- 1Department of Horticulture and Landscape Architecture, Colorado State University, Fort Collins, CO, United States
- 2Department of Entomology, Texas A&M University, College Station, TX, United States
There are many benefits to producing insects for food and feed; they require fewer resources to produce, process, and distribute. The digested and undigested waste along with insect feces (i.e., frass) from the mass production of insects can be considerable. Black soldier fly larvae (BSFL) digestion of organic residue produces frass that is high in macronutrients that are desirable for plants, potentially serving as a partial replacement for fertilizer or growing media, such as peat. Arugula and lettuce were grown in greenhouse pot studies with treatments comprised of BSFL frass (BSF), vermicompost (VC), and peat, and compared to a 100% peat control (CP). Yield, productivity, greenness, and tissue nutrient concentrations were measured. Arugula and lettuce produced the highest fresh weight and dry weight in the BSFL treatments. Primary macronutrients (N, P, K) and Mg in both crops were also highest in the BSFL treatments. Secondary macronutrients (Ca, Mg, S) and micronutrients produced more variable results (B, Fe, Mn, Cu, Zn). Lettuce produced larger yields but had much lower concentrations of nutrients compared to arugula. In small amounts, 10–20%, BSFL frass can serve as a good replacement for peat in leafy green crops. It is an especially beneficial way to recycle organic side streams in different industries and reduce waste production overall.
1 Introduction
Leafy vegetables are global staples because of their high fiber, antioxidant content, and extensively established cultivation methods (Shatilov et al., 2019). Lettuce (Lactuca sativa, L. Asteraceae), has important commercial value for the United States and can be successfully grown on a diversity of soil types, making it an ideal crop for this study. Additionally, romaine lettuce is known to thrive well in irrigated environments, like the western United States. For example, between 2015 and 2017, the ten major producing countries exported 5,010,843,109 kg of lettuce, which had an estimated value of $5,936,751,407 (Shatilov et al., 2019). Arugula (Eruca sativa, L. Brassicaceae) and lettuce are considered cool season crops because optimal temperatures do not rise above 23°C (Swiader and Ware, 2002). Arugula is a less commercially important crop than lettuce but offers more nutritional and culinary value (Shubha et al., 2019). For example, arugula is high in the anti-carcinogenic compound, sulforaphane (Shubha et al., 2019). Many of the cultivation and harvest techniques are similar between the two crops as well.
Plant growth requirements vary by species, but certain requirements, such as light, water, and nutrients are ubiquitous necessities across the plant kingdom. Important macronutrients that are often limiting for plants are: nitrogen (N), phosphorus (P), and potassium (K). Less limiting macronutrients, or secondary nutrients, are: calcium (Ca), magnesium (Mg), and sulfur (S). Micronutrients that are also required for plant health but needed in relatively small amounts are boron (B), iron (Fe), manganese (Mn), copper (Cu), Nickel (Ni), and zinc (Zn) (Swiader and Ware, 2002). Other nutrients like sodium (Na) and aluminum (Al) are only required by certain plants and under certain conditions (Chapin et al., 2011).
Black soldier fly (Hermetia illucens, L. Stratiomyidae) larvae (BSFL) have been industrialized in part because they are high in protein (Miranda et al., 2019), and they are efficient at converting organic by-products to insect biomass (Diener et al., 2009). Though they reduce the overall waste streams, residual material (i.e., frass) is generated. Frass is the material remaining after digestion by the BSFL; however, many describe the residual, which includes the undigested materials and shed exoskeletons together with the digested material, as frass. For the purpose of this publication, the definition of frass will be all inclusive.
The original waste material (such as feed stock) fed to the BSFL is altered by their gut microbiota in association with digestion (Kagata and Ohgushi, 2012). After digestion, N, P, and K become more bioavailable for potential plant uptake during development. Overall, total nutrients are reduced and any potential leaching and loss to neighboring environments may also be reduced (Kagata and Ohgushi, 2012). Due to its concentration of N and P, frass may serve as a supplement or substitute for inorganic fertilizers that are typically applied during the crop production cycle (Schmitt, 2020). Decreases in inorganic fertilizer application can reduce subsequent environmental problems, such as soil degradation and eutrophication (Xiao et al., 2018).
One area of interest for growers is to incorporate frass as a partial replacement for peat (Setti et al., 2019). Peat is currently one of the most common resources utilized in controlled environment agriculture (Niu and Masabni, 2018). Peat is comprised of decomposing plants, as well as minerals, and water, in marsh environments (Bloom, 1964; Cameron et al., 1989). Unfortunately, peat mining is currently the cause of serious environmental concerns. Additionally, the use of peat-based substrates is increasing at an unsustainable rate (i.e., use exceeds the rate of replacement). In fact, barren peat marshes resulting from extraction, or mining, experience severe disruption in microbial processes. In unaltered peat bogs, methanotrophic bacteria provide a ‘biofilter’ for methane, that balances the C and N cycles. When these ecosystems are disturbed, network connectivity is lost, and the marshes become large sources of methane emission (Kaupper et al., 2021). High methane emissions range from 1,300 to 3,800 kg per hectare per year (Couwenberg et al., 2011). Furthermore, wildlife diversity and richness also see significant reductions after mining occurs.
Several studies investigated the use of insect frass for application in horticultural production. Chavez et al. (2023) compared BSFL frass to vermicompost (VC) in tomato (Solanum lycopersicum, L., Solanaceae) production. In most treatments, BSFL frass produced comparable results in tomato fruit yield, quality, plant biomass, and greenness to the control. Klammsteiner et al. (2019) observed similar results in ryegrass (Lolium perenne, L., Poaceae). In this study BSFL frass was produced from chicken, fruits/vegetables, and grass-cutting feed and compared to a conventional fertilizer control. There were no differences in plant biomass between treatments and/or control indicating the BSFL frass could be a replacement. Other studies that utilized BSFL frass as a growth medium replacement for peat in tomato, basil (Ocimum basilicum, L., Lamiaceae), and lettuce crops discovered similar results (Setti et al., 2019). Peat mixtures with 10% frass adequately replaced a 100% peat based medium. Another study compared the application of BSFL frass (produced from digested coffee husks) with raw cow manure and undigested coffee husks to grow lettuce crops. The greatest lettuce dry and wet weights were found in the raw manure treatments, though the frass treatments did achieve significantly greater lettuce wet and dry weights than plants grown on the undigested coffee husks (Putra et al., 2017). BSFL frass was applied as a top dressing in two treatments (333 g/m2 and 667 g/m2) to sweet potato (Ipomoea batatas, L., Convolvulaceae) slips, produced similarities in length, number of nodes, stem diameter, and chlorophyll between the control and treatments (Romano et al., 2023).
Soil characteristics may also experience alterations with frass applications (Houben et al., 2020). The nutrient profiles of various raw manures and mealworm (Tenebrio molitar, L., Tenebrionidae) frass from Ÿnsect (ŸnFrass, Paris, France) were compared. Similar concentrations of N, P, and K were observed. Additionally, N mineralization and decomposition in the frass treatments occurred more quickly than in raw manure (Houben et al., 2020). Lower soluble P concentrations were also observed in the frass treatments. Increases in P bioavailability from BSFL frass treated samples have been reported in other studies (Klammsteiner et al., 2019). BSFL frass treatments have also produced improvements in soil organic matter (Zahn and Quilliam, 2017).
Insect frass has been studied mostly for the purpose of understanding its impacts on yield and other plant growth parameters, but only a few studies have monitored the impact on plant nutrition. The nutrient concentration in leaves of lettuce provided BSFL frass in a greenhouse were observed (Esteves et al., 2022). Treatments were comprised of varying blends of mineral fertilizer and BSFL frass to complete a 100% N profile. Once again, as with other previous studies discussed, there were no differences in K utilization; however, N, Fe, and Mg concentrations were highest in the mineral fertilizer only control. It should be noted P was lowest in the high BSFL frass treatments and Mg was highest in the high frass treatments. Na, Ca, Cu, and Zn were highest in the unfertilized control and the low frass treatment. These data indicate formulations are needed to optimize mineral uptake from BSFL frass by such plants.
To further demonstrate this point, a study compared the impact of raw cow (Bos taurus, L., Bovidae) manure with regular coffee husks and coffee husks that had been digested by BSFL on lettuce production (Putra et al., 2017). Differences in lettuce nutrient utilization depending on treatment were observed. N utilization was highest in the raw manure treatments. However, P and K utilization were highest in the BSFL treatment. Another study analyzed cabbage tissues after the application of insect frass compost compared to a commercial fertilizer (Choi et al., 2009). Contrary to the results from the coffee husk study, P was the only mineral content measured that produced significantly lower P concentrations in the insect frass compost treatment (Choi et al., 2009).
Another greenhouse pot study measured the NPK concentrations of barley (Hardeum vulgare, L., Poaceae) grown with a conventional fertilizer treatment, mealworm frass, a 50%frass/50% fertilizer, and an unfertilized control (Houben et al., 2020). There were no significant differences between the three treatments, but all NPK concentrations of barley were higher in the treatments than the control (Houben et al., 2020). Maize (Zea mays, L., Poaceae) provided insect frass, insect larval skins, insect adult bodies, organic fertilizers, or inorganic fertilizers was harvested and analyzed for nutrient content (Gärttling et al., 2020). N concentrations were lowest in the frass and larval skin treatments, and highest in the adult body and inorganic fertilizer treatments. The opposite was observed for P concentrations; percent P was highest in frass treatments and lowest in adult body and organic fertilizer treatments (Gärttling et al., 2020). In sweet potato slips there were no differences in mineral composition of treatments pertaining to the two top dressing dosages of BSFL frass, including K, P, Cu, Mg, Ni, and Zn. Cu and Fe were highest in the control and Mn was highest in the BSFL frass treatments (Romano et al., 2023).
A natural parallel to insect frass that is commonly used in horticultural production, and previously mentioned, is VC residual, which is the left-over product from worm digestion. This C-based substrate is commonly used in soil and fertility amendment for crop growth, especially in organic and specialty crop production (Tammam et al., 2022). Since insect frass and VC are produced through a similar process of digestion, it is likely that insect frass could also be adopted in a similar manner. While results vary, studies demonstrate that mixtures of VC and peat have positive impacts on crop production. The most effective treatments were augmented with 10 to 50% VC in peat based potting mixtures (Chavez and Uchanski, 2021). This is especially interesting for growers, considering several studies have observed significant increases in vegetable yields with VC application (with inorganic fertilizers) (Atiyeh et al., 2001; Arancon et al., 2003; Alam et al., 2007; Gutiérrez-Miceli et al., 2007; Ansari, 2008; Nongmaithem and Pal, 2011; Narayan et al., 2013; Yourtchi et al., 2013). The use of frass as a horticultural soil amendment can provide application for a growing (potentially local) waste stream in combination with inorganic fertilizers (Chavez et al., 2023). It can provide growers access to a beneficial amendment that is more affordable than VC, while also decreasing peat consumption (Chavez and Uchanski, 2021).
VC amendments have produced promising results for nutrient uptake in vegetable crops. Humic substances extracted from VC improved yields and increased protein and nitrates in field grown lettuce (Hernandez et al., 2015). In another study, VC was added to a greenhouse potting medium at increasing percentages to grow tomatoes. Resulting seedling tissues, with or without conventional fertilizers, had increases in N concentration when grown in high VC inclusion treatments (Atiyeh et al., 2001). Additionally, fertilizer combined with VC can increase the NPK content of potato tubers in the field (Yourtchi et al., 2013). In fact, the NPK uptake by field grown rosemary (Salvia Rosmarinus, Spenn., Lamiaceae) was highest in a conventional fertility treatment, but second highest in a slightly reduced fertilizer treatment supplemented with 0.4 kg per square meter of VC amendment. This improved nutrient utilization by various crops indicates greater uptake of nutrients from the soil media. Improved nutrient utilization and nutrient bioavailability indicate that an improvement in the quality of crops treated with frass applications could be anticipated (Singh and Wasnik, 2013).
Ultimately, all these formulations in supplying nutrients to plants may lead to decreases in leaching that occurs during agricultural production. Farmers applying nutrients through traditional means often provide higher dosages than is necessary for crop growth (Chapin et al., 2011). Nutrients that are not absorbed by the below ground biomass may infiltrate into the soil profile, or across landscapes, into surrounding environments. If these nutrients contaminate water sources, eutrophication can take place (Chapin et al., 2011). P run-off can be particularly problematic for surrounding environments because it increases with soil erosion, a common agricultural problem (Eghball and Gilley, 1999; Daverede et al., 2004). We conducted a greenhouse study from June to July of 2021. The study included two different leafy, cool season vegetable crop species, arugula and lettuce (Figure 1), in combination with various fertilizer treatments, including BSFL frass. Our major objective was to investigate the impact of BSFL frass on vegetable yield, biomass/ productivity, greenness, and nutrient concentrations and subsequent measurements in edible crop tissues. We compared the performance of crops grown in peat amended with insect frass and VC in a greenhouse setting. We predicted that BSFL frass applications will produce comparable or better arugula and lettuce fresh yield, dry weight, and greenness than the peat control and VC treatments. Additionally, we predicted plant tissue grown in BSFL frass will have greater concentrations of nutrients in harvested tissues than VC treatments and the control.
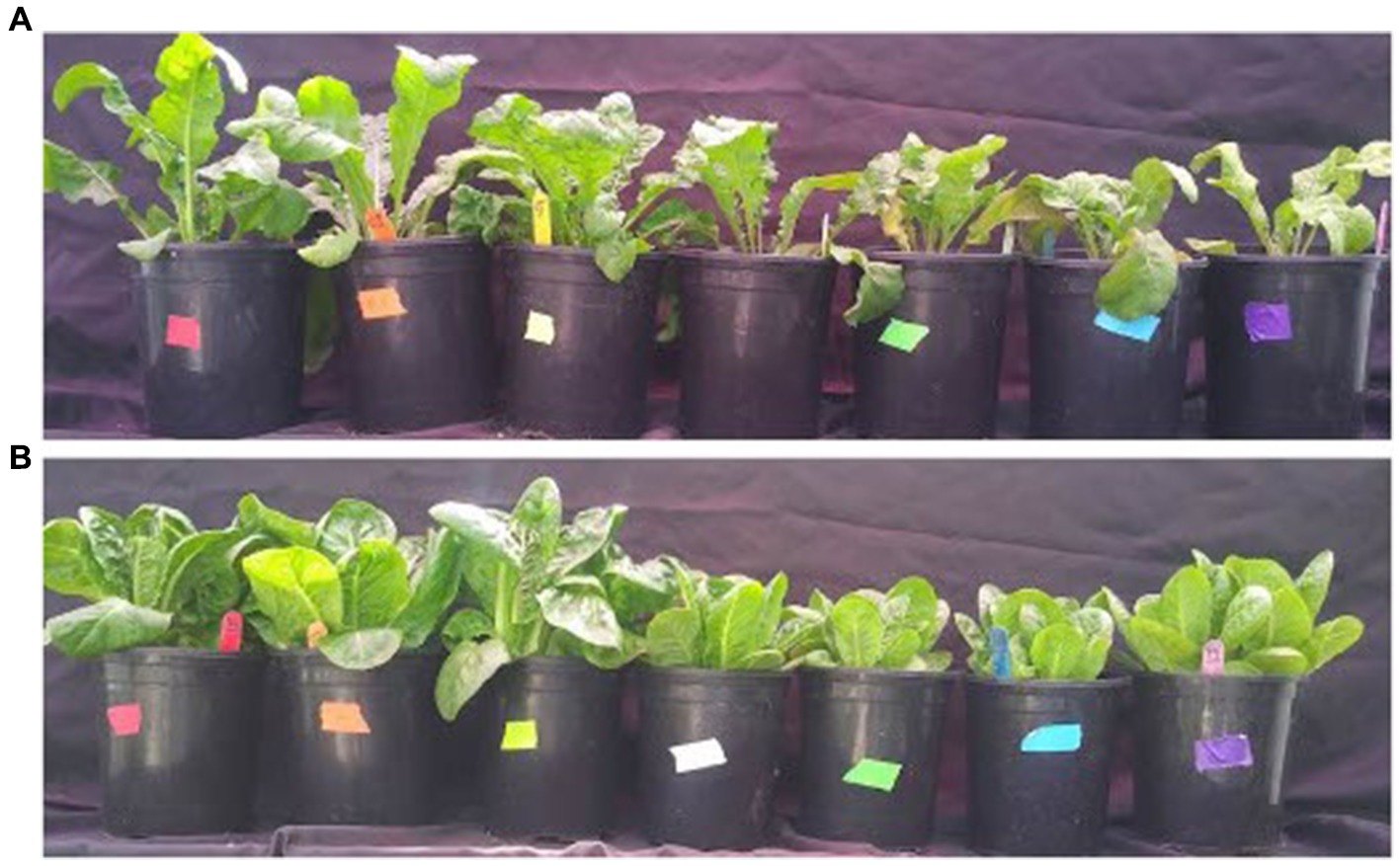
Figure 1. (A) Arugula and (B) lettuce grown in BSFL amended peat in a greenhouse study. Arugula and lettuce was grown with mixtures of commercial peat and black soldier fly larvae frass or vermicompost. Commercial peat (100%) served as the control.
2 Materials and methods
Preliminary studies were conducted in 2020 and produced the information needed to design the set of experiments that took place in 2021 (Table 1). This series of experiments was conducted in Fort Collins, CO, USA at the Colorado State University Horticulture Center greenhouses, elevation 1,525.22 m.1 They spanned the duration of June of 2021 to July 2021. The experiments (Table 1) were carried out as complete randomized designs using seven levels total (three treatments of each amendment and 100% commercial peat control), two crop species, and 10 replicates/level over the growing period. These amendments were: BSFL frass and VC (Figure 2). BSFL frass amendments were sourced from EVO Conversions System (College Station, TX, United States), the VC was from Rocky Mountain Soil Stewardship (Fort Collins, CO, United States), and the peat was a Berger B6 mix (Saint-Modeste, QC, CA, United States). Each amendment type was mixed with commercial peat at different percentages of amendment to peat (40%:60, 20%:80, 10%:90%), in addition to a 0%:100% peat control (Table 1). Inorganic fertilizer (24–8–16 NPK) Miracle Gro (Marysville, OH, United States) was applied at the label recommended rate (3.7 mL per 0.95 L water) for each crop, every other week. Productivity was assessed by weighing the vegetative biomass, or the leaves and petiole of the crop after harvest, 25 days after planting. Greenness was measured with a SPAD (soil plant analysis development) meter. Frass and plant tissue were sent to an external lab for compost and nutrient analysis.
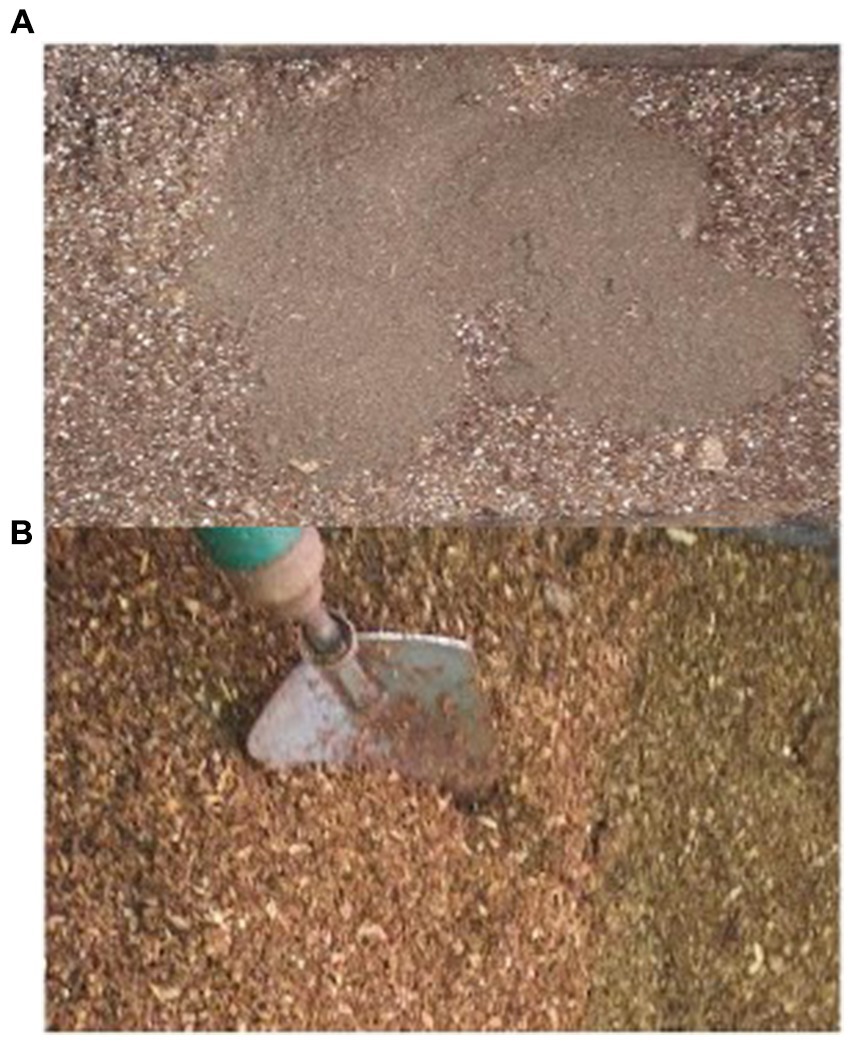
Figure 2. (A) VC and (B) BSFL frass amended into peat in a greenhouse pot study. Arugula and lettuce was grown with mixtures of commercial peat and black soldier fly larvae frass or vermicompost. Commercial peat (100%) served as the control.
Lettuce (cultivar: ‘Salvius MT0’) (Johnny’s Selected Seeds, Winslow, ME, United States), and arugula (cultivar: ‘Apollo’) (Seeds Savers Exchange, Decorah, IA, United States) were started on May 28, 2021as plugs in 288 cell trays with an organic germination mix: 3.79 L peat, 0.47 L VC, 29.57 mL blood meal, and 29.57 mL bone meal. They were grown on warming benches for two to 4 weeks in the greenhouse, and one to three plugs (depending on season and crop) were transplanted into 2-L pots on July 5, 2021. Harvest and data collection took place on July 30, 2021.
2.1 Yield
Crops were grown in pots placed on the greenhouse floor at 20–25°C. Each pot contained 1–3 plants. Four to five weeks after being transplanted, arugula and lettuce were harvested by cutting plants above the soil surface. The above ground fresh weight of each individual plant in each pot was weighed and prepared for drying (see below). Fresh weights were analyzed based on the average of the observational units, plants, within the experimental unit, which was the pot. Fresh weights of each plant/observational unit were averaged within replicate for the statistical analysis.
2.2 Primary productivity
The harvested plant material was placed in paper bags and left in the Horticulture Center drying oven at 70°C. Dried leaves were removed from the oven after 1 week and dry weights recorded. Similar to fresh weights, dry weights were taken on each individual plant in the pot. Weights of plants within the pot were then averaged as one replicate.
2.3 Greenness
Soil plant analysis development (SPAD) (Konica Minolta: Ramsey, NJ, United States) measurements were taken once before harvest. SPAD measures the difference of red and infrared light transmitted through the plant and is used as an indicator of greenness. The upper right side of the tallest leaf was measured. Each plant in the pot was measured separately, and the average for each pot was analyzed. SPAD is a relative metric with no units and is often used as an indicator of quality can because of its relationship correlation with N and chlorophyll content.
2.4 Nutrient concentrations
A plant tissue analysis was used to determine the nutrient concentrations in the plant tissue on a dry weight basis. After harvest, arugula and lettuce were dried in the drying oven at 70OCelsius. The dried plant samples were individually homogenized, ground, and sent to Brookside Labs (New Bremen, OH, United States) for plant tissue analysis on a dry weight basis with three replicates per treatment.
2.5 Compost analysis
There were four sources used in the potting mixes: distillery grain digested frass, brewery grain digested frass (EVO Conversions Systems, LLC), VC (Rocky Mountain Soil Stewardship, Fort Collins, CO, United States), and peat (Berger B6, Saint-Modeste, QC, Canada). Approximately 100 g of each unaltered material was sent to Brookside Labs (New Bremen, OH, United States) for a standard compost analysis (Table 2).
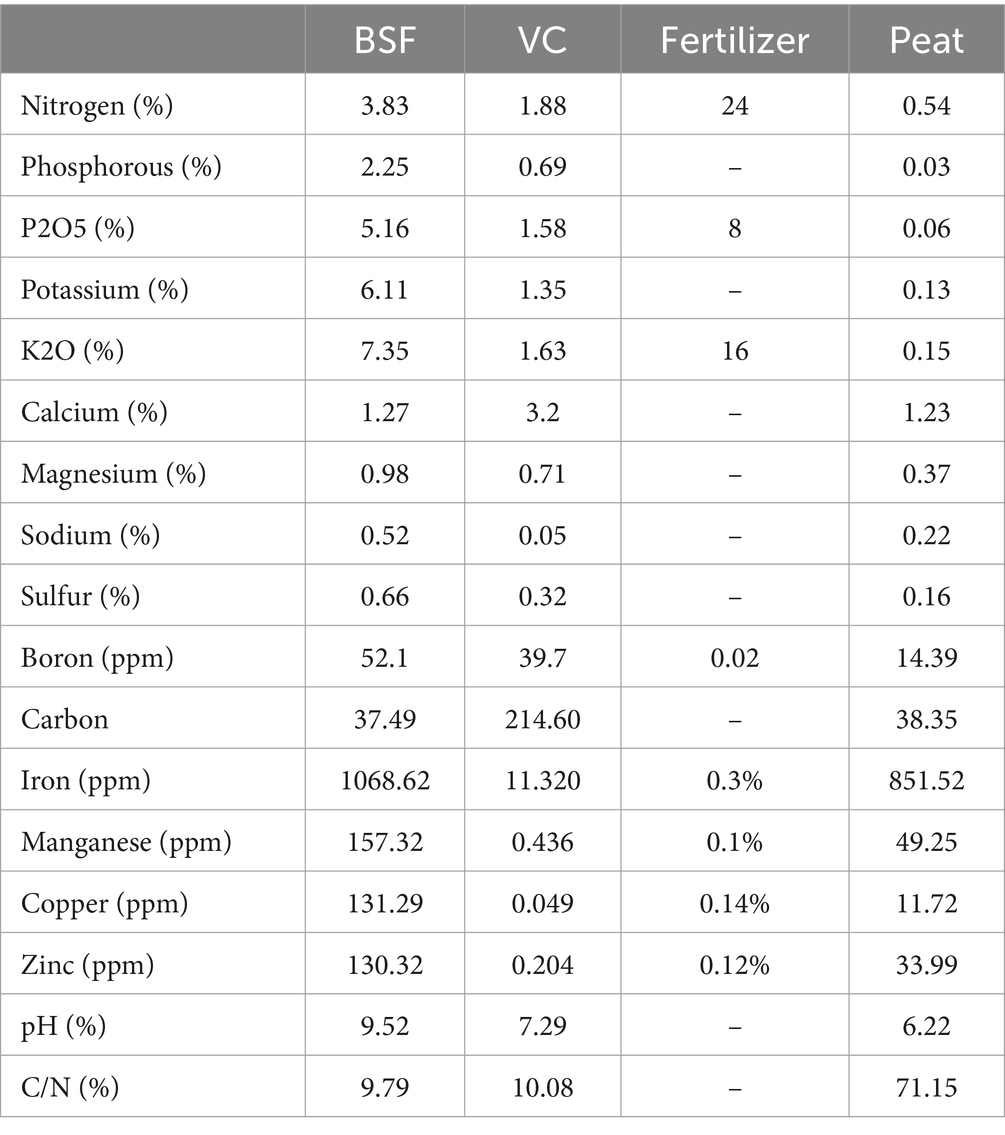
Table 2. Analysis of amendments used to create treatments 2021–2022: black soldier fly larvae (BSFL) frass, and vermicompost (VC) were the treatments, and peat was the control.
2.6 Statistical analysis
Arugula and lettuce plants, the observational units, were averaged within the pot (i.e., experimental replicates). Statistical analysis was conducted using JMP®, Pro 16 (SAS Institute Inc., Cary, NC, United States, 2022). Percent data was converted to proportions and transformed with the logit function before analysis. The Anderson-Darling test for normality and the Levene’s test for equal variance were conducted to determine if the assumptions for an ANOVA were met. When they were not met, non-parametric Kruskal Wallis rank sums tests were conducted in addition to the parametric tests. A threshold of p < 0.05 was considered as significant. Tukey HD and Dunn method for Joint Ranks pair wise comparisons were conducted to assess and test for differences between levels.
3 Results
3.1 Yield
Arugula (F = 16.65; df = 6; p < 0.0001) had a clear trend that the BSFL treatments produced significantly higher arugula (Figure 3) fresh yields (33.8–28.4 g) than the control (13.8 g) and the VC treatments (10.5–12.8 g). Lettuce (Figure 3) fresh yield (F = 56.16; df = 6; p < 0.0001) produced similar results to arugula in the same season. However, unlike most of the results, the highest concentration of frass, BSF 40%, produced the highest yield (72.6 g).
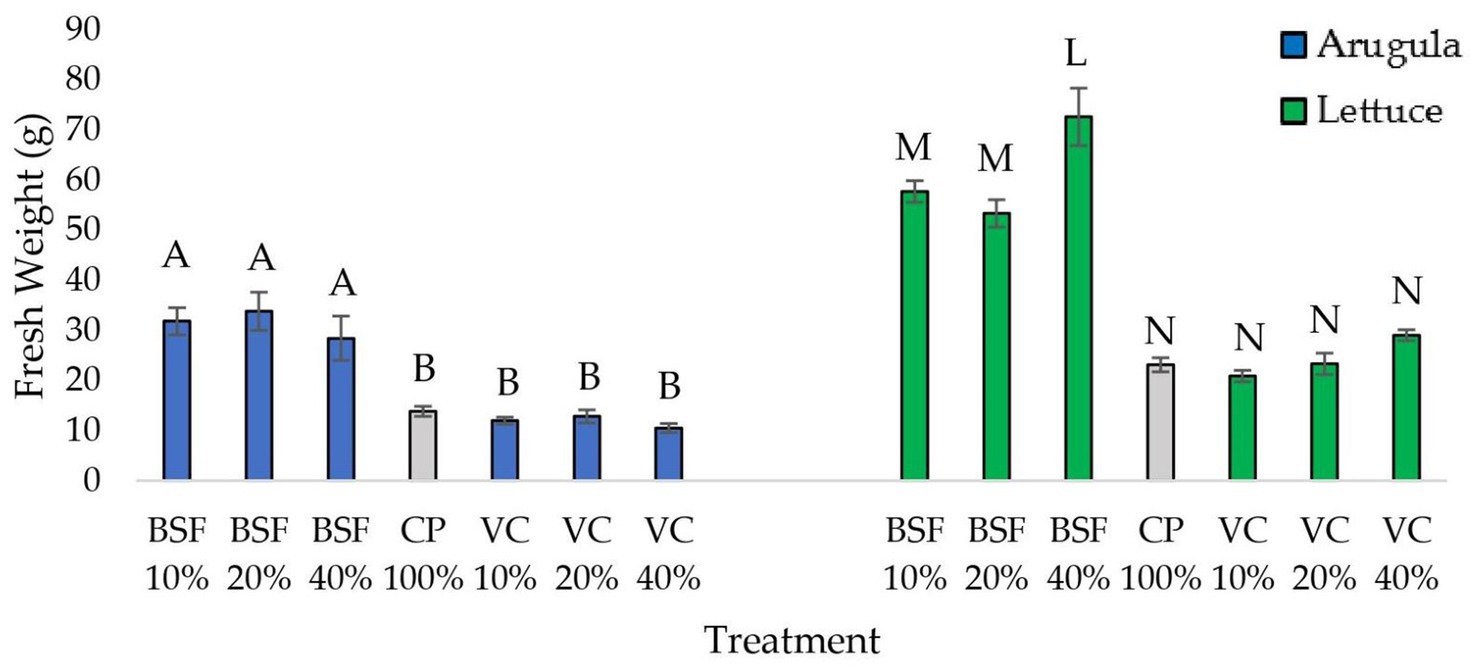
Figure 3. Fresh weight (g) ± SEM of arugula and lettuce grown in BSFL amended peat in a greenhouse. Arugula (blue columns, A–E) and lettuce (green columns, L–O) was grown with mixtures of commercial peat and black soldier fly larvae frass or vermicompost. Commercial peat (100%) served as the control pot (CP: grey column). Columns with different letters indicate differences determined by one-way ANOVAs and Tukey pairwise comparisons (p < 0.05).
3.2 Productivity
Dry weight (Figure 4) of arugula (F = 5.72; df = 6; p < 0.0001) showed similar results as fresh weight. BSF 20% (3.35 g) was significantly higher than a few of the VC treatments, VC 10% (1.7 g) and 40% (1.2 g), and statistically comparable to the other treatments and the control. Lettuce dry weight results (F = 56.16; df = 6; p < 0.0001) were also similar to the fresh weight. BSF 40% (9.97 g) was statistically the highest treatment, following BSF 20% (6.5 g) and BSF 10% (6.92 g), while the control (2.52 g) and VC treatments (1.82–2.7 g) were the lowest.
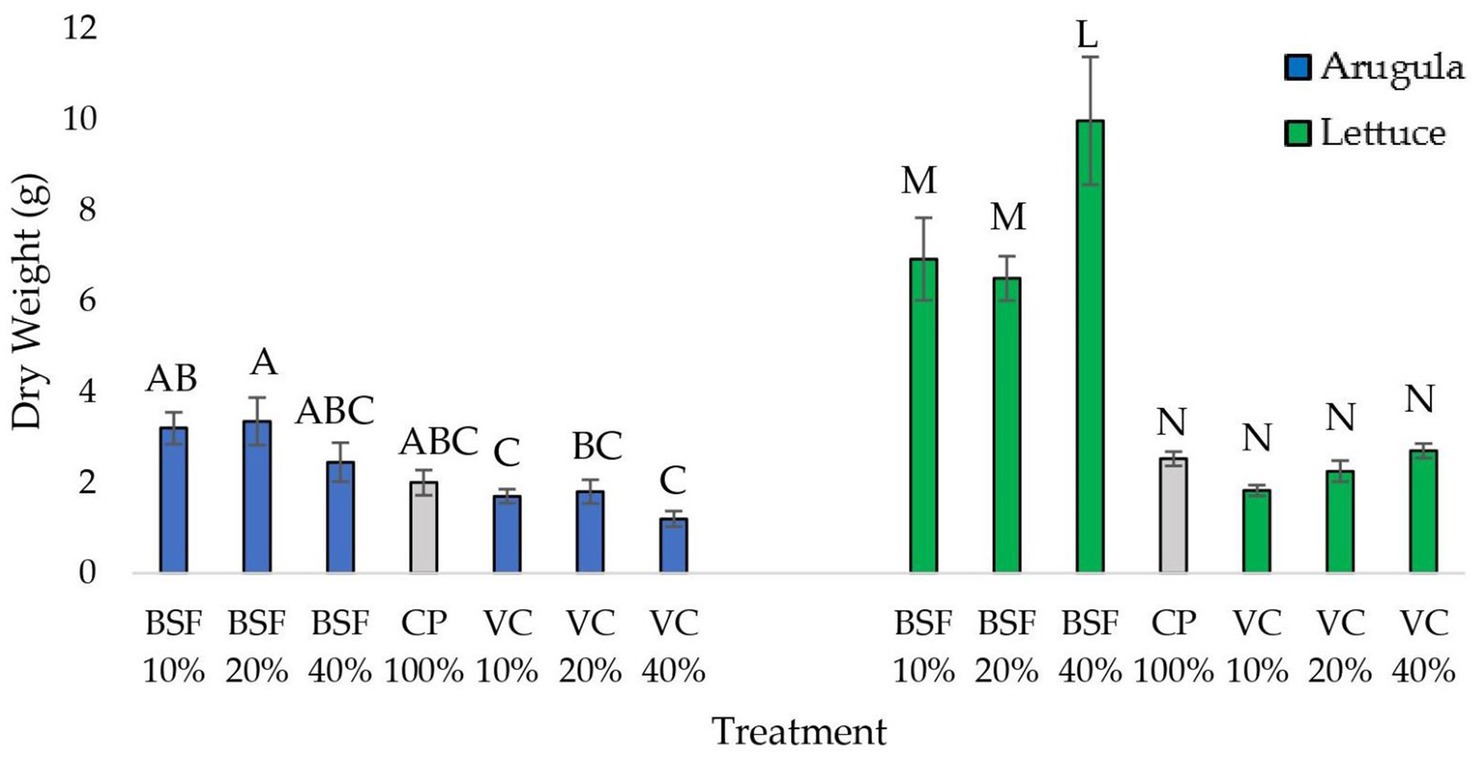
Figure 4. Dry weight (g) ± SEM of arugula and lettuce grown in BSFL amended peat in a greenhouse. Arugula (blue columns, A–E) and lettuce (green columns, L–O) was grown with mixtures of commercial peat and black soldier fly larvae frass or vermicompost. Commercial peat (100%) served as the control pot (CP: grey column). Columns with different letters indicate differences determined by one-way ANOVAs and Tukey pairwise comparisons (p < 0.05).
3.3 Greenness
Arugula greenness (F = 3.21; df = 6; p = 0.0082) showed some variability, but statistically most of these treatments were similar (Figure 5). BSF 10% (44.79 g) was greener (20% (36.65 g) and 40% (37.17 g)) than the higher concentrations of VC. Lettuce (Figure 5) produced similar SPAD results as arugula (F = 3.37; df = 6; p = 0.006). There were some significant differences in greenness between BSF treatments (44.32–46.23) and VC treatments (35.78–37.78). Overall, the BSF treatments were the greenest while BSF 10% (46.23) was statistically the greenest.
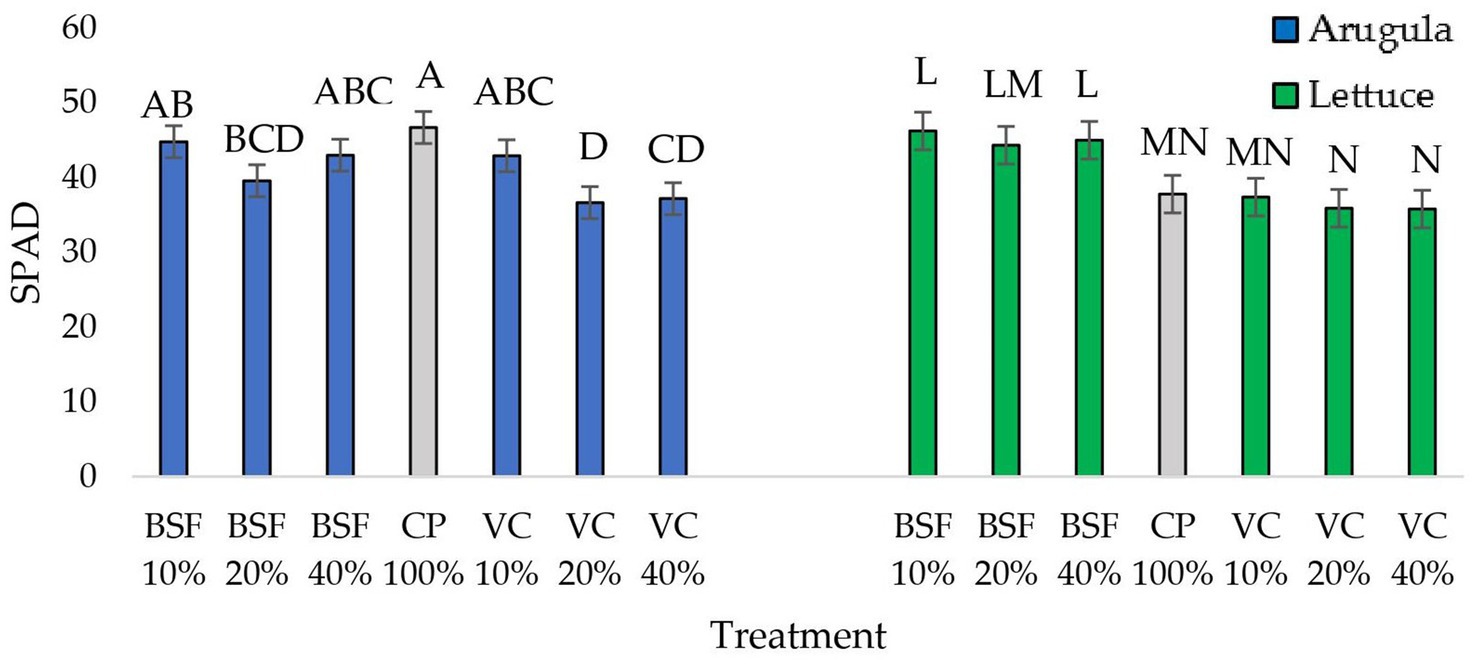
Figure 5. Greenness (SPAD) ± SEM of arugula and lettuce grown in BSFL amended peat in a greenhouse. Arugula (blue columns, A–E) and lettuce (green columns, L–O) was grown with mixtures of commercial peat and black soldier fly larvae frass or vermicompost. Commercial peat (100%) served as the control pot (CP: grey column). Columns with different letters indicate differences determined by one-way ANOVAs and Tukey pairwise comparisons (p < 0.05).
3.4 Nutrient concentration in plant tissue on a dry weight basis
3.4.1 Primary macronutrients: N, P, and K
N concentrations, Figure 6A, of both arugula (7%) (F = 109.41; df = 6; p < 0 0.0001), and lettuce (5%) (F = 54.66; d = 6; p < 0.0001) tissues were significantly higher in the BSF 40% treatment. The other BSF treatments (20 and 10%) were also significantly higher in N than the control and VC treatments. In both crops, the VC treatments were comparable to the control. This aligns with the results from the compost analysis. Distillery grains had high levels of N, VC had intermediate levels, while peat was very low in N.
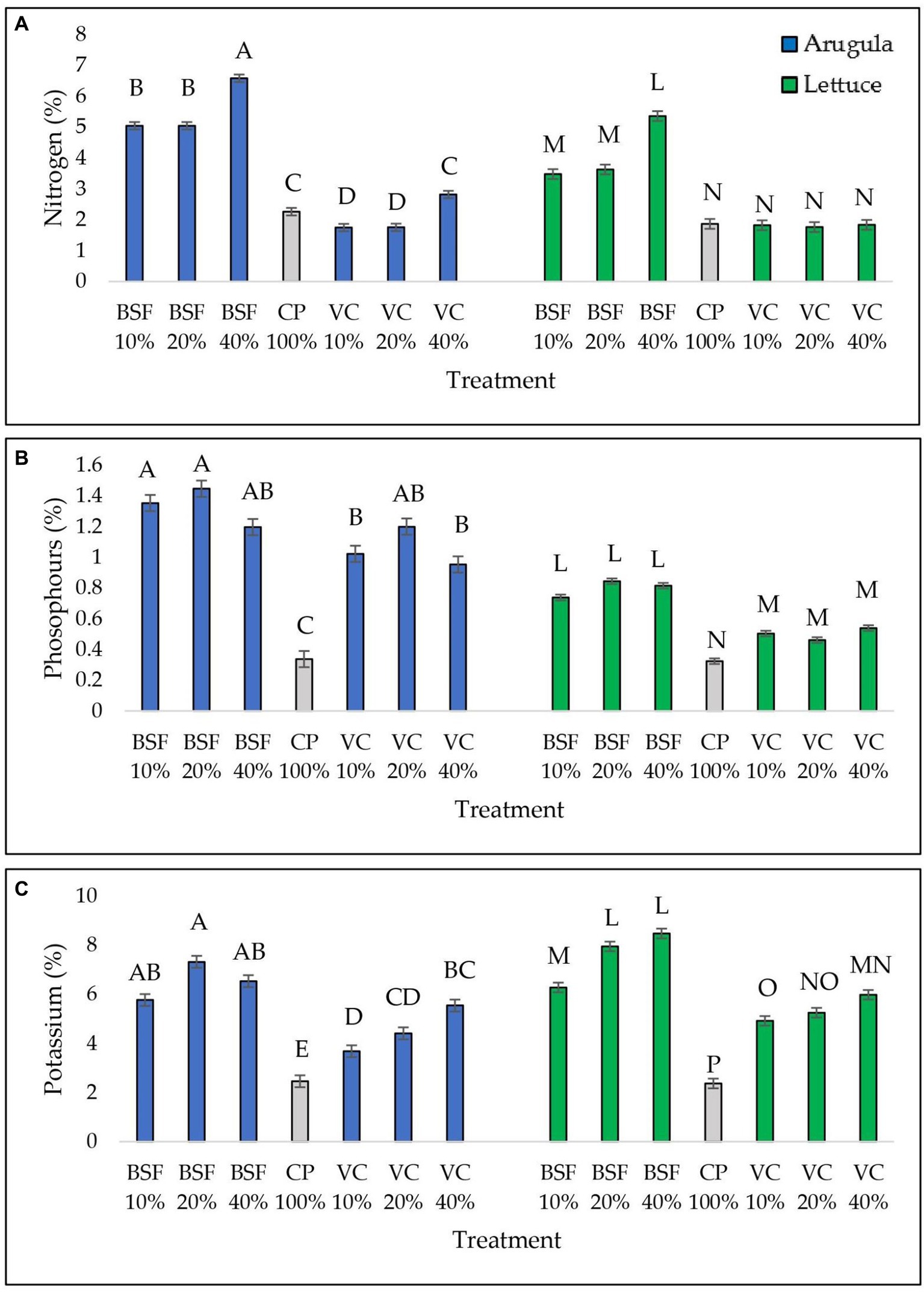
Figure 6. Concentrations of primary macronutrients (A) N (B) P, and (C) K, ± SEM of BSFL amended lettuce grown in a greenhouse. Arugula (blue columns, A–E) and lettuce (green columns, L–O) was grown with mixtures of commercial peat and black soldier fly larvae frass or vermicompost. Commercial peat (100%) served as the control pot (CP: grey column). Columns with different letters indicate differences determined by one-way ANOVAs and Tukey pairwise comparisons (p < 0.05).
P concentrations of arugula (Figure 6B) were comparable across all BSFL and VC treatments (F = 105.07; df = 6; p < 0.0001), though all treatments were significantly higher than the control (0.34%). Lettuce P concentrations were significantly higher in the BSFL treatments (0.74–0.84%) (Figure 6B), and the VC treatments (0.46–0.54%) were significantly higher than the control (0.32%) (F = 135.44; df = 6; p < 0.0001). K concentrations in arugula (F = 54.06; df = 6; p < 0.0001) and lettuce (F = 174.62; df = 6; p < 0.0001) were very similar (Figure 6C). BSF 20% produced the highest concentrations in arugula (7%) and BSF 40% was highest in lettuce (8%), while the control peat produced the lowest K concentrations in both crops. Additionally, the BSFL treatments were significantly higher than most of the VC treatments as well.
3.4.2 Secondary macronutrients: Ca, Mg, and S
In arugula plant tissues (Figure 7A) there were not many differences in percent Ca (F = 6.025; df = 6; p = 0.0027). The ANOVA results indicated significant differences, but the data did not pass the test for normality. The peat control (1%) had the lowest concentrations of Ca while BSF 10% (2%) had the highest. The BSFL and VC treatments were all statistically comparable. A non-parametric test was conducted to further evaluate the results. The χ2 (12.26) analysis indicated no significant differences between treatments (p = 0.056). The opposite effect appears in lettuce, where the control produced the greatest percentages of Ca (1%) (Figure 7A). This was statistically comparable to all the VC treatments, but higher than all the BSFL treatments (F = 11.084; df = 6; p = 0.0001). This information is slightly confounding to what we understand about the amendments, considering peat has the lowest amount of Ca and VC has the highest amount (Table 2).
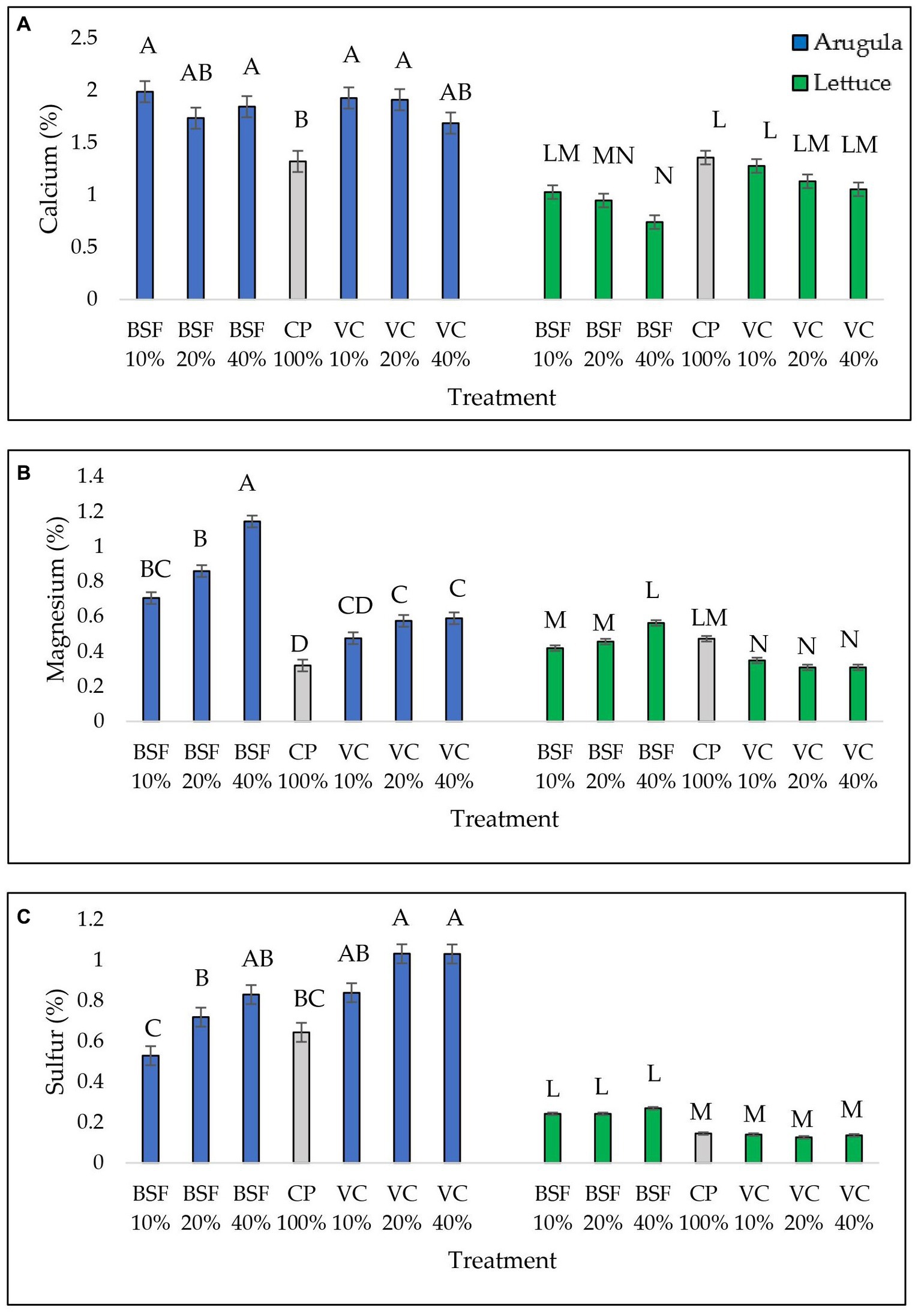
Figure 7. Concentrations of secondary macronutrients (A) Ca (B) Mg, and (C) S, ± SEM of BSFL amended lettuce grown in a greenhouse. Arugula (blue columns, A–E) and lettuce (green columns, L–O) was grown with mixtures of commercial peat and black soldier fly larvae frass or vermicompost. Commercial peat (100%) served as the control pot (CP: grey column). Columns with different letters indicate differences determined by one-way ANOVAs and Tukey pairwise comparisons (p < 0.05).
Arugula (F = 87.27; df = 6; p < 0.0001) and lettuce (F = 37.34; df = 6; p < 0.0001) amended with BSFL frass contains significantly higher percentages of Mg compared to the control and VC treatments (Figure 7B). For arugula (1.1%) and lettuce (0.5%) crops, BSF 40% was significantly greater than all the other treatments and control. In arugula leaves Mg is lowest in the control (0.3%), while in lettuce the lowest is VC 20% (0.31%) and VC 40% (0.31%). This corresponds to higher percentages of Mg in the frass (Table 2).
S concentration in arugula leaves (F = 20.53; df = 6; p < 0.0001) were greatest in the VC treatments, VC 20% (1%) and VC 40% (1%) and was lowest in the BSF 10% treatment (0.5%) (Figure 7C). Lettuce tissue analysis produced different results (F = 121.60; df = 6; p < 0.0001). The BSFL frass treatments (0.24–0.27%) were all significantly higher in percent S than the control (0.14%) and VC treatments (0.13–0.14%). The VC treatments were all comparable to the control (Figure 7C).
3.4.3 Micronutrients: B, Fe, Mg, Cu, and Zn
Arugula plant tissues produced the greatest amounts of B when grown in BSFL frass (5–57 ppm) (Figure 8A). These treatments were significantly higher than the control (29.67 ppm) and most of the VC treatments (34–45 ppm) (F = 22.42; df = 6; p < 0.0001). The control peat and VC 10% produced the lowest concentrations of B. B concentrations in lettuce tissue produced similar results (Figure 8A). The BSFL treatments (3,033 ppm) are all significantly higher than the control (21.67 ppm) and VC treatments (20–23 ppm) (F = 27.43; df = 6; p < 0.0001). This corresponds with the compost analysis that revealed high amounts of B in BSFL frass.
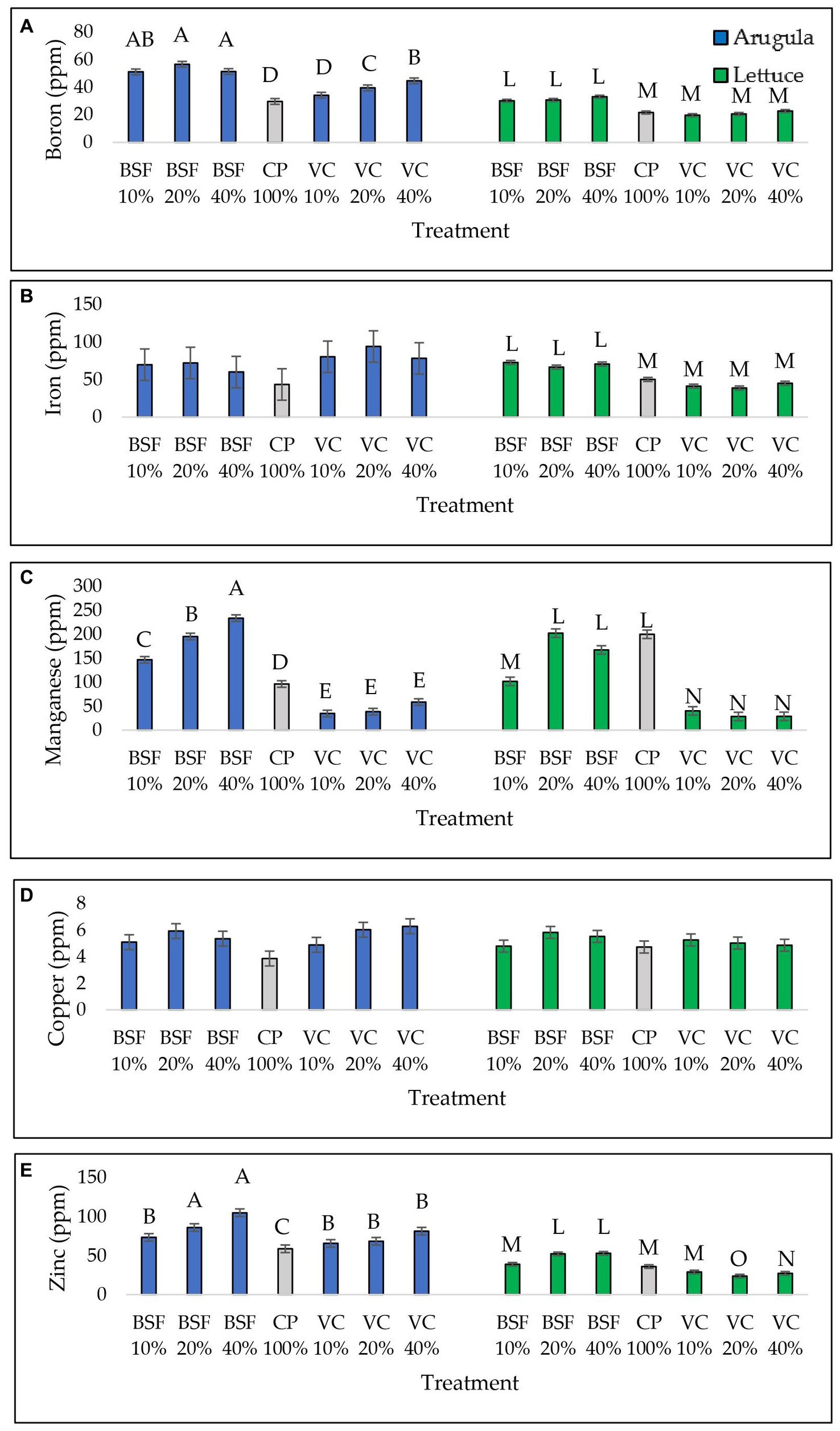
Figure 8. Concentrations of micronutrients (A) B (B) Fe (C) Mn (D) Cu, and (E) Zn, ± SEM of BSFL amended lettuce grown in a greenhouse. Arugula (blue columns, A–E) and lettuce (green columns, L–O) was grown with mixtures of commercial peat and black soldier fly larvae frass or vermicompost. Commercial peat (100%) served as the control pot (CP: grey column). Columns with different letters indicate differences determined by one-way ANOVAs and Tukey pairwise comparisons (p < 0.05).
There were no treatment differences in arugula Fe tissue concentrations (Figure 8B). Though not statistically significant, VC treatments produced the greatest concentrations of Fe in arugula tissues (F = 0.59; df = 6; p = 0.73). However, in lettuce tissues (F = 29.52; df = 6; p < 0.0001), the BSFL frass treatments (73–67 ppm) produced significantly higher concentrations of Fe compared to the control (50 ppm) and VC treatments (38.73–45.03) (Figure 8B). This both confirms and contradicts the compost analysis. BSFL frass had much higher levels of Fe than the other materials.
Mn concentrations in arugula (Figure 8C) was highest in the BSFL treatments and lowest in the VC treatments (F = 131.71; df = 6; p < 0 0.0001). BSF 40% (234 ppm) was significantly higher than the control (96.27 ppm) and all other treatments. Additionally, Mn concentrations in the control peat were significantly higher than the VC treatments in arugula tissue. In lettuce tissues (Figure 8C), Mn concentrations were significantly higher in the control (200 ppm) and the BSFL treatments, BSF 40% (167 ppm) and BSF 20% (202 ppm), compared to the VC treatments, which produced the lowest Mn concentrations (F = 81.70; df = 6; p < 0.0001). This corroborates the compost analysis that revealed higher concentrations of Mn in the BSFL frass (Table 2). However, there were low concentrations of Mn that were found in the peat.
Cu concentrations in arugula (F = 2.22; df = 6; p = 0.10) and lettuce (F = 0.83; df = 6; p = 0.56) tissues did not produce any significant differences based on treatment, Figure 8D. This cannot be explained by the compost analysis (Table 2) alone because the Cu levels are variable depending on the material.
Arugula tissues were highest in Zn (Figure 8E) when grown in the BSF 40% treatments (105 ppm) and lowest when grown in the control (59) (F = 10.07; df = 6; p = 0.0002). BSF 40% was significantly higher than all the treatments and control, except BSF 20% (86 ppm). In lettuce (Figure 8E), BSF 40% (53 ppm) and BSF 20% (52%) also produced the significantly higher concentrations of Zn (F = 26.75; df = 6; p < 0.0001). However, VC 40% (0.5%) and VC 20% (23%) contain the least amount of Zn. This corresponds with the compost analysis (Table 2) that revealed high amounts of percent Zn in the distillery grain frass.
3.4.4 Other elements: Al and Na
Al concentrations in both arugula (F = 1.25; df = 6; p = 0.34) and lettuce (F = 1.67; df = 6; p = 0.20) did not differ statistically based on treatment, Figure 9A. In lettuce, Al was much higher in the VC treatments, though not statistically significant. Al was not measured in the compost analysis and cannot be used to discuss these results.
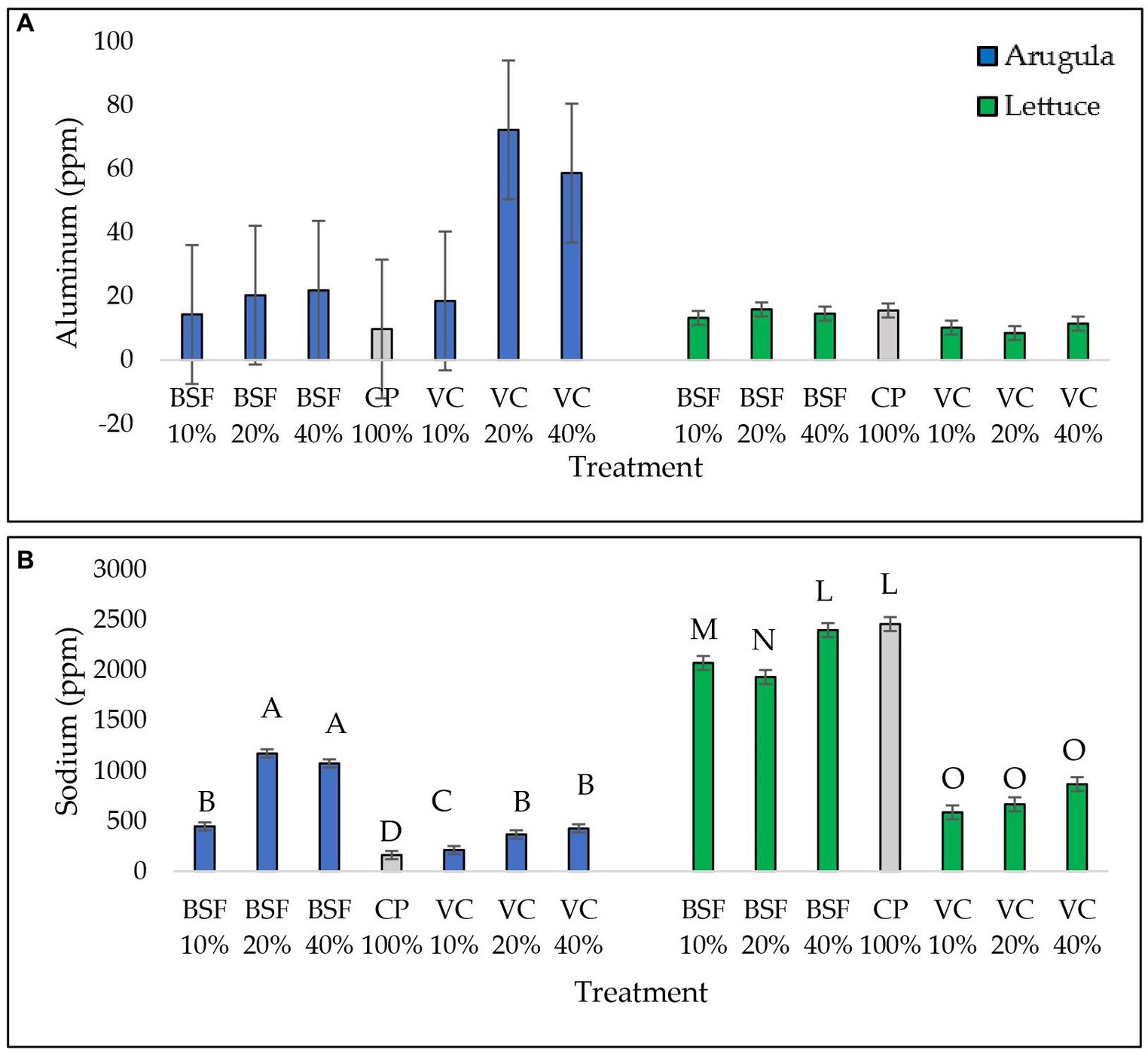
Figure 9. Concentrations of (A) aluminum and (B) sodium ± SEM in BSFL amended lettuce grown in a greenhouse. Arugula (blue columns, A-E) and lettuce (green columns, L-O) was grown with mixtures of commercial peat and black soldier fly larvae frass or vermicompost. Commercial peat (100%) served as the control pot (CP: grey column). Columns with different letters indicate differences determined by one-way ANOVAs and Tukey pairwise comparisons (p < 0.05).
Na concentrations were significantly greater in BSFL treatments (446–1,173 ppm) in arugula (Figure 9B). The control produced the lowest levels of Na (162 ppm) (F = 97.35; df = 6; p < 0.0001/F = 97.35). In lettuce tissues (F = 143.31; df = 6; p < 0.0001), Na was highest in the control (2,460 ppm) and lowest in the VC treatments (587–876 ppm) (Figure 9B) Distillery grains did have the highest level of Na, and VC had the lowest (Table 2), which validates the findings in the plant tissues.
4 Discussion
Arugula and lettuce experienced several of the same trends observed in the parameters measured. Higher fresh arugula and lettuce yields were produced in all the BSFL treatments compared to the VC treatments and control. A previous study observed the impacts of BSFL frass on tomato production utilizing the same methodology and treatments (Chavez et al., 2023). Total yields, greenness, dissolved solids, and vegetative biomass produced comparable results to the 100% peat control and the VC treatments, also indicating that BSFL frass is a neutral addition to a greenhouse potting media.
BSFL frass treatments produced higher SPAD and dry weight measurements in lettuce compared to arugula. Arugula treated with the BSFL frass did not produce profound differences in greenness, as the control produced the greenest plants. Lettuce greenness tended to be improved by low concentrations of BSFL frass, 10–20%. SPAD is a relative metric to indicate differences in greenness, which can often be correlated with N and chlorophyl concentrations. Studies with romaine lettuce grown in high tunnels revealed that an increase in SPAD measurements also resulted in increases in leaf N and chlorophyl concentrations (Mendoza-Tafolla et al., 2019). Dry weight is often a positive indicator of above ground net primary productivity in leafy plants (Smart et al., 2017). Increased dry weights observed in lettuce treated with BSFL frass could indicate increased photosynthetic activity. These results in combination provide a strong inference to suggest that BSFL frass has significant impacts in overall lettuce production.
Lettuce is a conventionally successful crop because of its ubiquitous acclimation to different soil conditions (Swiader and Ware, 2002). Arugula is known to be successful in arid environments due to its extensive root system (Bell and Wagstaff, 2019). Lettuce is not only a more domesticated crop but is also more commonly used in scientific research (Koukounaras et al., 2020), which highlights the importance of including both crops in this study. Overall lettuce seems to be more responsive to frass treatments compared to arugula. Water and nutrients in the soil are normally not readily available to plants growing in natural environments. Crops can forage for nearby resources by contributing energy to below ground productivity. Plasticity of root production can increase overall plant efficiency when resources are not immediately available (Huang and Eissenstat, 2000). Crops specialized to natural, arid environments are possibly more satiated in controlled environment systems. It is likely that pot studies may have limited the adaptive qualities of arugula, by limiting root growth, and their tendency to search for water and nutrients. Therefore, incorporating frass into future field studies will be essential in understanding individual crop performance.
Though we saw better yields utilizing BSFL frass, it is possible that other quality parameters, like post-harvest shelf life of leafy greens are also impacted by treatment. Arugula harvested in field trials during July have resulted in lower post-harvest quality compared to those harvested during cooler months (Koukounaras et al., 2020). Future research would benefit from replicating over several years and in different seasons. Restricting our research to an even more controlled environment, like growth chambers, could eliminate climate effects that may have impacted some of the results in this study.
Nutrient concentrations were often higher in the BSFL frass treatments for both crops. Lettuce SPAD measurements correspond with the results of the compost and plant tissue analysis, which also supports the significantly higher concentrations of macronutrients N, P, K, and Mg in both crops. Al concentrations observed in arugula tissues were significantly higher in the VC treatments. At low levels of pH (<5.5), high levels of Al can be toxic and damage root systems (Smith and Smith, 2015). That may explain the lower yields of arugula in the VC treatments. In the distillery grain treatments, there was a decrease in Ca concentrations of lettuce, compared to the peat control, despite the high Ca concentrations observed in distillery grain frass. The opposite trend was seen in the arugula, so response could vary by crop.
The ion exchange capacity of the soil, or the number of charged sites present on soil particles, is determined by soil texture and acidity. This controls how well cations (K+, Ca2+ and Mg2+) and anions (NO3−, H2PO4−, and SO42−) can stay in the soil solution, remaining available for plant uptake (Smith and Smith, 2015). The lower pH of the peat (6.22) could explain the lower nutrient concentrations often observed in the control plants. As soil acidity increases and pH decreases, it increases binding affinity for Al and decreases binding to other important cations like Ca and Na (Smith and Smith, 2015). This could explain the observations in Na concentration of arugula is significantly lower than all the other treatments. The same trend is not observed in lettuce, so the response may vary per species.
Though arugula and lettuce seem to retain most macronutrients in a similar manner; there was considerable variation in their response to micronutrients as well. Na levels in arugula were highest in the BSFL frass treatments, while Na concentrations in lettuce were highest in the control. Additionally, arugula did not seem to respond to Fe, while lettuce grown in the BSFL treatments produced significantly higher tissue Fe levels than the control and other treatments. Several biogeochemical processes are linked to soil pH (Neina, 2019). It is possible that the higher pH seen in the BSFL frass (9.52) amendments (Table 2) could have produced a growing media that was too basic for plant growth in large quantities, such as the 40% treatments. As alkalinity increases, so does nutrient leaching (Neina, 2019). Peats neutral pH (6.22) is a large reason for its adoption and continued use in controlled environment agriculture. It is likely that fertilizer applications will need to be more closely considered in future incorporation of frass into media amendments.
Overall, the addition of BSFL frass digested distillery grains can not only improve yield, but can also improve vegetable quality, for most macronutrients of concern. For the other macronutrients, additional steps could be considered to improve the frass as a growth amendment and fertility product. The addition of nutrient containing amendments, such as frass, could increase nutrient leaching from the growth media. A study by Bohara et al. (2019), observed increased nutrient concentrations of leachate after the application of poultry litter. Soils amended with pine biochar and poultry litter + pine biochar produced a significant decrease in nutrient leaching. Incorporating biochar into frass could improve the overall value as a peat replacement and fertilizer and provides another consideration for future studies.
Past studies have indicated that VC can alter the soil microbiome and increases the plant growth regulators present (Ravindran et al., 2016). Plant growth regulators are essential in signaling development during growth. Given the similar process of bioconversion, it is possible that an increase in PGR and an altered microbiome may be responsible for some of the results found in frass experiments, although this was not measured in this study. More experiments are warranted as this could explain why increases in yield were observed, but nutrient concentrations were not.
Fernández-Romero et al. (2016) observed the impacts of BSFL frass on lettuce production and found increases in soil organic matter and residual nutrient content. This increase in soil organic matter could indicate potential reductions in nutrient runoff, like those seen in VC (Elliott et al., 2007). Increases in the enzymatic activity of dehydrogenase and β-glucosidase have also been observed in soils treated with black soldier fly larvae frass (Esteves et al., 2022), which could lead to future improvements in soil health and function. By reducing peat consumption, redirecting waste streams, and improving local landscapes, the effects of frass adoption could have longer term impacts than just the potential improvements in crop yield. The general research on insect frass is sparse and variable. This research aimed to assess several potential crops, starting feeds, and quality and quantity metrics that can be used in the application of insect frass in horticultural production. Due to time and resource constraints, we were unable to replicate the same studies in the different years or seasons. However, it is still a worthy field of research in order explore novel improvements of the food system. Documented improvements in yield, vegetable quality, and soil health could be an incentive for growers to mitigate some of the harmful consequences of horticultural production, such as peat extraction.
5 Conclusion
Overall, we observed a range of impacts that insect frass may have on leafy green production. The nutrient profile of the compost is a large indicator for plant outcomes. Arugula and lettuce nutritional quality is improved with the supplement of BSFL frass. Additionally, lettuce, the more domesticated crop, responded more positively to treatments, in both productivity and greenness. However, arugula micronutrients seemed to benefit more from BSFL incorporation. Leafy greens are important staple crops, produced worldwide; Efforts to increase sustainability of these crops can only improve the accessibility of fresh, nutrient dense food. Mitigating practices to improve long term environmental outcomes of controlled environment agriculture can create long term production solutions. Recommendation to growers interested in adopting insect frass in their potting mixes is to first consider a 10% by volume peat replacement. Insect producers have made strides to reduce waste streams by incorporating food waste into their practices. Integrating the left-over substrates (frass) of insect production, in small amounts (10–20%), to reduce peat consumption further contributes to an industrial effort to improve circular economies (Chavez, 2021).
Data availability statement
The raw data supporting the conclusions of this article will be made available by the authors, without undue reservation.
Author contributions
MC: Conceptualization, Data curation, Formal analysis, Funding acquisition, Investigation, Methodology, Resources, Software, Visualization, Writing – original draft, Writing – review & editing. MU: Conceptualization, Project administration, Resources, Writing – original draft, Writing – review & editing. JT: Conceptualization, Formal analysis, Investigation, Methodology, Resources, Writing – original draft, Writing – review & editing.
Funding
The author(s) declare that financial support was received for the research, authorship, and/or publication of this article. This research was supported by the National Science Foundation Graduate Research Fellowship Program. Additional funds came through Colorado State University: the Graduate Degree Pro-gram in Ecology small grant (funded by Research Mentoring to Advance Inclusivity in STEM) and the Organic Agriculture Program Scholarship (funded by Aurora Organic Dairy). Material re-sources were provided by the Specialty Crop Program at CSU.
Acknowledgments
BSFL frass was donated by EVO Conversion Systems. Statistical analysis was consulted by the CSU Graybill statistical laboratory.
Conflict of interest
JT is a part owner of EVO Conversion Systems, LLC.
The remaining authors declare that the research was conducted in the absence of any commercial or financial relationships that could be construed as a potential conflict of interest.
Publisher’s note
All claims expressed in this article are solely those of the authors and do not necessarily represent those of their affiliated organizations, or those of the publisher, the editors and the reviewers. Any product that may be evaluated in this article, or claim that may be made by its manufacturer, is not guaranteed or endorsed by the publisher.
Footnotes
References
Alam, M. N., Jahan, M. S., Ali, M. K., Ashraf, M. A., and Islam, M. K. (2007). Effect of vermicompost and chemical fertilizers on growth, yield and yield components of potato in barind soils of Bangladesh. J. Appl. Sci. Res. 3, 1879–1888.
Ansari, A. A. (2008). Effect of vermicompost and vermiwash on the productivity of spinach (Spinacia oleracea), onion (Allium cepa) and potato (Solanum tuberosum). World J. Agric. Sci. 4, 554–557.
Arancon, N., Edwards, C., Bierman, P., Metzger, J., Lee, S., and Welch, C. (2003). Effects of vermicomposts on growth and marketable fruits of field-grown tomatoes, peppers and strawberriesThe 7th international symposium on earthworm ecology · Cardiff · Wales · 2002. Pedobiologia 47, 731–735. doi: 10.1016/S0031-4056(04)70260-7
Atiyeh, R. M., Edwards, C. A., Subler, S., and Metzger, J. D. (2001). Pig manure vermicompost as a component of a horticultural bedding plant medium: effects on physicochemical properties and plant growth. Bioresour. Technol. 78, 11–20. doi: 10.1016/S0960-8524(00)00172-3
Bell, L., and Wagstaff, C. (2019). Rocket science: a review of phytochemical & health-related research in Eruca & Diplotaxis species. Food Chem. X 1:100002. doi: 10.1016/j.fochx.2018.100002
Bloom, A. L. (1964). Peat accumulation and compaction in a Connecticut coastal marsh. J. Sediment. Res. 34, 599–603. doi: 10.1306/74D710F5-2B21-11D7-8648000102C1865D
Bohara, H., Dodla, S., Wang, J. J., Darapuneni, M., Acharya, B. S., Magdi, S., et al. (2019). Influence of poultry litter and biochar on soil water dynamics and nutrient leaching from a very fine sandy loam soil. Soil Tillage Res. 189, 44–51. doi: 10.1016/j.still.2019.01.001
Cameron, C. C., Esterle, J. S., and Palmer, C. A. (1989). The geology, botany and chemistry of selected peat-forming environments from temperate and tropical latitudes. Int. J. Coal Geol. 12, 105–156. doi: 10.1016/0166-5162(89)90049-9
Chapin, F. S., Matson, P. A., and Vitousek, P. (2011). Principles of terrestrial ecosystem ecology. New York, NY, USA: Springer Science & Business Media.
Chavez, M. (2021). The sustainability of industrial insect mass rearing for food and feed production: zero waste goals through by-product utilization. Curr. Opin. Insect Sci. 48, 44–49. doi: 10.1016/j.cois.2021.09.003
Chavez, M., and Uchanski, M. (2021). Insect left-over substrate as plant fertiliser. J. Insects Food Feed 7, 683–694. doi: 10.3920/JIFF2020.0063
Chavez, M. Y., Uchanski, M., and Tomberlin, J. K. (2023). Impacts of black soldier fly, (Diptera: Stratiomyidae) larval frass on tomato production. J. Econ. Entomol. 116, 1490–1495. doi: 10.1093/jee/toad150
Choi, Y. C., Choi, J. Y., Kim, J. G., Kim, M. S., Kim, W. T., Park, K. H., et al. (2009). Potential usage of food waste as a natural fertilizer after digestion by Hermetia illucens (Diptera: Stratiomyidae). Int. J. Ind. Entomol. 19, 171–174.
Couwenberg, J., Thiele, A., Tanneberger, F., Augustin, J., Bärisch, S., Dubovik, D., et al. (2011). Assessing greenhouse gas emissions from peatlands using vegetation as a proxy. Hydrobiologia 674, 67–89. doi: 10.1007/s10750-011-0729-x
Daverede, I. C., Kravchenko, A. N., Hoeft, R. G., Nafziger, E. D., Bullock, D. G., Warren, J. J., et al. (2004). Phosphorus runoff from incorporated and surface-applied liquid swine manure and phosphorus fertilizer. J. Environ. Qual. 33, 1535–1544. doi: 10.2134/jeq2004.1535
Diener, S., Zurbrügg, C., and Tockner, K. (2009). Conversion of organic material by black soldier fly larvae: establishing optimal feeding rates. Waste Manag. Res. 27, 603–610. doi: 10.1177/0734242X09103838
Eghball, B., and Gilley, J. E. (1999). Phosphorus and nitrogen in runoff following beef cattle manure or compost application. J. Environ. Qual. 28, 1201–1210. doi: 10.2134/jeq1999.00472425002800040022x
Elliott, A. L., Davis, J. G., Waskom, R. M., Self, J. R., and Christensen, D. K. (2007). Phosphorus fertilizers for organic farming systems. Master’s thesis Fort Collins, CO, USA: Colorado State University Libraries. doi: 10.3920/JIFF2022.0005
Esteves, C., Fareleira, P., Castelo-Branco, M. A., Lopes, I. G., Mota, M., Murta, D., et al. (2022). Black soldier fly larvae frass increases the soil’s residual nutrient content and enzymatic activity–a lettuce production trial. J. Insects Food Feed 8, 1–10.
Fernández-Romero, M. L., Clark, J. M., Collins, C. D., Parras-Alcántara, L., and Lozano-García, B. (2016). Evaluation of optical techniques for characterising soil organic matter quality in agricultural soils. Soil Tillage Res. 155, 450–460. doi: 10.1016/j.still.2015.05.004
Gärttling, D., Kirchner, S. M., and Schulz, H. (2020). Assessment of the N-and P-fertilization effect of black soldier Fly (Diptera: Stra-tiomyidae) by-products on maize. J. Insect Sci. 20, 1–11. doi: 10.1093/jisesa/ieaa089
Gutiérrez-Miceli, F. A., Santiago-Borraz, J., Molina, J. A. M., Nafate, C. C., Abud-Archila, M., Llaven, M. A. O., et al. (2007). Vermicompost as a soil supplement to improve growth, yield and fruit quality of tomato (Lycopersicum esculentum). Bioresour. Technol. 98, 2781–2786. doi: 10.1016/j.biortech.2006.02.032
Hernandez, O. L., Calderín, A., Huelva, R., Martínez-Balmori, D., Guridi, F., Aguiar, N. O., et al. (2015). Humic substances from vermicompost enhance urban lettuce production. Agron. Sustain. Dev. 35, 225–232. doi: 10.1007/s13593-014-0221-x
Houben, D., Daoulas, G., Faucon, M. P., and Dulaurent, A. M. (2020). Potential use of mealworm frass as a fertilizer: impact on crop growth and soil properties. Sci. Rep. 1, 1–9. doi: 10.1038/s41598-020-61765-x
Huang, B., and Eissenstat, D. M. (2000). Plant-Environment Interactions. New York, NY: Marcel Dekker, Inc.
Kagata, H., and Ohgushi, T. (2012). Positive and negative impacts of insect frass quality on soil nitrogen availability and plant growth. Popul. Ecol. 54, 75–82. doi: 10.1007/s10144-011-0281-6
Kaupper, T., Mendes, L. W., Harnisz, M., Krause, S. M. B., Horn, M. A., and Ho, A. (2021). Recovery of methanotrophic activity is not reflected in the methane-driven interaction network after peat mining. Appl. Environ. Microbiol. 87:e02355. doi: 10.1128/AEM.02355-20
Klammsteiner, T., Turan, V., Oberegger, S., Insam, H., and Juárez, M. F. D. (2019). Black soldier Fly (Hermetia illucens) Frass as plant fertilizer. 7th International Conference on Sustainable Solid Waste Management.
Koukounaras, A., Bantis, F., Karatolos, N., Melissas, C., and Vezyroglou, A. (2020). Influence of pre-harvest factors on postharvest quality of fresh-cut and baby leafy vegetables. Agronomy 10:172. doi: 10.3390/agronomy10020172
Mendoza-Tafolla, R. O., Juarez-Lopez, P., Ontiveros-Capurata, R. E., Sandoval-Villa, M., Iran, A. T., and Alejo-Santiago, G. (2019). Estimating nitrogen and chlorophyll status of romaine lettuce using SPAD and at LEAF readings. Not. Bot. Horti Agrobo. 47, 751–756. doi: 10.15835/nbha47311525
Miranda, C. D., Cammack, J. A., and Tomberlin, J. K. (2019). Life-history traits of the black soldier Fly, Hermetia illucens (L.) (Diptera: Stratiomyidae), reared on three manure types. Animals 9:281. doi: 10.3390/ani9050281
Narayan, S., Kanth, R. H., Narayan, R., Khan, F. A., Singh, P., and Rehman, S. U. (2013). Effect of integrated nutrient management practices on yield of potato. Potato J. 40, 84–86.
Neina, D. (2019). The role of soil pH in plant nutrition and soil remediation. Appl. Environ. Soil Sci. 2019, 1–9. doi: 10.1155/2019/5794869
Niu, G., and Masabni, J. (2018). Plant production in controlled environments. Horticulturae 4:28. doi: 10.3390/horticulturae4040028
Nongmaithem, D., and Pal, D. (2011). The effect of organic sources of nutrients on the growth attributes and yields of potato (Solanum tuberosum L.). J. Crop Weed 7, 67–69.
Putra, R. E., Hutami, R., Suantika, G., and Rosmiati, M. (2017). Application of compost produced by bioconversion of coffee husk by black soldier fly larvae (Hermetia illucens) as solid fertilizer to lettuce (Lactuca sativa var. crispa): impact to harvested bio-mass and utilization of nitrogen, phosphor, and potassium. In Proceedings of the International Conference on Green Technology, 8, 466–472.
Ravindran, B., Wong, J. W., Selvam, A., and Sekaran, G. (2016). Influence of microbial diversity and plant growth hormones in compost and vermicompost from fermented tannery waste. Bioresour. Technol. 217, 200–204. doi: 10.1016/j.biortech.2016.03.032
Romano, N., Webster, C., Datta, S. N., Pande, G. S. J., Fischer, H., Sinha, A. K., et al. (2023). Black soldier Fly (Hermetia illucens) Frass on sweet-potato (Ipomea batatas) slip production with aquaponics. Horticulturae 9:1088. doi: 10.3390/horticulturae9101088
Schmitt, E. (2020). Potential benefits of using Hermetia illucens frass as a soil amendment on food production and for environmental impact reduction. Curr. Opin. Green Sustain. Chem. 25:100335. doi: 10.1016/j.cogsc.2020.03.005
Setti, L., Francia, E., Pulvirenti, A., Gigliano, S., Zaccardelli, M., Pane, C., et al. (2019). Use of black soldier fly (Hermetia illucens (L.), Diptera: Stratiomyidae) larvae processing residue in peat-based growing media. Waste Manag. 95, 278–288. doi: 10.1016/j.wasman.2019.06.017
Shatilov, M. V., Razin, A. F., and Ivanova, M. I. (2019). Analysis of the world lettuce market. In IOP Conference Series: Earth and Environmental Science.
Shubha, K., Mukherjee, A., Tamta, M., and Koley, T. K. (2019). Arugula (Eruca vesicaria subsp. sativa (miller) Thell.): a healthy leafy vegetable. ICAR-Research Complex for Eastern Region, Patna.
Singh, M., and Wasnik, K. (2013). Effect of vermicompost and chemical fertilizer on growth, herb, oil yield, nutrient uptake, soil fertility, and oil quality of rosemary. Commun. Soil Sci. Plant Anal. 44, 2691–2700. doi: 10.1080/00103624.2013.813532
Smart, S. M., Glanville, H. C., Blanes, M. D. C., Mercado, L. M., Emmett, B. A., Jones, D. L., et al. (2017). Leaf dry matter content is better at predicting above-ground net primary production than specific leaf area. Funct. Ecol. 31, 1336–1344. doi: 10.1111/1365-2435.12832
Smith, T. M., and Smith, R. L. Elements of Ecology. 9th. (2015) Pearson Education, Inc. Hoboken, NJ.
Swiader, J. M., and Ware, G. W. Producing vegetable crops. (2002), Interstate Publisher Inc.: Danville, IL.
Tammam, A. A., Shehata, M. R. A. M., Pessarakli, M., and El-Aggan, W. H. (2022). Vermicompost and its role in alleviation of salt stress in plants–I. Impact of vermicompost on growth and nutrient uptake of salt-stressed plants. J. Plant Nutr. 46, 1–12. doi: 10.1080/01904167.2022.2072741
Xiao, X., Mazza, L., Yu, Y., Cai, M., Zheng, L., Tomberlin, J. K., et al. (2018). Efficient co-conversion process of chicken manure into protein feed and organic fertilizer by Hermetia illucens L.(Diptera: strati-omyidae) larvae and functional bacteria. J. Environ. Manag. 217, 668–676. doi: 10.1016/j.jenvman.2018.03.122
Yourtchi, M. S., Hadi, M. H. S., and Darzi, M. T. (2013). Effect of nitrogen fertilizer and vermicompost on vegetative growth, yield and NPK uptake by tuber of potato (Agria CV.). Int. J. Agric. Crop Sci. 5, 2033–2040.
Keywords: insect frass, vermicompost, macronutrients, micronutrients, productivity
Citation: Chavez MY, Uchanski M and Tomberlin JK (2024) Impacts of black soldier fly, Hermetia illucens, larval frass on lettuce and arugula production. Front. Sustain. Food Syst. 8:1399932. doi: 10.3389/fsufs.2024.1399932
Edited by:
Muhammad Saqlain Zaheer, Khwaja Fareed University of Engineering and Information Technology (KFUEIT), PakistanReviewed by:
Concepcion Paredes, Miguel Hernández University of Elche, SpainMuhammad Adnan Bodlah, Nanjing Agricultural University, China
Copyright © 2024 Chavez, Uchanski and Tomberlin. This is an open-access article distributed under the terms of the Creative Commons Attribution License (CC BY). The use, distribution or reproduction in other forums is permitted, provided the original author(s) and the copyright owner(s) are credited and that the original publication in this journal is cited, in accordance with accepted academic practice. No use, distribution or reproduction is permitted which does not comply with these terms.
*Correspondence: Maria Y. Chavez, bWFyaWEuY2hhdmV6QGNvbG9zdGF0ZS5lZHU=