4 Analysis of the biochemical composition of the experimental diets and fish
Ten fish after measuring biological indices of fish and 4 remaining fish from each tank were homogenized and utilized for proximate composition analysis after the 8-week feeding study. According to Association of Official Analytical Chemists (1990) standard method, the proximate composition for the experimental diets and fish were determined. Crude protein content was determined by Kjeldahl apparatus (Kjeltec 2,100 Distillation Unit, Foss Tecator, Hoganas, Sweden), and crude lipid content was determined by ether-extraction method (Soxtec TM 2043 Fat Extraction System, Foss Tecator, Hoganas, Sweden). Moisture content was determined by oven drying at 105°C for 24 h, and ash content was determined by using a muffle furnace at 550°C for 4 h. In order to analyze AA (apart from methionine and cysteine, and tryptophan), the experimental diets and the whole-body of olive flounder were hydrolyzed with 6 N HCl for 24 h at 110°C, followed by ion exchange chromatography with an AA analyzer (L-8800 Auto-analyzer: Hitachi, Tokyo, Japan). To measure methionine and cysteine content, the samples were oxidized with performic acid at below 5°C for 24 h to obtain methionine sulfone and cysteic acid, and they were then freeze-dried twice with deionized water. Then the freeze-dried samples were hydro-lysed and analyzed following similar process used for the other amino acids. Tryptophan analysis was conducted using high-performance liquid chromatography (S1125 HPLC pump system; Sykam GmbH, Eresing, Germany). Lipids for FA analyses in the experimental diets and the whole-body fish were extracted by a mixture of chloroform and methanol (2:1 v/v) according to the method of Folch et al. (1957). FA methyl esters were prepared by transesterification with 14% BF3-MeOH (Sigma, St. Louis, MO, United States) and analyzed by using gas chromatography (Trace GC, Thermo, Waltham, MA, United States).
2.5 Plasma parameters of fish
Three anesthetized fish from each tank had blood drawn from their caudal veins using heparinized syringes. Plasma was extracted and kept for subsequent analysis in separate aliquots in a freezer at −70°C after centrifugation (2,716 × g at 4°C) for 10 min. An automated chemistry system (Fuji Dri-Chem NX500i, Fujifilm, Tokyo, Japan) was used to analyze the following enzymes: aminotransferase (AST; 15,809,542, measurement range: 10–1,000 U/L), alanine aminotransferase (ALT; 16,654,035, measurement range: 10–1,000 U/L), alkaline phosphatase (ALP; 16,653,964, measurement range: 14–1,183 U/L), total bilirubin (T-BIL; 16,654,061, measurement range: 0.2–30.0 mg/dL), total cholesterol (T-CHO; 16,654,073, measurement range: 50–450 mg/dL), triglyceride (TG; 16,654,085, measurement range: 10–500 mg/dL), total protein (TP; 16,654,097, measurement range: 2.0–11.0 g/dL), and albumin (ALB; 16,653,952, measurement range: 1.0–6.0 g/dL).
2.6 Serum lysozyme activity and superoxide dismutase (SOD) of olive flounder
Three anesthetized fish from each tank had blood drawn from their caudal veins using syringes. Serum was extracted and kept for subsequent analysis in separate aliquots in a freezer at −70°C after centrifugation (2,716 × g at 4°C) for 10 min. Lysozyme activity was determined by the turbidimetric assay according to Lange et al. (2001). In brief, 100 μL test serum was added to a suspension (1.9 mL) of Micrococcus lysodeikticus (0.2 mg/mL; Sigma, St. Louis, MO, United States) in a 0.05 M sodium phosphate buffer, pH 6.2. The reaction was carried out at 25°C, and absorbance was measured at 530 nm on a spectrophotometer after 0 and 60 min. The quantity of enzyme needed to cause a 0.001/min reduction in absorbance was referred to as a lysozyme activity unit. SOD was determined by using a 19,160 SOD Determination Kit (Sigma-Aldrich Inc., St. Louis, MO, United States) following the manufacturer’s direction. The same methods and procedures for measurements of lysozyme activity and SOD of fish were adopted in Jeong and Cho (2023)’s study.
2.7 Statistical analysis
Prior to statistical analysis, all data were tested for normality and homogeneity. Significant differences in means were analyzed by one-way ANOVA and Tukey’s post hoc test with SPSS version 24.0 (SPSS Inc., Chicago, IL, United States). Percentage data were arcsine-transformed prior to statistical analysis. Furthermore, regression analysis was applied between weight gain, SGR, and feed consumption of fish and inclusion levels of JMM in diets substituting 50% FM by CGM.
3 Results
3.1 AA and FA profiles of the experimental feeds
The JMM is relatively high in essential AA (EAA) over FM; however, CGM is relatively rich in leucine and phenylalanine over FM (Table 2). Arginine, leucine, and lysine, as well as aspartic acid and glutamic acid are the abundant EAA and non-essential AA (NEAA), respectively, in all formulated diets. All EAA, except for phenylalanine tended to increase with dietary inclusion levels of JMM. The arginine and lysine content in all the experimental diets fulfilled the requirements of olive flounder, but were comparatively low for the dietary methionine requirement.
The FM is rich in total content of saturated FA (∑SFA), eicosapentaenoic acid (EPA, C20:5n-3), and total content of n-3 highly unsaturated FA (∑n-3 HUFA), while JMM is rich in total content of monounsaturated FA (∑MUFA), and docosahexaenoic acid (DHA, C22:6n-3) content (Table 3). The ∑MUFA and DHA content tended to increase with the inclusion of JMM in diets replacing 50% FM by CGM, but tended to decrease for the ∑SFA, except for the CJ50 diet, EPA, and ∑n-3 HUFA content.
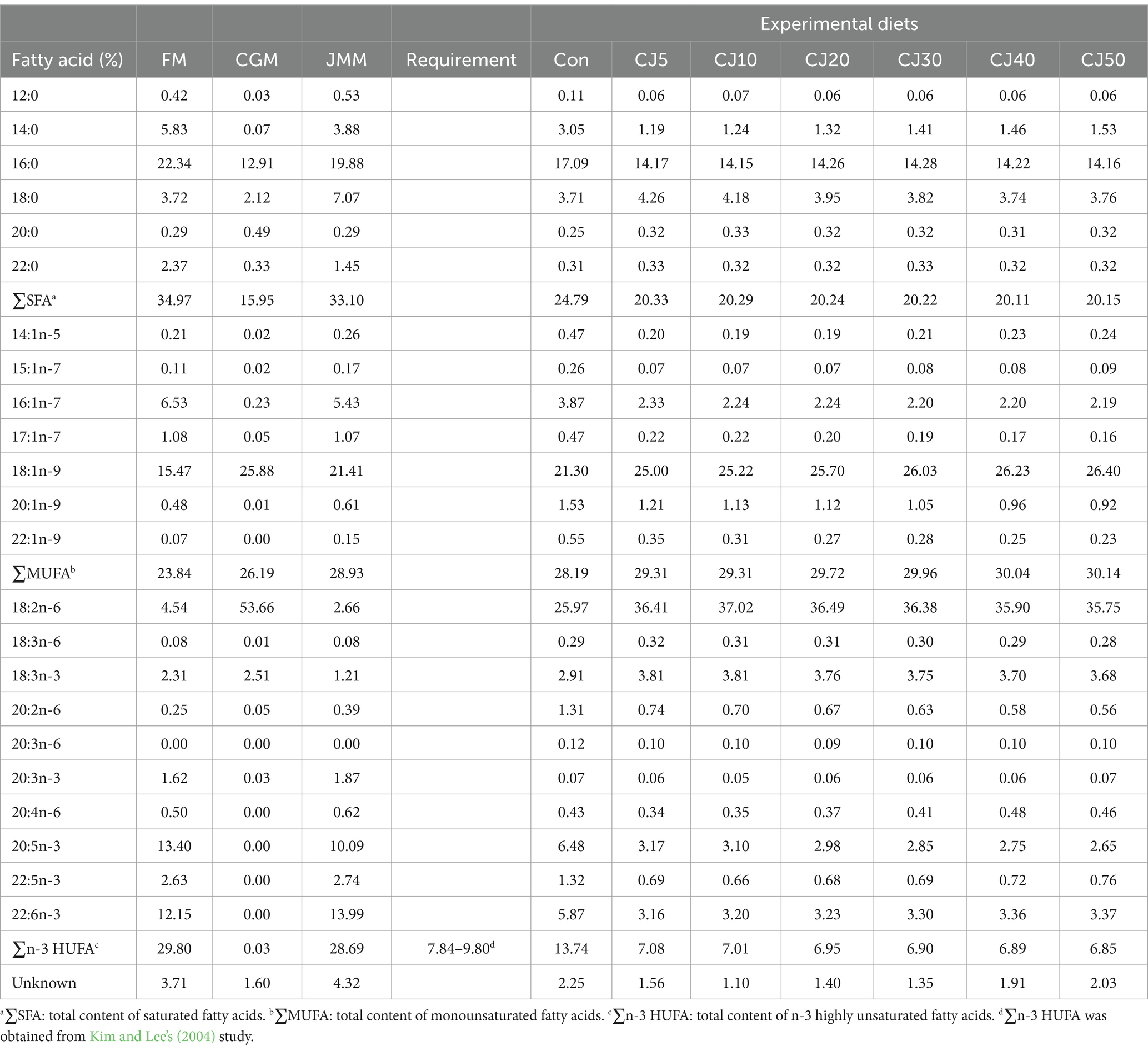
Table 3. Fatty acid (FA) profiles (% of total FA) of the main feed ingredients and experimental diets.
3.2 Growth performance, feed availability, and biological indices of fish
All olive flounder survived at the completion of the experiment (Table 4). Weight gain of olive flounder fed the Con (69.0 ± 0.16 g) diet was significantly (p < 0.001) higher than that of the fish fed the CJ5 (64.7 ± 0.14 g), CJ10 (64.9 ± 1.14 g), and CJ20 (66.5 ± 0.54 g) diets, but not significantly (p > 0.05) different from that of the fish fed the CJ30 (66.9 ± 0.20 g), CJ40 (67.3 ± 0.16 g), and CJ50 (67.4 ± 0.10 g) diets (Figure 1). The SGR of olive flounder fed the Con (2.81 ± 0.004%/day), CJ40 (2.78 ± 0.004%/day), and CJ50 (2.78 ± 0.003%/day) diets were significantly (p < 0.001) higher than that of fish fed the CJ5 (2.72 ± 0.003%/day) and CJ10 (2.72 ± 0.024%/day) diets, but not significantly (p > 0.05) different from that of olive flounder fed the CJ20 (2.76 ± 0.012%/day) and CJ30 (2.77 ± 0.004%/day) diets (Figure 2). In regression analysis, the linear models were found to be the best fit model between inclusion levels of JMM in the olive flounder diets replacing 50% FM with CGM and weight gain (linear; p = 0.0001, adjusted R2 = 0.577), and SGR (linear; p = 0.0001, adjusted R2 = 0.583), respectively (Figures 1 and 2, respectively).
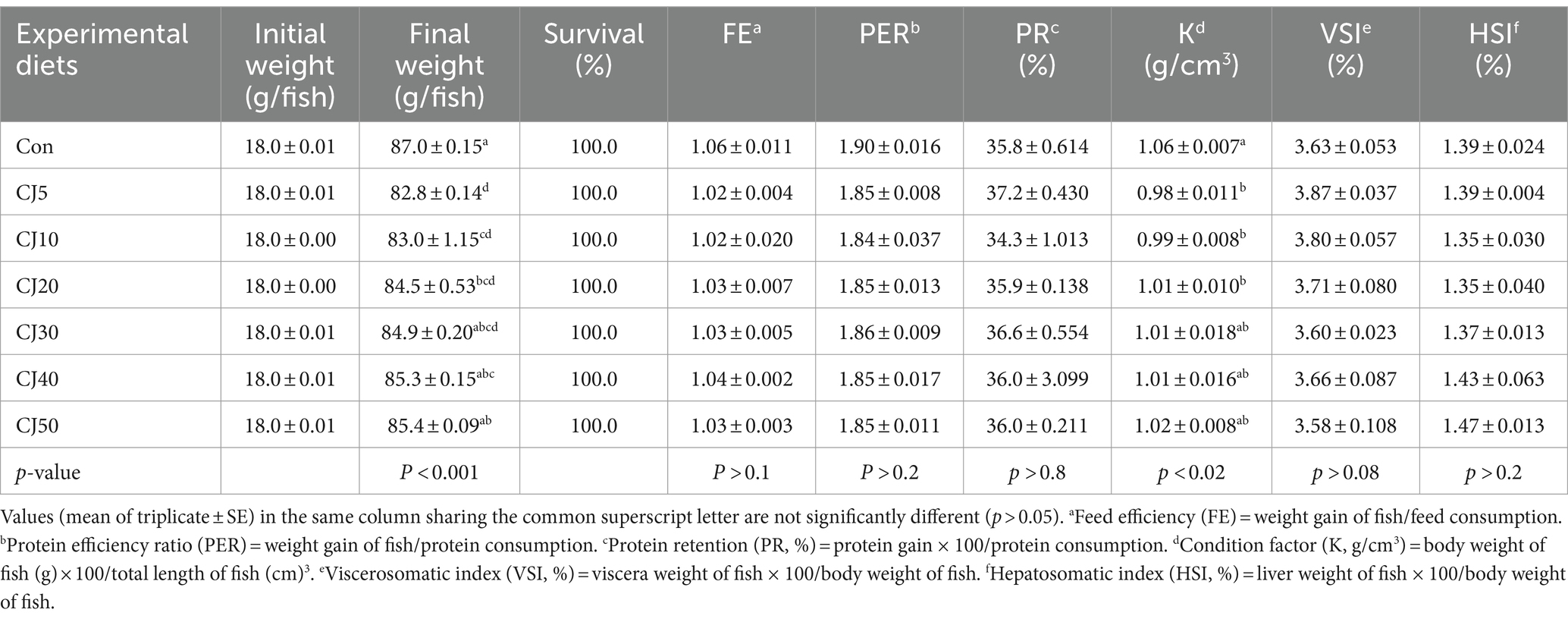
Table 4. Survival (%), feed efficiency (FE), protein efficiency ratio (PER), protein retention (PR), condition factor (K), viscerosomatic index (VSI), and hepatosomatic index (HSI) of olive flounder fed the experimental diets for 56 days.
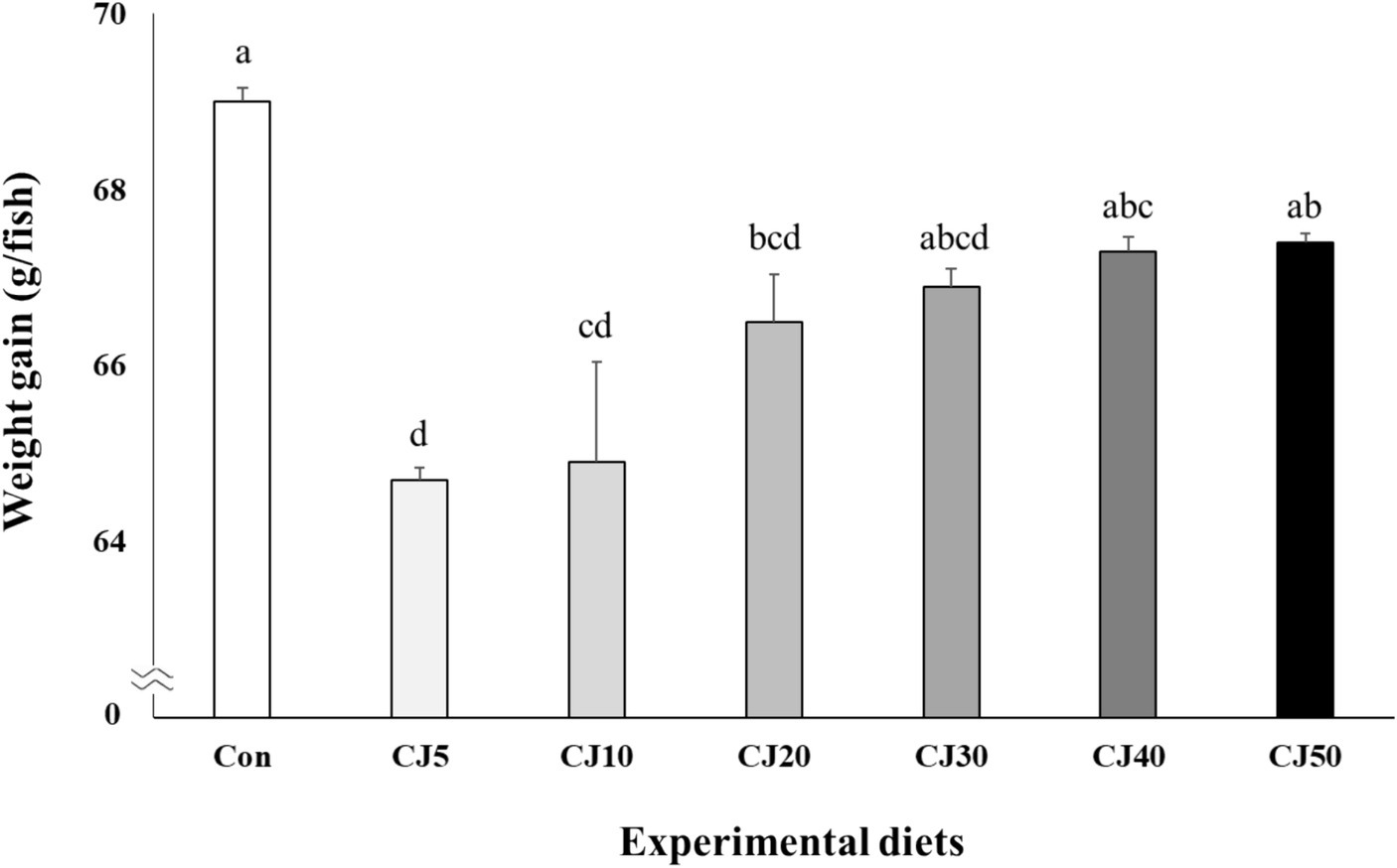
Figure 1. Weight gain (g/fish) of olive flounder (Paralichthys olivaceus) fed the experimental diets for 56 days (mean of triplicate ± SE) (p < 0.001). Regression analysis; Y = 0.596143X + 64.2077, p = 0.0001, adjusted R2 = 0.5773. Different letters indicate statistically significant differences (p < 0.05).
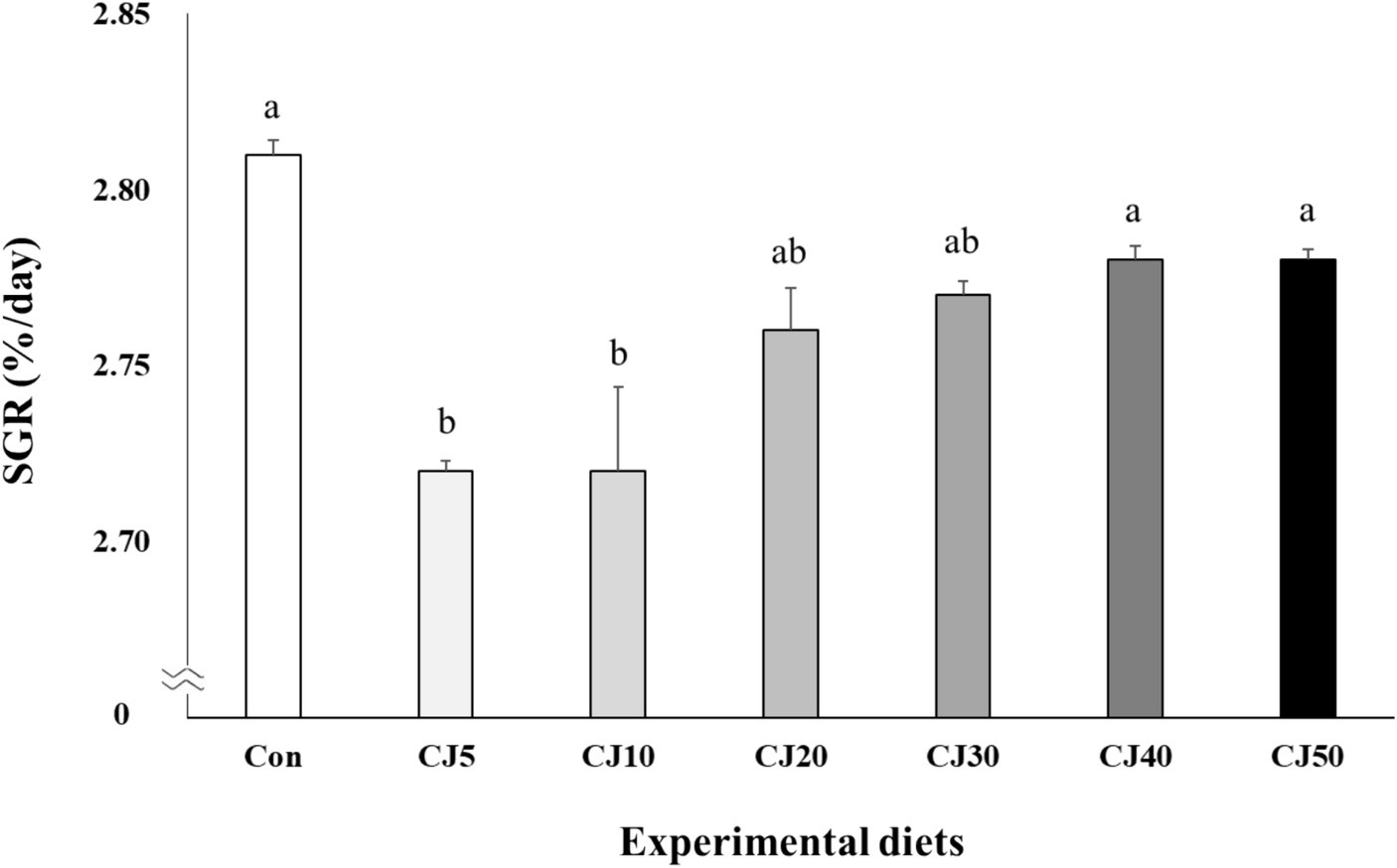
Figure 2. Specific growth rate (SGR, %/day) of olive flounder (Paralichthys olivaceus) fed the experimental diets for 56 days (mean of triplicate ± SE) (p < 0.001). Regression analysis; Y = 0.012979X+ 2.7094, p = 0.0001, adjusted R2 = 0.5830. Different letters indicate statistically significant differences (p < 0.05).
Feed consumption of olive flounder fed the Con (65.4 ± 0.44 g) and CJ50 (65.1 ± 0.46 g) diets was significantly (p < 0.04) higher than that of fish fed the CJ5 (63.5 ± 0.22 g) and CJ10 (63.8 ± 0.20 g) diets, but not significantly (p > 0.05) different from that of olive flounder fed the CJ20 (64.6 ± 0.18 g), CJ30 (64.8 ± 0.11 g), and CJ40 (65.0 ± 0.72 g) diets (Figure 3). In regression analysis, the linear model was found to be the best fit model between inclusion levels of JMM in olive the flounder diet replacing 50% FM with CGM and feed consumption (linear; p = 0.001, adjusted R2 = 0.482). The FE, PER, and PR of fish ranged from 1.02–1.06, 1.84–1.90, and 34.3–37.2%, respectively. These values were not significantly (p > 0.1, p > 0.2, and p > 0.8, respectively) affected by dietary treatments.
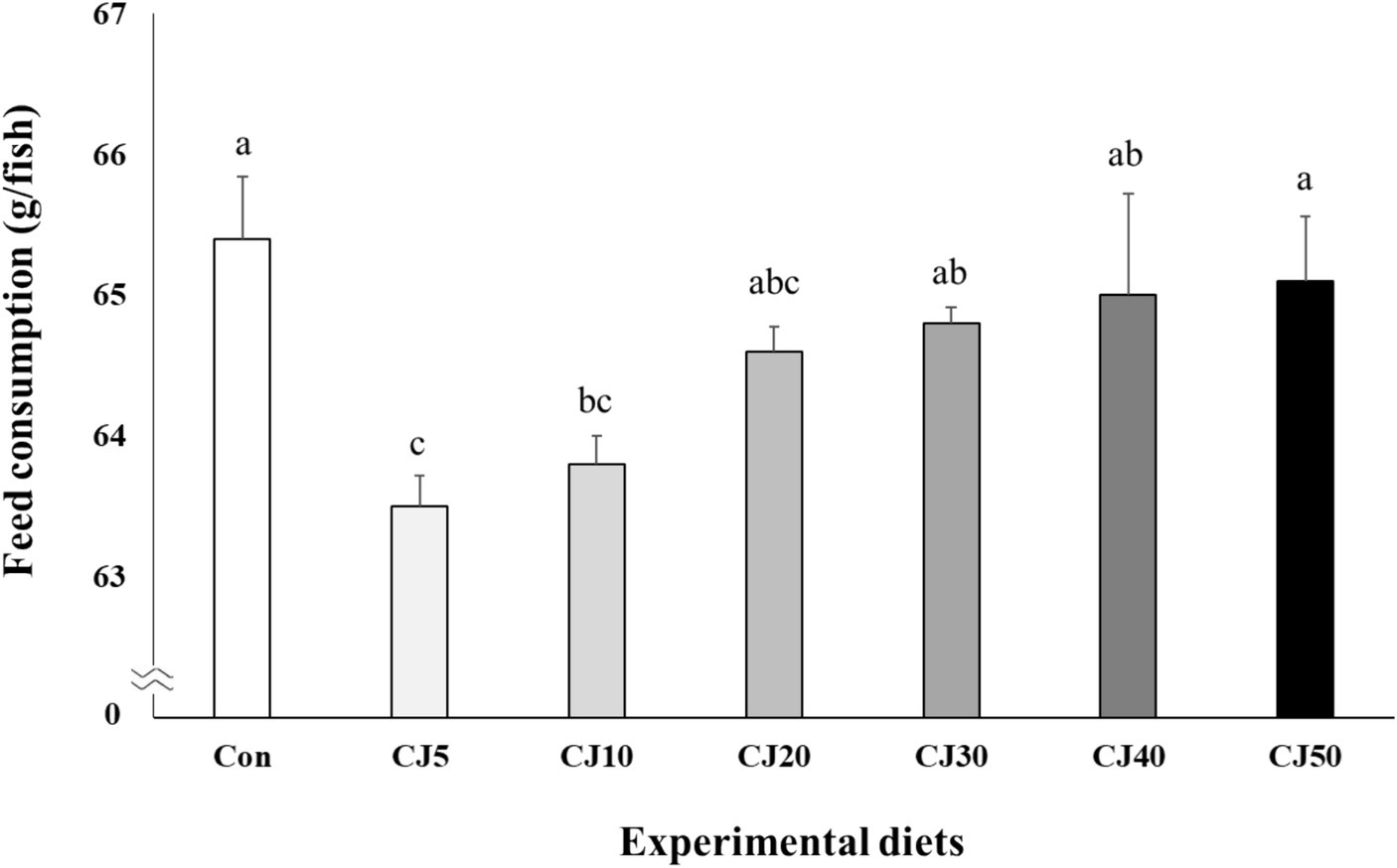
Figure 3. Feed consumption (g/fish) of olive flounder (Paralichthys olivaceus) fed the experimental diets for 56 days (mean of triplicate ± SE) (p < 0.04). Regression analysis; Y = 0.338438X + 63.3041, p = 0.001, adjusted R2 = 0.4819. Different letters indicate statistically significant differences (p < 0.05).
The K of olive flounder fed the Con diet was significantly (p > 0.02) higher than that of the olive flounder fed the CJ5, CJ10, and CJ20 diets, but not significantly (p > 0.05) different from that of the olive flounder fed the CJ30, CJ40, and CJ50 diets. However, VSI and HSI of olive flounder ranged 3.58–3.87% and 1.35–1.47%, respectively, and they were not significantly (p > 0.05) altered by dietary treatments.
3.3 Whole-body proximate composition of olive flounder
The moisture, crude protein, crude lipid, and ash content of the whole-body fish ranged 67.6–72.3%, 18.6–19.7%, 3.1–3.7%, and 3.5–4.3%, respectively (Table 5). Dietary interventions had no significant (p > 0.05) impact on these values.
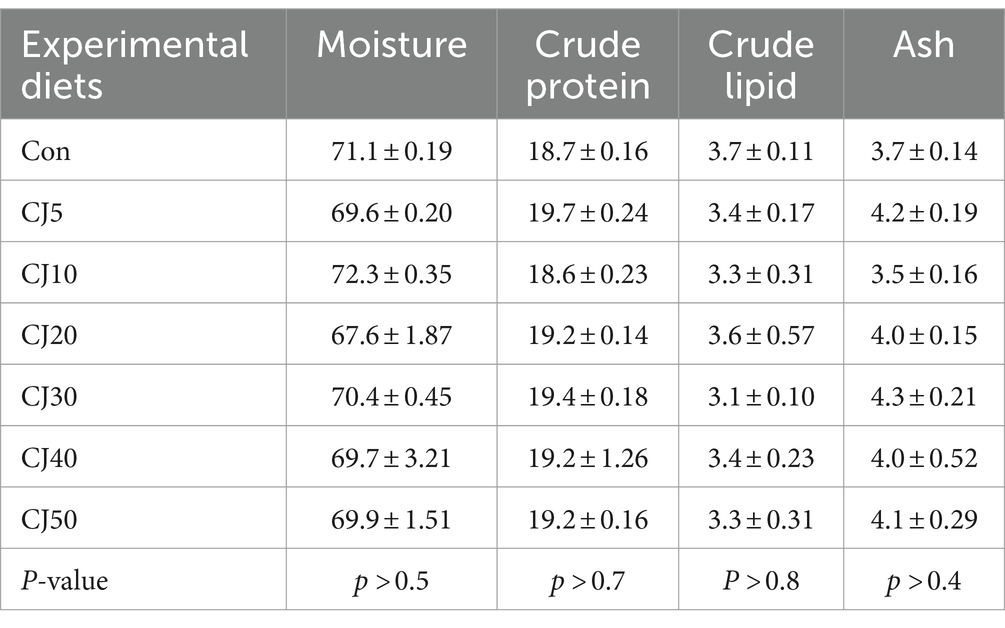
Table 5. Proximate composition (%, wet weight) of the whole-body olive flounder at the end of the 56-day feeding trial (mean of triplicate ± SE).
3.4 Plasma parameters of olive flounder
AST, ALT, ALP, TB, T-CHO, TG, TP, and ALB level of fish ranged 12.9–13.2 U/L, 6.3–6.4 U/L, 110.6–112.8 U/L, 0.7–0.8 mg/dL, 357.7–370.8 mg/dL, 440.3–452.9 mg/dL, 5.3–5.7 g/dL, and 1.0–1.1 g/dL, respectively (Table 6). The experimental diets did not significantly (p > 0.05) alter any hematological parameter of olive flounder.
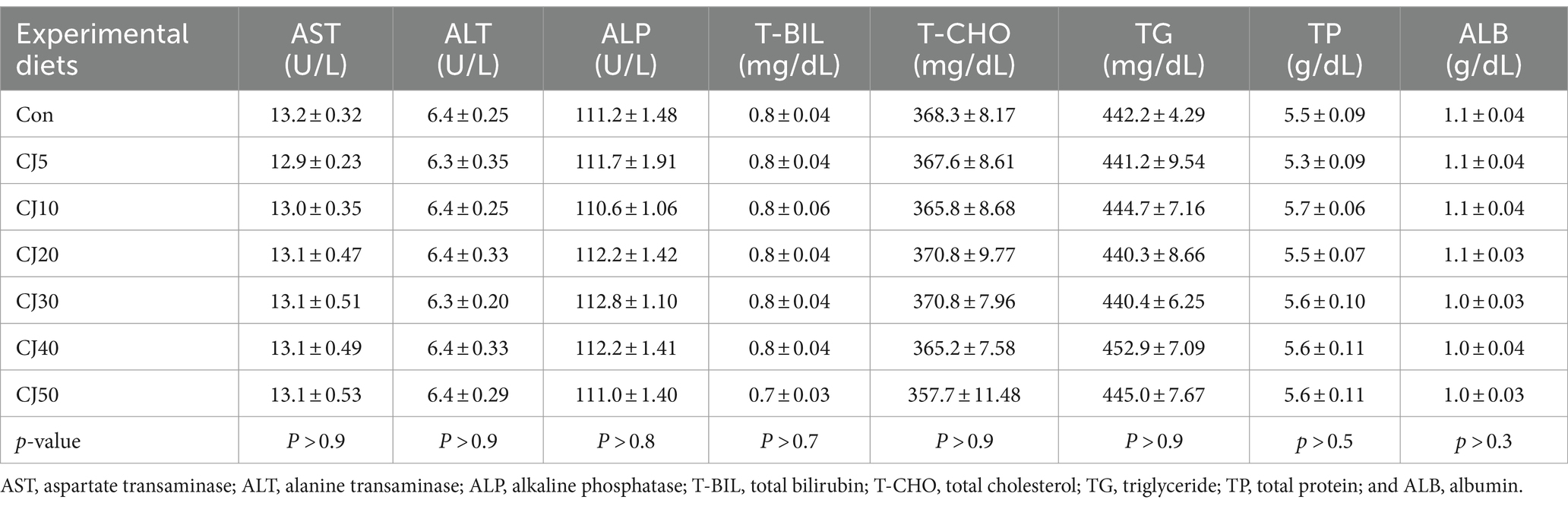
Table 6. Plasma parameters of olive flounder at the end of the 56-day feeding trial (means of triplicate ± SE).
3.5 Serum lysozyme activity and SOD of olive flounder
Serum lysozyme activity of olive flounder ranged 538.4–583.3 U/mL and SOD ranged 71.5–73.5% (Table 7). The experimental diets did not significantly (p > 0.9 and p > 0.8, respectively) affect serum lysozyme activity and SOD of olive flounder.
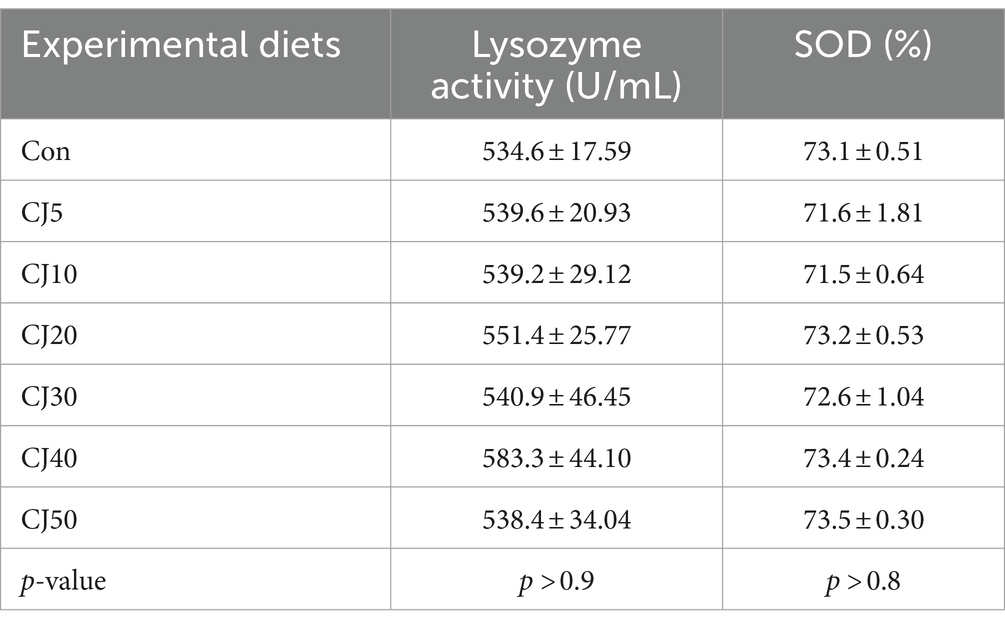
Table 7. Serum lysozyme activity and superoxide dismutase (SOD) of olive flounder at the end of the 56-day feeding trial (mean of triplicate ± SE).
3.6 AA and FA profiles of the whole-body olive flounder
AA profiles of the whole-body of olive flounder were not significantly (p > 0.05) altered by dietary treatments (Table 8).
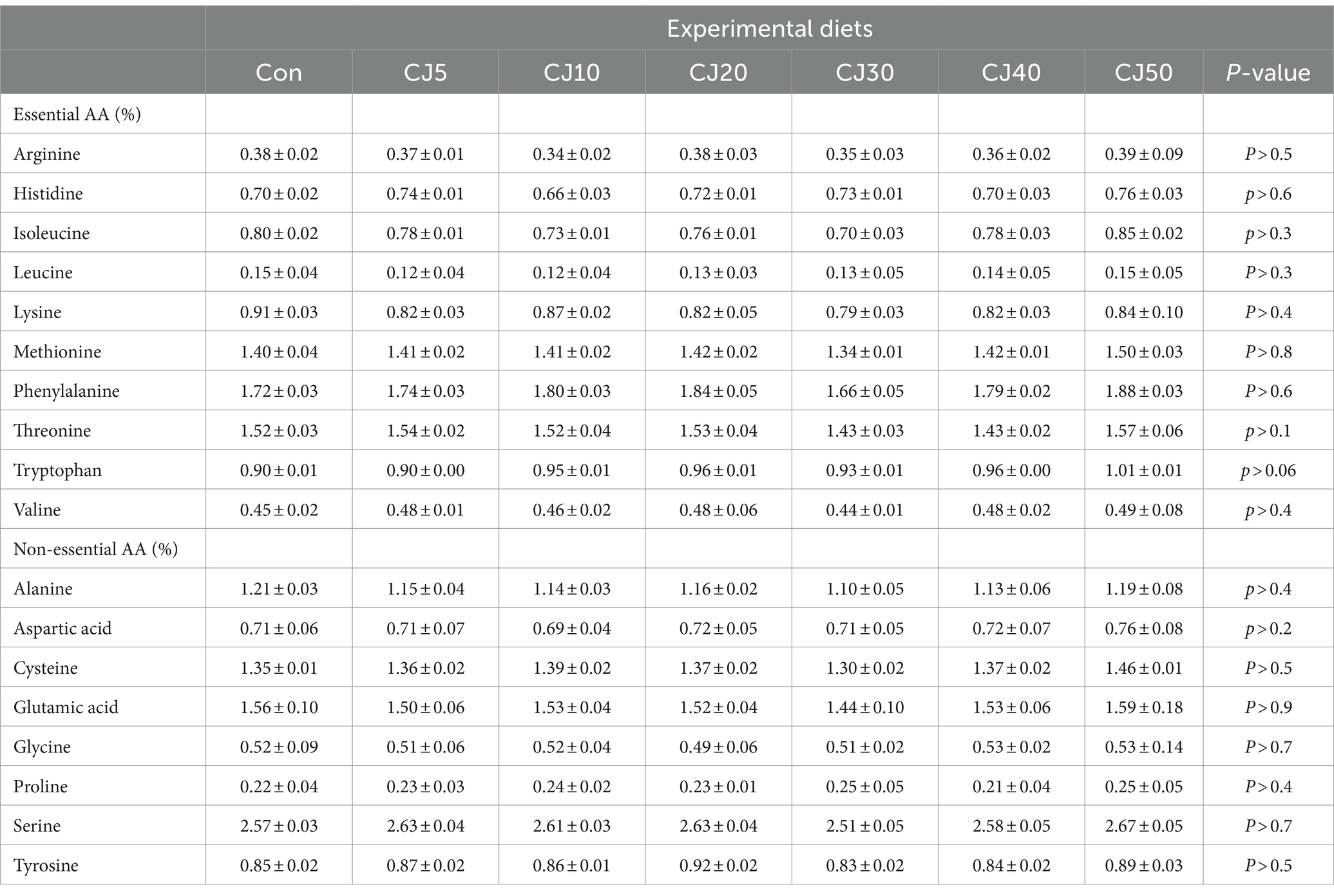
Table 8. Amino acid (AA) profiles (%, wet weight) of the whole-body of olive flounder at the end of the 56-day feeding trial (means of triplicate ± SE).
The ∑SFA of the whole-body olive flounder fed the Con diet was significantly (p < 0.0001) higher than that of the fish fed all other diets (Table 9). EPA and DHA content of the whole-body of olive flounder fed the Con diet were significantly (p < 0.0001 for both) higher than those of fish fed all other diets. In particular, EPA content of olive flounder tended to decrease with inclusion levels of JMM in the CJ diets. The ∑n-3 HUFA content of the whole-body of olive flounder fed the Con diet was significantly (p < 0.0001) higher than that of olive flounder fed all other feeds.
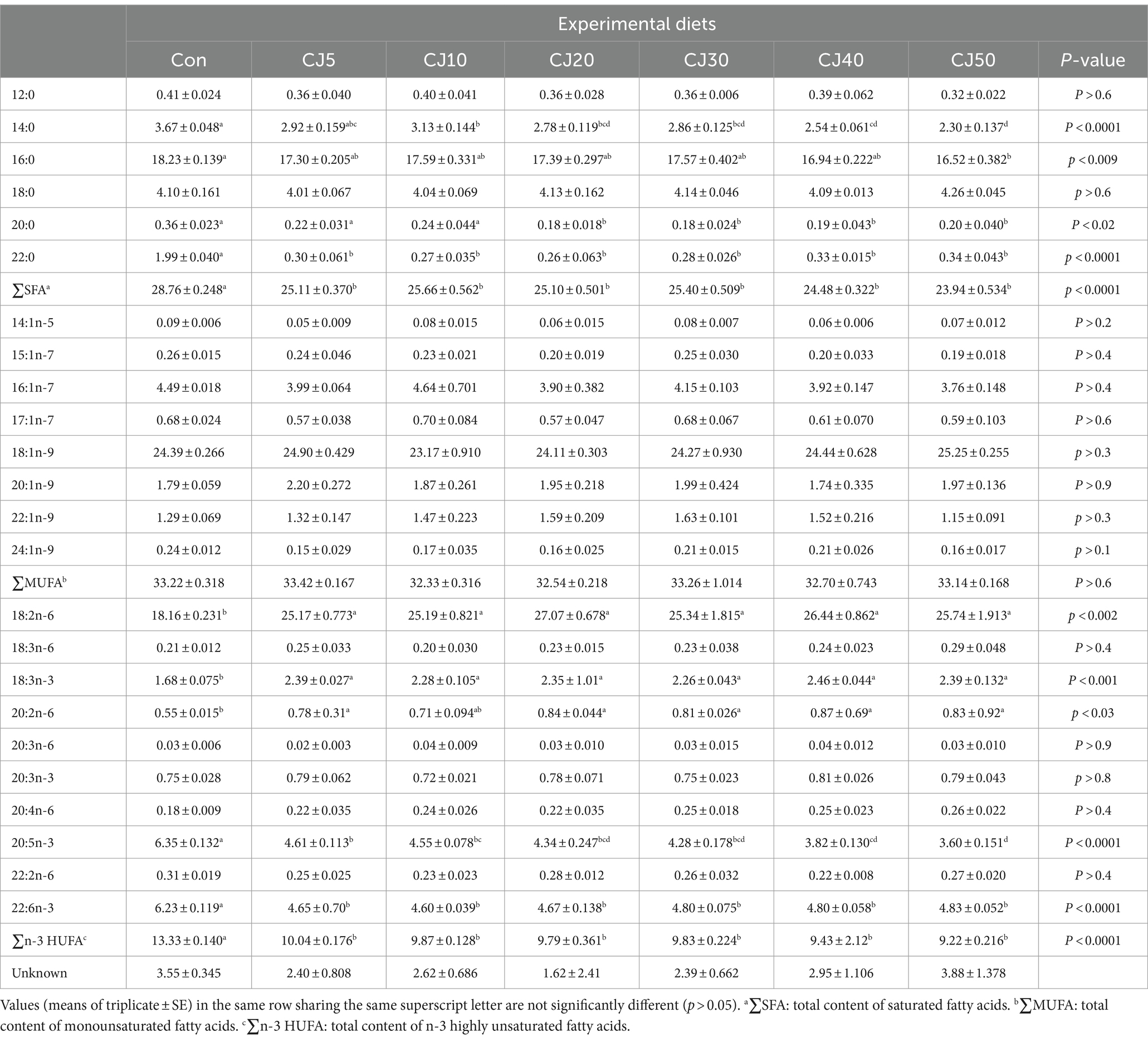
Table 9. Fatty acid (FA) profiles (% of total FA) of the whole body of olive flounder at the end of the 56-day feeding trial.
4 Discussion
Comparable weight gain and SGR of olive flounder fed the CJ30, CJ40, and CJ50 diets or CJ20, CJ30, CJ40, and CJ50 diets to fish fed the Con diet in this study implied that 50% FM could be replaceable with CGM without deteriorating growth and SGR of olive flounder as long as 30–50% and 20–50% JMM were included at the expense of FM in a 60% FM-based diet, respectively. Linear improvement in weight gain and SGR of olive flounder versus JMM inclusion levels in low-FM diets replacing 50% FM with CGM might indicate that inclusion of JMM in low-FM diets could bring about improved growth performance of fish. Comparable feed consumption of olive flounder fed the CJ20, CJ30, CJ40, and CJ50 diets to fish fed the Con diet in this study indicated that 50% FM could be replaceable with CGM without deteriorating feed consumption of olive flounder as long as 20–50% JMM was included in a 60% FM-based diet. Improved weight gain and SGR of olive flounder were directly reflected from improved feed consumption of fish fed the low-FM diets substituting 50% FM with CGM. However, inferior weight gain, SGR and feed consumption of olive flounder fed the CJ5 and CJ10 diets to fish fed the Con diet indicated that inclusion of 5–10% JMM in low-FM diets could not compensate for the 60% FM-based (Con) diet in this study. Since JMM is one of the most expensive FM sources, 30 and 20% JMM are the most advised strategy in low-FM diets substituting 50% FM with CGM in terms of weight gain, and SGR and feed consumption, respectively. Unlike this study, Kikuchi (1999a) emphasized that FM up to 40% could be replaced with CGM in the olive flounder diets supplemented with limiting AA without compromising growth and feed utilization, however, a diet substituting 40% FM with CGM without supplementation of limiting AA led to inferior growth and feed utilization compared to fish fed a 75% FM-basal diet or diet substituting 40% FM with CGM supplemented with limiting AA. In considering results of Kikuchi’s (1999a) study and this study, 50% FM could be replaceable by CGM without supplementation of limiting AA in low-FM diet as long as 20–50% JMM was included in olive flounder diet. Kim et al. (2019) and Jeong et al. (2020) also demonstrated that dietary inclusion of JMM led to improved growth performance and feed consumption of rockfish and olive flounder, respectively.
The CGM is a promising alternative to FM, but its utilization as a substitute for FM at an excess level deteriorated feed consumption and feed utilization, and eventually deteriorated the growth of turbot (Regost et al., 1999) and spotted rose snapper (Hernández et al., 2021). The imbalanced AA (deficiency in lysine and arginine) profiles of CGM in diets led to deteriorated growth of fish (Peter et al., 2000). Incorporated feed stimulants were found to be an effective method to enhance growth and feed consumption of fish fed the low-FM diet or non-FM diet, where FM was partially replaced with plant proteins (Papatryphon and Soares, 2000; Kader et al., 2012).
There were no significant differences in FE, PER, and PR of olive flounder fed all diets in this study. Similarly, dietary inclusion of graded levels of JMM as feed stimulants did not influenced feed utilization of olive flounder (Jeong et al., 2022; Jeong and Cho, 2023). Unlike this study, however, Kikuchi (1999b) revealed the greatest weight gain, feed consumption, FER, and PER in olive flounder fed a defatted soybean meal-basal diet as a replacer for FM with supplementation of blue mussel meat as feed enhancer. According to Hancz (2020) this disparity in the impact are possible due to the differences in type and doses of feed attractants and/or stimulants.
Fish require EAA to support their somatic and reproductive growth; hence, EAA requirements have to be taken into consideration when formulating low-FM diet (Hamidoghli et al., 2020). The arginine and lysine content of all experimental diets fulfilled the requirements (2.04–2.10% and 1.55–2.16%, respectively) of olive flounder (Forster and Ogata, 1998; Alam et al., 2002), but the methionine content of the experimental diets including the Con diet appeared to be comparatively lower than its requirement (1.44–1.49%) in the presence of 0.06% cysteine (Alam et al., 2000). The comparatively low methionine content in all experimental diets did not lower the growth of olive flounder because the relatively high cysteine content (0.85% in the CJ50 diet–1.02% in the Con diet) in all experimental diets could spare the methionine requirement (Finkelstein et al., 1988). Likewise, Harding et al. (1977) and Twibell et al. (2000) unveiled that cysteine could spare 50 and 60% of methionine in yellow perch (Perca flavescens) and channel catfish (Ictalurus punctatus) diets, respectively.
Marine fish including olive flounder need ∑n-3 HUFA for normal growth and development, which is mainly composed of EPA and DHA (Furuita et al., 2002). The EPA and DHA help to preserve the structural integrity of cell membranes, modulate carbohydrate and lipid metabolism, and regulate several inflammatory responses of fish (Lutfi et al., 2023). Farmed fish need to supply long-chain n-3 HUFA through diets because they have limited capacity to develop them in their bodies (Lee et al., 2020). The requirement (7.84–9.80% of total FA) of ∑n-3 HUFA for olive flounder (Kim and Lee, 2004) seemed to be met in the Con diet only in the current study. The ∑n-3 HUFA content in the experimental diets tended to decrease with increased JMM inclusion levels because FM contained a comparatively high ∑n-3 HUFA over JMM. Higher ∑SFA, EPA, DHA, and ∑n-3 HUFA content of whole-body of olive flounder fed the Con diet compared to those of fish fed all other diets were well responded from the FA profiles of the experimental diets. Likewise, the findings of this study are consistent with other studies, in which dietary FA profiles were well reflected in the whole-body FA profile of fish (Ha et al., 2021; Kim et al., 2021).
The biological parameters of fish including VSI and HSI in this study were not significantly impacted by dietary treatments. However, the K appeared to be well responded to weight gain of fish, which tended to increase with JMM inclusion levels in low-FM diets. The findings of this study were well proved by Baek et al. (2021)’s study, in which K of rockfish tended to increase with dietary inclusion levels of JMM. Likewise, Kader et al. (2010) explained that K of red sea bream (Pagrus major) tended to increase when crystalline AA, fish soluble, squid meal, and their combination were included as feed enhancer in low-FM diet.
Plasma parameters are useful indicators for the health status and stress level of fish (Satheeshkumar et al., 2012). In this study, no distinctive differences in plasma measurements of olive flounder were found among dietary treatments. Similarly, dietary inclusion of protein hydrolysates (shrimp, krill, and tilapia) showed no discernible differences in hematological measurements of olive flounder and red sea bream (Bui et al., 2014; Khosravi et al., 2015, 2018). Tusche et al. (2011) and Nagel et al. (2014) also reported that the inclusion of blood meal, mussel meal, and free AA mix as feed attractants in a plant protein concentrate-based diets did not affect the hematological parameters, except for the hematocrit level in rainbow trout (Oncorhynchus mykiss) and plasma protein level in turbot. No discernible difference in plasma chemistry of olive flounder in this study indicates that olive flounder fed the low-FM diets replacing 50% FM by CGM were in good health and nutritional condition.
The SOD protects cells from the toxic effects of superoxide radicals and lysozyme activity protects from pathogenic invasion (Sharf and Khan, 2020). However, lysozyme activity and SOD of olive flounder were not influenced by dietary treatments in this experiment. The findings of this study were supported by other studies, in which no remarkable changes in SOD and lysozyme activity were found in olive flounder fed diets substituting FM with fermented tuna by-product meal (Oncul et al., 2019) and meat meal (Ha et al., 2021). In contradiction to this study, inclusion of protein hydrolysate (shrimp, krill, and tilapia hydrolysate) tended to increase SOD and lysozyme activity of olive flounder fed a FM-basal feed (Khosravi et al., 2015) or low-FM feeds substituting 50% FM by soy protein concentrate (Khosravi et al., 2018). Bui et al. (2014) and Gunathilaka et al. (2021) reported that the protein hydrolysates prepared from fishery by-products contain low-molecular-weight peptides, which may induce the immunomodulatory activity in fish by triggering the activity of fish macrophages.
No distinctive differences in the proximate composition and AA profiles of the whole-body olive flounder were found in this study. Likewise, the inclusion of fishery protein hydrolysate and freeze-dried blue muscle meal as feed attractants in low-FM diets substituting various levels of FM with soy and rapeseed protein concentrate did not influence the proximate composition of olive flounder and turbot, respectively (Nagel et al., 2014; Khosravi et al., 2018). Similarly, no significant alterations in AA profiles were observed in the whole-body olive flounder fed diets replacing FM by various protein sources (Choi et al., 2020; Ha et al., 2021; Kim et al., 2021). Alam et al. (2002) also did not show any difference in AA profile of the whole-body olive flounder fed diets containing different AA patterns. However, there were some contradictory results presenting remarkable changes in the proximate composition (Kader et al., 2012; Kim et al., 2014; Peng et al., 2022) and AA profiles (Alam et al., 2018; Chen et al., 2019; Mastoraki et al., 2020) of fish by dietary replacement of FM with other protein sources.
5 Conclusion
Weight gain of olive flounder fed the CJ30, CJ40, and CJ50 diets was comparable to fish fed the Con diet. Furthermore, SGR and feed consumption of olive flounder fed the CJ20, CJ30, CJ40, and CJ50 diets were comparable to fish fed the Con diet. However, feed utilization, biological indices except for K, proximate composition, AA profiles, and hematological measurements of fish were not altered by dietary treatments. Inclusion of 30 and 20% JMM inclusion were the most recommendable in the olive flounder diets substituting 50% FM with CGM based on weight gain, and SGR and feed consumption, respectively.
Data availability statement
The original contributions presented in the study are included in the article/supplementary material, further inquiries can be directed to the corresponding author.
Ethics statement
The animal study was approved by Korea Maritime and Ocean University (KMOU IACUC 2021–05). The study was conducted in accordance with the local legislation and institutional requirements.
Author contributions
MI: Data curation, Investigation, Writing – original draft. SC: Conceptualization, Funding acquisition, Methodology, Project administration, Supervision, Writing – review & editing. TK: Funding acquisition, Writing – review & editing.
Funding
The author(s) declare that financial support was received for the research, authorship, and/or publication of this article. This research was supported by the Korea Institute of Marine Science & Technology Promotion (KIMST) funded by the Ministry of Oceans and Fisheries (RS-2018-KS181194).
Conflict of interest
The authors declare that the research was conducted in the absence of any commercial or financial relationships that could be construed as a potential conflict of interest.
Publisher’s note
All claims expressed in this article are solely those of the authors and do not necessarily represent those of their affiliated organizations, or those of the publisher, the editors and the reviewers. Any product that may be evaluated in this article, or claim that may be made by its manufacturer, is not guaranteed or endorsed by the publisher.
References
Alam, M. S., Teshima, S., Ishikawa, M., and Koshio, S. (2000). Methionine requirement of juvenile Japanese flounder Paralichthys olivaceus. J. World Aquac. Soc. 31, 618–626. doi: 10.1111/j.1749-7345.2000.tb00911.x
Alam, M. S., Teshima, S., Yaniharto, D., Koshio, S., and Ishikawa, M. (2002). Influence of different dietary amino acid patterns on growth and body composition of juvenile Japanese flounder, Paralichthys olivaceus. Aquaculture 210, 359–369. doi: 10.1016/S0044-8486(01)00892-4
Alam, M. S., Watanabe, W. O., Carroll, P. M., Gabel, J. E., Corum, M. A., Seaton, P., et al. (2018). Evaluation of genetically-improved (glandless) and genetically-modified low-gossypol cottonseed meal as alternative protein sources in the diet of juvenile southern flounder Paralichthys lethostigma reared in a recirculating aquaculture system. Aquaculture 489, 36–45. doi: 10.1016/j.aquaculture.2018.02.006
Association of Official Analytical Chemists (1990). “Association of official analytical chemists” in The official methods of analysis, association of official analytical chemists. 15th ed (Arlington, VA, USA: Association of analytical chemists,).
Bae, K. M., Kim, K. W., and Lee, S. M. (2015). Evaluation of rice distillers dried grain as a partial replacement for fish meal in the practical diet of the juvenile olive flounder Paralichthys olivaceus. Fish. Aquat. Sci. 18, 151–158. doi: 10.5657/FAS.2015.0151
Baek, S. I., Cho, S. H., and Kim, H. S. (2021). Dietary inclusion effect of various levels of jack mackerel meal on the growth performance, feed efficiency and whole body composition of rockfish (Sebastes schlegeli). Fish. Aquat. Sci. 24, 311–317. doi: 10.47853/FAS.2021.e30
Bui, H. T. D., Khosravi, S., Fournier, V., Herault, M., and Lee, K. J. (2014). Growth performance, feed utilization, innate immunity, digestibility and disease resistance of juvenile red seabream (Pagrus major) fed diets supplemented with protein hydrolysates. Aquaculture 418-419, 11–16. doi: 10.1016/j.aquaculture.2013.09.046
Chen, Y., Ma, J., Huang, H., and Zhong, H. (2019). Effects of the replacement of fishmeal by soy protein concentrate on growth performance, apparent digestibility, and retention of protein and amino acid in juvenile pearl gentian grouper. PLoS One 14:e0222780. doi: 10.1371/journal.pone.0222780
Choi, W., Hamidoghli, A., Bae, J., Won, S., Choi, Y. H., Kim, K. W., et al. (2020). On-farm evaluation of dietary animal and plant proteins to replace fishmeal in sub-adult olive flounder Paralichthys olivaceus. Fish. Aquatic Sci. 23, 1–8. doi: 10.1186/s41240-020-00169-4
Finkelstein, J. D., Martin, J. J., and Harris, B. J. (1988). Methionine metabolism in mammals. The methionine-sparing effect of cystine. J. Biol. Chem. 263, 11750–11754. doi: 10.1016/S0021-9258(18)37847-5
Folch, J., Lee, M., and Stanley, G. H. S. (1957). A simple method for the isolation and purification of total lipids from animal tissues. J. Biol. Chem. 226, 497–509. doi: 10.1016/S0021-9258(18)64849-5
Forster, I., and Ogata, H. Y. (1998). Lysine requirement of juvenile Japanese flounder Paralichthys olivaceus and juvenile red sea bream Pagrus major. Aquaculture 161, 131–142. doi: 10.1016/S0044-8486(97)00263-9
Furuita, H., Tanaka, H., Yamamoto, T., Suzuki, N., and Takeuchi, T. (2002). Effects of high levels of n-3 HUFA in brood stock diet on egg quality and egg fatty acid composition of Japanese flounder, Paralichthys olivaceus. Aquaculture 210, 323–333. doi: 10.1016/S0044-8486(01)00855-9
Gamboa-Delgado, J., Rojas-Casas, M. G., Nieto-López, M. G., and Cruz-Suárez, L. E. (2013). Simultaneous estimation of the nutritional contribution of fish meal, soy protein isolate and corn gluten to the growth of Pacific white shrimp (Litopenaeus vannamei) using dual stable isotope analysis. Aquaculture 380-383, 33–40. doi: 10.1016/j.aquaculture.2012.11.028
Gunathilaka, B. E., Khosravi, S., Shin, J., Shin, J., Herault, M., Fournier, V., et al. (2021). Evaluation of shrimp protein hydrolysate and krill meal supplementation in low fish meal diet for red seabream (Pagrus major). Fish. Aquat. Sci. 24, 109–120. doi: 10.47853/FAS.2021.e11
Ha, M. S., Cho, S. H., and Kim, T. (2021). Dietary substitution of fish meal by meat meal: effects on juvenile olive flounder (Paralichthys olivaceus) growth performance, feed utilization, haematology, biochemical profile, and disease resistance against Streptococcus iniae. Aquac. Nutr. 27, 1888–1902. doi: 10.1111/anu.13326
Hamidoghli, A., Won, S., Lee, S., Lee, S., Farris, N. W., and Bai, S. C. (2020). Nutrition and feeding of olive flounder Paralichthys olivaceus: a review. Rev. Fish. Sci. Aquac. 28, 340–357. doi: 10.1080/23308249.2020.1740166
Hancz, C. (2020). Feed efficiency, nutrient sensing, and feeding stimulation in aquaculture: a review. Acta Agr. Kapos. 24, 35–54. doi: 10.31914/aak.2375
Harding, D. E., Allen, O. W., and Wilson, R. P. (1977). Sulfur amino acid requirement of channel catfish: l-methionine and l-cystine. J. Nutr. 107, 2031–2035. doi: 10.1093/jn/107.11.2031
Hernández, C., Lizárraga-Velázquez, C. E., Contreras-Rojas, D., Sánchez-Gutiérrez, E. Y., Martínez-Montaño, E., Ibarra-Castro, L., et al. (2021). Fish meal replacement by corn gluten in feeds for juvenile spotted rose snapper (Lutjanus guttatus): effect on growth performance, feed efficiency, hematological parameters, protease activity, body composition, and nutrient digestibility. Aquaculture 531:735896. doi: 10.1016/j.aquaculture.2020.735896
Hosokawa, H., Takii, K., Shimeno, S., and Takeda, M. (2001). Identification of feeding stimulants for yellowtail in muscle extract of jack mackerel. Aquac. Sci. 49, 225–229. doi: 10.11233/aquaculturesci1953.49.225
Ikeda, I., Okamoto, Y., and Oda, K. (2012). Identification of feeding stimulants for Japanese flounder in muscle extract of jack mackerel. Aquac. Sci. 60, 195–198. doi: 10.11233/aquaculturesci.60.195
Jannathulla, R., Rajaram, V., Kalanjiam, R., Ambasankar, K., Muralidhar, M., and Dayal, J. S. (2019). Fishmeal availability in the scenarios of climate change: inevitability of fishmeal replacement in aquafeeds and approaches for the utilization of plant protein sources. Aquac. Res. 50, 3493–3506. doi: 10.1111/are.14324
Jeong, H. S., and Cho, S. H. (2023). Inclusion effect of jack mackerel meal as feed stimulants in diets replacing different levels of fish meal with various animal protein sources on growth performance of olive flounder (Paralichthys olivaceus). Aquac. Rep. 28:101450. doi: 10.1016/j.aqrep.2022.101450
Jeong, H. S., Choi, D. G., Lee, K. W., Cho, S. H., Lim, S. G., Lee, B. J., et al. (2020). Attractiveness of various crude feed ingredients to juvenile olive flounder (Paralichthys olivaceus, Temminck & Schlegel) and its application to aquaculture. Aquac. Res. 51, 4517–4532. doi: 10.1111/are.14797
Jeong, H. S., Kim, J., Olowe, O. S., and Cho, S. H. (2022). Dietary optimum inclusion level of jack mackerel meal for olive flounder (Paralichthys olivaceus, Temminck & Schlegel, 1846). Aquaculture 559:738432. doi: 10.1016/j.aquaculture.2022.738432
Kader, M. A., Bulbul, M., Koshio, S., Ishikawa, M., Yokoyama, S., Nguyen, B. T., et al. (2012). Effect of complete replacement of fishmeal by dehulled soybean meal with crude attractants supplementation in diets for red sea bream Pagrus major. Aquaculture 350-353, 109–116. doi: 10.1016/j.aquaculture.2012.04.009
Kader, M. A., Koshio, S., Ishikawa, M., Yokoyama, S., and Bulbul, M. (2010). Supplemental effects of some crude ingredients in improving nutritive values of low fishmeal diets for red sea bream, Pagrus major. Aquaculture 308, 136–144. doi: 10.1016/j.aquaculture.2010.07.037
Khosravi, S., Bui, H. T. D., Herault, M., Fournier, V., Kim, K., Lee, B., et al. (2018). Supplementation of protein hydrolysates to a low-fishmeal diet improves growth and health status of juvenile olive flounder, Paralichthys olivaceus. J. World Aquac. Soc 49, 897–911. doi: 10.1111/jwas.12436
Khosravi, S., Bui, H. T. D., Rahimnejad, S., Herault, M., Fournier, V., Jeong, J. B., et al. (2015). Effect of dietary hydrolysate supplementation on growth performance, non-specific immune response and disease resistance of olive flounder (Paralichthys olivaceus) challenged with Edwardsiella tarda. Aquac. Nutr. 21, 321–331. doi: 10.1111/anu.12157
Kikuchi, K. (1999a). Partial replacement of fish meal with corn gluten meal in diets for Japanese flounder Paralichthys olivaceus. J. World Aquac. Soc. 30, 357–363. doi: 10.1111/j.1749-7345.1999.tb00686.x
Kikuchi, K. (1999b). Use of defatted soybean meal as a substitute for fish meal in diets of Japanese flounder (Paralichthys olivaceus). Aquaculture 179, 3–11. doi: 10.1016/S0044-8486(99)00147-7
Kim, H. S., Baek, S. I., Lee, K. W., Jeong, H. S., and Cho, S. H. (2019). Attractiveness of various protein sources to juvenile rockfish (Sebastes schlegeli, Hilgendorf 1880). J. Appl. Aquac. 32, 205–220. doi: 10.1080/10454438.2019.1655516
Kim, H. S., and Cho, S. H. (2019). Dietary inclusion effect of feed ingredients showing high feeding attractiveness to rockfish (Sebastes schlegeli Hilgendorf 1880) on the growth performance, feed utilization, condition factor, and whole-body composition of fish. Comp. Biochem. Physiol. Part A Mol. Integr. Physiol. 231, 66–73. doi: 10.1016/j.cbpa.2019.01.011
Kim, J., Cho, S. H., Kim, T., and Hur, S. W. (2021). Substitution effect of fish meal with various sources of animal by-product meals in feed on growth, feed utilization, body composition, haematology and non-specific immune response of olive flounder (Paralichthys olivaceus, Temminck & Schlegel, 1846). Aquac. Res. 52, 2802–2817. doi: 10.1111/are.15132
Kim, H. S., Jung, W. G., Myung, S. H., Cho, S. H., and Kim, D. S. (2014). Substitution effects of fishmeal with tuna byproduct meal in the diet on growth, body composition, plasma chemistry and amino acid profiles of juvenile olive flounder (Paralichthys olivaceus). Aquaculture 431, 92–98. doi: 10.1016/j.aquaculture.2014.03.025
Kim, K., and Lee, S. (2004). Requirement of dietary n-3 highly unsaturated fatty acids for juvenile flounder (Paralichthys olivaceus). Aquaculture 229, 315–323. doi: 10.1016/S0044-8486(03)00356-9
KOSIS . (2023). Korean Statistical Information Service, Korea. Available at: https://kosis.kr/eng/ (Accessed February 25, 2023).
Kubitza, F., and Lovshin, L. L. (1999). Formulated diets, feeding strategies, and cannibalism control during intensive culture of juvenile carnivorous fishes. Rev. Fish. Sci. 7, 1–22. doi: 10.1080/10641269991319171
Lange, S., Guđmundsdottir, B. K., and Magnadottir, B. (2001). Humoral immune parameters of cultured Atlantic halibut (Hippoglossus hippoglossus L.). Fish Shellfish Immunol. 11, 523–535. doi: 10.1006/fsim.2000.0333
Lee, S., Aya, F. A., Won, S., Hamidoghli, A., and Bai, S. C. (2020). Effects of replacing dietary fish oil with beef tallow on growth performance, serological parameters, and fatty acid composition in juvenile olive flounder, Paralichthys olivaceus. J. World Aquac. Soc. 51, 393–406. doi: 10.1111/jwas.12654
Li, X., Fang, T., Wang, J., Wang, Z., Guan, D., Sun, H., et al. (2022). The efficiency of adding amino acid mixtures to a diet free of fishmeal and soybean meal as an attractant in yellow river carp (Cyprinus carpio var.). Aquac. Rep. 24:101189. doi: 10.1016/j.aqrep.2022.101189
Lim, S. J., and Lee, K. J. (2008). Supplemental iron and phosphorus increase dietary inclusion of cottonseed and soybean meal in olive flounder (Paralichthys olivaceus). Aquac. Nutr. 14, 423–430. doi: 10.1111/j.1365-2095.2007.00546.x
Lutfi, E., Berge, G. M., Bæverfjord, G., Sigholt, T., Bou, M., Larsson, T., et al. (2023). Increasing dietary levels of the n-3 long-chain PUFA, EPA and DHA, improves the growth, welfare, robustness and fillet quality of Atlantic salmon in sea cages. Br. J. Nutr. 129, 10–28. doi: 10.1017/S0007114522000642
Mastoraki, M., Ferrándiz, P. M., Vardali, S. C., Kontodimas, D. C., Kotzamanis, Y. P., Gasco, L., et al. (2020). A comparative study on the effect of fish meal substitution with three different insect meals on growth, body composition and metabolism of European sea bass (Dicentrarchus labrax L.). Aquaculture 528:735511. doi: 10.1016/j.aquaculture.2020.735511
Morais, S. (2017). The physiology of taste in fish: potential implications for feeding stimulation and gut chemical sensing. Rev. Fish. Sci. Aquac. 25, 133–149. doi: 10.1080/23308249.2016.1249279
Nagel, F., von Danwitz, A., Schlachter, M., Kroeckel, S., Wagner, C., and Schulz, C. (2014). Blue mussel meal as feed attractant in rapeseed protein-based diets for turbot (Psetta maxima L.). Aquac. Res. 45, 1964–1978. doi: 10.1111/are.12140
Oliva-Teles, A., Enes, P., and Peres, H. (2015). “Replacing fishmeal and fish oil in industrial aquafeeds for carnivorous fish” in Feed and feeding practices in aquaculture. ed. D. A. Davis (Sawston, Cambridge, United Kingdom: Elsevier), 203–233.
Oncul, F. O., Aya, F. A., Hamidoghli, A., Won, S., Lee, G., Han, K. R., et al. (2019). Effects of the dietary fermented tuna by-product meal on growth, blood parameters, non-specific immune response, and disease resistance in juvenile olive flounder, Paralichthys olivaceus. J. World Aquac. Soc. 50, 65–77. doi: 10.1111/jwas.12535
Papatryphon, E., and Soares, J. H. (2000). The effect of dietary feeding stimulants on growth performance of striped bass, Morone saxatilis, fed-a-plant feedstuff-based diet. Aquaculture 185, 329–338. doi: 10.1016/S0044-8486(99)00348-8
Peng, D., Peng, B., Li, J., Zhang, Y., Luo, H., Xiao, Q., et al. (2022). Effects of three feed attractants on the growth, biochemical indicators, lipid metabolism and appetite of Chinese perch (Siniperca chuatsi). Aquac. Rep. 23:101075. doi: 10.1016/j.aqrep.2022.101075
Pereira, T. G., and Oliva-Teles, A. (2003). Evaluation of corn gluten meal as a protein source in diets for gilthead sea bream (Sparus aurata L.) juveniles. Aquac. Res. 34, 1111–1117. doi: 10.1046/j.1365-2109.2003.00909.x
Peter, C. M., Han, Y., Boling-Frankenbach, S. D., Parsons, C. M., and Baker, D. H. (2000). Limiting order of amino acids and the effects of phytase on protein quality in corn gluten meal fed to young chicks. J. Anim. Sci. 78, 2150–2156. doi: 10.2527/2000.7882150x
Pham, M. A., Lee, K. J., Lim, S. J., and Park, K. H. (2007). Evaluation of cottonseed and soybean meal as partial replacement for fishmeal in diets for juvenile Japanese flounder Paralichthys olivaceus. Fish. Sci. 73, 760–769. doi: 10.1111/j.1444-2906.2007.01394.x
Rahimnejad, S., Lee, S. M., Park, H. G., and Choi, J. (2017). Effects of dietary inclusion of chlorella vulgaris on growth, blood biochemical parameters, and antioxidant enzyme activity in olive flounder, Paralichthys olivaceus. J. World Aquac. Soc. 48, 103–112. doi: 10.1111/jwas.12320
Regost, C., Arzel, J., and Kaushik, S. J. (1999). Partial or total replacement of fish meal by corn gluten meal in diet for turbot (Psetta maxima). Aquaculture 180, 99–117. doi: 10.1016/S0044-8486(99)00026-5
Satheeshkumar, P., Ananthan, G., Senthilkumar, D., Khan, A. B., and Jeevanantham, K. (2012). Comparative investigation on haematological and biochemical studies on wild marine teleost fishes from Vellar estuary, southeast coast of India. Comp. Clin. Path. 21, 275–281. doi: 10.1007/s00580-010-1091-5
Sevgili, H., Kurtoğlu, A., Oikawa, M., Aksoy, A., Kocakaya, S., Öztürk, E., et al. (2015). A combination of corn gluten and soybean meal as a substitute for fishmeal in diets of turbot (Scophthalmus maximus Linnaeus, 1758) in brackish water. J. Appl. Ichthyol. 31, 355–361. doi: 10.1111/jai.12683
Sharf, Y., and Khan, M. A. (2020). Effect of dietary isoleucine level on growth, protein retention efficiency, haematological parameter, lysozyme activity and serum antioxidant status of fingerling Channa punctatus (Bloch). Aquacul. Nutr. 26, 908–920. doi: 10.1111/anu.13049
Stieglitz, J. D., Hoenig, R. H., Baggett, J. K., Tudela, C. E., Mathur, S. K., and Benetti, D. D. (2021). Advancing production of marine fish in the United States: olive flounder, Paralichthys olivaceus, aquaculture. J. World Aquac. 52, 566–581. doi: 10.1111/jwas.12804
Tacon, A. G. J., and Metian, M. (2008). Global overview on the use of fish meal and fish oil in industrially com-pounded aquafeeds: trends and future prospects. Aquaculture 285, 146–158. doi: 10.1016/j.aquaculture.2008.08.015
Takakuwa, F., Masumoto, T., and Fukada, H. (2019). Identification of feeding stimulants for greater amberjack Seriola dumerili in muscle tissue of jack mackerel Trachurus japonicus. Fish. Sci. 85, 387–395. doi: 10.1007/s12562-018-01285-w
Tusche, K., Berends, K., Wuertz, S., Susenbeth, A., and Schulz, C. (2011). Evaluation of feed attractants in potato protein concentrate based diets for rainbow trout (Oncorhynchus mykiss). Aquaculture 321, 54–60. doi: 10.1016/j.aquaculture.2011.08.020
Twibell, R. G., Wilson, K. A., and Brown, P. B. (2000). Dietary sulfur amino acid requirement of juvenile yellow perch fed the maximum cystine replacement value for methionine. J. Nutr. 130, 612–616. doi: 10.1093/jn/130.3.612
Wang, L., Yin, N., Sagada, G., Hua, Y., Li, H., Zhang, J., et al. (2020). Partial replacement of fishmeal with corn gluten meal, pea protein isolate and their mixture in diet of black sea bream (Acanthopagrus schlegelii) juveniles: effects on growth performance, feed utilization and haematological parameters. Aquac. Res. 51, 2071–2083. doi: 10.1111/are.14558
Ye, J. D., Wang, K., Li, F. D., Sun, Y. Z., and Liu, X. H. (2011). Incorporation of a mixture of meat and bone meal, poultry by-product meal, blood meal and corn gluten meal as a replacement for fish meal in practical diets of Pacific white shrimp Litopenaeus vannamei at two dietary protein levels. Aquac. Nutr. 17, e337–e347. doi: 10.1111/j.1365-2095.2010.00768.x
Zeynali, M., Nafisi Bahabadi, M., Morshedi, V., Ghasemi, A., and Mozanzadeh, T. M. (2020). Replacement of dietary fishmeal with Sargassum ilicifolium meal on growth, innate immunity and immune gene mRNA transcript abundance in Lates calcarifer juveniles. Aquac. Nutr. 26, 1657–1668. doi: 10.1111/anu.13111
Keywords: jack mackerel meal, fish meal substitution, olive flounder, corn gluten meal, feed stimulants, feed utilization
Citation: Islam MR, Cho SH and Kim T (2024) Inclusion effect of jack mackerel meal in diets substituting fish meal with corn gluten meal on growth and feed utilization of olive flounder (Paralichthys olivaceus). Front. Sustain. Food Syst. 8:1414574. doi: 10.3389/fsufs.2024.1414574
Edited by:
Pallab Sarker, University of California, United StatesReviewed by:
Md. Tawheed Hasan, Sylhet Agricultural University, BangladeshWon Je Jang, Pukyong National University, Republic of Korea
Copyright © 2024 Islam, Cho and Kim. This is an open-access article distributed under the terms of the Creative Commons Attribution License (CC BY). The use, distribution or reproduction in other forums is permitted, provided the original author(s) and the copyright owner(s) are credited and that the original publication in this journal is cited, in accordance with accepted academic practice. No use, distribution or reproduction is permitted which does not comply with these terms.
*Correspondence: Sung Hwoan Cho, chosunh@kmou.ac.kr