- 1Department of Vegetable Science, HC&RI, Tamil Nadu Agricultural University, Coimbatore, India
- 2Department of Food Process Engineering, AEC&RI, Tamil Nadu Agricultural University, Coimbatore, India
- 3Department of Sustainable Organic Agriculture, Tamil Nadu Agricultural University, Coimbatore, India
“Biomimicry” is an acronym used to describe how people looked at nature for inspiration to tackle a variety of problems. The modern problems of fast-increasing urbanization, land degradation, climate change, pandemics, loss of biodiversity, and widespread use of pesticides and fertilizers seriously threaten our food supply chain. There is a growing consumer demand for nutrient-dense, flavourful plant-based cuisine with minimal environmental impact. Moreover, a considerable portion of food roughly 24% is lost before it reaches consumers, partly as a result of poor quality and protracted supply chains. Researching new methods of producing food is essential since, by 2050, there will be more than 9.7 billion people on the planet, 70% of whom will reside in cities. Vertical farming (VF), which relieves pressure on conventional agricultural land by using vertical space instead of horizontal expansion, is growing in popularity as a solution to these problems. Because VF incorporates soil-less growth techniques, it is well-suited for urban environments. This strategy may help to produce more premium products, such as fruits, vegetables, flowers, and herbs. It may also help to produce cosmetics and medications made from plants. Vertical farming, is becoming more favoured as an alternative to traditional agriculture, and provides avenues for enhancing sustainable food production given the growing challenges of climate change and population growth.
Introduction
Global warming, unchecked pesticide and chemical use, natural disasters, and fast urbanization have all had a deleterious effect on soil fertility. In addition, the amount of land available to each individual has decreased, and soil productivity and fertility have declined significantly (Lambin, 2012; Lal, 2015; Lehman et al., 2015). Concerns about uncontrolled groundwater levels, overuse of irrigation, and uncontrolled water contamination are among the causes threatening the watershed’s water resources (Bhanja et al., 2018). Over 9.7 billion people are predicted to live on the earth by 2050, which means that 50% more food must be produced globally. It will require more arable land for this, but there just is not enough of it (FAO, 2016). It is predicted that by 2050, the amount of arable land per person will be less than 0.20 hectares, or less than one-third of what it was in 1970 (Conforti, 2011; FAO, 2016). The conventional soil-based agricultural production techniques are seriously threatened by these problems, which makes the current state of food production quite challenging. Soil-based farming techniques need to be supplemented with modern, environmentally friendlier, and more productive farming technologies (Lambin and Meyfroidt, 2011). Soilless production methods, including hydroponics, aquaponics, and vertical farming, offer a potential solution to contemporary agricultural issues such as declining soil fertility, exhausted nutrient stocks, restricted access to irrigation water, and the effects of climate change (Albadwawi et al., 2022). These innovations can improve water and space conservation, open up urban areas for food production, and protect water resources (Sengupta and Banerjee, 2012; Fussy and Papenbrock, 2022). However, many variables, including soil type, terrain, climate, production scale, technological sophistication, and socioeconomic standing, affect how efficient these systems are (Obalum et al., 2011). Water is conserved and year-round food production is made possible by soilless culture methods like hydroponics and aeroponics in vertical farming (Gokul and Sheeja, 2016). While much attention has been focused on high-value crops, new research has shown that staple crops can be produced with far higher yields (Zhu and Marcelis, 2023). Vertical microgreen farming is a resource- and space-efficient method that promotes global food security (Rajan et al., 2019). Vertical farming methods could offer a different approach to addressing the present scarcity of arable land and water supplies (Benke and Tomkins, 2017) and provide a possible remedy for vulnerable urban communities since it can also solve global food shortages and environmental issues (Besthorn, 2013). This review focuses on cutting-edge methods and technology as it explores the most recent developments in vertical farming.
Vertical farming: a brief history
Vertical farming has its origins in the Babylonian period, exemplified by the Hanging Gardens, which were considered one of the Seven Wonders of the Ancient World by Greek civilization and date back to 600 BC (Al-Kodmany, 2018b). Life magazine published a full-page caricature of A.B. Walker in 1909. The cartoon depicts the open construction of a skyscraper with homes stacked one on top of the other against a rural background as an advertisement for a fictional real estate company. Architecture has been greatly influenced by illustration (Van Gerrewey et al., 2021). This served as the basis for Rem Koolhaas’s 1978 full-length novel Crazy New York. According to Koolhaas, this represents a theorem outlining the perfect characteristics of skyscrapers (Koolhaas, 2014). William Gericke’s book The Complete Guide to Soilless Gardening, published in the 1930s, set the framework for soilless cultivation. This book rose to prominence in the chronicles of vertical farming.
The term “vertical farming” took on a completely new meaning when geologist Gilbert Ellis Bailey used explosives for the first time in 1915 to achieve depth of root development and suggested tilling the earth more deeply (Bailey, 1915; Jackson, 2023). To ensure future food security, vertical farming the practice of cultivating crops in layers that are stacked vertically is essential (Kalantari et al., 2017). It has many advantages, such as increased nutrient and water efficiency, less pesticide and herbicide use, and less agricultural pollution (Zhu and Marcelis, 2023). With a yield that might be 70–80 times greater than that of normal farmland, it has the potential to greatly increase food production (Maurya P. et al., 2023; Zhu and Marcelis, 2023). Perceived sustainability influences consumer acceptability of vertical farming methods; larger systems are thought to be more sustainable (Jürkenbeck et al., 2019). Vertical farming is anticipated to be heavily reliant on technology-driven systems like the Internet of Things (IoT) in the future, although climate control and artificial lighting have substantial energy costs (Debdas et al., 2023; Ullah et al., 2023). Vertical farming is defined in various ways depending on factors such as size, density, layout, building type, location, level of control, and intended purpose. Consequently, stakeholders may perceive vertical farming as anywhere from a minor crop production method to a critical system necessary for ensuring future food security (Maurya P. et al., 2023; Taher Abdelfatah and Mahmoud El-Arnaouty, 2023; Zhu and Marcelis, 2023).
Vertical farming includes various growth systems that vary in scale, users, technologies, locations, and objectives. It is especially well-suited for growing horticultural crops like leafy vegetables (Agrilyst, 2017). The fundamental idea behind “vertical farming” is cultivating plants on several levels to increase yield in a constrained amount of area. Regarding the phrase “vertical farm,” it refers to a meticulously regulated indoor plant cultivation technique, as stated by SharathKumar et al. (2020) (or) an intricately designed indoor plant system that can produce fresh, high-quality produce all year round by carefully controlling every element of plant growth, such as temperature, light, CO2 concentration, humidity, water, and nutrients. Due to its independence from solar radiation and other environmental influences, this technology offers total control over the growing environment for the plant (Nair et al., 2017; SharathKumar et al., 2020).
Vertical farms are separated based on their size and intended use
A dedicated building houses the industrial-scale vertical farm known as Plant Factory with Artificial Lighting (PFAL) (Wang et al., 2023). A modular vertical farm housed inside a shipping container is known as a container farm (Rivero and Salvi, 2023). An indoor farm, or “in-store farm,” is a vertical farm situated in a retail or restaurant setting (Zhu and Marcelis, 2023). A vertical farm appliance fitted into a house or workplace is called an appliance farm (Maurya P. et al., 2023) (see Table 1).
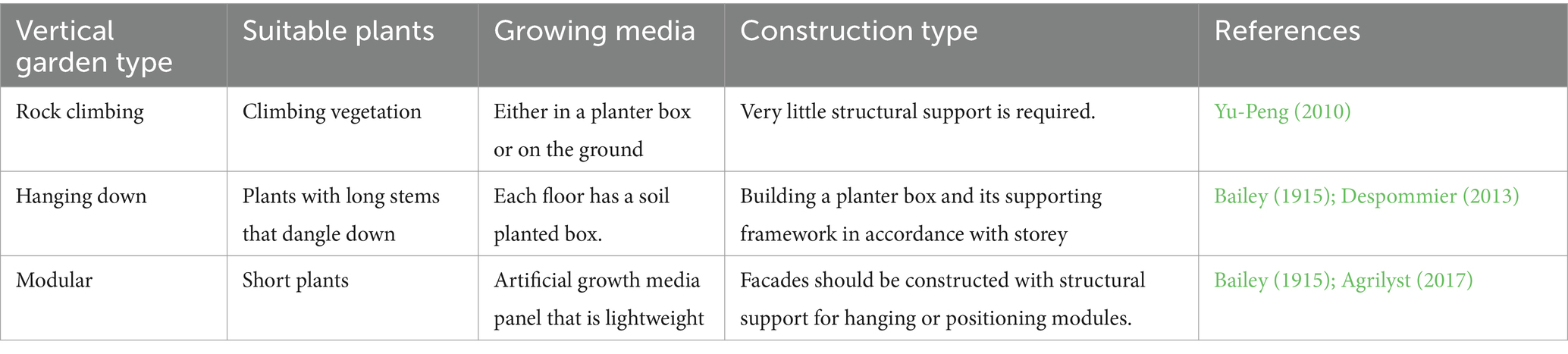
Table 1. Different types of vegetation and materials required for various kinds of vertical gardens (Yu-Peng, 2010).
The necessity of vertical farming
Food security
Global food security is vital in addressing the issue of food security worldwide. It ensures that all individuals have access to safe, nutritious, and affordable food. The impact of food security extends to various areas such as health, economic development, and social stability. Lack of access to adequate food can lead to malnutrition and other health issues, hindering the overall well-being of a population (Farms.com, 2016). Land use specialists including geologists, ecologists, and agronomists note a growing scarcity of farmland (Corvalan et al., 2005; Thomaier et al., 2015). The decreasing availability of traditional farmland is primarily due to factors such as urbanization, soil degradation, and climate change. These factors are impacting the ability to meet the growing food demand by shrinking the area available for food production (Lehman et al., 2015). As a result, there is increased pressure on the existing farmland to produce more food, leading to overuse and decreased soil fertility.
Vertical farming offers a feasible substitute by maximizing food output in a limited space (Touliatos et al., 2016). It is predicated on the idea of creating small, self-sufficient ecosystems that can perform a range of functions, including waste management and food production (Kalantari et al., 2017). In vertical farms, controlled settings lessen the effects of pests, runoff, nutritional deficits, contaminated water, and dust on crop cultivation. Furthermore, the establishment of vertical farming could contribute to the creation of much-needed “green collar” jobs in urban areas (Healy and Rosenberg, 2013).
Low crop yields in traditional agriculture
Traditional agriculture has been the backbone of food production for centuries, but it also comes with its environmental challenges. The environmental impact of traditional agriculture is a growing concern as it contributes to deforestation, soil erosion, water pollution, and greenhouse gas emissions (Rosenzweig et al., 2014). Low crop yields are a persistent problem for traditional agriculture, which can have a big influence on farmers’ income and productivity (Lal, 2009). Low crop yields in traditional agriculture are caused by several issues, including soil degradation, restricted access to modern agricultural methods, and the consequences of climate change (Spielman and Pandya-Lorch, 2009; Lambin and Meyfroidt, 2011). Farmers may enhance their livelihoods, boost crop yields, and add to the general resilience of agricultural systems by comprehending and addressing these factors (Basarir et al., 2022).
Vertical farming enhances crop yields
With precise control of environmental elements like light, temperature, and humidity, vertical farming may provide ideal growing conditions that accelerate growth, increase yields and year around growth (Ptak et al., 2024) and it effectively enhances the total output per unit of land or space and enhances the available growing area by stacking growing beds or shelves vertically (Oh and Lu, 2023). Vertical farming techniques generate larger yields than typical seasonal farming methods because they allow for continuous cultivation and several harvests throughout the year (Mishra et al., 2024). By making it easier to cultivate a wide range of crops, including ones that aren’t often cultivated in some climates, vertical farming can increase crop diversity and possibly yields overall (Qu et al., 2024). Increased crop yields and productivity in vertical farming systems are achieved by the use of cutting-edge technologies like robotics, artificial intelligence, and automated nutrient delivery systems (Zhu and Marcelis, 2023).
Climate change
According to the World Meteorological Organization, the average temperature increase in 2023 is 1.54 degrees Celsius above the pre-industrial period. Climate change increases the frequency and intensity of extreme weather events, such as floods, droughts, and storms, which can disrupt traditional agriculture but can be mitigated in controlled environments of vertical farms (FAO, 2017; Hernández et al., 2018). Changes in precipitation patterns and increased water scarcity due to climate change emphasize the importance of water-efficient farming methods like hydroponics and aeroponics, commonly used in vertical farming (Tilman et al., 2001; Naskali et al., 2022). Vertical farming provides climate-controlled environments that buffer against temperature variability, allowing for consistent crop production despite temperature fluctuations associated with climate change (Sani et al., 2020). Climate change alters pest and disease patterns, threatening crop productivity (Singh et al., 2023). Vertical farming offers resilience to climate change impacts by providing a flexible and adaptive production system. Its modular design allows for rapid adjustments in response to changing environmental conditions (Despommier, 2019). Vertical farming can further boost output and lessen its environmental impact by utilizing automation and technology (Benke and Tomkins, 2017).
Urbanization
According to the book of statistics 2023, the share of urban population was projected to have increased to 56.9 percent in 2022. It is generally higher in the developed (79.7 percent in 2022) than in the developing world (52.3 percent). As urban areas expand, available land for traditional agriculture decreases, necessitating innovative solutions like vertical farming to utilize limited space efficiently (Wang and Kintrea, 2021). Urbanization brings populations closer to markets, enhancing the viability of urban farming methods like vertical farming that can supply fresh produce directly to urban consumers (Specht et al., 2014). Urbanization fosters a growing interest in locally sourced food due to environmental concerns and the desire for fresher produce, driving the adoption of urban farming methods like vertical farming (Sanyé-Mengual et al., 2015). Moreover, the proximity of vertical farms to urban populations reduces transportation costs and emissions associated with food distribution (Specht et al., 2014). Additionally, vertical farming can repurpose underutilized urban spaces such as abandoned buildings or rooftops, contributing to urban revitalization efforts (Singh et al., 2023). There are multiple advantages to vertical urban farming, such as better food security, less of an influence on the environment, and the possibility of creating multipurpose urban areas (Al-Kodmany, 2018b; Khalil and Wahhab, 2020; Zaręba et al., 2021). In addition to lowering food miles and related greenhouse gas emissions, it can aid in addressing the problems of scarce green space, population expansion, and agricultural shortages (Al-Kodmany, 2018a). A 50% decrease in urban density would result in households needing to buy an extra 100 gallons of petrol each year, according to the National Highway Traffic Survey (NHTS).
Health
Traditional farming methods often lead to the destruction of both natural and human environments due to insufficient attention to environmental concerns (Despommier, 2010, 2013; Touliatos et al., 2016). This neglect results in soil erosion and pollution, resulting in notable water runoff. A WHO evaluation states that over half of farms worldwide continue to utilize raw animal manure as organic debris, which can attract flies and spread plant diseases and weed seeds that might be harmful to people who eat the food (Al-Kodmany, 2018a). Additionally, pesticide and herbicide use in traditional agriculture can contaminate agricultural runoff, but this risk can be mitigated through controlled indoor farming environments (Cho, 2011). Cho (2011) states that pest invasion and crop damage are also lessened in closed environments.
The ecosystem
Human agriculture, particularly its advancement and intensification, has had a profound impact on natural ecosystems, leading to habitat destruction, eutrophication, and a decline in biodiversity (Matson et al., 1997; Tilman et al., 2001; Emmerson et al., 2016) As stated by Dickson Despommier, “Farming has impacted the Earth’s ecosystems more than any other activity” (Corvalan et al., 2005). This has been primarily driven by the conversion of grasslands and woodlands into agricultural land to address the increasing needs of a burgeoning worldwide population (Walker, 1999). Over the past 50 years, about 1,812,992 km2 of hardwood forest in the Brazilian rainforest have been cleared for farmland (Corvalan et al., 2005). One potential solution to mitigate the negative impacts of climate change and restore biodiversity is the adoption of indoor vertical farming (Benke and Tomkins, 2017; Despommier, 2019; Maheshwari, 2021; Zaręba et al., 2021). Moreover, it is seen as a robust strategy for combating climate change, tackling issues including waste management, carbon emissions, and the consequences of urban heat islands (Maheshwari, 2021). The vertical farming approach is thought to be a workable way to boost urban food sufficiency, and it may also help to reduce resource use and restore forests (Despommier, 2019).
Vertical farming: unpacking the growing trend
Vertical farming is growing more and more prominent because it promotes efficient utilization of available space in urban places where there is a shortage of land. The restricted amount of land available in cities may be addressed by vertical farming, which makes effective use of available space. By harnessing vertical space in skyscrapers or other structures, vertical farming maximizes the use of available land and enables multiple layers of cultivation (Chatterjee et al., 2020). Vertical farming is also attractive because it offers better control over growing conditions. By employing controlled environments, vertical farms can optimize features such as humidity, lighting, and temperature to create ideal growing conditions (Maurya P. et al., 2023). This leads to enhanced crop yields and better-quality produce. Additionally, vertical farming reduces the need for extensive transportation of food from rural areas to urban centers (Van Delden et al., 2021). This avoids the carbon emissions associated with long transportation distances and reduces dependence on traditional agricultural systems (Maheshwari, 2021). Overall, people are choosing vertical farming because it allows for efficient use of space in urban centers, increases crop yields, provides better control over growing conditions, diminishes the demand for transportation, and promotes sustainability in food production. Vertical farming is becoming a popular choice for various reasons (Avgoustaki and Xydis, 2020).
Methods of vertical farming
Vertical farms employ one of three soilless techniques like hydroponics, aeroponics, and aquaponics to supply nutrients to the plants. This is a synopsis of the three systems:
Hydroponics
Hydroponics, the dominant cultivation method employed in vertical farming, entails growing plants in nutrient-enriched liquids without the use of soil (Maurya P. K. et al., 2023). Plant roots are immersed in a nutrient solution that is consistently monitored and circulated to uphold the appropriate chemical balance (Ahmed et al., 2021). This technique guarantees ideal growth and development by enabling fine control over the nutrients given to the plants (Sathyanarayan et al., 2023).
Aeroponics
NASA developed this revolutionary indoor growing process. NASA coined the word “aeroponics” in the 1990s to describe the efficient growth of plants in space without soil or much water. While still considered unconventional, aeroponic systems are increasingly prevalent in vertical farming (Alneyadi et al., 2024). These systems, which utilize significantly less water up to 90% less than hydroponic systems. Chittibomma et al. (2023) deemed the most efficient method for cultivating plants in vertical farms. These methods have been employed in an array of contexts, including seasonal plant cultivation, commercial production, and research (Wheeler, 2022). Studies on plant-microbe interactions and plant root development have also made extensive use of aeroponic systems, which offer a flexible and practical platform for investigation (Cai et al., 2023).
Aquaponics
Aquaponic systems integrate fish and plants within a unified environment, expanding upon the principles of hydroponics. In this setup, fish raised in indoor ponds generate nutrient-rich excrement, which is utilized to nourish plants within vertical farms. These plants, in turn, clarify the effluents, which is subsequently recycled back into the fishpond (Ramchiary et al., 2022; Vargas et al., 2022; Okomoda et al., 2023). While aquaponics finds application in lesser-scale vertical farming setups, commercial vertical farms typically focus on the cultivation of a limited range of fast-growing vegetable crops, omitting the aquaponic component. This streamlined approach simplifies both economics and production processes while optimizing efficiency (Birkby, 2016) (see Table 2).
High-tech vertical farming methods
Research on vertical and urban farming seeks to improve the long-term reliability of the food we consume by applying cutting-edge strategies that provide higher yields with less water usage than conventional agricultural practices (Kalantari et al., 2017). The agricultural sector is changing thanks to cutting-edge farming technology including closed plant production systems with artificial light (Kozai, 2012), smart aeroponic systems for IoT vertical agriculture (Belista et al., 2018), and LED lighting in vertical farming systems (Nájera et al., 2022). Herbicides and insecticides are no longer necessary thanks to these systems, which provide each plant with the precise nutrients and amount of light it needs. Additionally, as evidenced by the higher nutritional components and production in horticultural crops, they optimize the value of food and nutrition. In vertical aeroponic farming methods, the adoption of LED illumination has proven very effective in increasing photosynthetic capabilities and crop output (He, 2015). These innovations can greatly increase food security and sustainability. Furthermore, vertical farming offers the possibility of customizing product flavors to meet consumer preferences. These cutting-edge farming techniques mark a revolutionary change in the production of food and farming, and since they can flourish in little space, they are especially well-suited for urban settings as stated by Healy and Rosenberg (2013). Vertical farming minimizes water usage by utilizing innovative irrigation systems such as aeroponics or hydroponics, where plants are grown in a nutrient solution instead of soil. These systems can significantly reduce water consumption compared to traditional farming methods, making it a more sustainable option (Klassen et al., 2020).
The rise of vertical farming in vegetable production
The IPCC’s 2007 report prompted a shift toward exploring alternative methods of food production, such as permaculture, hydroponics, aeroponics, and aquaculture, in response to the escalating challenges posed by climate-induced crop failures. Futuristic ideas proposed by pioneers like Buckminster Fuller and John Todd in the 1930s and 1960s, respectively, gained practical relevance as traditional outdoor farming faced serious threats by the year 2000. By 2008, countries like Japan, Korea, Singapore, and Sweden started supporting demonstration programs, including the construction of Vertical Farms (VF), signaling a paradigm shift in food supply management.
Vertical farming has gained traction as a solution to the challenges posed by traditional agriculture, such as limited land availability and dependence on weather conditions. By utilizing vertically inclined surfaces, such as skyscrapers or indoor structures, vertical farming maximizes the use of space and resources. This means that vertical farming can cover a significant area for vegetable cultivation in urban settings, where land is scarce (Despommier, 2010). Nowadays vertical farming has been working as the skyscraper gardens or skyline farms in many cities. Vertical farming plays a crucial role in shaping the urban landscape and contributing to sustainable urban agriculture (Zaręba et al., 2021). With its innovative design and proper management, vertical farming can transform unused spaces into productive vegetable gardens. Moreover, vertical farming is considered a symbol of societal well-being and urban sustainable development. Vertical farming can cover a significant area for vegetable cultivation in urban settings, particularly in areas where land is limited (Khalil and Wahhab, 2020; Zhou et al., 2022). This allows for the adaptation of vertical farming to different available spaces in urban areas, making it a versatile and scalable solution for vegetable cultivation (Khalil and Wahhab, 2020). Overall, the area covered under vertical farming on vegetables can vary greatly depending on the specific design, patterns, and sizes of the vertical farm (Zhu and Marcelis, 2023) (see Table 3).
Technological trends in vertical farming
Sensing technology and actuators
For effective environmental management and to maximize plant development, sensors are essential in vertical farming (Chuah et al., 2019; Ullah et al., 2023). These sensors can be integrated into a range of systems, including electronic systems (CPS) (Chuah et al., 2019) mobile sensor networks and Internet of Things (IoT) founded applications (Abbasi et al., 2014). These sensors monitor variables like humidity, temperature, intensity of light, CO2 levels, level of nutrients, and pH. They offer real-time analytics and data, enabling remote monitoring and control as well as data-driven for decision-making (Chuah et al., 2019; Wang et al., 2021; Ullah et al., 2023). Furthermore, by tracking soil conditions and supplying crucial information for maximizing crop development, soil-based sensors and plant monitors can further improve precision agriculture (Yin et al., 2021).
In advanced vertical farming, the integration of sensors and actuators is vital for automation, efficiency, and simplified management (Singh and Singh, 2020). Actuators interact with environmental aspects and gather crucial data on temperature, pH alterations, light, decreasing the need for ongoing human intervention (Saad et al., 2021). Actuators respond to this data by regulating equipment associated with characteristics such as ventilation, cooling, refrigeration, humidity, illumination, pressurization, ensuring optimal agricultural conditions without heavy human involvement (Sivamani et al., 2013).
Temperature sensors utilizing thermostats or resistance temperature sensors provide real-time temperature measurements converted into electrical signals, while maintaining appropriate humidity levels is also key for plant development. Precise moisture conditions and exact temperature control are crucial for optimal plant development as various plant species necessitate different temperature ranges for photosynthesis (Lakhiar et al., 2018). Accurate measurement of CO2 levels is essential in vertical farming, with devices like chemical carbon dioxide sensors (CCDSs) and nondispersive infrared carbon dioxide sensors (NICDSs) aiding in monitoring and controlling CO2 concentrations, directly impacting plant growth and development and productivity of farm (Dhanaraju et al., 2022).
Texture sensor
It is employed to measure surface of soil, soil depth, soil density. Few research described many types of technical devices available to test soil electrical conductivity and texture (Martínez et al., 2021). These soil texture sensors also offer indirect data on soil health and electrical conductivity (Saad et al., 2021).
Organic matter sensing device
The component of soil known as organic matter is made up of substances produced by soil species, cells and tissues of soil microbes, and leftovers from plants and animals at various stages of decomposition (Ravansari et al., 2021). The physical and chemical characteristics in soil, in addition to the soil’s ability to supply balancing ecosystem services, may all be monitored with the use of soil organic matter sensors. For the consistency and functionality of soil, in particular, the presence of these sensors is thought to be crucial. Soil organic matter sensors monitor several aspects of soil function, such as nutrient cycling with storage, buffering capacity, water retention, fertility, pollutant adsorption and retention, changes in the composition of the soil, accumulation, and fertility (Frazão et al., 2019). Urban VF crops are produced using these sensors to choose sustainable soil (Saad et al., 2021).
Plants to develop healthily in indoor vertical farms, 8 to 10 h of artificial light are needed each day, the amount of light needed varies depending on the type of plant (Despommier, 2010; Kato et al., 2010). Light intensity sensors such as photodiodes, phototransistors and photoresistors, guarantee plants to assure the appropriate light amount, considering different plant varieties’ light requirements. These sensors ensures current information on intensity of light and particular wavelengths, ensuring optimal light conditions for vegetation development (Saad et al., 2021). Water-level sensors automate the monitoring of nutrient-solution reservoirs, contributing to accurate monitoring and control. Electrical conductivity (EC) and pH sensors are also utilized to measure nutrient solution parameters, augmenting nutritional balance and stability for improved nutrient absorption (Resh, 2022). Integrating sensors for light intensity, water level, temperature and EC/pH allows growers to obtain real-time insights, aiding in informed decision-making and enhancing crop quality and quantity in vertical farming settings (Yin et al., 2021).
Actuators serve as vital components in vertical farming, offering precise control over environmental conditions. These mechanisms transform electronic impulses into mechanical movements, enabling dynamic adjustments that foster plant growth. Actuators are indispensable in regulating airflow, modifying lighting positions, and managing irrigation systems for enhanced plant development outcomes (Sivamani et al., 2013; Saad et al., 2021). Actuators maintain constant temperature and air quality through ventilation system regulation, which is vital for the healthy development of plants. Actuators also control irrigation procedures by regulating pumps and valves to precisely supply the right quantity of water to the vegetation, reducing waste and guaranteeing steady hydration, and improving the effectiveness of water management (Despommier, 2010) (see Figure 1).
Nutrient-sensitive systems
Maintaining adequate nutrient levels in hydroponic setups is crucial because plants require varying quantities of nutrition during various growth phases for physical development and physiological maturity (Trejo-Téllez and Gómez-Merino, 2012). While, an excessive influx of stock solution into the nutrient mixing tank might trigger to toxicity in the intended nutrient solution, while insufficient flow might lead to a nutritional shortage. Both scenarios can hinder plants’ long-term growth and maturation (Bamsey et al., 2012). Traditionally, farmers have depended on visual cues in plants to identify nutrient deficiencies or toxicity, which are only visible after extended periods of inadequate nutrition (Horst et al., 2006). Furthermore, identical visual symptoms resulting from various nutrients shortages sometimes result in incorrect diagnoses and ineffective nutrient treatments (Marschner, 2011). A common method for monitoring nutrient levels in hydroponic solutions is to constantly measure pH levels and electrical conductivity. While this approach provides rapid information on nutrient levels, it falls short in pinpointing specific nutrient ions in the solution (see Figure 2).
Plant observation and governing systems
Monitoring and governing plant conditions is crucial for maximizing crop quality in vertical farming methods. In recent times, IoT technology has received significant acknowledgment and has been extensively employed in modern agriculture, especially vertical farming production and management (Marković et al., 2015; Dhanaraju et al., 2022). The crop-growing environment is continually monitored and controlled by IoT-enabled sensors and actuators, enabling real-time monitoring of critical parameters including humidity, temperature, soil moisture, water pH and controls the crop-growing environment, improving food output while consuming less resources (Aiswarya et al., 2023). The incorporation of IoT-based applications has garnered considerable attention due to its capacity to enhance monitoring and precise control, thereby enhancing agricultural sustainability. These interconnected systems utilize a variety of sensors, actuators, and data-analysis tools to offer real-time monitoring of essential environmental parameters (Morella et al., 2023). The advanced applications of the platform furnish farmers with insights into crop-growing circumstances, empowering them to execute prompt action and form decisions that contribute to ensuring crop quality and yield.
Growers can customize habitats for various crops using IoT-enabled devices, verifying regular growth parameters for specific plants from a distance (Quy et al., 2022). This lessens resource wastage, decreases reliance on pesticides, and minimizes the environmental effect in contrast to conventional farming methods. Sensors providing data on water and nutrient levels are utilized to tackle challenges related to the efficient management of water and nutrients. When crop demand is high, this knowledge directs the exact utilization of these resources. Moreover, real-time meteorological data may be readily integrated into IoT platforms, improving their capacity to forecast atmospheric conditions and allowing for proactive modifications (Kabir et al., 2023). The Internet of Things plays a crucial role in managing diseases and pests by using data analysis and contemporary technologies. As IoT devices are user-friendly in the agricultural domain, scientists are encouraged to combine Internet of Things solutions with machine learning techniques (Mehra et al., 2018; Rezk et al., 2021). By using sensors with picture recognition built-in, it is possible to quickly identify symptoms of illness or infestation. The potential for revolutionizing urban agriculture through the incorporation of IoT technologies in vertical farming is significant (Saad et al., 2021).
Visual sensors record visual information, which offers important insights into the growth and health of plants. Plants, foliage, and fruits are continually observed by high-resolution cameras, which provide real-time data on growth trends, disease signs, and stress indicators (Schima et al., 2016). Using computer-vision algorithms to evaluate plant density, size, and even fruit ripeness, visual sensors automate plant monitoring and improve operational efficiency by streamlining labour-intensive tasks. These data also help detect problems early and enable timely interventions (Paulus et al., 2014). By using visual sensor technology, vertical farmers may maximize resources, make educated judgments, and modify their planting operations. This data-driven strategy increases yields, promotes sustainable agricultural methods, and guarantees healthy crop growth (Paul et al., 2022).
Unmanned vertical farming systems
By fusing creativity and technology, automated vertical farming methods offer a cutting-edge agricultural inventions that will transform crop cultivation (Wang et al., 2021). These systems optimize plant development by combining automation, robotics, and cutting-edge sensing technologies to produce controlled circumstances in stacked layers. These systems provide precision management of environmental factors including temperature, light, humidity, and fertilizer supply, assuring ideal circumstances for plant development. They do this by combining real-time data with precise control mechanisms. This precision leads to increased crop yields and a more effective utilization of resources, which significantly lowers labour costs (Saad et al., 2021). Real-time monitoring and automated nutrient and water delivery systems enable more efficient use of resources, which preserves a lot of water and lessens reliance on outside nutrient sources (Avgoustaki et al., 2022). Automated vertical farming systems can produce crops year-round since they are not affected by seasonal or weather fluctuations, which adds to the security of food supply (Saad et al., 2021). The merging of sensors and data analytics enables immediate understanding of plant health and growth status, which also provides a platform for testing out novel crop varieties, cultivation techniques, and technology (Wang et al., 2021). To increase productivity, cut labour expenses, and improve overall efficiency, automated control systems are being included into a vast array of agricultural techniques, aquaculture, animal farming, and controlled greenhouses (Siskandar et al., 2022).
Automated vertical farming systems
Vertical farming automation provides a solution to problems including high labour costs, talent shortages, and increased productivity requirements. In vertical farming methods, fewer human interactions lower the risk of illness while also enhancing production and safety (Kozai, 2012; Van Delden et al., 2021). The ultimate triumph of vertical farming hinges on various automated processes, such as automated harvesting, bed maintenance and reloading, automated irrigation, lighting, fertilization, and crop surveillance through visual systems (Michael et al., 2021). These technologies permit IoT-connected farming for accurate surveillance and feedback on growth circumstances, reduce expenses, and provide critical data for optimal solutions (Bhowmick et al., 2019). The relevance of automation is shown by the emergence of tiny vertical farm systems intended for use in homes or small businesses. However, total automation and potential production optimization are now not attainable because of necessity on manual planting and harvesting (Hegedűs et al., 2023). On the other hand, entirely automated vertical farming systems demonstrate the potential of advanced software-driven setups by including a variety of functions like as lighting regulation, nutrient supplementation, harvesting, cleaning, and sowing. In vertical farming, white light-emitting diode (LED) lighting is an effective light source for commercial plant growth. A study was carried out to find out how white LED light sources affected the development and quality of butterhead and romaine lettuce. The highest light and energy consumption efficiencies were also observed. These findings support the use of certain white LED light sources in vertical farming and demonstrate the beneficial impacts of such light on lettuce growth and quality (Nguyen et al., 2021). An effective robot system for collecting cucumbers using a human-centered approach. In particular, to provide steady and effective harvesting, harvest ordering, visual servoing, and end-effector-based manipulation features were combined. The trials’ findings demonstrated that harvest ordering improved battery efficiency while reducing trip distance and harvesting time (Park et al., 2023). These systems exceed human capabilities by 10–30 times, enabling real-time monitoring, accurate planting, and swift seeding on a 20-layer vertical farm. The cost factor is still an issue, though, as this entirely autonomous system is two or three times costlier than the popular semiautomatic method (Kozai, 2012; Maurya P. K. et al., 2023).
Robotic vertical farming systems
Robotic technology is being used more and more in vertical farming due to the financial and physical limitations of using human labour (Shamshiri et al., 2018). Nevertheless, there are obstacles in the way of creating efficient robotic systems, such as the requirement for cooperation between humans and robots and the capacity to function in unstructured agricultural settings (Bechar and Vigneault, 2016). Because of the physical constraints of large structures and the great density of farmland, human labour is both impractical and wasteful. Complex tasks like precise harvesting, upholding environmental regulations, decreasing human error, and guaranteeing the consistency necessary for the highest possible crop yields and quality are all tasks that robotic systems excel at (Fountas et al., 2020). Robotic vertical farming systems decrease reliance on human labour by effectively and precisely managing various challenges. Labour expenses make up a considerable portion of overall spending (between 25 and 30 percent), robots prove to be accurate, reliable, and efficient workers in a diverse range of jobs, hence reducing both fixed and operating costs. Robotics and automation are enhanced by the use of smart technology, which raises production and efficiency (Starostin et al., 2023). In vertical farms, autonomous robotic platforms with flexible sensors can play a crucial role in achieving these objectives (Avgoustaki et al., 2022). iFarm is a customizable indoor vertical farming system that employs effective power management, LED lighting, and automatic climate control to enable year-round, pesticide-free plant growth. Among its cutting-edge technologies are a neural network that computes plant mass dynamics and a chatbot for analyzing plant images (Kabir et al., 2023). Low-altitude multispectral pictures may be used to identify plant growth and quality, which can reduce the amount of water and nutrients that plants consume (Avgoustaki et al., 2022). This non-intrusive vision method is perfect for self-governing robots in vertical farms. A robot for harvesting and planting on the City Crop automatic indoor farm was proposed by Marchant and Tosunoglu (2017).
Vertical farming with drones
A new era of agricultural innovation is brought about by the combination of drone technology with vertical farming, which reinvents crop growing in controlled environments. Drones and vertical farming together have the ability to transform agriculture, bringing sustainable food production and solving global issues (Tsouros et al., 2019). The confluence of these two innovative technologies holds immense promise to change agricultural practices and help address global challenges including the security of food, limited resources, and environmental sustainability. Drones provide accurate aerial photography and data-driven insights; they are complemented by the climate-controlled, space-efficient environments of vertical farming (Daponte et al., 2019). A revolutionary autonomous drone made exclusively for horticulture, such as flower recognition and seed germination, has been unveiled by Corvus Drones (Kabir et al., 2023). Additionally, they aid in conserving wild bee populations, which are diminishing due to factors like climate change, urban development, and pesticide usage, ultimately benefiting plants reliant on outdoor pollinators (Glick, 2023). Nokia Bell Labs and Aero Farms have collaborated since 2020 to investigate and improve ideas, including trials with commercial crops. Nokia Bell Labs is an expert in sensor data pipelines, AI for mobile sensors, improved photography, private wireless networks, and autonomous drone control (Kabir et al., 2023). Drones that operate on their own take pictures of vertical farm crops while collecting information on plant health markers including dimensions of leaf, stem height, coloration, bending, speckling, and damage (Ghazali et al., 2022). Drones with cutting-edge sensors and artificial intelligence (AI) can maximize resource utilization and deliver data on plant development and health in real-time (Kim et al., 2019). iFarm is effectively testing computer vision technology to use unmanned aerial vehicles (UAVs) (Nunes, 2023), to oversee the developmental phases of plants in vertical farming setups. Their work focuses on techniques for calculating plant weight from pictures and choosing the optimal lighting modes depending on energy usage and leaf growth rate (Bhushan and Negi, 2023). Additionally, they are looking at the use of drones that are unmanned to speed up data collection and enhance neural network performance (Lawrence et al., 2023). According to Puri et al. (2017), this method can greatly lower expenses while raising yields. The absence of a standardized UAV workflow in agriculture, however, continues to be a problem (Tsouros et al., 2019). Agricultural unmanned aerial vehicles (UAVs) appear to have a bright future despite this, as they continue to expand and improve (Kim et al., 2019).
Media selection
A substance that promotes plant development is called a growth medium. Numerous non-toxic porous materials, such as expanded clay, rock wool, pumice, perlite, volcanic minerals, and dust from coconut coir, are used as substrates for plant development (Gruda and Bragg, 2021). These media’s capacity to hold moisture and offer a steady environment for plant roots makes them very well-liked in vertical farming. However, the environmental problems raised by the usage of rock wool and peat in these mediums have prompted a quest for more sustainable replacements (Barrett et al., 2016; Gruda and Bragg, 2021). Using sustainable, reasonably priced, and renewable materials that satisfy environmental and quality standards is the way of the future for growth medium (Gruda, 2019; Sabatino, 2020). The choice of media used in vertical farming can vary based on the particular system and crops being grown (Kalantari et al., 2017). These non-soil mediums are commonly used in vertical farming due to their ability to retain moisture and provide a stable environment for plant roots. Some common types of media exploited in vertical farming include
Peat
The most popular medium for growing plants is peat. Peat generally tend to possess excellent chemical, physical and biological properties for plant growth (Krucker et al., 2010). These characteristics might differ greatly depending on the circumstances surrounding the production of the peat (Bragg, 1990; Michel, 2010). For example, when compared to older and more decomposed deposits, younger and less decomposed peats often have a better water-holding capacity (Schmilewski, 2008). Manufacturers of plant-growing media are moving toward a future with less peat owing to rising consumer knowledge of environmental issues, legislative pressure to restrict the mining of ecologically valuable peatlands, and an awareness of personal accountability (Gruda, 2019). In horticulture, a variety of organic compounds have been offered as a peat substitute for plant growth media (Barrett et al., 2016). As plant-growing media, only composted bark, wood fibre, green waste compost and coir pith have proven to be trustworthy.
Biostrate
It is a recently created microgreen growth medium that is extensively accessible on the market. It is a bio-based fabric called Biostrate that was created especially for hydroponic microgreens and baby salad greens it is utilized in soilless and hydroponic systems, is composed of biodegradable materials like wood Fibers and offers the perfect conditions for plant roots (Reddy and Yang, 2005). Because of its lightweight design and efficient moisture and nutrient retention, it is especially well-suited for hydroponic applications, including microgreen production (Du Jardin, 2015). Applying plant biostimulants can further increase the utilization of Biostrate by enhancing crop quality and nutrient absorption (Halpern et al., 2015). BioStrate is user-friendly and lightweight and ability to decompose naturally (Pandey et al., 2005). Therefore, Biostrate will be studied as a microgreen generation medium and its potential to provide a higher yield compared to other biodegradable environmentally friendly media.
Rockwool
Rockwool is originated by melting chalk and basalt rock together at very high temperatures. This method yields a substrate with a thick structure made up of long fiber strands that resemble steel wool in texture and appearance. According to Borošić et al. (2010), rock wool can be mulched over the soil, blended into the soil, or utilized as a chopped substrate component. Rockwool is a commonly used media in vertical farming due to its versatile properties. It provides excellent water retention capabilities, allowing for efficient nutrient uptake by plants. Additionally, rock wool offers good air circulation around the roots and provides stability and assistance for the plants during vertical growth (Gruda, 2019).
Coco coir: a sustainable growing medium
Natural fibre called coir is derived from the husks of coconuts and has a variety of uses (Stelte et al., 2023). Its physical characteristics, such its capacity to retain water and aeration, make it especially well-suited for various uses (Wang and Huang, 2009). Widely used growing media in vertical farming (Reddy and Reddy, 2019). Before being used as a growth medium, coir needs to be washed many times in fresh water and undergo a “buffering” procedure, which involves adding calcium nitrate to the material to replace dangerously high amounts of salt and potassium (Poulter, 2011; Gruda, 2019). It is biodegradable, renewable, and environmentally friendly. Coir is often used in vertical farming for growing a variety of crops, including vegetables, herbs, and ornamental plants. It can be utilized unprocessed or transformed into a variety of forms such as coir pellets, coir mats, or coir bricks for different growing applications (Reddy and Reddy, 2019).
Expanded clay pellets
Expanded clay pellets are lightweight and porous, providing excellent aeration and drainage for plant roots in vertical farming methods (Zhu and Marcelis, 2023). These pellets, which are made of clay, rice husk ash, and phosphate rock give plant roots superior drainage and aeration. Expanded clay pellets are often used in vertical farming due to their ability to promote efficient root development and nutrient absorption (Tala et al., 2020).
Perlite
Perlite is formed when volcanic rock undergoes further expansion after being superheated. It is light in weight, porous particles that have good aeration and drainage qualities (Varuzhanyan et al., 2006). Its applications extend to enhancing soil drainage and decreasing density when added to potting mixes. Plants may avoid waterlogging and root rot by using perlite, which holds onto some moisture while letting extra water to drain away quickly (Hasan and Dede, 2018). It is sterile, pH-neutral, and does not decompose, making it a durable and reusable growing medium. Perlite is often utilized in vertical farming systems, especially for growing plants that require good drainage and aeration, such as herbs, leafy greens, and strawberries.
Vermiculite
It belongs to the smectite mineral group, it contains significant quantities of potassium and magnesium. Despite being less durable compared to mediums like sand and perlite (Mir et al., 2022). Vermiculite is a very adaptable growth medium that provides several advantages to plants. Its superior water-retention qualities contribute to the roots’ continuous hydration. Vermiculite is also neutral in pH, lightweight, and offers adequate root aeration, making it a wonderful choice for a range of plant species (Papadopoulos et al., 2008). It’s crucial to remember that for best effects, vermiculite ought to be used in conjunction with other growth medium like perlite or coconut fibre (Landis et al., 1990).
Wood fibre
The term wood fibre which comes from both main (like fresh pine chips) and waste (like shredded pallets) streams of wood, is not well defined in the literature (Maher et al., 2008). The wood Fibers that are created through intensive secondary processing are the ones that are most frequently utilized in commercial soilless growth medium. Fresh wood chips are often extruded via a tiny opening. These chips are typically made from debarked softwoods like pine (Pinus spp.) or spruce (Picea spp.). Their structure is altered by the high pressure and high temperature environment, producing a secondary product that is more stable, sterile, and uniform (Domeño et al., 2010). To lessen microbial N immobilization and the ensuing instability (Gruda et al., 2000), the material is frequently saturated with nitrogen (Maher et al., 2008). Due to its inclination to get compressed and its capacity to hold inadequate plant-available water, it is rarely employed as a stand-alone growth medium component (Maher et al., 2008; Domeño et al., 2010). Rather, its purpose is to enhance the physical characteristics of other constituent materials, such as by decreasing bulk density, expanding air volume, and enhancing rewetting ability (Landis et al., 1990).
Trends and developments in vertical farming
One potential approach to addressing the problems of scarce land and rising urban food demand is vertical farming. It allows for efficient use of space by growing crops on vertically inclined surfaces such as skyscrapers (Avgoustaki and Xydis, 2020). While vertical farming is still considered a relatively new concept, its potential in vegetable cultivation is vast. Vertical farming enables vegetable growers to optimize space utilization and increase crop yields. The area covered under vertical farming for vegetable cultivation varies depending on the design, patterns, and sizes of the vertical farm (Zhu and Marcelis, 2023). Furthermore, vertical farming provides opportunities for sustainable agriculture by diminishing the necessity for transportation and preserving natural resources such as water and energy. This is backed by the development of affordable vertical farming models, particularly beneficial in remote areas experiencing challenging weather conditions (Carrasco et al., 2022). Some sources have suggested that vertical farming can range from small structures to large skyscrapers, offering a wide array of possibilities for vegetable cultivation in urban areas while minimizing environmental impact (Kalantari et al., 2017). Overall, the area covered under vertical farming on vegetables can encompass a broad array of structures and scales, from small-scale vertical gardens to multi-storied vertical farms. For example, smaller-scale vertical gardens may cover a relatively small area and be suitable for residential or community use (Kalantari et al., 2017). Conversely, large-scale vertical farms can cover significant areas, such as multi-storied buildings or even entire skyscrapers (Zhu and Marcelis, 2023). These larger vertical farms can maximize space utilization and greatly increase crop yields, providing a sustainable and effective solution for urban vegetable cultivation (Maurya P. et al., 2023). By putting cutting-edge technology like machine learning, artificial intelligence, and the Internet of Things into practice, VF’s quality and efficiency would increase (Swain, 2022).
Conclusion
Vertical farming is aimed to significantly improve food sustainability in urban areas, especially with the projected increase in urban populations. This method offers a range of benefits across social, environmental, and economic aspects compared to traditional rural farming methods. Innovations like hydroponics, aeroponics, and aquaponics are revolutionizing agricultural practices by reducing the reliance on soil-based farming. Vertical farming, which allows for year-round cultivation of crops like maize, potatoes, and vegetables in compact spaces with minimal labour, is particularly advantageous in regions with limited soil and water resources, benefiting marginalized populations. India expects substantial growth in this industry, necessitating the development of cost-effective technologies like low-cost hydroponics. However, ensuring affordability for low-income urban households remains a challenge, exacerbated by issues like slums and food deserts. The rise of vertical farming hinges on various factors including food supply, population size, technological advancements, cultural dietary habits, and access to energy and water resources.
As a technique, vertical farming seeks to improve crop output per unit of land area in order to alleviate the growing strain on agricultural production. It makes use of controlled settings and protected horticultural systems, such as glasshouses, with slope production surfaces or several layers of growth surfaces. Vertical farming has promise, but it’s also expensive and complicated. Technical considerations including lighting, growth systems, nutrition for crops, energy efficiency, building, and site selection must be addressed. To improve its technological and financial characteristics, with an emphasis on increasing production and cutting costs, more research is required.
Currently, vertical farming is predominantly directed by the industry, with limited academic research due to funding constraints, leading to a lack of standardization across different enterprises. Data on its feasibility and crop yield often stems from commercial sources or speculation, highlighting the requisite for more scientific investigations. Collaboration between the vertical farming sector and academia is vital to fully realizing its potential and determining its future expansion as a sustainable food production method.
Author contributions
CS: Conceptualization, Data curation, Formal analysis, Funding acquisition, Investigation, Methodology, Project administration, Resources, Software, Supervision, Validation, Visualization, Writing – original draft. MA: Conceptualization, Data curation, Formal analysis, Software, Supervision, Visualization, Writing – review & editing, Writing – original draft. CI: Data curation, Formal analysis, Investigation, Methodology, Project administration, Resources, Writing – review and editing. GA: Conceptualization, Data curation, Formal analysis, Methodology, Writing – original draft. PJ: Resources, Software, Supervision, Validation, Visualization, Writing – original draft.
Funding
The author(s) declare that no financial support was received for the research, authorship, and/or publication of this article.
Conflict of interest
The authors declare that the research was conducted in the absence of any commercial or financial relationships that could be construed as a potential conflict of interest.
Publisher’s note
All claims expressed in this article are solely those of the authors and do not necessarily represent those of their affiliated organizations, or those of the publisher, the editors and the reviewers. Any product that may be evaluated in this article, or claim that may be made by its manufacturer, is not guaranteed or endorsed by the publisher.
References
Abbasi, A. Z., Islam, N., and Shaikh, Z. A. (2014). A review of wireless sensors and networks’ applications in agriculture. Comput. Stand. Inter. 36, 263–270. doi: 10.1016/j.csi.2011.03.004
Ahmed, Z. F. R., Askri, A., Alnuaimi, A. K. H., Altamimi, A. S. H. R., and Alnaqbi, M. M. A. (2021). Liquid fertilizer as a potential alternative nutrient solution for strawberry production under greenhouse conditions. In III International Symposium on Soilless Culture and Hydroponics: Innovation and Advanced Technology for Circular Horticulture 1321 (pp. 165–172).
Aiswarya, L., Kambale, J., and Rajesh, G. (2023). Application of internet of things (IoT) in protected cultivation: a review. Int. J. Environ. Clim. Change 13, 1518–1529. doi: 10.9734/ijecc/2023/v13i92384
Albadwawi, M. A., Ahmed, Z. F., Kurup, S. S., Alyafei, M. A., and Jaleel, A. (2022). A comparative evaluation of aquaponic and soil systems on yield and antioxidant levels in basil, an important food plant in Lamiaceae. Agronomy 12:3007. doi: 10.3390/agronomy12123007
Al-Kodmany, K. (2018a). The vertical city: A sustainable development model. Johannesburg: WIT Press.
Al-Kodmany, K. (2018b). The vertical farm: a review of developments and implications for the vertical city. Buildings 8:24. doi: 10.3390/buildings8020024
Alneyadi, K. S. S., Almheiri, M. S. B., Tzortzakis, N., Di Gioia, F., and Ahmed, Z. F. R. (2024). Organic-based nutrient solutions for sustainable vegetable production in a zero-runoff soilless growing system. J. Agric. Food Res. 15:101035. doi: 10.1016/j.jafr.2024.101035
Aung, M. M., and Chang, Y. S. (2014). Temperature management for the quality assurance of a perishable food supply chain. Food Control 40, 198–207. doi: 10.1016/j.foodcont.2013.11.016
Avgoustaki, D. D., Avgoustakis, I., Miralles, C. C., Sohn, J., and Xydis, G. (2022). Autonomous mobile robot with attached multispectral camera to monitor the development of crops and detect nutrient and water deficiencies in vertical farms. Agronomy 12:2691. doi: 10.3390/agronomy12112691
Avgoustaki, D. D., and Xydis, G. (2020). “How energy innovation in indoor vertical farming can improve food security, sustainability, and food safety?” in Advances in food security and sustainability. ed. M. J. Cohen (Amsterdam: Elsevier), 1–51.
Bamsey, M., Graham, T., Thompson, C., Berinstain, A., Scott, A., and Dixon, M. (2012). Ion-specific nutrient management in closed systems: the necessity for ion-selective sensors in terrestrial and space-based agriculture and water management systems. Sensors 12, 13349–13392. doi: 10.3390/s121013349
Barrett, G., Alexander, P., Robinson, J., and Bragg, N. (2016). Achieving environmentally sustainable growing media for soilless plant cultivation systems–a review. Sci. Hortic. 212, 220–234. doi: 10.1016/j.scienta.2016.09.030
Basarir, A., Al Mansouri, N. M., and Ahmed, Z. F. (2022). Householders attitude, preferences, and willingness to have home garden at time of pandemics. Horticulturae 8:56. doi: 10.3390/horticulturae8010056
Bechar, A., and Vigneault, C. (2016). Agricultural robots for field operations: concepts and components. Biosyst. Eng. 149, 94–111. doi: 10.1016/j.biosystemseng.2016.06.014
Belista, F. C. L., Go, M. P. C., Luceñara, L. L., Policarpio, C. J. G., Tan, X. J. M., and Baldovino, R. G. (2018). “A smart aeroponic tailored for IoT vertical agriculture using network connected modular environmental chambers.” 2018 IEEE 10th International Conference on Humanoid, Nanotechnology, Information Technology, Communication and Control, Environment and Management (HNICEM).
Benke, K., and Tomkins, B. (2017). Future food-production systems: vertical farming and controlled-environment agriculture. Sustain. Sci. Pract. Policy 13, 13–26. doi: 10.1080/15487733.2017.1394054
Besthorn, F. H. (2013). Vertical farming: social work and sustainable urban agriculture in an age of global food crises. Aust. Soc. Work. 66, 187–203. doi: 10.1080/0312407X.2012.716448
Bhanja, S. N., Mukherjee, A., and Rodell, M. (2018). Groundwater storage variations in India. Groundwater South Asia, 49–59. doi: 10.1007/978-981-10-3889-1_4
Bhowmick, S., Biswas, B., Biswas, M., Dey, A., Roy, S., and Sarkar, S. K. (2019). Application of IoT-enabled smart agriculture in vertical farming. Advances in Communication, Devices and Networking: Proceedings of ICCDN 2018.
Bhushan, M., and Negi, A. (2023). “Impact of UAVs in agriculture” in Handbook of research on machine learning-enabled IoT for smart applications across industries. Eds N. Goel and R. K. Yadav (Hershey, Pennsylvania: IGI Global), 258–268.
Borošić, J., Benko, B., Fabek, S., Novak, B., Dobričević, N., and Bućan, L. (2010). Agronomic traits of soilless grown bell pepper. In XXVIII International Horticultural Congress on Science and Horticulture for People (IHC2010): International Symposium on 927 (pp. 421–428).
Bragg, N. (1990). A review of peat reserves and peat usage in horticulture and alternative materials. Petersfield, Hampshire, UK: Horticultural Development Council.
Cai, J., Veerappan, V., Arildsen, K., Sullivan, C., Piechowicz, M., Frugoli, J., et al. (2023). A modified aeroponic system for growing small-seeded legumes and other plants to study root systems. Plant Methods 19:21. doi: 10.1186/s13007-023-01000-6
Carrasco, G., Fuentes-Penailillo, F., Perez, R., Rebolledo, P., and Manriquez, P. (2022). “An approach to a vertical farming low-cost to reach sustainable vegetable crops.” 2022 IEEE International Conference on Automation/XXV Congress of the Chilean Association of Automatic Control (ICA-ACCA).
Chatterjee, A., Debnath, S., and Pal, H. (2020). “Implication of urban agriculture and vertical farming for future sustainability” in Urban horticulture-necessity of the future Ed. S. S. solankey (London, UK: IntechOpen).
Chittibomma, K., Yadav, N. K., and Reddy, M. G. (2023). Aeroponics: a Polytropic research tool in the new era of agriculture. Int. J. Environ. Clim. Change 13, 214–218. doi: 10.9734/ijecc/2023/v13i81946
Cho, R. (2011). “Vertical farms: from vision to reality.” State of the planet, blogs from the earth institute 13.
Chuah, Y., Lee, J., Tan, S., and Ng, C. (2019). Implementation of smart monitoring system in vertical farming. IOP Conf. Ser.: Earth Environ. Sci. 268:012083. doi: 10.1088/1755-1315/268/1/012083
Conforti, P. (2011) “Looking ahead in world food and agriculture: perspectives to 2050. 2011.” Available at: http://www.fao.org/docrep/014/i2280e/i2280e.pdf
Corvalan, C., Hales, S., and McMichael, A. J. (2005). Ecosystems and human well-being: Health synthesis. Geneva: World Health Organization.
Daponte, P., De Vito, L., Glielmo, L., Iannelli, L., Liuzza, D., Picariello, F., et al. (2019). A review on the use of drones for precision agriculture. IOP Conf. Ser.: Earth Environ. Sci. 275:012022. doi: 10.1088/1755-1315/275/1/012022
De Kreij, C., Voogt, W., and Baas, R. (2003). Nutrient solutions and water quality for soilless cultures. Applied Plant Research, Division Glasshouse.
Debdas, S., Chatterjee, S., Das, S., Das, D., Hazra, S., and Shah, P. B. (2023). “IoT edge based vertical farming.” 2023 World Conference on Communication & Computing (WCONF).
Despommier, D. (2010). The vertical farm: Feeding the world in the 21st century. United States: Macmillan.
Despommier, D. (2013). Farming up the city: the rise of urban vertical farms. Trends Biotechnol. 31, 388–389. doi: 10.1016/j.tibtech.2013.03.008
Despommier, D. (2019). Vertical farms, building a viable indoor farming model for cities. Field Actions Sci. Rep. J. Field Actions (Special Issue 20), 68–73.
Dhanaraju, M., Chenniappan, P., Ramalingam, K., Pazhanivelan, S., and Kaliaperumal, R. (2022). Smart farming: internet of things (IoT)-based sustainable agriculture. Agriculture 12:1745. doi: 10.3390/agriculture12101745
Domeño, I., Irigoyen, I., and Muro, J. (2010). New wood fibre substrates characterization and evaluation in hydroponic tomato culture. Eur. J. Hortic. Sci. 75:89.
Du Jardin, P. (2015). Plant biostimulants: definition, concept, main categories and regulation. Sci. Hortic. 196, 3–14. doi: 10.1016/j.scienta.2015.09.021
Emmerson, M., Morales, M. B., Oñate, J. J., Batary, P., Berendse, F., Liira, J., et al. (2016). “How agricultural intensification affects biodiversity and ecosystem services” in Advances in ecological research A. J. Dumbrell, R. L. Kordas, and G. Woodward. (United States: Elsevier), 43–97.
Farms.com. (2016). Vertical farming to help solve global food security issues. Available at: https://www.farms.com/ag-industry-news/vertical-farming-to-help-solve-global-foodsecurity-issues-007.aspx (Accessed on March 1, 2024).
Fountas, S., Mylonas, N., Malounas, I., Rodias, E., Hellmann Santos, C., and Pekkeriet, E. (2020). Agricultural robotics for field operations. Sensors 20:2672. doi: 10.3390/s20092672
Frazão, J., de Goede, R. G., Capowiez, Y., and Pulleman, M. M. (2019). Soil structure formation and organic matter distribution as affected by earthworm species interactions and crop residue placement. Geoderma 338, 453–463. doi: 10.1016/j.geoderma.2018.07.033
Fussy, A., and Papenbrock, J. (2022). An overview of soil and soilless cultivation techniques—chances, challenges and the neglected question of sustainability. Plan. Theory 11:1153. doi: 10.3390/plants11091153
Ghazali, M. H. M., Azmin, A., and Rahiman, W. (2022). Drone implementation in precision agriculture–a survey. Int. J. Emerg. Technol. Adv. Eng. 12, 67–77. doi: 10.46338/ijetae0422_10
Glick, M. (2023). Robotic bees could support vertical farms today and astronauts tomorrow. Sci. Am. 17. Available at: https://www.scientificamerican.com/article/robotic-bees-could-support-vertical-farms-today-and-astronauts-tomorrow/ (Accessed on 15 February, 2024).
Gokul, A., and Sheeja, P. (2016). Vertical farming: a novel farming technology. Int. J. Mod. Trends Eng. Res. 3, 7–11. doi: 10.21884/ijmter.v3.i7.gx2j5wu9
Gruda, N. S. (2019). Increasing sustainability of growing media constituents and stand-alone substrates in soilless culture systems. Agronomy 9:298. doi: 10.3390/agronomy9060298
Gruda, N. S., and Bragg, N. (2021). “Developments in alternative organic materials for growing media in soilless culture systems” in Advances in horticultural soilless culture. Ed. N. S. Gruda (Cambridge, UK: Burleigh Dodds Science Publishing), 73–106.
Gruda, N., Tucher, S. V., and Schnitzler, W. (2000). N-immobilization by wood fibre substrates in the production of tomato transplants (Lycopersicon lycopersicum (L.) karst. Ex Farw.). Angew. Bot. 74, 32–37.
Halpern, M., Bar-Tal, A., Ofek, M., Minz, D., Muller, T., and Yermiyahu, U. (2015). The use of biostimulants for enhancing nutrient uptake. Adv. Agron. 130, 141–174. doi: 10.1016/bs.agron.2014.10.001
Hasan, Ö., and Dede, Ö. H. (2018). Improving physical properties of plant growing medias using perlite. Sakarya Univ. J. Sci. 22, 1346–1350. doi: 10.16984/saufenbilder.382895
He, J. (2015). “Integrated vertical aeroponic farming systems for vegetable production in space limited environments.” ICESC2015: Hydroponics and Aquaponics at the Gold Coast 1176, 25–36.
Hegedűs, C., Frankó, A., Varga, P., Gindl, S., and Tauber, M. (2023). “Enabling scalable smart vertical farming with IoT and machine learning technologies.” NOMS 2023–2023 IEEE/IFIP Network Operations and Management Symposium.
Hernández, R., Trigal, C., Cañamás, T. P., and Escolà, A. (2018). Integration of renewable energies in urban vertical farms: a review. Renew. Sust. Energ. Rev. 82, 647–659.
Horst, W., Schenk, M., Bürkert, A., Claassen, N., Flessa, H., Frommer, W., et al. (2006). Plant nutrition: Food security and sustainability of agro-ecosystems through basic and applied research, vol. 92. New York, Boston, Moscow, Dordrecht, London: Kluwer Academic Publishers, Springer Science & Business Media.
Jackson, W. (2023). “New roots for agriculture” in Resettling America. Ed. J. Pretty (United Kingdom, United States: Routledge), 498–515.
Jürkenbeck, K., Heumann, A., and Spiller, A. (2019). Sustainability matters: consumer acceptance of different vertical farming systems. Sustain. For. 11:4052. doi: 10.3390/su11154052
Kabir, M. S. N., Reza, M. N., Chowdhury, M., Ali, M., Samsuzzaman, M. R., Ali, K. Y. L., et al. (2023). Technological trends and engineering issues on vertical farms: a review. Horticulturae 9:1229. doi: 10.3390/horticulturae9111229
Kalantari, F., Mohd Tahir, O., Mahmoudi Lahijani, A., and Kalantari, S. (2017). A review of vertical farming technology: a guide for implementation of building integrated agriculture in cities. Adv. Eng. Forum 24, 76–91. doi: 10.4028/www.scientific.net/AEF.24.76
Kato, K., Yoshida, R., Kikuzaki, A., Hirai, T., Kuroda, H., Hiwasa-Tanase, K., et al. (2010). Molecular breeding of tomato lines for mass production of miraculin in a plant factory. J. Agric. Food Chem. 58, 9505–9510. doi: 10.1021/jf101874b
Kawasaki, Y., and Yoneda, Y. (2019). Local temperature control in greenhouse vegetable production. Hortic. J. 88, 305–314. doi: 10.2503/hortj.UTD-R004
Khalil, H. I., and Wahhab, K. A. (2020). Advantage of vertical farming over horizontal farming in achieving sustainable city, Baghdad city-commercial street case study. IOP Conf. Ser.: Mater. Sci. Eng. 745:012173. doi: 10.1088/1757-899X/745/1/012173
Kim, J., Kim, S., Ju, C., and Son, H. I. (2019). Unmanned aerial vehicles in agriculture: a review of perspective of platform, control, and applications. IEEE Access 7, 105100–105115. doi: 10.1109/ACCESS.2019.2932119
Klassen, S. P., Bugbee, B., and Monje, O. (2020). “Aeroponic and hydroponic systems for the cultivation of plants” in Comprehensive biotechnology. Ed. M. Moo-Young Third ed (Springer: Academic Press), 330–344.
Koolhaas, R. (2014). Delirious New York: A retroactive manifesto for. Manhattan: The Monacelli Press, LLC.
Kozai, T. (2012). “Sustainable plant factory: closed plant production systems with artificial light for high resource use efficiencies and quality produce.” International Symposium on Soilless Cultivation 1004.
Krucker, M., Hummel, R. L., and Cogger, C. (2010). Chrysanthemum production in composted and noncomposted organic waste substrates fertilized with nitrogen at two rates using surface and subirrigation. HortScience 45, 1695–1701. doi: 10.21273/HORTSCI.45.11.1695
Lakhiar, I. A., Jianmin, G., Syed, T. N., Chandio, F. A., Buttar, N. A., and Qureshi, W. A. (2018). Monitoring and control systems in agriculture using intelligent sensor techniques: a review of the aeroponic system. J Sens 2018, 1–18. doi: 10.1155/2018/8672769
Lal, R. (2009). Soil degradation as a reason for inadequate human nutrition. Food Secur. 1, 45–57. doi: 10.1007/s12571-009-0009-z
Lal, R. (2015). Restoring soil quality to mitigate soil degradation. Sustain. For. 7, 5875–5895. doi: 10.3390/su7055875
Lambin, E. F. (2012). Global land availability: Malthus versus Ricardo. Glob. Food Sec. 1, 83–87. doi: 10.1016/j.gfs.2012.11.002
Lambin, E. F., and Meyfroidt, P. (2011). Global land use change, economic globalization, and the looming land scarcity. Proc. Natl. Acad. Sci. 108, 3465–3472. doi: 10.1073/pnas.1100480108
Landis, T. D., Jacobs, D. F., Wilkinson, K. M., and Luna, T. (1990). “Growing media” in The container tree nursery manual. vol 2. Eds. K. M. Wilkinson, T. D. Landis, D. L. Haase, B. F. Daley, and R. K. Dumroese (Department of Agriculture, Forest Service, Agriculture Handbook), 41–85.
Lawrence, I. D., Vijayakumar, R., and Agnishwar, J. (2023). “Dynamic application of unmanned aerial vehicles for analyzing the growth of crops and weeds for precision agriculture” in Artificial intelligence tools and technologies for smart farming and agriculture practices Eds. R. K. Gupta, A. Jain, J. Wang, S. K. Bharti, and S. B. Patel (USA: IGI Global), 115–132.
Lehman, R. M., Cambardella, C. A., Stott, D. E., Acosta-Martinez, V., Manter, D. K., Buyer, J. S., et al. (2015). Understanding and enhancing soil biological health: the solution for reversing soil degradation. Sustain. For. 7, 988–1027. doi: 10.3390/su7010988
Maher, M., Prasad, M., and Raviv, M. (2008). “Organic soilless media components” in Soilless culture: theory and practice. eds. M. Raviv and J. H. Lieth (Amsterdam: Elsevier), 459–504.
Maheshwari, S. (2021). “Vertical farming: resilience towards climate change” in Urban growth and environmental issues in India. eds. A. Kateja and R. Jain (Singapore: Springer), 207–221.
Marchant, W., and Tosunoglu, S. (2017). “Robotic implementation to automate a vertical farm system.” In Proceedings of the 30th Florida Conference on Recent Advances in Robotics (pp. 11–12). Available at: https://public.eng.fau.edu/design/fcrar2017/papers/RoboticImplementation.pdf (Accessed March 04, 2024).
Marković, D., Koprivica, R., Pešović, U., and Randić, S. (2015). Application of IoT in monitoring and controlling agricultural production. Acta Agric. Serbica 20, 145–153. doi: 10.5937/AASer1540145M
Marschner, H. (2011). Marschner’s mineral nutrition of higher plants. Exeter, England, United Kingdom: Academic Press.
Martínez, G., Laguna, A. M., Giráldez, J. V., and Vanderlinden, K. (2021). Concurrent variability of soil moisture and apparent electrical conductivity in the proximity of olive trees. Agric. Water Manag. 245:106652. doi: 10.1016/j.agwat.2020.106652
Massa, G. D., Kim, H.-H., Wheeler, R. M., and Mitchell, C. A. (2008). Plant productivity in response to LED lighting. HortScience 43, 1951–1956. doi: 10.21273/HORTSCI.43.7.1951
Matson, P. A., Parton, W. J., Power, A. G., and Swift, M. J. (1997). Agricultural intensification and ecosystem properties. Science 277, 504–509. doi: 10.1126/science.277.5325.504
Maurya, P., Jain, S., Kumar, V., Kiran, B., Singh, P., Nishad, U., et al. (2023). Vertical farming: an option in modern food production: a review. Int. J. Environ. Clim. Change 13, 883–893. doi: 10.9734/ijecc/2023/v13i92308
Maurya, P. K., Karde, R. Y., Bayskar, A. S., and Charitha, N. (2023). Innovative technologies such as vertical farming and hydroponics to grow crops in controlled environments. Adv. Farming Technol. 84–107.
Mehra, M., Saxena, S., Sankaranarayanan, S., Tom, R. J., and Veeramanikandan, M. (2018). IoT based hydroponics system using deep neural networks. Comput. Electron. Agric. 155, 473–486. doi: 10.1016/j.compag.2018.10.015
Michael, G., Tay, F., and Then, Y. (2021). Development of automated monitoring system for hydroponics vertical farming. J. Phys.: Conf. Ser. 1844:012024. doi: 10.1088/1742-6596/1844/1/012024
Mishra, N., Hangshing, L., Kadam, D. S., Tapang, T., and Shameena, S. (2024). Advances in Vertical Farming: Opportunities and Challenges. J. Sci. Res. doi: 10.9734/jsrr/2024/v30i82241
Michel, J.-C. (2010). The physical properties of peat: a key factor for modern growing media. Mires Peat 6, 1–6.
Mir, M. S., Naikoo, N. B., Kanth, R. H., Bahar, F., Bhat, M. A., Nazir, A., et al. (2022). Vertical farming: the future of agriculture: a review. Pharm. Innov. J. 11, 1175–1195.
Morella, P., Lambán, M. P., Royo, J., and Sánchez, J. C. (2023). Vertical farming monitoring: how does it work and how much does it cost? Sensors 23:3502. doi: 10.3390/s23073502
Nair, A., Chacko, A., Mohan, G. V. K., and Francis, T. K. (2017). “Smart vertical farming using hydroponics.”
Nájera, C., Gallegos-Cedillo, V. M., Ros, M., and Pascual, J. A. (2022). LED lighting in vertical farming systems enhances bioactive compounds and productivity of vegetables crops. Biol. Life Sci. Forum 16:24. doi: 10.3390/IECHo2022-12514
Naskali, A. T., Pinarer, O., and Tolga, A. C. (2022). Vertical farming: under climate change effect. Environment and climate-smart food production, 259–284. doi: 10.1007/978-3-030-71571-7_8
Nguyen, T. K. L., Cho, K. M., Lee, H. Y., Cho, D. Y., Lee, G. O., Jang, S. N., et al. (2021). Effects of white LED lighting with specific shorter blue and/or green wavelength on the growth and quality of two lettuce cultivars in a vertical farming system. Agronomy 11:2111. doi: 10.3390/agronomy11112111
Nunes, E. (2023). “Employing drones in agriculture: an exploration of various drone types and key Advantag.” arXiv [Preprint] arXiv:2307.04037.
Obalum, S., Ezenne, G., Watanabe, Y., and Wakatsuki, T. (2011). Contemporary global issue of rising water scarcity for agriculture: the quest for effective and feasible soil moisture and free-water surface conservation strategies. J. Water Resource Prot. 3, 166–175. doi: 10.4236/jwarp.2011.33021
Oh, S., and Lu, C. (2023). Vertical farming-smart urban agriculture for enhancing resilience and sustainability in food security. J. Hortic. Sci. Biotechnol. 98, 133–140. doi: 10.1080/14620316.2022.2141666
Okomoda, V. T., Oladimeji, S. A., Solomon, S. G., Olufeagba, S. O., Ogah, S. I., and Ikhwanuddin, M. (2023). Aquaponics production system: a review of historical perspective, opportunities, and challenges of its adoption. Food Sci. Nutr. 11, 1157–1165. doi: 10.1002/fsn3.3154
Olle, M., and Viršile, A. (2013). The effects of light-emitting diode lighting on greenhouse plant growth and quality. Agric. Food Sci. 22, 223–234. doi: 10.23986/afsci.7897
Pandey, J. K., Kumar, A. P., Misra, M., Mohanty, A. K., Drzal, L. T., and Palsingh, R. (2005). Recent advances in biodegradable nanocomposites. J. Nanosci. Nanotechnol. 5, 497–526. doi: 10.1166/jnn.2005.111
Papadopoulos, A. P., Bar-Tal, A., Silber, A., Saha, U. K., and Raviv, M. (2008). “Inorganic and synthetic organic components of soilless culture and potting mixes” in Soilless culture: theory and practice. eds. M. Raviv and J. H. Lieth (Amsterdam: Elsevier), 505–544.
Park, Y., Seol, J., Pak, J., Jo, Y., Kim, C., and Son, H. I. (2023). Human-centered approach for an efficient cucumber harvesting robot system: harvest ordering, visual servoing, and end-effector. Comput. Electron. Agric. 212:108116. doi: 10.1016/j.compag.2023.108116
Paul, K., Chatterjee, S. S., Pai, P., Varshney, A., Juikar, S., Prasad, V., et al. (2022). Viable smart sensors and their application in data driven agriculture. Comput. Electron. Agric. 198:107096. doi: 10.1016/j.compag.2022.107096
Paulus, S., Behmann, J., Mahlein, A.-K., Plümer, L., and Kuhlmann, H. (2014). Low-cost 3D systems: suitable tools for plant phenotyping. Sensors 14, 3001–3018. doi: 10.3390/s140203001
Poulter, R. (2011). “Quantifying differences between treated and untreated coir substrate.” In International Symposium on Organic Matter Management and Compost Use in Horticulture 1018.
Ptak, M., Wasieńko, S., and Makuła, P. (2024). A new approach for vertical plant cultivation maximizing crop efficiency. doi: 10.20944/preprints202406.1047
Puri, V., Nayyar, A., and Raja, L. (2017). Agriculture drones: a modern breakthrough in precision agriculture. J. Stat. Manag. Syst. 20, 507–518. doi: 10.1080/09720510.2017.1395171
Qu, B., Xiao, Z., Upadhyay, A., and Luo, Y. (2024). Perspectives on sustainable food production system: characteristics and green technologies. J. Agric. Food Res. 15:100988. doi: 10.1016/j.jafr.2024.100988
Quy, V. K., Hau, N. V., Anh, D. V., Quy, N. M., Ban, N. T., Lanza, S., et al. (2022). IoT-enabled smart agriculture: architecture, applications, and challenges. Appl. Sci. 12:3396. doi: 10.3390/app12073396
Rajan, P., Lada, R. R., and MacDonald, M. T. (2019). Advancement in indoor vertical farming for microgreen production. Am. J. Plant Sci. 10, 1397–1408. doi: 10.4236/ajps.2019.108100
Ramchiary, D., Nirmala, B., Baishya, S., and Raibaruah, A. K. (2022). “Aquaponics-a smart automated feedback control process for cultivation.” 2022 IEEE International Power and Renewable Energy Conference (IPRECON).
Ravansari, R., Wilson, S. C., Wilson, B. R., and Tighe, M. (2021). Rapid PXRF soil organic carbon and organic matter assessment using novel modular radiation detector assembly. Geoderma 382:114728. doi: 10.1016/j.geoderma.2020.114728
Reddy, N., and Reddy, N. (2019). “Agricultural applications of coir” in Sustainable applications of coir and other coconut by-products. Ed. N. reddy (Springer International Publishing). 31–54.
Reddy, N., and Yang, Y. (2005). Biofibers from agricultural byproducts for industrial applications. Trends Biotechnol. 23, 22–27. doi: 10.1016/j.tibtech.2004.11.002
Resh, H. M. (2022). Hydroponic food production: A definitive guidebook for the advanced home gardener and the commercial hydroponic grower. Boca Raton: CRC Press.
Rezk, N. G., Hemdan, E. E.-D., Attia, A.-F., El-Sayed, A., and El-Rashidy, M. A. (2021). An efficient IoT based smart farming system using machine learning algorithms. Multimed. Tools Appl. 80, 773–797. doi: 10.1007/s11042-020-09740-6
Rivero, W., and Salvi, D. (2023). “Vertical farming” in The produce contamination problem Eds. R. Karl and M. Deepti Selvi (USA: Academic Press), 267–295.
Rosenzweig, C., Elliott, J., Deryng, D., Ruane, A. C., Müller, C., Arneth, A., et al. (2014). Assessing agricultural risks of climate change in the 21st century in a global gridded crop model intercomparison. Proc. Natl. Acad. Sci. 111, 3268–3273. doi: 10.1073/pnas.1222463110
Saad, M. H. M., Hamdan, N. M., and Sarker, M. R. (2021). State of the art of urban smart vertical farming automation system: advanced topologies, issues and recommendations. Electronics 10:1422. doi: 10.3390/electronics10121422
Sabatino, L. (2020). Increasing sustainability of growing media constituents and stand-alone substrates in soilless culture systems—an editorial. MDPI 10. 1–3. doi: 10.3390/agronomy10091384
Sani, A., Mohammadi, M., and Moghimi, A. (2020). Potential of vertical farming and aeroponics for horticultural crop production. Hortic. Plant J. 6, 1–11.
Sanyé-Mengual, E., Orsini, F., Oliver-Solà, J., Rieradevall, J., Montero, J. I., and Gianquinto, G. (2015). Techniques and crops for efficient rooftop gardens in Bologna, Italy. Agronomy for Sustainable Development, 35, 1477–1488. doi: 10.1007/s13593-015-0331-0
Sathyanarayan, S. R., Warke, V. G., Mahajan, G. B., and Annapure, U. S. (2023). Soil free nutrient availability to plants. J. Plant Nutr. 46, 801–814. doi: 10.1080/01904167.2022.2071736
Schima, R., Mollenhauer, H., Grenzdörffer, G., Merbach, I., Lausch, A., Dietrich, P., et al. (2016). Imagine all the plants: evaluation of a light-field camera for on-site crop growth monitoring. Remote Sens. 8:823. doi: 10.3390/rs8100823
Schmilewski, G. (2008). The role of peat in assuring the quality of growing media. Mires Peat 3, 1–8.
Sengupta, A., and Banerjee, H. (2012). Soil-less culture in modern agriculture. World J. Sci. Technol 2, 103–108.
Shamshiri, R., Weltzien, C., Hameed, I. A., Yule, I. J., Grift, T. E., Balasundram, S. K., et al. (2018). Research and development in agricultural robotics: a perspective of digital farming. Int. J. Agric. Biol. Eng. 11, 1–14. doi: 10.25165/j.ijabe.20181104.4278
SharathKumar, M., Heuvelink, E., and Marcelis, L. F. (2020). Vertical farming: moving from genetic to environmental modification. Trends Plant Sci. 25, 724–727. doi: 10.1016/j.tplants.2020.05.012
Sharma, N., Acharya, S., Kumar, K., Singh, N., and Chaurasia, O. P. (2018). Hydroponics as an advanced technique for vegetable production: an overview. J. Soil Water Conserv. 17, 364–371. doi: 10.5958/2455-7145.2018.00056.5
Singh, B. K., Delgado-Baquerizo, M., Egidi, E., Guirado, E., Leach, J. E., Liu, H., et al. (2023). Climate change impacts on plant pathogens, food security and paths forward. Nat. Rev. Microbiol. doi: 10.1038/s41579-023-00900-7
Singh, N., and Singh, A. N. (2020). Odysseys of agriculture sensors: current challenges and forthcoming prospects. Comput. Electron. Agric. 171:105328. doi: 10.1016/j.compag.2020.105328
Siskandar, R., Santosa, S. H., Wiyoto, B. R. K., and Hidayat, A. P. (2022). “Control and automation: Insmoaf (integrated smart modern agriculture and fisheries) on the greenhouse model (Kontrol dan Otomatisasi: Insmoaf (integrated smart modern agriculture and fisheries) pada model Rumah Kaca).”
Sivamani, S., Bae, N., and Cho, Y. (2013). A smart service model based on ubiquitous sensor networks using vertical farm ontology. Int. J. Distrib. Sens. Netw. 9:161495. doi: 10.1155/2013/161495
Specht, K., Siebert, R., Hartmann, I., Freisinger, U. B., Sawicka, M., Werner, A., et al. (2014). Urban agriculture of the future: an overview of sustainability aspects of food production in and on buildings. Agric. Hum. Values 31, 33–51. doi: 10.1007/s10460-013-9448-4
Spielman, D. J., and Pandya-Lorch, R. (Eds.) (2009). Millions fed: Proven successes in agricultural development, vol. 873. Eds. Spielman, D. J., and Pandya-Lorch, R. Washington, DC, USA: Intl Food Policy Res Inst.
Starostin, I., Eshchin, A., and Davydova, S. (2023). Global trends in the development of agricultural robotics. IOP Conf. Ser.: Earth Environ. Sci. 1138:012042. doi: 10.1088/1755-1315/1138/1/012042
Stelte, W., Reddy, N., Barsberg, S., and Sanadi, A. R. (2023). Coir from coconut processing waste as a raw material for applications beyond traditional uses. Bioresources 18, 2187–2212. doi: 10.15376/biores.18.1.Stelte
Swain, M. (2022). “Vertical farming trends and challenges: a new age of agriculture using IoT and machine learning.” In R. Singh, A. K. Thakur, A. Gehlot, and A. K. Kaviti, eds, Internet of things for agriculture 4.0, 1–16. Burlington: Apple Academic Press
Taher Abdelfatah, M., and Mahmoud El-Arnaouty, S. (2023). A review of vertical farming for sustainable urban food security. J. Arts Humanit. 6, 214–231. doi: 10.21608/mjas.2023.204089.1139
Tala, S., Al-Ajlouni, M. G., Ayad, J. Y., Othman, Y. A., and Hilaire, R. S. (2020). Performance of six different soilless green roof substrates for the Mediterranean region. Sci. Total Environ. 730:139182. doi: 10.1016/j.scitotenv.2020.139182
Thomaier, S., Specht, K., Henckel, D., Dierich, A., Siebert, R., Freisinger, U. B., et al. (2015). Farming in and on urban buildings: present practice and specific novelties of zero-acreage farming (ZFarming). Renew. Agric. Food Syst. 30, 43–54. doi: 10.1017/S1742170514000143
Tilman, D., Fargione, J., Wolff, B., D’antonio, C., Dobson, A., Howarth, R., et al. (2001). Forecasting agriculturally driven global environmental change. Science 292, 281–284. doi: 10.1126/science.1057544
Touliatos, D., Dodd, I. C., and McAinsh, M. (2016). Vertical farming increases lettuce yield per unit area compared to conventional horizontal hydroponics. Food Energy Secur. 5, 184–191. doi: 10.1002/fes3.83
Trejo-Téllez, L. I., and Gómez-Merino, F. C. (2012). “Nutrient solutions for hydroponic systems” in Hydroponics – a standard methodology for plant biological researches. ed. T. Asao (London: InTech), 1–22.
Tsouros, D. C., Bibi, S., and Sarigiannidis, P. G. (2019). A review on UAV-based applications for precision agriculture. Information 10:349. doi: 10.3390/info10110349
Ullah, R., Asghar, I., Griffiths, M. G., Stacey, C., Stiles, W., and Whitelaw, C. (2023). “Internet of things based sensor system for vertical farming and controlled environment agriculture.” 2023 6th conference on cloud and internet of things (CIoT).
Van Delden, S., SharathKumar, M., Butturini, M., Graamans, L., Heuvelink, E., Kacira, M., et al. (2021). Current status and future challenges in implementing and upscaling vertical farming systems. Nat. Food 2, 944–956. doi: 10.1038/s43016-021-00402-w
Van Gerrewey, T., Boon, N., and Geelen, D. (2021). Vertical farming: the only way is up? Agronomy 12:2. doi: 10.3390/agronomy12010002
Vargas, S. A. V., Navarrete, Ó. L. G., and Gómez, M. A. C. (2022). Automated, technified and traditional aquaponic systems: a systematic review. Ing. Solidar. 18, 1–39. doi: 10.16925/2357-6014.2022.02.02
Varuzhanyan, A. A., Varuzhanyan, A. A., and Varuzhanyan, H. (2006). A mechanism of perlite expansion. Inorg. Mater. 42, 1039–1045. doi: 10.1134/S0020168506090202
Walker, B. (1999). The terrestrial biosphere and global change: implications for natural and managed ecosystems. Vol. 4: Cambridge University Press.
Wang, W., and Huang, G. (2009). Characterisation and utilization of natural coconut fibres composites. Mater. Des. 30, 2741–2744. doi: 10.1016/j.matdes.2008.11.002
Wang, X., Onychko, V., Zubko, V., Wu, Z., and Zhao, M. (2023). Sustainable production systems of urban agriculture in the future: a case study on the investigation and development countermeasures of the plant factory and vertical farm in China. Front. Sustain. Food Syst. 7:973341. doi: 10.3389/fsufs.2023.973341
Wang, T., Xu, X., Wang, C., Li, Z., and Li, D. (2021). From smart farming towards unmanned farms: a new mode of agricultural production. Agriculture 11:145. doi: 10.3390/agriculture11020145
Wang, Y. P., and Kintrea, K. (2021). Urban expansion and land use changes in Asia and Africa. Environment and Urbanization Asia. doi: 10.1177/0975425321999081
Wheeler, R. M. (2022). “NASA s contributions to vertical farming.” XXXI International Horticultural Congress (IHC2022): International Symposium on Advances in Vertical Farming 1369.
Yeh, N., and Chung, J.-P. (2009). High-brightness LEDs—energy efficient lighting sources and their potential in indoor plant cultivation. Renew. Sust. Energ. Rev. 13, 2175–2180. doi: 10.1016/j.rser.2009.01.027
Yin, H., Cao, Y., Marelli, B., Zeng, X., Mason, A. J., and Cao, C. (2021). Soil sensors and plant wearables for smart and precision agriculture. Adv. Mater. 33:2007764. doi: 10.1002/adma.202007764
Yu-Peng, Y. (2010). “Green Wall-the creative solution in response to the urban Heat Island effect.” National Chung-Hsing University.
Zaręba, A., Krzemińska, A., and Kozik, R. (2021). Urban vertical farming as an example of nature-based solutions supporting a healthy society living in the urban environment. Resources 10:109. doi: 10.3390/resources10110109
Zhou, H., Specht, K., and Kirby, C. K. (2022). Consumers’ and stakeholders’ acceptance of indoor Agritecture in Shanghai (China). Sustain. For. 14:2771. doi: 10.3390/su14052771
Zhu, X.-G., and Marcelis, L. (2023). Vertical farming for crop production. Mod. Agric. 1, 13–15. Available at: https://en.wikipedia.org/wiki/Vertical_farming#Technologies_and_devices
Keywords: vertical farming, types of vertical farming, sensors, drones, robots
Citation: Sowmya C, Anand M, Indu Rani C, Amuthaselvi G and Janaki P (2024) Recent developments and inventive approaches in vertical farming. Front. Sustain. Food Syst. 8:1400787. doi: 10.3389/fsufs.2024.1400787
Edited by:
Maria Inês Dias, Centro de Investigação de Montanha (CIMO), PortugalReviewed by:
Ajit Singh, University of Nottingham Malaysia Campus, MalaysiaZienab F. R. Ahmed, United Arab Emirates University, United Arab Emirates
Copyright © 2024 Sowmya, Anand, Indu Rani, Amuthaselvi and Janaki. This is an open-access article distributed under the terms of the Creative Commons Attribution License (CC BY). The use, distribution or reproduction in other forums is permitted, provided the original author(s) and the copyright owner(s) are credited and that the original publication in this journal is cited, in accordance with accepted academic practice. No use, distribution or reproduction is permitted which does not comply with these terms.
*Correspondence: M. Anand, anandhort@gmail.com; Chennu Sowmya, chennusowmyareddy@gmail.com