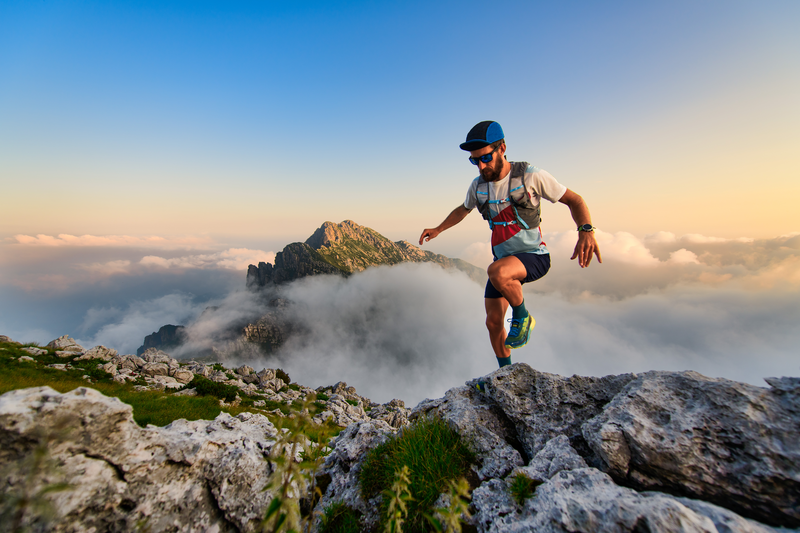
95% of researchers rate our articles as excellent or good
Learn more about the work of our research integrity team to safeguard the quality of each article we publish.
Find out more
ORIGINAL RESEARCH article
Front. Sustain. Food Syst. , 25 July 2024
Sec. Crop Biology and Sustainability
Volume 8 - 2024 | https://doi.org/10.3389/fsufs.2024.1412610
The present research explored the impact of varying concentrations of arbuscular mycorrhizal (AM) fungus inoculum and vermicompost on the growth, nutrient absorption, photosynthetic gas exchange, and quality parameters of ginger over a 2-year period in field conditions. In this study, the combination of 50 g each of AM and vermicompost increased plant height, number of tillers, and rhizome yield compared to the control. However, higher dry biomass (61 g) was observed with the combination of 75 g of each amendment. As expected, the application of arbuscular mycorrhizae (AM) positively affects spore count and mycorrhizal dependency percentage ranging from 58 to 70.5 spores per 50 g substrate and 19–36%, respectively. The combined use of vermicompost and AM led to a lower disease incidence of 10.5% in treatments with 25 g of each amendment and 10.1% in treatments with 50 g of each. Nutrient accumulations, particularly phosphorus (P), iron (Fe), and zinc (Zn), exhibited greater levels in ginger plants treated with vermicompost and arbuscular mycorrhizal (AM) inoculation, compared to uninoculated ginger rhizomes. The plants treated with AM and vermicompost increased the biomass accumulation by increasing the stomatal conductance and photosynthetic rate of leaves. AM and vermicompost improved ginger rhizome quality, increasing phenols by 37.8%, flavonoids by 35.7%, and essential oil by 29% compared to the control. The analysis revealed that the total flavonoid content was significantly higher in AM-treated samples compared to the control. However, the phenol content did not exhibit statistical significance across the treatments. Regarding essential oil (EO) content, our experiment highlighted that treatments with AM and vermicompost have consistently yielded higher EO content compared to other treatments. In contrast, there was no discernible trend in the fiber content with the application of AM and vermicompost amendments. PCA and correlation analyses revealed a positive influence on plant growth, nutrient absorption, and quality parameters, except for the incidence of diseases in ginger. Overall, our study finds that the concurrent use of vermicompost and arbuscular mycorrhizae (AM) makes a substantial contribution to the growth, nutrient uptake, photosynthetic, and quality parameters of ginger.
Ginger (Zingiber officinale Rosc.) is a medicinal herb belonging to the family of Zingiberaceae. It is mainly found in tropical regions, including India, China, Indonesia, and Nigeria. The rhizome is rich in phytochemicals such as zingiberene, α-curcumin, gingerols, and shogaols, which are the primary bioactive compounds in ginger supplements. These bioactive compounds exhibit a broad spectrum of biological activities, including anti-inflammatory, antioxidant, anticancer, antimicrobial, gastroprotective, and cardioprotective effects. These properties make ginger a valuable component in traditional and modern medicine for the prevention and treatment of various diseases (Ravindran et al., 1994). Ginger cultivation is fraught with challenges that significantly impact its production and yield. Insect pests such as shoot borers, rhizome scales, and aphids pose a direct threat to plant health. Pathogenic diseases, particularly bacterial wilt, soft rot, Fusarium yellows, and Pythium rhizome rot, can cause severe crop losses. In addition, non-pathogenic factors such as nutrient deficiencies, water stress, and soil pH imbalances further complicate ginger cultivation. Effective management strategies, including integrated pest management, proper disease control, and optimal cultivation practices, are essential to mitigate these challenges and ensure successful ginger production (Prasath et al., 2014; Bhai et al., 2019).
The increasing demand for organic products underscores the necessity of transitioning ginger production toward organic farming methods (Marsh et al., 2021). Conventional agricultural practices, while effective in boosting short-term yields, lead to soil degradation, nutrient imbalance, and environmental pollution (Igiehon and Babalola, 2018). In contrast, organic farming promotes sustainable practices that enhance soil health, conserve natural resources, and produce healthier products. By adopting organic methods, ginger farmers can contribute to a more sustainable and environmentally friendly agricultural system, meeting consumer demand while ensuring the long-term viability of their farming operations.
Various methods have been explored to decrease reliance on synthetic fertilizers in agroecosystems, with biostimulants emerging as a promising solution for promoting sustainability (Zhang et al., 2023; Xu et al., 2024). Biostimulants play a crucial role in assisting agroecosystems in managing environmental stress by enhancing the absorption of vital nutrients and plant-water balance, improving photosynthesis, and consequently contributing to the production of high-quality agricultural products (Hu et al., 2022; Li et al., 2022). As research and development in this field continue, biostimulants are likely to play an increasingly vital role in sustainable agriculture, helping to meet the growing demand for food while preserving natural resources and protecting the environment. Arbuscular mycorrhizae are a widely recognized biostimulant that can provide numerous benefits to plants and function not only as biofertilizers but also as bioprotectors and bioregulators, enhancing overall plant health and productivity through symbiosis.
In addition, this fungus can penetrate the root cortical cells and form specific haustoria-like structures termed arbuscules. These structures act as mediators for exchanging metabolites among the fungi and the host cytoplasm (Begum et al., 2019). Arbuscular mycorrhizae contribute not only to the breakdown of soil organic matter but also play a pivotal role in the plant’s ability to sequester atmospheric carbon dioxide (Qiu et al., 2023; Wang et al., 2024). This is achieved through the sink effect and facilitation of the transfer of photosynthates from aboveground organs to the roots. This function is crucial in enhancing the physical characteristics of soil like aggregation and assisting the plant in acquiring nutrients and water from the soil (Sharda and Koide, 2010; Wu et al., 2011; Khan et al., 2020). AM fungi have the capacity to influence the rhizosphere environment, potentially enhancing the production of plant metabolites (Falcao et al., 2024).
The use of bio-organic compounds provides a viable option for enhancing soil physicochemical properties, plant growth, and yield. Applying compost can improve the stability and physical characteristics of soil by augmenting organic matter, overall porosity, hydraulic conductivity, formation of aggregates, and the capacity to retain water. These modifications can result in shifts in the soil microbiome, ultimately enhancing plants’ ability to withstand abiotic stresses (Benaffari et al., 2022). Employing beneficial microbes along with compost is recognized as a vital and efficient strategy for enhancing nutrient utilization efficiency in less-than-ideal conditions. Co-amending soils with compost and microbial inocula can often result in a synergistic effect on plant growth. Vermicompost contains humic acid, which can promote spore production and hyphal growth in mycorrhizal fungi. The application of a small amount of compost together with AM inoculation can have a symbiotic effect, enhancing plant growth and phosphorus and zinc uptake. The substantial microbial diversity and nutrient richness present in compost and vermicompost can collaboratively contribute to the growth of AM fungi (Cavagnaro, 2014). Numerous horticultural crops, including bananas, potatoes, grapevine, apples, strawberries, artichokes, melons, and chilies, have stated the positive growth parameters as a result of AM inoculation (Schellenbaum et al., 1991; Vosátka and Gryndler, 2000; Borkowska, 2002; Declerck et al., 2002; Locatelli and Lovato, 2002; Fortunato et al., 2005; Benkebboura et al., 2024; Pereira et al., 2024). While Taber and Trappe (1982) were the first to investigate the presence of AM in rhizomes of ginger (Z. officinale) grown in Fiji and Hawaii, the impact and underlying mechanism of AM fungi, in conjunction with compost, on improving the growth of ginger, are still not fully understood. Arbuscular mycorrhizal fungi elevate photosynthetic gas exchange and augment water absorption by orchestrating intricate communication processes between the host and the fungus (Birhane et al., 2012). The addition of organic manure with AM fungi improved the soil respiration and other photosynthetic parameters in Lettuce (Chatzistathis et al., 2024). In addition, Govindjee (1995) reported that AM fungi enhanced photosynthesis through changes in chlorophyll fluorescence (Fv/Fm). Available information on the mechanisms of AM associated with photosynthetic light use is limited.
In this study, AM fungi (Rhizophagus sp.) with or without compost were tested on ginger plants under field conditions for 2 years. Previous research has demonstrated the positive effects of both AM fungi and vermicompost on plant growth and yield individually. However, limited studies have investigated the combined effects of these biostimulants on ginger plants under field conditions. Considering the synergistic potential of AM fungi and compost, it is hypothesized that their combined application will lead to improvements in ginger growth and yield compared to their individual application or absence thereof. With this hypothesis, we aimed (a) to evaluate the impact of AM fungi with vermicompost application on the plant growth, nutrient uptake, and disease incidence of ginger and (b) to assess the influence of AM fungi with vermicompost application on physiological measurements and quality parameters of ginger.
The experiment was conducted at the Experimental farm (11.29395°N, 75.82038°E), ICAR-Indian Institute of Spices Research, Chelavoor, Kozhikode, Kerala, India, for 2 years (2020–2021 and 2021–2022). Because ginger depletes nutrients from the soil, the experiment was not replicated in the same site but instead conducted in a new area within the location. The ginger crop (variety: IISR Varada) grown in the raised bed with the size 3 × 1 × 0.30 m (l X b X h) prepared and healthy disease-free seed rhizomes (25 g) with sprouts were used in the population of 40 plants per bed. The standard package of practices was implemented throughout the entire process from planting to harvesting (Rajeev and Thomas, 2015). The type of soil in the experimental site was a red lateritic clay loam, and their initial soil properties were pH 5.96, organic carbon 2.3 (%), nitrogen (N) 243.6 kg ha−1, phosphorus (P) 9.7 kg ha−1, potassium (K) 295 kg ha−1, Ca 2,132 ppm, Mg 114 ppm, Fe 12.4 ppm, Zn 1.34 ppm, Mn 35 ppm, and Cu 1.62 ppm. The study comprised of 10 treatments with three replications, as follows: T1: Control; T2: AM (25 g); T3: AM (50 g); T4: AM (75 g); T5: T2+ 25 g vermicompost; T6: T3+ 50 g vermicompost; T7: T4+ 75 g vermicompost; T8: 25 g vermicompost; T9:50 g vermicompost; T10:75 g vermicompost. Both amendments were applied at the time of planting. Observations on plant growth parameters such as plant height, tiller number, dry biomass, photosynthesis gas exchange parameters, mycorrhizal parameters, and disease incidence were recorded at 90 after planting (DAP) by destructive sampling. The rhizome yield was measured post-harvest at 240 DAP and expressed in t ha−1.
The inoculum of Arbuscular Mycorrhizal (AM) fungi, specifically Rhizophagus sp. (MN710507), was formulated using vermiculite as the carrier. Each gram of the inoculum contains 100 infective propagules, which include spores (100–125 μm in size), hyphae, and mycorrhizal roots. This formulation is designed to maximize the symbiotic association between the AM fungi and the plant roots, enhancing nutrient uptake and plant health. Vermicompost was produced using the composting worm Perionyx ceylanensis. The nutrient composition of the vermicompost is as follows: nitrogen (N) at 1%, phosphorus (P) at 1.3%, and potassium (K) at 0.58%. Both amendments were applied at the time of planting to enhance the soil fertility and plant growth.
To extract AM spores, a beaker containing 50 g of soil sample was meticulously mixed with half a liter of water to ensure the soil was well dispersed in the water, allowing the spores to be released from soil aggregates. Following a 1-h period, the beaker’s contents were filtered using a sequence of sieves organized in decreasing size order, ranging from 600 μm to 37 μm. The sifted materials were gathered in a Petri plate, and the spore count was conducted using a stereo zoom microscope (Gerdemann and Nicolson, 1963).
Mycorrhizal dependency (MD) denotes the extent of alterations in plant growth linked to arbuscular mycorrhizal (AM) fungi colonization. This relationship indicates how much a plant relies on its symbiotic association with AM fungi to achieve optimal growth and development.
MD was determined based on the formula of Plenchette et al. (1983):
Well-dried and powdered plants were taken for nutrient uptake analysis. Nitrogen (N) uptake was determined through the Kjeldahl method (Nelson and Sommers, 1973). Phosphorus (P) estimation involved digesting 1 g of powdered sample with a mixture of nitric acid (HNO3) and hydrochloric acid (HCl 60%) at 9:4 v:v ratio. The assessment was carried out using a spectrophotometer at 660 nm (Jackson, 1973). Analysis of exchangeable potassium (K), magnesium (Mg), and calcium (Ca) was conducted using an atomic absorption spectrophotometer. The zinc (Zn), iron (Fe), copper (Cu), and manganese (Mn) were estimated through diethylenetriaminepentaacetic acid (DTPA) extraction and subsequently analyzed using an atomic absorption spectrophotometer (Thomas, 1982).
During the 90th day, percentage disease incidence (PDI) was calculated (Praveena et al., 2021) based on infected plants divided by the total number of plants assessed as indicated below:
On the 90th day post-planting, measurements of net photosynthetic rate (Pn), stomatal conductance (Gs), transpiration rate (Tr), and leaf temperature (Tleaf) were conducted on the third fully expanded active set of leaves. The parameters were evaluated under controlled saturating photosynthetic photon flux (900 mmol m−2 s−1). This assessment took place during the daytime, specifically between 09:30 and 11:30 am, utilizing a portable photosynthesis system. Measurements were taken when both Pn and Gs reached a stable state. Three representative plants were randomly chosen from each treatment to record these parameters, and the average was calculated from five readings spaced evenly over 120 s.
Following the measurement of gas exchange parameters, the identical leaves were employed for chlorophyll fluorescence assessment. This evaluation took place during the daytime, specifically between 09:30 and 11:30 am, utilizing a portable fluorometer (Os-30 p). After the leaves underwent a 30-min adaptation to darkness, the obtained data were utilized to calculate the maximum efficiency of PSII (Fv/Fm), as described by Strasser et al. (1995). The average was derived from five readings.
For estimation of TPC in ginger, ginger extract was prepared by extracting the 1 g ground ginger with 80% methanol and making the extract up to the volume of 25 mL. From that, 0.2 mL of extract and 0.5 mL of Folin–Ciocalteu reagent were added. After mixing, it was kept incubated for 3 min at room temperature, and 2 mL of 10% sodium carbonate was added and mixed well. The tubes were kept in the dark at ambient temperature for 60 min after that the absorbance of the solution was measured in the spectrophotometer at 650 nm and expressed as a milligram of gallic acid equivalent phenol/g of extract (Sadasivam and Manickam, 2008).
To determine flavonoid levels, 0.2 mL of ginger extract was mixed with 0.1 mL of 1 M potassium acetate and 0.1 mL of 10% aluminum chloride. The blend was meticulously stirred and left to incubate at room temperature for a duration of 30 min, and the absorbance was measured at 415 nm, using quercetin as the standard (Miguel et al., 2010).
EO was extracted from the dried ginger sample (30 g) using the Clevenger-type hydrodistillation method (ASTA, 1997). After the extraction process, the remaining moisture was removed with anhydrous sodium sulfate (Na2SO4) and stored at 4°C. The percentage essential oil content was calculated using the formula:
The dried and powdered sample of the ginger was weighed 10 grams packed in cotton wool and placed in a glass column. Then, 50 mL of acetone was added and kept overnight. To determine the percentage of oleoresin, the filtrate was extracted and evaporated to dryness and weighed (ASTA, 1997), and the yield of oleoresin was calculated using the formula:
For crude fiber estimation, 1 g of crushed ginger was treated with 1.25% H2SO4 and boiled at 400°C for 45 min. Afterward, 1.25% NaOH was added and boiled, followed by washing and drying to a constant weight in an oven. To achieve the constant weight, the crucibles with residue were incinerated at 500°C. Total crude fiber content was calculated using the formula of ASTA (1997):
Statistical analysis was conducted on all replicates, with three replicates per treatment combination within a randomized block design. An analysis of variance (ANOVA) was performed using the “agricolae” package at a significance level of p < 0.05% in R (Version 4.3.2) to assess treatment effects. Subsequently, a post-hoc test for detailed comparisons among treatments, specifically the least significant difference (LSD) test, was conducted. To evaluate the interrelationships between various traits under both control conditions and the optimal treatment (T6), correlation plots were generated using the “corrplot” package. Principal component analysis (PCA) was additionally applied, utilizing the “factoextra” package in R, to investigate the connections between individual treatments and all the variables under study.
The colonization of adventitious roots by AM has the potential to enhance the growth and tillers of ginger. In the present study, 2 years of pooled analysis results showed that the plant height was increased in 50 g AM+ 50 g vermicompost dosage with 65.5 cm during the 90th day after planting. However, the impact of the different dosages of AM and vermicompost on growth was not statistically significant. The mean number of tillers in ginger (14; Figure 1A) was significantly higher in the AM (50 g) inoculated beds, and an increase in the dry biomass of ginger was also noticed in the 75 g AM+75 g vermicompost amended treatment (61 g; Figure 1B). Overall pooled analysis results showed that the use of vermicompost as a co-amendment with AM resulted in a lower disease incidence. Specifically, the treatments with 25 g of AM and 25 g of vermicompost, as well as 50 g of AM and 50 g of vermicompost, exhibited the lowest disease incidence rates, recording 10.5 and 10.1%, respectively (Figure 1C).
Figure 1. Effect of AM and vermicompost application on (A) number of tillers, (B) dry biomass, (C) disease incidence, and (D) yield of ginger.
Among the treatments, one with AM 50 g + and 50 g vermicompost and 75 g AM+75 g vermicompost registered significantly (p < 0.05) higher rhizome yield, and the increase was greater by 30.7 and 27.6%, respectively, compared to the control (Figure 1D).
The application of AM primarily affects mycorrhizal parameters such as spore count and mycorrhizal dependency percentage. The treatments with AM application showed a significant number of spores, ranging from 58 to 70.5 spores 50 g−1 of substrate (Figure 2A). The mycorrhizal dependency percentage is an indicator of the extent of growth transformation resulting from arbuscular mycorrhizal colonization. In ginger plants that were inoculated with various doses of AM, the mycorrhizal dependency percentage was recorded to be between 19 and 36% (Figure 2B).
Figure 2. Effect of AM and vermicompost application on (A) rhizosphere soil spore count and (B) percent mycorrhizal dependency of ginger.
The co-inoculation of arbuscular mycorrhizae and vermicompost in ginger plants resulted in a positive correlation with nutrient uptake. The macronutrient analysis report showed that all AM-inoculated treatments had a notable increase in the uptake of phosphorous, calcium, and magnesium in the ginger rhizome. Among the treatments, the 50 g AM +50 g vermicompost treatment showed the best uptake of phosphorous and calcium 0.45% (Figure 3A) and 0.08% (Figure 3B), respectively. In contrast, the uptake of magnesium was significantly greater in the treatment with 25 g of AM application (Figure 3B).
Figure 3. Effect of AM and vermicompost applications on uptake of (A) phosphorus, (B) calcium, (C) magnesium, (D) iron, and (E) zinc of ginger.
In terms of the micronutrient content of ginger rhizome, a significant amount of Fe (433.5 ppm; Figure 3C) and Zn (44 ppm; Figure 3D) was observed in 75 g AM +75 g vermicompost and 50 g AM+ 50 g vermicompost, respectively, when compared to the control. However, the Mn and Cu content of the rhizome did not show any significant differences among the treatments.
The effects of AM on all the photosynthesis gas exchange parameters were significant in ginger on 90 DAP. A significant increase in gas exchange parameters (p < 0.05) in 50 g AM +50 g vermicompost (Figure 4A)-treated plants indicated that AM enhanced the CO2 assimilation rate of ginger crops. The AM + vermicompost-treated plants showed significantly higher Pn (p < 0.05), Gs, and Tr (p < 0.05; Figure 4B). Furthermore, the photosynthetic rate (Pn) of plants treated solely with arbuscular mycorrhizal (AM) at different concentrations was significantly higher than that of plants treated solely with vermicompost at various concentrations (p < 0.05). Among the treatments, the treatment 50 g AM +50 g vermicompost exhibited the most positive effect on the efficiency of photosystem II (Fv/Fm; Figure 4C).
Figure 4. Influence of AM and vermicompost application on gas exchange measurements of ginger: (A) net photosynthetic rate, (B) stomatal conductance, and (C) chlorophyll fluorescence.
In the case of total phenolic content, treatment 6 (50 g AM+ 50 g vermicompost) was significantly higher followed by T5 (25g AM+ 25 g vermicompost) with 24.95 and 19.75 mg GAE g−1, respectively (Figure 5A). The total flavonoid content of AM-treated samples showed higher values in ginger rhizome as compared to the control. Treatment T7 which is treated with 75 g of AM and 50 g of vermicompost has shown the highest total flavonoid content of 1.705 mg quercetin equivalent g−1 among treatments (Figure 5B). In our experiment, we found that Treatment 4, Treatment 6, and Treatment 7 have given higher EO content of 40–42% compared to the control (Figure 5C). The oleoresin content was significantly higher (4%) with treatment 6 (50 g AM+ 50 g vermicompost; Figure 5D). No significant trend was observed in the fiber content upon the AM and vermicompost amendments. However, the crude fiber content was higher in the treatments T3 (50 g AM) and T9 (50 g vermicompost; Figure 5E).
Figure 5. Effect of AM and vermicompost application on quality parameters of ginger: (A) phenolics, (B) flavonoids, (C) essential oil, (D) oleoresin, and (E) crude fiber.
The correlation analysis conducted in this study revealed a significant and positive correlation among various variables when the combined application of arbuscular mycorrhizal (AM) fungi and vermicompost was employed. Specifically, the treatment with T6 (50 g AM+ 50 g vermicompost) exhibited a particularly strong positive correlation among the assessed variables, and their corresponding degree of association was given in Supplementary Table 1. These variables could include parameters related to plant growth, nutrient uptake, and quality of rhizomes. However, disease incidence and crude fiber content did not follow the general pattern of positive correlation observed in other parameters (Figure 6).
Figure 6. Correlation matrix analysis for the changes in plant growth, nutrient uptake, and quality parameters of ginger in response to AM and vermicompost application. The correlation plots represent Pearson’s r values corresponding to the size of the circle (n = 6). The color gradient from blue to red indicates the correlation between the studied variables ranging from positive to negative.
To comprehensively assess the impact of the dual application of arbuscular mycorrhizal (AM) fungi and vermicompost on ginger plants, we performed a principal component analysis (PCA) with morphological, physiological, nutrient, and quality parameters of the different treatments. The PCA revealed two principal components, collectively explaining a considerable 82.6% of the observed variability in the dataset. Of this, PC1 accounted for 72.5%, while PC2 contributed an additional 10.1% (Figure 7). PC1 had major contributions from dry biomass (8.2%), photosynthetic rate (8.0%), mycorrhizal dependency (8.0%), and lower disease incidence (7.5%). These variables all have positive contributions to PC1, which means that they are all positively correlated with each other, whereas PC2 was contributed majorly by crude fiber (52.3%). The biplot visualizes the interrelationships between variables and PCs. Closer proximity on the biplot denotes stronger correlations. For instance, dry biomass and yield are juxtaposed on the biplot, signifying a positive correlation. Similarly, most other parameters, except for disease incidence (DI) and crude fiber content (CF), cluster together, indicating positive correlations. Notably, DI’s position on the opposite side highlights its negative association with treatments T6 and T7. These treatments occupy the upper right quadrant of the PCA plot, revealing their advantageous effects relative to others. To sum up, the most favorable results in terms of plant growth parameters were observed in treatments T6 and T7, where a combination of AM fungi and vermicompost had a positive influence on dry biomass, yield, as well as the uptake of phosphorus and zinc and iron, alongside a decrease in disease incidence. It is noteworthy that treatments with higher levels of AM fungi tend to exhibit lower disease incidence in ginger, while those with higher vermicompost levels typically contain greater quantities of essential oil and oleoresin.
Figure 7. Distribution of plant growth, nutrition, and quality parameters of ginger on the biplot according to AM and vermicompost applications. DBM: dry biomass, DI: disease incidence, MD: mycorrhizal dependency, Yld: yield, P: phosphorus uptake, Fe: Fe uptake, Zn: Zn uptake, Phe: total phenolic content, Fav: flavonoids, EO: essential oil, Oleo: oleoresin, CF: crude fiber, fv.fm: chlorophyll fluorescence, Pn: photosynthetic rate, gs: stomatal conductance.
AM inoculation and amendment of vermicompost had improved their ginger plant growth, tillers, and ultimately biomass. Co-inoculation of AM (75 g) with 75 g vermicompost significantly increased the number of tillers (33%) and biomass (36%) over the uninoculated control. This is mainly because the mycorrhizal extra-radical hyphae network in the soil matrix absorbs nutrients from the surrounding soil and transports them to the plant roots in exchange for carbon (Harrier and Watson, 2004). Using vermicompost and AM-inoculated ginger plants dramatically stimulated biomass, compared to the effects of AM or vermicompost alone on the growth of ginger seedlings. This generally occurs through a substantial impact on water retention, hormone-like influences that stimulate root development, and the expansion of root hairs. Vermicompost significantly aided in the AM colonization and growth of hyphae (Paymaneh et al., 2023). As per Stoffel et al. (2021), there was a positive correlation between the effectiveness of mycorrhiza inoculant in enhancing the grain yield of soybean and the increase in biomass yield. The beneficial effect of AM inoculation on the growth and rhizome production of ginger was reported by many workers (da Silva et al., 2008; Uma et al., 2010; Samanhudi et al., 2014; Jabborova, 2022). The inoculated ginger plant roots showed evidence of structural colonization by AM, as depicted in Figure 8. The AM uninoculated samples in the morning displayed minimal spore counts, mainly attributed to the existence of an indigenous AM flora group with limited effects on growth and nutrient absorption (Pandey et al., 2020; Sarathambal et al., 2023). The level of mycorrhizal dependence represents an intrinsic characteristic of a particular crop species and significantly influences its reaction to inoculation (Urcoviche et al., 2014), and in the present study, vermicompost stimulated mycorrhizal colonization of approximately 36% in ginger roots. The extent to which crop plants depend on arbuscular mycorrhizal fungi for nutrient absorption is shaped by various root characteristics, including surface area, density and length of root hairs, growth rate, and their responses to soil conditions and root exudates. The mycorrhization parameters can be influenced by the type of materials that are added to the compost. Studies have indicated that integrating organic amendments into the soil can increase biomass, encourage mycorrhizal colonization, and stimulate the growth of AM fungal hyphae in the soil (Soussani et al., 2023).
The findings indicate a significant decrease in disease incidence as a result of the mutually beneficial interaction between AM and vermicompost in combatting pathogenic fungi such as Pythium spp. This reduction can be attributed to various biochemical processes, including the production of peroxidase and polyphenol oxidase enzymes, as well as an increase in total phenol content triggered by AM inoculation. These mechanisms ultimately enhance the host plant’s defense response against pathogens. In addition, the fungi enhanced vegetative growth, aiding plants in tolerating pathogen damage by improving nutrient uptake and root function. Bhai et al. (2012) mentioned that the co-inoculation of Glomus sp. and PGPR isolates promoted root growth in ginger without causing disease incidence. Similarly, Meng et al. (2017) revealed that ginger inoculated with Glomus versiforme exhibited the lowest disease index and the highest disease control effects.
Vermicompost, a nutrient-rich substrate, has the potential to enhance the multiplication of arbuscular mycorrhizal (AM) spores. The nutrient content of vermicompost likely promoted fungal growth, either directly or indirectly, by encouraging host root growth, mainly due to the microbiome and biomolecules associated with the vermicompost. Application of vermicompost typically increased soil mycorrhizal activity and root colonization in lentil, berseem clover, and sunflower (Koskey et al., 2023). This is attributed to its composition of beneficial microorganisms that foster both mycorrhizal growth and the development of host plants. Consequently, this promotes enhanced disease resistance in plants (Sarathambal et al., 2022). Several studies have recorded the impact of AM colonization on the defense mechanisms of host plants, including Oryza sativa, Triticum aestivum, Phaseolus vulgaris, Piper nigrum, Zingiber officinale, Solanum lycopersicum, and millets (Campos-Soriano et al., 2012; Song et al., 2015; El-Sharkawy et al., 2018; Eke et al., 2020; Bhai et al., 2021; Mythili and Ramalakshmi, 2022; Sarathambal et al., 2023).
Co inoculation of AM with vermicompost has increased ginger yields by up to 30%. The increase in the number of tillers and biomass is a result of enhanced plant nutrition and optimized photosynthesis. The increased biomass and yield resulting from the application of inoculants appear to be associated with the number of tillers per clump. The increase in yield can be attributed to the synergistic effect of arbuscular mycorrhizal (AM) activity, which enhances water and nutrient absorption (Rezaie et al., 2020). The associative action of mycorrhizal fungi in ginger has a great impact on root development and nutrient uptake, which results in the improvement of rhizome formulation. Numerous reports have highlighted the notable effect of AM fungi in enhancing the ginger yield, along with various other crops (Santos, 2010; Yunus et al., 2014; Espidkar et al., 2017; Zhu et al., 2017; Sharma et al., 2019; Wu et al., 2022).
The uptake of nutrients was positively affected by the co-inoculation of arbuscular mycorrhizae and vermicompost treatments rather than in uninoculated ginger plants. The hyphal network extends beyond the depletion zone, expanding the soil area accessible for phosphorus (P) uptake. Mycorrhizal symbiosis aids in the utilization and absorption of P. Despite being the second most vital nutrient element for plant growth and development, the uptake of phosphorus is hindered by its low mobility, limited solubility, and fixation in the soil (Lalitha et al., 2017). In contrast with Javanmardi et al. (2014), the presence of AM with vermicompost amendment did not exert a significant influence on nitrogen and potassium levels. Colonization of AM significantly (p < 0.05) increased Fe uptake. Fe uptake also significantly increased after colonization with AM and vermicompost application. Among the total Zn uptake, 24% of the Zn has been transferred through the mycorrhizal pathway (Bhantana et al., 2021). However, Mn and Cu uptake was not significantly influenced by the dual application. The augmentation of phosphorus nutrition and other mineral nutrients through the colonization of root systems by arbuscular mycorrhizal (AM) fungi varies depending on the host plant and the specific AM fungus involved (Ortas and Akpinar, 2006; Cavagnaro, 2008). Arbuscular mycorrhizal (AM) fungi enhance the mobilization of micronutrients by influencing the root morphology and physiology of the host plant, in addition to producing soil enzymes.
These findings are likely a consequence of the abundant organic material present in vermicompost, alongside the pivotal role played by arbuscular mycorrhizal (AM) fungi in metabolizing the various compounds released by plant roots (Rehman et al., 2023). The existence of organic matter in the soil can foster a robust provision of essential nutrients through mineralization. Arbuscular mycorrhizal (AM) fungi can improve the effectiveness of root surface area, facilitating more thorough exploration of the soil and overcoming water and nutrient depletion zones surrounding active root surfaces (Benaffari et al., 2022). Several studies have demonstrated that the use of AM can enhance plant nutrition in different crops, including Solanum lycopersicum L. (Kavatagi and Lakshman, 2014), Eleusine coracana L. (Patil et al., 2013), Sulla coronaria (Hidri et al., 2019), Piper nigrum (Sarathambal et al., 2023), and Triticum aestivum (Yadav et al., 2021). The introduction of arbuscular mycorrhizae (AM) holds the potential to notably elevate the concentration of diverse macro- and micronutrients, leading to increased production of photosynthates (Chen et al., 2017; Mitra et al., 2019). The increased photosynthesis rate observed in AM and vermicompost-treated samples in our study was corroborated by previous studies where AM-treated plants increased the net photosynthetic rate in black pepper (Sarathambal et al., 2022). The results of the present study agree with Ibrahim et al. (1990). Plants inoculated with arbuscular mycorrhizae (AM) experience an elevation in their photosynthetic rate attributed to the mutually beneficial carbon–phosphorus relationship established among the host and AM fungi (Allen et al., 1981; Kiers et al., 2011; Jiang et al., 2017).
The association with arbuscular mycorrhizae (AM) has been documented to boost the phyto-availability of major nutrients (Prasad et al., 2017; Li et al., 2022). Mycorrhizal inoculation markedly improved photosynthetic parameters under abiotic stress conditions (Elhindi et al., 2017; Pavithra and Yapa, 2018; Ait-El-Mokhtar et al., 2019). The enhancement in leaf gas exchange might be due to the enhanced uptake of essential nutrients and water facilitated by AM fungi (Zhu et al., 2010). Improved physiological traits indicate better photosynthetic performance and increased CO2 assimilation. Vermicompost is richer in macro- and micronutrients than other composts. Using vermicompost with arbuscular mycorrhizal fungi enhances water and nutrient uptake (N, Mg, K, Ca, and P), which are essential for stomatal opening and photosynthesis (Benaffari et al., 2022). Magnesium plays a vital role in the synthesis of chlorophyll content in leaves. In the present study, the elevation in the photosynthetic rate could be associated with an improved uptake of magnesium (Mg) and phosphorus (P) facilitated by AM (Zhu et al., 2014). In addition, the present study reported that the application of AM with vermicompost increases the uptake of K nutrients compared to control. In the pioneer study, the application of arbuscular mycorrhizae (AM) in plants has been found to enhance the uptake of potassium (K), potentially playing a crucial role in water transport through the hyphal network. This improvement contributes to enhanced hydraulic conductivity in the roots of plants treated with AM (el-Mesbahi et al., 2012).
The efficiency of PSII (Fv/Fm) was observed to be always higher in AM + vermicompost-treated plants than in untreated plants. This could be because the internal leaf temperature influences the photosystem II efficiency of ginger. This was reflected in the biomass production of these ginger. AM inoculation was advantageous to tropical crops, which consequently led to improved quantum yield of non-cyclic electrons of photosystems (Genty et al., 1989; Flagella et al., 1995). It was evident from this study that AM fungi conferred an effective function of the PSII system on ginger.
AM fungi can trigger various secondary metabolite pathways, including those involved in carotenoid, phenylpropanoid, and antioxidant production. These pathways yield compounds that serve different roles in the plant–AM fungi symbiosis, such as signaling, stress resilience, nutrient absorption, and defense against environmental challenges (Falcao et al., 2024). The arbuscular mycorrhizae (AM) and vermicompost application influenced the quality parameters of ginger rhizomes, resulting in elevated phenol (37.8%), flavonoids (35.7%), and essential oil (29%) compared to the control. Our results suggest that the increased aboveground biomass in colonized plants is likely due to enhanced nutrient absorption, as well as improved photosynthesis in AM plants. This leads to greater production of primary metabolites, which are essential precursors for the biosynthesis of phenolic compounds via the shikimic acid pathway (Duc et al., 2021). In plant–AM fungi interactions, flavonoids are crucial for initiating and limiting AM colonization. Specific flavonoids such as formononetin and ononin regulate symbiotic association by restricting AM colonization after a certain threshold. During later fungal colonization stages, flavonoids were upregulated in mycorrhizal roots (Schweiger and Müller, 2015). Moreover, inoculating with AM likely enhances the productivity of essential oil glands by elevating the levels of endogenous hormones, particularly cytokinin, indole-3-acetic acid, and gibberellin (Hazzoumi et al., 2017).
In addition, research indicates that AM can augment the content of secondary metabolites in different plant organs such as Lallemantia iberica seeds (Heydari and Pirzad, 2021), Solanum lycopersicum L. fruits (Pasković et al., 2021), and Zea mays L. leaves (Begum et al., 2019). These chemical compounds also function as bioprotectants against various plant pathogens (Ismail et al., 2011). However, in other instances, plants colonized by AM show a decrease in phenol concentration in wheat. This indicates the response of the functional compatibility of AM at the species level (Nahuelcura et al., 2022). According to da Silva et al. (2008), the inoculation of the AM fungus Gigaspora decipiens resulted in an increased yield of oleoresin in the ginger rhizome. In a study on basil, Copetta et al. (2006) noted variations in the concentration of essential oil based on the specific mycorrhizal inoculation employed. This observation aligns with studies on various host plants, including Mentha arvensis by Freitas et al. (2004), Foeniculum vulgare by Kapoor et al. (2004), and Origanum sp. by Khaosaad et al. (2006), which have also reported enhanced production of essential oils in both quantity and quality.
In summary, both PCA and correlation analyses indicated a positive impact on plant growth, nutrient absorption, and quality parameters, with the exception of disease incidence in ginger, in response to the application of arbuscular mycorrhizal (AM) fungi and vermicompost. These exceptions could imply that the dual application has improved disease resistance through the production of phenolic compounds and increased the activity of antioxidant enzymes (Sarathambal et al., 2023). The PCA results indicated that dry biomass, photosynthetic rate, mycorrhizal dependency, and disease incidence contributed more than the crude fiber. This suggests that the growth, physiological, and disease resistance characteristics may be closely associated with AM with vermicompost application in ginger particularly treatments 6 and 7 (Benaffari et al., 2022; Yilmaz, 2022). These findings underscore the synergistic benefits derived from the simultaneous use of AM fungi and vermicompost, reinforcing their potential to enhance multiple facets of ginger plant performance.
According to our findings, the positive correlation between the co-inoculation of arbuscular mycorrhizal (AM) fungi and vermicompost was associated with enhanced performance of ginger in field conditions (Figure 9). These amendments had symbiotic effects on the growth and nutrient uptake of the ginger plant when compared with the sole application. Plants inoculated with mycorrhizae exhibited elevated leaf photosynthetic parameters facilitated by enhanced uptake of essential nutrients. The application of AM with vermicompost facilitates a significant increase in the photosynthetic rate (Pn) by improving the quantum yield of the PSII system (chlorophyll fluorescence) of the ginger crop. Furthermore, co-amendment of AM with vermicompost can enhance the content of secondary metabolites in the ginger rhizome. In conclusion, the most effective and environmentally friendly treatment for enhancing the quality and quantity of rhizomes in ginger plants involved the inoculation of mycorrhizal fungi with vermicompost. Future research focuses on investigating the potential of these amendments as a promising candidate for integrated nutrient management in ginger cultivation under various agro-ecological regions. By doing so, we can gain valuable insights and a deeper understanding of the importance of incorporating these amendments into ginger-based cropping systems. Hence, the utilization of AM fungi, especially Rhizophagus sp., coupled with vermicompost, emerges as a viable strategy for promoting the eco-friendly cultivation of ginger, fostering sustainable practices while ensuring the yield of premium quality produce.
Figure 9. Graphical representation of synergistic effects of arbuscular mycorrhizal fungi and vermicompost on improving plant growth, nutrient absorption, and secondary metabolite production in ginger.
The original contributions presented in the study are included in the article/Supplementary material, further inquiries can be directed to the corresponding author.
CS: Conceptualization, Methodology, Visualization, Writing – original draft, Writing – review & editing. VS: Investigation, Methodology, Writing – original draft, Writing – review & editing. AJ: Investigation, Methodology, Writing – original draft, Writing – review & editing. RS: Investigation, Methodology, Writing – original draft, Writing – review & editing. MA: Investigation, Methodology, Writing – original draft, Writing – review & editing. MP: Investigation, Writing – original draft, Writing – review & editing. SM: Formal analysis, Writing – original draft, Writing – review & editing. PG: Investigation, Writing – original draft, Writing – review & editing. FD: Investigation, Writing – original draft, Writing – review & editing.
The author(s) declare that no financial support was received for the research, authorship, and/or publication of this article.
The authors declare that the research was conducted in the absence of any commercial or financial relationships that could be construed as a potential conflict of interest.
All claims expressed in this article are solely those of the authors and do not necessarily represent those of their affiliated organizations, or those of the publisher, the editors and the reviewers. Any product that may be evaluated in this article, or claim that may be made by its manufacturer, is not guaranteed or endorsed by the publisher.
The Supplementary material for this article can be found online at: https://www.frontiersin.org/articles/10.3389/fsufs.2024.1412610/full#supplementary-material
Ait-El-Mokhtar, M., Laouane, R. B., Anli, M., Boutasknit, A., Wahbi, S., and Meddich, A. (2019). Use of mycorrhizal fungi in improving tolerance of the date palm (Phoenix dactylifera L.) seedlings to salt stress. Sci. Hori. 253, 429–438. doi: 10.1016/j.scienta.2019.04.066
Allen, M. F., Smith, W. K., Moore, T. S., and Christensen, M. (1981). Comparative water relations and photosynthesis of mycorrhizal and nonmycorrhizal in Boutelouagracilis. New Phytol. 88, 683–693. doi: 10.1111/j.1469-8137.1981.tb01745.x
ASTA (1997). Official analytical methods of the American Spice Trade Association. (4th ed.). Englewood Cliffs, NJ: American Spice Trade Association (ASTA), Inc.
Begum, N., Qin, C., Ahanger, M. A., Raza, S., Khan, M. I., Ashraf, M., et al. (2019). Role of arbuscular mycorrhizal fungi in plant growth regulation: implications in abiotic stress tolerance. Front. Plant Sci. 10, 1–15. doi: 10.3389/fpls.2019.01068
Benaffari, W., Boutasknit, A., Anli, M., Ait-El-Mokhtar, M., Ait-Rahou, Y., Ben-Laouane, R., et al. (2022). The native Arbuscular Mycorrhizal Fungi and Vermicompost-based organic amendments enhance soil fertility, growth performance, and the drought stress tolerance of quinoa. Plan. Theory 11:393. doi: 10.3390/plants11030393
Benkebboura, A., Zoubi, B., Akachoud, O., Gholam, C., and Qaddoury, A. (2024). Field inoculation of arbuscular mycorrhizal fungi improves quality attributes and yield of melon (Cucumis melo). J. Hortic. Sci. Biotechnol. 99, 352–368. doi: 10.1080/14620316.2023.2272150
Bhai, R. S., Alex, R., Vadivukkarasi, P., and Shivakumar, M. S. (2021). Peroxidase activity as a marker to evaluate resistance in black pepper against Phytophthora infection. Indian Phytopathol. 74, 1099–1104. doi: 10.1007/s42360-021-00335-1
Bhai, R. S., Kishore, V. K., Kumar, A., Anandaraj, M., and Eapen, S. J. (2012). Screening of rhizobacterial isolates against soft rot disease of ginger (Zingiber officinale Rosc.). J Spices Aromatic Crops 14, 130–136.
Bhai, R. S., Prameela, T. P., Vincy, K., Biju, C. N., Srinivasan, V., and Babu, K. N. (2019). Soil solarization and amelioration with calcium chloride or Bacillus licheniformis - an effective integrated strategy for the management of bacterial wilt of ginger incited by Ralstoniapseudosolanacearum. Eur. J. Plant Pathol. 154, 903–917. doi: 10.1007/s10658-019-01709-y
Bhantana, P., Rana, M. S., Vista, S. P., RazanMalla, B. D. J., Timsina, G. P., and Hu, C.-X. (2021). Role of Mycorrhizal pathways in plant phosphorous and zinc uptake. Biomed J Sci & Tech Res 36, 28397–28411. doi: 10.26717/BJSTR.2021.36.005834
Birhane, E., Sterck, F., Fetene, M., Bongers, F., and Kuyper, T. (2012). Arbuscular mycorrhizal fungi enhance photosynthesis, water use efficiency, and growth of frankincense seedlings under pulsed water availability conditions. Oecologia 169, 895–904. doi: 10.1007/s00442-012-2258-3
Borkowska, B. (2002). Growth and photosynthetic activity of micropropagated strawberry plants inoculated with endomycorrhizal fungi (AMF) and growing under drought stress. Acta Physiol. Plant. 24, 365–370. doi: 10.1007/s11738-002-0031-7
Campos-Soriano, L., García-Martínez, J., and Segundo, B. S. (2012). The arbuscular mycorrhizal symbiosis promotes the systemic induction of regulatory defence-related genes in rice leaves and confers resistance to pathogen infection. Mol. Plant Pathol. 13, 579–592. doi: 10.1111/j.1364-3703.2011.00773.x
Cavagnaro, T. R. (2008). The role of arbuscularmycorrhizas in improving plant zinc nutrition under low soil zinc concentrations: a review. Plant Soil 304, 315–325. doi: 10.1007/s11104-008-9559-7
Cavagnaro, T. R. (2014). “Arbuscular Mycorrhizas and their role in plant zinc nutrition”, in Mycorrhizal fungi: use in sustainable agriculture and land restoration: Soil Biology. eds. Z. Solaiman, L. Abbott, and A. Varma. Vol 41. Berlin, Heidelberg: Springer.
Chatzistathis, T., Zoukidis, K., Vasilikiotis, C., Apostolidis, A., Giannakoula, A. E., Bountla, A., et al. (2024). Plant-growth-promoting Rhizobacteria and Arbuscular Mycorrhizal Fungi may improve soil fertility and the growth, nutrient uptake, and physiological performance of Batavia lettuce (Lactuca sativa L. var. longifolia) plants. Horticulturae 10:449. doi: 10.3390/horticulturae10050449
Chen, S., Zhao, H., Zou, C., Li, Y., Chen, Y., Wang, Z., et al. (2017). Combined inoculation with multiple arbuscular mycorrhizal fungi improves growth nutrient uptake and photosynthesis in cucumber seedlings. Front. Microbiol. 8, 25–16. doi: 10.3389/fmicb.2017.02516
Copetta, A., Lingua, G., and Berta, G. (2006). Effects of three AM fungi on growth, distribution of glandular hairs, and essential oil production in Ocimum basilicum L. var. Genovese. Mycorrhiza 16, 485–494. doi: 10.1007/s00572-006-0065-6
da Silva, MF, Pescador, R, and Rebelo, RA, Stu¨rmer SL (2008) The effect of arbuscular mycorrhizal fungal isolates on the development and oleoresin production of micropropagated Zingiber officinale. Braz. J. Plant Physiol. 20: 119–130, doi: 10.1590/S1677-04202008000200004
Declerck, S., Risede, J.-M., and Delvaux, B. (2002). Greenhouse response of micropropagated bananas inoculated with in vitro monxenically produced arbuscular mycorrhizal fungi. Sci. Hortic. 93, 301–309. doi: 10.1016/S0304-4238(01)00347-8
Duc, N. H., Vo, A. T., Haddidi, I., Daood, H., and Posta, K. (2021). Arbuscular Mycorrhizal Fungi improve tolerance of the medicinal plant Eclipta prostrata (L.) and induce Major changes in polyphenol profiles under salt stresses. Front. Plant Sci. 11:612299. doi: 10.3389/fpls.2020.612299
Eke, P., Adamou, S., Fokom, R., Nya, V. D., Fokou, P. V. T., Wakam, L. N., et al. (2020). Arbuscular mycorrhizal fungi alter antifungal potential of lemongrass essential oil against Fusarium solani, causing root rot in common bean (Phaseolus vulgaris L.). Heliyon 6:e05737. doi: 10.1016/j.heliyon.2020.e05737
Elhindi, K. M., El-Din, S. A., and Elgorban, A. M. (2017). The impact of arbuscular mycorrhizal fungi in mitigating salt-induced adverse effects in sweet basil(Ocimumbasilicum L.). Saudi J. Biol. Sci. 24, 170–179. doi: 10.1016/j.sjbs.2016.02.010
el-Mesbahi, M. N., Azcón, R., Ruiz-Lozano, J. M., and Aroca, R. (2012). Plant potassium content modifies the effects of arbuscular mycorrhizal symbiosis on root hydraulic properties in maize plants. Mycorrhiza 22, 555–564. doi: 10.1007/s00572-012-0433-3
El-Sharkawy, H. H., Rashad, Y. M., and Ibrahim, S. A. (2018). Biocontrol of stem rust disease of wheat using arbuscular mycorrhizal fungi and Trichoderma spp. Physiol. Mol. Plant Pathol. 103, 84–91. doi: 10.1016/j.pmpp.2018.05.002
Espidkar, Z., Yarnia, M., Ansari, M. H., Mirshekari, B., and Asadi, R. H. (2017). Differences in nitrogen and phosphorus uptake and yield components between barley cultivars grown under arbuscular mycorrhizal fungus and pseudomonas strains co-inoculation in rainfed condition. Appl. Ecol. Environ. Res. 15, 195–216. doi: 10.15666/aeer/1504_195216
Falcao, E. L., Qiang-Sheng, W., and da Silva, F. S. B. (2024). Arbuscular mycorrhizal fungi-mediated rhizospheric changes: what is the impact on plant secondary metabolism? Rhizosphere 30:100887. doi: 10.1016/j.rhisph.2024.100887
Figueiredo, A. F., Boy, J., and Guggenberger, G. (2021). Common mycorrhizae network: A review of the theories and mechanisms behind underground interactions. Front Fungal Biol 2:735299. doi: 10.3389/ffunb.2021.735299
Flagella, Z., Pastore, D., Campanile, R. G., and Di Fonzo, N. (1995). The quantum yield of photosynthetic electron transport evaluated by chlorophyll fluorescence as an indicator of drought tolerance in drum wheat. J. Agric. Sci. 125, 325–329. doi: 10.1017/S0021859600084823
Fortunato, I. M., Ruta, C., Castrignanò, A., and Saccardo, F. (2005). The effect of mycorrhizal symbiosis on the development of micropropagated artichokes. Sci. Hortic. 106, 472–483. doi: 10.1016/j.scienta.2005.05.006
Freitas, M. S. M., Martins, M. A., and Vieira, I. J. C. (2004). Yield and quality of essential oils of Mentha arvensis in response to inoculation with arbuscular mycorrhizal fungi Pesq. Agropec. Bras. 39, 887–894. doi: 10.1590/S0100-204X2004000900008
Genty, B., Briatntais, J. M., and Baker, N. R. (1989). The relationship between the quantum yield of photosynthesis electron transport and quenching of chlorophyll fluorescence. Biochim. Biophys. Acta 990, 87–92. doi: 10.1016/S0304-4165(89)80016-9
Gerdemann, J. W., and Nicolson, T. H. (1963). Spores of mycorrhizal Endogone species extracted from soil by wet sieving and decanting. Trans. Br. Mycol. Soc. 46, 235–244. doi: 10.1016/S0007-1536(63)80079-0
Govindjee, (1995). Sixty years since Kaustky: chlorophyll fluorescence. Aust. J. Plant Physiol. 22, 1–10.
Harrier, L. A., and Watson, C. A. (2004). The potential role of arbuscular mycorrhizal (AM) fungi in the bioprotectionofplants against soil borne pathogens in organic and/or other sustainable farming systems. Pest Manag. Sci. 60, 149–157. doi: 10.1002/ps.820
Hazzoumi, Z., Moustakime, Y., and Joutei, K. A. (2017). Effect of arbuscular mycorrhizal fungi and water stress on ultrastructural change of glandular hairs and essential oil compositions in Ocimum gratissimum. Chem. Biol. Technol. Agric. 4:20. doi: 10.1186/s40538-017-0102-z
Heydari, S., and Pirzad, A. (2021). Efficiency of Funneliformismosseae and Thiobacillus sp. on the secondary metabolites (essential oil, seed oil and mucilage) of Lallemantiaiberica under salinity stress. J. Hortic. Sci. Biotechnol. 96, 249–259. doi: 10.1080/14620316.2020.1833764
Hidri, R., Mahmoud, O. M.-B., Farhat, N., Cordero, I., Pueyo, J. J., Debez, A., et al. (2019). Arbuscular mycorrhizal fungus and rhizobacteria affect the physiology and performance of Sulla coronaria plants subjected to salt stress by mitigation of ionic imbalance. J. Plant Nutr. Soil Sci. 182, 451–462. doi: 10.1002/jpln.201800262
Hu, B., Das, P., Lv, X., Shi, M., Aa, J., Wang, K., et al. (2022). Effects of 'Healthy' fecal microbiota transplantation against the deterioration of depression in fawn-hooded rats. mSystems 7:e21822. doi: 10.1128/msystems.00218-22
Ibrahim, M. A., Campbell, W. F., Rupp, L. A., and Allen, E. B. (1990). Effects of mycorrhizae on sorghum growth, photosynthesis, and stomatal conductance under drought conditions. Arid Soil Res. Rehabil. 4, 99–107. doi: 10.1080/15324989009381237
Igiehon, N. O., and Babalola, O. O. (2018). Below-ground-above-ground plant-microbial interactions: focusing on soybean, Rhizobacteria and Mycorrhizal Fungi. Open Microbiol. J. 12, 261–279. doi: 10.2174/1874285801812010261
Ismail, Y., McCormick, S., and Hijri, M. (2011). A fungal symbiont of plant-roots modulates mycotoxin gene expression in the pathogen Fusarium sambucinum. PLoS One 6:e17990. doi: 10.1371/journal.pone.0017990
Jabborova, D. (2022). The effects of Pseudomonas koreensis IGPEB 17 and arbuscular mycorrhizal fungi on growth and physiological properties of ginger. Turk. J. Agric. For. 46, 488–495. doi: 10.55730/1300-011X.3020
Javanmardi, J., Zarei, M., and Saei, M. (2014, 2014). Influence of arbuscular mycorrhizal fungi on physiology and fruit quality of Pepino (Solanum muricatum Ait.) in vermicompost amended medium. Adv. Hortic. Sci. 28, 35–42.
Jiang, Y., Wang, W., Xie, Q., Liu, N., Liu, L., Wang, D., et al. (2017). Plants transfer lipids to sustain colonization by mutualistic mycorrhizal and parasitic fungi. Science 356, 1172–1175. doi: 10.1126/science.aam9970
Kapoor, R., Giri, B., and Mukerji, K. G. (2004). Improved growth and essential oil yield and quality in Foeniculum vulgare mill on mycorrhizal inoculation supplemented with Pfertilizer. Bioresour. Technol. 93, 3007–3311. doi: 10.1016/j.biortech.2003.10.028
Kavatagi, P. K., and Lakshman, H. C. (2014). Interaction between AMF and plant growth-promoting rhizobacteria on two varieties of Solanum lycopersicum L. World Appl. Sci. J. 32, 2054–2062. doi: 10.5829/idosi.wasj.2014.32.10.14164
Khan, A., Ding, Z. T., Ishaq, M., Khan, I., Ahmed, A. A., Khan, A. Q., et al. (2020). Applications of beneficial plant growth promoting Rhizobacteria and mycorrhizae in rhizosphere and plant growth: A review. Int. J. Agric. Biol. Eng. 13, 199–208. doi: 10.25165/j.ijabe.20201305.5762
Khaosaad, T., Vierheiling, H., Nell, M., Zitterl-Eglseer, K., and Novak, J. (2006). Arbuscular mycorrhiza alter the concentration of essential oils in oregano (Origanum sp., Lamiaceae). Mycorrhiza 16, 443–446. doi: 10.1007/s00572-006-0062-9
Kiers, E. T., Duhamel, M., Beesetty, Y., Mensah, J. A., Franken, O., Verbruggen, E., et al. (2011). Reciprocal rewards stabilize cooperation in the mycorrhizal symbiosis. Science 333, 880–882. doi: 10.1126/science.1208473
Koskey, G., Avio, L., Turrini, A., Sbrana, C., and Bàrberi, P. (2023). Biostimulatory effect of vermicompost extract enhances soil mycorrhizal activity and selectively improves crop productivity. Plant Soil 484, 183–199. doi: 10.1007/s11104-022-05783-w
Lalitha, M., Kumar, K. A., Dharumarajan, S., Balakrishnan, N., Srinivasan, R., Hedge, R., et al. (2017). “Role of vesicular-arbuscular mycorrhizae in mobilization of soil phosphorus,” in Agriculturally important microbes for sustainable. eds. V. S. Meena, et al. Springer Nature Singapore Pvt Ltd. 317.
Li, H., Zhang, T., Shaheen, S. M., Abdelrahman, H., Ali, E. F., Bolan, N., et al. (2022). Microbial inoculants and struvite improved organic matter humification and stabilized phosphorus during swine manure composting: multivariate and multiscale investigatio ns. Bioresour. Technol. 351:126976. doi: 10.1016/j.biortech.2022.126976
Locatelli, M. L., and Lovato, P. E. (2002). Inoculaçãomicorrízica e aclimatização de dois porta-enxertos de macieiramicropropagados. Pesq. Agropec. Bras. 37, 177–184. doi: 10.1590/S0100-204X2002000200009
Marsh, L., Hashem, F., and Smith, B. (2021). Organic ginger (Zingiber officinalerosc.) development in a short temperate growing season: effect of seedling transplant type and mycorrhiza application. Am. J. Plant Sci. 12, 315–328. doi: 10.4236/ajps.2021.123020
Meng, L. G., Peng, T. S., Xian, S. W., and Jin, L. R. (2017). Effects of arbuscular mycorrhizal fungi and plant growth-promoting rhizobacteria on bacterial wilt of ginger. J Fungal Res 15, 1–7.
Miguel, M. G., Nunes, S., Dandlen, S. A., Cavaco, A. M., and Antunes, M. D. (2010). Phenols and antioxidant activity of hydro-alcoholic extracts of propolis from Algarve south of Portugal. Food Chem. Toxicol. 48, 3418–3423. doi: 10.1016/j.fct.2010.09.014
Mitra, D., Navendra, U., Panneerselvam, U., Ansuman, S., Ganeshamurthy, A. N., and Divya, J. (2019). Role of mycorrhiza and its associated bacteria on plant growth promotion and nutrient management in sustainable agriculture. Int. J. Life Sci. Appl. Sci. 1, 1–10.
Mythili, M., and Ramalakshmi, A. (2022). Unravelling the distribution of AMF communities and their metabolites associated with soils of minor millets. Rhizosphere. 21:100473. doi: 10.1016/j.rhisph.2022.100473
Nahuelcura, J., Ruiz, A., Gomez, F., and Cornejo, P. (2022). The effect of arbuscular mycorrhizal fungi on the phenolic compounds profile, antioxidant activity and grain yields in wheat cultivars growing under hydric stress. J. Sci. Food Agric. 102, 407–416. doi: 10.1002/jsfa.11370
Nelson, D. W., and Sommers, L. E. (1973). Determination of total nitrogen in plant material. Agron. J. 65, 109–112. doi: 10.2134/agronj1973.00021962006500010033x
Ortas, I., and Akpinar, C. (2006). Response of kidney bean to arbuscular mycorrhizal inoculation and mycorrhizal dependency in P and Zn deficient soils, Acta Agric. Scand. Sect. B–Plant Soil Sci 56, 101–109. doi: 10.1080/09064710510029196
Pandey, R. R., Loushambam, S., and Srivastava, A. K. (2020). Arbuscular Mycorrhizal and dark septate endophyte fungal associations in two dominant ginger species of Northeast India. Proc. Natl. Acad. Sci., India, sect. B biol. Science 90, 885–894.
Pasković, I., Soldo, B., Ban, S. G., Radić, T., Lukić, M., Urlić, B., et al. (2021). Fruit quality and volatile compound composition of processing tomato as affected by fertilisation practices and arbuscular mycorrhizal fungi application. Food Chem. 359:129961. doi: 10.1016/j.foodchem.2021.129961
Patil, G. B., Lakshman, H. C., Mirdhe, R. M., and Agadi, B. S. (2013). Effect of co-inoculation of AM fungi and two beneficial microorganisms on growth and nutrient uptake of Eleusine coracana Gaertn. As. J. Plant Sci. Res. 13, 59–69. doi: 10.18052/www.scipress.com/ILNS.13.59
Pavithra, D., and Yapa, N. (2018). Arbuscular mycorrhizal fungi inoculation enhances drought stress tolerance of plants. Ground Water Sust. Dev. 7, 490–494. doi: 10.1016/j.gsd.2018.03.005
Paymaneh, Z., Sarcheshmehpour, M., Mohammadi, H., and Hesni, M. A. (2023). Vermicompost and/or compost and arbuscular mycorrhizal fungi are conducive to improving the growth of pistachio seedlings to drought stress. Appl. Soil Ecol. 182:104717. doi: 10.1016/j.apsoil.2022.104717
Pereira, J. A. P., Vieira, I. J. C., Freitas, M. S. M., Lima, T. C., and Mendonça, L. V. P. (2024). Effects of phosphorus and arbuscular mycorrhizal fungi on the quality of chili pepper fruits. J. Plant Nutr. 47, 1319–1330. doi: 10.1080/01904167.2024.2308192
Plenchette, C., Fortm, J. A., and Furlan, V. (1983). Growth response of several plant species to mycorrhizae in a soil of moderate fertility. Mycorrhizal dependency under field conditions, Plant Soil 70, 199–209.
Prasad, R., Bhola, D., Akdi, K., Cruz, C., Sairam, K. V. S. S., Tuteja, N., et al. (2017). “Introduction to mycorrhiza: historical development” in Mycorrhiza. eds. A. Varma, R. Prasad, and N. Tuteja (Cham: Springer), 1–7.
Prasath, D., Karthika, R., Habeeba, N. T., Suraby, E. J., Rosana, O. B., Shaji, A., et al. (2014). Comparison of the transcriptomes of ginger (Zingiber officinalerosc.) and mango ginger (Curcuma amadaRoxb.) in response to the bacterial wilt infection. PLoS One 9:e99731. doi: 10.1371/journal.pone.0099731
Praveena, R., Biju, C. N., and Sujatha, A. M. (2021). Deciphering primary incitant of rot complex of small cardamom plant by sequential inoculation of pathogens. Indian Phytopathol. 74, 133–143. doi: 10.1007/s42360-020-00309-9
Qiu, S., Yang, H., Zhang, S., Huang, S., Zhao, S., and Xu, X. (2023). Carbon storage in an arable soil combining field measurements, aggregate turnover modeling and climate scenarios. Catena 220:106708. doi: 10.1016/j.catena.2022.106708
Rajeev, P., and Thomas, L. (2015). Ginger-Extension Pamphlet. Kozhikode, Kerala, India: ICAR-Indian Institute of Spices Research, 12.
Ravindran, P. N., Sasikumar, B., Johnson, K. G., Ratnambal, M. J., Nirmal Babu, K., et al. (1994). Genetic resources of ginger (Zingiber officinalerosc.) and its conservation in India. Plant Genet Resour Newsl 98, 1–4.
Rehman, S. U., De Castro, F., Aprile, A., Benedetti, M., and Fanizzi, F. P. (2023). Vermicompost: enhancing plant growth and combating abiotic and biotic stress. Agronomy 13:1134. doi: 10.3390/agronomy13041134
Rezaie, M. A., Pasari, B., Mohammadi, K., Rokhzadi, A., and Karami, E. (2020). Study the effect of mycorrizal fungi, chitosan and cycocel on agronomic characteristics of rainfed chickpea. Legum. Res. 43, 546–551. doi: 10.18805/LR-509
Sadasivam, S., and Manickam, (2008). A biochemical method. 3rd Edn. New Delhi: New Age International Publishers.
Samanhudi, A. Y., Pujiasmanto, B., and Rahayu, M. (2014). Effect of organic manure and Arbuscular Mycorrhizal Fungi on growth and yield of young ginger (Zingiber officinalerosc.). IOSR J Agricul Vet Sci (IOSR-JAVS) 7, 2319–2372.
Santos, R. D. (2010). Effects of AMF and P fertilizer on post vitro growth of micropropagated Zingiber officinale R. Bras. Ci. Solo 34, 765–771. doi: 10.1590/S0100-06832010000300018
Sarathambal, C., Sivaranjani, R., Srinivasan, V., Alagupalamuthirsolai, M., Subila, K. P., and Anamika, B. (2023). Effect of arbuscular mycorrhizal inoculation on growth, mineral nutrient uptake, photosynthesis and antioxidant activities of black pepper cuttings. J. Plant Nutr. 46, 2508–2524. doi: 10.1080/01904167.2022.2160736
Sarathambal, C., Srinivasan, V., Dinesh, R., Jeevalatha, A., Prabhu, G., and Shahana, A. P. (2022). Bajra Napier hybrid: a perennial grass is an effective host for mass multiplication of Arbuscular Mycorrhizal inoculum. Range Manag Agroforestry. 43, 80–87.
Schellenbaum, L., Berta, G., Ravolanirina, F., Tisserant, B., Gianinazzi, S., and Fitter, A. H. (1991). Influence of endomycorrhizal infection on root morphology in a micropropagated woody plant species (Vitis vinifera L.). Ann. Bot. 68, 135–141. doi: 10.1093/oxfordjournals.aob.a088231
Schweiger, R., and Müller, C. (2015). Leaf metabolome in arbuscular mycorrhizal symbiosis. Curr. Opin. Plant Boil. 26, 120–126. doi: 10.1016/j.pbi.2015.06.009
Sharda, J., and Koide, R. (2010). Exploring the role of root anatomy in P-mediated control of colonization by Arbuscular Mycorrhizal Fungi. Botany 88, 165–173. doi: 10.1139/B09-105
Sharma, V., Sharma, S., Sharma, S., and Kumar, V. (2019). 2019. Synergistic effect of bio-inoculants on yield, nodulation and nutrient uptake of chickpea (Cicer arietinum L) under rainfed conditions. J. Plant Nutr. 42, 374–383. doi: 10.1080/01904167.2018.1555850
Song, Y., Chen, D., Lu, K., Sun, Z., and Zeng, R. (2015). Enhanced tomato disease resistance primed by arbuscular mycorrhizal fungus. Front. Plant Sci. 6:786. doi: 10.3389/fpls.2015.00786
Soussani, F. E., Boutasknit, A., Ben-Laouane, R., Benkirane, R., Baslam, M., and Meddich, A. (2023). Arbuscular mycorrhizal fungi and compost-based biostimulants enhance fitness, physiological responses, yield, and quality traits of drought-stressed tomato plants. Plants. 12:1856. doi: 10.3390/plants12091856
Stoffel, S. C. G., Cláudio, R. F. S. S., Edenilson, M., Paulo, E. L., and Admir, J. G. (2021). Yield increase of soybean inoculated with a commercial arbuscular mycorrhizal inoculant in Brazil. Afr. J. Agric. Res. 16, 702–713. doi: 10.5897/AJAR2020.14766
Strasser, R. J., Srivastava, A., and Govindjee, (1995). Polyphasic chlorophyll a fluorescence transient in plants and cyanobacteria. Photochem. Photobiol. 61, 32–42. doi: 10.1111/j.1751-1097.1995.tb09240.x
Taber, R. A., and Trappe, J. M. (1982). Vesicular-arbuscular mycorrhiza in rhizomes, scale-like leaves, roots, and xylem of ginger. Mycologia 74, 156–161. doi: 10.1080/00275514.1982.12021485
Thomas, G. W. (1982). “Exchangable cations: Methods of soil analysis, part 2, chemical and microbiological properties” in Agronomy. ed. A. L. Page (WI, USA: Madison), 159–165.
Uma, E., Muthukumar, T., Sathiyadash, K., and Muniappan, V. (2010). Mycorrhizal and dark septate fungal associations in gingers and spiral gingers. Botany 88, 500–511. doi: 10.1139/B10-021
Urcoviche, R. C., Castelli, M., and Gimenes, R. M. T. (2014). Spore density and diversity of arbuscular mycorrhizal fungi in medicinal and seasoning plant. Afr. J. Agric. Res. 9, 1244–1251. doi: 10.5897/AJAR2013.8025
Vosátka, M., and Gryndler, M. (2000). Response of micropropagated potatoes transplanted to peat media to post-vitro inoculation with arbuscular mycorrhizal fungi and soil bacteria. Appl. Soil Ecol. 15, 145–152. doi: 10.1016/S0929-1393(00)00090-1
Wang, Z., Liu, T., Yang, G., and Zhao, S. (2024). Preparation and research on cationic modified vermiculite with strong adsorption capacity for mineralizing bacteria. Mater. Lett. 363:136313. doi: 10.1016/j.matlet.2024.136313
Wu, Q., Li, G., and Zou, Y. (2011). Roles of Arbuscular Mycorrhizal Fungi on growth and nutrient Acquisition of Peach (Prunus Persica L. Batsch) seedlings. J. Anim. Plant Sci. 21, 746–750.
Wu, S., Shi, Z., Chen, X., Gao, J., and Wang, X. (2022). Arbuscular mycorrhizal fungi increase crop yields by improving biomass under rainfed condition: A meta-analysis. Peer J 10:e12861. doi: 10.7717/peerj.12861
Xu, Q., Zhang, T., Niu, Y., Mukherjee, S., Abou-Elwafa, S. F., Nguyen, N. S., et al. (2024). A comprehensive review on agricultural waste utilization through sustainable conversion techniques, with a focus on the additives effect on the fate of phosphorus and toxic elements during composting process. Sci. Total Environ. 942:173567. doi: 10.1016/j.scitotenv.2024.173567
Yadav, R., Ror, P., Rathore, P., Kumar, S., and Ramakrishna, W. (2021). Bacillus subtilis CP4, isolated from native soil in combination with arbuscular mycorrhizal fungi promotes biofortification, yield and metabolite production in wheat under field conditions. J. Appl. Microbiol. 131, 339–359. doi: 10.1111/jam.14951
Yilmaz, A. (2022). Karik U.AMF and PGPR enhance yield and secondary metabolite profile of basil (Ocimum basilicum L.). Ind. Crop. Prod. 176:114327. doi: 10.1016/j.indcrop.2021.114327
Yunus, S. A., Pujiasmanto, B., and Rahayu, M. (2014). Effect of organic manure and AMF on growth and yield of young ginger. J. Agric. Vet. Sci. 7, 1–5. doi: 10.9790/2380-07540105
Zhang, T., Li, H., Yan, T., Shaheen, S. M., Niu, Y., and Xie, S. (2023). Organic matter stabilization and phosphorus activation during vegetable waste composting: multivariate and multiscale investigation. Sci. Total Environ. 891:164608. doi: 10.1016/j.scitotenv.2023.164608
Zhu, Y., Lv, G. C., Chen, Y. L., Gong, X. F., Peng, Y. N., Wang, Z. Y., et al. (2017). Inoculation of arbuscular mycorrhizal fungi with plastic mulching in rainfed wheat: A promising farming strategy. Field Crop Res. 204, 229–241. doi: 10.1016/j.fcr.2016.11.005
Zhu, X. Q., Wang, C. Y., Chen, H., and Tang, M. (2014). Effects of arbuscular mycorrhizal fungi on photosynthesis, carbon content, and calorific value of black locust seedlings. Photosynthetica 52, 247–252. doi: 10.1007/s11099-014-0031-z
Keywords: mycorrhizal colonization, photosynthetic rate, nutrient uptake, rhizome quality, yield
Citation: Sarathambal C, Srinivasan V, Jeevalatha A, Sivaranjani R, Alagupalamuthirsolai M, Peeran MF, Mukesh Sankar S, George P and Dilkush F (2024) Unravelling the synergistic effects of arbuscular mycorrhizal fungi and vermicompost on improving plant growth,nutrient absorption, and secondary metabolite production in ginger (Zingiber officinale Rosc.). Front. Sustain. Food Syst. 8:1412610. doi: 10.3389/fsufs.2024.1412610
Received: 05 April 2024; Accepted: 04 July 2024;
Published: 25 July 2024.
Edited by:
Muhammad Saqlain Zaheer, Khwaja Fareed University of Engineering and Information Technology (KFUEIT), PakistanReviewed by:
Marika Pellegrini, University of L’Aquila, ItalyCopyright © 2024 Sarathambal, Srinivasan, Jeevalatha, Sivaranjani, Alagupalamuthirsolai, Peeran, Mukesh Sankar, George and Dilkush. This is an open-access article distributed under the terms of the Creative Commons Attribution License (CC BY). The use, distribution or reproduction in other forums is permitted, provided the original author(s) and the copyright owner(s) are credited and that the original publication in this journal is cited, in accordance with accepted academic practice. No use, distribution or reproduction is permitted which does not comply with these terms.
*Correspondence: C. Sarathambal, c2FyYXRoYTZAZ21haWwuY29t
†Present Addresses: A. Jeevalatha and M. Alagupalamuthirsolai, ICAR Sugarcane Breeding Institute, Coimbatore, Tamil Nadu, India
Disclaimer: All claims expressed in this article are solely those of the authors and do not necessarily represent those of their affiliated organizations, or those of the publisher, the editors and the reviewers. Any product that may be evaluated in this article or claim that may be made by its manufacturer is not guaranteed or endorsed by the publisher.
Research integrity at Frontiers
Learn more about the work of our research integrity team to safeguard the quality of each article we publish.