- Agronomy Department, Federal University of Espírito Santo, Alegre, Brazil
Phosphorus (P) is found in the soil in organic (Po) and inorganic (Pi) forms and in compartments with different lability, normally being separated into labile, moderately labile, restricted lability and non-labile forms. The different sources of fertilizers used in the long term may change the form and availability of P over time, increasing or not its availability to plants, which can positively influence coffee productivity. This work aimed to evaluate in the field the effects of phosphate fertilizers with associated technology on the availability of P in soil with high P adsorption capacity after successive fertilizations in Coffea canephora Pierre ex A.Froehner crop. In the experiment, three phosphate sources (conventional MAP – MAP, polymer-coated MAP – MAPPOL and MAP + filter cake – MAPTF); two doses (100 and 150 % of the recommendation); and two nitrogen sources (conventional urea – UC and urea coated with polymer and sulfur – UPS) were used. After 3 years of field experimentation, the soil was collected at a depth of 0–10 cm and then, the labile, moderately labile, and restricted lability fractions were determined. Phosphate fertilizers with associated technology obtained better results in relation to MAP in terms of Pi and Po contents for the labile compartment over time, with an average increase of 66.02 mg dm−3 P in the soil. For the moderately labile fraction, fertilizers with associated technology increased the Pi content (HCl 1M) in the soil in relation to MAP, by 2.56 mg dm−3, representing an increase of 37.92%. These compartments are considered an important reserve of available P for crops grown in soils with high adsorption capacity. Phosphate fertilizers with integrated protection (MAPPOL + MAPTF) compared to MAP increased productivity by 6.79 and 11.62 % in the 2017/2018 and 2018/2019 harvests, respectively. Phosphate fertilizers with associated technology promote P dynamics in different soil compartments, increasing Coffea canephora Pierre ex A. Froehner productivity and sustainability.
1 Introduction
The total area destined for coffee growing in Brazil in 2023, for arabica and conilon coffee, totals 2.24 million hectares. With a 70.2% share of coffee production in the country, it is estimated that 38.16 million bags of Arabica coffee will be harvested, representing an increase of 16.6% over the previous harvest. For conilon coffee, estimated production is 16.2 million bags of 60 kg, with a 10% reduction in productivity due to adverse weather conditions (Companhia Nacional de Abastecimento - CONAB, 2019). The growing need to increase crop productivity to attend the population's demand for coffee results in a greater consumption of phosphate fertilizers and the need to increase their efficiency, given that P reserves are not renewable.
In Brazil, coffee growing is one of the crops with the highest consumption of fertilizers. Among the nutrients applied, P is considered the most limiting nutrient in tropical soils. These soils, specifically the Oxisols, are characterized by a marked degree of weathering, with iron (Fe) and aluminum (Al) oxides predominating in the clay fraction. This condition gives them a high capacity for adsorption of P (Abdala et al., 2015; De Campos et al., 2016), causing low availability of this nutrient in the soil solution (Fink et al., 2014) and low efficiency of use of P fertilizer in crops, which is around 20%, ensuring the need to be applied in quantities greater than those required by the crop. In this scenario, adequate fertilizer management is essential for the efficient use of P and the sustainability of coffee growing.
Currently, technological alternatives are being sought to increase the efficiency of phosphate fertilizers, such as covering the soluble fertilizer granule with polymers or biochars or adding organic material, such as filter cake (Borges et al., 2019; Li et al., 2020; Lustosa Filho et al., 2020). The new technologies have the potential to minimize environmental impact and improve the efficiency of fertilizer use. Besides that, they increase the nutrient release process to soil, reducing the movement of P from labile to non-labile forms, promoting greater P absorption by plants and improving crop productivity/sustainability.
The efficiency of technologies associated with the soluble source of P consists in altering the kinetics of nutrient release into the soil. This allows its transport and the minimization of reactions between P and the soil components that surround the fertilizer granules. By altering the kinetics of P release there is also a reduction in the contact time of P with Fe and Al oxides, favoring release over a longer period, making it more available to plants (Gazola et al., 2013).
Phosphorus fertilization management influences P availability to the plants. Then, there is a need to know how P is affected by different soil management and long-term fertilization strategies (Qiong et al., 2022).
In the soil, P occurs in inorganic (Pi) and organic (Po) forms, with different degrees of lability (Dalai, 1977), namely: labile fraction, moderately labile fraction; restricted lability fraction and non-labile fraction, all with their organic and inorganic compartments (Hedley et al., 1982; Camargo et al., 1999). Knowledge of these compartments has the advantage of obtaining information about the availability of P in the short, medium, and long term, and can also be used to monitor changes in the forms of P in the soil (Araujo et al., 1993), especially in situations involving the use of fertilizers and soil management (Rheinheimer et al., 2000).
Oliveira et al. (2022) found that P levels in the soil varied depending on the sources (MAP and polymer-coated MAP) and doses applied to sugarcane, with the coated MAP providing the highest nutrient levels (12.72 mg dm−3 P) while conventional MAP presented a content of 10.89 mg dm−3 P in a readily labile compartment.
In this scenario, it is important to understand how each of the phosphate fertilizer technologies can act on soil P dynamics, increasing P availability and improving fertilizer efficiency in coffee crops, making the production process more sustainable. This work aimed to evaluate P compartments through the application of phosphate fertilizers with associated technology after successive fertilization in coffee crops and its productivity/sustainability.
2 Material and methods
The experiment was carried out using Coffea canephora Pierre ex A.Froehner as a test plant, grown on a farm in the municipality of Jerônimo Monteiro - ES (20°49′58′′ S and 41°24′51′′ W. The soil in the region is a Argissolo Vermelho Amarelo, the relief is wavy and the region's climate is Cwa, according to Koppen's classification (Alvares et al., 2013). Precipitation (mm) and temperature (°C) data are presented in Figure 1.
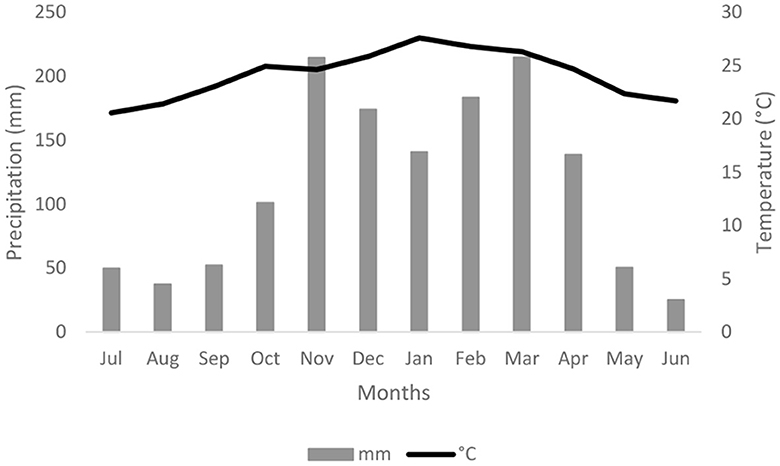
Figure 1. Average rainfall (mm) and temperature (°C) in the municipality of Jerônimo Monteiro – ES during the 2017/2018 and 2018/2019 harvests.
The cultivation was carried out during the 2017/2018 and 2018/2019 harvests. The coffee plants were 5 years old and the spacing used was 2.5 × 1.5 m. During the experimental period, no irrigation was performed.
The research was carried out with 12 treatments (3 × 2 × 2), with three replications, so that the total experimental units were 36 samples, with six coffee plants per experimental unit. The experimental design was in randomized complete block design, with the factors being: three phosphate fertilizer sources (conventional monoammonium phosphate – MAP, Kimcoat® polymer-coated MAP – MAPPOL and MAP + filter cake – MAPTF), two doses, being 100 and 150% of the recommended phosphate fertilizer for coffee plants according to Prezotti et al. (2007) and two nitrogen sources (conventional urea – UC and urea coated with polymer and sulfur – UPS). The experimental design is shown in the following Figure 2.
Soil samples were collected (depth of 0–20 cm), in the projection of the plant crown, for physical (Teixeira et al., 2017) and soil chemical (Silva and Da Silva, 2009) characterization in the 2017/2018 and 2018/2019 harvests. After the analyses, liming was applied on the soil surface, in the projection of the plant crown, to increase base saturation to 60 % (Prezotti et al., 2007) in each coffee harvest.
Fertilization with N and P was carried out for a productivity of 4,260–6,000 kg ha−1 according to Prezotti et al. (2007), which corresponds to a dose per harvest of 440 and 75 kg ha−1 of N, P2O5, respectively. The amount of phosphate fertilizer for the 100 % dose is 75 kg ha−1 of P2O5 and for the 150 % dose it is 112.5 kg ha−1 of P2O5.
After two harvests had elapsed, soil was collected in the projection of the coffee tree crown, at a depth of 0–10 cm, to do P fractionation and determine the organic and inorganic P compartments in all experimental units. The method developed by Hedley et al. (1982) was used, with adaptations (Condron et al., 1985; Araujo et al., 1993; Tiessen and Moir, 1993; Cross and Schlesinger, 1995; Camargo et al., 1999) described by Andrade and de Villani (2017). This method aims to determine the labile (readily available), moderately labile (medium-term availability) and restricted lability (long-term availability) forms. At the end, the sequential extraction of P aimed to determine inorganic P (Pi) and total P (Pt) in each extractor, except in the first stage, which is the anion exchange resin, in which the P adsorbed to the resin was quantified. Organic P (Porg) was determined by the difference between Pt and Pi.
The collected 0.5 g of soil was used to perform the extraction steps, the first of which was extraction in anion exchange resin, followed by NaHCO3 0,5 mol L−1 pH 8,5, NaOH 0,1 mol L−1, HCl 1 mol L−1, NaOH 0,5 mol L−1. The final step consisted of digesting 0.25 g of remaining soil to determine the residual P in the extract, according to Figure 3. Pi and Porg contents were determined at different degrees of lability for each of the extractors and evaluated their relationship with the technologies of phosphate and nitrogen fertilizer used, specially their effect on P availability after successive crops.
The coffee fruits were harvested to determine the productivity of each treatment, starting when 90% of the fruits were ripe. The fruits of the four central plants of each treatment were harvested, washed and separated into green and mature grains and then weighed individually. Subsequently, a simple sample of 0.5 kg of mature grains was taken from each plant to form a composite sample of 2 kg per treatment. The samples were dried in a forced circulation oven at 45°C until obtaining humidity between 11 and 12%, controlled using a Gehaka AGRI model G800 humidity meter. Subsequently, the samples were piled and weighed to obtain productivity in kg/ha.
The data were subjected to analysis of variance and the treatments were evaluated by the F test at levels of 5 and 10% probability and evaluated by comparing means using orthogonal contrasts (Alvarez and Alvarez, 2006), according to Table 1. For data analysis, the statistical program R was used.
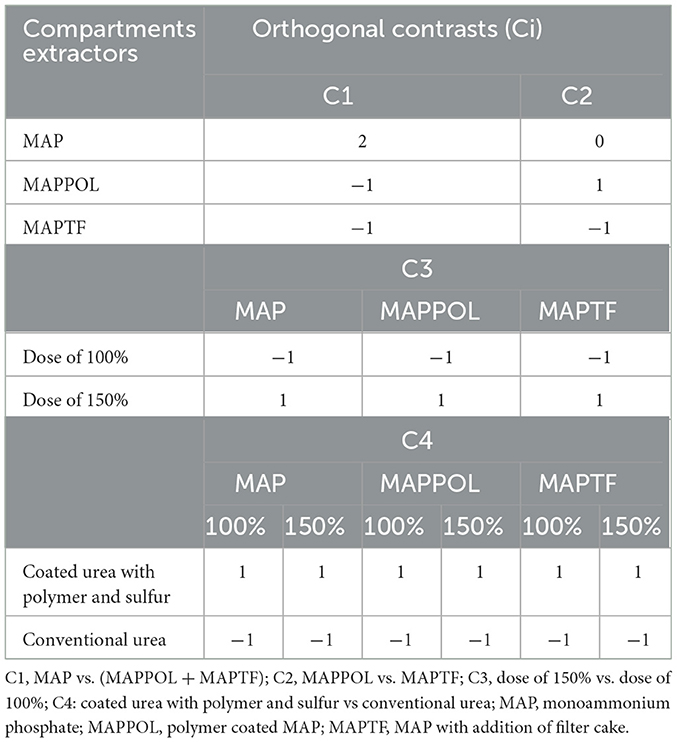
Table 1. Orthogonal contrast (Ci) of P compartments and extractors, considering phosphate fertilizers, doses of P and nitrogen fertilizers.
3 Results
The P content in the soil varied depending on the phosphate sources applied to the conilon coffee crop (Table 2). In general, the associated technologies promoted higher P content in the readily labile and restricted lability compartment (C1, Table 3). MAP, in turn, increased the P content in the non-labile fraction in relation to associated technologies (C1, Table 3).
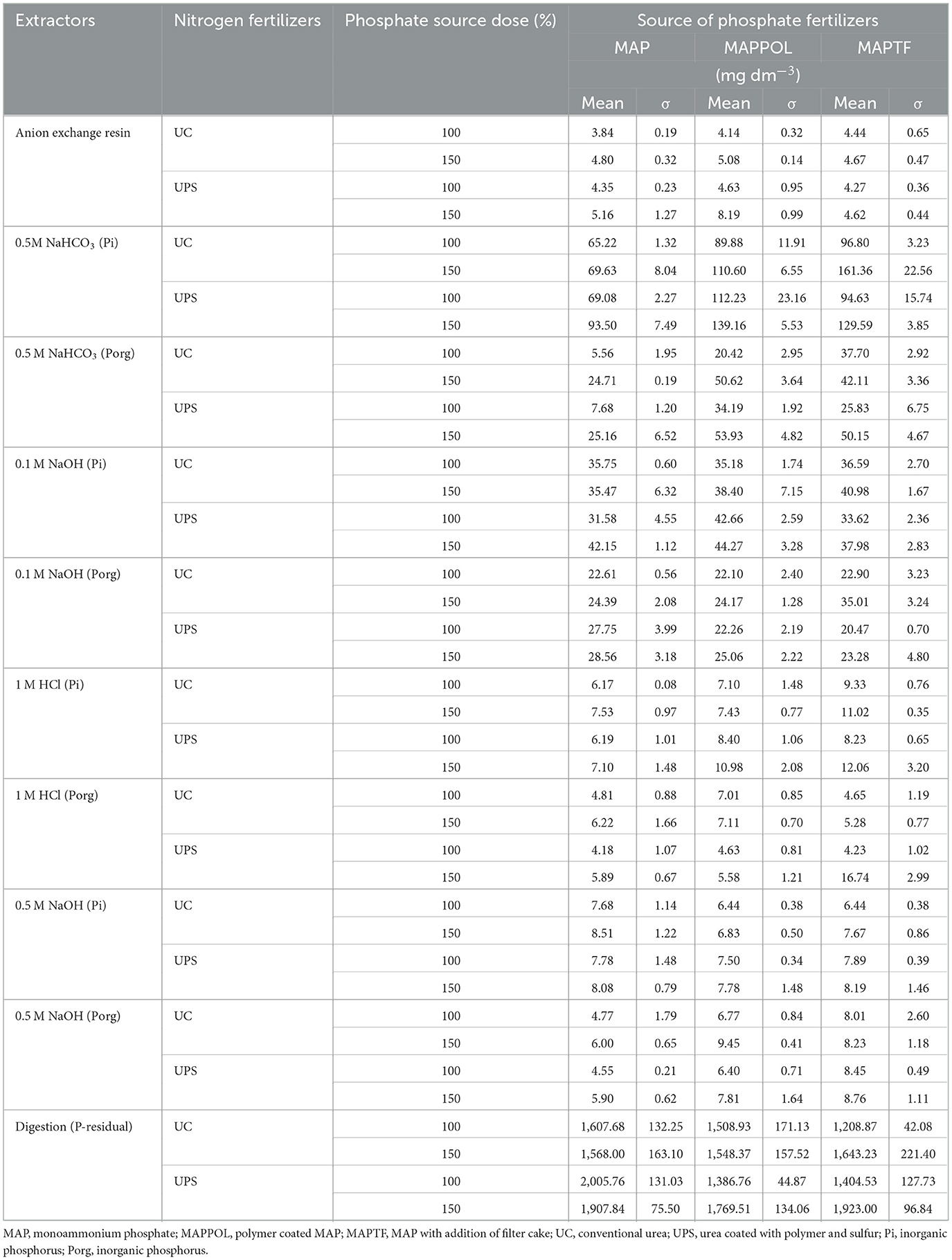
Table 2. Average levels and standard deviation of available P depending on the extractors after application of phosphate fertilizers considering the doses and N fertilizers.
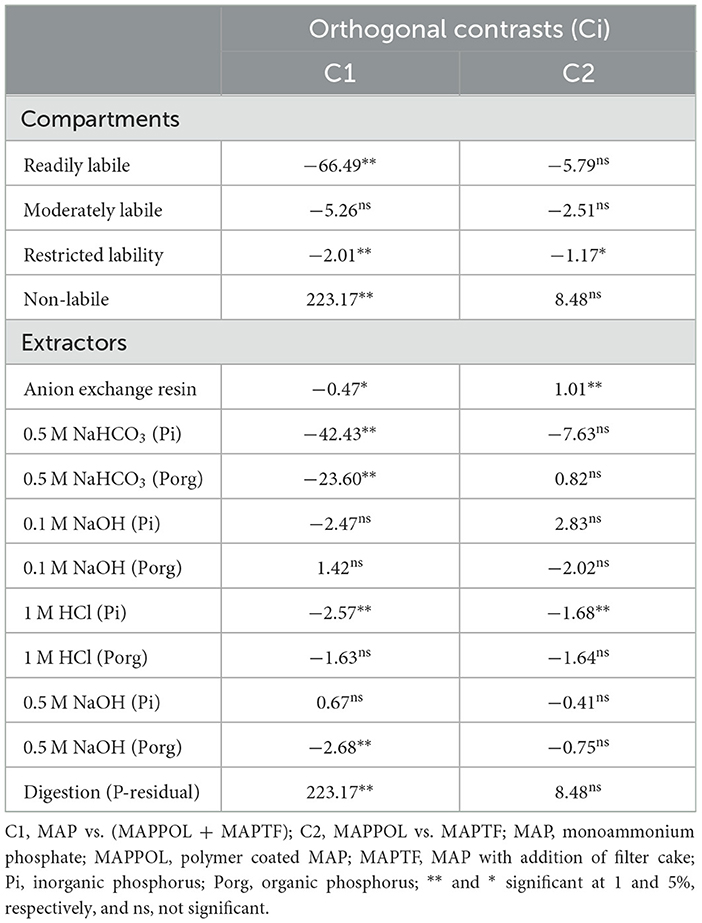
Table 3. Orthogonal contrasts of P extractors considering phosphate fertilizers regardless of the amounts of phosphate fertilizers and N fertilizers.
Phosphate fertilizers with associated technology provided higher levels of readily labile Pi and Porg (extracted in 0.5M NaHCO3) in relation to MAP (C1, Table 3). In turn, fertilizers with associated technology did not differ in terms of readily labile P content (C2, Table 3). The MAP presented an average of 74.36 mg dm−3 for Pi and 15.78 mg dm−3 for Porg while the associated technologies presented values of 116.78 mg dm−3 (Pi) and 39.38 mg dm−3 (Porg) (Table 2).
In general, there were no differences between sources with associated technology and MAP or between MAPPOL and MAPBIO for the moderately labile compartment. However, in this compartment, fertilizers with associated technology showed better results in relation to MAP and MAPTF was better in relation to MAPPOL for the content of moderately labile Pi extracted in 1 M HCl (C1 and C2, Table 3).
Fertilizers with associated technology presented average levels (Pi) of 9.32 mg dm−3 while MAP recorded a value of 6.75 mg dm−3 for the 1 M HCl extractor (Table 2). MAPTF presented a value 19.81% higher in relation to MAPPOL for this same variable.
In the restricted lability compartment, the associated technologies provided better results in relation to the MAP for the Porg contents extracted in 0.5 M NaOH (C1, Table 3). The average obtained by MAP was 5.30 mg dm−3 while the associated technolo-gies recorded 7.98 mg dm−3 (increase of 50.56%).
MAP presented higher P-values for the non-labile compartment in relation to the associated technologies (C1, Table 3). While fertilizers with associated technology record-ed average values of 1,549.15 mg dm−3, MAP presented a value of 1,772.32 mg dm−3, as conventional MAP tends to present higher levels of P in more stable forms in soil.
The Pi and Porg contents in the restricted lability compartment (0.5 M NaOH) and the non-labile P contents showed no differences between the associated technologies (C2, Table 3). These results demonstrate that the addition of filter cake or coating with polymers presented similar results for these compartments.
When considering the doses of P, MAP and MAPTF at a dose of 150% presented higher levels of P readily labile, of restricted lability and non-labile P in relation to 100 % (C3, Table 4). In turn, MAPPOL showed higher P levels in the readily labile and restricted lability compartments at a dose of 150% compared to 100% (C3, Table 4). As expected, the increase in the dose of phosphate fertilizer suggests higher P contents, especially in the more labile compartments (C3, Table 4). This occurs because, due to the increase in the applied P dose, the P adsorption sites in the regions adjacent to the granules can be saturated, increasing the availability of P.
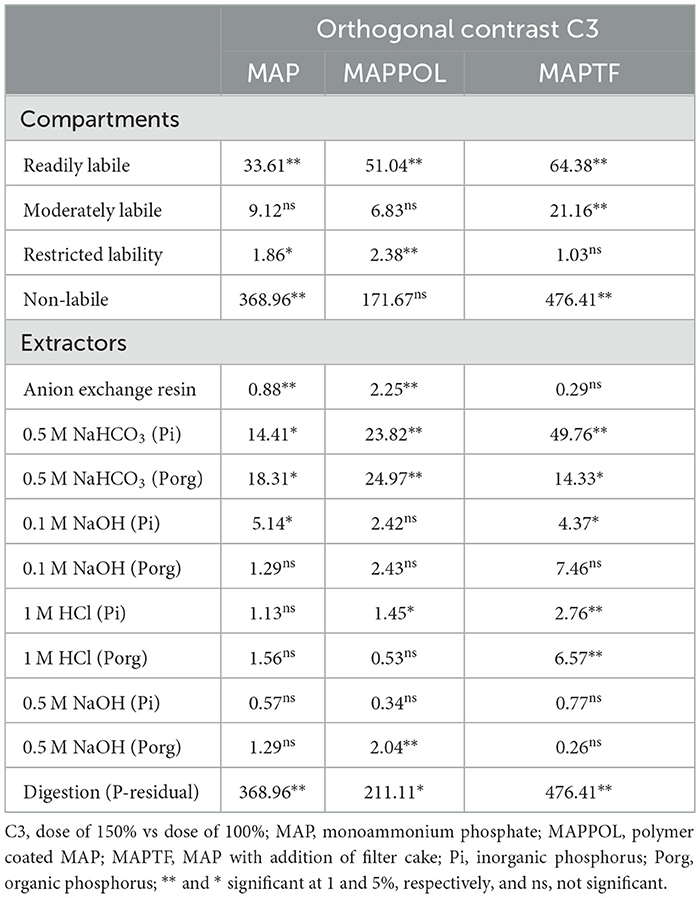
Table 4. Orthogonal contrast (Ci) of P extractors, considering the doses of phosphate fertilizers within phosphate fertilizers, independently of nitrogen fertilizers.
The dose effect on MAP and MAPPOL occurred mainly in readily labile and non-labile P extractors (C3, Table 4). MAPTF also provided greater results in extractors with moderate lability, with Pi extracted in 0.1 M NaOH and Pi and Porg extracted in 1M HCl at a dose of 150% in relation to 100% (C3, Table 4).
For the restricted lability extractor, 0.5 M NaOH, the 150% dose was only higher than the 100% dose for the Porg content recorded by MAPPOL. For all sources, the highest dose provided the highest P-residual content.
In general, there was no interaction for the use of N fertilizers, which was expected in a long-term experiment. Data regarding N sources were not presented due to the lack of significance for the contrasts regarding the use of N fertilizers (conventional urea – UC x urea coated with polymer and sulfur – UPS).
Productivity data per fertilizer is presented in Figure 4.
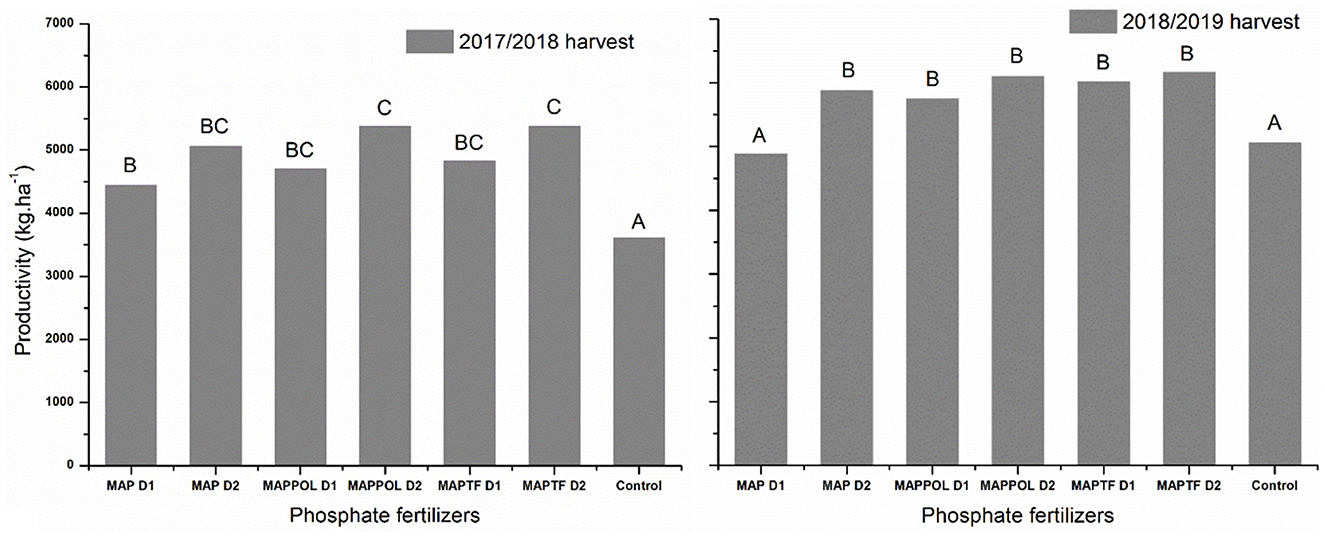
Figure 4. Average productivity of the conilon coffee in the 2017/2018 and 2018/2019 harvests depending on the application of phosphate fertilizers and doses of phosphate fertilizers. Equal letters do not differ among fertilizers at the 5% significance level using Tukey test.
The average yields of processed grains in the 2017/2018 and 2018/2019 harvests due to the application of phosphate fertilizers with integrated protection (MAPPOL + MAPTF) were 5,067.6 and 6,004.8 kg ha−1, while the application of MAP generated production of 4,745.4 and 5,379.6 ka ha−1, respectively. This demonstrates that phosphate fertilizers with integrated protection (MAPPOL + MAPTF) compared to MAP increased productivity by 6.79 and 11.62% in the 2017/2018 and 2018/2019 harvests, respectively.
When comparing phosphate fertilizers with associated technology to each other in the 2017/2018 and 2018/2019 harvests, there were no statistical differences between the MAPPOL and the MAPTF for the variables analyzed (Figure 4). Even though there are no differences between them, the application of these fertilizers provided high productivity in relation to the average productivity for conilon coffee in the State of Espírito Santo in the 2017/2018 and 2018/2019 harvests (CONAB, 2019).
4 Discussion
The increase in availability of P with the use of fertilizers with technology was relevant (C1, Table 3), indicating that, in general, the more labile fraction of P in the soil was influenced by the addition of technology to fertilization management, whether by coating with polymers or addition of filter cake to MAP. These results were relevant, as they demonstrate that the use of technology can increase the sustainability of phosphate fertilization, improving the availability of P for crops.
The lower levels of soil P availability recorded by MAP may be associated with the high solubility of the soluble source and the absence of P release mechanisms under favorable soil conditions, causing immediate adsorption and migration to less labile compartments. The shorter P remains in labile compartments, the faster its transit to less labile compartments, reducing the efficiency of phosphate fertilizer (Amaral et al., 2011).
The addition of technology to the soluble source had the potential to increase the labile fractions of P (Borges et al., 2019; Lustosa Filho et al., 2020), as it promotes the release of P in favorable soil conditions (high volumetric content of water) and/or improves the environment in the region of P release (addition of organic matter). As a result, there was an increase in the organic matter content, an increase in negative charges, the release of organic acids and an improvement in the environmental quality of the soil, increasing production sustainability. Furthermore, it reduced the contact time of P with the soil matrix, reducing forms that are less available through the adsorption process (Wadt and Silva, 2011; da Cruz et al., 2017).
There are particularities in the functioning of each associated technology. MAPPOL has a coating layer responsive to soil moisture and the solubilization of the fertilizer inside occurs according to the humidity (Mikula et al., 2020). This release dynamic allowed the P to be released under more favorable conditions. In addition, the greater volumetric water content provides greater biological activity and, consequently, greater presence of P in the readily labile compartment, suggesting greater environmental sustainability of the production.
MAPTF, as it is an organo-mineral fertilizer, the organic matter present in its constitution acts by coating Fe and Al oxides and the organic acids released compete for P adsorption sites in clay minerals, which reduces the adsorption intensity and increases P availability (Andrade et al., 2003; Fernandes et al., 2015), increasing P levels in the labile compartment. Furthermore, the phosphorus-metal-humic compounds formed between P and organic matter tend to have greater reversibility compared to oxides, which may have increased the P contents extracted in anion exchange resin and 0.5 M NaHCO3 (Lustosa Filho et al., 2020).
Soltangheisi et al. (2019) found an increase in labile P fractions by up to 45.7% when adding filter cake to a soluble source (triple superphosphate). The authors attributed these results to the fact that the filter cake is an organic material with a high cation exchange capacity, which can reduce the intensity of P adsorption and increase its availability.
Oliveira et al. (2022), using polymer-coated MAP, found that in the labile and moderately labile fractions, the polymer-coated fertilizer was more efficient. However, Gazola et al. (2013) did not find an interaction between MAP (conventional and coated) and residual phosphorus content in the soil, which requires further studies to establish the relationship between polymerized fertilizers and the availability of P in the soil by different extractors.
Much has been said about the sustainability of P use in agriculture and the importance of reusing or recycling sources of this element, especially as it is one of the most limiting nutrients for agricultural production in tropical soils. In the case of Brazil, there is a great dependence on imports of phosphate fertilizers. In this context, the addition of technology to phosphate sources and the increase in the P content in the labile fraction has the potential to increase the efficiency of fertilization and, consequently, the sustainability of agriculture, requiring fewer inputs to maintain high levels of productivity.
The best results presented by the associated technologies in relation to MAP in the moderately labile fraction for the Pi content extracted in 1 M HCl may be associated with the kinetics of P release, the coating of Fe and Al oxides by organic matter and the formation of organic compounds with P (Borges et al., 2019; Li et al., 2020; Lustosa Filho et al., 2020). Such mechanisms contribute to reducing the intensity of P adsorption on Fe and Al oxides, which begins with the reversible adsorption-precipitation of the orthophosphate ion from the soil solution until its irreversibility, from an agronomic point of view (Novais et al., 2007; Fernández et al., 2008), decreasing the lability of P, a phenomenon that is observed more pronouncedly for soluble sources, such as MAP.
The higher P levels obtained by MAPTF in relation to MAPPOL for moderate lability may be related to the migration of P from the most labile compartments, which occurs over time. It is also noteworthy that this compartment is important in P dynamics and contributes to the absorption of P by crops, especially perennials (Tisdale et al., 1993; Li et al., 2020).
It is observed that even in a compartment with restricted lability there was an increase in P levels, a fact that may be related to successive fertilization during harvests, which, in addition to increasing P levels in labile and moderately labile compartments, contributed to the increase of associated P to fulvic and humic acids within aggregates (Condron et al., 1985).
The differences between lability and fertilization levels for the fertilizers studied (MAP and MAPPOL – readily labile and non-labile; MAPTF – moderate lability) may be related to the P release kinetics, as the addition of filter cake to the soluble source generates a pelletized product, which changes the mechanical characteristics of the final product and, consequently, the P release rate, depending on the volumetric water con-tent of the medium.
Authors have already reported a longer release period for MAPTF in relation to other associated technologies, increasing P levels in the soil and maintaining it at agronomically adequate levels for up to 2 years (Soltangheisi et al., 2019), in labile and moderately labile, a fact that may have occurred in this work.
The increase in P adsorption intensity caused by the use of MAP tends to occur because it is a highly soluble source. MAP releases P more quickly into the soil solution and in less favorable conditions, such as the absence of organic matter in the P release region, which the increased contact time with Fe and Al oxides tends to reduce the most available forms of P (Wadt and Silva, 2011). All these together promote greater losses of P to less labile compartments, reducing coffee production sustainability.
The lack of interaction for the use of nitrogen fertilizers can be explained by the long term of the experiment. However, the short-term effect of N on P availability may occur. Hanway and Olson (1980) observed that there is an increase in P absorption when applied together with ammonia N in the planting furrow. This occurs because UPS has sulfur in its composition and when added to the soil, the released sulfur can compete with phosphate for adsorption sites on Fe and Al oxides, especially in highly weathered soil (Casagrande et al., 2003). With this, adsorption intensity can be reduced, especially in phosphate sources of P with associated technology, which alter the nutrient release kinetics, as well as UPS, improving the availability of N and P for plants and improving the use of nutrients add-ed via fertilization.
Regarding productivity data (Figure 4), it is observed that the greater efficiency of fertilizers with associated technology, MAPPOL and MAPTF, can be attributed to the polymer coating of the granules or to the organic additive, which prevents direct contact of the P with soil colloids, reducing the intensity of P adsorption (Erro et al., 2012; Figueiredo et al., 2012), and thus, may have increased the availability of P for the coffee plant.
The results found corroborate those obtained by Pelá et al. (2019) for corn and soybean crops. The authors observed that monoammonium phosphate coated with polymers compared to uncoated monoammonium phosphate provided an increase of 30.2% (11,367 kg ha−1) and 21.1% (3,595 kg ha−1) in crop productivity of corn and soybeans, respectively.
Evaluating corn grain productivity in response to phosphate fertilizer, Grohskopf et al. (2019) also found an increase in productivity with the application of phosphate fertilizer with associated technology. The authors observed that monoammonium phosphate mixed with poultry litter provided greater corn productivity in the 3 years of cultivation, presenting an agronomic efficiency 20% higher than that of conventional fertilizer.
These results demonstrated that the intensity of P adsorption intensified by using MAP tends to be greater because it is a highly soluble source. MAP releases P more quickly into the soil solution and in less favorable conditions, such as the absence of organic matter in the P release region, which the increased contact time with Fe and Al oxides tends to reduce the most available forms of P (Wadt and Silva, 2011). All these together promote greater losses of P to less labile compartments, reducing the productivity and sustainability of coffee production.
5 Conclusions
Phosphate fertilizers with associated technology influenced the availability of P in the soil in the labile, moderately labile and restricted lability fractions. These results demonstrate the feasibility of using technologies associated with soluble phosphate fertilizers in field crops, increasing the availability of P for successive crops or perennial crops.
The main effect of fertilizers with associated technology occurred on the labile fraction of the soil, which indicates greater productive potential for short-cycle and perennial crops over time.
These results indicate that the use of phosphate sources with technology has the potential to improve the efficiency of fertilizer use, maintaining higher P levels in forms that are more available in the soil and promoting the sustainability of coffee production.
Phosphate fertilizers with associated technology were efficient in increasing coffee productivity when compared to conventional monoammonium phosphate.
Data availability statement
The raw data supporting the conclusions of this article will be made available by the authors, without undue reservation.
Author contributions
FS: Investigation, Writing – original draft. FA: Conceptualization, Funding acquisition, Project administration, Supervision, Writing – original draft, Writing – review & editing. ES: Formal analysis, Investigation, Methodology, Writing – original draft. EM: Conceptualization, Resources, Validation, Visualization, Writing – review & editing.
Funding
The author(s) declare that financial support was received for the research, authorship, and/or publication of this article. We acknowledge FAPES (Fundação de Amparo à Pesquisa e Inovação do Estado do Espírito Santo) and CNPq (Conselho Nacional de Desenvolvimento Científico e Tecnológico) for granting financial support; and CAPES (Coordenação de Aperfeiçoamento de Pessoal de Nível Superior) to the master's scholarship of FS.
Conflict of interest
The authors declare that the research was conducted in the absence of any commercial or financial relationships that could be construed as a potential conflict of interest.
Publisher's note
All claims expressed in this article are solely those of the authors and do not necessarily represent those of their affiliated organizations, or those of the publisher, the editors and the reviewers. Any product that may be evaluated in this article, or claim that may be made by its manufacturer, is not guaranteed or endorsed by the publisher.
References
Abdala, D. B., Northrup, P. A., Arai, Y., and Sparks, D. L. (2015). Surface loading effects on orthophosphate surface complexation at the goethite/water interface as examined by extended X-ray absorption fine structure (EXAFS) spectroscopy. J. Colloid Interface Sci. 437, 297–303. doi: 10.1016/j.jcis.2014.09.057
Alvares, C. A., Stape, J. L., Sentelhas, P. C., Gonçalves, J. L. M., and Sparovek, G. (2013). Koppen's climate classification map for Brazil. Meteorol. Z. 22, 711–728. doi: 10.1127/0941-2948/2013/0507
Alvarez, V. V. H., and Alvarez, G. A. M. (2006). Comparações de médias ou testes de hipóteses? Contrastes! Bol. Inf. SBCS. 3, 24–33.
Amaral, J. F. T. D., Martinez, H. E. P., Laviola, B. G., Tomaz, M. A., Fernandes Filho, E. I, and Cruz, C. D. (2011). Produtividade e eficiência de uso de nutrientes por cultivares de cafeeiro. Coffee Sci. 6, 65–74.
Andrade, F. V., and de Villani, E. M. (2017). “Fracionamento de Fósforo,” in Manual de Métodos de análise de solos. 3rd Edn, eds. P. C. Teixeira, G. K. Donagema, A. Fontana, and W. G. Teixeira (Brasília: Embrapa), 343–358.
Andrade, F. V., Mendonça, E. S., Alvarez, V. H., and Novais, R. F. (2003). Adição de ácidos orgânicos e húmicos em Latossolos e adsorção de fosfato. RBCS 27, 1003–1011. doi: 10.1590/S0100-06832003000600004
Araujo, M. B., Salcedo, I. H., and Sampaio, E. B. (1993). Efeito de fertilizações fostatadas anuais em solo cultivado com cana-de-açúcar. I: Intensidade e formas de acumulação. Rev. Bras. Ciênc. Solo 17, 389–396.
Borges, B. M. M. N., Abdala, D. B., De Souza, M. F., Viglio, L. M., Coelho, M. J. A., Pavinato, P. S., et al. (2019). Organomineral phosphate fertilizer from sugarcane by product and its effects on soil phosphorus availability and sugarcane yield. Geoderma 339, 20–30. doi: 10.1016/j.geoderma.2018.12.036
Camargo, F. A. O., Gianello, C., Rheinheimer, D., Kaminski, J., and Bissani, C. A. (1999). “Fracionamento do N, P e S orgânicos,” in Fundamentos da matéria orgânica do solo: ecossistemas tropicais e subtropicais, eds. G. A. Santos, and F. A. O. Camargo (Porto Alegre: Gênesis), 373–376.
Casagrande, J. C., Alleoni, L. R. F., Camargo, O. D., and Borges, M. (2003). Adsorção de fosfato e sulfato em solos com cargas elétricas variáveis. Rev. Bras. Ciênc. Solo 27, 51–59. doi: 10.1590/S0100-06832003000100006
Companhia Nacional de Abastecimento - CONAB (2019). Acompanhamento da safra brasileira: café, v.5 - Safra 2019, n. 4 - quarto levantamento, Brasília. Available online at: http://www.conab.gov.br/info-agro/safras/cafe/boletim-da-safra-de-cafe (accessed Jan 02, 2020).
CONAB (2019). Companhia Nacional de Abastecimento – CONAB. Acompanhamento da safra brasileira: café, v.5 – Safra 2019, n. 4 – quarto levantamento, Brasília, dez. 2019. Available online at: http://www.conab.gov.br/info-agro/safras/cafe/boletim-da-safra-de-cafe (accessed January 2, 2020).
Condron, L. M., Goh, K. M., and Newman, R. H. (1985). Nature and distribution of soil phosphorus as revealed by a sequential extraction method followed by 31P nuclear magnetic resonance analysis. J. Soil Sci. 36, 199–207. doi: 10.1111/j.1365-2389.1985.tb00324.x
Cross, A. F., and Schlesinger, W. H. (1995). A literature review and evaluation of the. Hedley fractionation: applications to the biogeochemical cycle of soil phosphorus in natural ecosystems. Geoderma 64, 197–214. doi: 10.1016/0016-7061(94)00023-4
da Cruz, D. F., Bortoletto-Santos, R., Guimarães, G. G. F., Polito, W. L., and Ribeiro, C. (2017). Role of polymeric coating on the phosphate availability as a fertilizer: insight from phosphate release by castor polyurethane coatings. J. Agric. Food Chem. 65, 5890–5895. doi: 10.1021/acs.jafc.7b01686
Dalai, R. C. (1977). Soil organic phosphorus. Adv Agron. 29, 83–117. doi: 10.1016/S0065-2113(08)60216-3
De Campos, M., Antonangelo, J. A., and Alleoni, L. R. F. (2016). Phosphorus sorption index in humid tropical soils. Soil Till Res. 156, 110–118. doi: 10.1016/j.still.2015.09.020
Erro, J., Urrutia, O., Baigorri, R., Aparicio-Tejo, P., Irigoyen, I., Storino, F., et al. (2012). Organic Complexed Superphosphates (CSP): physicochemical characterization and agronomical properties. J. Agric. Food Chem. 60, 2008–2017. doi: 10.1021/jf204821j
Fernandes, D. M., Grohskopf, M. A., Gomes, E. R., Ferreira, N. R., and Büll, L. T. (2015). Fósforo na solução do solo em resposta à aplicação de fertilizantes fluidos mineral e organomineral. Irriga 1, 14–27. doi: 10.15809/irriga.2015v1n1p14
Fernández, R. I. E, Novais, R. F., Nunes, F. N., and Ker, J. C. (2008). Reversibilidade de fósforo não-lábil em solos submetidos à redução microbiana e química: II-extrações sucessivas do fósforo pela resina de troca aniônica. Rev. Bras. Ciênc. Solo 32, 2319–2330. doi: 10.1590/S0100-06832008000600011
Figueiredo, C. C., Barbosa, D. V., Oliveira, S. A., Fagioli, M., and Sato, J. H. (2012). Adubo fosfatado revestido com polímero e calagem na produção e parâmetros morfológicos de milho. Rev. Ciênc. Agron. 43, 446–452. doi: 10.1590/S1806-66902012000300005
Fink, J. R., Inda, A. V., Bayer, C., Torrent, J., and Barrón, V. (2014). Mineralogy and phosphorus adsorption in soils of south and central-west Brazil under conventional and no-tillage systems. Acta Sci. Agron. 36, 379–387. doi: 10.4025/actasciagron.v36i3.17937
Gazola, R. N., Buzetti, S., Dinalli, R. P., Teixeira Filho, M. C. M., and De Souza Celestrino, T. (2013). Efeito residual da aplicação de fosfato monoamônio revestido por diferentes polímeros na cultura de milho. Rev. Ceres. 60, 876–884. doi: 10.1590/S0034-737X2013000600016
Grohskopf, M. A., Corrêa, J. C., Fernandes, D. M., Benites, V. M., Teixeira, P. C., and Cruz, C. V. (2019). Phosphate fertilization with organomineral fertilizer on corn crops on a Rhodic Khandiudox with a high phosphorus contente. Pesqui. Agr. Bras. 54:e00434. doi: 10.1590/s1678-3921.pab2019.v54.00434
Hanway, J. J., and Olson, R. A. (1980). “Phosphate nutrition of corn, sorghum, soybeans, and small grains,” in The Role of Phosphorus in Agriculture, 681–692. doi: 10.2134/1980.roleofphosphorus.c25
Hedley, M. J., Stewart, J. W. B., and Chauhan, B. (1982). Changes in inorganic and organic soil phosphorus fractions induced by cultivation practices and by laboratory incubations. Soil Sci. Soc. Am. J. 46, 970–976. doi: 10.2136/sssaj1982.03615995004600050017x
Li, H., Li, Y., Xu, Y., and Lu, X. (2020). Biochar phosphorus fertilizer effects on soil phosphorus availability. Chemosphere 244:125471. doi: 10.1016/j.chemosphere.2019.125471
Lustosa Filho, J. F., Da Silva Carneiro, J. S., Barbosa, C. F., De Lima, K. P., Do Amaral Leite, A., and Melo, L. C. A. (2020). Aging of biochar-based fertilizers in soil: effects on phosphorus pools and availability to Urochloa brizantha grass. Sci. Total Environ. 709:136028. doi: 10.1016/j.scitotenv.2019.136028
Mikula, K., Izydorczyk, G., Skrzypczak, D., Mironiuk, M., Moustakas, K., Witek-Krowiak, A., et al. (2020). Controlled release micronutrient fertilizers for precision agriculture – A review. Sci. Total Environ. 712:136365. doi: 10.1016/j.scitotenv.2019.136365
Novais, R. F., Smyth, T. J., and Nunes, F. N. (2007). “Fósforo,” in Fertilidade do solo, eds. R. F. Novais, V. Alvarez, N. F. Barros, R. L. F. Fontes, R. B. Cantarutti, and J. C. L. Neves (Viçosa, MG: SBCS), 471–550.
Oliveira, C. L. B. D., Cassimiro, J. B., Lira, M. V. D. S., Boni, A. D. S., Donato, N. D. L., Reis, J. R., et al. (2022). Sugarcane ratoon yield and soil phosphorus availability in response to enhanced efficiency phosphate fertilizer. Agronomy 12:2817. doi: 10.3390/agronomy12112817
Pelá, A., Bento, R. U., Crispim, L. B. R., and Reis Junior, R. A. (2019). Enhanced efficiency of phosphorus fertilizer in soybean and maize. Am. J. Cult. Sociol. 13, 1638–1642. doi: 10.21475/ajcs.19.13.10.p1853
Prezotti, L. C., Oliveira, J., Gomes, J., and Dadalto, G. (2007). Manual de recomendação de calagem e adubação para o estado do Espírito Santo: 5th Edn. Vitória: SEEA, INCAPER; CEDAGRO, 305.
Qiong, W. A. N. G., Qin, Z. H., Zhang, W. W., Chen, Y. H., Ping, Z. H. U., Chang, P. E. N. G., et al. (2022). Effect of long-term fertilization on phosphorus fractions in different soil layers and their quantitative relationships with soil properties. J. Integr. Agric. 21, 2720–2733. doi: 10.1016/j.jia.2022.07.018
Rheinheimer, D. D., Santos, E. J. S., Kaminski, J., Bortoluzzi, E. C., and Gatiboni, L. C. (2000). Alterações de atributos do solo pela calagem superficial e incorporada a partir de pastagem natural. RBCS 24, 797–805. doi: 10.1590/S0100-06832000000400012
Silva, F. C. D., and Da Silva, F. C. (2009). Manual de análises químicas de solos, plantas e fertilizantes. Brasília, DF: Embrapa Informação Tecnológica; Rio de Janeiro: Embrapa Solos.
Soltangheisi, A., Santos, V. R. D., Franco, H. C. J., Kolln, O., Vitti, A. C., Dias, C. T. D. S., et al. (2019). Phosphate sources and filter cake amendment affecting sugarcane yield and soil phosphorus fractions. Rev. Ciênc. Agron. 43:227. doi: 10.1590/18069657rbcs20180227
Teixeira, P. C., Donagemma, G. K., Fontana, A., and Teixeira, W. G. (2017). Manual de métodos de análise de solo. Rio de Janeiro, 4.
Tiessen, H., and Moir, J. O. (1993). “Characterization of available P by sequential extraction,” in Soil Sampling and Methods of Analysis, ed. M. R. Carter (Boca Raton, FL: Lewis Publishers). 75–86.
Tisdale, L. S., Nelson, W. L., and Beaton, J. D. (1993). Soil Fertility and Fertilizers. New York, NY: Lehig Press.
Keywords: monoammonium Phosphate, filter cake, fertilizer efficiency, technology, phosphorus, extractors
Citation: Siman FC, Andrade FV, Stauffer E and Mendonça EdS (2024) Reservoirs of P and fertilizer with technology on the path to sustainability in coffee production. Front. Sustain. Food Syst. 8:1403744. doi: 10.3389/fsufs.2024.1403744
Received: 19 March 2024; Accepted: 17 June 2024;
Published: 04 July 2024.
Edited by:
Paulo Sergio Pavinato, University of São Paulo, BrazilReviewed by:
Arup Ghosh, Council of Scientific and Industrial Research (CSIR), IndiaTrio Santoso, Lampung University, Indonesia
Copyright © 2024 Siman, Andrade, Stauffer and Mendonça. This is an open-access article distributed under the terms of the Creative Commons Attribution License (CC BY). The use, distribution or reproduction in other forums is permitted, provided the original author(s) and the copyright owner(s) are credited and that the original publication in this journal is cited, in accordance with accepted academic practice. No use, distribution or reproduction is permitted which does not comply with these terms.
*Correspondence: Eduardo de Sá Mendonça, ZWR1YXJkby5tZW5kb25jYUB1ZmVzLmJy