- Department of Botany, Faculty of Science, Dayalbagh Educational Institute, Agra, India
Agroecology has emerged as a fundamental paradigm for developing innovative solutions to major global concerns such as food security, biodiversity loss, and climate change. The core subject of biodiversity conservation emphasizes agroecology’s role in the preservation of native species, pollinators, and beneficial creatures through practices such as agroforestry, cover crops, and decreased chemical inputs. Agroecological principles such as polyculture, crop diversity, and integrated pest control help to improve food security by increasing stability and nutrition. Agroecology encourages carbon sequestration, soil health, and greenhouse gas reductions, resulting in climate-resilient farming systems. The literature review revealed that no article thoroughly discusses all of the critical hitting global challenges, such as food security, biodiversity loss, and climate change, in a single study that can be addressed using agroecological principles. This review article attempts to make a connection between numerous challenges that may be solved using agroecological techniques in order to facilitate sustainable growth while protecting the environment. The study examines the benefits of incorporating agroecological methodologies into sustainable agriculture, with an emphasis on increasing agroecosystem resilience, improving socioeconomic circumstances for smallholder farmers, rural livelihoods, and local communities, and contributing to climate change mitigation. It provides a complete review of agroecological concepts and their numerous advantages, making it an excellent guide for policymakers, academics, and practitioners who is working to build a sustainable and resilient global food system. Agroecology emerges as a light of hope, boosting agricultural output while also protecting the environment, with the ultimate goal of achieving harmonious cohabitation between mankind and the natural world.
Introduction
Agroecology integrates ecological principles into agriculture for sustainability. It promotes biodiversity, crop rotation, soil health, and natural pest control (Yadav et al., 2021). Agroforestry and local knowledge are valued (Saliu et al., 2023). This approach enhances food security, conserves biodiversity, mitigates climate change, and reduces environmental impact (Patel et al., 2020). Resilient farming systems and social equity are key components, supporting communities and traditions (Reyes et al., 2020). Agroecology offers a solution for sustainable agriculture, addressing food security, biodiversity loss, and climate change while prioritizing ecosystem and human well-being (De La Riva et al., 2023).
Major global challenges are interwoven with the security of our fundamental resources: food and water. Climate change and global warming disrupt weather patterns, resulting in extreme events that threaten agricultural productivity and amplify the vulnerability of already precarious food systems (Tofu et al., 2022). Biodiversity loss compounds these issues, as shrinking ecosystems undermine the resilience of ecosystems essential for pollination, soil health, and disease control. Moreover, agrochemicals, although intended to boost yields, can inadvertently harm ecosystems and human health, highlighting the demand for more environmentally friendly farming methods (World Health Organization, 2021). In order to solve these issues and guarantee the security of food and water, as well as the intricate interactions among human activity, the environment, and the delicate balance of nature, swift action is needed (Mishra et al., 2021).
Agroecological practices play a pivotal role in mitigating the impacts of climate change. Strategies such as carbon sequestration in soils, reduced dependence on synthetic fertilizers and pesticides, and the implementation of agroforestry systems collectively contribute to the reduction of greenhouse gas emissions (Muhie, 2022). Additionally, the diversified cropping systems fostered by agroecology enhance soil health and augment resilience against climate-induced stresses (Amede et al., 2023). Amid the concerning biodiversity loss, agroecology emerges as a beacon of hope. By advocating for diverse crop varieties, the establishment of mixed farming systems, and the preservation of habitats, agroecology actively promotes biodiversity (Calo et al., 2023). The reduction in chemical inputs and the promotion of natural pest control mechanisms not only enhance ecosystem health but also significantly contribute to the conservation of global biodiversity (Kremen, 2020).
In the realm of food security, agroecological practices play a vital role in ensuring sustainable nourishment. By bolstering soil fertility, encouraging crop diversity, and supporting local food production, agroecology acts as a buffer against the challenges posed by a changing climate (McLennon et al., 2021; Madsen et al., 2021). The emphasis on sustainable practices equips communities to adapt to evolving environmental conditions and ensures consistent access to wholesome food. Water scarcity, a growing concern, finds a potential ally in agroecology (Nabhan et al., 2020). The adoption of practices like rainwater harvesting, drip irrigation, and soil conservation techniques enables efficient water management. The improvement of soil structure and water retention capacities within agroecological systems not only curtails water demand but also maintains agricultural productivity (Odhiambo et al., 2021; Roohi et al., 2022). In addressing health pandemics, agroecology champions local and diversified food systems. By circumventing the reliance on intricate global supply chains, the spread of diseases can be mitigated. Moreover, the promotion of robust ecosystems through agroecological practices offers a shield against disease transmission risks (Barrett et al., 2020).
Agroecology’s impact on poverty reduction and inequality is profound (Kroll, 2021). By empowering small-scale farmers with knowledge and tools, agroecology diversifies income sources and promotes equitable opportunities. This translates into a tangible upliftment of livelihoods and the creation of a more inclusive society. Agroecology’s contribution to mitigating environmental degradation is of paramount importance. It decreases chemical pollution, prevents soil erosion, and promotes soil health through sustainable land management techniques (Anderson et al., 2022). The fight against environmental degradation that agroecology undertakes is characterised by the protection of ecosystems and the promotion of regenerative agriculture (Wojtynia et al., 2021).
Agroecology is a holistic approach (Figure 1) that tackles multiple global issues at once. Its emphasis on biodiversity, ecological sustainability, strengthening local communities, and developing resilient agricultural systems has the potential to bring back a harmonious relationship between humans and the environment (Chable et al., 2020). The shift towards holistic, locally suited farming practices forms the pivotal foundation of agroecology’s transformative contribution toward creating a world that is both more sustainable and equitable.
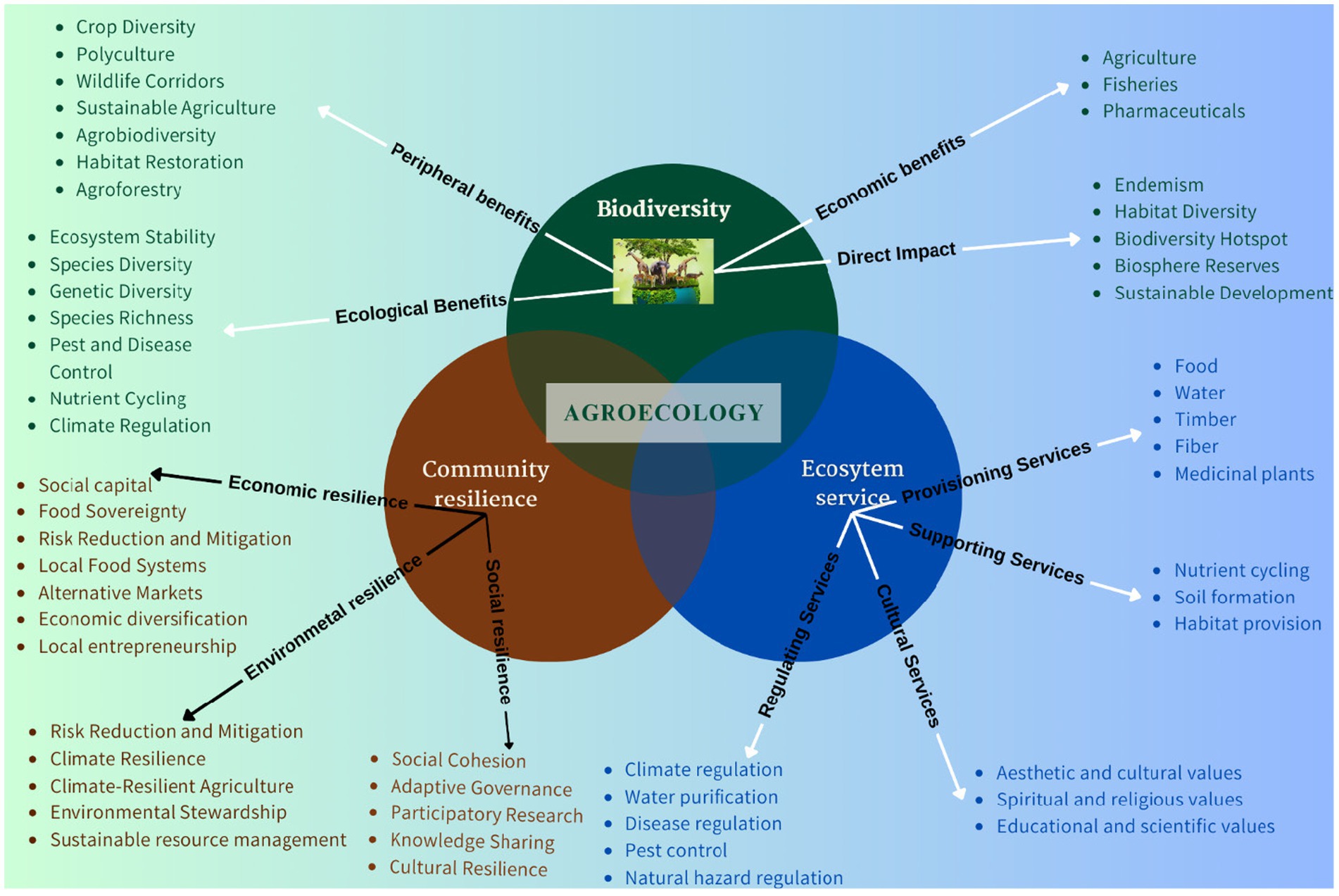
Figure 1. Agroecology’s multifaceted contribution to biodiversity and sustainable development. This figure illustrates how agroecology, as a holistic agricultural approach, interconnects with various components to foster biodiversity, community resilience.
Agroecology and food security
Agroecology significantly contributes to food security by promoting diverse food production through a wide array of crops and livestock, mitigating risks associated with single crop failures and disease outbreaks, thus ensuring a consistent food supply. It emphasizes localized, small-scale farming systems, reducing dependency on distant food sources and minimizing transportation-related losses (Figure 2). Furthermore, the ability of agroecological systems to adapt improves their resilience against climatic variability, strengthening their ability to endure catastrophic weather events and guaranteeing a consistent supply of food (Kyriakopoulos and Sebos, 2023; Karandish et al., 2021).
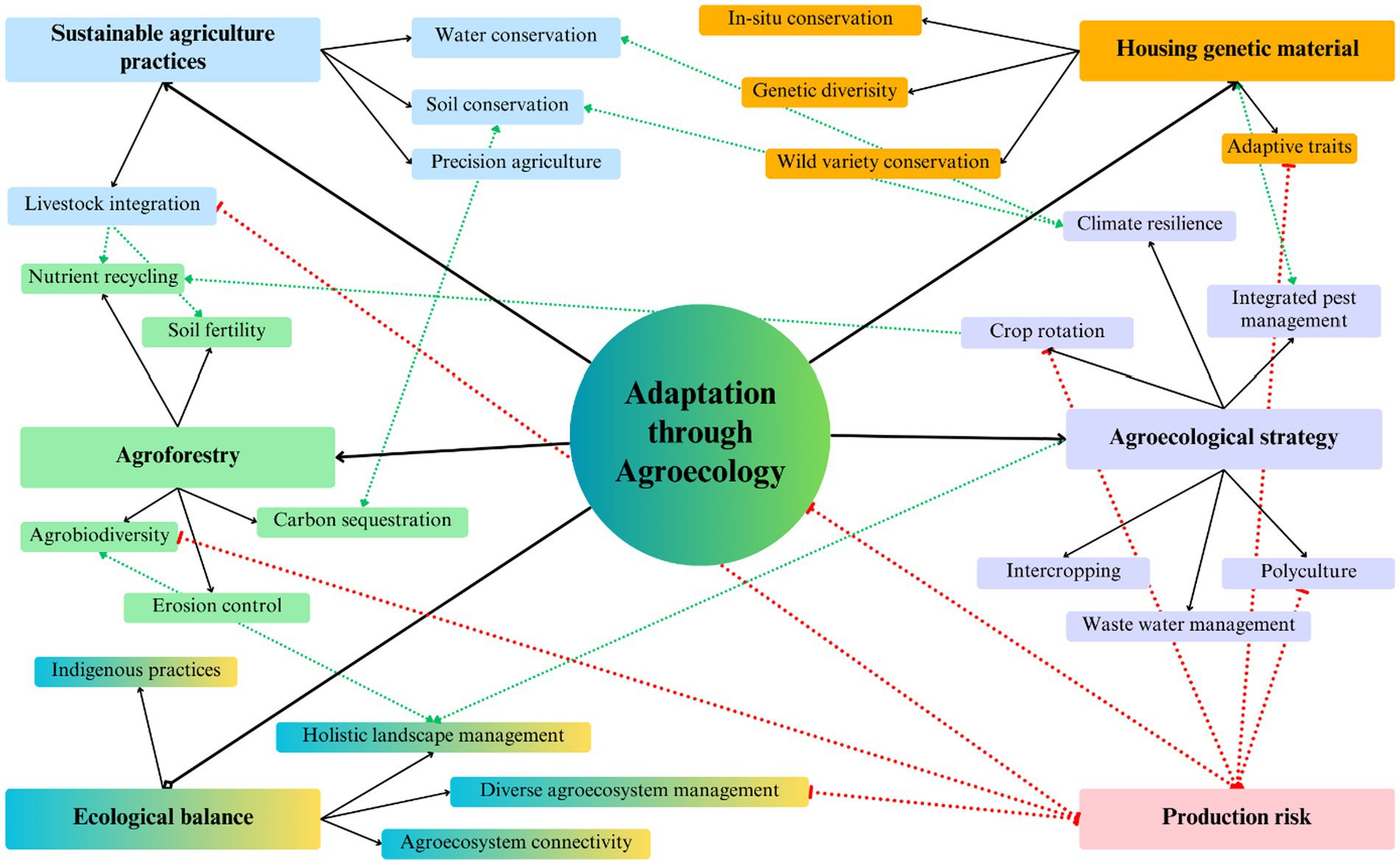
Figure 2. This figure illustrates the intricate web of interconnections among various sustainable agriculture practices, highlighting the synergy between different components of agroecology.
Role of agroecological practices in ensuring stable food production
Stable food production, denoting the consistent and dependable generation of food resources to fulfill the nutritional requirements of a populace, is fundamental for upholding food security, defined as the ample accessibility and attainment of adequate quantity and quality of food for all members of a society (Ayinde et al., 2020). Agroecological methodologies serve as a cornerstone in ensuring stable food production by fostering resilient, sustainable, and diversified agricultural systems. These practices engender stability through various mechanisms: Diversification mitigates vulnerability by advocating for a wide array of crops and livestock species, thus offsetting the impact of crop failures (Carlile and Garnett, 2021). Adaptive management encourages farmers to tailor their practices to local ecosystems, ensuring continued production amidst changing conditions (Sinclair et al., 2019). Resilience to climate change is bolstered through biodiversity, resource management, and ecological equilibrium, rendering agroecological systems more adept at withstanding environmental fluctuations (Jhariya et al., 2021; Solankey et al., 2021). Natural pest management techniques circumvent the pitfalls of chemical reliance, averting pest resistance and fostering long-term crop stability (Deguine et al., 2021). Soil health and fertility are upheld via techniques like cover cropping and crop rotation, providing a sturdy foundation for consistent yields (McLennon et al., 2021). Reduced input dependency insulates farmers from price fluctuations, stabilizing production costs and food prices (Bonnet et al., 2021). Localized production diminishes reliance on distant sources, curbing supply chain disruptions and ensuring stability within local communities (Grilli et al., 2023). Furthermore, the preservation of traditional knowledge integrated into agroecological practices harnesses generations of wisdom, contributing to stable production under diverse conditions (Einbinder et al., 2022).
Crop diversification, polyculture, and integrated pest management for enhanced yield stability
i. Crop diversification: Crop diversification involves planting a variety of crops within a farming system, diverging from monoculture. This practice mitigates risks by safeguarding against crop failures from pests or adverse weather (Wang et al., 2021; Kalinga et al., 2022). Diverse crops enhance resilience, as different species tolerate various conditions. Nutrient optimization is achieved through varied nutrient requirements and nitrogen-fixing crops. Pests and diseases are managed naturally, while soil health improves from diverse root structures (Thakur et al., 2023). Market opportunities expand with diverse produce, and cultural traditions are preserved. Adaptation to climate change is also facilitated. Diversification methods include intercropping, crop rotation, and polyculture. This sustainable approach aligns with agroecology, bolstering food systems’ resilience and sustainability (Kerr et al., 2023).
ii. Polyculture: Polyculture involves cultivating diverse crops together or in rotation, fostering biodiversity, nutrient cycling, and pest control. Unlike monoculture, it enhances ecosystem stability, resilience, and resource efficiency. With varied plants sharing resources, soil health improves, while risk from pests and climate variability decreases (Wilson and Lovell, 2016). Polyculture’s cultural diversity and ecological services, like carbon sequestration, amplify its value. Examples include the “Three Sisters” technique of corn, beans, and squash, and legume-cereal combos. This sustainable practice reflects agroecological principles, promoting resilient and productive food systems while preserving local traditions and enhancing ecosystem health (Al Aysh, 2016).
iii. Integrated pest management (IPM): Integrated pest management (IPM) is a sustainable approach to pest control in agriculture. It combines techniques like monitoring, prevention, cultural adjustments, biological control, and limited chemical use. IPM focuses on minimizing pests’ impact while reducing reliance on synthetic chemicals. Strategies include promoting natural predators, adjusting planting practices, and setting action thresholds. By considering ecosystem dynamics and long-term solutions, IPM maintains crop health, preserves beneficial species, and prevents pesticide resistance. This approach fosters resilient and environmentally friendly agricultural systems, ensuring both productivity and ecological balance (Kabir and Rainis, 2015; Lamichhane et al., 2015).
Improved nutritional outcomes through agroecology
Agroecology prioritizes improved nutritional outcomes by cultivating nutrient-rich crops, promoting biodiversity, and emphasizing traditional and local foods. Reduced chemical use ensures safer, healthier produce. Soil health enhances crop nutrition, while decentralized systems provide fresher foods (Hunter et al., 2019). Agroecology’s climate resilience sustains nutritional access amid environmental challenges. Empowering small-scale farmers improves food diversity and livelihoods. Knowledge-sharing fosters informed dietary choices. This approach integrates ecology, agriculture, and nutrition, contributing to better overall health and well-being.
Agrobiodiversity and adaptive capacity
Recognizing the significance of agrobiodiversity in adapting to evolving environmental circumstances is crucial for the establishment of robust and sustainable agricultural systems. Diverse crop varieties possess unique traits that enable them to endure stressors, aiding in breeding resilient strains (Kole et al., 2015; Dar et al., 2021). Agrobiodiversity aids climate adaptation by housing genetic material for crops thriving in evolving conditions. Examples highlight agrobiodiversity’s impact, from flood-resilient rice to adaptable tomatoes. Agroecological practices further reduce production risks by emphasizing diversification, integrated pest management, and soil health. Examples, including agroforestry in drought-prone regions and rice-fish farming, showcase successful implementation. These strategies collectively enhance adaptive capacity and promote sustainable food systems (Rao et al., 2016; Rozas et al., 2022).
Significance of agrobiodiversity in adapting to changing environmental conditions
The significance of agrobiodiversity in adapting to changing environmental conditions is paramount for resilient and sustainable agricultural systems. Agrobiodiversity, encompassing diverse plants, animals, and microorganisms within agroecosystems, plays a pivotal role due to its genetic resilience. Varied crop varieties possess unique traits that enable them to endure environmental stressors like drought, pests, and diseases, offering potential for breeding resilient strains (Adeleke and Babalola, 2021). Moreover, agrobiodiversity aids climate adaptation by housing genetic material for developing crops thriving in evolving conditions (Kozicka et al., 2020). Its scope extends to ecosystem services, crop and livestock health, nutritional diversity, local adaptation, and cultural heritage preservation. By reducing reliance on limited varieties, encouraging research and innovation, and fostering community-led conservation, agrobiodiversity becomes central to addressing uncertainties in the future. This ensures food security, ecological balance, and sustainable development (Agnoletti and Santoro, 2022).
Examples illustrate agrobiodiversity’s real-world impact. Traditional rice varieties like India’s “Sahbhagi Dhan” show flood resilience (Dar et al., 2021), aiding inundation-prone areas. Heirloom tomato types like “Brandywine” highlight adaptability to varied climates, while Icelandic sheep exhibit hardiness in harsh conditions (Swett et al., 2023). Community-driven initiatives, such as the “Slow Food Presidia,” safeguard crops like Andean “Tarwi,” preserving cultural heritage and genetics. These instances underscore agrobiodiversity’s rich genetic resources, equipping us to tackle environmental challenges while fostering sustainable food systems.
Agroecological practices reducing production risks
Diversification of crops and livestock, crop rotation, and agroforestry are core strategies employed to minimize risks. Natural pest control mechanisms and integrated pest management reduce reliance on chemical pesticides, mitigating the threat of pest resistance. Emphasis on soil health and local knowledge fortifies system adaptability (Bale et al., 2008; Harvey et al., 2014; Piwowar, 2021). Recent examples highlight the efficacy of agroecological practices. In response to climate change, farmers in drought-prone regions have adopted agroforestry, planting trees to alleviate water stress and buffer crops from extreme weather (Akinnagbe and Irohibe, 2014). In Cambodia, integrated rice-fish farming enhances pest control and boosts productivity (Halwart, 1998). In the drought-affected regions of Australia, agroecological practices have been successfully implemented to mitigate water scarcity impacts (Wakchaure et al., 2020). Farmers have adopted a combination of practices such as conservation tillage, cover cropping, and rotational grazing. These approaches enhance soil water retention and minimize water runoff, allowing crops and pastures to better withstand extended dry periods (Carr et al., 2013). The result has been increased resilience to drought conditions and reduced vulnerability to water-related production risks.
Agroecological strategies that promote adaptive capacity
These agroecological strategies have been put into practice worldwide, effectively enhancing the adaptive capacity of agricultural systems. Here are examples of each:
i. Diverse crop rotations: In the United States, the corn-soybean-wheat rotation is a classic agroecological strategy that has been widely adopted. This rotation involves alternating the cultivation of corn, soybeans, and wheat on the same field over consecutive seasons. This practice enhances soil fertility by varying nutrient demands and reducing pest buildup, leading to sustainable and resilient crop yields (Mirsky et al., 2013; Huang et al., 2021).
ii. Agroforestry systems: The “taungya” agroforestry system in Southeast Asia combines tree planting with crop cultivation (Paudel and Shrestha, 2022). In Indonesia, farmers plant fast-growing tree species alongside crops like maize and beans (Siarudin et al., 2021). The trees provide shade, improve soil fertility, and serve as a source of wood products. This strategy enhances local adaptation to climate changes and promotes ecosystem services.
iii. Climate-resilient crop varieties: The development of drought-tolerant maize varieties by the International Maize and Wheat Improvement Center (CIMMYT) has empowered farmers in sub-Saharan Africa to withstand changing climate patterns (Simtowe et al., 2019). These improved maize varieties have shorter growth cycles, enabling farmers to avoid drought periods and harvest more reliable yields.
iv. Water management techniques: In India, the System of Rice Intensification (SRI) promotes water-efficient rice cultivation. SRI uses reduced water inputs and spacing between plants, resulting in increased yields and decreased water requirements. This technique has helped farmers adapt to erratic monsoon patterns and water scarcity (Biswas et al., 2019).
v. Cover cropping and mulching: In Brazil, farmers practicing no-till agriculture have adopted cover cropping and residue mulching to improve soil structure and reduce erosion. These practices enhance water infiltration, reduce soil evaporation, and promote overall soil health, enabling adaptation to fluctuating rainfall patterns (Ofstehage and Nehring, 2021).
vi. Intercropping and polyculture systems: In parts of Africa, the “push-pull” intercropping system has been employed. It involves planting maize and sorghum intercropped with forage grasses and desmodium, a nitrogen-fixing legume. Desmodium repels stem borers from the maize and sorghum while providing nitrogen to the soil. This agroecological strategy enhances pest control and soil fertility (Khan et al., 2014).
vii. Traditional and local knowledge integration: The “zai” technique in Burkina Faso involves small pits filled with organic matter, which increase water retention and enhance soil fertility. This technique, based on local knowledge, enables farmers to adapt to drought conditions and improve crop yields (Danjuma and Mohammed, 2015).
viii. Livestock integration: In Australia, holistic planned grazing involves rotating livestock across pastures in a controlled manner. This practice promotes grassland health, reduces soil erosion, and enhances biodiversity. It adapts to changing climatic conditions by ensuring proper land management and sustainable forage utilization (Drewry and Paton, 2000).
Biodiversity conservation through agroecology
Agroecology plays a pivotal role in preserving native species and upholding biodiversity. This holistic approach resonates with ecological harmony and diversity, offering a unique platform for the harmonious coexistence of agricultural practices and native ecosystems. At its heart, agroecology encompasses an array of practices that collectively contribute to the creation of habitats, enhancement of natural pest control mechanisms, and the conservation of genetic diversity. This comprehensive overview delves into the paramount role that agroecology plays in conserving native species, supported by recent and effective implementations (Amekawa et al., 2010; Nikezic, 2022; Shukla et al., 2022). Table 1 presents a diverse range of agroecological activities across 20 countries, showcasing positive impacts on ecosystems. Examples include Spain’s “Dehesa” system, preserving critical habitats, and Thailand’s rice-fish farming enhancing aquatic biodiversity and providing additional income for farmers. These initiatives demonstrate agroecology’s potential to contribute to environmental sustainability and biodiversity conservation globally.
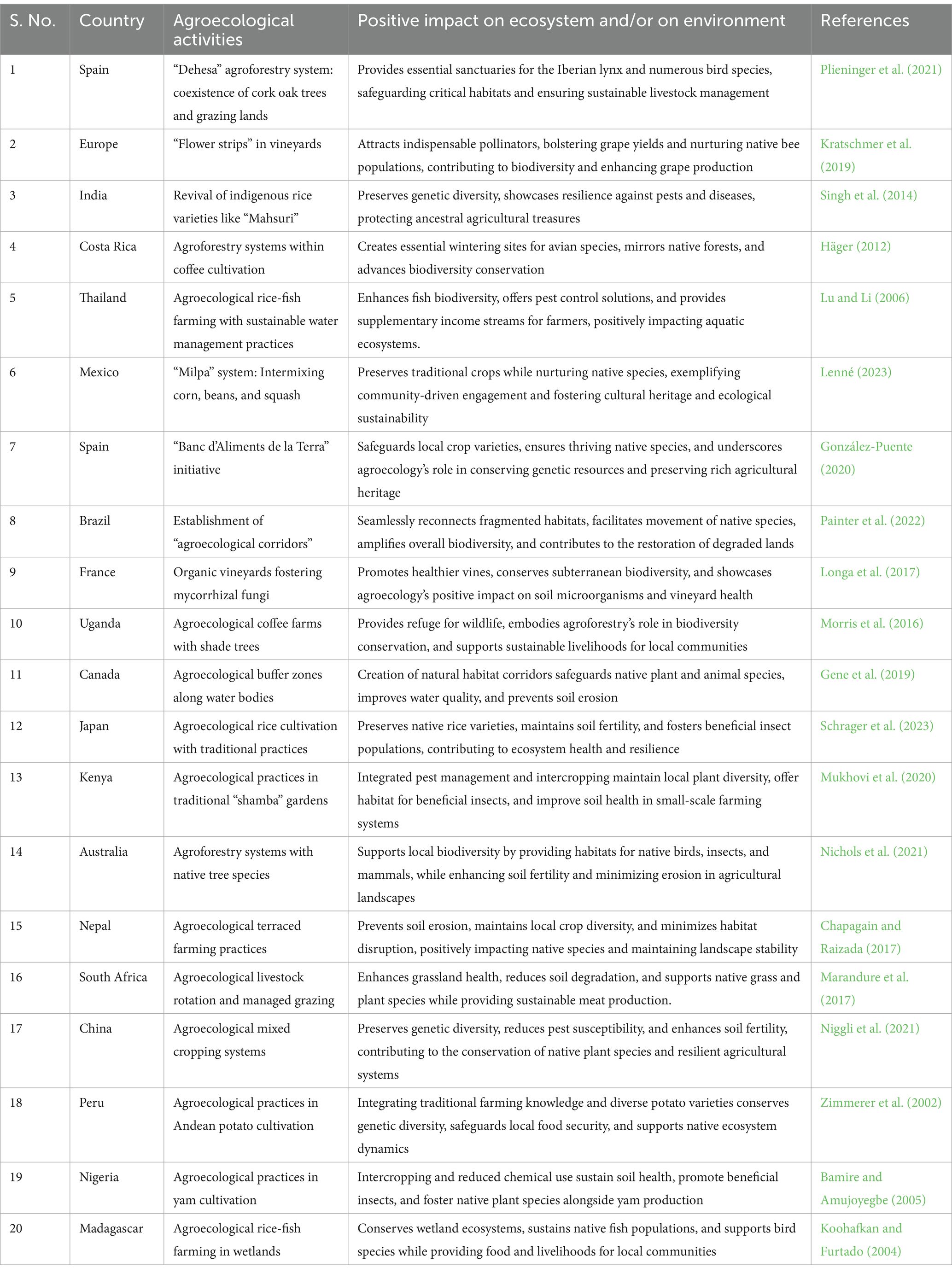
Table 1. Agroecological activities across countries and their positive impacts on ecosystems and the environment.
Biodiversity-boosting farming techniques
Agroforestry, cover cropping, and reduced chemical inputs are sustainable farming methods that significantly enhance biodiversity (Rosati et al., 2021). Agroforestry involves integrating trees into agricultural areas, providing habitats for wildlife and stabilizing soil (Yadav et al., 2019). Cover cropping, using diverse non-cash crops, protects soil from erosion, enriches it with organic matter, and fosters beneficial insects and pollinators. Minimizing synthetic pesticides and fertilizers reduces harm to non-target organisms, allowing natural pest control and soil health recovery (Kocira et al., 2020). These practices create multifaceted habitats, improve soil structure, and encourage diverse microbial communities. By mirroring natural ecosystems, these approaches establish resilient environments for various species. Together, they promote ecological balance while maintaining agricultural productivity.
Agroecology’s role in climate change mitigation
Agroecology fosters a symbiotic link between carbon sequestration and soil health, promoting practices like cover cropping and reduced tillage (Giri and Madla, 2017). By mitigating emissions through crop diversification and eco-friendly approaches, agroecology emerges as a resilient, community-driven solution, contingent on supportive policies and resources.
Carbon sequestration
The process of removing carbon dioxide (CO2) from the atmosphere and storing it for a long time is known as carbon sequestration. This process, as it relates to agriculture, entails storing carbon in soils, plants, and other natural systems after it has been captured through a variety of methods. Increasing the amount of organic matter in soils by techniques like cover crops, reduced tillage, and adding organic amendment is one of the main ways to sequester carbon (Nogia et al., 2016).
Soil health improvement
Soil health encompasses the physical, chemical, and biological well-being of soils. Healthy soils are more resilient, productive, and better able to support plant growth. Practices that improve soil health include adding organic matter, practicing crop rotation, reducing soil disturbance, and minimizing chemical inputs (El-Ramady et al., 2014). These practices enhance soil structure, water retention, nutrient availability, and beneficial microbial communities.
Carbon sequestration and soil health are closely linked. Increasing organic matter in soils not only stores carbon but also improves soil structure and water-holding capacity. This, in turn, supports plant growth, enhances nutrient cycling, and reduces erosion. Healthy soils with adequate organic matter provide a more favourable environment for beneficial soil organisms, such as earthworms and mycorrhizal fungi, which aid in nutrient uptake and contribute to overall ecosystem resilience.
Agroecological practices that reduce greenhouse gas emissions
Crop rotation and diversification interrupt disease and pest cycles, diminishing the reliance on synthetic pesticides and fertilizers (Tariq et al., 2019). Moreover, these practices bolster nutrient cycling, enrich soil health, and curtail the release of nitrous oxide, a potent greenhouse gas, from fertilized soils. Cover cropping, adopted during fallow periods, captures atmospheric carbon dioxide and mitigates soil erosion. Integration of agroforestry systems intertwines trees with crops or livestock, storing carbon within woody biomass and soil repositories. Trees also provide shading, ameliorating heat stress in livestock and crops and subsequently reducing emissions from energy-intensive cooling systems (Medhi et al., 2021).
Progressive livestock management, particularly rotational grazing, fosters pasture recovery and curtails methane emissions resulting from enteric fermentation. This strategy also enhances carbon sequestration in grasslands. Integrated pest management (IPM) prioritizes natural predators and beneficial insects for pest control, mitigating reliance on chemical pesticides. This approach maintains ecological equilibrium, limiting ecosystem disruption and potential greenhouse gas emissions linked to pesticide production and application (Alliance Environnement, 2019; Desneux et al., 2022).
Agroecological nutrient management emphasizes organic amendments, elevating soil organic matter content and diminishing synthetic fertilizer necessity. This, in turn, reduces emissions linked to fertilizer production and restrains nitrous oxide release from fertilized soils. Localized food systems decrease emissions linked to long-haul transportation and storage. Consumption of locally sourced produce enables communities to mitigate the carbon footprint of their diets. Traditional indigenous practices, grounded in generations of wisdom, often underscore agroecological techniques that maintain soil health and resource sustainability, resulting in reduced emissions compared to conventional methods (Raza et al., 2022; Enthoven and Van den Broeck, 2021).
Climate-resilient farming systems
Agroecology offers a promising path towards climate-resilient farming systems by integrating ecological principles into agriculture. Diversification of crops and livestock, prioritizing soil health through reduced tillage and organic practices, and promoting biodiversity for pollination and pest control are central to its approach (Leippert et al., 2020). Agroecology also emphasizes the use of traditional and locally adapted crop varieties, which are more resilient to changing climate patterns. By reducing dependency on external inputs and enhancing water management strategies, it helps farmers cope with water scarcity and extreme weather events (Sinclair et al., 2019). Moreover, agroecological practices foster community-based knowledge sharing, enabling flexible adaptation to local conditions. To fully unlock its potential, supportive policies, education, and resources are crucial for enabling farmers to implement these practices effectively.
Case studies and success stories
Diverse agroecological initiatives worldwide, showcasing their transformative impact on agriculture, livelihoods, and sustainability. Highlighting cases from Andhra Pradesh (India), Mexico, Malawi, Cuba, Asia, Africa, and Latin America, it illustrates how community-managed natural farming, research-driven programs, agroforestry, agroecological revolutions, systematic rice intensification, conservation tillage, and farmer-driven knowledge sharing contribute to enhanced food security, biodiversity preservation, and climate change mitigation.
Andhra Pradesh community managed sustainable agriculture
The Rythu Sadhikara Samstha, Government of Andhra Pradesh, is driving a transformation impacting 50 million people, including 6 million farmers and 8 million hectares of land. Named the Andhra Pradesh Community-Managed Natural Farming initiative, it shifts toward systemic agro-ecology, empowering small-scale farmers and maintaining ecological balance. Noteworthy for involving local communities, the initiative advocates agroecological practices like organic farming and integrated pest management. The initiative enhances farmer livelihoods, soil fertility, and biodiversity, demonstrating the integration of agroecological principles for resilience, sustainability, and community empowerment (Bharucha et al., 2020; Prabhu et al., 2022).
MasAgro in Mexico
MasAgro is a research for development program in Mexico that aims to increase the productivity and sustainability of maize and wheat production. It stands for “Mas Alimentos, Mas Ingresos, y Mas Sustentabilidad en el Agro” in Spanish, which translates to “More Food, More Income, and More Sustainability in Agriculture” in English. The program is designed to address the challenges of food security, poverty alleviation, and environmental sustainability (Martinez-Cruz, 2020; González-Abraham et al., 2023).
Agroforestry in Malawi
Intercropping maize with Faidherbia albida trees boosts soil fertility, yielding up to 280% more maize. The Changu Changu Moto Stove Project distributes efficient stoves, promoting sustainable woodlot management and curbing deforestation. Smallholders thrive by integrating fruit trees like mangoes, enhancing nutrition, income, and crop shading. These cases highlight agroforestry’s role in bolstering yields, curbing deforestation, and diversifying livelihoods. To harness its benefits, knowledge sharing, supportive policies, and research are vital. Malawi’s embrace of agroforestry promises a resilient and prosperous agricultural future (Ekyaligonza et al., 2022; Dierks et al., 2021).
Cuba’s Agroecological revolution
Cuba’s Agroecological Revolution has evolved over decades, reflecting a continuous journey of change and innovation. In the 1960s–1970s, the country leaned on chemicals and machinery, aided by the Soviet Union, embracing monoculture practices. The 1990s brought the “Special Period” post-Soviet collapse, inducing economic hardships. Without external support, agricultural reliance on imported resources declined, causing food production setbacks. Responding to the crisis, Cuba initiated sustainable alternatives in the 1990s. Organic and agroecological experiments surfaced, focusing on smaller-scale, self-reliant systems. In the late 1990s and 2000s, Cuba officially endorsed agroecology, championing urban farming via the “Programa de Agricultura Urbana.” The nation turned to diversified, community-based approaches, emphasizing local cultivation in home and urban gardens. Throughout the 2010s, agroecology’s influence expanded across urban and rural domains. Organic farming, traditional seed conservation, and natural pest control garnered attention. Vibrant farmers’ markets and local networks bolstered access to fresh produce (Altieri and Toledo, 2011; Machado, 2017).
Systematic Rice Intensification
The System of Rice Intensification (SRI) has achieved remarkable success across Asia. From India to China, Indonesia to Vietnam, and Bangladesh to Cambodia, SRI’s innovative practices have led to significant yield increases and improved sustainability (Upboff, 2008). By optimizing planting techniques, water management, and organic matter incorporation, SRI has become a vital tool in enhancing rice production, boosting food security, and promoting sustainable agricultural practices throughout the region. SRI has been practiced in various provinces of China, with substantial yield increases observed. Some regions have reported yield improvements of 30–50%. Chinese farmers have adapted SRI techniques to suit local conditions and water availability (Ly et al., 2012).
African Conservation Tillage Network
African Conservation Tillage Network: This network promotes conservation tillage practices across Africa, minimizing soil disturbance and maintaining crop residues on the field. These practices improve soil structure, water retention, and nutrient availability, leading to enhanced yields and reduced erosion (Steiner and Bwalya, 2003).
Campesino a campesino movement in Latin America
This movement facilitates knowledge sharing among small-scale farmers. By exchanging traditional farming practices and adapting them to local conditions, farmers across Latin America have improved their yields, diversified their crops, and reduced their reliance on external inputs (Holt-Giménez, 2006).
Challenges and barriers
Agroecological adoption encounters hurdles like knowledge gaps, technological constraints, and risk aversion. Policy challenges involve regulatory frameworks, limited support, and economic pressures, while cultural barriers include traditional beliefs and societal norms. Overcoming these challenges is vital for sustainable agriculture.
Obstacles in adopting agroecological practices
Agroecological practices represent a promising avenue for achieving sustainable agriculture, yet their widespread integration encounters multifaceted challenges requiring meticulous examination. A significant impediment lies in the gap of knowledge and awareness, as farmers, extension services, and policymakers often possess limited comprehension of agroecological principles, necessitating comprehensive educational initiatives for effective implementation (Leippert et al., 2020). Moreover, technological and infrastructural constraints hinder adoption, with farmers facing barriers in accessing requisite innovations, thereby impeding the transition from conventional methods (Boulestreau et al., 2021). Additionally, risk aversion stemming from uncertainties surrounding agroecology may dissuade farmers from embracing novel practices, mandating the demonstration of benefits and risk mitigation strategies (Chèze et al., 2020). Concerns over short-term yields further exacerbate reluctance, underscoring the importance of conveying the long-term advantages such as enhanced soil health and resilience (Kipling et al., 2019). Policy and market challenges compound the issue, with inadequate support and incentives discouraging agroecological adoption, necessitating policy interventions like subsidies and market promotion (Mockshell et al., 2023). Fragmentation of agroecological knowledge exacerbates adoption hurdles, highlighting the imperative of integrating and disseminating localized knowledge (Takahashi et al., 2020). Labor and time intensity associated with some practices pose additional barriers, particularly in regions grappling with labor shortages. Socioeconomic factors further complicate matters, as poverty, limited access to credit, and gender disparities inhibit adoption, underscoring the necessity for inclusive solutions addressing these challenges (Rao and Moharaj, 2023). Moreover, resistance to change, entrenched by farmers’ attachment to conventional practices, necessitates showcasing successful case studies and engaging farmers in experimentation to foster acceptance (Antwi-Agyei and Stringer, 2021). Lastly, efforts to scale up agroecological practices encounter limitations in extension services and technical support, warranting bolstering of these services to facilitate broader adoption (Autio et al., 2021).
Policy, economic, and cultural challenges
Agroecological practices represent a promising avenue for achieving sustainability and resilience in agricultural systems, yet their widespread adoption encounters multifaceted challenges spanning regulatory, economic, and cultural spheres. Regulatory frameworks, often shaped by industrial agricultural paradigms, may pose barriers to the incorporation of diverse and environmentally friendly methods (Morel et al., 2020). Additionally, limited government support, including insufficient funding, extension services, and incentives, impedes the transition to agroecology, particularly when policies favoring large-scale monocultures and chemical inputs are entrenched (Blair, 2020). Economic hurdles further complicate matters, as short-term profit pressures and inadequate access to resources deter risk-averse farmers from embracing agroecology, perpetuating disparities within the agricultural sector (Boswijk, 2021; Migliorati, 2023). Cultural challenges, including entrenched traditional beliefs and knowledge systems resistant to change, present significant obstacles, necessitating a nuanced approach to reconcile indigenous wisdom with modern agroecological insights (Swiderska et al., 2022). Moreover, social norms and perceptions, influenced by peer pressure and societal expectations favoring conventional practices, impede the widespread adoption of agroecological methods, underscoring the importance of fostering a shift in mindset and fostering social acceptance (Oteros-Rozas et al., 2019).
Future directions and recommendations
Promoting agroecology adoption involves diverse strategies like education, demonstration farms, policy support, research, extension services, market access, networks, incentives, partnerships, advocacy, and awareness. Crucial advancements in research, policy, and education underpin agroecological success.
Strategies to promote wider adoption of agroecology
Promoting the wider adoption of agroecology necessitates a comprehensive and interdisciplinary approach that encompasses agricultural, policy, educational, and community dimensions. Agroecology, grounded in sustainable and holistic farming principles that emulate natural ecosystems, holds promise for both environmental conservation and agricultural productivity. Strategies to foster its broader acceptance include educational initiatives aimed at empowering farmers with agroecological knowledge through workshops and resource dissemination, alongside the establishment of demonstration farms to tangibly exhibit the benefits of agroecology. Advocacy for pro-agroecology policies, such as incentivizing sustainable practices and integrating them into national agricultural frameworks, is imperative. Furthermore, investments in research and technological innovation are crucial for validating and optimizing agroecological methods. Strengthening extension services to provide tailored guidance on agroecology, developing local markets and supply chains for agroecological products, and fostering collaborative networks among farmers are essential components of the approach. Financial incentives, including grants and tax breaks, can serve as catalysts for farmers to embrace sustainable practices, while partnerships with various stakeholders amplify resources and outreach efforts. Advocating for policy changes grounded in evidence and promoting public awareness of agroecology’s multifaceted benefits are vital for driving its widespread adoption. Additionally, providing support and assistance to farmers transitioning to agroecological practices is essential for overcoming challenges and ensuring a smooth transition. Overall, a concerted effort across multiple fronts is indispensable in realizing the transformative potential of agroecology in modern agriculture.
Research, policy, and education to advance agroecological principles
Advancing agroecological principles necessitates a coordinated and multidisciplinary approach encompassing research, policy development, and education. These three key areas play a pivotal role in driving the adoption and integration of sustainable farming practices that align with agroecology’s principles.
i. Research: In research, prioritize comprehensive, long-term studies comparing agroecological practices to conventional methods. Beyond yields, assess ecological, economic, and social impacts. Rigorous research offers empirical evidence, highlighting agroecology’s advantages and challenges. Investigate its contributions to vital ecosystem services like pollination, soil health, and water conservation for broader adoption. Understand agroecological principles’ role in climate resilience, exploring diversified cropping and agroforestry’s impact on shifting climate patterns. Collaborate with indigenous communities, merging traditional knowledge with modern science to enhance sustainability. Explore innovative technologies aligned with agroecology, such as precision farming for low-input systems, providing practical solutions.
ii. Policy: In policy, fostering agroecology requires subsidies and incentives for transitioning from chemical-intensive methods. Clear standards and certifications boost credibility and adoption. Land tenure security prevents displacement. Adequate research funding empowers innovative, sustainable solutions. Mandating government food procurement from agroecological farms supports local farmers and sustainability.
iii. Education: In education, integrating agroecological principles into curricula nurtures informed agricultural professionals who understand sustainability. Training extension workers and farmer field schools offer practical support. Model farms exemplify agroecology’s success, while digital platforms share resources, benefiting those with limited formal education. This approach equips individuals with skills for implementing sustainable practices, driving agroecological adoption.
Conclusion
The review covers agroecology’s numerous contributions to biodiversity protection, climate change mitigation, sustainable food systems, and adaptive ability. Agroecological approaches, which emphasize crop diversification, polyculture, and integrated pest management, improve yield stability and resilience in food production. Furthermore, agroecology improves nutritional results while addressing global food security challenges. Agrobiodiversity emerges as a significant tool for reducing production risks and increasing adaptive ability in the context of changing environmental conditions. The interdependent link between agroecology and biodiversity conservation is investigated, illustrating how agroecological practices protect local species while increasing biodiversity in agricultural environments. Notably, agroecology aids in climate change mitigation by sequestering carbon, enhancing soil health, and lowering greenhouse gas emissions, so encouraging the development of climate-resilient farming systems. Case studies from varied locations like Andhra Pradesh, Mexico, Malawi, and Cuba demonstrate both the effectiveness and scalability of agroecological techniques in a variety of agricultural situations. Nonetheless, the analysis recognizes challenges to wider adoption, such as farm-level limits and broader legal, economic, and cultural problems. Overcoming these limitations is critical to realizing agroecology’s full potential in promoting sustainable agriculture. Looking ahead, the article proposes measures for integrating agroecology, such as encouraging research, adopting supporting policies, and expanding educational programmes to spread agroecological ideas. Finally, the review closes with a positive prognosis on agroecology’s revolutionary potential to advance sustainable and resilient agricultural systems.
Author contributions
Vikas: Conceptualization, Formal analysis, Writing – original draft. RR: Supervision, Writing – review & editing.
Funding
The author(s) declare that no financial support was received for the research, authorship, and/or publication of this article.
Acknowledgments
The authors wish to acknowledge the contributions of several individuals who have played a critical role in the successful completion of this study. The authors are grateful to the director of the institute for providing the necessary resources and support to conduct this study. The authors are also grateful to Ms. Shivangi Mathur and Ms. Deeksha Singh for their valuable time, effort, and feedback in writing and proofreading the manuscript.
Conflict of interest
The authors declare that the research was conducted in the absence of any commercial or financial relationships that could be construed as a potential conflict of interest.
Publisher’s note
All claims expressed in this article are solely those of the authors and do not necessarily represent those of their affiliated organizations, or those of the publisher, the editors and the reviewers. Any product that may be evaluated in this article, or claim that may be made by its manufacturer, is not guaranteed or endorsed by the publisher.
References
Adeleke, B. S., and Babalola, O. O. (2021). Biotechnological overview of agriculturally important endophytic fungi. Hortic. Environ. Biotechnol. 62, 507–520. doi: 10.1007/s13580-021-00334-1
Agnoletti, M., and Santoro, A. (2022). Agricultural heritage systems and agrobiodiversity. Biodivers. Conserv. 31, 2231–2241. doi: 10.1007/s10531-022-02460-3
Akinnagbe, O. M., and Irohibe, I. J. (2014). Agricultural adaptation strategies to climate change impacts in Africa: a review. Bangladesh J. Agric. Res. 39, 407–418. doi: 10.3329/bjar.v39i3.21984
Al Aysh, F. M. (2016). Intercropping is a missing ring for sustainability. LS Int. J. Life Sci. 5, 119–126. doi: 10.5958/2319-1198.2016.00018.X
Alliance Environnement (2019). Evaluation study of the impact of the CAP on climate change and greenhouse gas emissions. European Commission, Directorate-General for Agriculture and Rural Development: Brussels, Belgium.
Altieri, M. A., and Toledo, V. M. (2011). The agroecological revolution in Latin America: rescuing nature, ensuring food sovereignty and empowering peasants. J. Peasant Stud. 38, 587–612. doi: 10.1080/03066150.2011.582947
Amede, T., Konde, A. A., Muhinda, J. J., and Bigirwa, G. (2023). Sustainable farming in practice: building resilient and profitable smallholder agricultural systems in Sub-Saharan Africa. Sustainability 15:5731. doi: 10.3390/su15075731
Amekawa, Y., Sseguya, H., Onzere, S., and Carranza, I. (2010). Delineating the multifunctional role of agroecological practices: toward sustainable livelihoods for smallholder farmers in developing countries. Journal of Sustainable Agriculture 34, 202–228.
Anderson, J. R., Changxiao, L., Jiang, S., Chen, F., Yin, X., and He, J. (2022). Restoring and managing agricultural ecosystems as climate changes. A Compendium of Thematic Papers 6, 6–21.
Antwi-Agyei, P., and Stringer, L. C. (2021). Improving the effectiveness of agricultural extension services in supporting farmers to adapt to climate change: insights from northeastern Ghana. Clim. Risk Manag. 32:100304. doi: 10.1016/j.crm.2021.100304
Autio, A., Johansson, T., Motaroki, L., Minoia, P., and Pellikka, P. (2021). Constraints for adopting climate-smart agricultural practices among smallholder farmers in Southeast Kenya. Agric. Syst. 194:103284. doi: 10.1016/j.agsy.2021.103284
Ayinde, I. A., Otekunrin, O. A., Akinbode, S. O., and Otekunrin, O. A. (2020). Food security in Nigeria: impetus for growth and development. J. Agric. Econ. 6, 808–820.
Bale, J. S., Van Lenteren, J. C., and Bigler, F. (2008). Biological control and sustainable food production. Phil. Trans. R. Soc. B 363, 761–776. doi: 10.1098/rstb.2007.2182
Bamire, A. S., and Amujoyegbe, B. J. (2005). Economic analysis of land improvement techniques in smallholder yam-based production systems in the agro-ecological zones of Southwestern Nigeria. J. Hum. Ecol. 18, 1–12. doi: 10.1080/09709274.2005.11905799
Barrett, C. B., Benton, T. G., Fanzo, J., Herrero, M. T., Nelson, R., Bageant, E., et al. (2020). Socio-technical innovation bundles for Agri-food systems transformation. Cham: Palgrave Macmillan.
Bharucha, Z. P., Mitjans, S. B., and Pretty, J. (2020). Towards redesign at scale through zero budget natural farming in Andhra Pradesh, India. Int. J. Agric. Sustain. 18, 1–20. doi: 10.1080/14735903.2019.1694465
Biswas, B., Timsina, J., Patra, S. R., De, D., Mishra, B., Chakraborti, R., et al. (2019). Climate change-resilient rice production technology: a high yielding, water efficient and remunerative option for south Asian farmers. Global J. Agric. Allied sci. 1, 20–29. doi: 10.35251/gjaas.2019.003
Blair, E. (2020). “Overcoming barriers to agroecological practices: assessing the role of financial incentive programs in facilitating cover crop adoption” in Doctoral dissertation (Ann Arbor, MI: University of Michigan).
Bonnet, C., Gaudio, N., Alletto, L., Raffaillac, D., Bergez, J. E., Debaeke, P., et al. (2021). Design and multicriteria assessment of low-input cropping systems based on plant diversification in southwestern France. Agron. Sustain. Dev. 41, 1–19. doi: 10.1007/s13593-021-00719-7
Boswijk, K. D. (2021). “A farmers’ perspective on the tractions and frictions for transitioning to a more agroecological approach to dairy farming” in Master’s thesis (Utrecht: Utrecht University).
Boulestreau, Y., Casagrande, M., and Navarrete, M. (2021). Analyzing barriers and levers for practice change: a new framework applied to vegetables’ soil pest management. Agron. Sustain. Dev. 41:44. doi: 10.1007/s13593-021-00700-4
Calo, A., Shields, K., and Iles, A. (2023). Using property law to expand agroecology: Scotland’s land reforms based on human rights. J. Peasant Stud. 50, 2075–2111. doi: 10.1080/03066150.2022.2083506
Carlile, R., and Garnett, T. (2021). What is agroecology? TABLE explainer series. Oxford: TABLE, University of Oxford, Swedish University of Agricultural Sciences and Wageningen University & Research.
Carr, P. M., Gramig, G. G., and Liebig, M. A. (2013). Impacts of organic zero tillage systems on crops, weeds, and soil quality. Sustainability 5, 3172–3201. doi: 10.3390/su5073172
Chable, V., Nuijten, E., Costanzo, A., Goldringer, I., Bocci, R., Oehen, B., et al. (2020). Embedding cultivated diversity in society for agro-ecological transition. Sustainability 12:784. doi: 10.3390/su12030784
Chapagain, T., and Raizada, M. N. (2017). Agronomic challenges and opportunities for smallholder terrace agriculture in developing countries. Front. Plant Sci. 8:213080. doi: 10.3389/fpls.2017.00331
Chèze, B., David, M., and Martinet, V. (2020). Understanding farmers' reluctance to reduce pesticide use: a choice experiment. Ecol. Econ. 167:106349. doi: 10.1016/j.ecolecon.2019.06.004
Danjuma, M. N., and Mohammed, S. (2015). Zai pits system: a catalyst for restoration in the dry lands. J. Agric. Vet. Sci. 8, 1–4. doi: 10.9790/2380-08210104
Dar, M. H., Bano, D. A., Waza, S. A., Zaidi, N. W., Majid, A., Shikari, A. B., et al. (2021). Abiotic stress tolerance-progress and pathways of sustainable 602 rice production. Sustainability 13:2078. doi: 10.3390/su13042078
de la Riva, E. G., Ulrich, W., Batáry, P., Baudry, J., Beaumelle, L., Bucher, R., et al. (2023). From functional 605 diversity to human well-being: a conceptual framework for agroecosystem 606 sustainability. Agric. Syst. 208:103659. doi: 10.1016/j.agsy.2023.103659
Deguine, J. P., Aubertot, J. N., Flor, R. J., Lescourret, F., Wyckhuys, K. A., and Ratnadass, A. (2021). 608 integrated pest management: good intentions, hard realities. A review. Agron. Sustain. Dev. 41:38. doi: 10.1007/s13593-021-00689-w
Desneux, N., Han, P., Mansour, R., Arnó, J., Brévault, T., Campos, M. R., et al. (2022). Integrated pest management of Tuta absoluta: 612 practical implementations across different world regions. J. Pest. Sci. 95, 17–39. doi: 10.1007/s10340-021-01442-8
Dierks, J., Blaser-Hart, W. J., Gamper, H. A., Nyoka, I. B., Barrios, E., and Six, J. (2021). Trees enhance 614 abundance of arbuscular mycorrhizal fungi, soil structure, and nutrient retention in low-input maize 615 cropping systems. Agric. Ecosyst. Environ. 318:107487. doi: 10.1016/j.agee.2021.107487
Drewry, J. J., and Paton, R. J. (2000). Effects of cattle treading and natural amelioration on soil physicalproperties and pasture under dairy farming in Southland, New Zealand. N. Z. J. Agric. Res. 43, 377–386. doi: 10.1080/00288233.2000.9513438
Einbinder, N., Morales, H., Mier y Terán Giménez Cacho, M., Ferguson, B. G., Aldasoro, M., and Nigh, R. (2022). Agroecology from the ground up: a critical analysis of sustainable soil management in the highlands of Guatemala. Agric. Hum. Values 39, 979–996. doi: 10.1007/s10460-022-10299-1
Ekyaligonza, D. M., Kahigwa, T. T., Dietrich, P., Akoraebirungi, B., Kagorora, J. P., Friedel, J. K., et al. (2022). Biomass contribution and nutrient recycling of organic matter management practices in tropical smallholder annual farming systems. Acta Agric. Scand. B 72, 945–956. doi: 10.1080/09064710.2022.2134819
El-Ramady, H. R., Alshaal, T. A., Amer, M., Domokos-Szabolcsy, É., Elhawat, N., Prokisch, J., et al. (2014). “Soil quality and plant nutrition” in Sustainable agriculture reviews 14: agroecology and global change (Cham: Springer), 345–447.
Enthoven, L., and Van den Broeck, G. (2021). Local food systems: reviewing two decades of research. Agric. Syst. 193:103226. doi: 10.1016/j.agsy.2021.103226
Gene, S. M., Hoekstra, P. F., Hannam, C., White, M., Truman, C., Hanson, M. L., et al. (2019). The role of vegetated buffers in agriculture and their regulation across Canada and the United States. J. Environ. Manag. 243, 12–21. doi: 10.1016/j.jenvman.2019.05.003
Giri, R. K. K. V., and Madla, V. R. (2017). Study and evaluation of carbon sequestration using remote sending and GIS: a review on various techniques. Int. J. Civil Eng. Technol. 8, 287–300.
González-Abraham, C., Flores-Santana, C., Rodríguez-Ramírez, S., Olguín-Álvarez, M., Flores-Martínez, A., Torres Rojo, J. M., et al. (2023). Long-term pathways analysis to assess the feasibility of sustainable land-use and food systems in Mexico. Sustain. Sci. 18, 469–484. doi: 10.1007/s11625-022-01243-7
González-Puente, M. (2020). Accés a la terra, desagrarització i reagrarització: discursos des d’un banc de terres al Camp de Tarragona. Arxiu d’Etnografia de Catalunya 21, 69–96.
Grilli, G., Cofré, N., Marro, N., Videla, M., and Urcelay, C. (2023). Shifts from conventional horticulture to agroecology impacts soil fungal diversity in Central Argentina. Mycol. Prog. 22:20. doi: 10.1007/s11557-023-01872-x
Häger, A. (2012). The effects of management and plant diversity on carbon storage in coffee agroforestry systems in Costa Rica. Agrofor. Syst. 86, 159–174. doi: 10.1007/s10457-012-9545-1
Harvey, C. A., Chacon, M., Donatti, C. I., Garen, E., Hannah, L., Andrade, A., et al. (2014). Climate-smart landscapes: opportunities and challenges for integrating adaptation and mitigation in tropical agriculture. Conserv. Lett. 7, 77–90. doi: 10.1111/conl.12066
Holt-Giménez, E. (2006). Campesino a Campesino: voices from Latin America’s farmer to farmer movement for sustainable agriculture. New York: Food First Books.
Huang, Y., Tao, B., Xiaochen, Z., Yang, Y., Liang, L., Wang, L., et al. (2021). Conservation tillage increases corn and soybean water productivity across the Ohio River basin. Agric. Water Manag. 254:106962. doi: 10.1016/j.agwat.2021.106962
Hunter, D., Borelli, T., Beltrame, D. M., Oliveira, C. N., Coradin, L., Wasike, V. W., et al. (2019). The potential of neglected and underutilized species for improving diets and nutrition. Planta 250, 709–729. doi: 10.1007/s00425-019-03169-4
Jhariya, M. K., Meena, R. S., and Banerjee, A. (2021). “Ecological intensification of natural resources towards sustainable productive system” in Ecological intensification of natural resources for sustainable agriculture (Singapore: Springer), 1–28.
Kabir, M. H., and Rainis, R. (2015). Adoption and intensity of integrated pest management (IPM) vegetable farming in Bangladesh: an approach to sustainable agricultural development. Environ. Dev. Sustain. 17, 1413–1429. doi: 10.1007/s10668-014-9613-y
Kalinga, A. S., Kangalawe, R. Y., and Lyimo, J. G. (2022). The significance of the diversification of food crops in abating impacts of climate variability: the case of Rungwe District, Tanzania. J. Geogr. Assoc. Tanzania 41, 1–21. doi: 10.56279/jgat.v41i2.191
Karandish, F., Nouri, H., and Brugnach, M. (2021). Agro-economic and socio-environmental assessments of food and virtual water trades of Iran. Sci. Rep. 11:15022. doi: 10.1038/s41598-021-93928-9
Kerr, R. B., Postigo, J. C., Smith, P., Cowie, A., Singh, P. K., Rivera-Ferre, M., et al. (2023). Agroecology as a transformative approach to tackle climatic, food, and ecosystemic crises. Curr. Opin. Environ. Sustain. 62:101275. doi: 10.1016/j.cosust.2023.101275
Khan, Z. R., Midega, C. A., Pittchar, J. O., Murage, A. W., Birkett, M. A., Bruce, T. J., et al. (2014). Achieving food security for one million sub-Saharan African poor through push–pull innovation by 2020. Philosophical Transactions of the Royal Society B: Biological Sciences 369:20120284.
Kipling, R. P., Topp, C. F., Bannink, A., Bartley, D. J., Blanco-Penedo, I., Cortignani, R., et al. (2019). To what extent is climate change adaptation a novel challenge for agricultural modellers? Environ. Model Softw. 120:104492. doi: 10.1016/j.envsoft.2019.104492
Kocira, A., Staniak, M., Tomaszewska, M., Kornas, R., Cymerman, J., Panasiewicz, K., et al. (2020). Legume cover crops as one of the elements of strategic weed management and soil quality improvement. A review. Agriculture 10:394. doi: 10.3390/agriculture10090394
Kole, C., Muthamilarasan, M., Henry, R., Edwards, D., Sharma, R., Abberton, M., et al. (2015). Application of genomics-assisted breeding for generation of climate resilient crops: progress and prospects. Front. Plant Sci. 6:153364. doi: 10.3389/fpls.2015.00563
Koohafkan, P., and Furtado, J. (2004). Traditional rice-fish systems as globally important ingenious agricultural heritage systems. Int. Rice Comm. Newsl. 53, 66–73.
Kozicka, M., Gotor, E., Ocimati, W., de Jager, T., Kikulwe, E., and Groot, J. C. (2020). Responding to future regime shifts with agrobiodiversity: a multi-level perspective on small-scale farming in Uganda. Agric. Syst. 183:102864. doi: 10.1016/j.agsy.2020.102864
Kratschmer, S., Pachinger, B., Schwantzer, M., Paredes, D., Guzmán, G., Goméz, J. A., et al. (2019). Response of wild bee diversity, abundance, and functional traits to vineyard inter-row management intensity and landscape diversity across Europe. Ecol. Evol. 9, 4103–4115. doi: 10.1002/ece3.5039
Kremen, C. (2020). Ecological intensification and diversification approaches to maintain biodiversity, ecosystem services and food production in a changing world. Emerg. Top. Life Sci. 4, 229–240. doi: 10.1042/ETLS20190205
Kroll, F. (2021). Agroecology and the metropolitan biopolitics of food in Cape Town and Johannesburg. Urban Agriculture & Regional Food Systems 6:e20010.
Kyriakopoulos, G. L., and Sebos, I. (2023). Enhancing climate neutrality and resilience through coordinated climate action: review of the synergies between mitigation and adaptation actions. Climate 11:105. doi: 10.3390/cli11050105
Lamichhane, J. R., Arendse, W., Dachbrodt-Saaydeh, S., Kudsk, P., Roman, J. C., van Bijsterveldt-Gels, J. E., et al. (2015). Challenges and opportunities for integrated pest management in Europe: a telling example of minor uses. Crop Prot. 74, 42–47. doi: 10.1016/j.cropro.2015.04.005
Leippert, F., Darmaun, M., Bernoux, M., Mpheshea, M., Müller, A., Geck, M., et al. (2020). The potential of agroecology to build climate-resilient livelihoods and food systems. Rome: FAO.
Lenné, J. (2023). Current agricultural diversification strategies are already agroecological. Outlook Agric. 52, 273–280. doi: 10.1177/00307270231199796
Longa, C. M., Nicola, L., Antonielli, L., Mescalchin, E., Zanzotti, R., Turco, E., et al. (2017). Soil microbiota respond to green manure in organic vineyards. J. Appl. Microbiol. 123, 1547–1560. doi: 10.1111/jam.13606
Lu, J., and Li, X. (2006). Review of rice-fish-farming systems in China—one of the globally important ingenious agricultural heritage systems (GIAHS). Aquaculture 260, 106–113. doi: 10.1016/j.aquaculture.2006.05.059
Ly, P., Jensen, L. S., Bruun, T. B., Rutz, D., and de Neergaard, A. (2012). The system of rice intensification: adapted practices, reported outcomes and their relevance in Cambodia. Agric. Syst. 113, 16–27. doi: 10.1016/j.agsy.2012.07.005
Machado, M. R. (2017). Alternative to what? Agroecology, food sovereignty, and Cuba’s agricultural revolution. Hum. Geogr. 10, 7–21. doi: 10.1177/194277861701000302
Madsen, S., Bezner Kerr, R., Shumba, L., and Dakishoni, L. (2021). Agroecological practices of legume residue management and crop diversification for improved smallholder food security, dietary diversity and sustainable land use in Malawi. Agroecol. Sustain. Food Syst. 45, 197–224. doi: 10.1080/21683565.2020.1811828
Marandure, T., Mapiye, C., Makombe, G., and Dzama, K. (2017). Indicator-based sustainability assessment of the smallholder beef cattle production system in South Africa. Agroecology and Sustainable Food Systems 41, 3–29.
Martinez-Cruz, T. E. (2020). “On continuities and discontinuities: the making of technology-driven interventions and the encounter with the MasAgro Programme in Mexico” in Doctoral dissertation (Wageningen: Wageningen University and Research).
McLennon, E., Dari, B., Jha, G., Sihi, D., and Kankarla, V. (2021). Regenerative agriculture and integrative permaculture for sustainable and technology driven global food production and security. Agron. J. 113, 4541–4559. doi: 10.1002/agj2.20814
Medhi, K., Bhardwaj, R., and Laxmi, R. (2021). “Climate change with its impacts on soil and soil microbiome regulating biogeochemical nutrient transformations” in Climate change and the microbiome. Soil biology (Cham: Springer), 95–138.
Migliorati, L. (2023). “Exploring the impacts of the institutionalization of agroecology for a just and fair transition in India and Senegal” in Master’s thesis (Enschede: University of Twente).
Mirsky, S. B., Ryan, M. R., Teasdale, J. R., Curran, W. S., Reberg-Horton, C. S., Spargo, J. T., et al. (2013). Overcoming weed management challenges in cover crop– based organic rotational no-till soybean production in the eastern United States. Weed Technol. 27, 193–203. doi: 10.1614/WT-D-12-00078.1
Mishra, B. K., Kumar, P., Saraswat, C., Chakraborty, S., and Gautam, A. (2021). Water security in a changing environment: concept, challenges and solutions. Water 13:490. doi: 10.3390/w13040490
Mockshell, J., Ogutu, S., Alvarez, D., Nielsen, T., Steinke, J., Remans, R., et al. (2023). Transitioning to agroecological food systems: a review of incentives for adoption of agroecological practices and outcomes. Montpellier: CGIAR.
Morel, K., Revoyron, E., San Cristobal, M., and Baret, P. V. (2020). Innovating within or outside dominant food systems? Different challenges for contrasting crop diversification strategies in Europe. PLoS One 15:e0229910. doi: 10.1371/journal.pone.0229910
Morris, K. S., Méndez, V. E., Zonneveld, M. V., Gerlicz, A., and Caswell, M. (2016). Agroecology and climate change resilience: in smallholder coffee agroecosystems of Central America. Rome, Italy: Bioversity International.
Muhie, S. H. (2022). Novel approaches and practices to sustainable agriculture. J. Agric. Food Res. 10, –100446. doi: 10.1016/j.jafr.2022.100446
Mukhovi, S. M., Jacobi, J., Llanque, A., Rist, S., Delgado, F., Kiteme, B., et al. (2020). Social self-organization and social-ecological resilience in food systems: lessons from smallholder agriculture in Kenya and indigenous Guaraní communities in Bolivia. Food Stud. 10, 19–42. doi: 10.18848/2160-1933/CGP/v10i01/19-42
Nabhan, G. P., Riordan, E. C., Monti, L., Rea, A. M., Wilder, B. T., Ezcurra, E., et al. (2020). An Aridamerican model for agriculture in a hotter, water scarce world. Plants People Planet 2, 627–639. doi: 10.1002/ppp3.10129
Nichols, J. D., Lee, S. M., Reid, R., and Grant, J. C. (2021). “Ecosystem services from agroforestry systems in Australia” in Agroforestry and ecosystem services (Cham: Springer), 431–449.
Niggli, U., Wang-Müller, Q., Willer, H., and Fuchs, J. (2021). Innovation in agroecological and organic farming systems. Chinese Journal of Eco-Agriculture 29, 423–430.
Nikezic, A. (2022). Enhancing Biocultural Diversity of Wild Urban Woodland through Research-Based Architectural Design: Case Study—War Island in Belgrade, Serbia. Sustainability 2022:11445.
Nogia, P., Sidhu, G. K., Mehrotra, R., and Mehrotra, S. (2016). Capturing atmospheric carbon: biological and nonbiological methods. Int. J. Low-Carbon Technol. 11, 266–274. doi: 10.1093/ijlct/ctt077
Odhiambo, K. O., Iro Ong'or, B. T., and Kanda, E. K. (2021). Optimization of rainwater harvesting system design for smallholder irrigation farmers in Kenya: a review. J. Water Supply: Res. Technol. - AQUA 70, 483–492. doi: 10.2166/aqua.2021.087
Ofstehage, A., and Nehring, R. (2021). No-till agriculture and the deception of sustainability in Brazil. Int. J. Agric. Sustain. 19, 335–348. doi: 10.1080/14735903.2021.1910419
Oteros-Rozas, E., Ravera, F., and García-Llorente, M. (2019). How does agroecology contribute to the transitions towards social-ecological sustainability? Sustainability 11:4372. doi: 10.3390/su11164372
Painter, K. R., Buschbacher, R., Souto Silva, L. C., and Costa e Silva, E. (2022). “Agroecology and Forest conservation in three types of land reform communities in the cacao region of Bahia, Brazil” in Biodiversity islands: strategies for conservation in human-dominated environments (Cham: Springer), 569–599.
Patel, S. K., Sharma, A., and Singh, G. S. (2020). Traditional agricultural practices in India: an approach or environmental sustainability and food security. Energy Ecol. Environ. 5, 253–271. doi: 10.1007/s40974-020-00158-2
Paudel, Y., and Shrestha, S. (2022). Agroforestry practices prevailing in SAARC countries: a review. Indones. J. Soc. Environ. Issues 3, 10–18. doi: 10.47540/ijsei.v3i1.390
Piwowar, A. (2021). The use of pesticides in polish agriculture after integrated pest management (IPM) implementation. Environ. Sci. Pollut. Res. 28, 26628–26642. doi: 10.1007/s11356-020-12283-w
Plieninger, T., Flinzberger, L., Hetman, M., Horstmannshoff, I., Reinhard-Kolempas, M., Topp, E., et al., (2021). Dehesas as high nature value farming systems: a social-ecological synthesis of drivers, pressures, state, impacts, and responses.
Prabhu, R., Dhyani, S. K., Nayak, D., and Rizvi, J. (2022). “Transformative agroecology-based alternatives for a sustainable and biodiverse future” in Indian agriculture towards 2030 (Singapore: Springer), 183.
Rao, C. S., Gopinath, K. A., Prasad, J. V. N. S., and Singh, A. K. (2016). Climate resilient villages for sustainable food security in tropical India: concept, process, technologies, institutions, and impacts. Adv. Agron. 140, 101–214. doi: 10.1016/bs.agron.2016.06.003
Rao, R., and Moharaj, P. (2023). Empowering women in climate-resilient farming through sustainable agriculture technologies. Int. J. Multidiscip. Res. Growth Eval. 4, 157–265.
Raza, S. T., Wu, J., Rene, E. R., Ali, Z., and Chen, Z. (2022). Reuse of agricultural wastes, manure, and biochar as an organic amendment: a review on its implications for vermicomposting technology. J. Clean. Prod. 360:132200. doi: 10.1016/j.jclepro.2022.132200
Reyes, S. R. C., Miyazaki, A., Yiu, E., and Saito, O. (2020). Enhancing sustainability in traditional agriculture: indicators for monitoring the conservation of globally important agricultural heritage systems (GIAHS) in Japan. Sustainability 12:5656. doi: 10.3390/su12145656
Roohi, M., Arif, M. S., Guillaume, T., Yasmeen, T., Riaz, M., Shakoor, A., et al. (2022). Role of fertilization regime on soil carbon sequestration and crop yield in a maize-cowpea intercropping system on low fertility soils. Geoderma 428:116152. doi: 10.1016/j.geoderma.2022.116152
Rosati, A., Borek, R., and Canali, S. (2021). Agroforestry and organic agriculture. Agrofor. Syst. 95, 805–821. doi: 10.1007/s10457-020-00559-6
Rozas, P., Kessi-Pérez, E. I., and Martínez, C. (2022). Genetically modified organisms: adapting regulatory frameworks for evolving genome editing technologies. Biol. Res. 55:31. doi: 10.1186/s40659-022-00399-x
Saliu, F., Luqman, M., and Alkhaz’leh, H. S. (2023). A review on the impact of sustainable agriculture practices on crop yields and soil health. Int. J. Res. Adv.Agric. Sci. 2, 1–13.
Schrager, B., Ikeda, H., and Yukitsugu, T. (2023). Successes and challenges of a university-based agroecological community garden and educational program in Japan. J. Agric. Food Syst. Community Dev. 12, 1–15. doi: 10.5304/jafscd.2023.124.011
Shukla, J., Dhyani, S., Pujari, P., Mishra, A., and Verma, P. (2022). Impact of agriculture intensification on forest degradation and tree carbon stock; promoting multi‐criteria optimization for restoration in Central India. Land Degradation & Development 33, 3103–3117.
Siarudin, M., Rahman, S. A., Artati, Y., Indrajaya, Y., Narulita, S., Ardha, M. J., et al. (2021). Carbon sequestration potential of agroforestry systems in degraded landscapes in West Java, Indonesia. Forests 12:714. doi: 10.3390/f12060714
Simtowe, F., Amondo, E., Marenya, P., Sonder, K., and Erenstein, O. (2019). Impacts of drought-tolerant maize varieties on productivity, risk, and resource use: evidence from Uganda. Land Use Policy 88:104091. doi: 10.1016/j.landusepol.2019.104091
Sinclair, F., Wezel, A., Mbow, C., Chomba, S., Robiglio, V., and Harrison, R. (2019). The contribution of agroecological approaches to realizing climate-resilient agriculture. Rotterdam: Global Commission on Adaptation.
Singh, R. K., Singh, A., and Pandey, C. B. (2014). Agro-biodiversity in rice–wheat-based agroecosystems of eastern Uttar Pradesh, India: implications for conservation and sustainable management. Int. J. Sustain. Dev. World Ecol. 21, 46–59. doi: 10.1080/13504509.2013.869272
Solankey, S. S., Kumari, M., Akhtar, S., Singh, H. K., and Ray, P. K. (2021). “Challenges and opportunities in vegetable production in changing climate: mitigation and adaptation strategies” in Advances in research on vegetable production under a changing climate (Cham: Springer), 13–59.
Steiner, K., and Bwalya, M. (2003). “The African conservation tillage network enhancing conservation tillage in Africa” in Conservation agriculture (Dordrecht: Environment, Farmers Experiences, Innovations, Socio-economy, Policy, Springer), 111–122.
Swett, C. L., Del Castillo Múnera, J., Hellman, E., and Rodriguez, F. (2023). Monitoring for a new I3 resistance gene-breaking race of F. oxysporum f. sp. lycopersici (Fusarium wilt) in California processing tomatoes following recent widespread adoption of resistant (F3) cultivars: challenges with race 3 and 4 differentiation methods. Front. Plant Sci. 14:1088044. doi: 10.3389/fpls.2023.1088044
Swiderska, K., Argumedo, A., Wekesa, C., Ndalilo, L., Song, Y., Rastogi, A., et al. (2022). Indigenous peoples’ food systems and biocultural heritage: addressing indigenous priorities using decolonial and interdisciplinary research approaches. Sustainability 14:11311. doi: 10.3390/su141811311
Takahashi, K., Muraoka, R., and Otsuka, K. (2020). Technology adoption, impact, and extension in developing countries’ agriculture: a review of the recent literature. Agric. Econ. 51, 31–45. doi: 10.1111/agec.12539
Tariq, M., Ali, H., Hussain, N., Nasim, W., Mubeen, M., Ahmad, S., et al. (2019). “Fundamentals of crop rotation in agronomic management” in Agronomic crops (Singapore: Springer), 545–559.
Thakur, N., Nigam, M., Mann, N. A., Gupta, S., Hussain, C. M., Shukla, S. K., et al. (2023). Host-mediated gene engineering and microbiome-based technology optimization for sustainable agriculture and environment. Funct. Integr. Genomics 23:57. doi: 10.1007/s10142-023-00982-9
Tofu, D. A., Woldeamanuel, T., and Haile, F. (2022). Smallholder farmers’ vulnerability and adaptation to climate change induced shocks: the case of northern Ethiopia highlands. J. Agric. Food Res. 8:100312:100312. doi: 10.1016/j.jafr.2022.100312
Upboff, N. (2008). The System of Rice intensification (SRI) as a system of agricultural innovation. Jurnal Ilmu Tanah dan Lingkungan 10, 27–40. doi: 10.29244/jitl.10.1.27-40
Urs, N., Qiyan, W. M., Helga, W., and Jacques, F. (2021). Innovation in agroecological and organic farming systems. Chin. J. Eco-Agric. 29, 423–430. doi: 10.13930/j.cnki.cjea.200469
Wakchaure, G. C., Minhas, P. S., Meena, K. K., Kumar, S., and Rane, J. (2020). Effect of plant growth regulators and deficit irrigation on canopy traits, yield, water productivity and fruit quality of eggplant (Solanum melongena L.) grown in the water scarce environment. J. Environ. Manag. 262:110320. doi: 10.1016/j.jenvman.2020.110320
Wang, G., Bei, S., Li, J., Bao, X., Zhang, J., Schultz, P. A., et al. (2021). Soil microbial legacy drives crop diversity advantage: linking ecological plant-soil feedback with agricultural intercropping. J. Appl. Ecol. 58, 496–506. doi: 10.1111/1365-2664.13802
Wilson, M. H., and Lovell, S. T. (2016). Agroforestry—the next step in sustainable and resilient agriculture. Sustainability 8:574. doi: 10.3390/su8060574
Wojtynia, N., van Dijk, J., Derks, M., Groot Koerkamp, P. W., and Hekkert, M. P. (2021). A new green revolution or agribusiness as usual? Uncovering alignment issues and potential transition complications in agri-food system transitions. Agron. Sustain. Dev. 41:77. doi: 10.1007/s13593-021-00734-8
World Health Organization (2021). Nature, biodiversity and health: an overview of interconnections. Bonn: World Health Organization.
Yadav, S. K., Banerjee, A., Jhariya, M. K., Raj, A., Khan, N., Meena, R. S., et al. (2021). “Agroecology towards environmental sustainability” in Sustainable intensification for agroecosystem services and management (Cham: Springer), 323–352.
Yadav, A., Gendley, M. K., Sahu, J., Patel, P. K., Chandraker, K., and Dubey, A. (2019). Silvopastoral system: a prototype of livestock agroforestry. Pharma Innov. J. 8, 76–82.
Zimmerer, K., Cleveland, D. A., and Soleri, D. (2002). “Social and agroecological variability of seed production and the potential collaborative breeding of potatoes in the Andean countries” in Farmers, scientists, and plant breeding: integrating knowledge and practice. eds. D. A. Cleveland and D. Soleri (Wallingford: CABI Publishing), 83–106.
Keywords: agroecology, biodiversity, climate change, food security, sustainable development
Citation: Ranjan R (2024) Agroecological approaches to sustainable development. Front. Sustain. Food Syst. 8:1405409. doi: 10.3389/fsufs.2024.1405409
Edited by:
Pankaj Kumar Arora, M. J. P. Rohilkhand University, IndiaReviewed by:
Ebrahim Jahanshiri, Crops For the Future UK, United KingdomBruno Condori, Universidad Pública de El Alto, Bolivia
Copyright © 2024 Vikas and Ranjan. This is an open-access article distributed under the terms of the Creative Commons Attribution License (CC BY). The use, distribution or reproduction in other forums is permitted, provided the original author(s) and the copyright owner(s) are credited and that the original publication in this journal is cited, in accordance with accepted academic practice. No use, distribution or reproduction is permitted which does not comply with these terms.
*Correspondence: Rajiv Ranjan, cmFqaXZyYW5qYW5idEBnbWFpbC5jb20=