- Department of Soils, Water and Agricultural Engineering, College of Agricultural and Marine Sciences, Sultan Qaboos University, Muscat, Oman
Drying food is an effective method to preserve fruits and vegetables, increasing their shelf life and preventing germination. Bottle gourds, known for their high nutritional value, can rot quickly due to their high moisture content, causing biochemical and microbial changes. To improve shelf life, moisture can be removed to prevent microbial activity. Different drying methods are used to preserve gourd family vegetables for long periods and off-season. Indirect solar drying shields foods from UV rays, dust, insects, and other contamination by avoiding direct exposure. This study aimed to utilize an indirect solar dryer to research the potential for drying bottle gourds and conduct physicochemical analyses for the dried products to compare the drying techniques. In this experiment, different samples of bottle gourd slices were dried using an indirect solar dryer and compared with sun and shade drying. Under physicochemical properties, moisture content (MC), retention of color, thickness, and shrinkage of dried samples were analyzed and compared. The result showed that the time taken to reach 10% moisture was 13 h for samples dried with sun drying. While shade and indirect solar drying took 17 h. The Midilli-Kucuk model outperforms other approaches for drying bottle gourd, with the greatest R2 values (0.9967, 0.9950, 0.9936) and the lowest RMSE values (0.0182, 0.0220, 0.0277) for direct sun, shade, and indirect solar drying, respectively. The indirect sun dryer showed good color retention compared to the fresh bottle gourd samples with L* value of 44.08, b* value of 15.40 and a* value of 7.01. The volumetric shrinkage was 97.64% in the direct sun drying method after 15 h, which is higher compared to sun and shade dryers due to the effect of time and temperature. In addition, there was a significant difference (p-value <0.05) between the thickness of the three drying methods. The use of indirect solar drying methods can be highly recommended to get high-quality dried food with zero energy cost. For future studies it is recommended to enhance the function and design of the indirect solar dryer to speed up drying times and improve the quality of dried samples.
1 Introduction
Bottle gourd [Lagenaria siceraria (Mol.) Standl.], often known as calabash, is a popular cucurbitaceous vegetable growing in tropical and subtropical regions of the world. It is frequently grown in tropical Africa, Colombia, Brazil, China, Hong Kong, India, Sri Lanka, Indonesia, Malaysia, the Philippines, and the Philippines (Dhatt and Khosa, 2015). Bottle gourd fruit is high in vitamins, antioxidants, minerals, and dietary fibers. In addition to having moderate levels of vitamin C, it is a rich source of choline and the B complex (Gajera et al., 2017). The value of moisture content of a bottle gourd is 94.5 ± 0.06 (Barot et al., 2015). According to Zambrano et al. (2019), this heightened moisture content value, if not adequately controlled, may precipitate swift degradation, provoking consequential biochemical and microbiological alterations. Consequently, fruit and vegetable preservation and shelf-life extension have been achieved by the use of drying. Fruits can be preserved by drying them, which also reduces their water content, weight, and size, makes them easier to transport, takes up less storage space, and increases the variety of foods available (Patel and Gajera, 2019).
Since ancient times, sun drying has been the only way to preserve vegetables and fruits, and until now, some countries are still using this technique. Because of the limitations of sun drying contamination with dust, pests, or insects; loss of color, aroma, or nutritional compounds (Al-Hamdani et al., 2022). Furthermore, plants dried in the sun are susceptible to loss due to birds, insects, and other animals, damage from rain, or other weather events. The product can even lose color, nutrients, and aroma. Another disadvantage is labor intensity and limited capacity (Kumar and Kalita, 2017). Shade drying also utilizes solar energy to heat the bottle gourds. It uses shade to avoid the direct sun damaging the bottle gourds. By heating the surrounding air, it dries the bottle gourds and lowers the humidity of the air required. Light-sensitive substances can be preserved through this drying method, which minimizes light-induced chemical reactions such as oxidation. A shade drying process takes longer to dry than sun drying, which is already considered a lengthy process (Pirbalouti et al., 2013). In comparison with other drying methods such as hot air drying, sun drying, microwave drying, and freeze drying, shade drying retains more essential oil content and color in dried products than other drying methods such as sun drying, microwave drying, and freeze drying (Thamkaew et al., 2021). Many studies were conducted on bottle gourds with different drying techniques, such as those by Vishal and Sanjay (2019), who used fluidized bed drying, and tray dryers (Ingle et al., 2019). A food product’s quality and appearance can be affected by physiochemical and nutritional changes during the drying process (Waskale and Bhong, 2017). Examining the food’s color, moisture content, and shrinkage is crucial because these factors affect the dried food’s quality. Food deterioration is largely caused by microbial development, which is correlated with moisture content (Vera Zambrano et al., 2019). Furthermore, food material shrinkage negatively affects the quality of the dehydrated product, structural changes, volume loss, and increased hardness typically give the consumer a bad impression (Hafezi et al., 2015).
Oman, with its abundant solar radiation throughout the year, has an excellent chance for harnessing and developing solar energy resources (Alharbi and Csala, 2021). It is essential to use sustainable energy to dry agricultural products, especially because of the high post-harvest losses. With the ability to overcome the drawbacks of conventional systems sustainable drying techniques are becoming more and more popular as the best substitutes. According to Lamidi et al. (2019), conventional drying techniques that rely on fossil fuels are not only uneconomical but also losing popularity worldwide. Fruits and vegetables that are sun-dried have several challenges, such as being susceptible to bird and insect attacks and unfavorable weather conditions that can cause color, nutrient, and aroma losses. Sun drying also requires a lot of labor (Kumar and Kalita, 2017). In response, a stand-alone solar-powered indirect solar dryer that offers superior temperature and humidity control and preserves the quality of dried samples appears to be a suitable substitute for direct sun drying. Consequently, this research aims to evaluate the performance of a pre-developed indirect solar dryer in drying bottle gourds by comparing it with sun drying and shade drying. In addition, to analyze the moisture content, color, shrinkage, and thickness of bottle gourds.
2 Materials and methods
2.1 Sample preparation
This experiment was conducted with the bottle gourd samples which were purchased from a local market in As-Seeb, Muscat, Oman. The samples were cut into thin slices and uniformly distributed into trays without overlapping before drying, with an initial moisture content of 94%.
2.2 Drying process and experimental design
Three drying methods were used in this experiment: sun drying, shade drying and indirect solar dryer to dry bottle gourd samples. The low-cost indirect solar dryer at AES (Agricultural Experiment Station) shade house in Sultan Qaboos University (Figure 1) consists of a drying chamber with dimensions of 75 cm length, 75 cm width and 50 cm height, a solar collector and an effective airflow channel. The solar collector uses black body radiation and a thin-layer drying concept. Where, the solar panel powers an air blower indefinitely, and a drying chamber with nine trays is used. The solar collector absorbs heat and an air blower is connected to the collector intake. As shown in Figure 2 a PVC pipe ducting system with insulation is used to connect the collector and drying chamber. Seven thermocouples are used to measure temperatures at various points in a solar dryer and six relative humidity sensors (HOBO BRAND) to monitor humidity within the drying chamber. Nine traces were placed in the drying chamber in an indirect solar dryer, three under sun and shade drying, and five samples were selected from each. Weight, color, and diameter measurements were taken every half an hour for 9 h per day.
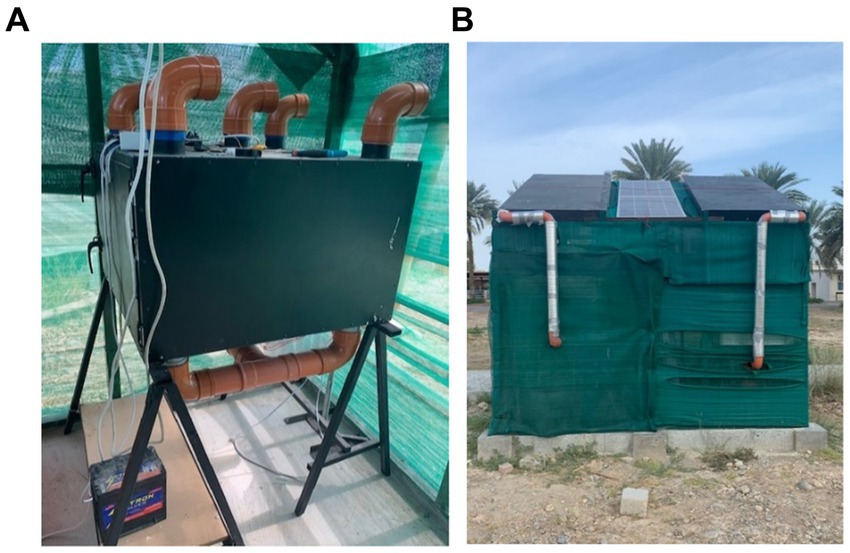
Figure 1. (A) Indirect solar dryer used in experiments (B) Shade house with solar collectors and solar panels.
2.3 Evaluating the heating characteristics of the solar collector
To confirm the surface temperature of a solar collector at various blower speeds and day fluctuations, the study employed temperature distribution modeling. A digital laser sensor and an infrared thermometer were used to measure the temperature. The optimal performance condition was displayed using the contour map modeling program, with temperature levels fixed (20°C, 25°C, 32°C, and 40°C) and airflow varying between 2 and 8 m/s values.
2.4 Mathematical modeling of drying kinetics
The moisture ratio during drying was calculated using the following Equation (1) Jayasuriya et al. (2023):
Where MR represents the moisture ratio, Mo represents initial moisture content [%], and Mt is the moisture content [%]. For a longer drying time, Mt/Mo can be interpreted as the moisture ratio since the equilibrium moisture (Me) content is very small when compared to Mt and Mo (Ashtiani et al., 2017).
In this research for bottle gourds, some drying kinetic models were selected out of a large number of drying models developed for similar agricultural products. The selected models are listed in Table 1. A comparison of the selected models to bottle gourd drying characteristics was undertaken. The best-fitting models were identified using the criteria of the highest value of R2 and the lowest value of RMSE.
2.5 Measurements of physical properties
2.5.1 Measurement of moisture content
The initial moisture content of bottle gourds samples was measured using the Hotbox Oven (Gallenkamp, Cambridge, United kingdom), kept at 105°C for 24 h. Drying trials were conducted, and the averages were used for further analyses. The moisture content was measured in solar, shade, and sun drying at regular intervals until the final moisture level reached 10%. The drying rate curve was generated by plotting moisture content against time.
2.5.2 Color measurements
A portable colorimeter (3nh Precision Colorimeter, NR110, Shenzhen, China) equipped with a D65 illuminant and ten observers was used to evaluate the color quality of bottle gourd drying. Using L*, a*, and b* as benchmarks, the color values were determined. Whereas the a* numbers identify the red as negative values and the green as positive values, the L* values show the lightness of the color from 0 to 100 (Black to White). Alternatively, when it is positive, the chromaticity coordinate b* defines yellow, and when it is negative, blue (Pathare et al., 2013). The total color difference (ΔE), hue, and Chroma were used to calculate color change using the following Equations (2−4) Jayasuriya et al. (2023):
2.5.3 Volumetric shrinkage and thickness measurements
To measure the volume of the bottle gourd, the thickness and diameters of bottle gourd samples were measured every hour using a vernier caliper, respectively. The volumetric shrinkage percentage was calculated concerning the initial volume of the bottle gourd sample using the following Equation (5) Seerangurayar et al. (2019).
2.6 Analysis of data
The data were analyzed using Excel 2016, and RStudio for Windows 10 version 21H2. The experiments were conducted on five replicate data, and the results are presented as the mean standard deviation (SD). One-way analysis of variance and Tukey’s test were used to study the impact of the experimental variables, namely, drying methods (Indirect solar, direct sun, shade drying), on physical properties (moisture content and thickness), using mean values at a 5% significance level. Regression analyses such as coefficient of determination (R2) (Equation 6), and root mean square error (RMSE) (Equation 7) were done to identify the best fit of the evaluated experimental data. The best-fitting quality parameters were those with the highest R2 and lowest RMSE. For parameter estimation, the equations listed below were employed (Inyang et al., 2018).
3 Results and discussion
3.1 Evaluation of ambient parameters
The experiments were conducted in the winter season in the Agricultural Excrement Station (AES) at Sultan Qaboos University (23°35′56.4”N 58°09′43.7″ E). The ambient parameters such as temperature and relative humidity were recorded because they affect the drying process. The solar radiation in the two days of the experiment was 595.7 and 704.0 W/m2, respectively. Lower solar radiation may affect the drying time. The ambient temperature reached maximum values at mid-day at 47.3°C on the second day of experiments. Even though the minimum temperature reached 18°C on the first day of the experiment. The relative humidity reached a maximum value of 79.3%. Higher relative humidity formed obstructions to the drying process such as slow drying rate and destruction of physicochemical properties.
3.2 Performance of the indirect solar dryer
The indirect solar dryer protects the drying materials from the surrounding environment such as insects, dust, and other pollutants as compared to direct sun and shade drying where the samples are directly exposed to the surrounding environment. In addition, the indirect solar dryer preserves the change in the shape of the samples instead of sun and shade drying where the change was higher and non-uniform. The solar collector reached a maximum temperature of 61.03°C, whereas the dryer chamber reached a maximum temperature of 45.2°C, as illustrated in Figure 3. The temperature dropped by 9.5 to 15.0°C between the drying chamber’s intake and the solar collector’s exit due to heat loss via the insulated pipes. This was confirmed by Al-Hamdani et al. (2022) where the temperature dropped by 14.26°C. The parameters of the samples’ drying process can also be impacted by cloud cover.
3.2.1 Heating characteristics of the solar dryer
3.2.1.1 Solar collector
Modeling of temperature distribution is very important to know the position, sun direction, and tilted angle of the solar collector. The heat characteristics of solar collectors may be affected by different parameters such as airflow. Figure 4 shows how the temperature is distributed at different airflow speeds. It is clear that as the fan speed increased from 2.1 to 8 m/s, the temperature of the solar collector decreased immediately.
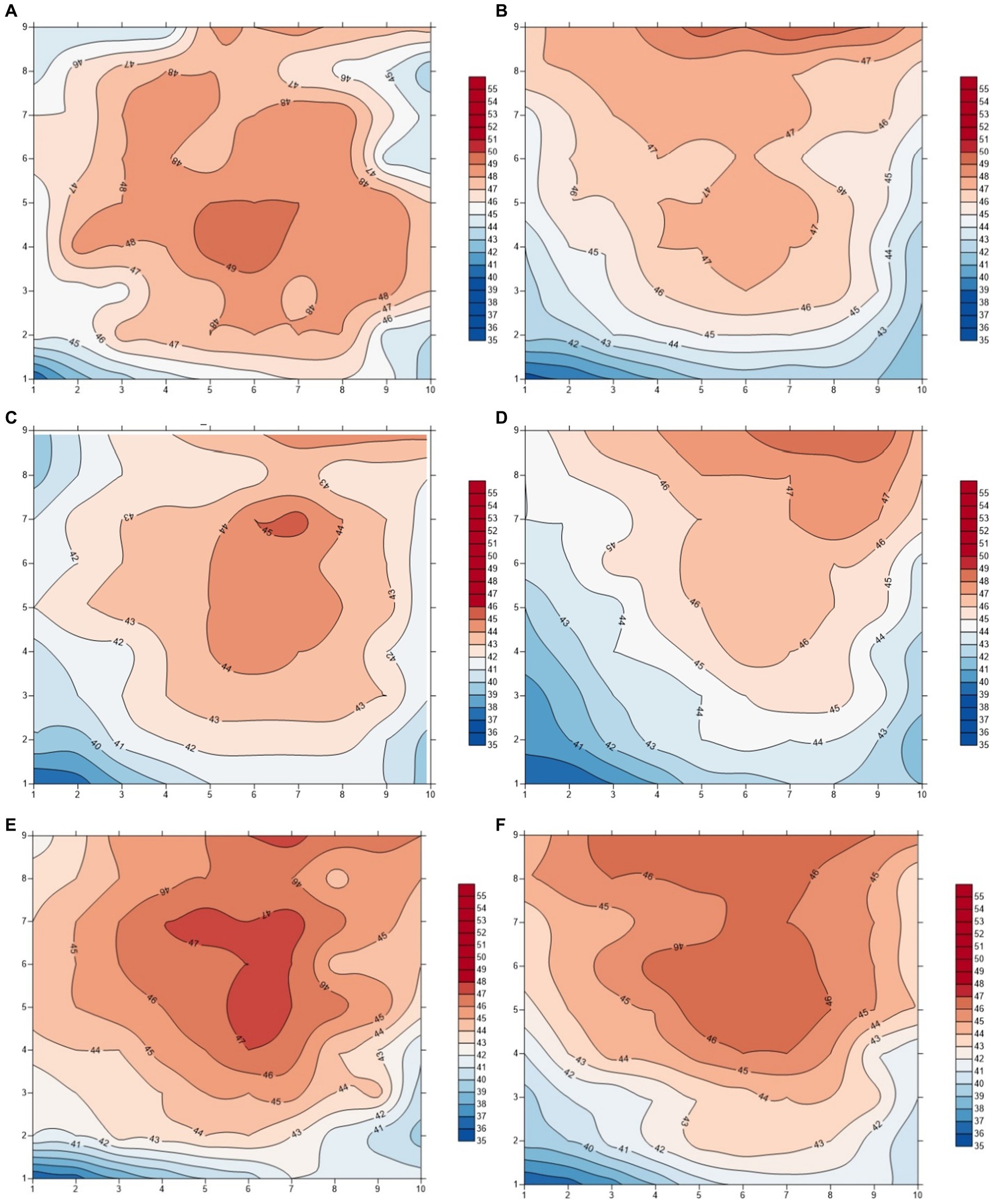
Figure 4. Solar collector heating characteristics illustrated in contour maps at different airflow speeds. (A) Airflow Speed 2.1 m/s, 11:30 a.m. (B) Airflow Speed 3.0 m/s, 10:50 a.m. (C) Airflow Speed 4.1 m/s, 10:35 a.m. (D) Airflow Speed 4.5 m/s, 10:55a.m. (E) Airflow Speed 6.5 m/s, 11:05 a.m. (F) Airflow Speed 8.1 m/s, 11:45 a.m.
The study demonstrates a decrease in solar collector temperature at both inlet and outlet with an increase in fan speed from 2.1 to 8.1 m/s as shown in Figure 5. The outlet temperature drops further through the insulated pipe connection when air reaches the dryer chamber. Even though the temperature of the fan inlet was increased as the fan speed increased. Straight at similar fan speeds, the fan inlet temperature varies slightly, changing at 4.1 m/s and 4.5 m/s (25.0°C and 25.5°C, respectively). Similarly, higher chamber inlet speeds are associated with higher chamber inlet temperatures. The maximum temperature (30.5°C) is recorded with a speed of 8.1 m/s.
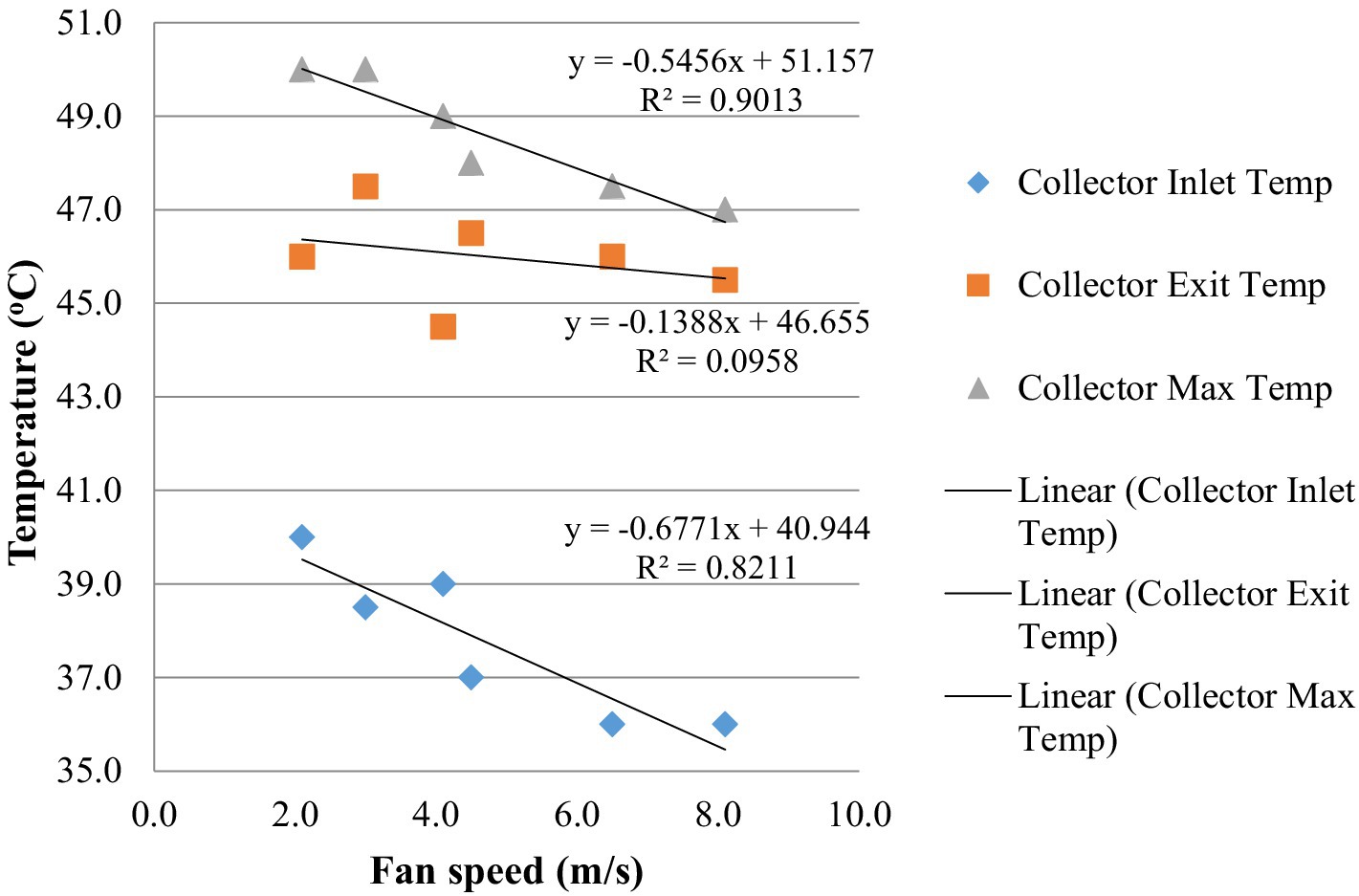
Figure 5. Solar collector heating characteristics with different airflow speeds: Collector temperature drops with an increase in fan speed.
3.3 Moisture content evaluation of bottle gourd
The bottle gourd had an initial moisture content of 94%. The drying methods used to dry bottle gourds significantly affect the moisture content (p-value<0.05). Generally, moisture content decreased over time for all dried samples for all drying methods. This reduction is unstable due to the clouds at that time which affected the solar radiation influx for drying. Sun drying showed a rapid decrease in moisture content reaching 6.4% in 13 h. While shade drying tends to retain moisture content compared with the sun and indirect solar dryers. The indirect solar dryer took 17 h to reduce the weight of the bottle gourd from 94 to 10%. Final moisture content was reduced to around 10% in bottle gourd by three drying methods were 6.4, 12.3 and 10%, respectively (direct sun, shade, and indirect solar drying), which was indicated by the chart Figure 6. Therefore, the open sun was the faster method, indirect solar dryer, and then shade drying. This is associated with the effect of temperature on drying time, as air temperature increase the total drying time reduced (Yasmin et al., 2022). To clarify, higher temperature generates a vapor pressure differential and a partial vapor pressure in the air at a certain temperature, which is one of the driving forces behind drying. Therefore, a higher temperature causes a greater driving force for heat transfer and accelerates the product’s drying process (Daliran et al., 2023). This result was resembled with results by Al-Hamdani et al. (2022) which conclude that the faster drying methods for drying fresh basil and mint is open sun followed by indirect solar dryer and shade drying. Moreover, Daliran et al. (2023) showed that the open sun drying take less time to dry mint leaves with 4.4 h compared with the shade dryer which take 6 h.
3.4 Drying kinetic models of bottle gourd
Figure 7 highlights the relationship between moisture ratio and time. The final moisture ratio of the bottle gourd by using direct sun, shade, and indirect solar drying reached 0.068, 0.131, and 0.107 (MC around 10%), respectively. After 12 h, the shade drying illustrates a higher moisture ratio compared to the indirect sun drying and that may be associated with the temperature difference between shade dryer and indirect solar dryer, where the drying chamber of indirect solar dryer achieved a higher temperature. As shown in Figure 8, the best-fit model for the moisture content curve of drying bottle gourd using different drying methods is the Midilli – Kucuk model. This model had the highest R2 = 0.9967, 0.9950, 0.9936 and lowest RMSE = 0.0182, 0.0220, 0.0277 for direct sun, shade, and indirect solar drying, respectively as shown in Table 2. Similarly, it was found that the best fit model for moisture ratio curves for drying basil and Vernonia amygdalina leaves using open sun and shade drying is Midilli-Kucuk model (Alara et al., 2018; Al-Hamdani et al., 2022). In addition, Al-Hamdani et al. (2022) found that the best fir model for Basil drying using indirect solar dryer in summer is Midilli-Kucuk model.
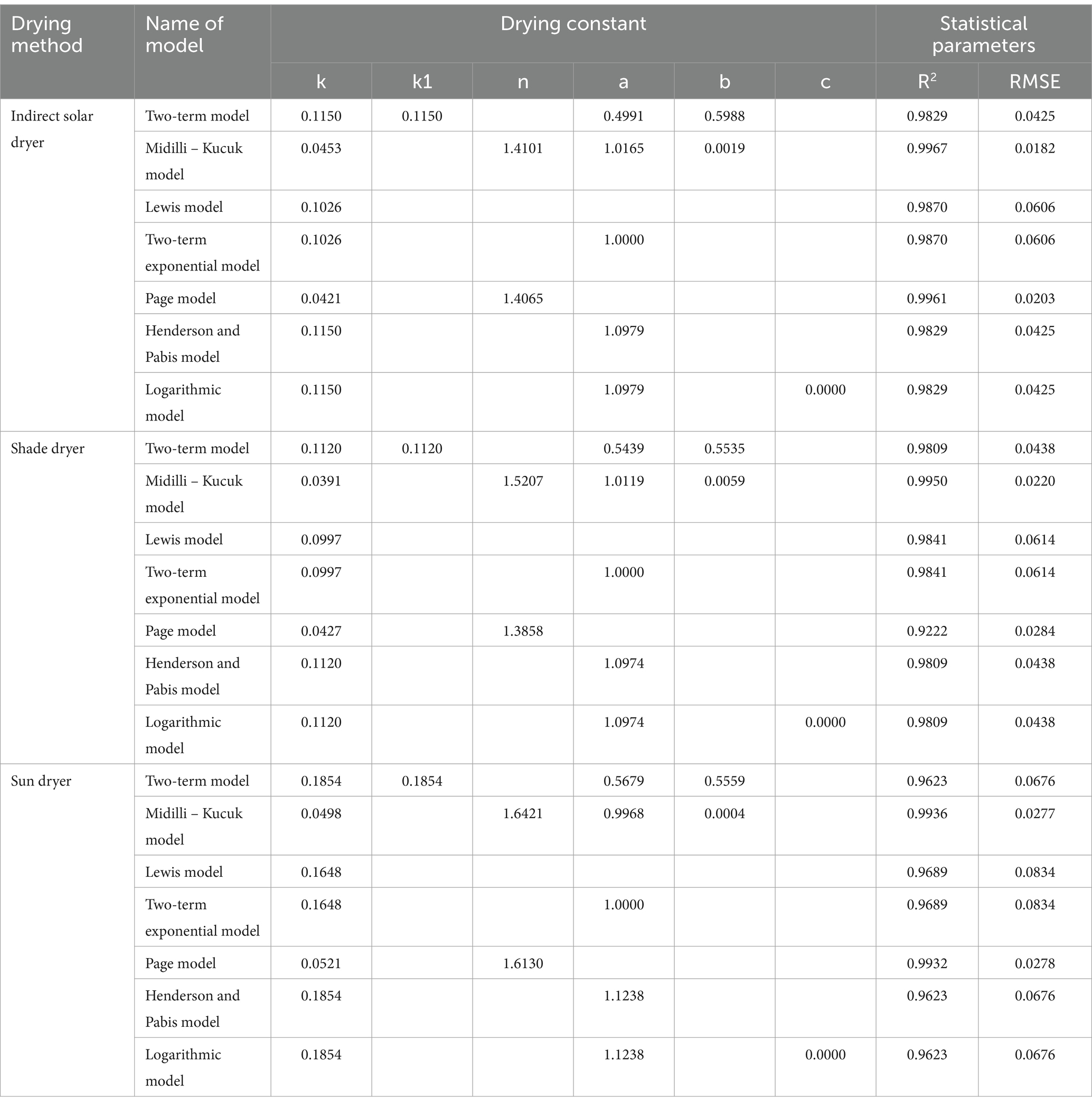
Table 2. Statistical analysis and model parameters of drying kinetic models for bottle gourd using different three drying methods.
3.5 Physicochemical quality parameters
Under physicochemical property evaluations, retention of color and volumetric shrinkage were evaluated to reflect the final quality of the dried samples of bottle gourd.
3.5.1 Color change of bottle gourds
Product quality is greatly influenced by color and appearance, which can either warn of a rotting product or encourage consumption through an appealing appearance (Meng et al., 2018). Table 3 shows the change in bottle gourd color using different drying methods. Bottle gourd L* measurements decreased for each sun, shade, and indirect solar dryer at the end of the experiment, indicating a potential darkening impact. Samples dried in the shade preserve a mid-range lightness, but samples dried in an indirect sun dryer exhibit a lower lightness. The reason behind that is that the sample dried with shade drying tends to retain more moisture content compared with the sample dried with an indirect sun dryer. That can be explained by the linear relationship between lightness and moisture content. Higher moisture content decreased the lightness value due to a change in the spectral properties of light reflected from the dried sample (Özkan et al., 2003). According to Daliran et al. (2023), the lightness of dried mint leaves with a shade dryer showed a lower difference from the lightness of fresh mint leaves compared to the sun drying and Greenhouse Solar Dryer (GSD). The* values of bottle gourds were consistently low and positive, although they increased with every drying technique. Higher greenness is indicated by lower a* values, as indicated by Yasmin et al. (2022). The b* values usually increased with time under all drying methods, indicating a change toward more prominent yellow tones. The indirect solar dryer had less effect, while the shade and sun dryer displayed the greatest b* values. This finding could be attributed to high-temperature drying causing enzymatic and non-enzymatic browning reactions, which result in oxidation when the sample comes into direct contact with oxygen. As a result, the products’ original color was easily destroyed and browning occurred due to the heat exposure (Wang et al., 2014; Meng et al., 2018; Yan et al., 2019). Moreover, The direct sun drying showed greater b* and hue values than indirect solar dryer. Same result was found by Elangovan and Natarajan (2022) that the b* and hue values of dried ivy gourd sample with open sun drying was higher than natural and forced convection drying. In addition, the color difference showed highest change with direct sun drying compare with other drying methods. Al-Hamdani et al. (2022) explained that this higher change in color difference is due to the effect of temperature and time. Where, higher ΔE values associated with the higher drying temperature as indicated by Aksoy et al. (2019).

Table 3. Comparison of Color “L*, a*, b*”, Hue (H), Chroma (C), and color difference (ΔE) values change of drying bottle gourd by three drying methods.
3.5.2 Volumetric shrinkage of bottle gourd during drying
The volumetric shrinkage is an important parameter that indicates how much water is reduced from the samples over time and is important in the value addition process. Food product shrinkage affects drying pace and quality because it causes surface cracking, volume, porosity, and hardness variations (Mugi and Chandramohan, 2021). As shown in Figure 9, the volumetric shrinkage of bottle gourd after 12 h was 96.22, 84.06 and 95.53% for direct sun, shade and indirect solar drying methods, respectively. The sun drying methods showed the highest percentage of volumetric shrinkage within 12 h followed by indirect solar and shade drying. Similarly, Seerangurayar et al. (2019) observed that date dried with the open sun dryer has the higher shrinkage compared to the other solar dryer methods. The reason behind that is associated with the relationship between shrinkage and moisture content, where the highest shrinkage associated with the highest moisture content (Wang et al., 2014; Seerangurayar et al., 2019). Where, the food’s cellular structure is stressed by heat and water loss, which results in a change in shape and a dimension reduction (Hafezi et al., 2015). After 15 h of drying the indirect solar dryer shows the higher volumetric shrinkage (97.64%). This is related to the effect of time and temperature in dried samples whereas time and temperature increase the moisture content decrease, since the food material will exposed to heat for longer period (Abbasi et al., 2009).
3.5.3 Thickness change in bottle gourd during drying
Slice thickness is a crucial factor that influences the characteristics of color quality and drying time. In this study, bottle gourds were initially 5.20 mm, 5.85 mm, and 4.86 mm thick when dried with direct sun, shade, and indirect solar drying methods, respectively. According to the ANOVA test, there was a significant difference in thickness values among the three drying methods (p-value <0.05). A significant difference was observed between direct sun and shade drying methods and between indirect solar and shade drying. However, the was no significant difference between the direct sun and indirect solar drying method (p-value >0.05). As shown in Figure 10, the thickness of the bottle gourd decreased for all drying methods. This indicates longer drying time will lead to thinner bottle gourd slices. After 10 h of drying process, the bottle gourds dried with the direct sun showed a thinner slice (0.77 mm) compared with the shade drying (2.15 mm) and indirect solar dryer (0.88 mm). This occurred as a result of an increased moisture gradient, which also resulted in microstructure stress, and permanent structural alteration (Turkmen et al., 2020).
4 Conclusion
The study focused on evaluating the physiochemical properties of bottle gourds using three different methods: sun drying, shade drying, and indirect solar drying, which used the concept of black body radiation. The study showed significant effects of three drying methods on moisture content, thickness, and color parameters. Evaluation of the moisture content showed that the fastest method to reduce the moisture content of bottle gourds from 94 to 10% was sun drying, followed by an indirect solar dryer that took 17 h. In addition, the best-fit model for the moisture ratio curve using different three drying method is Midilli – Kucuk model. Compared to other drying techniques, the indirect sun dryer demonstrated good color retention ability. Longer drying times produced thinner bottle gourd slices, according to thickness measurements, but volumetric shrinkage analysis revealed that the sun-drying approach produced the largest shrinkage (96.22%) within 12 h. When compared to sun and shade drying, the stand-alone, solar-powered indirect solar dryer eliminates problems like dust and insects. It is recommended that this drier should be evaluated on other foods that are appropriate for thin-layer drying. Further research could focus on improving the indirect solar dryer’s design and function to increase drying rates and improve the quality of dried samples. Enhancing this drying technique in terms of sustainability, quality, and efficiency is the goal of these recommendations.
Data availability statement
The original contributions presented in the study are included in the article/supplementary material, further inquiries can be directed to the corresponding author.
Author contributions
OA-G: Data curation, Formal analysis, Investigation, Writing – original draft. MA-K: Data curation, Formal analysis, Investigation, Writing – original draft. HJ: Conceptualization, Funding acquisition, Investigation, Methodology, Project administration, Resources, Supervision, Writing – review & editing. PP: Conceptualization, Methodology, Resources, Supervision, Validation, Writing – review & editing. MA-B: Methodology, Resources, Writing – review & editing.
Funding
The author(s) declare financial support was received for the research, authorship, and/or publication of this article. The authors would like to thank Sultan Qaboos University, Oman for their financial support under the internal grant project with codes: IG/AGR/SWAE/20/01 and IG/AGR/SWAE/23/02.
Acknowledgments
The authors would like to thank Mr. Seif Al-Adawi and Mr. Khalifa Al-Hinai for their assistance in dryer development and executing the experiment.
Conflict of interest
The authors declare that the research was conducted in the absence of any commercial or financial relationships that could be construed as a potential conflict of interest.
The author(s) declared that they were an editorial board member of Frontiers, at the time of submission. This had no impact on the peer review process and the final decision.
Publisher’s note
All claims expressed in this article are solely those of the authors and do not necessarily represent those of their affiliated organizations, or those of the publisher, the editors and the reviewers. Any product that may be evaluated in this article, or claim that may be made by its manufacturer, is not guaranteed or endorsed by the publisher.
References
Abbasi, S., Mousavi, S. M., Mohebi, M., and Kiani, S. (2009). Effect of time and temperature on moisture content, shrinkage, and rehydration of dried onion.
Aksoy, A., Karasu, S., Akcicek, A., and Kayacan, S. (2019). Effects of different drying methods on drying kinetics, microstructure, color, and the rehydration ratio of minced meat. Food Secur. 8:216. doi: 10.3390/foods8060216
Alara, O. R., Abdurahman, N. H., Mudalip, S. K. A., and Olalere, O. A. (2018). Mathematical modeling of thin layer drying using open sun and shade of Vernonia amygdalina leaves. Agric. Nat. Resour. 52, 53–58. doi: 10.1016/j.anres.2018.05.013
Al-Hamdani, A., Jayasuriya, H., Pathare, P. B., and Al-Attabi, Z. (2022). Drying characteristics and quality analysis of medicinal herbs dried by an indirect solar dryer. Foods 11:4103. doi: 10.3390/foods11244103
Alharbi, F. R., and Csala, D. (2021). Gulf cooperation council countries’ climate change mitigation challenges and exploration of solar and wind energy resource potential. Appl. Sci. 11:2648. doi: 10.3390/app11062648
Ashtiani, S. H. M., Salarikia, A., and Golzarian, M. R. (2017). Analyzing drying characteristics and modeling of thin layers of peppermint leaves under hot-air and infrared treatments. Inform. Process. Agric. 4, 128–139. doi: 10.1016/j.inpa.2017.03.001
Barot, A., Pinto, S., Balakrishnan, S., and Prajapati, J. P. (2015). Composition, functional properties and application of bottle gourd in food products. Research & Reviews: Journal of Dairy Science and Technology 4, 15–27.
Chauhan, P. S., Kumar, A., Nuntadusit, C., and Mishra, S. S. (2018). Drying kinetics, quality assessment, and economic analysis of bitter gourd flakes drying inside forced convection greenhouse dryer. J. Sol. Energy Eng. 140:051001.
Daliran, A., Taki, M., Marzban, A., Rahnama, M., and Farhadi, R. (2023). Kinetic analysis, mathematical modeling and quality evaluation of mint drying in greenhouse solar dryer. Therm. Sci. Eng. Progr. 46:102252. doi: 10.1016/j.tsep.2023.102252
Dhatt, A. S., and Khosa, J. S. (2015). “Bottle gourd,” in Handbook of vegetables. Vol. III. eds. K. V. Peter and P. Hazra (Houston: Studium Press LLC), 49–78.
Elangovan, E., and Natarajan, S. K. (2022). Physicochemical characteristics of dried ivy gourd in a single slope solar dryer. Environ. Prog. Sustain. Energy 41:e13812. doi: 10.1002/ep.13812
Gajera, R. R., Joshi, D. C., and Ravani, A. (2017). Processing potential of bottle gourd (L. siceraria) fruits: an overview. ~ 106 ~ international. J. Herb. Med. 5, 106–109
Hafezi, N., Sheikhdavoodi, M. J., and Sajadiye, S. M. (2015). The effect of drying kinetic on shrinkage and colour of potato slices in the vacuum- infrared drying method. Int. J. Agric. Food Res. 4, 24–31. doi: 10.24102/ijafr.v4i1.476
Ingle, M., Tapre, A. R., and Nawkar, R. (2019). Drying kinetics and mathematical modeling of bottle gourd. Curr. J. Appl. Sci. Technol. 1–8, 1–8. doi: 10.9734/cjast/2019/v38i530382
Inyang, U. E., Oboh, I. O., and Etuk, B. R. (2018). Kinetic models for drying techniques—food materials. Adv. Chem. Eng. Sci. 8, 27–48. doi: 10.4236/aces.2018.82003
Jayasuriya, H., Pathare, P. B., Al-Attabi, Z., and Al-Hamdani, A. (2023). Drying kinetics and quality analysis of coriander leaves dried in an indirect stand-alone solar dryer. Processes 11:1596. doi: 10.3390/pr11061596
Kumar, D., and Kalita, P. (2017). Reducing postharvest losses during storage of grain crops to strengthen food security in developing countries. Food Secur. 6, 1–22. doi: 10.3390/foods6010008
Lamidi, R. O., Jiang, L., Pathare, P. B., Wang, Y., and Roskilly, A. P. (2019). Recent advances in sustainable drying of agricultural produce: a review. Appl. Energy 233-234, 367–385. doi: 10.1016/j.apenergy.2018.10.044
Meng, Q., Fan, H., Li, Y., and Zhang, L. (2018). Effect of drying methods on physico-chemical properties and antioxidant activity of Dendrobium officinale. J. Food Meas. Charact. 12, 1–10. doi: 10.1007/s11694-017-9611-5
Mugi, V. R., and Chandramohan, V. P. (2021). Shrinkage, effective diffusion coefficient, surface transfer coefficients and their factors during solar drying of food products – a review. Sol. Energy 229, 84–101. doi: 10.1016/j.solener.2021.07.042
Özkan, M., Kirca, A., and Cemeroğlu, B. (2003). Effect of moisture content on CIE color values in dried apricots. Eur. Food Res. Technol. 216, 217–219. doi: 10.1007/s00217-002-0627-6
Patel, H. A., and Gajera, R. R. (2019). Chemical science review and letters effect of dehydration on quality of bottle gourd shreds. Chem. Sci. Rev. Lett. 8, 216–219.
Pathare, P. B., Opara, U. L., and Al-Said, F. A. J. (2013). Colour measurement and analysis in fresh and processed foods: a review. Food Bioproc. Technol. 6, 36–60. doi: 10.1007/s11947-012-0867-9
Pirbalouti, A. G., Mahdad, E., and Craker, L. (2013). Effects of drying methods on qualitative and quantitative properties of essential oil of two basil landraces. Food Chem. 141, 2440–2449. doi: 10.1016/j.foodchem.2013.05.098
Seerangurayar, T., Al-Ismaili, A. M., Jeewantha, L. J., and Al-Nabhani, A. (2019). Experimental investigation of shrinkage and microstructural properties of date fruits at three solar drying methods. Sol. Energy 180, 445–455. doi: 10.1016/j.solener.2019.01.047
Thamkaew, G., Sjöholm, I., and Galindo, F. G. (2021). A review of drying methods for improving the quality of dried herbs. Crit. Rev. Food Sci. Nutr. 61, 1763–1786. doi: 10.1080/10408398.2020.1765309
Turkmen, F., Karasu, S., and Karadag, A. (2020). Effects of different drying methods and temperature on the drying behavior and quality attributes of cherry laurel fruit. PRO 8:761. doi: 10.3390/pr8070761
Vera Zambrano, M., Dutta, B., Mercer, D. G., MacLean, H. L., and Touchie, M. F. (2019). Assessment of moisture content measurement methods of dried food products in small-scale operations in developing countries: a review. Trends Food Sci. Technol. 88, 484–496. doi: 10.1016/j.tifs.2019.04.006
Vijayan, S., Arjunan, T. V., and Kumar, A. (2016). Mathematical modeling and performance analysis of thin layer drying of bitter gourd in sensible storage based indirect solar dryer. Innovative Food Sci. Emerg. Technol. 36, 59–67. doi: 10.1016/j.ifset.2016.05.014
Vishal, D. K., and Sanjay, H. A. (2019). Drying behavior and mathematical modelling of bottle gourd. Int. J. Agric. Environ. Biotechnol. 12, 299–306. doi: 10.30954/0974-1712.12.2019.1
Wang, H., Zhang, M., and Mujumdar, A. S. (2014). Comparison of three new drying methods for drying characteristics and quality of shiitake mushroom (Lentinus edodes). Dry. Technol. 32, 1791–1802. doi: 10.1080/07373937.2014.947426
Waskale, H. S., and Bhong, M. G. (2017). Physicochemical and colour properties changes in fruits and vegetables during drying process: A review. Int. J. Innov. Res. Sci. Eng. Technol. 4, 1–8.
Yan, J. K., Wu, L. X., Qiao, Z. R., Cai, W. D., and Ma, H. (2019). Effect of different drying methods on the product quality and bioactive polysaccharides of bitter gourd (Momordica charantia L.) slices. Food Chem. 271, 588–596. doi: 10.1016/j.foodchem.2018.08.012
Yasmin, S., Hasan, M., Sohany, M., and Sarker, M. S. H. (2022). Drying kinetics and quality aspects of bitter gourd (Momordica charantia) dried in a novel cabinet dryer. Food Res. 6, 180–188. doi: 10.26656/fr.2017.6(4).437
Keywords: bottle gourd, indirect solar dryer, moisture content, color, volumetric shrinkage
Citation: Al-Ghadani O, Al-Khayari M, Jayasuriya H, Pathare PB and Al-Belushi M (2024) Drying characteristics and kinetics of bottle gourd using stand-alone indirect solar dryer. Front. Sustain. Food Syst. 8:1400938. doi: 10.3389/fsufs.2024.1400938
Edited by:
Poonam Rani, Teagasc Food Research Centre (Ireland), IrelandReviewed by:
R. Pandiselvam, Central Plantation Crops Research Institute (ICAR), IndiaSeyed-Hassan Miraei Ashtiani, Dalhousie University, Canada
Copyright © 2024 Al-Ghadani, Al-Khayari, Jayasuriya, Pathare and Al-Belushi. This is an open-access article distributed under the terms of the Creative Commons Attribution License (CC BY). The use, distribution or reproduction in other forums is permitted, provided the original author(s) and the copyright owner(s) are credited and that the original publication in this journal is cited, in accordance with accepted academic practice. No use, distribution or reproduction is permitted which does not comply with these terms.
*Correspondence: Pankaj B. Pathare, cGFua2FqQHNxdS5lZHUub20=