- Facultad de Ciencias Agrarias, Universidad del Cauca, Popayán, Colombia
The production of instant flour constitutes a fast-expanding sector, and, this is an innovative area, that is being modified adjusting continually its methodologies to enhance production efficiency, optimizing its resources, fostering innovation in its applications, and increasing its economic income. Among the methods widely cited for precooked flours production are spray drying, drum drying, and extrusion cooking, the latter emerging as a high-potential and versatile solution to produce such commodities. In this regard, a comprehensive understanding of the extrusion process, its mechanical principles, and its effects on the physical characteristics of extruded raw materials is necessary. Analyzing process parameters (specifical mechanical energy and mean residence time) is essential to achieve the desired outcomes. Furthermore, it was analyzed the effect of the process modification conditions (temperature, screw speed, and moisture content) on the physical characteristics of the extruded instant flours. This review offers insights into the most reported system parameters as Specifical Mechanical Energy (SME), Pressure, Torque and, physical properties assessed in different instant flour obtained by extrusion such as Water Absorption Index (WAI), Water Solubility Index (WSI), Swelling Power (SP), Rehydration Capacity, Wetting Capacity, Sinking, Dispersibility, Pasting properties, Thermal properties, and Color. The review summarized and discussed the behavior of the hygroscopic properties and the water affinity of different instant flours obtained by extrusion.
1 Introduction
The growing urban metropolises, involving lifestyles, and the sustained economic growth of industrialized nations significantly influence the dynamics of food production and its concurrent demand. It is estimated that the global market for pre-cooked flours reached sales of $1.3 trillion in 2020, with projections indicating an expected increase to approximately $1.6 trillion by 2027, constituting a compound annual growth rate of 0.4% (Markets, 2022). Pre-cooked flours exhibit a wide spectrum of utility, serving as intermediate or final products. Between the applications are the formulation of infant food, cereal blends, cereal breakfast, porridge, soups, and dairy formula development. The food industry that specializes in pre-cooked flour, also reports advancements in their utilization as essential components, encompassing pre-cooked flours, hydrolyzed flours, bakery premixes, powdered beverage substrates, stabilizing agents, gelling agents, and binding agents (Transparency, 2019b). Beneficiary sectors of these developments include the pharmaceutical, cosmetic, and animal nutrition industries. The market segmentation for pre-cooked flours can be based on the source raw materials like wheat, corn, rice, and legumes, among others. An alternative classification criterion is the origin of these flours (organic or conventional). Additional categorization is feasible based on distribution channels, spanning hypermarkets, supermarkets, specialized markets, and retail outlets. Final criterion is related to the geographical delineations encompassing Europe, South Asia, East Asia, the Middle East and Africa, Oceania, North America, and South America (Transparency, 2019a; MMR, 2021).
The most reported techniques for the pre-cooking of flours include spray drying (SD), drum drying (DD), and extrusion processes (E). Pre-cook represents an efficient process because of capacity to enhance modify flour attributes at industrial level. Also, pre-cook is environmentally friendly because of its low use of water and reduced by-products amount (Ma et al., 2022). The properties of the resultant flour depend on the specific technique employed, for example, the pre-cooked of starchy flours by spray drying generates a starch modification with granular integrity, this effect influence the generates a starch modification with granular integrity, this effect influence properties such as water solubility, particle density, wettability, dispersibility. Conversely, extrusion or drum drying exert strong starch modifications, enhancing starch solubility and stability of viscous cold water (Ma et al., 2022).
Comparing the three techniques (SD, DD, E) available for precooking flour, the extrusion process is efficient in the use of resources. Extrusion generates a high level of conversion in the components of raw materials (starch, protein, fiber) at low moisture content. The extrusion equipment is only one process unit that involves kneading, mixing, cooking, shaping and others. The extrusion process has several effects on the modification of raw material, such as starch gelatinization, protein denaturation, reduction of lipid oxidation, enhancement of soluble dietary fibers, and others (Nikmaram et al., 2017; Pismag et al., 2023). In this regard, the purpose of this review is to analyze the effect of the changes in process parameters (temperature, moisture, screw speed) on the extruder performance (system parameters), at same time, discuss the behavior of physical and hygroscopic properties (product parameters) of different instant flours obtained by extrusion.
2 Extrusion as a method for obtaining pre-cooked flours
Food processing methods exert a strong influence on the physical and chemical attributes of food products. Emerging techniques of food processing yield a diverse range of products. However, it is imperative to align these changes with the requirements of customers (He et al., 2020). The aim behind the pre-cooking or pre-gelatinization of flours or starches is to produce instant preparation foods with high digestibility and distinctive flavor profiles, extending beyond conventional cooking (He et al., 2020). Pre-gelatinization of starches or flours modifies their physical properties, including water absorption, water solubility, pasting properties, and storage stability, enabling numerous applications (Wiriyawattana et al., 2018).
Extrusion cooking is a high-temperature, short-time (HTST) process with high efficiency in food processing, characterized by low levels of waste and emissions (Guy, 2001; Ali et al., 2016). Extrusion cooking provides enough thermomechanical energy to induce substantial physicochemical transformations in raw food materials. It facilitates the mixing, dispersion, and homogenization of food constituents. The melted materials are subsequently transported within a cylinder and pulled through a die designed for shaping purposes (Riaz, 2000). Extrusion is a process unit that involves different unit operations at the same time, including cooking, conveying, mixing, kneading, pasteurization, cooling, shaping, and cutting (Riaz, 2000; Ding et al., 2005; Egas et al., 2010; Offiah et al., 2018).
Extrusion cooking is an alternative technique in the production of pre-cooked flour, as it promotes the gelatinization of starches (Mouquet et al., 2003). Those conditions cause the breakaging of covalent bonds in amylose and amylopectin, resulting in the loss of particle integrity and partial depolymerization of the starch components. This strong structural breakdown promotes changes in the functional properties of starch (Ma et al., 2022). Concurrently, extrusion cooking has an influence on other nutritional components, enhancing protein digestibility while diminishing the anti-nutritional factors such as trypsin inhibitors (Mouquet et al., 2003; Ali et al., 2016).
3 Extrusion process parameters
The extrusion process can be analyzed by examining the effects of modifying independent parameters that influence a set of dependent parameters. The multidimensional nature of the extrusion process has led to the development of an analytical model described in Figure 1. The process parameters (independent) can be adjusted according to the desired characteristics of the final product. These parameters can be classified as either adjustable or variable parameters. Adjustable parameters should be configured prior to equipment operation, and they are related to equipment design, such as screw configuration, screw rotation, die design, and others. On the other hand, variable parameters can be adjusted during equipment operation, for example, barrel temperature, moisture content, screw speed, and others (Rubin, 2012).
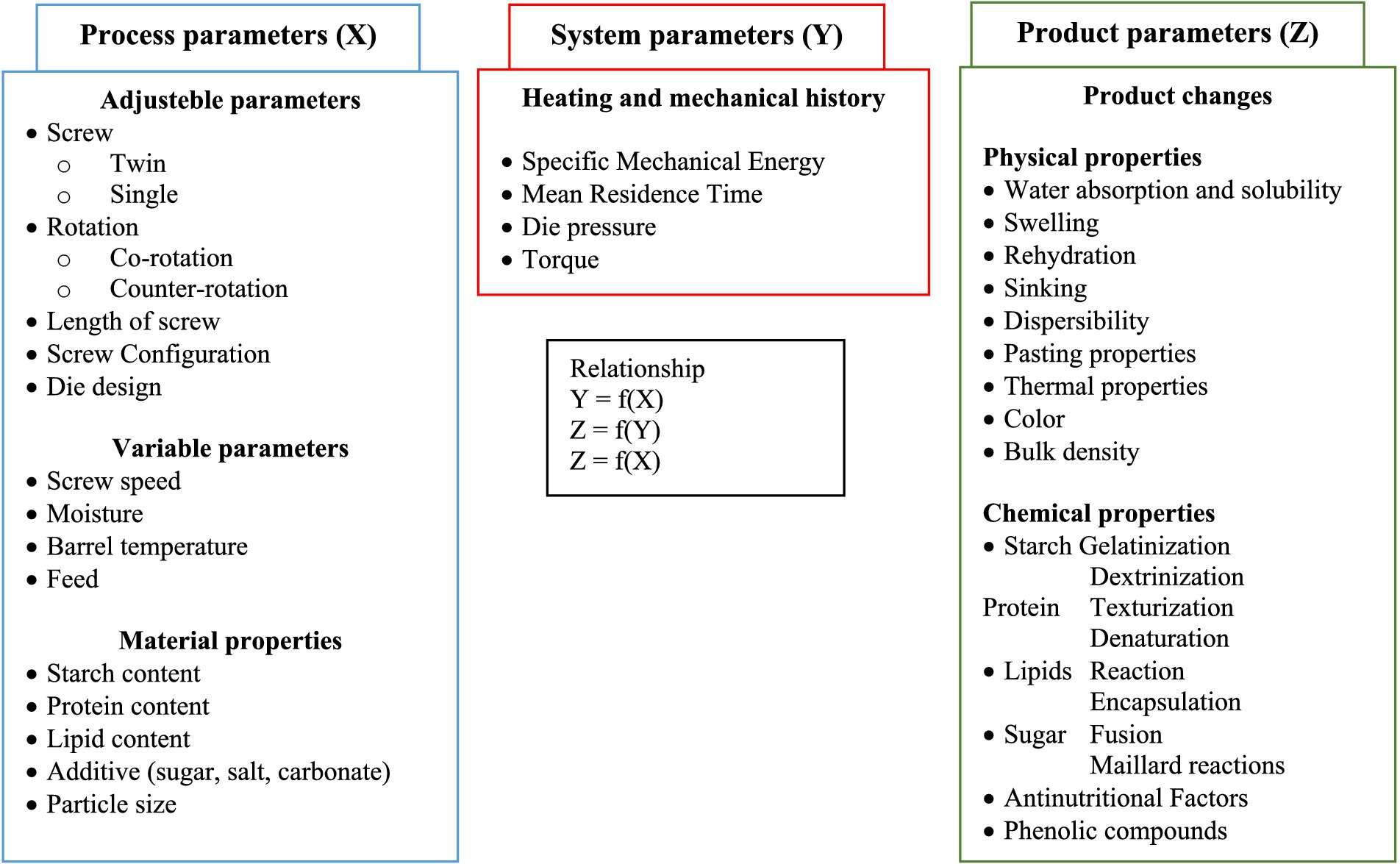
Figure 1. Multidimensional analysis of the extrusion process. [Modified of Rubin (2012)].
The dependent parameters are classified into two categories: system parameters, related to the equipment operation, and product parameters, associated with the transformations by the raw materials fed to the extruder. The Specific Mechanical Energy (SME) and Torque (T) are the system parameters that allow estimate the energy consumption and the mechanical force exerted during the extrusion process. Likewise, it is possible to estimate the time at which the raw material crosses through the extruder barrel, quantified as the Mean Residence Time (MRT). Furthermore, it is possible to establish the pressure level inside the equipment, this condition can also be partially controlled, and its behavior depends on the process parameters.
Conversely, product parameters describe the changes in the physicochemical attributes of the final product. These parameters represent the outcomes observed at the end of the process, with their values depending on the manipulation of process and system parameters. The analytically model depicted in the Figure 1 offers a comprehensive overview of the unit employed, enabling the anticipation of likely changes in the raw materials, the mechanisms governing their occurrence, and their development with the extrusion equipment (Ding et al., 2005; Patil et al., 2007; Pansawat et al., 2008). This schematic analysis of the extrusion process is essential because it aids in understanding how modifying independent parameters affects dependent parameters during flour processing using this technique. The following sections of the document will explore the interaction of the most commonly reported parameters during the production of extruded flours.
3.1 Effect of extrusion on system parameters
The assessment of system parameters within the extrusion process explains the values engendered by the equipment during operation in response to the modifications in process parameters. The system parameters serve as a descriptor of equipment performance during the cooking of raw materials. Specific Mechanical Energy (SME), torque (T), and pressure (P) are the most frequently documented system parameters in the context of extrusion to obtain pre-cooked flours.
Table 1 shows a compilation of different plant-based raw materials, either pure or mixed, used in the development of precooked flours by extrusion process. Throughout these processes the behavior of the extrudate materials has been evaluated. The work applied by the main motor to move the melted raw materials through the barrel is represented as SME. This crucial process parameter is intrinsically associated with the amount of energy required to facilitate the transformation of raw materials. For instance, the increasing SME corresponds to a dextrinization of starches and denaturation of proteins (Heredia et al., 2019).
The temperature during the process influences the viscosity of the melted material, while moisture content acts as a lubricant, enhancing the flow of the materials inside the extruder. Together, these factors contribute to a reduction in energy consumption, resulting in a lower SME. Conversely, increasing the screw speed in the extruder elevates shear and friction forces, thereby increasing the SME (Pathania et al., 2013; Ai et al., 2016; Ali et al., 2016).
As with SME, torque (T) is closely linked to the interaction between the screw speed and the flow of the melted materials. Torque is strongly influenced by changes in temperature, moisture content, and screw speed, as shown in Table 1. A decrease in Torque can be attributed to the reduction in material viscosity, achieved through increased parameters such as temperature, moisture content, and screw speed during the extrusion process (Ai et al., 2016; Li et al., 2020).
On the other hand, the variation in pressure (P) inside the extruder results from the restriction encountered by the melted material troughing the die at the exit in the extruder, often causing accumulation just before the exit (Ai et al., 2016). According to the reports in Table 1, higher temperatures, moisture content, and screw speed typically lead to a decrease in pressure during extrusion. Overall, changes in process parameter can modify the viscosity of melted material and affect SME, T, and P, as system parameters (Pathania et al., 2013).
3.2 Effect of extrusion on product parameters
The extrusion is a versatile operation because it facilitates the processing of a wide range of raw materials, including cereals, legumes, and mixtures, to yield pre-cooked flours with specific physical attributes. The development of the physical properties in the pre-cooked flours results from the process conditions in the extruder and the consequent chemical modifications in the processed materials. The outcomes stemming from the process conditions encompass a wide range of results, which will be discussed below. While the following discussion clarifies several physical properties of precooked flours obtained by extrusion, it is important to highlight that the industry leading its innovations toward properties such as flowability, water holding capacity, consistency, and thickening among others (Transparency, 2019b; Markets, 2022).
3.2.1 Water absorption index and water solubility index
The Water Absorption Index (WAI) and Water Solubility Index (WSI) are classified as product parameters and are considered indirect indicators of the degree of starch conversion within the raw materials. The WSI is denoted as the proportion of water-soluble constituents within the extruded material, expressed as a percentage of the dry matter. This parameter is related to starch dextrinization and its extent of gelatinization during the extrusion (González et al., 2007; Atukuri et al., 2019). Aditionally, the WSI measures the capacity of solubilization in solvents of pre-cooked flours or food powders. The behavior of this property depends on the molecular weight of the soluble fractions and their capacity to develop hydrogen bonds with water (Gandhi et al., 2018; Atukuri et al., 2019). The behavior of WSI in extruded materials is variable, with its response depends on the modification of single process parameters or their combinations (see Table 2).
Many of the listed samples in Table 2 show an increase in WSI due to the increase in the extrusion temperature (Pathania et al., 2013; Ali et al., 2016; Cheng et al., 2020; Pasqualone et al., 2021; Ruiz et al., 2022; Zhang et al., 2023). The temperature facilitates starch gelatinization, leaching of amylose, and dextrinization through the breaking of starch into lower-weight molecules (Gandhi et al., 2018; Atukuri et al., 2019). At the same, the increase in screw speed engenders heightened shear forces, advancing structural starch changes, causing fractionation and beraking of starch particles with an increase in the quantity of soluble material (Pathania et al., 2013; Sarawong et al., 2014; Kowalski et al., 2016; Arora et al., 2020; Pasqualone et al., 2021; Ruiz et al., 2022). Otherwise, increased moisture content inversely affects shear forces, reducing the mean residence time of extruded material within the extruder, thereby constraining the thermo-mechanical modification of accessible starch and other macromolecules (Ali et al., 2016).
On the other hand, the WAI is the ability of the extruded material to form a gel in excess of water also described as moisture holding capacity of pre- gelatinized starches (Pathania et al., 2013). During extrusion, gelatinization affects the molecular interaction within starch structures (amorphous and crystalline zones), disrupting of intermolecular or intramolecular bonds and exposing hydroxyl groups to form hydrogen bonds with water (Sotelo et al., 2023).
In many cases from Table 2, WAI shows an inverse correlation with WSI, indicating higher WAI values correspond to lesser structural change in starches and reduced soluble fraction (WSI). Decerasing WAI con occur with higher temperatures and screw speeds during extrusion, linked to the starch gelatinization extent. Elevated extrusion temperatures enhance the degree of gelatinization starch, reducing the WAI. Similarly, heightened screw speeds and lower moisture intensify shear and friction forces, causing starch granulate changes by fragmentation, resulting in damage by mechanical effect.
However, González et al. (2007), Kowalski et al. (2016), and Cheng et al. (2020) report unusual behavior of WAI and WSI parameters in theirstudies, they observe high WAI with increased moisture content and extrusion temperature. In contrast, Dogan and Karwe (2003) suggest that WAI is influenced by interactions involving low-molecular-weight compounds released during starch gelatinization and protein denaturation. These interactions form new complexes with hydrophilic properties, thereby increasing both WAI and WSI simultaneously.
3.2.2 Swelling power
Swelling Power (SP) refers to the ability of starches to absorb water and increase in size (Otondi et al., 2020). It indirectly measures the conformation, distribution, and proportion of amylopectin within the starch structure (Cheng et al., 2020). However, these properties alone cannot fullypredict swelling capacity. Starch structure variations derived from the same botanical species from different origins can yield different results (Zhu, 2016). Exploring these attributes in pre-cooked flours helps define potential applications in formulating highly viscous foods such as soups, purées, and porridge.
Table 3 reports indicate that the interaction between extrusion temperatures and moisture content influences the swelling capacity of starches in pre-cooked flours (Qi et al., 2020). Severe extrusion conditions significantly alter starch structure, leading to dextrinization and reduced swelling capacity (Zhang et al., 2016).
Cheng et al. (2020) studied buckwheat flour extrusion and observed an increase of the swelling power (SP) with higher barrel temperatures and moisture content. They attribute SP increase to damage of starch crystalline structures, exposing more active sites for water binding. Conversely, low moisture content during extrusion intensifies the SME, fragmentation starches and reducing SP in the pre-gelatinized flour.
In contrast, Otondi et al. (2020) noted significantly higher SP with increased moisture content during extrusion, this phenomenon was attributed to enhanced internal exposure of starch granulates during the gelatinization induced by extrusion. The increasing trend of SP based on the high level of temperature and moisture content during the extrusion process was reported by Martínez et al. (2014a) in extruded rice flour. They suggest that starch gelatinization leads to loss of starch granule integrity and higher SP.
Most authors find an improved SP in pre-cooked compared to native flours. However, the presence of proteins and lipids in whole flours can modify SP behavior in extruded products. Martínez et al. (2014a, b) suggest that interaction among components, particularly proteins, influence physical properties post-extrusion.
Kesselly et al. (2023) report that increasing extrusion temperature reduces the SP in cowpea flour, while higher moisture content increases SP by facilitating starch gelatinization. Moisture acts as a plasticizer, regulating starch gelatinization, and preventing their dextrinization. However, increasing the temperature can alter SP due to protein interactions with the starch granules. Vegetable proteins with their high water affinity, further modify flour SP behavior turning hydrophobic under high temperatures during extrusion (Wang et al., 2019).
Jafari et al. (2017) found a reduction SP in sorghum flour with higher barrel temperatures and lower the moisture content, promoting amylose-lipid complexes formation that hinder water absorption. Lower extrusion temperatures and moisture content favor the starch packing, reducing water absorption and SP values.
3.2.3 Rehydration capacity
Rehydration capacity, also known as rehydration rate (Shan et al., 2015) or hydration power (Bhati et al., 2022), evaluate structural changes in the constituents of extruded flours and their interaction with water. This parameter is crucial for prospective applications like porridge, soups, or infant foods, where the hydration is necessary before consumption. Table 4 confirms that rehydration rate in extruded flours varies with changes in extrusion parameters (Kaur et al., 2019), influenced by starch and protein molecular weight reductions that increase water-binding sities (Bhandari et al., 2013).
Increased extrusion temperature enhances starch gelatinization and protein denaturation, boosting water-binding capacity (Kaur et al., 2019). However, excessive temperatures favor starch dextrinization with reduced hydration rates (Bhati et al., 2022). Conversely, higher moisture content during extrusion decrease rehydration rate due to shorter residence time of the melted material and promoting gentler extrusion conditions (Yu et al., 2013; Kaur et al., 2019). Moreover, higher screw speeds increase the rehydration rates by mechanical altering starch and protein structures (Kaur et al., 2019).
3.2.4 Wetting capacity
Wetting capacity is the first stage of the rehydration cycle of food powders, pre-cooked, or instant flours and is measured as a function of time (Barbosa, 2021). This parameter measures the ability of the powder to become wet upon contact with liquid (Fitzpatrick et al., 2016; Oliveira et al., 2019; Eke et al., 2021). Changes in wetting capacity depend on extrusion process parameters (see Table 5), flour composition, the fat content, particle size, and affinity for reconstituting liquid (Fournaise et al., 2021). According to Dhanalakshmi et al. (2011), larger particle sizes, high porosity, and lipid presence on particle surfaces contribute to optimal wetting capacity in pre-cooked or instant flours.
Giraldo et al. (2019) observed that the increasing extrusion temperature and screw speed reduced the wetting capacity of commercial extruded banana flour over time. They also noted that the particle size significantly influenced wetting capacity. This study concluded that under the evaluated process conditions can produce extruded banana flour suitable for instant preparation.
Conversely, Filli et al. (2013) investigated the wetting capacity of an extruded blend of millet and soy flours over time. They examined soy incorporation levels, blend moisture content, and screw speed. Their findings indicated that a higher soy content prolonged wetting capacity, likely due to an increase in fat content. This underscores the substantial impact of raw material compositional on wetting capacity. Filli et al. (2013) also highlighted the significant role of particle size in influencing wetting capacity.
While none of the presented cases directly relate the modification of process variables like temperature, moisture, and screw speed to the wetting capacity of flours, it is important to consider the series of phenomena occurring during the extrusion. These include thermo-mechanical effects, starch gelatinization and protein denaturation. These phenomena significantly influence particle sizes modification and agglomerate formation in water, which are key factors affecting wetting capacity (Dhanalakshmi et al., 2011; Fitzpatrick et al., 2016; Wang et al., 2020; Eke et al., 2021). Despite wetting capacity being essential for the quick preparation of modified flours, there is insufficient information on how process parameters modifications affect the wetting capacity in pre-cooked flours obtained by extrusion.
3.2.5 Sinking
When determining the properties of pre-cooked flour or instant food, three key phenomena must be considered: wetting, sinking and dispersion (Bhandari et al., 2013; Crowley et al., 2016; Nahemiah et al., 2016, 2017). Sinking occurs immediately after the powder particles become sufficiently wet, causing them to precipitate in the solvent due to increased density (Crowley et al., 2016; Eke et al., 2021). This process is rapid, making it challenging to accurately measure the rate at which a particles sink. While commonly evaluated in powdered milk, a standardized methodology is still developing.
Sinking begins with the wetting, followed by exchange of within the particles and surrounding liquid. Due to its speed, obtaining precise information about sinking is difficul. This is why specific studies on this property are rare, as it is often considered the final stage of the wetting process. Barbosa (2021) and Crowley et al. (2016) agree that sinking is part of the wetting process of food powders, can refer to the same process. Like wetting, the sinking capacity of instant powdered food is influenced by its particle size (Hogekamp and Schubert, 2003; Wang et al., 2020), process conditions (temperature, moisture, screw speed), and the composition of raw materials, particularly the relationship between starch, proteins, fat or lipids (Bhandari et al., 2013).
3.2.6 Dispersibility
Dispersibility is the third stage of the rehydration process of instant food flours or powders. The process begins with wetting, followed by the sinking and then the dispersion of the wet agglomerates into individual particles which then solubilize (Barbosa, 2021; Fournaise et al., 2021). Dispersibility dependents on the particle size, porosity, material density (Eke et al., 2021; Lee and Yoo, 2023), and the chemical composition of the particle surface (Fournaise et al., 2021). Nahemiah et al. (2016) found that pre-cooked flour from extruded rice and cowpea mixtures improves dispersion when processed at low temperatures and low moisture contents. These process parameters influence dispersion of the extruded mixture.
However, as shown in Table 6, most studies reports increased dispersion with higher extrusion temperatures. According to Tanhehco and Ng (2005), an increase in SME during extrusion, under certain conditions (temperature and moisture), can decrease dispersion due to the formation of aggregates during rehydration.
The energy requirement of the extruder (SME) during raw material processing is directly influenced by factors such as temperature, which affect the viscosity of the material as it passes through the extruder. Low process temperatures increase SME due to the challenges in starch gelatinization and protein denaturation, leading to aggregate formation. Similarly, high moisture content during extrusion, reduce SME by facilitating starch gelatinization, thus improving dispersibility of processed flour (Tanhehco and Ng, 2005).
Dispersion, like wettability and sinking characteristics of the pre-cooked flours, is sensitivity to particle size. Generally, large particle size enhance these properties. However, excessive fine particles may form agglomerates, hindering effective wetting mechanisms (Giraldo et al., 2019).
3.2.7 Pasting properties
The pasting properties helps to verify the structural damage to the starches caused by hydrothermal processes during extrusion (Akande et al., 2017). Generally, these properties decrease in extruded flours compared with their native counterparts (Mahasukhonthachat et al., 2010; Menegassi et al., 2011; Sarawong et al., 2014; Qi et al., 2020), due to the loss of the starch structural integrity. Hydrothermal treatments like extrusion induce starch gelatinization by swelling and water absorption in its amorphous regions, disrupting the crystalline regions in the starch granules (Martínez et al., 2014b).
Table 7 describes how extrusion parameters such as temperature, moisture content, and screw speed affect pasting properties like temperature of extent (TE), the peak viscosity (PV), gel instability or break down (BD), and the final viscosity (FV). The interaction between the process parameters in the extrusion system influences the pasting properties of the extruded flour.
This evaluation helps assess starch integrity but the test can be influenced by the presence of proteins, lipids, and fibers available in the flours (Qi et al., 2020). Another important consideration is that in all the cases reported, the native flours consistently show higher pasting values in comparison with extruded flours, indicating significant structural modification during extrusion (Menegassi et al., 2011). Table 7 highlights that extrusion temperature reduces all pasting parameters, crucial for designing instant foods (Menegassi et al., 2011; Ai et al., 2016; Qi et al., 2020).
During heating of the starch solution, viscosity gradually increase to the maximum peak viscosity (PV), considered as the balance between the swelling and rupture of the starch granules. High extrusion temperatures cause significant starch damage due to a starch gelatinization, showing low values in PV (Menegassi et al., 2011; Ai et al., 2016; Qi et al., 2020). The increase in moisture content also reduce pasting parameters, including gel instability or breakdown (BD), indicating gelatinized starch resistance to temperature and shear (Qi et al., 2020). The final viscosity decreases due to higher extrusion temperatures, reflecting the re-association of starch molecules, especially amylopectin during cooling (Menegassi et al., 2011).
Increasing moisture content and screw speed during the extrusion process both reduce pasting properties. Higher moisture content provides sufficient water for starch gelatinization, causing greater structural damage and resulting in lower pasting values (Rodríguez et al., 2015). Similarly, higher screw speeds exert significant mechanical effect on starches, leading to their modification and a reduction in pasting properties (Mahasukhonthachat et al., 2010), as shown in Table 7.
However, Sarawong et al. (2014), reported that lower moisture content cause greater starch degradation due to increase in shear due to a mechanical effect of the screws in the extruder. Although there are few reports on the effects of screw speed on pasting properties, it can be inferred that higher screw speeds reduce the residence time inside the extruder, insufficient for starch gelatinization. This was observed by Sarawong et al. (2014) during the extrusion of green banana flour. This pattern is also seen in parameters like BD and final viscosity.
3.2.8 Thermal properties
In the extrusion processes of starchy raw materials, thermal properties change to the loss of molecular order in starch fraction (Table 8). The behavior is attributed to the loss in the molecular order of the starch fraction and is related to the gelatinization process due to starch swelling and a consequent loss in its structural order during the extrusion process. The thermo-mechanical effects from change in extrusion parameters can significant alter starch structural (Lai et al., 2022).
The changes in thermal properties following the extrusion process led to variations in To, Tp, and Tc, and reduce endotherms (see Figure 2). Garcia et al. (2019) found that the extrusion of two types of plantain flour reduce the endotherm and gelatinization enthalpy (ΔH), indicating complete starch gelatinization.
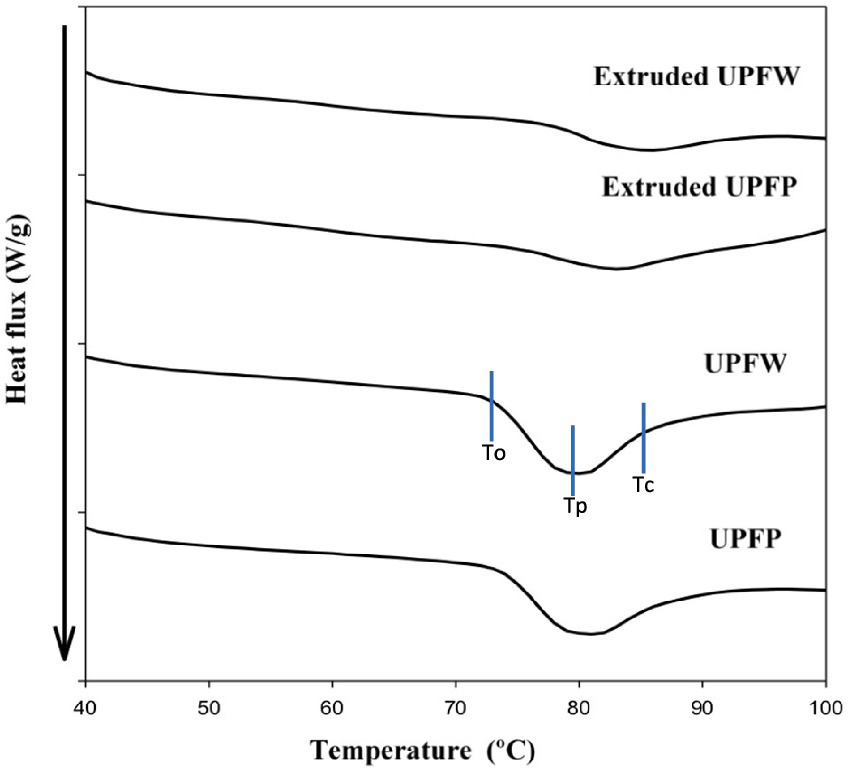
Figure 2. Differential scanning calorimetry of native and extruded flours of unripe plantain pulp (UPFP) and whole fruit (UPFW). [Modified of Garcia et al. (2019)].
Menegassi et al. (2011) explain that low ΔH values in pre-gelatinized flours by extrusion are due to the structural starch damage. Typically, the parameters defining the endothermic peak (T0, Tp, Tc) slightly increase in extruded flours compared to native flours, likely due to a structural alteration in starch molecules and to the presence of starchy fractions that resist gelatinization, requering more energy (Martínez et al., 2014a).
Table 8 shows a direct relationship between thermal properties and pasting properties, resulting from structural modifications in starch, proteins and the formation of lipid complexes during extrusion (Menegassi et al., 2011). The evaluation of pasting and thermal parameters indicates that the greater structural and morphological alteration due to cooking extrusion result in lower magnitudes in the pasting properties (PV, BD, FV, and TE), reduced ΔH and increased values of To, Tp, and Tc.
3.2.9 Color
Color changes in extruded products are mainly related to a non-enzymatic reactions, such as Maillard reactions or caramelization, resulting from the starch degradation into sugars and their interaction with free amino acids during extrusion (Menegassi et al., 2011; Martínez et al., 2014a,b; Wang et al., 2020). Controlling color is crucial, as it directly influences consumer perception of quality (Menegassi et al., 2011; Kowalski et al., 2016).
Color changes in flour extrusion are typically marked by a loss of luminosity (L*) and an increasein red (+a*) and yellow (+b*) hues (Menegassi et al., 2011; He et al., 2020). As shownin Table 9, thermal effects are major contributors to darker colors during extrusion (He et al., 2020). In pre-cooked flours, increased process temperatures and moisture lead to reduced L* values and higher a* and b* values due to Maillard reaction and pigment oxidation, resulting in browner products (Jafari et al., 2017).
Jafari et al. (2017) observed that increasing moisture content during extrusion of sorghum flour controls the loss of lightness (L*), resulting in lighter shades similar to native flour. This effect is due to reduced residence time inside the extruder, insufficient for the non-enzymatic browning reactions. Similar findings reported by Gulati et al. (2016) and Wang et al. (2020), who noted that higher moisture content during extrusion helps maintain L* values and reduces red (a*) and yellow (b*) color production by minimizing the thermomechanical effects.
However, Kowalski et al. (2016) and Martínez et al. (2014a, b) found contrary results, observing that increased moisture content intensifies brown colors extruded flours. This darkening results from a higher degree of gelatinization, leading to more reducing sugars interacting with the amino groups in the flour proteins, triggering the Maillard reaction (Kowalski et al., 2016). On the contrary, increasing screw speed during extrusion reduces the residence time of the sample, preventing the formation of brown colors and increasing a* and b* parameters (Gulati et al., 2016; Kowalski et al., 2016).
3.2.10 Bulk density
Bulk density is an important quality criterion for packing, affecting storage costs, transportation, product standardization, and process efficiency. Bulk density is commonly defined as the mass of the powder (m) filling a vessel of known volume (V), expressed as m/V (Barbosa, 2021). Whiles some studies measure the bulk of the pellets directly after the extrusion, this property is closely related to the expansion capacity of the melt material as it exits the extruder. The measurement is typically estimated as the weight of the pellet pieces per unit volume (Nyombaire et al., 2011; Wang et al., 2019).
During the extrusion process, the melted material undergoes a sharp pressure change, causing it to puff and reducing the bulk density of extruded flour. Low bulk density powders have high porosity and specific surface area, enhancing their interaction with water molecules improving wettability of the extruded flour. Analyzing this property is particularly important when extrusion conditions (parameter process) are modified.
Table 10 shows that temperature and screw speed significantly reduce the bulk density of all the extruded flours. This reduction is related to the pressure drop from inside the extruder to atmospheric pressure. During the extrusion increased temperature and screw speed create high-pressure conditions. When the melted material exits the die, the rapid transfer from high internal pressure to atmospheric pressure causes the internal moisture content to flash off suddenly. This promotes bubble formation, which becomes entrapped in the melted material, resulting in highly expanded, low-density extrudates (Ali et al., 2016; Wang et al., 2019, 2020).
Moisture content is the main factor affecting extrudate density and expansion. As shown in Table 10, high moisture levels reduce bulk density by altering amylopectin networks in the starch-based materials. This change reduce dough elasticity through plasticization of the melt, resulting in reduced SME and gelatinization, which in turn decreases expansion and increases the density of the extrudates (Pathania et al., 2013; Ali et al., 2016).
4 Conclusion
Extrusion is a spreadable operation that is being used as an alternative mechanism to obtain pre-cooked flours useful in the development of instant preparation foods. It is a process widely used on an industrial scale. However, it is a technique that is once again gaining interest in the development of food, using as a production base unconventional raw materials such as quinoa, amaranth, native beans, native corn, and even fruits such as bananas and agroindustrial byproducts from the processing of other industries such as soybean cakes, all processed by the same technique to obtain finished products or bases for the development of new foods.
In this sense, the physical behavior of pre-cooked flours obtained by the extrusion process is influenced by its chemical composition and the changes that each of its components may undergo due to the change in extrusion conditions. The thermal and mechanical effects developed inside the equipment can modify the availability of sufficient active sites that facilitate the raw materials to interact with the solvent medium. Likewise, it is necessary to consider that the intensity of the thermal and mechanical effect developed inside the equipment and applied to the raw materials will promote their fractionation or even the formation of complexes that can alter their behavior against the solvent medium.
On the other hand, the hydration of pre-cooked flours used in the development of instant preparation foods follows a pattern that has been fully identified and is described in four stages starting with wetting, sinking, dispersion, and solubilization. However, information about methodologies that help their quantification in flours pre-cooked by extrusion is not yet fully developed. The reporting of this type of protocol or results could provide clarity on the possible uses of flours modified by extrusion, and it is necessary to continue expanding knowledge about the physical behavior of pre-cooked flours.
4.1 Prospective
The production of instant flours obtained by different methods such as spray dry, drum dry, and extrusion, is a sector that covers more and more markets, some of them very selective in terms of consumption, however, this type of product is gaining importance in the production of food for low-income populations. In this last aspect, the usefulness of efficient techniques in the usage of resources, space, versatility, and production capacity, such as extrusion, has been very important.
Extrusion is a widely studied technique that has allowed the transformation of unconventional raw materials such as Amaranth, Buckwheat Tartary, Sesame, Sorghum, Millet, and different native species of Beans and Maize, all of them produced in regions where the development of foods with high nutritional potential is indispensable to cover the requirements of vulnerable populations.
However, it is important to know how to understand the changes that the use of extrusion generates on the processed raw materials by evaluating operating conditions such as changes in temperature, moisture content, and screw speed, but it is still necessary to investigate other equally important variables such as feeding speed, nozzle size, screw configuration, and others.
Likewise, it is important to study the physical properties of extruded flours and their capacity for instant preparation, understanding in detail the process of interaction with solvents such as water. Unfortunately, the information available regarding this type of study is scarce, and the methodologies for the physical evaluations of instant products are still under development, for this reason, it is necessary to continue researching equipment and protocols to obtain accurate answers regarding many of the hydration properties of instant products obtained by extrusion.
Author contributions
RP: Conceptualization, Investigation, Methodology, Writing – original draft, Writing – review & editing. JR: Investigation, Methodology, Writing – original draft, Writing – review & editing. JH: Resources, Validation, Writing – original draft, Writing – review & editing. JB: Conceptualization, Resources, Writing – original draft, Writing – review & editing. DR: Supervision, Writing – original draft, Writing – review & editing.
Funding
The author(s) declare financial support was received for the research, authorship, and/or publication of this article. This work has been fully financed by the Colombian General Reimbursement System, Universidad del Cauca, and SEGALCO, within the framework of the SGR BPIN 2020000100052 project.
Conflict of interest
The authors declare that the research was conducted in the absence of any commercial or financial relationships that could be construed as a potential conflict of interest.
Publisher’s note
All claims expressed in this article are solely those of the authors and do not necessarily represent those of their affiliated organizations, or those of the publisher, the editors and the reviewers. Any product that may be evaluated in this article, or claim that may be made by its manufacturer, is not guaranteed or endorsed by the publisher.
References
Ai, Y., Cichy, K., Harte, J., Kelly, J., and Ng, P. (2016). Effects of extrusion cooking on the chemical composition and functional properties of dry common bean powders. Food Chem. 211, 538–545. doi: 10.1016/j.foodchem.2016.05.095
Akande, O., Nakimbugwe, D., and Mukisa, I. (2017). Optimization of extrusion conditions for the production of instant grain amaranth-based porridge flour. Food Sci. Nutr. 5, 1205–1214. doi: 10.1002/fsn3.513
Ali, S., Singh, B., and Sharma, S. (2016). Response surface analysis and extrusion process optimization of maize mungbean based instant weaning food. Int. J. Food Sci. Technol. 51, 2301–2312. doi: 10.1111/ijfs.13186
Arora, B., Yoon, A., Sriram, M., Singha, P., and Rizvi, S. (2020). Reactive extrusion: a review of the physicochemical changes in food systems. Innov. Food Sci. Emerg. Technol. 64:102429. doi: 10.1016/j.ifset.2020.102429
Atukuri, J., Odong, B., and Muyonga, H. (2019). Multi response optimization of extrusion conditions of grain amaranth flour by response surface methodology. Food Sci. Nutr. 7, 4147–4162. doi: 10.1002/fsn3.1284
Basilio, J., Condezo, L., and Repo, R. (2020). Effect of extrusion cooking on the physical-chemical properties of whole kiwicha (Amaranthus caudatus L) flour variety Centenario: process optimization. LWT Food Sci. Technol. 128:109426. doi: 10.1016/j.lwt.2020.109426
Bhandari, B., Bansal, N., Zhang, M., and Schuck, P. (2013). Handbook of food powders. 1st Edn. London: Wooden Publishing Limited.
Bhati, D., Singh, B., Arashdeep, S., Sharma, S., and Pandiselvam, R. (2022). Assessment of physicochemical, rheological, and thermal properties of Indian rice cultivars: implications on the extrusion characteristics. J. Texture Stud. 53, 854–869. doi: 10.1111/jtxs.12678
Cheng, W., Gao, L., Wu, D., Gao, C., Meng, L., Feng, X., et al. (2020). Effect of improved extrusion cooking technology on structure, physiochemical and nutritional characteristics of physically modified buckwheat flour: its potential use as food ingredients. LWT 133:109872. doi: 10.1016/j.lwt.2020.109872
Crowley, S., Kelly, A., Jeantet, R., and O’Mahony, J. (2016). “Rehydration and solubility characteristics of high-protein dairy powders” in Advanced dairy chemistry. eds. P. L. H. McSweeney and P. A. O’Mahony (Berlin: Springer), 99–131.
Dhanalakshmi, K., Ghosal, S., and Bhattacharya, S. (2011). Agglomeration of food powder and applications. Crit. Rev. Food Sci. Nutr. 51, 432–441. doi: 10.1080/10408391003646270
Ding, Q., Ainsworth, P., Tucker, G., and Marson, H. (2005). The effect of extrusion conditions on the physicochemical properties and sensory characteristics of rice based expanded snacks. J. Food Eng. 66, 283–289. doi: 10.1016/j.jfoodeng.2004.03.019
Dogan, H., and Karwe, M. (2003). Physicochemical properties of quinoa Extrudates. Food Sci. Technol. Int. 9, 101–114. doi: 10.1177/1082013203009002006
Egas, L., Villacrés, E., García, E., and Ruiloval, M. (2010). Elaboración de un cereal para desayuno con base a quinua (Chenopodium quinoa) expandida. Rev. Tecnol. 23, 9–15.
Eke, J., Ojimadu, A., Wordu, J., and Ofoedu, C. (2021). Functional properties of complementary food from millet (Pennisetum glaucum), African yam bean (Sphenostylis stenocarpa), and jackfruit (Artocarpus heterophyllus) flour blends: a comparative study. Asian Food Sci. J. 20, 45–62. doi: 10.9734/afsj/2021/v20i930342
Fernandes, M., Wang, S., Ascheri, J., Oliveira, M., and Costa, S. (2003). Efeito da temperatura de extrusão na absorção de água, solubilidade e dispersibilidade da farinha précozida de milho-soja (70,30). Food Sci. Technol. 23, 234–239. doi: 10.1590/S0101-20612003000200023
Filli, K., Nkama, I., Jideani, V., and Ibok, I. (2013). Effect of process variables on the hydration properties and acceptability of extruded millet-soybean blends for fura manufacture. Br. Food J. 115, 884–898. doi: 10.1108/BFJ-May-2010-0091
Fitzpatrick, J., Lauwe, A., Coursol, M., Brien, A., Fitzpatrick, K., Ji, J., et al. (2016). Investigation of the rehydration behaviour of food powders by comparing the behaviour of twelve powders with different properties. Powder Technol. 297, 340–348. doi: 10.1016/j.powtec.2016.04.036
Fournaise, T., Petit, J., and Gaiani, C. (2021). Main powder physicochemical characteristics in fluencing their reconstitution behavior. Powder Technol. 383, 65–73. doi: 10.1016/j.powtec.2021.01.056
Gandhi, N., Singh, B., Sharma, S., and Kapoor, S. (2018). Extrusion process optimization of corn starch to develop instant vegetable soup mix. Int. J. Curr. Microbiol. Appl. Sci. 7, 2886–2910. doi: 10.20546/ijcmas.2018.702.352
Garcia, D., Agama, E., Nuñez, M., Alvarez, J., and Bello, L. (2021). Extrusion pregelatinization improves texture, viscoelasticity and in vitro starch digestibility of mango and amaranth flours. J. Funct. Foods 80, 104441–104411. doi: 10.1016/j.jff.2021.104441
Garcia, D., Bello, L., Flores, P., Agama, E., and Tovar, J. (2019). Extruded unripe plantain flour as an indigestible carbohydrate-rich ingredient. Front. Nutr. 6:2. doi: 10.3389/fnut.2019.00002
Gborie, S., Mugabi, R., and Byaruhanga, Y. (2022). Effect of Extrusion on the Functional and Pasting Properties of High-quality Cassava Flour (HQCF). J. Food Res. 11, 1–12. doi: 10.5539/jfr.v11n4p1
Ge, F., Sun, Y., Yang, C., Cheng, W., Wang, Z., Xia, X., et al. (2024). Exploring the relationship between starch structure and physicochemical properties: the impact of extrusion on highland barley flour. Food Res. Int. 183, 114226–114212. doi: 10.1016/j.foodres.2024.114226
Ghumman, A., Kaur, A., Singh, N., and Singh, B. (2016). Effect of feed moisture and extrusion temperature on protein digestibility and extrusion behaviour of lentil and horsegram. LWT Food Sci. Technol. 70, 349–357. doi: 10.1016/j.lwt.2016.02.032
Giraldo, G., Rodríguez, S., and Sanabria, N. (2019). Preparation of instant green banana flour powders by an extrusion process. Powder Technol. 353, 437–443. doi: 10.1016/j.powtec.2019.05.050
González, R., Carrara, C., Tosi, E., Anon, M., and Pilosof, A. (2007). Amaranth starch rich fraction properties modified by extrusion and fluidized bed heating. LWT Food Sci. Technol. 40, 136–143. doi: 10.1016/j.lwt.2005.09.003
Gulati, P., Weier, S., Santra, D., Subbiah, J., and Rose, D. (2016). Effects of feed moisture and extruder screw speed and temperature on physical characteristics and antioxidant activity of extruded proso millet (Panicum miliaceum) flour. Int. J. Food Sci. Technol. 51, 114–122. doi: 10.1111/ijfs.12974
Gulzar, B., Hussain, S., Naseer, B., and Naik, H. (2021). Enhancement of resistant starch content in modified rice flour using extrusion technology. Cereal Chem. 98, 634–641. doi: 10.1002/cche.10407
Guy, R. (2001). Extrusion cooking: Technologies and applications. Sawston, UK: Woodhead publishing Limited.
He, C., Zheng, J., Liu, F., Wai, M., Xiong, H., and Zhao, Q. (2020). Fabrication and characterization of oat flour processed by different methods. J. Cereal Sci. 96:103123. doi: 10.1016/j.jcs.2020.103123
Heredia, E., Contreras, M., Perez, E., Oth, S., and Rosa, J. (2019). Assessment of the techno-functionality, starch digestion rates and protein quality of rice flour whey protein instant powders produced in a twin extruder. Int. J. Food Sci. Technol. 55, 878–890. doi: 10.1111/ijfs.14376
Hogekamp, S., and Schubert, H. (2003). Rehydration of food powders. Food Sci. Technol. Int. 9, 223–235. doi: 10.1177/1082013203034938
Jafari, M., Koocheki, A., and Milani, E. (2017). Effect of extrusion cooking on chemical structure, morphology, crystallinity and thermal properties of sorghum flour extrudates. J. Cereal Sci. 75, 324–331. doi: 10.1016/j.jcs.2017.05.005
Kaur, P. (2021). Modification of rice flour through extrusion for its application in beverage processing. Punjab: Punjab Agricultural University.
Kaur, N., Singh, B., and Sharma, S. (2019). Comparison of quality protein maize (QPM) and normal maize with respect to properties of instant porridge. LWT Food Sci. Technol. 99, 291–298. doi: 10.1016/j.lwt.2018.09.070
Kesselly, S., Mugabi, R., and Byaruhanga, Y. (2023). Effect of soaking and extrusion on functional and pasting properties of cowpeas flour. Sci. Afr. 19, e01532–e01538. doi: 10.1016/j.sciaf.2022.e01532
Kowalski, R., Medina, I., Thapa, B., Murphy, K., and Ganjyal, G. (2016). Extrusion processing characteristics of quinoa (Chenopodium quinoa Willd) var. cherry Vanilla. J. Cereal Sci. 70, 91–98. doi: 10.1016/j.jcs.2016.05.024
Lai, S., Zhang, T., Wang, Y., Ouyang, K., Hu, H., Hu, X., et al. (2022). Effects of different extrusion temperatures on physicochemical, rheological and digestion properties of rice flour produced in a pilot-scale extruder. Int. J. Food Sci. Technol. 57, 6773–6784. doi: 10.1111/ijfs.16026
Lee, H., and Yoo, B. (2023). Particule agglomeretion and properties of pregelatinized potato starch powder. Gels 9, 1–14. doi: 10.3390/gels9020093
Li, W., Chen, S., Peng, J., Pan, L., and Tu, K. (2020). Effects of twin-screw extrusion processing variables on physicochemical properties and antioxidant activity of rice incorporated with Agriophyllum squarrosum flour. J. Food Proc. Preserv. 44:14524. doi: 10.1111/jfpp.14524
Ma, H., Liu, M., Liang, Y., Zheng, X., Sun, L., Dang, W., et al. (2022). Grain & oil science and technology research progress on properties of pre-gelatinized starch and its application in wheat fl our products. Grain Oil Sci. Technol. 5, 87–97. doi: 10.1016/j.gaost.2022.01.001
Mahasukhonthachat, K., Sopade, P., and Gidley, M. (2010). Kinetics of starch digestion and functional properties of twin-screw extruded sorghum. J. Cereal Sci. 51, 392–401. doi: 10.1016/j.jcs.2010.02.008
Martínez, M., Calviño, A., Rosell, C., and Gómez, M. (2014a). Effect of different extrusion treatments and particle size distribution on the physicochemical properties of Rice flour. Food Bioprocess Technol. 7, 2657–2665. doi: 10.1007/s11947-014-1252-7
Martínez, M., Rosell, C., and Gómez, M. (2014b). Modification of wheat flour functionality and digestibility through different extrusion conditions. J. Food Eng. 143, 74–79. doi: 10.1016/j.jfoodeng.2014.06.035
Menegassi, B., Pilosof, A. M. R., Arêas, J. A. G., Areas, J. A. G., Arêas, J. A. G., and Areas, J. A. G. (2011). Comparison of properties of native and extruded amaranth (Amaranthus cruentus L. - BRS Alegria) flour. LWT Food Sci. Technol. 44, 1915–1921. doi: 10.1016/j.lwt.2011.04.008
MMR. (2021). Pre-cooked flour market- global industry analysis and forecast (2021–2027) by source, nature, application, and region. Available at: https://www.maximizemarketresearch.com/market-report/global-pre-cooked-flour-market/110997/.
Mohmmed, B., Anusha, S., and Abdullahi, F. (2022). Effect of extrusion cooking on some physical and functional properties of instant porridge from blends of sorghum and groundnut: process optimization. Amsterdam, Netherlands: Elsevier, 250–271.
Mouquet, C., Salvignol, B., Hoan, N., Monvois, J., and Tre, S. (2003). Ability of a ‘“very low cost extruder”’ to produce instant infant flours at a small scale in Vietnam. Food Chem. 82, 249–255. doi: 10.1016/S0308-8146(02)00545-9
Nahemiah, D., Nkama, I., Bada, M., and Gbenyi, D. (2017). Multiple parameter optimization of hydration characteristics and proximate compositions of Rice-soybean extruded foods. Open Access Libr. J. 4, 1–22. doi: 10.4236/oalib.1102930
Nahemiah, D., Nkama, I., and Badau, M. (2016). Application of response surface methodology (RSM) for the production and optimization of extruded instant porridge from broken Rice fractions blended with cowpea. Int. J. Food Nutr. Food Sci. 5, 105–116. doi: 10.11648/j.ijnfs.20160502.13
Nikmaram, N., Ying, S., Koubaa, M., and Zhu, Z. (2017). Effect of extrusion on the anti-nutritional factors of food products: an overview. Food Control 79, 62–73. doi: 10.1016/j.foodcont.2017.03.027
Nyombaire, G., Siddiq, M., and Dolan, K. (2011). Physico-chemical and sensory quality of extruded light red kidney bean (Phaseolus vulgaris L.) porridge. LWT Food Sci. Technol. 44, 1597–1602. doi: 10.1016/j.lwt.2011.02.016
Offiah, V., Kontogiorgos, V., and Falade, K. (2018). Extrusion processing of raw food materials and by- products: a review. Crit. Rev. Food Sci. Nutr. 59, 2979–2998. doi: 10.1080/10408398.2018.1480007
Oliveira, C., Ramírez, J., Piler, C., Hidalgo, D., Araújo, I., Deliza, R., et al. (2019). Impact of extruded sorghum genotypes on the rehydration and sensory properties of soluble beverages and the Brazilian consumers perception of sorghum and cereal beverage using word association. J. Cereal Sci. 89:102793. doi: 10.1016/j.jcs.2019.102793
Otondi, E., Nduko, J., and Omwamba, M. (2020). Physico-chemical properties of extruded cassava-chia seed instant flour. J. Agric. Food Res. 2:100058. doi: 10.1016/j.jafr.2020.100058
Ouyang, J., Fan, K., Li, Q., Wang, F., Li, W., and Su, X. (2024). Mechanism of feed moisture levels in extrusion treatment to improve the instant properties of Chinese yam (Dioscorea opposita Thunb) flour. Food Chem. 431:137056. doi: 10.1016/j.foodchem.2023.137056
Pansawat, N., Jangchud, K., Jangchud, A., Wuttijumnong, P., Saalia, F., Eitenmiller, R., et al. (2008). Effects of extrusion conditions on secondary extrusion variables and physical properties of fish, rice based snacks. LWT Food Sci. Technol. 41, 632–641. doi: 10.1016/j.lwt.2007.05.010
Pasqualone, A., Costantini, M., Labarbuta, R., and Summo, C. (2021). Production of extruded-cooked lentil flours at industrial level: effect of processing conditions on starch gelatinization, dough rheological properties and techno-functional parameters. Food Sci. Technol. 147, 111580–111588. doi: 10.1016/j.lwt.2021.111580
Pathania, S., Singh, B., and Sharma, S. (2013). Development of low cost multipurpose instant mix by extrusion process using RSM. Food Sci. 2, 317–320. doi: 10.36106/IJSR
Patil, R., Berrios, J., Tang, J., and Swanson, B. (2007). Evaluation of methods for expansion properties of legume extrudates. Appl. Eng. Agric. 23, 777–783. doi: 10.13031/2013.24044
Pismag, Y., Polo, M., Hoyos, J., Bravo, J., and Roa, D. (2023). Effect of extrusion cooking on the chemical and nutritional properties of instant flours: a review. F1000Research 12:1356. doi: 10.12688/f1000research.140748.1
Qi, M., Zhang, G., Ren, Z., He, Z., Peng, H., and Zhang, D. (2020). Impact of extrusion temperature on in vitro digestibility and pasting properties of pea flour. Plant Foods Hum. Nutr. 76, 26–30. doi: 10.1007/s11130-020-00869-1
Rodríguez, N., Salazar, M., Ramírez, B., Islas, A., Platt, L., Morales, I., et al. (2015). Effect of malting and Nixtamalization processes on the physicochemical properties of instant extruded corn flour and tortilla quality. Plant Foods Hum. Nutr. 70, 275–280. doi: 10.1007/s11130-015-0490-9
Rubin, C. (2012). “Cereal products” in Ullmann’s encyclopedia of industrial chemistry. ed. C. Rubin (Weinheim: Wiley-VCH Verlag GmbH and Co. KGaA), 639–656.
Ruiz, X., Ruiz, J., Espinoza, R., Gutiérrez, R., Aguilar, E., Zazueta, J., et al. (2022). Use of sesame by-product and optimized extrusion to obtain a functional flour with improved techno-functional, nutritional and antioxidant properties Resumen Introducción. Acta Univ. 32, 1–20. doi: 10.15174/au.2022.3494
Sarawong, C., Schoenlechner, R., Sekiguchi, K., Berghofer, E., and Ng, P. (2014). Effect of extrusion cooking on the physicochemical properties, resistant starch, phenolic content and antioxidant capacities of green banana flour. Food Chem. 143, 33–39. doi: 10.1016/j.foodchem.2013.07.081
Shan, S., Sulaiman, R., Sanny, M., and Hanani, N. (2015). Effect of extrusion barrel temperatures on residence time and physical properties of various flour extrudates. Int. Food Res. J. 22, 965–972.
Sotelo, L., Igual, M., Martínez, J., and García, P. (2023). Techno functional properties of corn flour with cowpea (Vigna unguilata) powders obtained by extrusion. Food Secur. 12, 1–14. doi: 10.3390/foods12020298
Tanhehco, E., and Ng, P. (2005). The effects of extrusion cooking and milling on the instant properties of wheat powders. Food Sci. Biotechnol. 14, 758–765.
Transparency, M. R. (2019b). Pregelatinized flour market. Global Industry Analysis, Size, Share, Growth, Trends, and Forecast 2019–2028.
Wang, S., Nickerson, M., Niefer, S., and Nickerson, M. (2019). Effect of barrel temperature and feed moisture on the physical properties of chickpea, sorghum, and maize extrudates and the functionality of their resultant flours part 1. AACCI Cereals and Grains Association, February, pp. 609–620.
Wang, H., Wu, J., Luo, S., Zou, P., Guo, B., Liu, Y., et al. (2020). Improving instant properties of kudzu powder by extrusion treatment and its related mechanism. Food Hydrocoll. 101, 105475–105478. doi: 10.1016/j.foodhyd.2019.105475
Wiriyawattana, P., Suwonsichon, S., and Suwonsichon, T. (2018). Effects of drum drying on physical and antioxidant properties of riceberry flour. Agric. Nat. Resour. 52, 445–450. doi: 10.1016/j.anres.2018.11.008
Yu, L., Ramaswamy, H., and Boye, J. (2012). Twin screw extrusion of corn flour and soy protein isolate (SPI) blends: a response surface analysis. Food Bioprocess Technol. 5, 485–497. doi: 10.1007/s11947-009-0294-8
Yu, L., Ramaswamy, H., and Boye, J. (2013). Protein rich extruded products prepared from soy protein isolate-corn flour blends. LWT 50, 279–289. doi: 10.1016/j.lwt.2012.05.012
Zhang, X., Chen, Y., Zhang, R., Zhong, Y., Luo, Y., Xu, S., et al. (2016). Effects of extrusion treatment on physicochemical properties and in vitro digestion of pregelatinized high amylose maize flour. J. Cereal Sci. 68, 108–115. doi: 10.1016/j.jcs.2016.01.005
Zhang, Z., Zhu, M., Xing, B., Liang, Y., Zou, L., and Li, M. (2023). Effects of extrusion on structural properties, physicochemical properties and in vitro starch digestibility of Tartary buckwheat flour. Food Hydrocoll. 135:108197. doi: 10.1016/j.foodhyd.2022.108197
Keywords: precooked flour, process parameters, physical properties, instant flour, drum dry, spray dry
Citation: Pismag RY, Rivera JD, Hoyos JL, Bravo JE and Roa DF (2024) Effect of extrusion cooking on physical and thermal properties of instant flours: a review. Front. Sustain. Food Syst. 8:1398908. doi: 10.3389/fsufs.2024.1398908
Edited by:
Debabandya Mohapatra, Indian Council of Agricultural Research, IndiaReviewed by:
Farhana Allai, Islamic University of Science and Technology, IndiaChanthima Phungamngoen, Khon Kaen University, Thailand
Copyright © 2024 Pismag, Rivera, Hoyos, Bravo and Roa. This is an open-access article distributed under the terms of the Creative Commons Attribution License (CC BY). The use, distribution or reproduction in other forums is permitted, provided the original author(s) and the copyright owner(s) are credited and that the original publication in this journal is cited, in accordance with accepted academic practice. No use, distribution or reproduction is permitted which does not comply with these terms.
*Correspondence: Diego Fernando Roa, ZHJvYUB1bmljYXVjYS5lZHUuY28=