- 1Department of Fisheries, Faculty of Fisheries, University of Rajshahi, Rajshahi, Bangladesh
- 2Department of Oceanography and Blue Economy, Faculty of Fisheries, Hobiganj Agricultural University, Hobiganj, Bangladesh
- 3Bangamata Sheikh Fojilatunnesa Mujib Science & Technology University, Jamalpur, Bangladesh
- 4Environmental Studies Department, University of California, Santa Cruz, CA, United States
- 5Environmental and Life Sciences Programme, Faculty of Science, Universiti Brunei Darussalam, Gadong, Brunei Darussalam
- 6Department of Zoology, College of Science, King Saud University, Riyadh, Saudi Arabia
- 7Department of Fisheries and Marine Science, Noakhali Science and Technology University, Noakhali, Bangladesh
- 8School of Engineering and Built Environment, Griffith University, Brisbane, QLD, Australia
Nano-sized Zn particles are recognized for its ability to elevate fish health and well-being, enhancing antimicrobial and antioxidant capacities and reinforcing the immune system. In the present study we explored the effects of Zn-NPs supplementation on augmenting the growth performance and nutritional indicators and physiological aspects of the catfish, Clarias batrachus. Zn-NPs were prepared by heating the mixture of [Zn(CH3COO)2.2H2O], ethylene glycol and polymer surfactant (PVP) at 70°C for 45 min using an oil bath heater. The resultant solution was centrifuged at 6000 rpm on a heated plate at 70°C until the precipitation of Zn-NPs was achieved. Crystal structure, growth mechanisms and shapes of Zn-NPs were characterized by the atomic force microscopy. Produced Zn-NPs was characterized by spectrophotometer and the zeta potential and mean size was recorded as −32.5 ± 1.0 mV and 14.7 ± 2.5 nm, respectively. The experiment comprised six distinct test diets, categorized by the incorporation of Zn-NPs at levels of 0, 10, 20, 30, 40, and 50 mg/kg into the feed where the initial diet was employed as a control group, devoid of any Zn-NPs supplementation. Three glass aquariums were employed for each diet. In each aquarium, 12 fish with an initial mean weight of 5.23 ± 0.06 g were stocked. The fish underwent experimental feeding for 60 days, with the feed amount set at 3% of their body weight. After the experimental period, growth performance (weight gain, length gain, average daily gain, specific growth rate, thermal growth co-efficient, condition factor and survival rate), nutritional indices (feed conversion ratio, protein efficiency ratio, annual net protein utilization), proximate composition of muscle (protein, lipid, carbohydrate, ash and moisture), hematological parameters (RBC, WBC, hemoglobin, hematocrit, MCV, MCH, MCHC, total protein, albumin and globulin), serum lipid and enzymatic parameters (total cholesterol, HDL, LDL, triglycerides, ALP, AST, ALT, amylase, lipase and protease), and bioaccumulation of Zn in different organs of fish were estimated following standard methods. Growth analysis revealed that C. batrachus fed with Zn-NPs at 40 mg/kg of feed had significantly (p < 0.05) improved growth performance (WG = 18.87 ± 0.91 g, SGR = 2.52 ± 0.06% g/day, TGC = 1.09 ± 0.05 and CF = 1.47 ± 0.12), nutritional indices (FCR = 1.35 ± 0.06, PER = 2.24 ± 0.10 and ANPU = 41.46 ± 2.56%), hematological parameters, and enzymatic activity compared to control and other groups. The optimal dietary supplementation of Zn-NPs for final weight, weight gain and specific growth rate of C. batrachus was estimated to be ranged between 30.4 and 30.5 mg/kg per diet, respectively.
1 Introduction
Aquaculture, a rapidly expanding agricultural sector, plays a vital role in meeting global demand for animal protein while maintaining symbiotic relationships with the environment (Moss et al., 2019; FAO, 2020). Despite aquaculture playing a crucial role in ensuring food security and providing employment opportunities for millions of people around the world, the sector is confronted with various challenges. These include water pollution, disease outbreaks, climate change impacts, and a lack of technical knowledge. Bangladesh, currently ranked third globally in aquaculture, has been witnessing an impressive annual growth rate of about 45% in fish production (Moller et al., 2023), while concurrently encountering similar challenges in the industry. Thus, the adoption and application of new technologies are crucial for addressing these multifaceted issues and ensuring the sustainability, growth, and resilience of the aquaculture sector. Incorporating advanced technologies into fish feed can revolutionize aquaculture, meeting rising demands for seafood, improving food security, and promoting sustainable practices on a global scale.
The production of fish in aquaculture is significantly influenced by two key factors: feed quality and water conditions (Moller et al., 2023). Optimal feed composition ensures proper nutrition while maintaining water quality is crucial for providing a conducive environment for fish growth and survival (Hossain et al., 2022; Jewel et al., 2023; Akter et al., 2024). Because these two variables directly impact the health, growth, and overall performance of farmed fish. The integration of nanoparticles (any particle <100 nm, e.g., ZnO and CuO) containing essential trace elements holds promise for enhancing the growth and development of fish (Umalatha Sridhar et al., 2016; Fasil et al., 2019; Kumar et al., 2023; Ramana et al., 2023) and maintaining good water quality.
Nanoparticles have been shown to have a substantial impact on increasing growth and immunological factors in fish (Kumar et al., 2022, 2023, 2024). Currently, there is a substantial body of published material that provides a thorough review of the many uses of nanoparticle in aquaculture (Luis et al., 2017; Masoomi Dezfooli et al., 2018; Shah and Mraz, 2020; Jewel et al., 2023). Nutrients in Nanoform are attributed to their enhanced bioavailability and efficient absorption through tiny cellular structures. This allows for the encapsulation of traditional bulk minerals into nanoparticle forms, addressing issues such as expense and residue buildup (Karthikeyan et al., 2020). However, it is essential to consider potential risks associated with excessive Zn-NPs concentrations, as they can lead to acute toxicity in fish (Kumar et al., 2020).
Zinc nanoparticles (Zn-NPs) are widely recognized for their effectiveness in enhancing fish health and growth (Wang et al., 2008). They offer various benefits, including environmentally sustainable properties and diverse applications such as anticancer and antimicrobial activities (Jiang et al., 2018). The utilization of zinc oxide nanoparticles (Zn-NPs) as a dietary supplement for various fish and aquatic species has garnered significant attention in scientific research (Faiz et al., 2015; Kumar et al., 2022, 2023, 2024). Studies have illustrated that different concentrations of Zn-NPs can elicit varied effects on fish health and growth, contingent upon the species and their nutritional requirements. For instance, Kumar et al. (2018) showcased that a dosage of 20 mg/kg of Zn-NPs enhanced growth performance, mitigated oxidative stress, and bolstered immunological responses in Pangasius hypophthalmus, while Faiz et al. (2015) observed that a concentration of 30 mg/kg augmented growth and improved hematological parameters in grass carp, Ctenopharyngodon idella. Similarly, Sallama et al. (2020) reported significant growth enhancement and improved protein utilization in Siganus rivulatus with the supplementation of 30 mg/kg ZnO-NPs, while Thangapandiyan and Monika (2019) noted highest growth and metabolic function in Labeo rohita with a diet supplemented with 10 mg/kg ZnO-NPs. Furthermore, the incorporation of ZnO-NPs into diets has demonstrated enhanced heat tolerance in Pangasius hypophthalmus exposed to lead contamination, offering protection against oxidative and cellular metabolic stress (Kumar et al., 2017).
The Asian Catfish, Clarias batrachus, is readily found and bred in the ditches, ponds, lakes, and rivers of Bangladesh. It exhibits a strong resistance to several environmental stresses, rendering it very adaptable to changes in water quality and climate (Ali et al., 2013). Moreover, the species exhibits a rapid development rate and possesses a high capacity for reproduction, rendering it economically feasible for aquaculture production. In addition, Asian Catfish is widely consumed and socially embraced as a food fish in Bangladesh, resulting in a consistent market demand. The fish is a valuable and protein-dense food source that plays a crucial part in fulfilling the nutritional requirements of the Bangladeshi population (Ali et al., 2013). Moreover, the cultivation of this crop offers employment prospects for a multitude of small-scale farmers, so aiding in the reduction of poverty and the advancement of rural areas. Through the promotion of sustainable techniques, such as effective water management and appropriate feed utilization. Nevertheless, the cultivation of C. batrachus, in Bangladesh has numerous challenges. The factors of feed quality, availability, and cost have an impact on the growth and performance of catfish farms (Ahmed et al., 2010). Again, intensive catfish farming practices can lead to environmental difficulties such as water pollution and habitat destruction. Therefore, it is necessary to implement sustainable management approaches to address these issues. To tackle these issues, extensive research is needed to analyze the growth and production performances of the fish, while minimizing costs and maximizing benefits. Previous research has concentrated on determining the best amounts of inclusion for regionally accessible ingredients (mustard oil cake, fishmeal, and soybean meal) in catfish diets in order to prepare affordable, nutritionally balanced feeds. However, research on the utilization of Zn-NPs in Bangladesh remains limited, with no studies found on the effects of dietary Zn-NPs specifically on freshwater catfish species like Clarias batrachus (Linnaeus, 1758). Based on the established function of zinc in multiple physiological functions and the possibility of increasing its bioavailability via nanoparticle forms, we hypothesized that adding Zn-NPs to the diet will improve C. batrachus growth, survival rates, physiological parameters, and nutritional values. In particular, we anticipated that fish fed with Zn-NP supplemented diets would show higher growth rates, higher survival rates, changes in physiological biomarkers indicating better health and stress tolerance, and improvements in nutritional parameters like protein content, lipid profile, and mineral composition in comparison to fish fed control diets. Therefore, this study aimed to explore the effects of dietary Zn-NPs on the growth performance, survival, physiological parameters, and nutritional values of C. batrachus. Understanding these effects is pivotal for optimizing fish culture techniques, ensuring food security, and promoting sustainable aquaculture practices on a global scale.
2 Materials and methods
2.1 Synthesizing and characterization of Zn-NPs
The synthesis of zinc nanoparticles (Zn-NPs) followed the procedure by Alam et al. (2015) with modifications. Liquid phase synthesis was conducted using an acoustic method. In this approach, Zinc acetate ([Zn(CH3COO)2.2H2O], 99.99%) and ethylene glycol (EG, (CH2OH)2, 99.8%) were sourced from Sigma Aldrich (Merck KGaA, Darmstadt, Germany). Polyvinylpyrrolidone (PVP(C6H9NO)n, 99.99%) was obtained from AcrosOrganics BVBA (Belgium). All chemicals were employed as received without additional purification. A mixture of 1.75 mmol [Zn(CH3COO)2.2H2O], 20 mL EG and 2% w/v polymer surfactant (PVP) were dissolved in 25 mL of water and heated at 70°C for 45 min using an oil bath heater (Oil Bath Digital (13 L) SH-WB-10GDH SH Scientific Korea). The nanoparticles that were produced underwent purification through a precipitation technique. The solutions were mixed and magnetically centrifugated at 6000 rpm (Centrifuge Machine 6,800 rpm 8 × 15 mL RB / 4 × 15 mL Tube Z 207 A HERMLE Germany) on a heated plate at 70°C until white Zn-NPs precipitates appeared. For acetate removal, the precipitate was washed multiple times and dried overnight in a 60°C electric oven. The manipulation of variables such as reagent surfactant concentrations (e.g., PVP), precursor materials, solvents (EG or other polyols), gas introduction, temperatures, and heating rates facilitated the production of desired nanostructures in high yields (Alam et al., 2015). The atomic force microscope (AFM-Park Systems, XE-70, South Korea) was utilized to examine the crystal structures and growth mechanisms of the nanoparticles, as well as to analyze their shapes. For AFM measurements, samples were prepared by placing a drop of the colloidal solution onto glass slides. The Zn-NPs produced in the present study are mostly needle-shaped nanorods in morphology. The characterization of Zn-NPs was performed using a spectrophotometer (Varian Cary 6000i, Santa Clara, CA, United States) at 300–500 nm which results a peak of 260–380 nm. The Zeta potential was measured by using the Zetasizer Nano ZS (Malvern Instruments Ltd., GB). Zeta potential and mean size of the produced Zn-NPs in the present study was −32.5 ± 1.0 mV and 14.7 ± 2.5 nm, respectively.
2.2 Feed formulation
Fish feed was formulated by combining locally available ingredients like fish meal, mustard oil cake, soybean meal, maize bran, wheat bran, rice bran, and soybean oil. The feed was enhanced with minerals and a vitamin pre-mixture devoid of zinc. All components were ground, sifted, weighed, and meticulously blended to create the final diet. The base feed was mixed with synthesized Zn-NPs in five different levels (10, 20, 30, 40, and 50 mg/kg of dry feed). This mixture was homogenized with water until it reached a dough-like consistency, followed by manual pelletization using a 3 mm diameter die. Once the moisture content dropped below 10%, the resultant pellets were dried in a thermostatic oven (Microsil, India). The formulated diets were stored at 20°C until employed for the experiment. The feed ingredient ratios and the nutritional composition of the prepared diet can be found in Table 1.
2.3 Experimental design and feeding trials
The investigation was conducted by the University’s guidelines for using experimental animals in research. A total of 216 C. batrachus individuals with an average body weight of 5.23 ± 0.06 g (mean ± SEM) were obtained from a fish seed hatchery, and transported in an aerated plastic sack to the laboratory at the University of Rajshahi, Bangladesh. The fish were gradually introduced to the experimental basal diet over a week-long period in a circular cement tank, with water continuously flowing. Following the acclimatization phase, the fish were randomly allocated into 18 glass tanks, with 12 fish per tank, which included three tanks for control purposes. Each tank had dimensions of 1.5 m × 0.8 m × 0.8 m and water capacities of 500 L. Six different concentrations of Zn-NPs were tested: 10, 20, 30, 40, and 50 mg/kg, alongside a control group (0 mg/kg). These concentrations were applied to separate tanks, with each concentration having three replicate tanks. The fish were manually fed twice daily using the experimental diets, at a rate corresponding to 3% of their body weight per day. The experiment ran for 60 days, during which regular siphoning was carried out to remove leftover food particles from each aquarium after feeding. Throughout the culture period, daily water exchange occurred in all tanks, with about half of the water volume replaced by pre-treated water. Monitoring of physical and chemical factors (Temperature, °C; DO, mg/L, pH, and ammonia, mg/L) took place every 15 days. Temperature was gaged using a Celsius thermometer, dissolved oxygen with a HACH kit (FF-2, United States), and pH using a Jenwary 3,020 pH meter, United Kingdom. The recorded ranges for dissolved oxygen, water temperature, pH, and ammonia were 5.87–6.07 mg/L, 27.76–28.93°C, 6.97–7.16, and 0.001–0.002 mg/L, correspondingly. The initial zinc concentration of the water used in the aquariums was measured using atomic absorption spectrophotometry (Shimadzu, AA-6800). The level measured was 0.18 ± 0.08 mg/L (Figure 1).
2.4 Sampling and measurement of fish specimens
Fish sampling was conducted at a fixed interval of 15 days. All the individuals from each aquarium were used for the measurement of growth parameters on each sampling day. The precise weight and length of the entire body were determined using an electronic balance and a digital slide caliper, accurate to 0.1 g and 0.1 cm, respectively. The initial fish biomass was established at the start of the feeding trial and then re-evaluated at the trial’s conclusion.
2.5 Growth parameters and nutritional indices
Upon completion of the feeding trial, growth indicators [average daily gain, weight gain, specific growth rate (SGR), survival rate (SR), condition factor (CF), thermal growth co-efficient (TGC), as well as nutritional metrics (feed conversion ratio (FCR), protein efficiency ratio (PER), and apparent net protein utilization (ANPU)] were computed using the following established equations (Nordgarden et al., 2003; Mohanty, 2015):
2.6 Proximate composition of fish muscle
The diets and fish muscles were assessed for their proximate compositions, which encompassed protein, lipid, carbohydrate, ash, and moisture (AOAC, 2016). Proximate composition analysis was conducted both at the beginning and the end of the fish harvest. For determining crude protein, the Kjeldahl method (JEQ-16B, India) was employed, while crude lipid was measured using the Soxhlet method (LABORATE, LT-SOXA001, India) involving petroleum ether extraction. Samples were heated to 550°C in a muffle furnace (SH-FU-5MG SH Scientific Korea) for 5 h to determine the amount of ash present. Moisture levels were determined by consistently weighing samples subjected to oven drying at temperatures of 70°C and 105°C, respectively.
2.7 Collection and processing of blood sample
Toward the experiment’s end, 15 fish from each feeding group were randomly chosen for analysis of proximate composition, including protein, lipid, ash, and moisture. Anesthesia was induced in 15 fish per treatment group using clove oil (Merck, Germany) at a concentration of 50 μL per L of water, for blood sampling. A 2.0-ml hypodermal syringe was used to draw blood samples from the caudal vein. About 500–600 μL of blood were divided into two vials; one vial contained EDTA as an anticoagulant, while the other did not for serum collection. After gentle agitation, the EDTA-coated vial prevented clotting and hemolysis. Serum was obtained by collecting blood without anticoagulant, followed by centrifugation at 2000 g for 15 min at 4°C. The resulting serum was stored at −20°C. From the same fish, the liver and muscles were dissected, washed in distilled water, and preserved in 10% formalin.
2.8 Analysis of hematological parameters
Before assessing total erythrocyte (RBC) and leukocyte (WBC) counts, blood samples were diluted using a mixture of Turk’s solution and Toisson’s solution. The quantification was performed using a Neubauer hemocytometer, as outlined by Behera et al. (2014). The calculation of cell counts followed the method detailed in Parida et al. (2011). For RBCs, the formula was: Total RBCs per mm3 = 20,050 N / 10,000 N (N = number of RBCs counted, dilution factor = 200). For WBCs, the formula was: Total WBCs per mm3 = (201 L * 0.4) / 50 L (L = number of WBCs counted, dilution factor = 50). Hemoglobin concentration was determined via the cyanomethemoglobin method using Drabkin’s solution (Drabkin, 1950). Hematocrit was measured through the standard micro-hematocrit technique (Bull et al., 2000). Utilizing micro-hematocrit tubes and formulas from Dacie and Lewis (1999), parameters including Mean Corpuscular Hemoglobin (MCH), Mean Corpuscular Volume (MCV), and Mean Corpuscular Hemoglobin Concentration (MCHC) were determined. Total protein and albumin levels were assessed spectrophotometrically, while globulin levels were derived by subtracting albumin from total protein.
2.9 Lipid profile and enzymatic analysis
Atomic absorption spectrophotometry employing Crest Biosystems® kits was utilized to gage total cholesterol, triglycerides, low-density lipoprotein (LDL), high-density lipoprotein (HDL), aspartate aminotransferase (AST), alanine aminotransferase (ALT), and alkaline phosphatase (ALP) levels in the blood. Assessment of serum amylase, lipase, and protease activity was conducted via a colorimetric enzymatic approach.
2.10 Bioaccumulation of Zn
After eliminating formalin using filter paper, preserved liver and muscle samples were freeze-dried. Quantification of zinc uptake from zinc nanoparticles (Zn-NPs) in liver, muscle, and serum was conducted through inductively coupled plasma mass spectrometry (ICP-MS) analysis, following the method of Shahzad et al. (2017). A mixture of 10 mL concentrated nitric acid and 2 mL perchloric acid was applied to 1 g freeze-dried samples. Heat treatment at 100°C on a hot plate initiated the digestion process. The digestion process involved the addition of two drops of hydrogen peroxide, followed by dilution using distilled water and filtration using a Whatman filter paper. Zinc concentration was reported as μg/g. Hot plate was used as it was cost-effective and readily available alternative at our lab. At the time of our study, microwave equipment was not accessible. While microwave digestion offers advantages in terms of speed and uniformity, the analysis method itself may not be sensitive to any differences resulting from the use of a hot plate. Furthermore, the choice between hot plate and microwave digestion may depend on factors such as sample size and type. Hot plates are often preferred for larger sample volumes or samples not requiring rapid digestion, whereas microwave systems are commonly used for smaller sample sizes or materials necessitating rapid digestion processes.
2.11 Statistical analysis
All of the data were presented in Mean ± S.E. format. The Shapiro–Wilk test was used to evaluate the normality of the data. The untransformed form of the data was examined for treatment comparisons, except for percentage and ratio statistics. Before analysis, percentage and ratio data underwent an arc-sign transformation. One-way analysis of variance (ANOVA) using SPSS 25 ver. Was used to examine the impact of various Zn-NPs levels on growth metrics, nutritional indices, hematological parameters, serum lipid profile, and enzymatic parameters. Duncan’s multiple range test (DMRT) was used for mean comparison when significant F values appeared at a 5% probability level.
3 Results
3.1 Growth performance
The growth performance of C. batrachus fed on varying levels of Zn-NPs is summarized in Table 2. Initially, there were no significant differences in the initial body weight and length of the fish, with weights ranging from 5.21 ± 0.09 to 5.25 ± 0.07 g and lengths ranging from 7.38 ± 0.12 to 7.74 ± 0.72 cm. After being fed a diet supplemented with Zn-NPs, C. batrachus exhibited significantly enhanced growth performance across various parameters. A concentration-dependent enhancement in growth was observed, with the 40 mg/kg Zn-NPs supplemented diet whereas FW and WG showed 42.74 and 54.05%, respectively increment compared to control group. However, the 50 mg/kg Zn-NPs supplemented diet exhibited poorer performance across these parameters. Importantly, there was no significant effect of Zn-NPs supplemented diet on the survival rate of C. batrachus among the experimental groups. Polynomial regression analysis revealed a relationship between FW, WG, and SGR against dietary Zn-NPs levels, estimating the optimal dietary supplementation of Zn-NPs for C. batrachus to be between 30.39 to 30.52 mg/kg per diet, respectively (Figure 2).
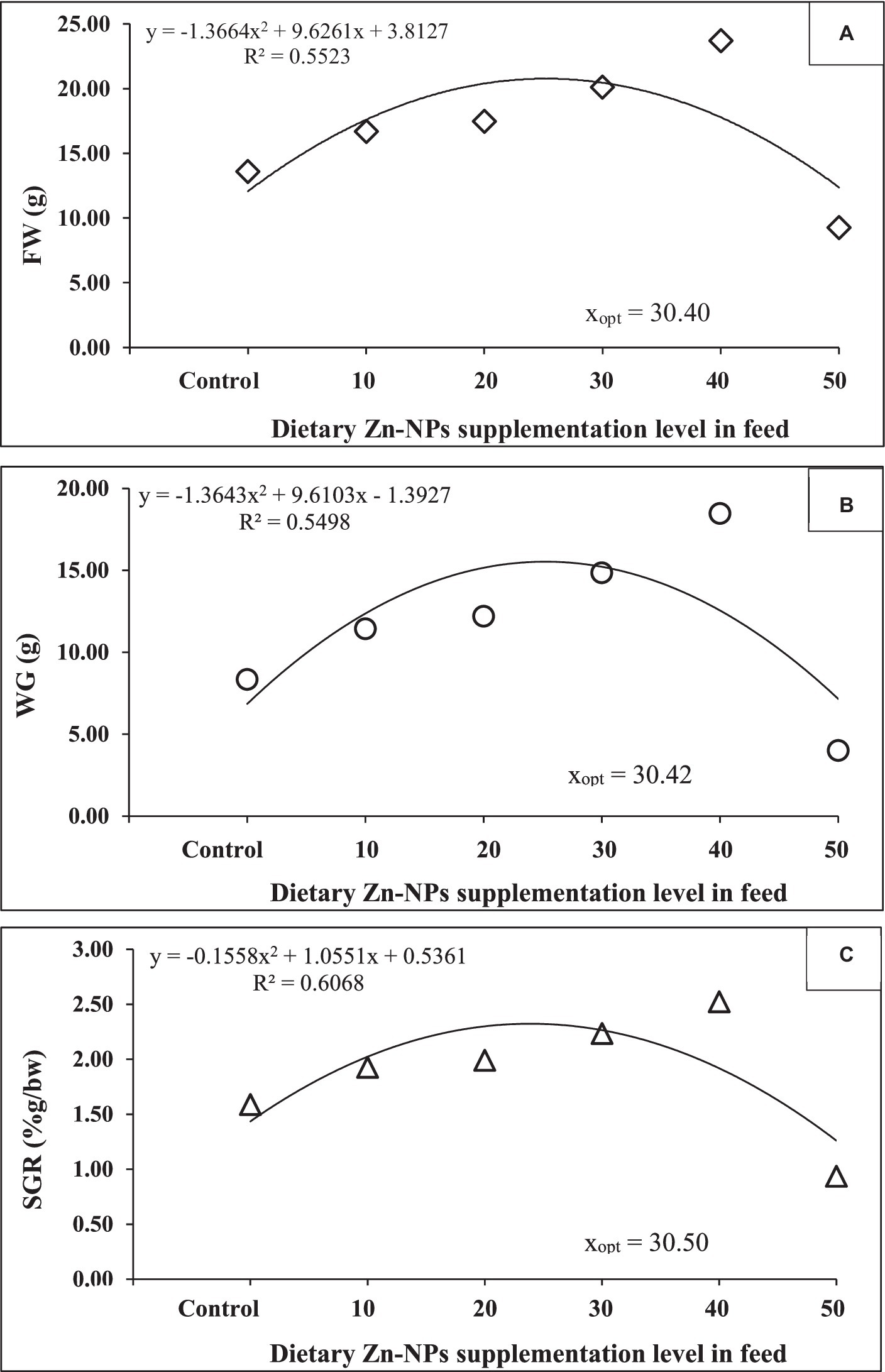
Figure 2. The relationship between (A) final weight (g), (B) weight gain (g) and (C) specific growth rate (SGR; %g/day), of C. batrachus fed diets supplemented with varying levels of Zn-NPs for 60 days.
3.2 Nutritional indices
The results of the feed utilization parameters, including feed consumed, feed conversion ratio (FCR), protein efficiency ratio (PER), and apparent net protein utilization (ANPU), are furnished and presented in Table 1. At the initial stage, no significant differences were observed in the amount of feed consumed by the experimental groups, indicating comparable feeding behavior among the fish. However, significant concentration-dependent variations were noted among the experimental groups for FCR, PER, and ANPU. The FCR values ranged from 1.35 ± 0.06 to 6.81 ± 2.59, with the most improved performance observed in the 40 mg/kg Zn-NPs supplemented group and the poorest performance recorded in the 50 mg/kg Zn-NPs supplemented group. FCR showed an improvement of 55.44% compared to the control group. This suggests that at 40 mg/kg Zn-NPs supplementation, the fish utilized the feed more efficiently compared to other concentrations. Similarly, PER and ANPU exhibited considerably better performance in the 40 mg/kg Zn-NPs supplemented group, with values ranging from 0.48 ± 0.15 to 2.24 ± 0.10 for PER and 9.82 ± 1.84 to 41.46 ± 2.65 for ANPU. These results indicate that the fish in the 40 mg/kg Zn-NPs supplemented group utilized dietary protein more effectively compared to other groups. Conversely, significantly lower values for all feed utilization parameters were recorded in the 50 mg/kg Zn-NPs supplemented group, even lower than those of the control group. This suggests that supplementation with 50 mg/kg Zn-NPs negatively impacted feed utilization efficiency, resulting in poorer performance compared to other experimental groups.
3.3 Proximate composition
The whole-body proximate composition exhibited significant differences among the feeding groups (Figure 3). Protein, lipid, and ash content demonstrated an increasing trend with the elevation of Zn-NPs concentrations, particularly up to a concentration of 40 mg/kg Zn-NPs. However, the fish group fed with 50 mg/kg Zn-NPs exhibited significantly reduced levels of protein, lipid, and ash compared to both the control group and the other feeding groups. In contrast to these parameters, carbohydrate content decreased significantly with increasing Zn-NPs concentration, albeit showing a slight increase at the 50 mg/kg Zn-NPs concentration. The lowest and maximum moisture content values were observed at 40 mg/kg and 30 mg/kg Zn-NPs concentrations, respectively. Moisture content displayed irregular fluctuations with varying concentrations of Zn-NPs. These findings suggest that Zn-NPs supplementation influenced the proximate composition of the whole body, with notable changes in protein, lipid, ash, carbohydrate, and moisture content. The most significant alterations were observed at the 40 mg/kg Zn-NPs concentration, while the 50 mg/kg Zn-NPs concentration resulted in contrasting effects, particularly in protein, lipid, and ash content.
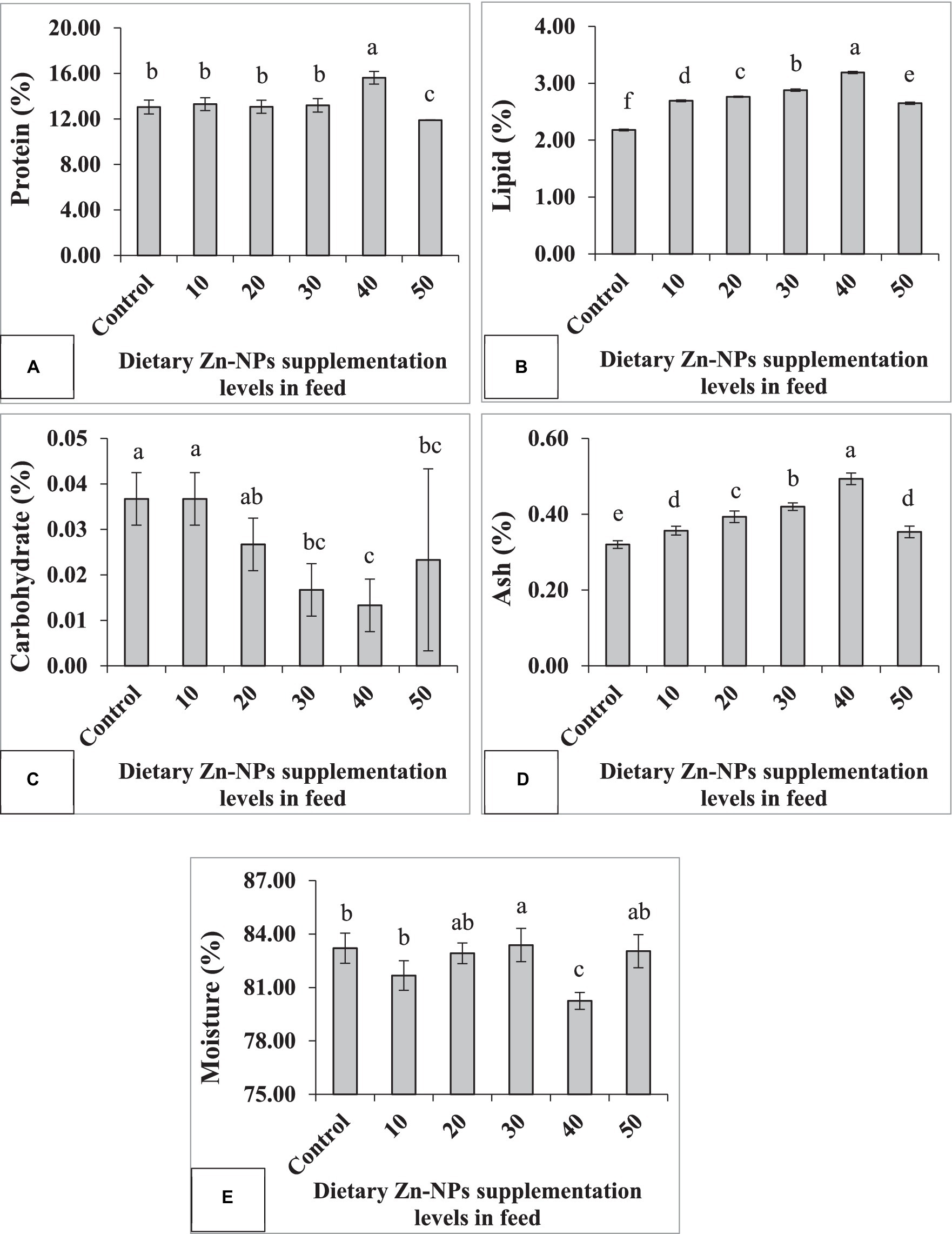
Figure 3. Proximate composition of C. batrachus fed on different levels of Zn-NPs enriched diets. Different superscript letters indicate significant difference (p < 0.05) among the feeding groups (A, Protein F = 15.93, p < 0.01; B, Lipid F = 1338.62, p < 0.01; C, Carbohydrates F = 8.67, p < 0.01; D, Ash F = 65.99, p < 0.01; E, Moisture F = 7.24, p < 0.01).
3.4 Hematological parameters
Hematological parameters examined during the present experiment are shown in Table 3. Dietary Zn-NPs supplementation in feed was found to have a significant toxic effect on hematological parameters, however in a dose-dependent manner. RBC count was decreased significantly in Zn-NPs treated fish from that of the respective control group. RBC count was 25.28% higher at 40 mg/kg Zn-NPs group compared to the control group. However, the WBC count increased significantly in Zn-NPs-treated fish compared to the control group. A significant increasing trend was also observed in both Hb (hemoglobin) and Hct (hematocrit) content with an increasing Zn-NPs concentration up to 40 mg/kg Zn-NPs group; however, an abrupt decreasing trend was observed in the 50 mg/kg Zn-NPs group. Hematological indices such as MCV and MCH were showed irregular ups and downs and significantly (p < 0.05) higher values in 50 mg/kg Zn-NPs group, while a significantly lower value was evident in the control group. No significant difference was observed in MCHC value for all the concentrations and control groups. Total protein, albumin, and globulin were significantly different between the control and various Zn-NPs concentrations, according to an analysis of variance (F-values). A considerable increment in serum total protein, albumin, and globulin was seen in 50 mg/kg Zn-NPs group when compared to the control groups about the effect of the investigated Zn-NPs concentrations.
3.5 Serum lipid and enzyme profile
The effects of Zn-NPs supplementation in feed on the serum lipid and enzyme profile of C. batrachus are presented in Table 4. Zn-NPs supplementation in feed significantly (p < 0.05) increased the values of all the parameters compared to the control group. Elevated levels of total cholesterol, HDL, triglycerides, ALP, AST, ALT, amylase, lipase, and protease at 50 mg/kg Zn-NPs enriched diet indicated the toxic effect of Zn-NPs at higher doses. However, optimal growth performance, nutritional indices, and hematological parameters suggest the effective role of 40 mg/kg Zn-NPs supplementation in feed for improving serum lipid and enzyme profile of C. batrachus.
3.6 Bioaccumulation of Zn
The results demonstrate a clear correlation between the dietary concentration of Zn-NPs and the zinc levels found in the muscle, serum, and liver of the fish. As the concentration of Zn-NPs in the diet increased, there was a consistent elevation in zinc levels across all tissues (Figure 4). Particularly noteworthy is the group of fish fed with a diet containing 50 mg/kg Zn-NPs, which exhibited significantly higher zinc content in the muscle, serum, and liver compared to other dietary concentrations. Furthermore, the liver consistently showed the highest accumulation of zinc among the three tissues examined, followed by the muscle and serum, with the order of zinc accumulation being liver > muscle > serum. These findings suggest that dietary supplementation of Zn-NPs leads to increased zinc accumulation in fish tissues, with the liver being the primary site of accumulation, followed by muscle tissue and serum. Accumulation of Zn in muscle, serum and liver was 64.50, 77.41 and 62.58%, respectively higher at 50 mg/kg Zn-NPs supplemented group compared to the control group. Furthermore, accumulation of Zn at 50 mg/kg Zn-NPs supplemented group was 14.13 and 58.86% in liver compared to muscle and serum, respectively.
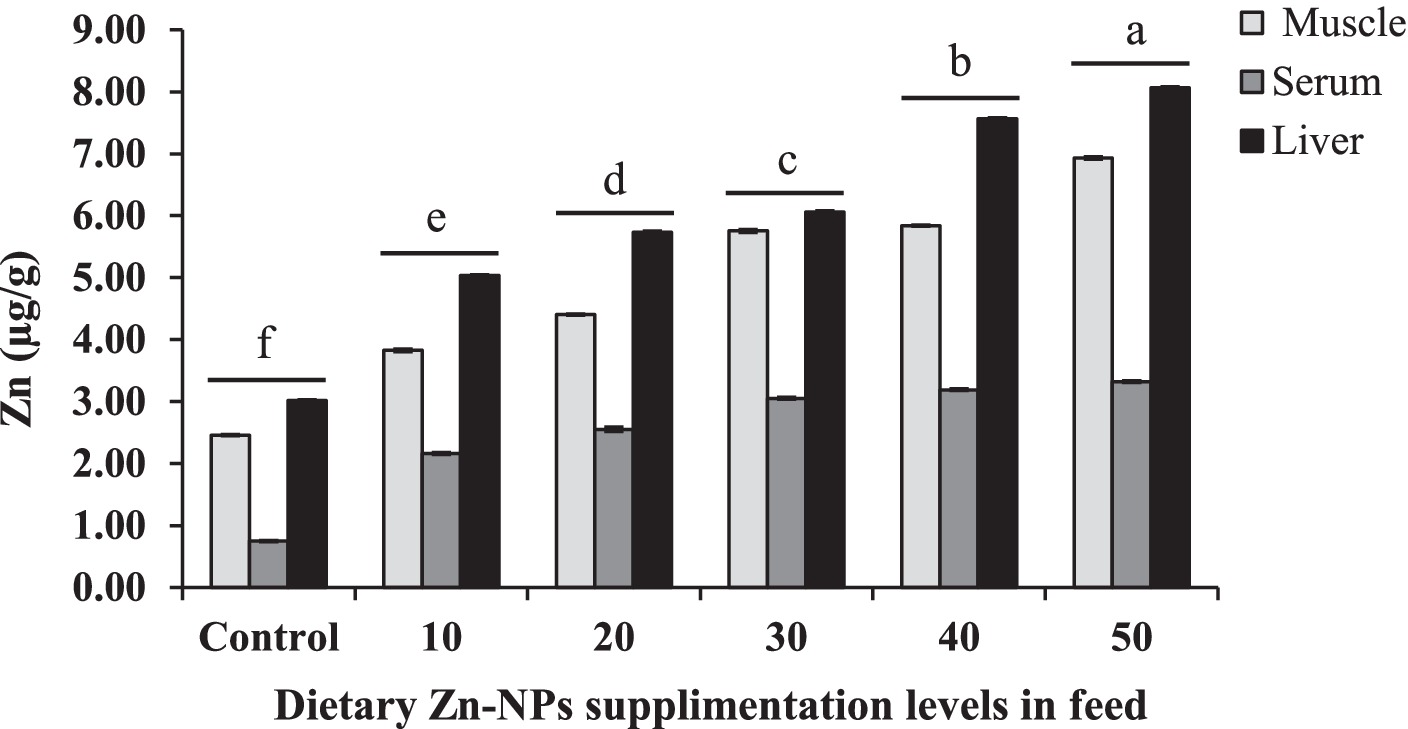
Figure 4. Concentrations of Zn in muscle, serum, and liver of C. batrachus fed on different levels of Zn-NP enriched diets. Different superscript letters indicate significant difference (p < 0.05) among the feeding groups (Muscle F = 26158.39, p < 0.01; Serum F = 7374.54, p < 0.01; Liver F = 49716.83, p < 0.01).
4 Discussion
The results of this study demonstrated that including Zn-NPs in the diet of C. batrachus promoted optimal growth and favorable adaptation to its environment. Importantly, water quality measurements consistently remained within optimal ranges for tropical fish farming conditions across all treatments, showing no significant differences. This underscores the maintenance of a favorable rearing environment throughout the experiment. Moreover, the incorporation of Zn-NPs into the diet led to a significant improvement in the growth performance of C. batrachus in a dose-dependent manner compared to the control group. Notably, no mortalities were observed throughout the study period.
Specifically, fish receiving 40 mg/kg Zn-NPs supplementation showed notable increases (p < 0.05) in parameters such as fish weight (FW), fish length (FL), weight gain (WG), percentage weight gain (%WG), average daily gain (ADG), specific growth rate (SGR), and condition factor (CF), surpassing both other treatment groups and the control. Similar synergistic effects of zinc on growth performance have been reported for various fish and prawn species (Liang et al., 2012; Chen et al., 2015). The ability of nanoparticles to enhance intestinal absorption, bioavailability, growth hormone synthesis, and catalytic activity has been acknowledged to support fish growth, development, and physiology (Albrecht et al., 2006; Eide, 2006; Maret and Krężel, 2007; Dube et al., 2010; Alishahi et al., 2011). The enhanced growth performance observed in C. batrachus may be attributed to the stimulation of DNA and RNA synthesis, as well as cell division, induced by Zn-NPs supplementation. These processes likely contribute to somatic growth and development in the fish. Faiz et al. (2015) observed similar findings in Grass carp (C. idella), while Shahpar and Johari (2019) reported comparable results in rainbow trout (Oncorhynchus mykiss) larvae. However, although a Zn-NPs concentration of 40 mg/kg enhanced growth, a slightly higher concentration of 50 mg/kg Zn-NPs significantly reduced the growth rate. Similarly, decreased growth was noted in juveniles of the Grass carp, C. idella, exposed to 50 mg/kg ZnO-NP supplementation compared to 30 mg/kg ZnO-NP supplementation (Faiz et al., 2015). This suggests potential zinc toxicity at the highest concentration tested in this study. Although Zn poisoning is uncommon, it is best to avoid supplementing with excessive Zn levels as this prevents the uptake of other minerals preferably iron, copper, and cadmium required for fish to grow and function normally (Buentello et al., 2009). As per Jewel et al. (2023), administering Fe-NPs and Zn-NPs at 30 mg/kg improved survival and growth in L. rohita, whereas adverse effects were observed at 50 mg/kg. Thus, it is crucial to determine the optimal Zn-NPs dosage for fish feed to effectively boost fish growth and production performance.
Throughout the experimental phase, the FCR of the fishes demonstrated superior performance in the group treated with nanoparticles (NPs), with variations observed based on concentration levels. Significant enhancements in feed utilization parameters were noted in the 40 mg/kg Zn-NPs group compared to the control group. However, feeding parameters sharply declined in the 50 mg/kg Zn-NPs group, resulting in reduced growth performance in fish within this group. This finding is consistent with a similar observation documented by Swain et al. (2018) in their study on the treatment of L. rohita with ZnONPs, where a reduction in feed utilization was observed in the NP-fed group compared to the control. Similarly, Kumar et al. (2020) and Rajan and Rohini (2021) observed enhanced feed efficiency in Cirrhinus mrigala through dietary ZnO-NPs, which was attributed to elevated digestive enzyme activity. Thangapandiyan and Monika (2019) also reported improved feed utilization (FCR) in Zn-NPs-enriched diets for L. rohita.
The increasing levels of protein, lipid, and ash observed in fish muscle provide evidence that dietary Zn-NPs influence the absorption, synthesis, and storage of these parameters in C. batrachus. Consistent with our findings, similar concentration-dependent increases and decreases in proximate composition have been reported in L. rohita fed diets enriched with 30 mg/kg NPs, with a subsequent decline observed in these parameters for diets containing 40–50 mg/kg NPs supplementation (Jewel et al., 2023). Additionally, Tawfik et al. (2017) demonstrated elevated levels of muscle protein in tilapia fish fed a diet supplemented with different concentrations of ZnONPs. Thangapandiyan and Monika (2019) also reported an increased level of carbohydrate, total protein, and lipid in L. rohita fed with a diet supplemented with 10 mg/kg ZnONPs. This is consistent with the crucial role that zinc (Zn) plays in the metabolism of lipids, proteins, carbohydrates, and nucleic acids.
Our study revealed significant changes in blood parameters of catfish fed diets supplemented with various NP concentrations compared to the control. The decrease observed in red blood cells (RBCs), hemoglobin, and hematocrit, in a dose-dependent manner, may be attributed to red cell swelling causing hemolysis. Similar erythrocyte hemolysis has been documented in Heteroclarias as well (Oti and Avoaja, 2005; Kori-Siakpere and Ubogu, 2008), induced by sublethal Zn concentrations in the diet. Studies have suggested that nanoparticles can have adverse effects on red blood cell populations, potentially leading to the development of anemia. The adverse impact is primarily ascribed to either the reduction in red blood cell lifespan or the inhibition of bone marrow stem cell activity (Faiz et al., 2015). The increase in RBC, Hb, and Hct levels up to the 40 mg/kg Zn-NPs diet may be attributed to the potent antioxidant properties of nanoparticles, which help maintain cell stability and integrity within organisms, thus guarding against hemolysis. However, at higher levels, nanoparticles can become toxic, leading to adverse effects (Khan et al., 2016). The sudden decrease in RBC, Hb, and Hct at the 50 mg/kg Zn-NPs group in our study suggests the onset of anemia due to injury to hematopoietic tissue, suppression of erythropoiesis, or malfunction of transferrin (Javed et al., 2016). The increase in WBC levels observed in our study may be attributed to nanoparticles bioaccumulating in various tissues, thereby inducing toxicity. Earlier investigations have indicated that heightened NP concentrations notably affect spleen cell production (Firat, 2007). Additionally, it has been observed that this increase in NPs leads to a greater secretion of corticosteroid hormones, which play a crucial role in the prevention and healing of inflammation (Celik et al., 2013). Our findings suggest that the increase in WBCs recorded in the 50 mg/kg NPs feeding group may be linked to the stimulation of the immune system in response to exposure to toxic high doses of Zn-NPs (Vani et al., 2011; Bantu et al., 2017). The observed alterations in erythrocyte indices in the present study are likely a result of stress induced by the same toxic effect. At higher concentrations (< 50 mg/kg), ZnO-NPs have been reported to affect erythrocyte indices by reducing the blood’s ability to transport oxygen and causing abnormalities in blood tissue (Hrubec and Smith, 2010; Saravanan et al., 2011; Suganthi et al., 2015). Similarly, an increase in erythrocyte indices has been documented in L. rohita fish exposed to various stressors such as paint, dye, and petroleum industry effluents (Zutshi et al., 2010).
It was observed that dietary Zn-NPs resulted in a significant rise in the concentrations of total protein, albumin, and globulin in the blood serum of C. batrachus within the 50 mg/kg Zn-NPs group. Zinc nanoparticles (Zn-NPs) administered at doses ranging from 10 to 40 mg/kg in the feed did not exhibit any notable differences compared to the control group, except for the levels of globulin. However, at a dosage of 50 mg/kg in the feed, zinc nanoparticles (Zn-NPs) notably elevated these markers above those observed in the control and other experimental groups. The observed increase in albumin and globulin levels suggests an induced immune response in fish treated with NPs. Moreover, there is a documented positive correlation between elevated serum protein and globulin levels and a robust innate immune response in various fish species (Kumar et al., 2013).The presence of an elevated immune response suggests that fish are experiencing a compromised and stressful state, rendering them susceptible to diseases (Kanwal et al., 2019). The investigation conducted by Yonar et al. (2019) examined the significant increase in serum total protein levels in rainbow trout that were fed curcumin. Similarly, Manal (2018) studied the augmentation of serum total protein in Nile tilapia fish through the supplementation of curcumin. Additionally, Abdel-Tawwab et al. (2022) investigated the effect of Cu-NP supplementation on serum total protein levels. According to Moghadam et al. (2021), it was observed that feeding L. vannamei with diets enriched with Cu-NPs resulted in elevated levels of serum total protein and albumin. The observed elevation of these proteins in the groups treated with NP can be attributed to the enhanced synthesis of proteins, which is necessary to meet the increased energy demands caused by the higher concentration of NPs in the feed. This heightened protein synthesis is essential to address the immunotoxic challenge posed by the NPs.
The results of the present investigation showed that total cholesterol, LDL, triglycerides, and HDL activity in fish serum were all affected by Zn-NPs in a dose-dependent manner. Specifically, Zn-NPs were found to decrease the activity of total cholesterol, LDL, and triglycerides, while increasing the activity of HDL, when compared to the control group. Zinc nanoparticles (Zn-NPs) have been recognized for their antioxidant properties, which have been attributed to the enhancement of the aforementioned parameters in Asian catfish when compared to the control group (Mahboub et al., 2020). Zn is also recognized as essential for lipid metabolism, which includes lipid synthesis, storage, and breakdown processes. By modifying the activity of enzymes involved in lipid synthesis and metabolism, such as lipoprotein lipase, hormone-sensitive lipase, and fatty acid synthase, Zn-NPs supplementation might have an impact on lipid metabolism. Safawo et al. (2018) observed a significant free radical scavenging activity of ZnO nanoparticles, with an IC50 value of 127.74 μg/mL, indicating a 50% inhibition concentration. The concentration-dependent increase in the free radical scavenging activity of nanoparticles was also reported by Tettey and Shin (2019) and Ramana et al. (2023). El-Bahr et al. (2020) found that two doses of ZnO NPs (30 and 60 mg/kg) enhance liver function in birds by lowering blood total cholesterol and triglyceride, which corresponds well with our present findings. However, when administered at higher doses (40 and 50 mg/kg Zn-NPs feed), a notable elevation in the concentration may suggest the presence of stress in fish. In a study conducted by Levesque et al. (2002), it was demonstrated that chronic exposure of Perca flavescens to sub-lethal concentrations of copper (Cu), cadmium (Cd), and zinc (Zn) resulted in significant alterations in triglyceride levels. The assessment of triglyceride concentration is crucial for the evaluation of lipid metabolism, as elevated levels of triglycerides have been observed in individuals with nephritic syndrome and glycogen storage impairment. Hence, the findings of this study demonstrate that elevated doses of triglyceride are associated with impaired liver function in C. batrachus in the present study. When compared to the control group, serum ALP, AST, ALT, amylase, lipase, and protease activities in C. batrachus exhibited a usually very significant rise as the quantity of Zn-NPs in the diet increased. A comparable finding was also documented by Alkaladi et al. (2015), who noted that the release of serum enzymes increased significantly with higher concentrations of ZnONPs. This phenomenon is likely attributed to hepato-pancreatic injury, indicating potential harm to various fish tissues, including parenchymal cells, muscles, intestines, and liver (Kandeel, 2004). In their study, Firat and Kargan (2010) observed that the serum activities of ALT and AST in Oreochromis niloticus were elevated when exposed to Zn and Cd, in comparison to the control group. Furthermore, in a study conducted by Younis et al. (2012), it was also observed that there was a notable rise in the levels of AST and ALT in Zn-treated O. niloticus following a brief period of exposure. The observed elevation in serum enzyme activity suggests that the addition of Zn-NPs has an impact on the secretion of serum enzymes in C. batrachus. Supporting this observation, Li et al. (2007) reported increased activities of protease, amylase, and lipase in tilapia (O. niloticus and O. aureus) fed with feed supplemented with zinc. Similarly, Jewel et al. (2023) found increased activities of these enzymes in the L. rohita when fed with zinc-supplemented feed.
In the current study, it was observed that C. batrachus absorbed more nanoparticles (NPs) than the control group when fed a diet enriched with 50 mg/kg of Zn-NPs. These findings indicate that the level of supplementation in the diet influenced the absorption of Zn-NPs in catfish, with the highest concentration of Zn-NPs (50 mg/kg diet) resulting in a significant increase in zinc concentration in the liver. In a related study, Kaya et al. (2015) discovered that the liver of juvenile carp (Cyprinus carpio) consistently accumulated zinc compared to the gills, kidneys, and muscles. Shahzad et al. (2017) and Kurian and Elumalai (2021) showed similar results, with increased Zn accumulation in the liver of tilapia (O mossambicus) compared to muscles and gills. The collective findings of these prior studies consistently demonstrate a positive correlation between the dosage concentration of ZnO-NPs and the accumulation of zinc in the liver of fish, but not necessarily other tissues, as was also found in the present study. The liver chemically assimilates, excretes, and detoxifies metals (Marijić and Raspor, 2006). Due to liver detoxification, toxic substances accumulate and change hepatocyte physiology and histology (Sharma et al., 2012).
5 Conclusion
Dietary Zn-NPs were found to influence the physiological status, growth performance, and nutritional consumption of C. batrachus in a dose-dependent manner. Growth, nutritional indices, and physiology of C. batrachus were found to be enhanced due to the supplementation of Zn-NPs at a concentration of 40 mg/kg when compared to the control and other groups. According to the polynomial regression analysis, the optimal concentration of Zn-NPs supplementation in the diet of C. batrachus was determined to be between 30.4 and 30.5 mg/kg of feed. Additionally, the research demonstrated that the liver was the most prominent bioaccumulator organ for Zn from Zn-NPs, and the bioaccumulation was highest at higher concentrations of Zn-NPs in the diet. Despite these promising findings, further research is needed to fully assess the potential of Zn-NPs to be used in a range of fish species.
Data availability statement
The original contributions presented in the study are included in the article/Supplementary material, further inquiries can be directed to the corresponding authors.
Author contributions
AJ: Conceptualization, Data curation, Funding acquisition, Resources, Writing – original draft. AH: Investigation, Methodology, Writing – original draft. NA: Investigation, Methodology, Writing – original draft. SA: Data curation, Formal analysis, Writing – original draft. AS: Methodology, Writing – original draft. PS: Funding acquisition, Resources, Writing – review & editing. DM: Resources, Writing – review & editing. BP: Funding acquisition, Writing – review & editing. MH: Funding acquisition, Resources, Validation, Writing – review & editing.
Funding
The author(s) declare that financial support was received for the research, authorship, and/or publication of this article. This study was supported by Researchers Supporting Project Number (RSP2024R144), King Saud University, Riyadh, Saudi Arabia.
Acknowledgments
Thanks are extended to Lab Assistants. The authors acknowledge the support from Researchers Supporting Project Number (RSP2024R144), King Saud University, Riyadh, Saudi Arabia.
Conflict of interest
The authors declare that the research was conducted in the absence of any commercial or financial relationships that could be construed as a potential conflict of interest.
The author(s) declared that they were an editorial board member of Frontiers, at the time of submission. This had no impact on the peer review process and the final decision.
Publisher’s note
All claims expressed in this article are solely those of the authors and do not necessarily represent those of their affiliated organizations, or those of the publisher, the editors and the reviewers. Any product that may be evaluated in this article, or claim that may be made by its manufacturer, is not guaranteed or endorsed by the publisher.
Supplementary material
The Supplementary material for this article can be found online at: https://www.frontiersin.org/articles/10.3389/fsufs.2024.1410557/full#supplementary-material
References
Abdel-Tawwab, M., Eissa, E. -S. H., Tawfik, W. A., Abd Elnabi, H. E., Saadony, S., Bazina, W. K., et al. (2022). Dietary curcumin nanoparticles promoted the performance, antioxidant activity, and humoral immunity, and modulated the hepatic and intestinal histology of Nile tilapia fingerlings. Fish Physiol. Biochem. 48, 585–601. doi: 10.1007/s10695-022-01066-4
Ahmed, N., Alam, M. F., and Hasan, M. R. (2010). The economics of sutchi catfish (Pangasianodon hypophthalmus) aquaculture under three different farming systems in rural Bangladesh. Aquaculture Res. 41, 1668–1682. doi: 10.1111/j.1365-2109.2010.02549.x
Akter, S., Haque, M. A., Sarker, M. A. A., Atique, U., Iqbal, S., Sarker, P. K., et al. (2024). Efficacy of using plant ingredients as partial substitute of fishmeal in formulated diet for a commercially cultured fish. Labeo rohita. Front. Sustain. Food Syst. 8:1376112. doi: 10.3389/fsufs.2024.1376112
Alam, M. J., Sultana, F., and Iqbal, M. T. (2015). The potential of iron nanoparticles to increase germination and growth of wheat seedlings. J. Nanosci. Adv. Tech. 1, 14–20. doi: 10.24218/jnat.2015.12
Albrecht, M. A., Evans, C. W., and Raston, C. L. (2006). Green chemistry and the health implications of nanoparticles. Green Chem. 8, 417–432. doi: 10.1039/b517131h
Ali, H., Haque, M. M., and Belton, B. (2013). Striped catfish (Pangasianodon hypophthalmus, Sauvage, 1878) aquaculture in Bangladesh: an overview. Aquaculture Res. 44, 950–965. doi: 10.1111/j.1365-2109.2012.03101.x
Alishahi, A., Mirvaghefi, A., Tehrani, M. R., Farahmand, H., Shojaosadati, S. A., Dorkoosh, F. A., et al. (2011). Shelf life and delivery enhancement of vitamin C using chitosan nanoparticles. Food Chem. 126, 935–940. doi: 10.1016/j.foodchem.2010.11.086
Alkaladi, A., El-Deen, N. A. M. N., Afifi, M., and Zinadah, O. A. A. (2015). Hematological and biochemical investigations on the effect of vitamin E and C on Oreochromis niloticus exposed to zinc oxide nanoparticles. Saudi J. Biol. Sci. 22, 556–563. doi: 10.1016/j.sjbs.2015.02.012
AOAC (2016). “Official methods of analyses” in Association of Official Analytical Chemist. 20th ed. Ed. G. Latimer (Washington, DC, USA: AOAC International).
Bantu, N., Chaithnaya karri, K., Krishnan VK, G., Kumari, N., and Vakita, R. (2017). Histological alteration in different tissues of Indian major carp, Labeo rohita (Hamilton) exposed to Profenofos 50% EC and Carbosulfan 25% EC formulations. J. biol. today's world 6, 38–52. doi: 10.15412/J.JBTW.01060301
Behera, T., Swain, P., Rangacharulu, P. V., and Samanta, M. (2014). Nano-Fe as feed additive improves the hematological and immunological parameters of fish, Labeo rohita H. Applied Nanosci. 4, 687–694. doi: 10.1007/s13204-013-0251-8
Buentello, J. A., Goff, J. B., and Gatlin, D. M. III (2009). Dietary zinc requirement of hybrid striped bass Morone chrysops × Morone saxatilis and bioavailability of two chemically different zinc compounds. J. World Aquacult. Soc. 40, 687–694. doi: 10.1111/j.1749-7345.2009.00288.x
Bull, B. S., Koepke, J. A., Simson, E., and van Assendelft, O. W. (2000). Procedure for determining packed cell volume by microhematocrit method; approved standard, vol. 20. third Edn. USA: Clinical and laboratory standards institute, 1–7.
Celik, E. S., Kaya, H., Yilmaz, S., Akbulut, M., and Tulgar, A. (2013). Effects of zinc exposure on the accumulation, haematology and immunology of Mozambique tilapia, Oreochromis mossambicus. Afr. J. Biotechnol. 12, 744–753. doi: 10.5897/AJB12.1408
Chen, Q., Liu, H. Y., Tan, B. P., Dong, X. H., Chi, S. Y., Yang, Q. H., et al. (2015). Effects of dietary cholesterol level on growth performance, blood biochemical parameters and lipid metabolism of juvenile cobia (Rachycentron canadum). J. Guangdong Ocean Univ. 36, 35–43. doi: 10.3969/j.issn.1673-9159.2016.01.007
Dacie, J. V., and Lewis, S. M. (1999). Practical hematology. 6th Edn. London, UK: Churchill Livingstone, 1999.
Drabkin, D. L. (1950). The distribution of the chromoproteins, hemoglobin, myoglobin, and cytochrome C, in the tissues of different species, and the relationship of the total content of each chromoprotein to body mass. J. Biol. Chem. 182, 317–333. doi: 10.1016/s0021-9258(18)56550-9
Dube, A., Nicolazzo, J. A., and Larson, I. (2010). Chitosan nanoparticles enhance the intestinal absorption of the green tea catechins (+) catechin and (−) -epigallocatechin gallate. Eur. J. Pharm. Sci. 41, 219–225. doi: 10.1016/j.ejps.2010.06.010
Eide, D. J. (2006). Zinc transporters and the cellular trafficking of zinc. Biochim. Biophys. Acta 1763, 711–722. doi: 10.1016/j.bbamcr.2006.03.005
El-Bahr, S. M., Shousha, S., Albokhadaim, I., Shehab, A., and Khattab, W. (2020). Impact of dietary zinc oxide nanoparticles on selected serum biomarkers, lipid peroxidation and tissue gene expression of antioxidant enzymes and cytokines in Japanese quail. BMC Vet. Res. 16:349. doi: 10.1186/s12917-020-02482-5
Faiz, H., Zuberi, A., Nazir, S., Rauf, M., and Younus, N. (2015). Zinc oxide, zinc sulfate and zinc oxide nanoparticles as source of dietary zinc: comparative effects on growth and hematological indices of juvenile grass carp (Ctenopharyngodon idella). Int. J. Agric. Biol. 17, 568–574. doi: 10.17957/IJAB/17.3.14.446
FAO (2020). The state of world fisheries and aquaculture 2020. Rome: Contributing to food security and nutrition for all, 220.
Fasil, D. M., Paritosh, P., Parashar, S. K. S., and Biswadeep, D. (2019). Mechanistic insights into diverse nano-based strategies for aquaculture enhancement: a holistic review. Aquaculture 519:734770. doi: 10.1016/j.aquaculture.2019.734770
Firat, O. (2007). Effects of metal (Zn, cd) and metal mixtures (Zn + cd) on physiological and biochemical parameters in blood tissues of Oreochromis niloticus. Ph. D Thesis, Çukurova University, Turkey.
Firat, O., and Kargan, F. (2010). Individual and combined effects of heavy metals on serum biochemistry of Nile Tilapia, Oreochromis niloticus. Arch. Environ. Contam. Toxicol. 58, 151–157. doi: 10.1007/s00244-009-9344-5
Hossain, M. A., Hossain, M. A., Haque, M. A., Mondol, M. M. R., Harun-Ur-Rashid, M., and Das, S. K. (2022). Determination of suitable stocking density for good aquaculture practice-based carp fattening in ponds under drought-prone areas of Bangladesh. Aquaculture 547:737485. doi: 10.1016/j.aquaculture.2021.737485
Hrubec, T. C., and Smith, S. A. (2010). “Schalm’s veterinary hematology” in Hematology of fishes. eds. B. F. Feldman, J. G. Zinkl, and N. C. Jain. 5th ed (Philadelphia, PA: Lippincott Williams & Wilkins), 1120–1125.
Javed, M., Ahmad, I., Ahmad, A., Usmani, N., and Ahmad, M. (2016). Studies on the alterations in haematological indices, micronuclei induction and pathological marker enzyme activities in Channa punctatus (spotted snakehead) perciformes, channidae exposed to thermal power plant effluent. Springerplus 5:761. doi: 10.1186/s40064-016-2478-9
Jewel, M. A. S., Haque, M. A., Pervin, M. E., Akter, S., Ali, S. W., Noor, N. M., et al. (2023). Regulatory mechanisms of nutrient metabolism and the impacts of iron and zinc nanoparticles on growth and physiology of Rohu. Labeo rohita. Anim. Feed Sci. Technol. 304:115759. doi: 10.1016/j.anifeedsci.2023.115759
Jiang, J., Pi, J., and Cai, J. (2018). The advancing of zinc oxide nanoparticles for biomedical applications. Bioinorg. Chem. Appl. 3, 1–18. doi: 10.1155/2018/1062562
Kandeel, N. M. S. (2004). Toxicological and metabolic studies of some molluscicides on harmful terrestrial snails (M.Sc. thesis). Zoology Dep., Faculty of Science, Cairo University.
Kanwal, Z., Raza, M. A., Manzoor, F., Riaz, S., Jabeen, G., Fatima, S., et al. (2019). A comparative assessment of nanotoxicity induced by metal (silver, nickel) and metal oxide (cobalt, chromium) nanoparticles in Labeo rohita. Nano 9:309. doi: 10.3390/nano9020309
Karthikeyan, A., Senthil, N., and Min, T. (2020). Nanocurcumin: a promising candidate for therapeutic applications. Front. Pharmacol. 11:487. doi: 10.3389/fphar.2020.00487
Kaya, H., Aydın, F., Gürkanc, M., Yılmaza, S., Atesd, M., Demire, V., et al. (2015). Effects of zinc oxide nanoparticles on bioaccumulation and oxidative stress in different organs of tilapia (Oreochromis niloticus). Environ. Toxicol. Pharmacol. 40, 936–947. doi: 10.1016/j.etap.2015.10.001
Khan, K. U., Zuberi, A., Nazir, S., Fernandes, J. B., Jamil, Z., and Sarwar, H. (2016). Effects of dietary selenium nanoparticles on physiological and biochemical aspects of juvenile Tor putitora. Turkish J. Zool. 40, 704–712. doi: 10.3906/zoo-1510-5
Kori-Siakpere, O., and Ubogu, E. O. (2008). Sublethal haematological effects of zinc on the freshwater fish, Heteroclarias sp. (Osteichthyes: Clariidae). Afr. J. Biotechnol. 7, 2068–2073. doi: 10.5897/ajb07.706
Kumar, N., Chandan, N. K., Wakchaure, G. C., and Singh, N. P. (2020). Synergistic effect of zinc nanoparticles and temperature on acute toxicity with response to biochemical markers and histopathological attributes in fish. Comp. Biochem. Physiol. Part C Toxicol. Pharmacol. 229:108678. doi: 10.1016/j.cbpc.2019.108678
Kumar, N., Krishnani, K., Kumar, P., and Singh, N. P. (2017). Zinc nanoparticles potentiates thermal tolerance and cellular stress protection of Pangasius hypophthalmus reared under multiple stressors. J. Therm. Biol. 70, 61–68. doi: 10.1016/j.jtherbio.2017.10.003
Kumar, N., Krishnani, K. K., and Singh, N. P. (2018). Effect of dietary zinc-nanoparticles on growth performance, anti-oxidative and immunological status of fish reared under multiple stressors. Biol. Trace Elem. Res. 186, 267–278. doi: 10.1007/s12011-018-1285-2
Kumar, N., Kumar, S., Singh, A. K., Gite, A., Patole, P. B., and Thorat, S. T. (2022). Exploring mitigating role of zinc nanoparticles on arsenic, ammonia and temperature stress using molecular signature in fish. J. Trace Elem. Med. Biol. 74:127076. doi: 10.1016/j.jtemb.2022.127076
Kumar, S., Raman, R. P., Pandey, P. K., Mohanty, S., Kumar, A., and Kumar, K. (2013). Effect of orally administered azadirachtin on non-specific immune parameters of goldfish Carassius auratus (Linn. 1758) and resistance against Aeromonas hydrophila. Fish Shellfish Immunol. 34, 564–573. doi: 10.1016/j.fsi.2012.11.038
Kumar, N., Singh, D. K., Chandan, N. K., Thorat, S. T., Patole, P. B., Gite, A., et al. (2023). Nano-zinc enhances gene regulation of non-specific immunity and antioxidative status to mitigate multiple stresses in fish. Sci. Rep. 13:5015. doi: 10.1038/s41598-023-32296-y
Kumar, N., Thorat, S. T., Chavhan, S. R., and Reddy, K. S. (2024). Potential role of dietary zinc on gene regulation of growth performance and immunity in Pangasianodon hypophthalmus against multiple stresses. Aquaculture 580:740271. doi: 10.1016/j.aquaculture.2023.740271
Kurian, A., and Elumalai, P. (2021). Study on the impacts of chemical and green synthesized (Leucas aspera and oxy-cyclodextrin complex) dietary zinc oxide nanoparticles in Nile tilapia (Oreochromis niloticus). Environ. Sci. Pollut. Res. Int. 28, 20344–20361. doi: 10.1007/s11356-020-11992-6
Levesque, H. M., Moon, T. W., Campbell, P. G. C., and Hontela, A. (2002). Seasonal variation in carbohydrate and lipid metabolism of yellow perch (Perca flavescens) chronically exposed to metals in the field. Aquat. Toxicol. 60, 257–267. doi: 10.1016/S0166-445X(02)00012-7
Li, J. -S., Li, J. -L., and Wu, T. -T. (2007). The effects of copper, iron and zinc on digestive enzyme activity in the hybrid tilapia Oreochromis niloticus (L.) × Oreochromis aureus (Steindachner). J. Fish Biol. 71, 1788–1798. doi: 10.1111/j.1095-8649.2007.01643.x
Liang, J. J., Yang, H. J., Liu, Y. J., Tian, L. X., and Liang, G. Y. (2012). Dietary zinc requirement of juvenile grass carp (Ctenopharyngodon idella) based on growth and mineralization. Aquac. Nutr. 18, 380–387. doi: 10.1111/j.1365-2095.2011.00935.x
Linnaeus, C. (1758). Systema Naturae per regna tria naturae, secundum classes, ordines, genera, species, cum characteribus, differentiis, synonymis, locis. Editio decima, reformata [10th revised edition], vol. 1: 824 pp. Laurentius Salvius: Holmiae. Available at: https://biodiversitylibrary.org/page/726886 (Accessed January 23, 2023).
Luis, A. I. S., Campos, E. V. R., de Oliveira, J. L., and Fraceto, L. F. (2017). Trends in aquaculture sciences: from now to use of nanotechnology for disease control. Rev. Aquac. 11, 119–132. doi: 10.1111/raq.12229
Mahboub, H. H., Rashidian, G., Hoseinifar, S. H., Kamel, S., Zare, M., Ghafarifarsani, H., et al. (2020). Protective effects of Allium hirtifolium extract against foodborne toxicity of zinc oxide nanoparticles in common carp (Cyprinus carpio). Comp. Biochem. Physiol. Part C: Toxicol. Pharmacol. 257:109345. doi: 10.1016/j.cbpc.2022.109345
Manal, I. (2018). Impact of garlic and curcumin on the hepatic histology and cytochrome P450 gene expression of aflatoxicosis Oreochromis niloticus using RT-PCR. Turk. J. Fish. Aquat. Sci. 18, 405–415. doi: 10.4194/1303-2712-v18_3_06
Maret, W., and Krężel, A. (2007). Cellular zinc and redox buffering capacity of metallothionein/thionein in health and disease. Mol. Med. 13, 371–375. doi: 10.2119/2007-00036.Maret
Marijić, V. F., and Raspor, B. (2006). Age- and tissue-dependent metallothionein and cytosolic metal distribution in a native Mediterranean fish, Mullus barbatus, from the eastern Adriatic Sea. Comp Biochem Physiol C Toxicol Pharmacol 143, 382–387. doi: 10.1016/j.cbpc.2005.05.019
Masoomi Dezfooli, S., Gutierrez-Maddox, N., Alfaro, A., and Seyfoddin, A. (2018). Encapsulation for delivering bioactives in aquaculture. Rev. Aquac. 11, 631–660. doi: 10.1111/raq.12250
Moghadam, H., Sourinejad, I., and Johari, S. A. (2021). Growth performance, haemato-immunological responses and antioxidant status of Pacific white shrimp Penaeus vannamei fed with turmeric powder, curcumin and curcumin nanomicelles. Aquac. Nutr. 27, 2294–2306. doi: 10.1111/anu.13363
Moller, K., Eeswaran, R., Nejadhashemi, A. P., and Hernandez-Suarez, J. S. (2023). Livestock and aquaculture farming in Bangladesh: current and future challenges and opportunities. Cogent Food Agricult. 9:2241274. doi: 10.1080/23311932.2023.2241274
Moss, A. S., Ishikawa, M., Koshio, S., Yokoyama, S., and Dawood, M. A. (2019). Effects of different levels of marine snail shells in the diets of juvenile Kuruma shrimps Marsupenaeus japonicus as a source of calcium. N. Am. J. Aquac. 81, 55–66. doi: 10.1002/naaq.10066
Nordgarden, U., Oppedal, F., Taranger, G. L., Hemre, G. I., and Hansen, T. (2003). Seasonally changing metabolism in Atlantic salmon (Salmo salar L.) I —growth and feed conversion ration. Aquac. Nutr. 9, 287–293. doi: 10.1046/j.1365-2095.2003.00256.x
Oti, E. E., and Avoaja, D. A. (2005). Haematological assessment of freshwater catfishes, Clarias gariepinus (Burch) and “Heteroclarias” (hybrid) exposed to sublethal concentrations of zinc. Pak. J. Zool. 37, 101–105.
Parida, S. P., Dutta, S. K., and Pal, A. (2011). Hematological and plasma biochemistry in Psammophilus blanfordanus (Sauria: Agamidae). Comp. Clin. Pathol. 21, 1387–1394. doi: 10.1007/S00580-011-1303-7
Rajan, M. R., and Rohini, R. (2021). Impact of different quantity of zinc oxide nanoparticles on growth and hematology of Mrigal Cirrhinus mrigala. J. Water Environ. Nanotechnol. 6, 62–71. doi: 10.22090/jwent.2021.01.006
Ramana, V., Rajeshkumar, S., and Jagadeesh, K. (2023). Review of the environmentally friendly production of zinc oxide nanoparticles and its anti-oxidant, anti-hyperlipidemic, and antidiabetic properties. J. Survey in Fish. Sci. 10, 117–127. doi: 10.17762/sfs.v10i1S.154
Safawo, T., Sandeep, B., Pola, S., and Tadesse, A. (2018). Synthesis and characterization of zinc oxide nanoparticles using tuber extract of anchote (Coccinia abyssinica (lam.) Cong.) for antimicrobial and antioxidant activity assessment. OpenNano. 3, 56–63. doi: 10.1016/j.onano.2018.08.001
Sallama, A. E., Mansour, A. T., Alsaqufi, A. S., Salema, M. E. S., and El-Fekya, M. M. M. (2020). Growth performance, anti-oxidative status, innate immunity, and ammonia stress resistance of Siganus rivulatus fed diet supplemented with zinc and zinc nanoparticles. Aquac. Rep. 18:100410. doi: 10.1016/j.aqrep.2020.100410
Saravanan, M., Kumar, P., and Ramesh, M. (2011). Haematological and biochemical responses of freshwater teleost fish Cyprinus carpio (Actinopterygii: Cypriniformes) during acute and chronic sublethal exposure to lindane. Pestic. Biochem. Physiol. 100, 206–211. doi: 10.1016/j.pestbp.2011.04.002
Shah, B. R., and Mraz, J. (2020). Advances in nanotechnology for sustainable aquaculture and fisheries. Rev. Aquac. 12, 925–942. doi: 10.1111/raq.12356
Shahpar, Z., and Johari, S. A. (2019). Effects of dietary organic, inorganic, and nanoparticulate zinc on rainbow trout, Oncorhynchus mykiss larvae. Biol. Trace Elem. Res. 190, 535–540. doi: 10.1007/s12011-018-1563-z
Shahzad, K., Khan, M. N., Jabeen, F., Kosour, N., Sohail, M., Khan, M. K. A., et al. (2017). Bioaccumulation of manufactured titanium dioxide (TiO2), copper oxide (CuO) and zinc oxide (ZnO) nanoparticles in soft tissues of tilapia (Oreochromis mossambicus). Punjab Univ. J. Zool. 32, 237–243.
Sharma, V., Anderson, D., and Dhawan, A. (2012). Zinc oxide nanoparticles induce oxidative DNA damage and ROS-triggered mitochondria mediated apoptosis in human liver cells (HepG2). Apoptosis 17, 852–870. doi: 10.1007/s10495-012-0705-6
Suganthi, P., Murali, M., Sadiq, B. A., Syed, M. H. E., Basu, H. B., and Singhal, R. K. (2015). Haematological studies on freshwater tilapia treated with ZnO nanoparticles. J. Adv. Appl. Scienti. Res. 1, 41–67. doi: 10.46947/joaasr1120154
Swain, P., Das, R., Das, A., Padhi, S. K., Das, K. C., and Mishra, S. S. (2018). Effects of dietary zinc oxide and selenium nanoparticles on growth performance, immune responses and enzyme activity in rohu, Labeo rohita (Hamilton). Aquac. Nutr. 25, 486–494. doi: 10.1111/anu.12874
Tawfik, M. M. M., Moustafa, M. M., Abumourad, I. M. K., El-Meliegy, E. M., and Refai, M. K. (2017). Evaluation of nano zinc oxide feed additive on tilapia growth and immunity. 15th international conference on environmental science and technology Rhodes, Greece.
Tettey, C. O., and Shin, H. M. (2019). Evaluation of the antioxidant and cytotoxic activities of zinc oxide nanoparticles synthesized using Scutellaria baicalensis root. Sci. Afr. 6:e00157. doi: 10.1016/j.sciaf.2019.e00157
Thangapandiyan, S., and Monika, S. (2019). Green synthesized zinc oxide nanoparticles as feed additives to improve growth, biochemical, and hematological parameters in freshwater fish Labeo rohita. Biol. Trace Elem. Res. 195, 636–647. doi: 10.1007/s12011-019-01873-6
Umalatha Sridhar, N., Kushwaha, J. P., and Gangadhar, B. (2016). Digestive enzyme activities in different size groups and segments of the digestive tract in Labeo rohita (day, 1878). JAMB 4:00098. doi: 10.15406/jamb.2016.04.00098
Vani, T., Saharan, N., Mukherjee, S., Ranjan, R., Kumar, R., and Brahmchari, R. (2011). Deltamethrin induced alterations of hematological and biochemical parameters in fingerlings of Catla catla (ham.) and their amelioration by dietary supplement of vitamin C. Pestic. Biochem. Physiol. 101, 16–20. doi: 10.1016/j.pestbp.2011.05.007
Wang, Y., Li, K., Han, H., Zheng, Z., and Bureau, D. P. (2008). Potential of using a blend of rendered animal protein ingredients to replace fish meal in practical diets for Malabar grouper (Epinephelus malabaricus). Aquaculture 281, 113–117. doi: 10.1016/j.aquaculture.2008.03.033
Yonar, M. E., Mişe Yonar, S., İspir, Ü., and Ural, M. Ş. (2019). Effects of curcumin on haematological values, immunity, antioxidant status and resistance of rainbow trout (Oncorhynchus mykiss) against Aeromonas salmonicida subsp. achromogenes. Fish Shellfish Immunol. 89, 83–90. doi: 10.1016/j.fsi.2019.03.038
Younis, E. M., Abdel-Warith, A. A., and Al-Asgah, N. A. (2012). Hematological and enzymatic responses of Nile tilapia Oreochromis niloticus during short and long term sublethal exposure to zinc. Afr. J. Biotechnol. 11, 4442–4446. doi: 10.5897/ajb11.3987
Keywords: zinc-nanoparticles, feed formulation, growth performance, blood indices, enzymatic activity
Citation: Jewel AS, Haque A, Akter N, Akter S, Satter A, Sarker PK, Marshall DJ, Paray BA and Hossain MB (2024) Effects of dietary supplementation of Zn-nanoparticles on the growth performance and nutritional quality of Asian catfish, Clarias batrachus. Front. Sustain. Food Syst. 8:1410557. doi: 10.3389/fsufs.2024.1410557
Edited by:
Mehdi Raissy, Azad University, IranReviewed by:
Neeraj Kumar, National Institute of Abiotic Stress Management (ICAR), IndiaAhmed M. Saad, Zagazig University, Egypt
Randhir Makkar, Guild BioSciences, United States
Copyright © 2024 Jewel, Haque, Akter, Akter, Satter, Sarker, Marshall, Paray and Hossain. This is an open-access article distributed under the terms of the Creative Commons Attribution License (CC BY). The use, distribution or reproduction in other forums is permitted, provided the original author(s) and the copyright owner(s) are credited and that the original publication in this journal is cited, in accordance with accepted academic practice. No use, distribution or reproduction is permitted which does not comply with these terms.
*Correspondence: M. Belal Hossain, YmVsYWwuaG9zc2FpbkBuc3R1LmVkdS5iZA==; Pallab Kumer Sarker, cHNhcmtlckB1Y3NjLmVkdQ==