- 1Faculty of Agriculture, The University of Zagreb, Zagreb, Croatia
- 2UWA School of Agriculture and Environment, The University of Western Australia, Perth, WA, Australia
- 3Institute for Adriatic Crops and Karst Reclamation, Split, Croatia
Global food security (GFS) is challenged by increasing food demand due to population growth and climate change. International trade and globalization have underpinned GFS until the most recent public health, geopolitical and economic crises, when virtually overnight, the focus of governments has shifted towards domestically and locally produced foods. However, the agri-food sector has limited flexibility, and relatively long periods are needed for fundamental/sustainable changes. One of the crucial factors enabling GFS is the efficient water management, but the required knowledge and capabilities are often lacking regionally/locally. We propose the Centers for Optimizing Water Management in Agroecosystems as a long-term solution. The Centers would be the specialized hubs for promotion of research, innovation and technology transfer, raising the knowledge of stakeholders (farmers, extension and government officials, scholars, students, policymakers and other professionals) and their capacities in water management. These Centers would operate as research/education/technology demonstration entities tailored to the specifics of a particular country/region, aiming to address the most important and pertinent goals and outcomes with a high-spatial-resolution outreach. Finally, the Centers will improve farmers’ livelihoods, contribute to sustainable and efficient use of agro-environmental resources, and increase productivity and food quality, ultimately supporting GFS.
The background: an old problem in a new context
The bioeconomy (based primarily on biogenic rather than fossil resources) has become a contemporary and global imperative in the creation and implementation of (inter) national economic strategies and policies. A driving force for the current paradigm is that the bioeconomy can provide sustainable and long-term solutions for some of the most-pressing challenges for humans, notably economic growth and increasing demand for food (Ahmed et al., 2022), water and raw materials (and their recycling to ensure circular bioeconomy), without compromising the managed and native environments (e.g., von Braun, 2018). Global agri-food sector provides more than a billion jobs and contributes 3% to the global GDP, but 20 to >50% of national GDP in developing countries (FAO, 2020). The protection of agri-food systems and environment is the major pillar of the bioeconomy policy agenda (Galanakis et al., 2022; von Braun, 2018), given its important contribution to sustainable development (Ansari et al., 2023) through the creation of new businesses/jobs, opportunities and innovations (e.g., efarm-to-market-to-table food systems).
Global food security is fundamental to human development (Chen et al., 2022), with 12 out of 17 Sustainable Development Goals (SDGs) having direct relationships with agri-food systems (Bartram et al., 2018; FAO, 2022). Contemporary agroecosystems are resource- and energy-intensive, covering ~43% of the world’s ice-free land (excluding deserts); moreover, around 2/3 of freshwater withdrawals are used in irrigated agroecosystems, driving 90 to 95% of global scarcity-weighted water use (Poore and Nemecek, 2018; FAO, 2022). However, global food security is challenged by (i) increasing food demand due to population growth (Sharma et al., 2023; Sakrabani, 2024) and (ii) climate change (Dorward and Giller, 2022; Mbiafeu et al., 2024) (Table 1), both of which have intensified over the last decades and are expected to intensify further by 2050 (Parkinson, 2021; FAO, 2022).
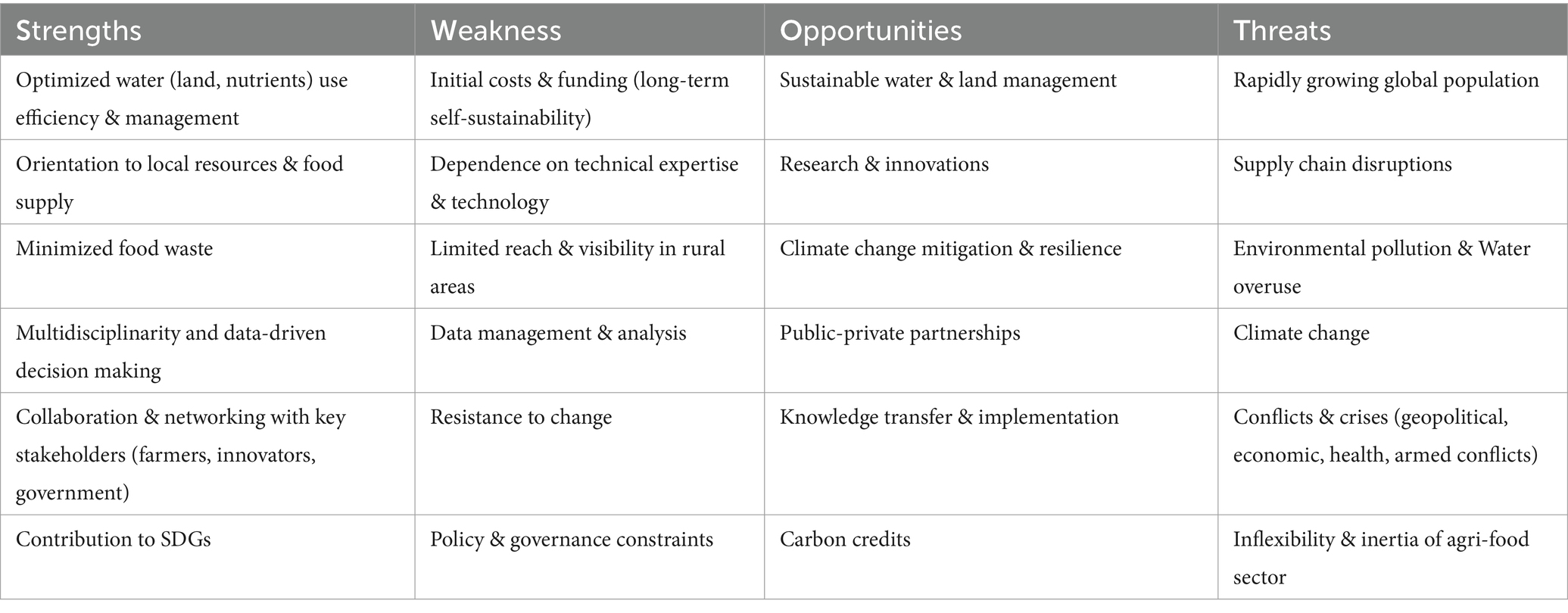
Table 1. SWOT analysis of global food security & proposed solutions – the centers for optimizing water management in agroecosystems.
Climate change impacts principal environmental components (soil, water) underpinning food production, gravely threatening rain-fed agroecosystems and grasslands as the predominant (>80%) global agri-food factories. Namely, conventional rain-fed agroecosystems on 1.21 billion ha (78% of cropland in the world) contribute 60% of the global food supply, whereas the rest of 349 Mha (22%) of cropland are irrigated agroecosystems providing 40% of the global food supply (Ondrasek et al., 2023). Furthermore, as exemplified by the impact of global warming, with ~1°C rise over the last 50-year period, the freshwater supply in many regions has been impacted by the increased severity of precipitation extremes (floods and droughts, causing frequent and pronounced water stresses) and increasing evapotranspiration rates (e.g., by ~10% between 2003 and 2019) (UNESCO, 2022).
The global population has more than tripled from 1950 (2.5 billion) to the present (8+ billion), and projections suggest a global population of almost 10 billion by 2050 as a medium scenario. To meet the increasing needs for food, the production of the most consumed foodstuff by 2050 will need to increase by up to 110% of current levels (Sharma et al., 2023). However, the total number of livestock has more than tripled, from 7.3 billion units in 1970 to 24.2 billion units in 2011 (UNESCO, 2022), and global irrigated areas increased by more than 4-fold, from ~100 Mha in 1950s to ~470 Mha in 2000s (Thenkabail et al., 2009). If we continue with current management practices, water demand will exceed available water supply by 40% by 2030 (FAO, 2022); thus, it is highly unlikely we can continue with such intensive exploitation of water resources, especially given the current global climate change.
Until recently, globalization was the main driving force (via international trade) in ensuring the increased global food (commodities) demands were met (Muscat et al., 2020). However, the most recent health and geopolitical crises, such as the COVID-19 pandemic (Abu-Bakar et al., 2021), Russian invasion of Ukraine (Hellegers, 2022; Shumilova et al., 2023), and escalated Middle East conflicts (Altemur et al., 2024) have shown how fragile the globalism is and how colossal the impacts its fracturing can have on food safety and security (Abu-Bakar et al., 2021; Liera et al., 2023), global financial markets (Altemur et al., 2024), energy, raw materials, and the total environment (Hellegers, 2022; Shumilova et al., 2023). Self-sufficiency and domestically/locally produced foods have rapidly become the high priority for governments.
The interrupted or impeded commodity export (or uncertainty in supply) due to lockdowns and war-caused shortages impacted agri-food markets severely and increased prices (or made them volatile at least) (Dorward and Giller, 2022). Further price increases are expected due to extreme weather events such as floods, droughts and heat waves in Pakistan, Australia, and African and other countries. The recent food and energy crises have prompted governments worldwide to implement a variety of short-term (ad hoc) interventions (Gars et al., 2022; Bergquist et al., 2023). For example, a restriction on agri-food being used as a raw material for bio-energy, temporarily reducing livestock numbers and redirecting animal feed, switching from meat/dairy- to plant-based diet, freezing the prices for basic foodstuffs, and utilization of tax policy instruments (Gars et al., 2022; Sgaravatti et al., 2022). However, agri-food sector is scarcely flexible (Table 1), and relatively long periods are needed for fundamental and sustainable changes to permeate through.
Proposed solution – centers for optimizing water management in agroecosystems
Crop yield quantity and quality are closely related to crop water consumption (Valmassoi and Keller, 2022), whereby increases in water consumption (to a certain extent) are related positively to increases in yield and vice versa (Ondrasek et al., 2014). We argue that significant help in improving water management and promoting and intensifying adoption of sustainable agriculture to underpin global food safety and security can be provided by the Centers for Optimizing Water Management in Agroecosystems (Figure 1; Table 1). These Centers would be the specialized hubs for promotion of research, innovation and technology transfer, raising the knowledge of the stakeholders (farmers, extension and government officials, scholars, students, policy-makers and other professionals) and their capacities in water management (Figure 1). Specifically, each Center would operate as an research/education/technology demonstration entity tailored to the specifics of a particular country or region, aiming to address the most important and pertinent goals and outcomes.
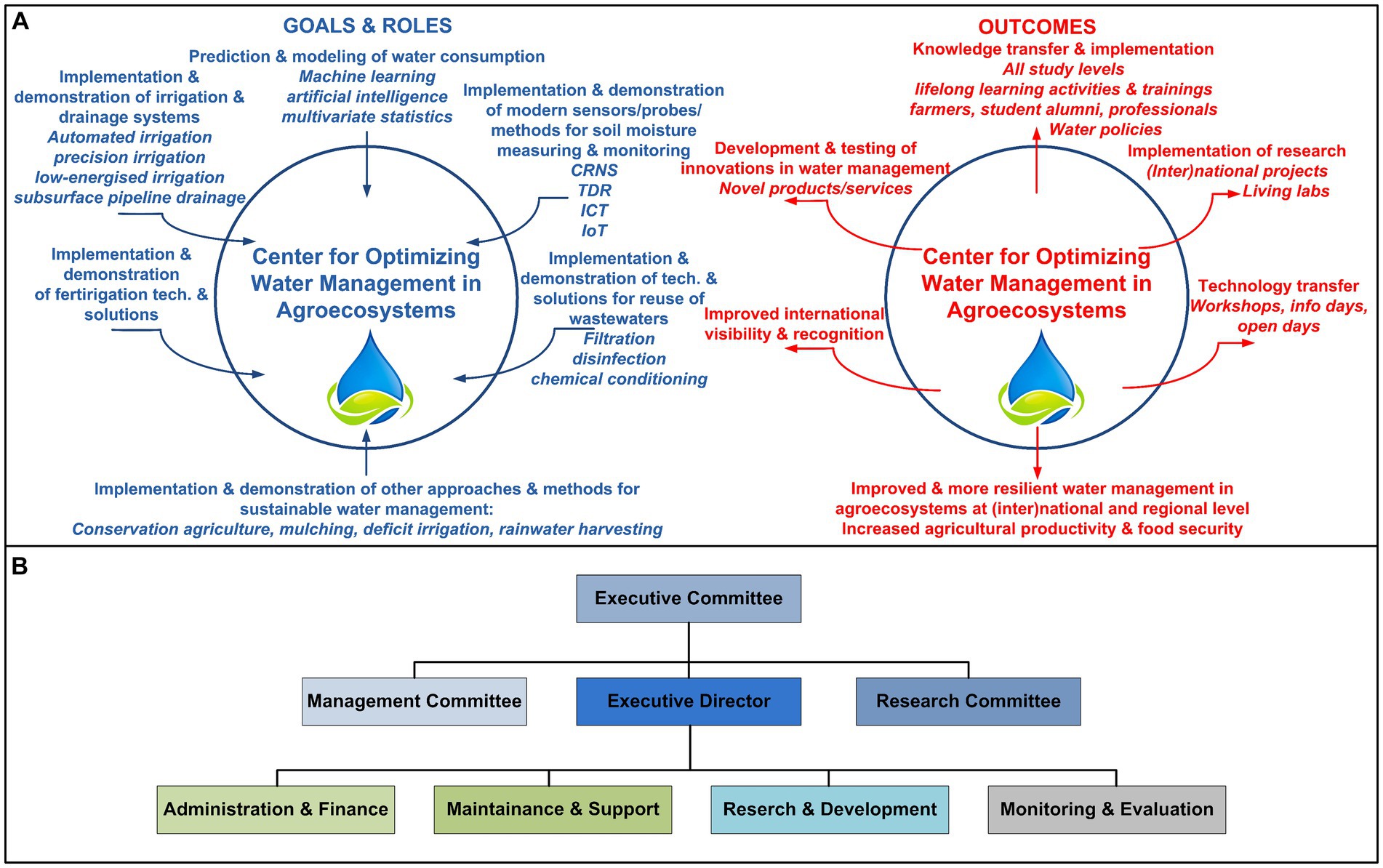
Figure 1. The most important goals, roles, and expected outcomes (A), with a proposed organization structure of the centres for optimizing water management in Agroecosystems (B).
Leveraging Information & Communication Technology (ICT) to foster interactions among particular Center subdomains (Figure 1) is crucial to accomplishing long-term goals and outcomes. For instance, simulation modeling by using machine learning and artificial intelligence (AI) can allow forecasting of crop water requirements in a particular area and the likelihood of droughts and floods (e.g., Gorlapalli et al., 2022; Narita et al., 2023). The AI algorithms can be used to optimize the performance of water treatment facilities and irrigation systems by analyzing real-time data on water quality, flow rates, soil moisture, precipitation, evapotranspiration, etc. Other (agro)environmental abiotic parameters that vary spatially, such as land constrains, water scarcity/quality, biodiversity, conservation/organic farming practices (Ondrasek et al., 2019; Romić et al., 2020), etc., can be some of input model variables.
There are many soil-based approaches to improving water management and increasing water-use efficiency, such as implementation of conservation practices (terracing, rainwater harvesting, crop residues incorporation, reduced tillage), irrigation technique (deficit irrigation, partial root zone drying), moisture monitoring (advanced sensors coupled with Internet of things – IoT) (Ondrasek et al., 2014; Jones et al., 2023), and their applicability and effectiveness in specific agroecosystems would vary in different countries and regions. For instance, IoT coupled with large-scale and real-time data analytics are currently receiving considerable attention (Reljić et al., 2023; Dong et al., 2024) as possible solutions to the issue of global food security. As of 2015, there were ~ 30 million IoT devices deployed in agroecosystems (e.g., for water and soil monitoring programs), a figure that more than doubled to >75 million by 2020 (Sharma et al., 2023).
It is expected that the implementation of IoT, data analytics and AI (Figure 1) will ensure a strong support for smart agriculture, leading to increased operational and water-use efficiency and agri-food productivity. Advanced wireless sensors/networks are used in numerous applications related to smart/precision agriculture (Reljić et al., 2023; Dong et al., 2024), food production, and water management (Rani et al., 2022; Jiang et al., 2023). The data and capacity sharing may empower the testing of extremely complex water scenarios on big spatiotemporal scales; such centralized comprehensive assessments of water management in agri-food sector with a high-spatial resolution outreach is sorely lacking. Moreover, the integration of IoT, data analytics and AI technologies is expected to complement the success of multi-scale data integration methods in smart/precision agriculture, facilitating increased operational efficiency and productivity while enhancing water management across diverse (agro)ecosystems. For example, multi-scale data fusion, incorporating (i) Cosmic Ray Neutron Sensors (CRNS) for in situ soil moisture detection, (ii) land surface models (e.g., ERA5-Land, GLDAS-Noah, GLEAM) and (iii) satellite data (e.g., Sentinel-1 SSM, SCAT/Sentinel-1 SWI, SMAP/Sentinel-1 L2), has been successful in assessing soil moisture across various temporal scales within national German ecosystems (grassland, cropland, forest), with promising global applicability (Schmidt et al., 2024).
One of the main obstacles to the long-term self-sustainability of the Centers could be external funding. To manage this obstacle, the transition from research and innovation to practical applications must be in the core of the Centers mission together with multidisciplinary collaboration to ensure the self-sustainability and national/international visibility and recognition of the Centers (Figure 1; Table 1). One of the successful stories regarding regional-to-international centers is CGIAR (Consultative Group on International Agricultural Research) that has spearheaded agricultural R&D for half a century through its 15 research centers/institutes (e.g., International Water Management Institute – IWMI, Africa Rice Center – ARC, International Center for Agricultural Research in the Dry Areas – ICARDA, etc.), numerous partner organizations, and programs aimed at creating more resilient agroecosystems (Meinke et al., 2023); such activities align to goals, activities and outcomes of the proposed Centers (Figure 1). High-income nations have recognized the importance of R&D (Howard et al., 2021), and in their long-term policies significant funds have been allocated to it; e.g. in the United States, National Science Foundation supports research and education currently with an $8.8 billion annual budget, and the EU €95+ billion were allocated for research and innovation in just one program (Horizon Europe 2021–2027).
Acknowledging public-private partnerships (PPPs), as well as the self-interest and investment of countries, can significantly contribute to overcoming funding hurdles and enhance the sustainability, effectiveness, and impact of the proposed Centers (Table 1). PPPs are recognized as potential solutions for governments, aiming to maximize value for money and finance the necessary investments for infrastructure provision and public service management, particularly in the water sector (Lima et al., 2021). Moreover, there has been a notable increase in studding of the PPP phenomenon in the water sectors of Asia, Europe, and Africa over the past decade (Lima et al., 2021). Recent findings underscore a significant correlation within the Water-Energy-Food (WEF) nexus framework, indicating that countries with superior WEF governance tend to excel in enhancing WEF accessibility, emphasizing the crucial role of governance within the WEF nexus security framework (He et al., 2024). Evidence suggests that countries’ self-interests and investments in the Centers could an enable the development of local capacity in research, innovation, and management (Cho et al., 2023), allowing them to address specific national priorities (e.g., establishment of the Nitrate Directive) and implement more effective solutions (e.g., reshaping of nitrate-vulnerable zones) (Ondrasek et al., 2021). Additionally, investing in regional centers has been shown to facilitate trans-regional/national collaboration, knowledge sharing (Cho et al., 2023; Torre et al., 2023), pooling of resources, and protection of water resources from pollution/overuse (Ondrasek et al., 2021; Cho et al., 2023). Studies also indicate that countries investing in the Centers demonstrate their commitment to SDGs related to water security, climate resilience and environmental sustainability (e.g., Carvalho and Spataru, 2023; Cho et al., 2023) (Table 1). This commitment can enhance standing of the Centers in the global community and attract additional support and partnerships from international donors, investors and institutions.
The resistance to change and poor adoption of new technologies/practices, high implementation costs, cultural resistance as well as limited outreach to farmers and other stakeholders, especially in developing countries and remote areas, are some of the potential barriers. Next, a wide variety of sensors, with different interfaces and incompatible protocols, poses a challenge in terms of software/hardware footprint and hinders the future growth of IoT systems; moreover, such systems rely on the availability of fast wireless connections that are often deficient or even non-existent in remote rural agricultural areas. Therefore, the efficiency of data providing (e.g., encoding) needs to be improved to ensure that the system can function effectively in real time (Sharma et al., 2023).
To overcome some of other barriers (i) innovative and demonstrated solutions need to be reproducible and scalable (e.g., from small to large farms) and (ii) governments should encourage widespread adoption of demonstrated practices through infrastructural projects in rural areas. Some other top-to-bottom strategies and approaches that can be implemented to overcome the challenges associated with the Centers targets include:
• capacity building, e.g., specific online or onsite programs/workshops for knowledge transfer among farmers and other stakeholders (Figure 1);
• investments in cloud computing through directly transferring data to reduce the development and management costs (Mytton, 2021; Ramesh et al., 2023), and even contribute to carbon neutrality (Cao and Bian, 2021; Parkinson, 2021) (Table 1);
• standardization, development and implementation of industry-wide standards for advanced ICT solutions (e.g., IoT systems; Mytton, 2021) can help ensure interoperability of protocols and interfaces;
• policy and regulation play a crucial role in promoting the adoption and implementation of advanced systemic solutions (Bartram et al., 2018) in agriculture; therefore, governments must ensure that data privacy and security are guaranteed.
Finally, the principal strengths and opportunities that distinguish proposed Centers from existing structures (e.g., IWMI, ARC, ICARDA) include (Table 1; Figure 1):
• focus on optimized water management, whereas existing structures may have broader mandates that encompass various aspects of agriculture, livestock production, food security, and rural development,
• multidisciplinary approach, integrating expertise from diverse areas (agronomy, hydrology, ecology, pedology, statistics, ICT, engineering, etc.) to address complex water challenges comprehensively. In contrast, some existing structures may focus on specific areas like crop improvement (e.g., rice or wheat), livestock or agricultural policy,
• technology & innovation in water management is strongly emphasized, which might not be the case in some existing structures, and.
• close and proactive relationship with water policies and governance. This presents an opportunity for the Centers to actively engage in policy advocacy and institutional capacity building by disseminating knowledge through national/regional pilot irrigation projects, living labs, and other initiatives, aiming to reshape water policies effectively.
Conclusion
Recent health and geopolitical crises have shown the fragility of globalism and the importance of prioritizing self-sufficiency and domestically produced food over international trade. However, relatively long timeframes are required for fundamental and sustainable changes in the agri-food sector. As food production is closely linked to water consumption, more efficient water management is crucial for food safety and security. A possible, long-term solution to promote sustainable agriculture and improve water management is to establish the Centers for Optimizing Water Management in Agroecosystems. These country and/or regional Centers would promote research, innovation and technology transfer among all key stakeholders in water management of agroecosystems, using contemporary approaches (e.g., big-data analytics coupled with machine learning and AI) and techniques (e.g., implementation of IoT, precision and smart irrigation).
Implementation should ensure that Centers are accessible to all stakeholders, especially smallholder farmers who may have limited resources and technical expertise. It is important to tailor the services of the Centers to the specific needs and challenges of each region or country, considering local conditions, cultures and traditions. Collaboration with local governments, research institutions and non-government organizations can help ensure the relevance and effectiveness of the Centers. Funding is a crucial aspect of the Centers sustainability; thus, building public-private partnerships and involving international organizations can help secure the necessary resources for their long-term operations. In addition, alternative funding models such as crowdfunding, PPPs or impact investing could be explored to support the Centers mission and encourage community engagement.
The proposed Centers are distinguished from existing structures by their specialized focus on optimized water management, interdisciplinary approach, emphasis on technology and innovation, and proactive engagement in water policies and governance. Finally, the proposed Centers offer a promising solution to improving food security and promoting sustainability in the agri-food sector. By providing guidance and tools for effective water management, the Centers can help mitigate the impacts of climate change and water scarcity in food production while contributing to broader environmental and social goals.
Data availability statement
The raw data supporting the conclusions of this article will be made available by the authors, without undue reservation.
Author contributions
GO: Conceptualization, Writing – original draft, Writing – review & editing. ZR: Data curation, Writing – review & editing.
Funding
The author(s) declare that financial support was received for the research, authorship, and/or publication of this article. This study has been partly conducted under the project IP-2022-10-7906, founded by Croatian Science Foundation.
Conflict of interest
The authors declare that the research was conducted in the absence of any commercial or financial relationships that could be construed as a potential conflict of interest.
Publisher’s note
All claims expressed in this article are solely those of the authors and do not necessarily represent those of their affiliated organizations, or those of the publisher, the editors and the reviewers. Any product that may be evaluated in this article, or claim that may be made by its manufacturer, is not guaranteed or endorsed by the publisher.
References
Abu-Bakar, H., Williams, L., and Hallett, S. H. (2021). Quantifying the impact of the COVID-19 lockdown on household water consumption patterns in England. NPJ Clean Water 4:13. doi: 10.1038/s41545-021-00103-8
Ahmed, M., Mavukkandy, M. O., Giwa, A., Elektorowicz, M., Katsou, E., Khelifi, O., et al. (2022). Recent developments in hazardous pollutants removal from wastewater and water reuse within a circular economy. NPJ Clean Water 5:12. doi: 10.1038/s41545-022-00154-5
Altemur, N., Eren, B. S., and Karaca, S. S. (2024). The impact of the Hamas-Israel conflict on stock market indices in the middle east and Turkey: an event study analysis. J. Econ. Bus. Issues 4, 77–85.
Ansari, A., Wuryandani, S., Pranesti, A., Telaumbanua, M., Ngadisih, H., Hardiansyah, M. Y., et al. (2023). Optimizing water-energy-food nexus: achieving economic prosperity and environmental sustainability in agriculture. Front. Sustain. Food Syst. 7:1207197. doi: 10.3389/fsufs.2023.1207197
Bartram, J., Brocklehurst, C., Bradley, D., Muller, M., and Evans, B. (2018). Policy review of the means of implementation targets and indicators for the sustainable development goal for water and sanitation. NPJ Clean Water 1:3. doi: 10.1038/s41545-018-0003-0
Bergquist, P., De Roche, G., Lachapelle, E., Mildenberger, M., and Harrison, K. (2023). The politics of intersecting crises: the effect of the COVID-19 pandemic on climate policy preferences. Br. J. Polit. Sci. 53, 707–716. doi: 10.1017/S0007123422000266
Cao, Y., and Bian, Y. (2021). Improving the ecological environmental performance to achieve carbon neutrality: the application of DPSIR-improved matter-element extension cloud model. J. Environ. Manag. 293:112887. doi: 10.1016/j.jenvman.2021.112887
Carvalho, P., and Spataru, C. (2023). Overcoming resource Nexus conflicts with a normative-institutional approach: a case study of Brazil. Front. Water 3:747208. doi: 10.3389/frwa.2021.747208
Chen, C., Chaudhary, A., and Mathys, A. (2022). Dietary change and global sustainable development goals. Front. Sustain. Food Syst. 6:771041. doi: 10.3389/fsufs.2022.771041
Cho, S. J., Klemz, C., Barreto, S., Raepple, J., Bracale, H., Acosta, E. A., et al. (2023). Collaborative watershed modeling as stakeholder engagement tool for science-based water policy assessment in São Paulo, Brazil. Water 15:401. doi: 10.3390/w15030401
Dong, Y., Werling, B., Cao, Z., and Li, G. (2024). Implementation of an in-field IoT system for precision irrigation management. Front. Water 6:1353597. doi: 10.3389/frwa.2024.1353597
Dorward, A., and Giller, K. E. (2022). Change in the climate and other factors affecting agriculture, food or poverty: an opportunity, a threat or both? A personal perspective. Glob. Food Sec. 33:100623. doi: 10.1016/j.gfs.2022.100623
FAO (2022). The state of the World’s land and water resources for food and agriculture 2021 – systems at breaking point. Rome, Italy: FAO.
Galanakis, C. M, Brunori, G., Chiaramonti, D., Matthews, R., Panoutsou, C., and Fritsche, U. R. (2022). Bioeconomy and green recovery in a post-COVID-19 era. Sci. Total Environ. 808:152180. doi: 10.1016/j.scitotenv.2021.152180
Gars, J., Spiro, D., and Wachtmeister, H. (2022). The effect of European fuel-tax cuts on the oil income of Russia. Nat. Energy 7, 989–997. doi: 10.1038/s41560-022-01122-6
Gorlapalli, A., Kallakuri, S., Sreekanth, P. D., Patil, R., Bandumula, N., Ondrasek, G., et al. (2022). Characterization and prediction of water stress using time series and artificial intelligence models. Sustain. For. 14:6690. doi: 10.3390/su14116690
He, B., Zheng, H., and Guan, Q. (2024). Toward revolutionizing water-energy-food nexus composite index model: from availability, accessibility, and governance. Front. Water 6:1338534. doi: 10.3389/frwa.2024.1338534
Hellegers, P. (2022). Food security vulnerability due to trade dependencies on Russia and Ukraine. Food Secur. 14, 1503–1510. doi: 10.1007/s12571-022-01306-8
Howard, G., Nijhawan, A., Flint, A., Baidya, M., Pregnolato, M., Ghimire, A., et al. (2021). The how tough is WASH framework for assessing the climate resilience of water and sanitation. NPJ Clean Water 4:39. doi: 10.1038/s41545-021-00130-5
Jiang, J., Men, Y., Pang, T., Tang, S., Hou, Z., Luo, M., et al. (2023). An integrated supervision framework to safeguard the urban river water quality supported by ICT and models. J. Environ. Manag. 331:117245. doi: 10.1016/j.jenvman.2023.117245
Jones, K., Nowak, A., Berglund, E., Grinnell, W., Temu, E., Paul, B., et al. (2023). Evidence supports the potential for climate-smart agriculture in Tanzania. Glob. Food Sec. 36:100666. doi: 10.1016/j.gfs.2022.100666
Liera, C., Dickin, S., Rishworth, A., Bisung, E., Moreno, A., and Elliott, S. J. (2023). Human rights, COVID-19, and barriers to safe water and sanitation among people experiencing homelessness in Mexico City. Front. Water 5:1054182. doi: 10.3389/frwa.2023.1054182
Lima, S., Brochado, A., and Marques, R. C. (2021). Public-private partnerships in the water sector: a review. Util. Policy 69:101182. doi: 10.1016/j.jup.2021.101182
Mbiafeu, M. F., Molua, E. L., Sotamenou, J., and Ndip, F. E. (2024). Climate, agroecology, and farm returns: differential impacts with implications for agricultural progress in the face of climate change. Front. Sustain. Food Syst. 8:1322568. doi: 10.3389/fsufs.2024.1322568
Meinke, H., Ash, A., Barrett, C. B., Smith, A. G., Graff Zivin, J. S., Abera, F., et al. (2023). Evolution of the one CGIAR’s research and innovation portfolio to 2030: approaches, tools, and insights after the reform. NPJ Sustain Agric. 1:6. doi: 10.1038/s44264-023-00005-x
Muscat, A., de Olde, E. M., de Boer, I. J. M., and Ripoll-Bosch, R. (2020). The battle for biomass: a systematic review of food-feed-fuel competition. Glob. Food Sec. 25:100330. doi: 10.1016/j.gfs.2019.100330
Mytton, D. (2021). Data Centre water consumption. NPJ Clean Water 4:11. doi: 10.1038/s41545-021-00101-w
Narita, K., Matsui, Y., Matsushita, T., and Shirasaki, N. (2023). Screening priority pesticides for drinking water quality regulation and monitoring by machine learning: analysis of factors affecting detectability. J. Environ. Manag. 326:116738. doi: 10.1016/j.jenvman.2022.116738
Ondrasek, G., Bakić Begić, H., Romić, D., Brkić, Ž., Husnjak, S., and Bubalo Kovačić, M. (2021). A novel LUMNAqSoP approach for prioritising groundwater monitoring stations for implementation of the nitrates directive. Environ. Sci. Eur. 33:23. doi: 10.1186/s12302-021-00467-1
Ondrasek, G., Bakić Begić, H., Zovko, M., Filipović, L., Meriño-Gergichevich, C., Savić, R., et al. (2019). Biogeochemistry of soil organic matter in agroecosystems & environmental implications. Sci. Total Environ. 658, 1559–1573. doi: 10.1016/j.scitotenv.2018.12.243
Ondrasek, G., Horvatinec, J., Kovačić, M. B., Reljić, M., Vinceković, M., Rathod, S., et al. (2023). Land resources in organic agriculture: trends and challenges in the twenty-first century from global to Croatian contexts. Agronomy 13:1544. doi: 10.3390/agronomy13061544
Ondrasek, G., Rengel, Z., Petosic, D., and Filipovic, V. (2014). “Land and Water Management Strategies for the Improvement of Crop Production” in Emerging Technologies and Management of Crop Stress Tolerance. eds. P. Ahmad and S. Rasool (Cambridge, MA: Academic Press), 291–313.
Parkinson, S. (2021). Guiding urban water management towards 1.5 °C. NPJ Clean Water 4:34. doi: 10.1038/s41545-021-00126-1
Poore, J., and Nemecek, T. (2018). Reducing food’s environmental impacts through producers and consumers. Science 360, 987–992. doi: 10.1126/science.aaq0216
Ramesh, D., Mishra, R., Atrey, P. K., Edla, D. R., Misra, S., and Qi, L. (2023). Blockchain based efficient tamper-proof EHR storage for decentralized cloud-assisted storage. Alex. Eng. J. 68, 205–226. doi: 10.1016/j.aej.2023.01.012
Rani, A., Snyder, S. W., Kim, H., Lei, Z., and Pan, S.-Y. (2022). Pathways to a net-zero-carbon water sector through energy-extracting wastewater technologies. NPJ Clean Water 5:49. doi: 10.1038/s41545-022-00197-8
Reljić, M., Romić, M., Romić, D., Gilja, G., Mornar, V., Ondrasek, G., et al. (2023). Advanced continuous monitoring System & Tools for water resource management and decision support system in salt Affected Delta. Agriculture 13:369. doi: 10.3390/agriculture13020369
Romić, D., Castrignanò, A., Romić, M., Buttafuoco, G., Bubalo Kovačić, M., Ondrašek, G., et al. (2020). Modelling spatial and temporal variability of water quality from different monitoring stations using mixed effects model theory. Sci. Total Environ. 704:135875. doi: 10.1016/j.scitotenv.2019.135875
Sakrabani, R. (2024). Opportunities and challenges organo-mineral fertiliser can play in enabling food security. Front. Sustain. Food Syst. 8:1296351. doi: 10.3389/fsufs.2024.1296351
Schmidt, T., Schrön, M., Li, Z., Francke, T., Zacharias, S., Hildebrandt, A., et al. (2024). Comprehensive quality assessment of satellite- and model-based soil moisture products against the COSMOS network in Germany. Remote Sens. Environ. 301:113930. doi: 10.1016/j.rse.2023.113930
Sharma, R. P., Dharavath, R., and Edla, D. R. (2023). IoFT-FIS: internet of farm things based prediction for crop pest infestation using optimized fuzzy inference system. Internet Things 21:100658. doi: 10.1016/j.iot.2022.100658
Shumilova, O., Tockner, K., Sukhodolov, A., Khilchevskyi, V., De Meester, L., Stepanenko, S., et al. (2023). Impact of the Russia–Ukraine armed conflict on water resources and water infrastructure. Nat. Sustain. doi: 10.1038/s41893-023-01068-x
Sgaravatti, G., Tagliapietra, S., Trasi, C., and Zachmann, Z. (2021). ‘National policies to shield consumers from rising energy prices’, Bruegel Datasets Available at: https://www.bruegel.org/dataset/national-policies-shield-consumers-rising-energy-prices (Accessed February 20, 2024)
Thenkabail, P. S., Biradar, C. M., Noojipady, P., Dheeravath, V., Li, Y., Velpuri, M., et al. (2009). Global irrigated area map (GIAM), derived from remote sensing, for the end of the last millennium. Int. J. Remote Sens. 30, 3679–3733. doi: 10.1080/01431160802698919
Torre, A., Wallet, F., and Huang, J. (2023). A collaborative and multidisciplinary approach to knowledge-based rural development: 25 years of the PSDR program in France. J. Rural. Stud. 97, 428–437. doi: 10.1016/j.jrurstud.2022.12.034
UNESCO (2022). The United Nations world water development report 2022: groundwater: making the invisible visible title. Available at: https://unesdoc.unesco.org/search/N-EXPLORE-c44ef867-a60d-46c5-8d15-0fc0ff4c1214
Valmassoi, A., and Keller, J. D. (2022). A review on irrigation parameterizations in earth system models. Front. Water 4:906664. doi: 10.3389/frwa.2022.906664
Keywords: food security, food safety, sustainable water management, demonstration center, climate change, global population
Citation: Ondrasek G and Rengel Z (2024) Centers for optimizing water management in agroecosystems & global food security. Front. Sustain. Food Syst. 8:1398454. doi: 10.3389/fsufs.2024.1398454
Edited by:
Richard Graham Taylor, University College London, United KingdomReviewed by:
La Zhuo, Northwest A&F University, ChinaCopyright © 2024 Ondrasek and Rengel. This is an open-access article distributed under the terms of the Creative Commons Attribution License (CC BY). The use, distribution or reproduction in other forums is permitted, provided the original author(s) and the copyright owner(s) are credited and that the original publication in this journal is cited, in accordance with accepted academic practice. No use, distribution or reproduction is permitted which does not comply with these terms.
*Correspondence: Gabrijel Ondrasek, gondrasek@agr.hr