- 1IRCAF (Invernizzi Reference Centre on Agri-Food), Università Cattolica del Sacro Cuore, Cremona, Italy
- 2Department of Biosciences and Pediatric Clinical Research Center Romeo ed Enrica Invernizzi, University of Milan, Milan, Italy
- 3Department of Veterinary Medical Sciences, University of Parma, Parma, Italy
- 4Department of Veterinary Medicine and Animal Sciences, University of Milan, Milan, Italy
Introduction: A common thought is that extensive and organic breeding systems are associated with lower prevalence of infections in livestock animals, compared to intensive ones. In addition, organic systems limit the use of anti-microbial drugs, which may lead to lower emergence of antimicrobial resistances (AMR).
Methods: To examine these issues, avoiding any a priori bias, we carried out a systematic literature search on dairy cattle breeding. Search was targeted to publications that compared different types of livestock farming (intensive, extensive, conventional, organic) in terms of the circulation of infectious diseases and AMR.
Results and discussion: A total of 101 papers were finally selected. These papers did not show any trend in the circulation of the infections in the four types of breeding systems. However, AMR was more prevalent on conventional dairy farms compared to organic ones. The prevalence of specific pathogens and types of resistances were frequently associated with specific risk factors that were not strictly related to the type of farming system. In conclusion, we did not find any evidence suggesting that extensive and organic dairy farming bears any advantage over the intensive and conventional ones, in terms of the circulation of infectious agents.
1 Introduction
It is a common view that intensive livestock farming facilitates the circulation of infectious agents within herds, possibly facilitating spill-over events of infections from animals to humans and the diffusion of emerging infectious diseases (EID) (Bartlett et al., 2022). Factors that are expected to facilitate the circulation of infections in intensive management are: high density and numerosity of animals; movement of animals (or animal-derived products) to food-processing industries (or markets); sub-optimal animal welfare conditions (Bellet et al., 2021). However, this view has been called into question, at least for some types of farming management systems (FMS) (Temple and Manteca, 2020; Bartlett et al., 2022). In addition, reliable opinions on this topic requires that intensive and extensive FMS are precisely defined (Bartlett et al., 2022). Intensive animal farming typically ensures a higher production per unit area (Bartlett et al., 2022). Intensive farming is thus expected to reduce the extension of the land used to support animal breeding, for a given amount of produce (e.g., milk, meat, eggs). Furthermore, intensive livestock farming is frequently based on large herds, with animals placed in dense aggregations, as opposed to extensive farming in which herds are generally small, and animals not over crowded (Gilbert et al., 2021). Therefore, according to Bartlett et al. (2022), but differently from other views (e.g., Gilbert et al., 2021), extensive farming increases the risk of circulation of infectious agents, since it is associated with the fragmentation of farming into small holdings, with higher number of animals in a given area, in order to obtain the same production that is achieved in intensive farming with fewer animals. The fragmentation of the farming system may increase contact with wildlife and the sylvatic environment. Thus, while it has been suggested that intensive farming is associated with increased circulation of infectious agents, an alternative hypothesis is that extensive breeding is more likely to favor infections in livestock. This issue can also regard organic farming, defined by specific requirements that differ among countries, and which shares some features with extensive systems, such as the space available to animals or the fact that high production yield is generally not a primary goal in either system. In addition, extensive farming is generally assumed to be associated with a high level of animal welfare, similar to organic farming (Hemsworth et al., 1995; Wagner et al., 2021). In turn, animal welfare is thought to be associated with increased resistance to infectious agents (von Keyserlingk et al., 2009).
Along with the scientific literature, non-specialist publications (e.g., news publications, magazines and web sites devoted to scientific dissemination) have also discussed the “pros” and “cons” of intensive and conventional farming, at times in the absence of solid scientific evidence. The issue is thus well suited to be addressed through systematic analyses of the literature. A systematic review implies that search keys and inclusion and exclusion criteria are defined a priori, in order to obtain unbiased retrieval of relevant publications. Systematic reviews are frequently performed according to the PRISMA protocol (Page et al., 2021). The resulting scientific literature is then examined to build a general, unbiased view of the topic, rather than an “advocacy publication.”
In this systematic review we focused on dairy cattle farming, to evaluate the current knowledge on the circulation of infectious agents and the diffusion of antimicrobial resistance in relation to the type of FMS. We specifically defined the search keys to retrieve publications in which different FMS (intensive and extensive; conventional and organic) have directly been compared. In order to retrieve as many articles as possible in which FMS had been compared in relation to the circulation of pathogens, we used general terms referring to infections or infectious agents (see search strings in Materials and methods). Furthermore, we reasoned that studies on antimicrobial and antiparasitic drugs could also provide information on the prevalence or incidence of infections or infectious agents. We have therefore also included terms related to these issues. Then, since the terms in the search strings were suited to also retrieve documents related to antimicrobial resistance, and not only those related to infections or infectious agents per se, we decided to conduct an analysis of the retrieved documents also in relation to antimicrobial resistance, but limiting our analysis to antimicrobial resistance in bacterial pathogens. We emphasize that the circulation of infectious agents in herds, in terms of both acute and chronic infections, may impact on the quality of animal-derived foods. Therefore, a systematic review, or meta-analysis, to determine whether the prevalence of infections in herd is influenced by the type of management systems might also provide indirect information on the quality of food production, and, in this specific case, quality of milk and milk-derived products, as recently emphasized by the Food and Agriculture Organization of the United Nations (FAO, 2023). The present meta-analysis is not specifically addressed to livestock veterinary practitioners and stakeholders of milk production chain, whose interest is likely focused on specific risk factors associated with cattle infections. Rather, the aim is to target those “non-specialist” readers such as journalists, science communicators, and politicians, who have the responsibility to inform the general public, orienting (and taking) decisions on laws and regulations. Indeed, the issue of intensive and extensive production (or conventional and organic) is hotly debated in governmental institutions (e.g., European Parliament Research Service, 2016), non-governmental organizations (e.g., Ferma gli Allevamenti Intensivi, 2024), and newspapers (e.g., Chang, 2024). In this context, the public deserves information based on unbiased reports to prevent the creation and circulation of ideological positions. The purpose of this article is thus to respond to this need for information, with a search on the scientific literature that is not biased by preconceptions.
2 Methods
In this study, we followed the PRISMA guidelines (Page et al., 2021) and carried out the bibliographic search on the Scopus database (search day: 4/3/2023). Literature retrieval strategy consisted in the search for publications in which dairy cattle management systems have been compared. Specifically, the type of searched comparisons was as follows: intensive VS extensive (IvsE) or conventional VS organic (CvsO). The different types of management systems were compared in relation to the following: (1) infectious agents (IA) or infectious diseases (ID); (2) antimicrobial resistance/susceptibility (AMR/AMS). The search terms were selected according to synonyms present in the literature. In addition, we assumed that publications focused on anti-parasitic and antimicrobial drug use (AMU) might provide results relevant to the issues of IA/ID and AMR/AMS, and we thus included terms related with the use of these drugs (even though the specific issue of AMU was out of the scope of this study). The following search terms and Boolean operators were used; the two query strings are reported below. Two query strings were used rather than combining all search terms into a single string in order to obtain two separate outputs for IvsE and CvsO.
((TITLE-ABS-KEY (intensive*) AND TITLE-ABS-KEY (cow) OR TITLE-ABS-KEY (cattle) AND TITLE-ABS-KEY (milk) OR TITLE-ABS-KEY (dairy) OR TITLE-ABS-KEY (cheese) AND TITLE-ABS-KEY (infect*) OR TITLE-ABS-KEY (parasit*) OR TITLE-ABS-KEY (pathogen*) OR TITLE-ABS-KEY (zoono*) OR TITLE-ABS-KEY (microb*) OR TITLE-ABS-KEY (vir*) OR TITLE-ABS-KEY (protozo*) OR TITLE-ABS-KEY (mico*) OR TITLE-ABS-KEY (fungi*) OR TITLE-ABS-KEY (nematod*) OR TITLE-ABS-KEY (helmint*) OR TITLE-ABS-KEY (antibiotic*) OR TITLE-ABS-KEY (antimicrobial*) OR TITLE-ABS-KEY (amr) OR TITLE-ABS-KEY (antiparasit*) OR TITLE-ABS-KEY (drug*) AND TITLE-ABS-KEY (extensive*) OR TITLE-ABS-KEY (pastur*) OR TITLE-ABS-KEY (graz*)))
((TITLE-ABS-KEY (conventional*) AND TITLE-ABS-KEY (cow) OR TITLE-ABS-KEY (cattle) AND TITLE-ABS-KEY (milk) OR TITLE-ABS-KEY (dairy) OR TITLE-ABS-KEY (cheese) AND TITLE-ABS-KEY (infect*) OR TITLE-ABS-KEY (parasit*) OR TITLE-ABS-KEY (pathogen*) OR TITLE-ABS-KEY (zoono*) OR TITLE-ABS-KEY (microb*) OR TITLE-ABS-KEY (vir*) OR TITLE-ABS-KEY (protozo*) OR TITLE-ABS-KEY (mico*) OR TITLE-ABS-KEY (fungi*) OR TITLE-ABS-KEY (nematod*) OR TITLE-ABS-KEY (helmint*) OR TITLE-ABS-KEY (antibiotic*) OR TITLE-ABS-KEY (antimicrobial*) OR TITLE-ABS-KEY (amr) OR TITLE-ABS-KEY (antiparasit*) OR TITLE-ABS-KEY (drug*) AND TITLE-ABS-KEY (organic*)))
Outputs generated by the two query strings were subjected to the same eligibility criteria and the same PRISMA procedure. The inclusion criteria were: (1) a publication must be an original study, in which conventional management was compared to organic management (CvsO), or intensive management was compared to extensive management (IvsE), of dairy cattle farms; (2) results must refer to: the presence, prevalence, or incidence of an infectious agent or infectious disease; the use of antimicrobials; the presence of drug-resistant (AMR) or drug-susceptible (AMS) microbes or parasites (where “drug” is intended as an antimicrobial product).
The exclusion criteria were as follows: publications classified as reviews, or other types of secondary studies such as book chapters, conference publications, notes and incomplete text articles. The full texts of all potentially relevant studies were downloaded in their entirety. A further round of screening was applied through careful reading of the title and abstracts. In particular, the reading of the title and abstract was carried out by three of the authors, independently. After a first screening of retrieved paper through the automatic exclusion tool provided by the Scopus platform, each of the three authors had to fill a table composed of five columns and 286 lines (plus the title line). Column 1 was used to list the 286 papers that passed the automatic selection. Columns 2 and 3 were to be flagged, to indicate whether a given paper was to be included or excluded, based on the eligibility criteria. Column 4 was to be flagged to indicate uncertain situations. Column 5 was used to indicate the reason(s) for the exclusion. At the end of this process, the three authors worked collegially, to analyze the matching in excluded and included papers, to discuss reasons for the exclusion, and to discuss uncertain cases. This collegial work led also to a precise formulation of the reasons for the exclusion. The process is summarized in the PRISMA flow chart (Figure 1). The reasons for the exclusion of papers, after the collegial work described above, are listed as A-F in the legend to Figure 1.
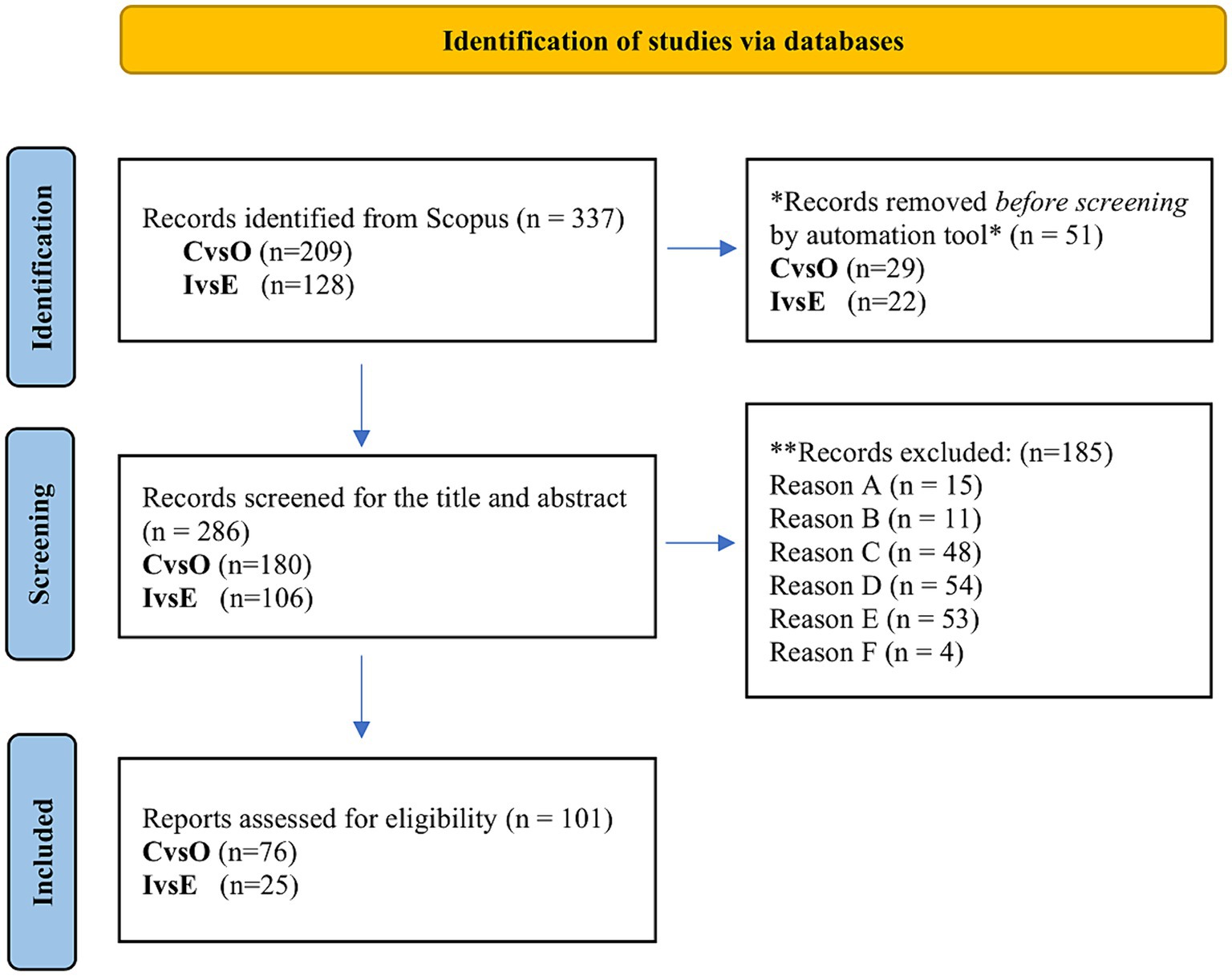
Figure 1. PRISMA flow chart presenting the results of the searching strategy and the exclusion process. *An automatic filter was applied to automatically exclude publications classified as “note,” “conference publications,” “book chapter,” “review.” **A manual operation to identify publications defined “not pertinent” for the follow reasons (A, B, C, D, E, F); A: items focused on the issue of “consumer perception” or “farming policy”; B: items that did not refer to “dairy cow” (e.g., those focused on dairy buffalo or other dairy animals); C: items that did not perform, in the same study, a direct comparison CvsO or IvsE; D: the terms “extensive “intensive” “organic,” “conventional” did not refer to the farming management system; E: items that did not compare CvsO or IvsE according to eligibility criteria no 2; F: publications classified as “note,” “conference publications,” “book chapter,” “review.”
3 Results and discussion
3.1 Overall results
A total of 336 items were retrieved from the Scopus platform. According to pre-defined eligibility criteria, as reported in the PRISMA flow-chart (Figure 1), we finally selected 101 publications, 77 dealing with the comparison CvsO, and 25 with IvsE (one publication addressed both comparisons). We emphasize that part of these publications presented two or more studies, focused on different ID/IA or on both ID/IA and AMR. Therefore, the total number of studies (132) was higher than the number of publications (101). These 132 studies are reported in Tables 1–3, indicated as study numbers Sn1-Sn132, with the corresponding references. Also in the main text, the quoted studies will be referred to by indicating the Sn code, as reported in the tables. The countries where the studies had been conducted and year of publication are reported in Supplementary Tables S1–S3. The reader is referred to Table 4 for a precise matching between a given Sn and the corresponding reference. In total, 102 studies focused on f ID/IA and 30 on AMR. A higher number of studies was focused on CvsO (73 on ID/IA; 27 AMR), compared with IvsE (29 on ID/IA; 3 AMR). The countries where the studies had been conducted are also presented in Figures 2, 3. Comparative studies on IvsE were mainly conducted in Mediterranean and sub-Saharan countries, Far East Asia, and South America, while studies on CvsO were mainly conducted in North and South America, North and Central Europe, and Mediterranean countries. Studies on AMR/AMS came mainly from CvsO comparisons, mostly from North America and Europe. The two major ID groups that had been investigated in both CvsO and IvsE are: (1) intra-mammary infections (IMIs), with 31 studies (3 IvsE and 28 CvsO), mainly from North America and Europe and (2) gastrointestinal parasitic infections (GPIs), with 20 studies (4 IvsE and 16 CvsO) mainly from European countries. A full report and discussion on IMIs and GPIs can be found below in the main text. For the remaining IDs/IAs, for which the number of studies is limited, a complete list is reported below, with results and comments summarized in Supplementary Table S4. In general, the different dairy FMS (C, O, I, E) do not always appear to be associated with the incidence or prevalence of a given infection or disease. Furthermore, most of the studies did not clearly define the criteria for the attribution of a farm to the O or C group, even if several of the studies referred to the national transposition of the FAO guidelines on organic farms (Organically Produced Foods, 2023). Similarly, different studies classified I and E farms based on different criteria. Furthermore, a number of studies evaluated the risk factors associated with the prevalence/incidence of IDs, IAs or AMR, with less attention to the relevance of these risk factors in relation to the type of FMS in terms of C, O, I, E. However, since the goal of this study was to contribute to the public debate on animal welfare and ecological sustainability issues associated with the different FMS, we will only report briefly on the specific risk factors (RFs).
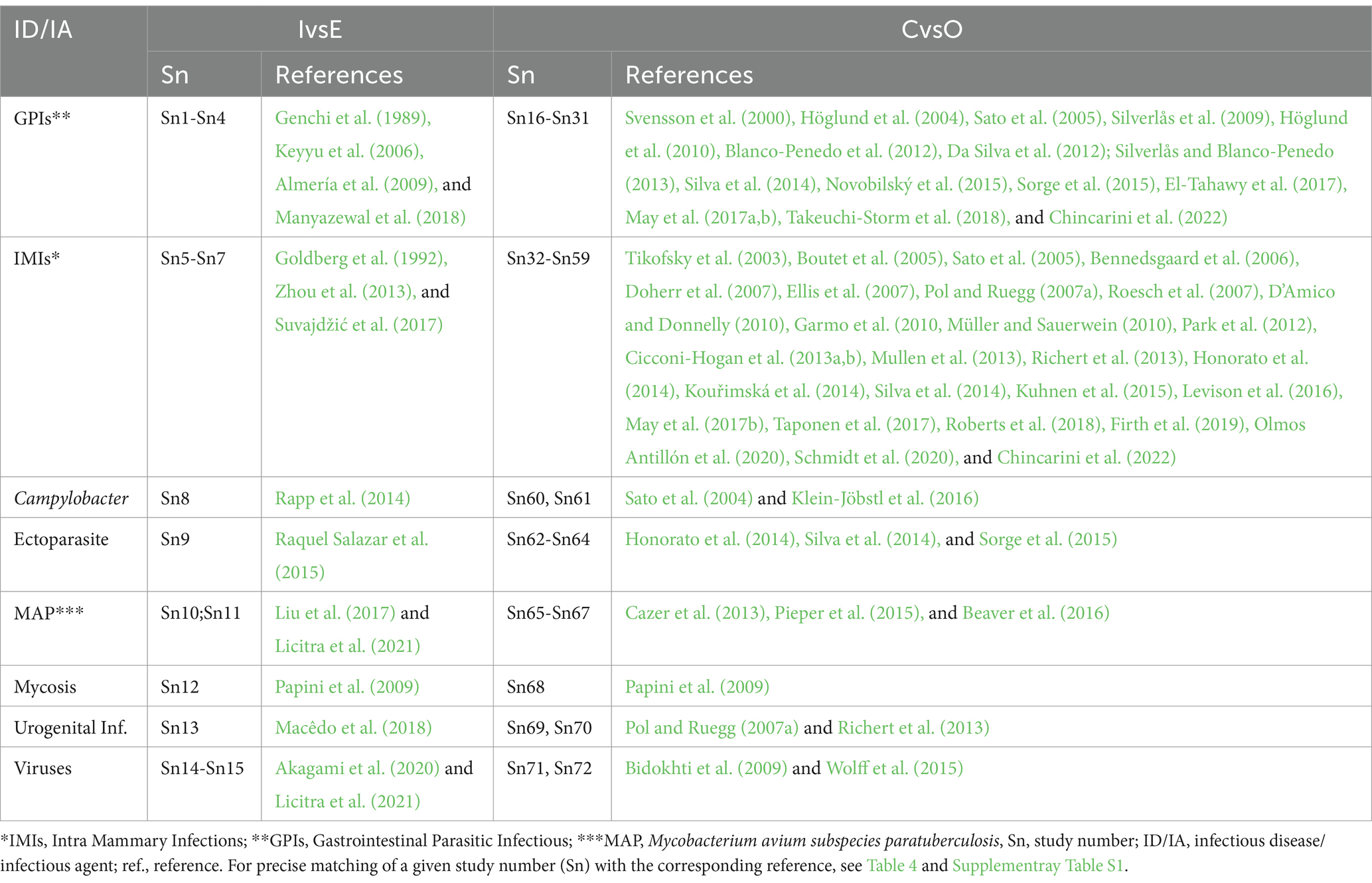
Table 1. Comparative studies on the presence of infectious diseases or infectious agents (ID/IA), in intensive versus extensive management (IvsE, on the left) and conventional versus organic management (CvsO, right), in which the investigated ID/IA coincide.
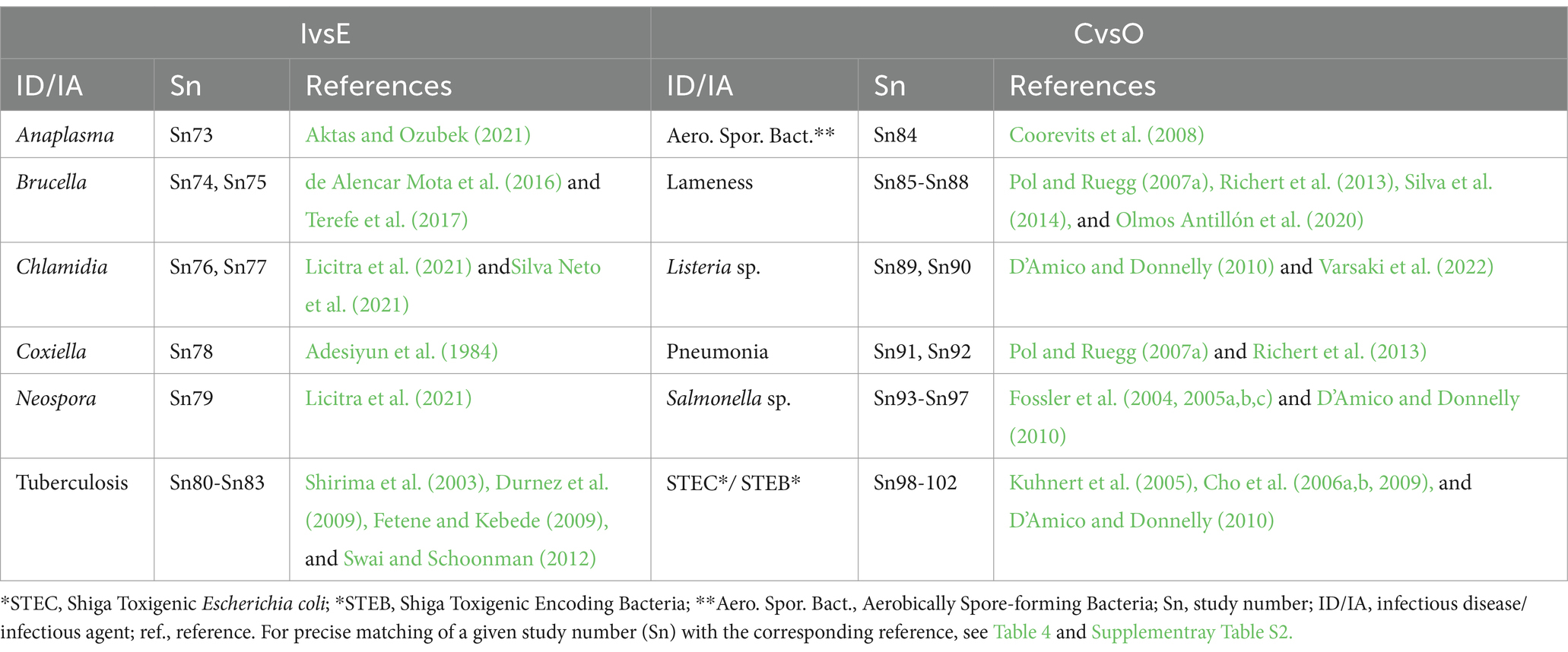
Table 2. Comparative studies on the presence of infectious diseases or infectious agents (ID/IA), in intensive versus extensive management (IvsE, on the left) and conventional versus organic management (CvsO, right), in which the investigated ID/IA do not coincide.
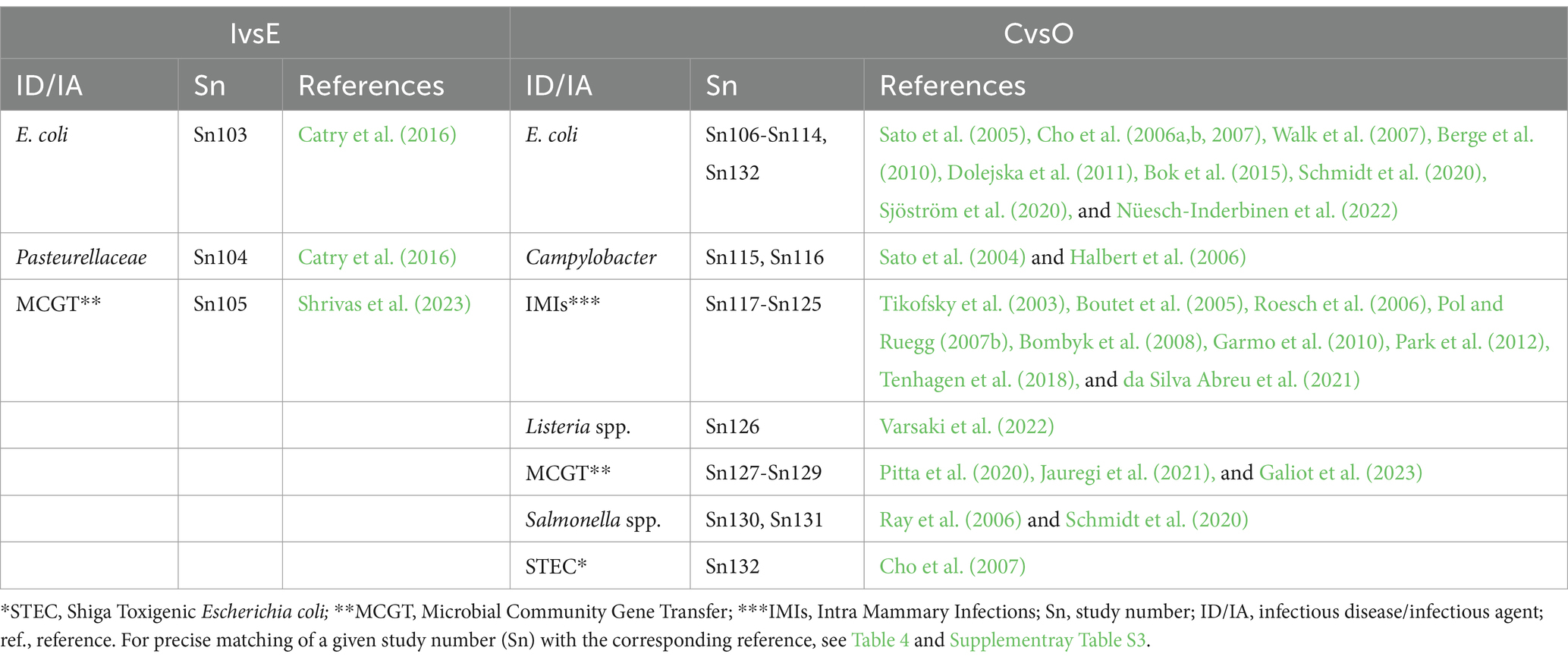
Table 3. Comparative studies of antimicrobial resistance/antimicrobial susceptibility (AMR/AMS) in infectious diseases or infectious agents (ID/IA) in intensive versus extensive management (IvsE, on the left) and conventional versus organic management (CvsO, right).
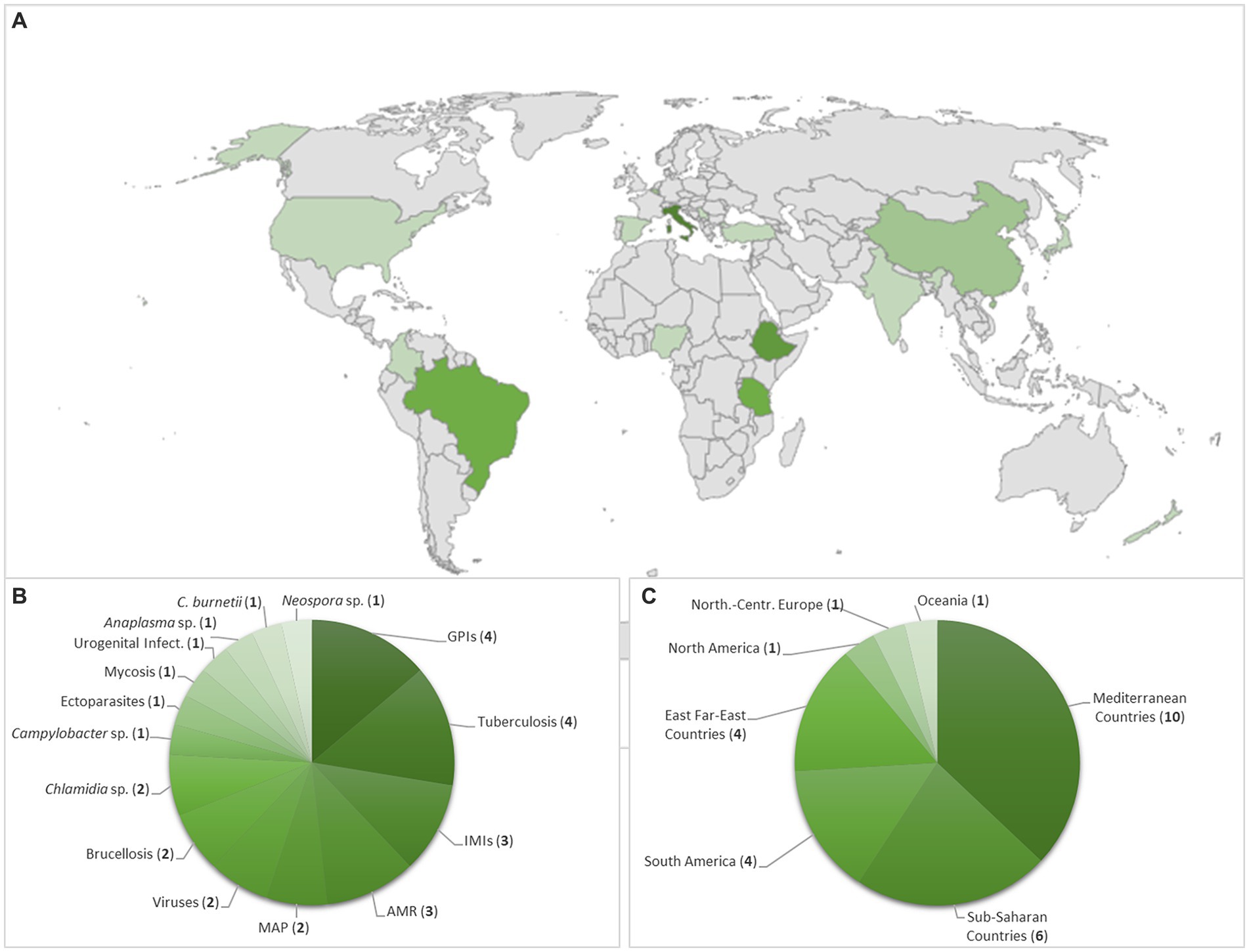
Figure 2. World map with the countries where IvsE investigations were conducted (A); number of studies on the different ID/IA/AMR (B); number of studies for macro region (C).
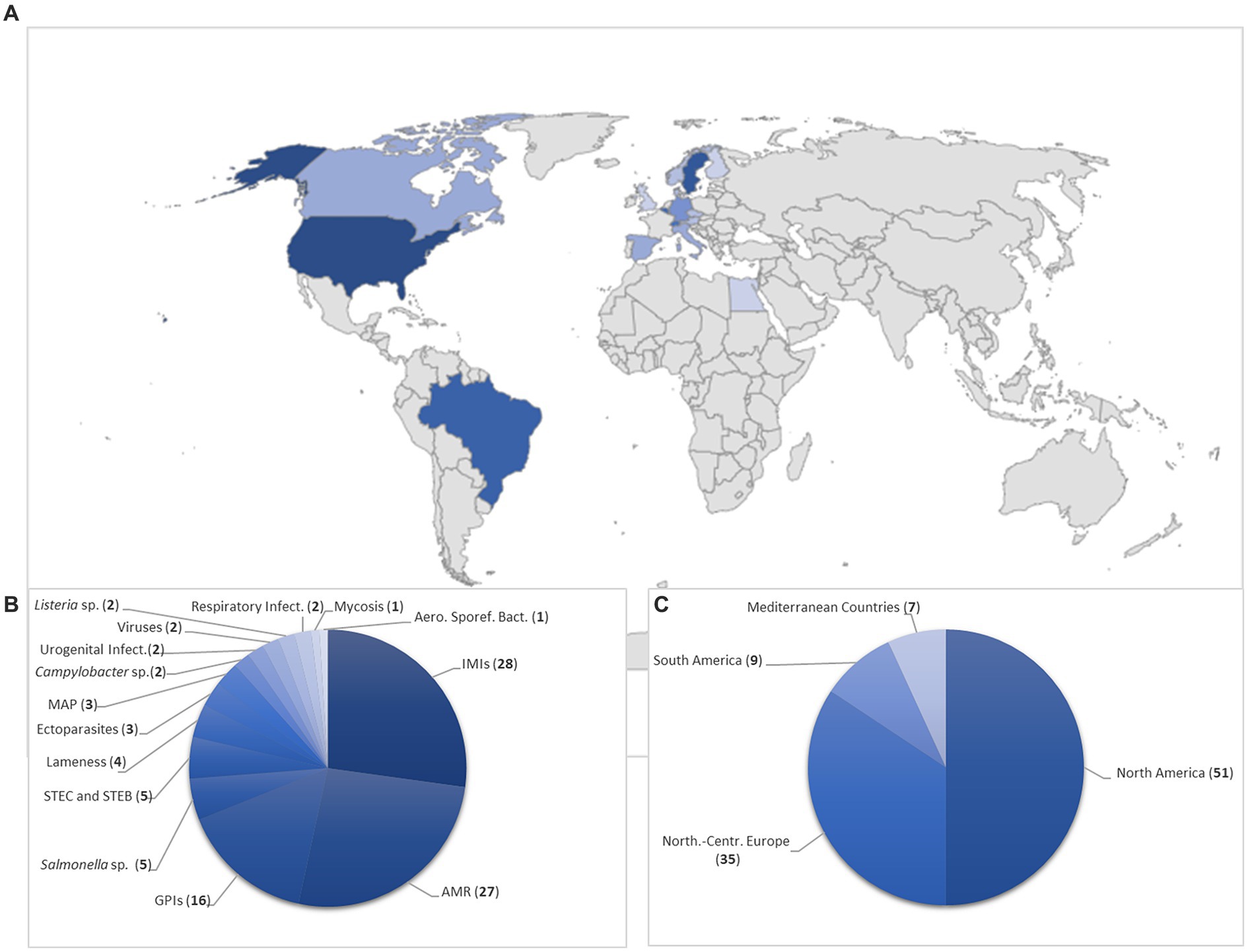
Figure 3. World map with the countries where CvsO investigations were conducted (A); number of studies on the different ID/IA/AMR (B); number of studies for macro region (C).
3.2 Intramammary infections
Thirty-one studies focused on IMIs were retrieved. Twenty-eight of these dealt with CvsO (Sn32-Sn59) (Tikofsky et al., 2003; Boutet et al., 2005; Sato et al., 2005; Bennedsgaard et al., 2006; Doherr et al., 2007; Ellis et al., 2007; Pol and Ruegg, 2007a; Roesch et al., 2007; D’Amico and Donnelly, 2010; Garmo et al., 2010; Müller and Sauerwein, 2010; Park et al., 2012; Cicconi-Hogan et al., 2013a,b; Mullen et al., 2013; Richert et al., 2013; Honorato et al., 2014; Kouřimská et al., 2014; Silva et al., 2014; Kuhnen et al., 2015; Levison et al., 2016; May et al., 2017b; Taponen et al., 2017; Roberts et al., 2018; Firth et al., 2019; Olmos Antillón et al., 2020; Schmidt et al., 2020; Chincarini et al., 2022); three with IvsE (Sn5-Sn7) (Goldberg et al., 1992; Zhou et al., 2013; Suvajdžić et al., 2017).
Eight out of the 28 studies on CvsO did not report any significative difference between C and O (Sn32; Sn35; Sn40; Sn44; Sn46; Sn49; Sn57; Sn58), six studies reported a higher prevalence/incidence of IMI in C (Sn38; Sn41; Sn42; Sn47; Sn52; Sn59); one study reported a higher prevalence/incidence of IMI in O (Sn56). Among the remaining 13 studies, six were focused on the risk factors associated with IMI (see below); seven highlighted apparently contrasting results. Sn55 recorded higher somatic cell counts (SCCs) in organic farms at day 31 DIM (day in milking), which were however similar to that of conventional herds at 102 DIM; in addition, higher prevalence of non-agalactiae streptococci was recorded at both 31 DIM and102 DIM in O compared to C, but the prevalence of coagulase-negative staphylococci was lower in O at 102 DIM. These fluctuations in cellular and microbial counts were not associated with clinical or sub-clinical mastitis. Similarly, in Sn41, higher counts of mesophilic and coliform bacteria were found in milk samples from O, but with no significant correlations with IMIs. A Norwegian study (Sn50) highlighted a higher proportion of dried off quarters in O vs. C, but did not find any difference in the number of quarters positive for mastitis bacteria, even if lower SCC in O cows was noted. Another study conducted in Sweden (Sn33) reported higher prevalence of IMI in C, even though bulk milk SCCs was higher in O. A German study (Sn51) did not report any difference in bulk milk SCCs, but reported a higher portion of organic vs. conventional individual cows with SCC > 150,000 at both 3 months before and 3 months after the dry period. A study conducted in the USA (Sn48) monitored several farms for 3 years during the transition from C to O, highlighting a higher incidence of mastitis in O at parturition, but no differences at dry-off. Finally, Sn39 reported that the incidence rate of clinical mastitis was higher in C compared to O (23.7 vs. 13.2 cases per 100 cows per year), however, bulk tank SCC tended to be lower in C.
Among the three retrieved studies on IvsE, in one the prevalence of mycotic mastitis in China was higher under extensive management (Sn6). One study, focused on S. aureus biofilm-producer strains in different regions of Serbia, did not find any differences under intensive or semi-extensive dairy farms (Sn5). Finally, a study conducted in the USA (Sn7) focused on the risk factors associated to grazing management.
Part of the studies retrieved with the query string for CvsO (namely: Sn34, Sn44, Sn47, Sn53, Sn54) were focused on the risk factors associated with IMI, and only to a lesser extent on the effects of the type of management, C or O, on the prevalence or incidence of these infections. Highlighted risk factors include: lactation number, farming part time, poor cleanliness of udders, size of the herd, use of mineral feed supplements, irregular milking intervals, milk urea concentrations, water temperature for washing the milking system, bedding area, timing of antibiotic treatments in relation to dry period (C only), hygiene, extent in the use of external resources, number of people responsible for mastitis treatment, age of the premises, percentage of cows with three or fewer quarters, use of fore stripping, proactive detection of mastitis during postpartum (and thus treatment), and stall barn housing. In addition, environmental temperature and duration of the infection in positive cows were associated with the incidence of mycotic mastitis (Sn6). Evidence for the role of genetic susceptibility to IMI was also reported in Sn36. A reduction in the risk of mastitis was associated to regular access to pasture, automatic milking shut-off and access to feed immediately after milking (Sn34). None of these factors appear to be specifically associated with C, O, I, E. However, they represent important leverage points for management toward healthier animals in terms of rational grazing (e.g., through rotational grazing rather than confined grazing) that are not specifically linked to intensive or extensive FM (Sn7).
3.3 Gastrointestinal parasitic infections
Twenty studies focused on GIPs were retrieved. Sixteen of these dealt with CvsO (Sn16-Sn31) (Svensson et al., 2000; Höglund et al., 2004; Sato et al., 2005; Silverlås et al., 2009; Höglund et al., 2010; Blanco-Penedo et al., 2012; Da Silva et al., 2012; Silverlås and Blanco-Penedo, 2013; Silva et al., 2014; Novobilský et al., 2015; Sorge et al., 2015; El-Tahawy et al., 2017; May et al., 2017a,b; Takeuchi-Storm et al., 2018; Chincarini et al., 2022), while the remaining four dealt with IvsE (Sn1-Sn4) (Genchi et al., 1989; Keyyu et al., 2006; Almería et al., 2009; Manyazewal et al., 2018).
Three out of 16 studies comparing CvsO (Sn21, Sn24, Sn25) did not report any significative difference, four studies reported a higher GPIs in conventional farming (Sn16, Sn18, Sn19, Sn30), five studies reported a higher GPIs in organic farming (Sn22, Sn23, Sn27, Sn29, Sn31), and the remaining four studies (Sn17, Sn20, Sn26, Sn28) were focused on risk factors associated to GPIs, not strictly related with the type of management. Studies on Cryptosporidium spp. infection provided apparently contradictory results. According to Sn24, there was no difference in the prevalence in either calves or cows; however, another study (Sn28) found higher levels of parasite shedding in organic farms, but a variety of factors not strictly related with the type of management might be associated with parasite shedding. Studies focused on fascioliasis (Sn21; Sn27), reported no significant difference between the two management types, while another (Sn18) detected significantly lower prevalence in O farms, probably due to continuous exposure to the parasite, leadings to better resilience (Sn16). Studies considering Ostertagia ostertagi highlighted contrasting results: two studies (Sn25; Sn29) found no correlation between infection and FMS, while another (Sn27) reported the opposite. Studies on the lungworm Dictyocaulus viviparus also reported contrasting results: Sn30 detected D. viviparus only on C, while Sn27 reported a prevalence of 18% in O and 9% in C herds. Interestingly, infected conventional herds were located near infected organic herds. Two studies (Sn22; Sn23), conducted in North and South America, reported a significatively higher prevalence of strongly-type fecal eggs in O farms, while a German study (Sn19), highlighted a significantly higher prevalence in C farms and pointed out the risk factors associated to seasonality. Another German study (Sn20) considered the issue of bovine genetics as a risk factor for parasitic infections, not strictly related to different FMS, even if particular genetic traits could be preferred in relation to the type of farming. Moreover, as highlighted in a Danish study (Sn17), estimations GPIs can also vary in relation with the type diagnostic method used.
Two out of four studies comparing IvsE reported higher GPIs on extensive farms (Sn2, Sn4), one reported higher GPIs in intensive farming, but also considered other risk factors (Sn1), while the other was focused only on risk factors (Sn3). Sn2 (conducted in Spain) reported a higher prevalence of Ostertagia ostertagi in EvsI, but the different environmental contexts could explain this result. Similar findings came from Sn4 (conducted in Italy) that compared two intensive farms (located in the Po River Valley) with an extensive farm (in an Alpine Mountain region), but again the differences in the sampling areas could represent an important confounding factor. Sn1, conducted in Ethiopia, highlighting a lower prevalence for cryptosporidiosis in IvsE; however, the authors note that the infection was significantly associated not only with the FMS but also with same others RFs like farm location, herd size, source of drinking water, weaning age, presence of bedding, pen cleanness and cleanness of hindquarters. Sn3, conducted in Tanzania, showed that prevalences of nematodes and flukes vary widely with geographic location and grazing management, further highlighting that several RFs not specifically related to the type of farming, (e.g., communal grazing and watering management practices) play a role in the circulation of parasitic worms.
3.4 Miscellaneous of retrieved IDs/IAs with limited comparative studies
This paragraph presents a complete list of the IDs/IAs for which the number of comparative studies is limited. Results and comments are summarized in Supplementary Table S4.
Comparative studies of IDs/IAs present on both CvsO and IvsE: five studies on MAP, Sn65-Sn67 on CvsO (Cazer et al., 2013; Pieper et al., 2015; Beaver et al., 2016), and Sn10-Sn11 on IvsE (Liu et al., 2017; Licitra et al., 2021). Four studies on viral infections, Sn71-Sn72 on CvsO (Bidokhti et al., 2009; Wolff et al., 2015), and Sn14-Sn15 on IvsE (Akagami et al., 2020; Licitra et al., 2021). Three studies on urogenital infections, Sn69-Sn70 on CvsO (Pol and Ruegg, 2007a; Richert et al., 2013), and Sn13 on IvsE (Macêdo et al., 2018). Three studies on Campylobacter sp., Sn60-Sn61 on CvsO; (Sato et al., 2004; Klein-Jöbstl et al., 2016), and Sn8 on IvsE (Rapp et al., 2014). Four studies on ectoparasites, Sn62-Sn64 on CvsO; (Honorato et al., 2014; Silva et al., 2014; Sorge et al., 2015), and Sn9 on IvsE (Raquel Salazar et al., 2015). Two studies on zoonotic dermatophyte, Sn12 on CvsO; (Papini et al., 2009), and Sn68 on IvsE (Papini et al., 2009).
Comparative studies of IDs/IAs present only on CvsO: Shiga Toxigenic Escherichia coli (STEC) and Shiga Toxigenic Bacteria (STEB) (Sn98-Sn102) (Kuhnert et al., 2005; Cho et al., 2006a,b, 2009; D’Amico and Donnelly, 2010), Lameness (Sn85-Sn88) (Pol and Ruegg, 2007a; Richert et al., 2013; Silva et al., 2014; Olmos Antillón et al., 2020), Salmonella sp. (Sn93-Sn97) (Fossler et al., 2004, 2005a,b,c; D’Amico and Donnelly, 2010), pneumonia (Sn91-Sn92) (Pol and Ruegg, 2007a; Richert et al., 2013), Listeria sp. (Sn89-Sn90) (D’Amico and Donnelly, 2010; Varsaki et al., 2022) and aerobic spore-forming bacteria (Sn84) (Coorevits et al., 2008).
Comparative studies of IDs/IAs present only on the IvsE: tuberculosis (Sn80-Sn83) (Shirima et al., 2003; Durnez et al., 2009; Fetene and Kebede, 2009; Swai and Schoonman, 2012), Brucellosis (Sn74-Sn75) (de Alencar Mota et al., 2016; Terefe et al., 2017), Chlamidia sp. (Sn76-Sn77) (Licitra et al., 2021; Silva Neto et al., 2021), Coxiella burnetii (Sn78) (Adesiyun et al., 1984), Neospora sp. (Sn79) (Licitra et al., 2021), and Anaplasma marginale (Sn73) (Aktas and Ozubek, 2021).
3.5 Antibiotic resistance/susceptibility (AMR/AMS)
Among the 30 studies retrieved, 27 compared CvsO (Sn106-Sn132) and three IvsE (Sn103-Sn105). In general, a higher circulation of AMR genes was found on conventional and intensive FMS.
AMR/AMS in CvsO. Ten studies on AMR/AMS strains of Escherichia coli (Sn106-Sn114, Sn132) (Sato et al., 2005; Cho et al., 2006a, 2007; Walk et al., 2007; Berge et al., 2010; Dolejska et al., 2011; Bok et al., 2015; Schmidt et al., 2020; Sjöström et al., 2020; Nüesch-Inderbinen et al., 2022) were retrieved. Six were conducted in the USA. Sn113 did not find any significant difference across FMS for the abundance of E. coli O157 virulence marker genes, antimicrobial susceptibility profiles, and genotypes. On the contrary, Sn107 found significantly more abundant resistance genes in animals bred on conventional farms, but no significant differences in carcasses or beef trimmings. E. coli strains isolated from fecal samples showed a significantly higher resistance to seven out of 17 antimicrobial molecules on conventional farms (Sn114). Furthermore, AMR was strongly influenced by animal age, geographical region (dairy-intense flat land or more extensive foothill pasture) and whether cattle were raised for dairy or beef (Sn111). Sn112 assessed the association between age of cattle, and AMR/AMS in E. coli phylogroups isolated from both FMS. Here the authors used a hierarchical log-linear modeling approach that accounts for additional predictors, resulting in more robust relationships; the study provided evidence of clonal resistance (ampicillin) and genetic hitchhiking (tetracycline), reporting a significant association between low multidrug resistance, organic herds and numerically dominant phylogroup B1 strains, suggesting that the genetic composition of the herds may influence the AMR/AMS. Additionally, authors estimated that it would take from three to 15 years to have a significant change in bacterial populations passing from conventional to organic farming. These studies clearly demonstrates that there exist possible confounding factors/risk factors, that, if not properly accounted for in models, can potentially bias the results. Another study (Sn132) found a significantly higher proportion of non-susceptible spectinomycin in Shiga Toxigenic E. coli (STEC) isolates from conventional farms. Resistance to sulphadimethoxine in calves (but not in adult milking cows) was significantly higher on conventional farms. Multidrug resistant (MDR) patterns were more commonly found in non-O157 STEC vs. O157 STEC and the percentage of MDR on the two farm types was similar. A Swedish study (Sn108) found little significant difference for resistance to single antimicrobials on conventional farms. Most conventional herds had a rather high proportion of isolates resistant to at least one antimicrobial, but MDR strains were rare. A study conducted in Czech Republic (Sn110), revealed a higher prevalence of E. coli isolates producing an extended-spectrum beta-lactamase (ESBL) on conventional farms compared to organic ones. A Swiss study (Sn106) did not find any significative difference in the AMR of E. coli strains isolated from young dairy calves, but did report that the ESBL-producing Enterobacteriaceae were more prevalent in conventional farms.
Nine studies on AMR/AMS on IMIs were retrieved (Sn117-Sn125) (Tikofsky et al., 2003; Boutet et al., 2005; Roesch et al., 2006; Pol and Ruegg, 2007b; Bombyk et al., 2008; Garmo et al., 2010; Park et al., 2012; Tenhagen et al., 2018; da Silva Abreu et al., 2021). Three out the four studied conducted in USA (Sn125, Sn121, Sn122), found that Staphylococcus aureus isolates from milk samples from C were significantly more resistant for the majority of tested antimicrobial molecules (Sn121, Sn125). Sn122 found that AMR of IMIs-associated pathogens were more common in C, yet the prevalence of bacteria responsible for mastitis was higher on O (with the exception of coliforms). Finally, a high proportion of sulfadimethoxine-resistant isolates were observed in both FMS, and were higher on O. Another study from USA (Sn119) was focused on the conversion process from conventional to organic management over a 3-year period; coagulase-negative staphylococci (CNS) were the most prevalent bacteria responsible for mastitis. They were significantly less resistant to β-lactam antibiotics after herd transitioned to O. AMR significatively decreased for ampicillin, cephalothin, cloxacillin, and penicillin for CNS, but not for S. aureus. This suggests that cessation of antibiotic use, in combination with organic management, reduced AMR of mastitis bacteria, even if the prevalence of S. aureus did not change significantly as herds transitioned from conventional to organic FMS. Similar results were reported in a Belgium study (Sn124) which found that the three most frequently isolated pathogens (Streptococcus uberis, S. aureus, and Streptococcus dysgalactiae) were significantly more resistant to antimicrobials on C. On the contrary, a Norwegian study (Sn120), did not find a significant difference in penicillin resistance against coagulase-negative staphylococci isolated from sub-clinically infected quarters, while a Swiss study (Sn123), found that AMR of Staphylococcus spp. and Streptococcus spp. was not significantly different in CvsO, except for S. uberis, which tended to have more single gene resistance on OvsC ones. Finally, a Brazilian study (Sn117), on Staphylococcus strains sampled in Minas Frescal cheese found no significant prevalence related to FMS.
Three studies on AMR/AMS in microbial communities and gene transfer phenomena MCGT (Sn127-Sn129) (Pitta et al., 2020; Jauregi et al., 2021; Galiot et al., 2023). A Canadian study (Sn127) analyzed publicly available metagenomics data, showing that organic practices are generally associated with lower prevalence of AMR Genes (AMRGs). Sn129 affirmed that the abundance and diversity of ARGs in feces was significantly higher in conventional vs. organic herds. All manure storage and soil samples had a diversity (albeit low abundance) of AMRGs conferring resistance to several antibiotics. Antimicrobial use on farms significantly influenced specific groups of AMRGs in feces, but not in manure storages or soil samples. Similar results from a Spanish study (Sn128), based on the monitoring AMRGs and mobile genetic elements (MGEs) in different types of comparisons (amended vs. unamended, CvsO, slurry vs. fresh or aged manure), found that the spread of AMRGs-MGEs cannot be inferred directly from any of the individual comparisons (including CvsO).
Two studies on AMR/AMS in Salmonella strains (Sn130, Sn131) (Ray et al., 2006; Schmidt et al., 2020) (both conducted in the USA). Sn130 found significantly higher circulation of AMRGs on C, but differences in carcasses or beef trimmings were not significant. Sn131, using logistic proportional hazards models, found that isolates from C were significantly associated with higher MIC for only two out of nine antimicrobials (streptomycin and sulfamethoxazole). Moreover, Salmonella isolates resistant to five or more antimicrobial agents were found on both FMS.
Two studies on AMR/AMS conducted on Campylobacter strains (Sn115, Sn116) (Sato et al., 2004; Halbert et al., 2006) (both conducted in the USA). Sn115 found that resistance to one out of four antimicrobial molecules tested was more prevalent in C, while Sn116, did not find any difference.
Similar results came from the only recovered study focused on Listeria monocytogenes (Sn126) (Varsaki et al., 2022), conducted in USA.
AMR/AMS in IvsE comparisons. Three studies on IvsE (Sn103-Sn105) (Catry et al., 2016; Shrivas et al., 2023).
An Indian study (Sn105) (Shrivas et al., 2023), comparing intensive farms vs. farms were animal had access to grazing, found that fecal bacteria from intensive farms were characterized by higher prevalence of AMR, which was also affected by feeding practices and nutrient concentration. Two studies, conducted by the same research group in Belgium (Sn103-Sn104) (Catry et al., 2016), focused on AMR profiles of both E. coli, (retrieved from the rectum) and Pasteurellaceae bacteria (retrieved from the nasal cavity) found a strong relationship between antimicrobial treatment and resistance profiles of bacterial isolates, in particular on intensive farms.
4 Conclusion
This systematic review has not specifically been focused on the issues of zoonoses, EID of human relevance, and spillover phenomena. Rather, we developed search strings with the objective of a wide-spectrum retrieval of research publications, dealing with the issue of the circulation of infections and bacterial AMR in dairy farms, in relation with the type of management system. However, results are relevant to the scenarios associated with zoonotic spillover events, an issue that has attracted a great deal of attention following the COVID19 pandemics (Shepon et al., 2023). The search strings, with wide-spectrum terms such as infect*, pathogen*, zoono*, microb*, should really have caught papers dealing with pathogens that have the potential to infect humans. We emphasize that the risks of infection in general, and zoonoses in particular, associated with intensive and extensive breeding systems are still debated (e.g., Shepon et al., 2023). The present systematic review would suggest that the circulation of infectious agents is not significantly influenced by the type of management system in dairy farms, whether it is conventional or organic, intensive or extensive. Indeed, only a few studies reported significant differences, but with contrasting results regarding the higher or lower circulation of different pathogens under the different types of management. As anticipated in the introduction, the overall interpretation of our results suggest that CvsO and IvsE comparisons can be regarded as partially equivalent in some respects, for example in relation to the consumers’ perception of issues such as animal welfare and sustainability (e.g., Harper and Makatouni, 2002; Anders et al., 2023; Clark et al., 2024). Therefore, the main outcome of our study, in relation to the public debate on the “pros” and “cons” of the different types of animal management, suggests that organic and extensive farming is not correlated with a decrease of circulation of infectious agents, compared to conventional and intensive dairy FMS.
Despite consumer perception that organic and extensive production are approximately the same thing, the present study used two separate strings for literature search, in order to separate the two comparisons, CvsO and IvsE. In-depth examination of the retrieved publications highlight that: (1) the definitions of intensive and extensive farming differ among studies, making difficult the comparison of studies that are apparently similar; (2) Conventional and organic farming are not clearly defined in all publications: 52 studies refer to the national transposition of international guidelines on organic farming, such as the FAO guidelines (Migliorini and Wezel, 2017; Seufert et al., 2017; Organically Produced Foods, 2023), or to specific national norms; 33 declare that the Organic certification was issued by an authority/agency, but they do not specify the norms that have been followed (even though we might assume that the norms are those of the country or region where the studies have been conducted); only 18 do not refer to any national or international norm or guideline, or to any certification. It follows that basic requirements for Organic production are likely to present some consistency across different studies, at least for the 85 studies that refer to some type of norm (52 studies), or certification (33 studies) (for comparisons of norms and guidelines on Organic farming, see Migliorini and Wezel, 2017; Seufert et al., 2017). This suggests that comparisons between studies on Organic productions are more reliable, with respect to studies on Extensive management system, for which there are not clear definitions. Furthermore, different studies evaluated other risk factors, not necessarily associated with a given FMS.
Comparison of CvsO showed greater prevalence of antibiotic resistances in conventional farms. This is consistent with the expectation that the use of antibiotics is minimal in organic farms, with a consequent low selective pressure for antibiotic resistance. However, AMR was in general uncommon in both farming systems. Moreover, many confounding factors suggest that different bacterial species may behave differently depending on a variety of management and environmental variables in different environmental contexts and in different countries. For instance, age distribution, time of the year at sampling, an early or extended period of cow-calf contact, diagnostic methodology, type of drugs used, environmental context, etc. are quite different across the sampled farms, affecting interpretation of the significance. Herd size is particularly important because conventional herds are frequently larger than organic ones, and reliable comparisons in the presence of highly variable herd sizes are virtually impossible. As for the comparison IvsE, AMR was more prevalent in intensive farming, but results are to be interpreted with caution, since only three studies were retrieved for this comparison.
As already emphasized, the reading of the publications listed in Tables 1–3 revealed that terms like C, O, I, E are not always univocally defined (even though the existence of norms, formal definitions and certification authorities and agencies make more reliable the attribution of the O status, compared to that of the E status – see above and Supplementary Table S5). Furthermore, the results of the statistical tests in some of the examined studies might have been flawed by confounding factors, thus reducing the power of statistical analysis, which may have detected false significant correlations or not detected significant ones. For this reason, future studies on the impact of the management system on the circulation of infectious agents and AMR should apply statistical approaches exploiting generalized linear models (GLM) or similar statistical tools, instead of tests which only consider the variable of interest. Since GLMs can include additional factors, they can disentangle their contributions to the dependent variable, to better highlight significant differences in the predictor of interest (e.g., FMS). This would require recording additional information related to the farms and herds under comparison in a form that is possible to use in such models; to note that GLMs can also exploit categorical and continuous predictors together, enabling high modeling flexibility. Other modeling strategies can also be envisioned, for instance cases with many predictors, most of which are expected to be non-influent on the outcome, can benefit from a regularization and/or variable selection step like in LASSO regression.
Besides specific statistical approaches, our recommendation is that future studies comparing different types of management in animal productions should include a clear presentation of all possible confounding factors, as well as of all of the possible risk factors that are not intrinsically associated with the type of management under investigation. Another recommendation is that the management systems under analyses are clearly defined.
The systematic review approach, or metanalysis, was developed in the context of the medical area, with the goal of generating the so-called evidence-based medicine, in relation to the need to provide the international medical community with firm evidence regarding the efficacy of a given therapeutic approach. Following an agenda raised by Archie Cochrane, Iain Chalmers played a key role in the establishments of this approach in the medical field, and in the launching of the Cochrane Collaboration, now referred to as the Cochrane Organization (Winkelstein, 2009). We hope that the application of the systematic review approach into the area of primary food production, where our present work is just an example, could promote an international initiative aimed at proposing a novel Cochrane Centre, devoted to security in animal husbandry and food production. Should such an initiative be established, this would guide future studies toward more uniform approaches, and thus a higher comparability.
Data availability statement
The original contributions presented in the study are included in the article/Supplementary material, further inquiries can be directed to the corresponding author.
Author contributions
MP: Writing – review & editing, Writing – original draft, Methodology, Investigation. MB: Writing – review & editing, Writing – original draft, Methodology, Formal analysis, Conceptualization. GP: Writing – review & editing, Investigation, Formal analysis, Data curation. LK: Writing – review & editing, Data curation. PM: Writing – review & editing, Conceptualization. CB: Writing – review & editing, Writing – original draft, Validation, Supervision, Resources, Funding acquisition, Conceptualization. MV: Writing – review & editing, Data curation, Investigation.
Funding
The author(s) declare that financial support was received for the research, authorship, and/or publication of this article. This work was supported by IRCAF - Invernizzi Reference Centre on Agri-Food “Romeo ed. Enrica Invernizzi.” Pro-ject Milk Quality: a multifaceted approach. Partial further support by NextGeneration EU-MUR PNRR Extended Partnership initiative on Emerging Infectious Diseases (Project no. PE00000007, INF-ACT).
Acknowledgments
MP sincerely thanks Indira Fassioni and Nicola Ferrari for their kind support. MP and CB thank Lorenzo Morelli for catalyzing the start of this project and for his engaging encouragement.
Conflict of interest
The authors declare that the research was conducted in the absence of any commercial or financial relationships that could be construed as a potential conflict of interest.
Publisher's note
All claims expressed in this article are solely those of the authors and do not necessarily represent those of their affiliated organizations, or those of the publisher, the editors and the reviewers. Any product that may be evaluated in this article, or claim that may be made by its manufacturer, is not guaranteed or endorsed by the publisher.
Supplementary material
The Supplementary material for this article can be found online at: https://www.frontiersin.org/articles/10.3389/fsufs.2024.1397095/full#supplementary-material
References
Adesiyun, A. A., Jagun, A. G., and Tekdek, L. B. (1984). Coxiella burnetii antibodies in some Nigerian dairy cows and their suckling calves. Int. J. Zoonoses 11, 155–160,
Akagami, M., Seki, S., Kashima, Y., Yamashita, K., Oya, S., Fujii, Y., et al. (2020). Risk factors associated with the within-farm transmission of bovine viral diarrhea virus and the incidence of persistently infected cattle on dairy farms from Ibaraki prefecture of Japan. Res. Vet. Sci. 129, 187–192. doi: 10.1016/j.rvsc.2020.02.001
Aktas, M., and Ozubek, S. (2021). Genetic diversity of major surface protein 1a of Anaplasma marginale in dairy cattle. Infect. Genet. Evol. 89:104608. doi: 10.1016/j.meegid.2020.104608
Almería, S., Adelantado, C., Charlier, J., Claerebout, E., and Bach, A. (2009). Ostertagia ostertagi antibodies in milk samples: relationships with herd management and milk production parameters in two Mediterranean production systems of Spain. Res. Vet. Sci. 87, 416–420. doi: 10.1016/j.rvsc.2009.05.001
Anders, S., Malzoni, M., and An, H. (2023). Altruism and anti-anthropocentrism shape individual choice intentions for pro-environmental and ethical meat credence attributes. PLoS One 18:e0294531. doi: 10.1371/journal.pone.0294531
Bartlett, H., Holmes, M. A., Petrovan, S. O., Williams, D. R., Wood, J. L. N., and Balmford, A. (2022). Understanding the relative risks of zoonosis emergence under contrasting approaches to meeting livestock product demand. R. Soc. Open Sci. 9:211573. doi: 10.1098/rsos.211573
Beaver, A., Ruegg, P. L., Gröhn, Y. T., and Schukken, Y. H. (2016). Comparative risk assessment for new cow-level Mycobacterium avium ssp. paratuberculosis infections between 3 dairy production types: organic, conventional, and conventional-grazing systems. J. Dairy Sci. 99, 9885–9899. doi: 10.3168/jds.2016-11360
Bellet, C., Hamilton, L., and Rushton, J. (2021). Re-thinking public health: towards a new scientific logic of routine animal health care in European industrial farming. Palgrave Commun. 8, 1–11. doi: 10.1057/s41599-021-00890-y
Bennedsgaard, T. W., Thamsborg, S. M., Aarestrup, F. M., Enevoldsen, C., Vaarst, M., and Christoffersen, A. B. (2006). Resistance to penicillin of Staphylococcus aureus isolates from cows with high somatic cell counts in organic and conventional dairy herds in Denmark. Acta Vet. Scand. 48:24. doi: 10.1186/1751-0147-48-24
Berge, A. C., Hancock, D. D., Sischo, W. M., and Besser, T. E. (2010). Geographic, farm, and animal factors associated with multiple antimicrobial resistance in fecal Escherichia coli isolates from cattle in the western United States. J. Am. Vet. Med. Assoc. 236, 1338–1344. doi: 10.2460/javma.236.12.1338
Bidokhti, M. R. M., Tråvén, M., Fall, N., Emanuelson, U., and Alenius, S. (2009). Reduced likelihood of bovine coronavirus and bovine respiratory syncytial virus infection on organic compared to conventional dairy farms. Vet. J. 182, 436–440. doi: 10.1016/j.tvjl.2008.08.010
Blanco-Penedo, I., Höglund, J., Fall, N., and Emanuelson, U. (2012). Exposure to pasture borne nematodes affects individual milk yield in Swedish dairy herds. Vet. Parasitol. 188, 93–98. doi: 10.1016/j.vetpar.2012.02.024
Bok, E., Mazurek, J., Stosik, M., Wojciech, M., and Baldy-Chudzik, K. (2015). Prevalence of virulence determinants and antimicrobial resistance among commensal Escherichia coli derived from dairy and beef cattle. Int. J. Environ. Res. Public Health 12, 970–985. doi: 10.3390/ijerph120100970
Bombyk, R. A. M., Bykowski, A. L., Draper, C. E., Savelkoul, E. J., Sullivan, L. R., and Wyckoff, T. J. O. (2008). Comparison of types and antimicrobial susceptibility of Staphylococcus from conventional and organic dairies in west-Central Minnesota, USA. J. Appl. Microbiol. 104, 1726–1731. doi: 10.1111/j.1365-2672.2007.03681.x
Boutet, P., Detilleux, J., Motkin, M., Deliege, M., Piraux, E., Depinois, A., et al. (2005). A comparison of somatic cell count and antimicrobial susceptibility of subclinical mastitis pathogens in organic and conventional dairy herds. Ann. Med. Vet. 149, 173–182,
Catry, B., Dewulf, J., Maes, D., Pardon, B., Callens, B., Vanrobaeys, M., et al. (2016). Effect of antimicrobial consumption and production type on antibacterial resistance in the bovine respiratory and digestive tract. PLoS One 11:e0146488. doi: 10.1371/journal.pone.0146488
Cazer, C. L., Mitchell, R. M., Cicconi-Hogan, K. M., Gamroth, M., Richert, R. M., Ruegg, P. L., et al. (2013). Associations between Mycobacterium avium subsp. paratuberculosis antibodies in bulk tank milk, season of sampling and protocols for managing infected cows. BMC Vet. Res. 9:234. doi: 10.1186/1746-6148-9-234
Chang, K. (2024) Organic food vs. conventional food. Well. https://archive.nytimes.com/well.blogs.nytimes.com/2012/09/04/organic-food-vs-conventional-food/ (Accessed January 25, 2024).
Chincarini, M., Lanzoni, L., Di Pasquale, J., Morelli, S., Vignola, G., Paoletti, B., et al. (2022). Animal welfare and parasite infections in organic and conventional dairy farms: a comparative pilot study in Central Italy. Animals 12:351. doi: 10.3390/ani12030351
Cho, S., Bender, J. B., Diez-Gonzalez, F., Fossler, C. P., Hedberg, C. W., Kaneene, J. B., et al. (2006a). Prevalence and characterization of Escherichia coli O157 isolates from Minnesota dairy farms and county fairs. J. Food Prot. 69, 252–259. doi: 10.4315/0362-028X-69.2.252
Cho, S., Diez-Gonzalez, F., Fossler, C. P., Wells, S. J., Hedberg, C. W., Kaneene, J. B., et al. (2006b). Prevalence of Shiga toxin-encoding bacteria and Shiga toxin-producing Escherichia coli isolates from dairy farms and county fairs. Vet. Microbiol. 118, 289–298. doi: 10.1016/j.vetmic.2006.07.021
Cho, S., Fossler, C. P., Diez-Gonzalez, F., Wells, S. J., Hedberg, C. W., Kaneene, J. B., et al. (2007). Antimicrobial susceptibility of Shiga toxin-producing Escherichia coli isolated from organic dairy farms, conventional dairy farms, and county fairs in Minnesota. Foodborne Pathog. Dis. 4, 178–186. doi: 10.1089/fpd.2006.0074
Cho, S., Fossler, C. P., Diez-Gonzalez, F., Wells, S. J., Hedberg, C. W., Kaneene, J. B., et al. (2009). Cattle-level risk factors associated with fecal shedding of Shiga toxin-encoding bacteria on dairy farms, Minnesota, USA. Can. J. Vet. Res. 73, 151–156
Cicconi-Hogan, K. M., Gamroth, M., Richert, R., Ruegg, P. L., Stiglbauer, K. E., and Schukken, Y. H. (2013a). Associations of risk factors with somatic cell count in bulk tank milk on organic and conventional dairy farms in the United States. J. Dairy Sci. 96, 3689–3702. doi: 10.3168/jds.2012-6271
Cicconi-Hogan, K. M., Gamroth, M., Richert, R., Ruegg, P. L., Stiglbauer, K. E., and Schukken, Y. H. (2013b). Risk factors associated with bulk tank standard plate count, bulk tank coliform count, and the presence of Staphylococcus aureus on organic and conventional dairy farms in the United States. J. Dairy Sci. 96, 7578–7590. doi: 10.3168/jds.2012-6505
Clark, B., Panzone, L. A., Stewart, G. B., Kyriazakis, I., Niemi, J. K., Latvala, T., et al. Consumer attitudes towards production diseases in intensive production systems. PLoS One 14:e0210432. doi: 10.1371/journal.pone.0210432
Coorevits, A., De Jonghe, V., Vandroemme, J., Reekmans, R., Heyrman, J., Messens, W., et al. (2008). Comparative analysis of the diversity of aerobic spore-forming bacteria in raw milk from organic and conventional dairy farms. Syst. Appl. Microbiol. 31, 126–140. doi: 10.1016/j.syapm.2008.03.002
D’Amico, D. J., and Donnelly, C. W. (2010). Microbiological quality of raw milk used for small-scale artisan cheese production in Vermont: effect of farm characteristics and practices. J. Dairy Sci. 93, 134–147. doi: 10.3168/jds.2009-2426
da Silva Abreu, A. C., Matos, L. G., da Silva Cândido, T. J., Barboza, G. R., de Souza, V. V. M. A., Munive Nuñez, K. V., et al. (2021). Antimicrobial resistance of Staphylococcus spp. isolated from organic and conventional Minas Frescal cheese producers in São Paulo, Brazil. J. Dairy Sci. 104, 4012–4022. doi: 10.3168/jds.2020-19338
Da Silva, J. B., Soares, J. P. G., and Da Fonseca, A. H. (2012). Gastrointestinal parasites occurrence in dairy cows kept in organic and conventional production system. Semin. Agrar. 33, 2375–2382. doi: 10.5433/1679-0359.2012v33n6p2375
de Alencar Mota, A. L. A., Ferreira, F., Ferreira Neto, J. S., Dias, R. A., Amaku, M., Hildebrand Grisi-Filho, J. H., et al. (2016). Large-scale study of herd-level risk factors for bovine brucellosis in Brazil. Acta Trop. 164, 226–232. doi: 10.1016/j.actatropica.2016.09.016
Doherr, M. G., Roesch, M., Schaeren, W., Schallibaum, M., and Blum, J. W. (2007). Risk factors associated with subclinical mastitis in dairy cows on Swiss organic and conventional production system farms. Vet. Med. 52, 487–495. doi: 10.17221/2060-VETMED
Dolejska, M., Jurcickova, Z., Literak, I., Pokludova, L., Bures, J., Hera, A., et al. (2011). IncN plasmids carrying blaCTX-M-1 in Escherichia coli isolates on a dairy farm. Vet. Microbiol. 149, 513–516. doi: 10.1016/j.vetmic.2010.11.032
Durnez, L., Sadiki, H., Katakweba, A., Machang’u, R. R., Kazwala, R. R., Leirs, H., et al. (2009). The prevalence of Mycobacterium bovis-infection and atypical mycobacterioses in cattle in and around Morogoro, Tanzania. Trop. Anim. Health Prod. 41, 1653–1659. doi: 10.1007/s11250-009-9361-4
Ellis, K. A., Innocent, G. T., Mihm, M., Cripps, P., McLean, W. G., Howard, C. V., et al. (2007). Dairy cow cleanliness and milk quality on organic and conventional farms in the UK. J. Dairy Res. 74, 302–310. doi: 10.1017/S002202990700249X
El-Tahawy, A. S., Bazh, E. K., and Khalafalla, R. E. (2017). Epidemiology of bovine fascioliasis in the Nile Delta region of Egypt: its prevalence, evaluation of risk factors, and its economic significance. Vet. World 10, 1241–1249. doi: 10.14202/vetworld.2017.1241-1249
European Parliament Research Service (2016). Available at: https://www.europarl.europa.eu/RegData/etudes/STUD/2016/581922/EPRS_STU(2016)581922_EN.pdf (Accessed January 25, 2024).
FAO (2023). Contribution of terrestrial animal source food to healthy diets for improved nutrition and health outcomes. Rome, Italy: FAO.
Ferma gli Allevamenti Intensivi. Greenpeace Ital Available at: https://www.greenpeace.org/italy/attivati/ferma-gli-allevamenti-intensivi/ (Accessed January 25, 2024).
Fetene, T., and Kebede, N. (2009). Bovine tuberculosis of cattle in three districts of northwestern Ethiopia. Trop. Anim. Health Prod. 41, 273–277. doi: 10.1007/s11250-008-9186-6
Firth, C. L., Laubichler, C., Schleicher, C., Fuchs, K., Käsbohrer, A., Egger-Danner, C., et al. (2019). Relationship between the probability of veterinary-diagnosed bovine mastitis occurring and farm management risk factors on small dairy farms in Austria. J. Dairy Sci. 102, 4452–4463. doi: 10.3168/jds.2018-15657
Fossler, C. P., Wells, S. J., Kaneene, J. B., Ruegg, P. L., Warnick, L. D., Bender, J. B., et al. (2005a). Herd-level factors associated with isolation of Salmonella in a multi-state study of conventional and organic dairy farms. II. Salmonella shedding in calves. Prev. Vet. Med. 70, 279–291. doi: 10.1016/j.prevetmed.2005.04.002
Fossler, C. P., Wells, S. J., Kaneene, J. B., Ruegg, P. L., Warnick, L. D., Bender, J. B., et al. (2005b). Herd-level factors associated with isolation of Salmonella in a multi-state study of conventional and organic dairy farms: I. Salmonella shedding in cows. Prev. Vet. Med. 70, 257–277. doi: 10.1016/j.prevetmed.2005.04.003
Fossler, C. P., Wells, S. J., Kaneene, J. B., Ruegg, P. L., Warnick, L. D., Bender, J. B., et al. (2004). Prevalence of Salmonella spp on conventional and organic dairy farms. J. Am. Vet. Med. Assoc. 225, 567–573. doi: 10.2460/javma.2004.225.567
Fossler, C. P., Wells, S. J., Kaneene, J. B., Ruegg, P. L., Warnick, L. D., Eberly, L. E., et al. (2005c). Cattle and environmental sample-level factors associated with the presence of Salmonella in a multi-state study of conventional and organic dairy farms. Prev. Vet. Med. 67, 39–53. doi: 10.1016/j.prevetmed.2004.10.005
Galiot, L., Monger, X. C., and Vincent, A. T. (2023). Studying the association between antibiotic resistance genes and insertion sequences in metagenomes: challenges and pitfalls. Antibiotics 12:175. doi: 10.3390/antibiotics12010175
Garmo, R. T., Waage, S., Sviland, S., Henriksen, B. I. F., Østerås, O., and Reksen, O. (2010). Reproductive performance, udder health, and antibiotic resistance in mastitis Bacteria isolated from Norwegian red cows in conventional and organic farming. Acta Vet. Scand. 52:11. doi: 10.1186/1751-0147-52-11
Genchi, C., Madonna, M., and Traldi, G. (1989). Epidemiology of Ostertagia ostertagi in dairy cow from different breeding systems. Parassitologia 31, 123–132.
Gilbert, W., Thomas, L. F., Coyne, L., and Rushton, J. (2021). Review: mitigating the risks posed by intensification in livestock production: the examples of antimicrobial resistance and zoonoses. Anim. Int. J. Anim. Biosci. 15:100123. doi: 10.1016/j.animal.2020.100123
Goldberg, J. J., Wildman, E. E., Pankey, J. W., Kunkel, J. R., Howard, D. B., and Murphy, B. M. (1992). The influence of intensively managed rotational grazing, traditional continuous grazing, and confinement housing on bulk tank Milk quality and udder health. J. Dairy Sci. 75, 96–104. doi: 10.3168/jds.S0022-0302(92)77743-1
Halbert, L. W., Kaneene, J. B., Ruegg, P. L., Warnick, L. D., Wells, S. J., Mansfield, L. S., et al. (2006). Evaluation of antimicrobial susceptibility patterns in Campylobacter spp isolated from dairy cattle and farms managed organically and conventionally in the midwestern and northeastern United States. J. Am. Vet. Med. Assoc. 228, 1074–1081. doi: 10.2460/javma.228.7.1074
Harper, G. C., and Makatouni, A. (2002). Consumer perception of organic food production and farm animal welfare. Br. Food J. 104, 287–299. doi: 10.1108/00070700210425723
Hemsworth, P. H., Barnett, J. L., Beveridge, L., and Matthews, L. R. (1995). The welfare of extensively managed dairy cattle: a review. Appl. Anim. Behav. Sci. 42, 161–182. doi: 10.1016/0168-1591(94)00538-P
Höglund, J., Dahlström, F., Engström, A., Hessle, A., Jakubek, E.-B., Schnieder, T., et al. (2010). Antibodies to major pasture borne helminth infections in bulk-tank milk samples from organic and nearby conventional dairy herds in south-Central Sweden. Vet. Parasitol. 171, 293–299. doi: 10.1016/j.vetpar.2010.04.002
Höglund, J., Viring, S., and Törnqvist, M. (2004). Seroprevalence of Dictyocaulus viviparus in first grazing season calves in Sweden. Vet. Parasitol. 125, 343–352. doi: 10.1016/j.vetpar.2004.07.018
Honorato, L. A., Machado Filho, L. C. P., Barbosa Silveira, I. D., and Hötzel, M. J. (2014). Strategies used by dairy family farmers in the south of Brazil to comply with organic regulations. J. Dairy Sci. 97, 1319–1327. doi: 10.3168/jds.2012-6532
Jauregi, L., Epelde, L., Alkorta, I., and Garbisu, C. (2021). Antibiotic resistance in agricultural soil and crops associated to the application of cow manure-derived amendments from conventional and organic livestock farms. Front. Vet. Sci. 8:633858. doi: 10.3389/fvets.2021.633858
Keyyu, J. D., Kassuku, A. A., Msalilwa, L. P., Monrad, J., and Kyvsgaard, N. C. (2006). Cross-sectional prevalence of helminth infections in cattle on traditional, small-scale and large-scale dairy farms in Iringa district, Tanzania. Vet. Res. Commun. 30, 45–55. doi: 10.1007/s11259-005-3176-1
Klein-Jöbstl, D., Sofka, D., Iwersen, M., Drillich, M., and Hilbert, F. (2016). Multilocus sequence typing and antimicrobial resistance of Campylobacter jejuni isolated from dairy calves in Austria. Front. Microbiol. 7:72. doi: 10.3389/fmicb.2016.00072
Kouřimská, L., Legarová, V., Panovská, Z., and Pánek, J. (2014). Quality of cows’ milk from organic and conventional farming. Czech J. Food Sci. 32, 398–405. doi: 10.17221/510/2012-cjfs
Kuhnen, S., Stibuski, R. B., Honorato, L. A., and Filho, L. C. P. M. (2015). Farm management in organic and conventional dairy production systems based on pasture in southern Brazil and its consequences on production and milk quality. Animals 5, 479–494. doi: 10.3390/ani5030367
Kuhnert, P., Dubosson, C. R., Roesch, M., Homfeld, E., Doherr, M. G., and Blum, J. W. (2005). Prevalence and risk-factor analysis of Shiga toxigenic Escherichia coli in faecal samples of organically and conventionally farmed dairy cattle. Vet. Microbiol. 109, 37–45. doi: 10.1016/j.vetmic.2005.02.015
Levison, L. J., Miller-Cushon, E. K., Tucker, A. L., Bergeron, R., Leslie, K. E., Barkema, H. W., et al. (2016). Incidence rate of pathogen-specific clinical mastitis on conventional and organic Canadian dairy farms. J. Dairy Sci. 99, 1341–1350. doi: 10.3168/jds.2015-9809
Licitra, F., Perillo, L., Antoci, F., Piccione, G., Giannetto, C., Salonia, R., et al. (2021). Management factors influence animal welfare and the correlation to infectious diseases in dairy cows. Animals 11:3321. doi: 10.3390/ani11113321
Liu, X., Li, J., Yang, X., Wang, D., Wang, J., and Wu, J. (2017). The seroprevalence of Mycobacterium avium subspecies paratuberculosis in dairy cattle in Xinjiang, Northwest China. Ir. Vet. J. 70:1. doi: 10.1186/s13620-016-0079-0
Macêdo, A. A. M., Oliveira, J. M. B., Silva, B. P., Borges, J. M., Soares, L. B. F., Silva, G. M., et al. (2018). Occurrence of mycoplasma bovigenitalium and Ureaplasma diversum in dairy cattle from to Pernambuco state, Brazil. Arq. Bras. Med. Vet. E Zootec. 70, 1798–1806. doi: 10.1590/1678-4162-10132
Manyazewal, A., Francesca, S., Pal, M., Gezahegn, M., Tesfaye, M., Lucy, M., et al. (2018). Prevalence, risk factors and molecular characterization of Cryptosporidium infection in cattle in Addis Ababa and its environs, Ethiopia. Vet. Parasitol. Reg. Stud. Rep. 13, 79–84. doi: 10.1016/j.vprsr.2018.03.005
May, K., Brügemann, K., König, S., and Strube, C. (2017a). Patent gastrointestinal nematode infections in organically and conventionally pastured dairy cows and their impact on individual milk and fertility parameters. Vet. Parasitol. 245, 119–127. doi: 10.1016/j.vetpar.2017.08.024
May, K., Brügemann, K., Yin, T., Scheper, C., Strube, C., and König, S. (2017b). Genetic line comparisons and genetic parameters for endoparasite infections and test-day milk production traits. J. Dairy Sci. 100, 7330–7344. doi: 10.3168/jds.2017-12901
Migliorini, P., and Wezel, A. (2017). Converging and diverging principles and practices of organic agriculture regulations and agroecology. A review. Agron. Sustain. Dev. 37:63. doi: 10.1007/s13593-017-0472-4
Mullen, K. A. E., Sparks, L. G., Lyman, R. L., Washburn, S. P., and Anderson, K. L. (2013). Comparisons of milk quality on North Carolina organic and conventional dairies. J. Dairy Sci. 96, 6753–6762. doi: 10.3168/jds.2012-6519
Müller, U., and Sauerwein, H. (2010). A comparison of somatic cell count between organic and conventional dairy cow herds in West Germany stressing dry period related changes. Livest. Sci. 127, 30–37. doi: 10.1016/j.livsci.2009.08.003
Novobilský, A., Sollenberg, S., and Höglund, J. (2015). Distribution of Fasciola hepatica in Swedish dairy cattle and associations with pasture management factors. Geospat. Health 9, 293–300. doi: 10.4081/gh.2015.351
Nüesch-Inderbinen, M., Hänni, C., Zurfluh, K., Hartnack, S., and Stephan, R. (2022). Antimicrobial resistance profiles of Escherichia coli and prevalence of extended-spectrum beta-lactamase-producing Enterobacteriaceae in calves from organic and conventional dairy farms in Switzerland. MicrobiologyOpen 11:e1269. doi: 10.1002/mbo3.1269
Olmos Antillón, G., Sjöström, K., Fall, N., Sternberg Lewerin, S., and Emanuelson, U. (2020). Antibiotic use in organic and non-organic Swedish dairy farms: a comparison of three recording methods. Front Vet Sci 7:568881. doi: 10.3389/fvets.2020.568881
Organically Produced Foods (2023). FAO. Available at: https://www.fao.org/family-farming/detail/en/c/288137/ (Accessed December 13, 2023).
Page, M. J., McKenzie, J. E., Bossuyt, P. M., Boutron, I., Hoffmann, T. C., Mulrow, C. D., et al. (2021). The PRISMA 2020 statement: an updated guideline for reporting systematic reviews. BMJ 372:n71. doi: 10.1136/bmj.n71
Papini, R., Nardoni, S., Fanelli, A., and Mancianti, F. (2009). High infection rate of Trichophyton verrucosum in calves from Central Italy. Zoonoses Public Health 56, 59–64. doi: 10.1111/j.1863-2378.2008.01157.x
Park, Y. K., Fox, L. K., Hancock, D. D., McMahan, W., and Park, Y. H. (2012). Prevalence and antibiotic resistance of mastitis pathogens isolated from dairy herds transitioning to organic management. J. Vet. Sci. 13, 103–105. doi: 10.4142/jvs.2012.13.1.103
Pieper, L., Sorge, U. S., DeVries, T., Godkin, A., Lissemore, K., and Kelton, D. (2015). Comparing ELISA test-positive prevalence, risk factors and management recommendations for Johne’s disease prevention between organic and conventional dairy farms in Ontario, Canada. Prev. Vet. Med. 122, 83–91. doi: 10.1016/j.prevetmed.2015.09.004
Pitta, D. W., Indugu, N., Toth, J. D., Bender, J. S., Baker, L. D., Hennessy, M. L., et al. (2020). The distribution of microbiomes and resistomes across farm environments in conventional and organic dairy herds in Pennsylvania. Environ. Microb. 15:21. doi: 10.1186/s40793-020-00368-5
Pol, M., and Ruegg, P. L. (2007a). Treatment practices and quantification of antimicrobial drug usage in conventional and organic dairy farms in Wisconsin. J. Dairy Sci. 90, 249–261. doi: 10.3168/jds.S0022-0302(07)72626-7
Pol, M., and Ruegg, P. L. (2007b). Relationship between antimicrobial drug usage and antimicrobial susceptibility of gram-positive mastitis pathogens. J. Dairy Sci. 90, 262–273. doi: 10.3168/jds.S0022-0302(07)72627-9
Rapp, D., Ross, C. M., Cave, V., and Muirhead, R. W. (2014). Prevalence, concentration and genotypes of Campylobacter jejuni in faeces from dairy herds managed in farm systems with or without housing. J. Appl. Microbiol. 116, 1035–1043. doi: 10.1111/jam.12425
Raquel Salazar, B., Rolando Barahona, R., Julián Chará, O., and Pinzon, M. S. S. (2015). Productivity and tick load in Bos indicus X B. taurus cattle in a tropical dry forest silvopastoral system. Trop. Subtrop. Agroecosyst. 18, 103–112,
Ray, K. A., Warnick, L. D., Mitchell, R. M., Kaneene, J. B., Ruegg, P. L., Wells, S. J., et al. (2006). Antimicrobial susceptibility of Salmonella from organic and conventional dairy farms. J. Dairy Sci. 89, 2038–2050. doi: 10.3168/jds.S0022-0302(06)72271-8
Richert, R. M., Cicconi, K. M., Gamroth, M. J., Schukken, Y. H., Stiglbauer, K. E., and Ruegg, P. L. (2013). Risk factors for clinical mastitis, ketosis, and pneumonia in dairy cattle on organic and small conventional farms in the United States. J. Dairy Sci. 96, 4269–4285. doi: 10.3168/jds.2012-5980
Roberts, M. C., Garland-Lewis, G., Trufan, S., Meschke, S. J., Fowler, H., Shean, R. C., et al. (2018). Distribution of Staphylococcus species in dairy cows, workers and shared farm environments. FEMS Microbiol. Lett. 365:fny146. doi: 10.1093/femsle/fny146
Roesch, M., Doherr, M. G., Schären, W., Schällibaum, M., and Blum, J. W. (2007). Subclinical mastitis in dairy cows in Swiss organic and conventional production systems. J. Dairy Res. 74, 86–92. doi: 10.1017/S002202990600210X
Roesch, M., Perreten, V., Doherr, M. G., Schaeren, W., Schällibaum, M., and Blum, J. W. (2006). Comparison of antibiotic resistance of udder pathogens in dairy cows kept on organic and on conventional farms. J. Dairy Sci. 89, 989–997. doi: 10.3168/jds.S0022-0302(06)72164-6
Sato, K., Bartlett, P. C., Erskine, R. J., and Kaneene, J. B. (2005). A comparison of production and management between Wisconsin organic and conventional dairy herds. Livest. Prod. Sci. 93, 105–115. doi: 10.1016/j.livprodsci.2004.09.007
Sato, K., Bartlett, P. C., Kaneene, J. B., and Downes, F. P. (2004). Comparison of prevalence and antimicrobial susceptibilities of Campylobacter spp. isolates from organic and conventional dairy herds in Wisconsin. Appl. Environ. Microbiol. 70, 1442–1447. doi: 10.1128/AEM.70.3.1442-1447.2004
Sato, K., Bartlett, P. C., and Saeed, M. A. (2005). Antimicrobial susceptibility of Escherichia coli isolates from dairy farms using organic versus conventional production methods. J. Am. Vet. Med. Assoc. 226, 589–594. doi: 10.2460/javma.2005.226.589
Schmidt, J. W., Vikram, A., Arthur, T. M., Belk, K. E., Morley, P. S., Weinroth, M. D., et al. (2020). Antimicrobial resistance at two U.S. cull cow processing establishments. J. Food Prot. 83, 2216–2228. doi: 10.4315/JFP-20-201
Seufert, V., Ramankutty, N., and Mayerhofer, T. (2017). What is this thing called organic? – how organic farming is codified in regulations. Food Policy 68, 10–20. doi: 10.1016/j.foodpol.2016.12.009
Shepon, A., Wu, T., Kremen, C., Dayan, T., Perfecto, I., Fanzo, J., et al. (2023). Exploring scenarios for the food system-zoonotic risk interface. Lancet Planet Health 7, e329–e335. doi: 10.1016/S2542-5196(23)00007-4
Shirima, G. M., Kazwala, R. R., and Kambarage, D. M. (2003). Prevalence of bovine tuberculosis in cattle in different farming systems in the eastern zone of Tanzania. Prev. Vet. Med. 57, 167–172. doi: 10.1016/S0167-5877(02)00214-3
Shrivas, V. L., Choudhary, A. K., Hariprasad, P., and Sharma, S. (2023). Nutrient concentrations affect the antimicrobial resistance profiles of cattle manures. Environ. Sci. Pollut. Res. 30, 25141–25147. doi: 10.1007/s11356-021-16700-6
Silva, J. B., Fagundes, G. M., Soares, J. P. G., Fonseca, A. H., and Muir, J. P. (2014). A comparative study of production performance and animal health practices in organic and conventional dairy systems. Trop. Anim. Health Prod. 46, 1287–1295. doi: 10.1007/s11250-014-0642-1
Silverlås, C., and Blanco-Penedo, I. (2013). Cryptosporidium spp. in calves and cows from organic and conventional dairy herds. Epidemiol. Infect. 141, 529–539. doi: 10.1017/S0950268812000830
Silverlås, C., Emanuelson, U., de Verdier, K., and Björkman, C. (2009). Prevalence and associated management factors of Cryptosporidium shedding in 50 Swedish dairy herds. Prev. Vet. Med. 90, 242–253. doi: 10.1016/j.prevetmed.2009.04.006
Sjöström, K., Hickman, R. A., Tepper, V., Antillón, G. O., Järhult, J. D., Emanuelson, U., et al. (2020). Antimicrobial resistance patterns in organic and conventional dairy herds in Sweden. Antibiotics 9, 1–17. doi: 10.3390/antibiotics9110834
Sorge, U. S., Moon, R. D., Stromberg, B. E., Schroth, S. L., Michels, L., Wolff, L. J., et al. (2015). Parasites and parasite management practices of organic and conventional dairy herds in Minnesota. J. Dairy Sci. 98, 3143–3151. doi: 10.3168/jds.2014-9031
Suvajdžić, B., Teodorović, V., Vasilev, D., Karabasil, N., Dimitrijević, M., Dordević, J., et al. (2017). Detection of ICAA and ICAD genes of Staphylococcus aureus isolated in cases of bovine mastitis in the republic of Serbia. Acta Vet. 67, 168–177. doi: 10.1515/acve-2017-0015
Svensson, C., Hessle, A., and Höglund, J. (2000). Parasite control methods in organic and conventional dairy herds in Sweden. Livest. Prod. Sci. 66, 57–69. doi: 10.1016/S0301-6226(00)00155-X
Swai, E. S., and Schoonman, L. (2012). Differences in prevalence of tuberculosis in indigenous and crossbred cattle under extensive and intensive management systems in Tanga region of Tanzania. Trop. Anim. Health Prod. 44, 459–465. doi: 10.1007/s11250-011-9919-9
Takeuchi-Storm, N., Denwood, M., Petersen, H. H., Enemark, H. L., Stensgaard, A.-S., Sengupta, M. E., et al. (2018). Patterns of Fasciola hepatica infection in Danish dairy cattle: implications for on-farm control of the parasite based on different diagnostic methods. Parasit. Vectors 11:674. doi: 10.1186/s13071-018-3248-z
Taponen, S., Liski, E., Heikkilä, A.-M., and Pyörälä, S. (2017). Factors associated with intramammary infection in dairy cows caused by coagulase-negative staphylococci, Staphylococcus aureus, Streptococcus uberis, Streptococcus dysgalactiae, Corynebacterium bovis, or Escherichia coli. J. Dairy Sci. 100, 493–503. doi: 10.3168/jds.2016-11465
Temple, D., and Manteca, X. (2020). Animal welfare in extensive production systems is still an area of concern. Front. Sustain. Food Syst. 4:545902. doi: 10.3389/fsufs.2020.545902
Tenhagen, B.-A., Alt, K., Pfefferkorn, B., Wiehle, L., Käsbohrer, A., and Fetsch, A. (2018). Short communication: methicillin-resistant Staphylococcus aureus in conventional and organic dairy herds in Germany. J. Dairy Sci. 101, 3380–3386. doi: 10.3168/jds.2017-12939
Terefe, Y., Girma, S., Mekonnen, N., and Asrade, B. (2017). Brucellosis and associated risk factors in dairy cattle of eastern Ethiopia. Trop. Anim. Health Prod. 49, 599–606. doi: 10.1007/s11250-017-1242-7
Tikofsky, L. L., Barlow, J. W., Santisteban, C., and Schukken, Y. H. (2003). A comparison of antimicrobial susceptibility patterns for Staphylococcus aureus in organic and conventional dairy herds. Microb. Drug Resist. Larchmt N 9 Suppl 1, S39–S45. doi: 10.1089/107662903322541883
Silva Neto, A. L., Silva, B. P., Lúcio, É. C., Nascimento, S. A., and Pinheiro Júnior, J. W. (2021). Soroepidemiological survey of chlamydia abortus infection in bovine in the state of Pernambuco, Brazil. Semin. Cienc Agrar. 42, 229–240. doi: 10.5433/1679-0359.2021v42n1p229
Varsaki, A., Ortiz, S., Santorum, P., López, P., López-Alonso, V., Hernández, M., et al. (2022). Prevalence and population diversity of Listeria monocytogenes isolated from dairy cattle farms in the Cantabria region of Spain. Animals 12:2477. doi: 10.3390/ani12182477
von Keyserlingk, M., Rushen, J., de Passillé, A. M., and Weary, D. M. (2009). Invited review: the welfare of dairy cattle--key concepts and the role of science. J. Dairy Sci. 92, 4101–4111. doi: 10.3168/jds.2009-2326
Wagner, K., Brinkmann, J., Bergschmidt, A., Renziehausen, C., and March, S. (2021). The effects of farming systems (organic vs. conventional) on dairy cow welfare, based on the welfare quality® protocol. Animal 15:100301. doi: 10.1016/j.animal.2021.100301
Walk, S. T., Mladonicky, J. M., Middleton, J. A., Heidt, A. J., Cunningham, J. R., Bartlett, P., et al. (2007). Influence of antibiotic selection on genetic composition of Escherichia coli populations from conventional and organic dairy farms. Appl. Environ. Microbiol. 73, 5982–5989. doi: 10.1128/AEM.00709-07
Winkelstein, W. (2009). The remarkable Archie: origins of the Cochrane collaboration. Epidemiol. Camb. Mass. 20:779. doi: 10.1097/EDE.0b013e3181aff391
Wolff, C., Emanuelson, U., Ohlson, A., Alenius, S., and Fall, N. (2015). Bovine respiratory syncytial virus and bovine coronavirus in Swedish organic and conventional dairy herds. Acta Vet. Scand. 57:2. doi: 10.1186/s13028-014-0091-x
Keywords: intensive, extensive, organic, dairy farm, infectious disease, infectious agents, antimicrobial resistance
Citation: Pajoro M, Brilli M, Pezzali G, Vadalà M, Kramer L, Moroni P and Bandi C (2024) Do organic, conventional, and intensive approaches in livestock farming have an impact on the circulation of infectious agents and antimicrobial resistance? A systematic review, focused on dairy cattle. Front. Sustain. Food Syst. 8:1397095. doi: 10.3389/fsufs.2024.1397095
Edited by:
Fatima Zahra Jawhari, Higher Institute of Nursing and Health Techniques, MoroccoReviewed by:
Mohammed Bassouya, Sidi Mohamed Ben Abdellah University, MoroccoAbdelkrim Agour, Sidi Mohamed Ben Abdellah University, Morocco
Copyright © 2024 Pajoro, Brilli, Pezzali, Vadalà, Kramer, Moroni and Bandi. This is an open-access article distributed under the terms of the Creative Commons Attribution License (CC BY). The use, distribution or reproduction in other forums is permitted, provided the original author(s) and the copyright owner(s) are credited and that the original publication in this journal is cited, in accordance with accepted academic practice. No use, distribution or reproduction is permitted which does not comply with these terms.
*Correspondence: Claudio Bandi, Y2xhdWRpby5iYW5kaUB1bmltaS5pdA==