- 1College of Food and Biological Engineering, Chengdu University, Chengdu, China
- 2Institute of Quality Standards and Testing Technology for Agro-Products, Chinese Academy of Agricultural Sciences, Beijing, China
Fresh-cut fruit has been popularized for use by consumers in recent years, but its shelf life is very short. Plant essential oils have received attention for their bioactive properties, such as antibacterial and antioxidant properties, but their volatile nature limited their application in foods. In this study, the bacteriostasis of lemon essential oil (LEO) was verified, and LEO nanoemulsion was prepared to preserve fresh-cut kiwifruit. Compared with those of gram-negative bacteria, the inhibition zone diameters of LEO against gram-positive bacteria were significantly greater, measuring 12.20 ± 0.67 mm (Staphylococcus aureus) and 14.23 ± 0.61 mm (Listeria monocytogenes), compared to 9.50 ± 1.08 mm (Escherichia coli). At a concentration of 50 mg/mL, LEO exhibited DPPH and ABTS radical scavenging rates of 84.88 and 83.01%, respectively, which were comparable to those of 10 mg/L vitamin C (VC). Konjac glucomannan, whey protein and LEO were used to prepare nanoemulsion. When 2.0% LEO was added, the nanoemulsion exhibited great stability, which resulted in a decrease in particle size to 220.80 nm, a decrease in PDI to 0.238, and an increase in zeta potential to-27.83 mV. The stability of the nanoemulsion with added LEO was superior to that of the nanoemulsion without LEO on the 15th day. Preservation tests on fresh-cut kiwifruit demonstrated that, on the 8th day, compared with no treatment, nanoemulsion containing 2.0% LEO reduced the weight loss rate from 3.20 to 1.16%, increased the firmness from 20.459 to 38.23, and decreased the decay rate from 80.69 to 41.74% compared to untreated kiwifruit. It also effectively maintained the original color of fresh-cut kiwifruit and had positive effects on the soluble solid content, titratable acid content, VC content, and total colony count. LEO has great bacteriostatic and antioxidant activity, and the use of LEO nanoemulsions on fresh-cut kiwifruit can effectively maintain various indicators of fresh kiwifruit after a 8-day storage period.
1 Introduction
Fresh fruits are indispensable foods in people’s daily life. Fresh-cut fruits are minimally processed fruits that are widely welcomed by consumers, especially those at food service establishments, schools and company cafeterias (Li et al., 2021), for their convenience, high nutrition value and freshness. However, after mechanical treatment (such as being sliced), the fruit’s own barrier will be destroyed, leading to increased respiratory intensity, enzyme activity and microbial reproduction rate, all of which result in rapid deterioration of the fruit (Tapia et al., 2008). In view of the perishable nature of postharvest fruits, how to store them safely, economically, and efficiently is a continuous challenge for the food industry.
Kiwifruit is a of the famous tropical seasonal fruit produced worldwide and is popular due to its unique flavor and high nutritional value (Shao et al., 2022). Kiwifruit is a perishable food that have a short shelf life, and start to decrease in quality immediately after harvesting (Yang et al., 2024). Usually, kiwifruit slices are consumed fresh as a salad or in confectionery (Manzoor et al., 2021). To extend the shelf life of fresh-cut fruits, recent research has focus mainly on chemical preservation (Ali et al., 2024), air-condition preservation (Gorzelany et al., 2022), ultraviolet light preservation (Liu et al., 2023) and biological preservation (Song et al., 2024). Plant essential oil is a biological preservative contain bioactive substances and meet consumer demands for fewer chemical additives and safety. The Food and Drug Administration recognizes essential oils as Generally Recognized as Safe (GRAS) (U. S. Food and Drug Administration, 2019). Therefore, they have been widely studied by researchers in recent years (Yang et al., 2023). However, using essential oil alone as a means of preservation will lead to changes in food flavor, and the volatility and hydrophobicity of essential oil are its limitations as a preservation technology (Yousuf et al., 2021).
Encapsulating lipophilic compounds in nanoemulsions has emerged as an effective approach to overcome the limitations of insolubility, volatility, and strong odors of essential oil (Meng et al., 2022a). Nanoemulsions can efficiently encapsulate, protect, and deliver lipophilic compounds (Meng et al., 2020). Additionally, the nanoemulsions offer several advantages, such as small size, minimal polydispersity, slow release of bioactive chemicals (Meng et al., 2022b). Therefore, nanoemulsions can serve as an effective system to enhance the physical stability and biological activity of essential oils. Researchers have been dedicated to designing essential oil nanoemulsions for food processing and preservation purposes. For example, previous studies confirmed that the antimicrobial efficacy of garlic essential oil was enhanced by emulsification (Katata-Seru et al., 2017). In recent years, plant essential oil nanoemulsions have shown strong potential in the food preservation industry. Several essential oil nanoemulsions can maintain the original color of food (Lee et al., 2019) and control the release of active ingredients in food, enhancing the efficacy of bioactive substances (Gardesh et al., 2016). Therefore, in this study, the antibacterial and antioxidant effects of lemon essential oil (LEO) were evaluated. To overcome the limitations of volatility and hydrophobicity LEO, two natural wall material (konjac glucomannan, whey protein) were chosen to prepare nanoemulsions, and the preservation effect of LEO nanoemulsion on fresh-cut kiwifruit were systematically studied.
2 Materials and methods
2.1 Materials
Kiwifruits were purchased from local suppliers in Sichuan, China. The plants were selected for their uniform size, shape, color, and ripening stage without injury and decay. Lemon essential oil (LEO) was purchased from Sichuan Anyue County Lvyuan Lemon Development Co., Ltd. Escherichia coli, Staphylococcus aureus, and Listeria monocytogenes were obtained from Beijing Biowe Technology Co., Ltd. Dimethyl sulfoxide (DMSO) was purchased from Shanghai Yuanye Bio-Technology Co., Ltd. 2,2-Diphenyl-1-picrylhydrazyl (DPPH) was purchased from Sigma Aldrich (Shanghai) Trading Co., Ltd. 2,2′-azino-bis (ABTS) was purchased from Shanghai Hualan Chemical Technology Co., Ltd. KGM (Mw = 2.895 × 106 g/mol, 95.0% purity) was supplied by the Anfu Konjac Development Co., Ltd. (Mianyang, China). All other chemicals were of analytical grade.
2.2 Antibacterial activity of LEO
2.2.1 Strain preparation
E. coli and S. aureus were cultured in Luria-Bertani (LB) liquid medium (1% of tryptone, 0.5% of yeast extract, 1% of sodium chloride, pH 7.2–7.4) at 37°C with shaking of 100 r/min. L. monocytogenes was cultured in tryptic soy broth (TSB) medium (1.7% of tryptone, 0.3% of soy peptone, 0.5% of sodium chloride, 0.25% of dipotassium phosphate, 0.25% of glucose, pH = 7.1–7.5) at 37°C with shaking of 100 r/min. All the strains were cultured to the log phase, and the bacterial dilutions were obtained by adjusting the final cell density to 107 CFU/mL according to OD600-cell count standard curve, and then the bacterial dilutions were used for subsequent experiments.
2.2.2 Determination of the minimum bacteriostatic concentration and the minimal bactericidal concentration
The MIC and MBC of LEO was determined according to previous study (Yazgan, 2020) with slightly modification: 1.5% DMSO was added to the LB liquid medium or TSB liquid medium. Then, different amounts of LEO were added to achieve final concentrations of 0.08, 0.16, 0.31, 0.63, 1.25, 2.50, 5.00, 10.00, 20.00, 40.00, 60.00, and 80.00 μL/mL. Only the tubes containing DMSO were used as the control group, while only the tubes containing culture medium were used as the blank group. One hundred microliters of the bacterial dilutions was added, and the mixture was cultured at 37°C for 24 h. The MIC values represent the lowest concentration of the sample that inhibited visible growth of the tested microorganism. To determine the MBC, the contents of the tubes with the MIC were subcultured onto solid medium and incubated at 37°C. The minimal concentration of the samples that showed no growth after 24 h of incubation was considered as the MBC.
2.2.3 Determination of the inhibitory zone of LEO
The agar diffusion test was slightly modified (Luo et al., 2023) and used to determine the antibacterial activity of LEO in vitro. The Petri plate medium (liquid medium supplemented with 1.5% agar) surface was inoculated by spreading 10 μL of the bacterial dilutions over the entire surface of the agar. After air-drying, three filter paper discs with a diameter of 8 mm were placed on each culture medium, ensuring a certain distance between the discs. Ten microliters of LEO was pipetted onto the filter paper discs at 37°C for 24 h. The essential oil diffused from the center to the outer edge of the agar plate, forming transparent inhibitory zones. The blank group was not treated with LEO. The diameter of the inhibitory zones was measured. The standards for determining the bacteriostatic effect were as follows: “highly sensitive”: diameter ≥ 20 mm; “medium sensitive”: 20 mm ≥ diameter ≥ 12 mm; and “slightly sensitive”: diameter ≤ 12 mm.
2.2.4 Growth curve
According to previous methods with minor modifications (Costa et al., 2022), E. coli, S. aureus, and L. monocytogenes culture mediums were mixed with 1.5% of DMSO, and then the culture mediums were mixed with LEO at concentrations of 2 MIC, MIC, 0.5 MIC, respectively. For the blank group: only E. coli culture medium was used, while for the S. aureus culture medium, L. monocytogenes culture medium was used. In the control group, E. coli, S. aureus, and L. monocytogenes culture mediums were mixed with 1.5% of DMSO. Then the cells were cultured at 37°C with shaking, and the OD600 values of each group were measured at 0, 2, 4, 6, 8, 10, 12, and 24 h, respectively, by using a 1,550 microplate reader (Thermo Scientific, MA, United States).
2.3 The DPPH and ABTS radical scavenging ability of LEO
DPPH and ABTS radical scavenging ability of LEO was determined according to previous study (Wang et al., 2023) with some modification: LEO was diluted to different concentrations in sample solution with absolute ethanol (10, 20, 30, 40, 50 mg/mL). The control group was 10 mg/mL VC solution. One hundred microliters of sample was mixed with 100 μL of 0.01 mM DPPH methanol solution and incubated at room temperature in the dark for 30 min. The absorbance of the sample was measured at 517 nm using a Synergy H1 microplate reader (BioTek, VT, USA). The results were calculated by Formula (1):
where A0 is the absorbance value of the ultrapure water, A1 is the absorbance value of the sample with DPPH solution, and A2 is the absorbance value of the sample with ultrapure water. VC was used as a positive reference antioxidant in the DPPH radical scavenging test.
An equal volume of 7 mM ABTS solution and 2.45 mM K2S2O8 were mixed and incubated in the dark for 14 h. The mixture was diluted with phosphate buffer (0.2 M, pH = 7.4) to an absorbance of 0.70 ± 0.02 at 734 nm. Thirty microliters of sample was mixed with 170 μL of ABTS solution and incubated at 15°C and in the dark for 6 min. The absorbance was measured at 734 nm. The results were calculated by Formula (2):
where A0 is the absorbance value of the ultrapure water, and A1 is the absorbance value of the sample with ABTS solution. VC was used as a positive reference antioxidant in the ABTS radical scavenging test.
2.4 Preparation of LEO nanoemulsions
Aqueous phase: 0.3% of KGM was mixed with ultrapure water for swelling at 60°C for 30 min. The mixture was subsequently mixed with 2% whey protein, stirred them at 25°C for 1 h, and preserve them at 4° C for preparation.
The aqueous phase and 0.5, 1.0, 1.5, and 2.0% of LEO were mixed, and the mixture was subsequently homogenized for 3 min (10,000 r/min). Coarse emulsions were placed on a GJJ-60 high-pressure homogenizer (Zhengzhou Yuxiang Machinery Equipment Co., Ltd., China) to make LEO nanoemulsions (30 MPa, 90 s).
2.5 Average particle size, polydispersity index, zeta potential, and state of the LEO nanoemulsion
LEO nanoemulsions were diluted (1:100) with ultrapure water. A ZEN3600 nanoparticle size analyzer (Malvern, Britain) with a He-Ne laser (633 nm) and 90° collecting optical element were used to measure the average diameter, PDI and zeta potential of the LEO nanoemulsion on Days 0 and 15.
The LEO nanoemulsions were stored at room temperature for 15 days, after which the state of the nanoemulsions were observed on Days 0 and 15.
2.6 Effects of LEO nanoemulsions on the fresh-cut kiwifruit storage
2.6.1 Preparation and coating on fresh-cut kiwifruit
Kiwifruits were preserved at 4°C until they reached the edible stage (soluble solids content ≥10%), after which they were subjected to fresh-cut processing (Hu et al., 2022). Undamaged samples (90 ± 5 g) of a uniform size, at the edible stage, were rinsed. Kiwifruit tissue was cut into 1 cm thick thin slices using a stainless steel knife after peeling. The slices were randomly divided into 6 groups: the blank group was soaked in ultrapure water. The control group was soaked in an equal concentration of nanoemulsion without embedding essential oils. 0.5, 1.0, 1.5, and 2.0% LEO nanoemulsion groups were soaked in 0.5, 1.0, 1.5, and 2.0%(v/v) LEO nanoemulsion, respectively. Both samples were air-dried for 10 min after soaking in different concentrations of nanoemulsion for 3 min. Afterward, both samples were stored in plastic containers at 4°C for 8 days. During storage, the samples were sampled at 2-d intervals for analysis.
2.6.2 Weight loss
The weight loss rate was determined by the weighing method, and the samples were weighed once every 2 days during storage. The weight loss rate was calculated by Formula (3):
where Pi is the weight of the sample before storage (g) and Pf is the weight of the sample after storage (g).
2.6.3 Firmness
The firmness of kiwifruit was measured with a TA-XT plusC texture instrument (StableMicro System, Godalming, United Kingdom). The parameter settings were as follows: 2 mm probe with a puncture speed of 1 mm−1 and a puncture distance of 5 mm. The firmness was the maximum force (N) required to puncture the slices.
2.6.4 Color
The color values (a* and L*) of the samples were measured from 3 random points on each sample using an NH300 colorimeter (Threenh Technology Co., Ltd., China) during the storage period. Color values were determined by taking the average of these points.
The total chlorophyll content was analyzed using commercial assay kits according to the manufacturer’s instructions (Nanjing Jiancheng Biological Engineering Research Institute, China).
2.6.5 Decay rate
The decay rate was assessed by visually inspecting the presence of spoilage on the surface of kiwifruit slices at the beginning and end of each storage interval. Visually spoiled slices, characterized by changes in color, visible structural integrity, and appearance, were removed when approximately 70% of the fruit surface area decayed. The decay rate was calculated as the number of decayed slices divided by the initial number of slices, multiplied by 100.
2.6.6 Determination of soluble solids content titratable acid content and pH
The SCC of the kiwifruit was determined using a PAL-1 Akabe refractometer (ATAGO CO., Ltd., Guangzhou, China), and the results are expressed as percentages.
TA was determined by the titration method. Ten grams of sample was homogenized and subsequently diluted to 100 mL with distilled water. Then the samples were filtered for 30 min to collect the filtrate. Three to five drops of 1% phenolphthalein indicator were added to 25 mL of filtrate. The mixture was stirred and titrated with 0.1 M NaOH solution until the solution turned pink and did not fade within 30 s. The conversion Factor f (lemon acid) is based on the consumption of NaOH titrant and the results are expressed as the mass fraction (%) of the titratable content of the sample (Su et al., 2023). The TA content was calculated by Formula (4)
where V is the total volume of extraction solution (mL); C is the molar concentration of NaOH (mol/L); V1 is the volume of NaOH consumed by the filtrate (mL); V0 is the volume of NaOH consumed by distilled water (mL); VS is the volume of extraction solution (mL); W is the weight of the samples (g); the f is the conversion factor (g/mmol).
After the kiwifruit slices were homogenized, use a PHSJ-4F pH meter (INASE Scientific Instrument Co., Ltd., Shanghai, China) to measure the pH of the sample.
2.6.7 VC content and DPPH radical scavenging ability of fresh-cut kiwifruit
VC was analyzed using commercial assay kits according to the manufacturer’s instructions (Nanjing Jiancheng Biological Engineering Research Institute, China).
Five grams of kiwifruit were homogenized in an ice bath, then it was centrifuged at 4°C, and 10,000 × g for 10 min and collected as a test sample. The DPPH radical scavenging ability of the kiwifruit was determined according to the methods of section 2.3.
2.6.8 Antioxidant enzyme activity
Peroxidase (POD), catalase (CAT), phenylalaninammo-nialyase (PAL), and polyphenol oxidase (PPL) were analyzed using commercial assay kits according to the manufacturer’s instructions (Nanjing Jiancheng Biological Engineering Research Institute, China).
2.6.9 Microbiological analysis
According to the Chinese national standard GB4789.2—2016, the total colony count of freshly cut kiwifruit on the 8th day of storage was determined and expressed as log10 (CFU/g).
2.7 Statistical analysis
The data are reported as means ± standard deviations (SD). Statistical computations and analyses were conducted using SPSS 26.0 (IBM Analytics, Tulsa, United States). Data were subjected to analysis of variance (ANOVA), and Tukey’s HSD test was carried out to compare the means. Differences were considered significant at p < 0.05 and are represented by different lowercase letters.
3 Results and discussion
3.1 Antibacterial activity of LEO
3.1.1 Inhibitory zone
As shown in Figure 1A and Table 1, the studied LEOs act differently on the tested bacteria, L. monocytogenes has the best antibacterial effect, with an antibacterial circle diameter of 14.23 ± 0.61 mm, followed by S. aureus (12.20 ± 0.67 mm), both of which are highly sensitive; however, LEO has the worst antibacterial effect on E. coli (9.50 ± 1.08 mm), which is slightly sensitive and significantly less than the antibacterial effect of LEO on the other two kinds of bacteria. One possible reason is that limonene, a main volatile component of LEO, can damage the cell membranes of S. aureus and L. monocytogenes, leading to the leakage of biomolecules from the cells (Han et al., 2021), while the outer membranes of gram-negative bacteria are rich in lipopolysaccharides and are almost unable to penetrate lipophilic compounds, thus preventing the penetration of various antibacterial components of the essential oil. The presence of hydrolases in the periplasmic space may also help hydrolyze antibacterial substances. However, LEO can still act on gram-negative bacteria because other volatile substances, such as γ-terpinene and β-pinene in LEO still have antibacterial effects on E. coli (Tang et al., 2023), these two small molecular compounds can act on gram-negative bacteria through a sufficiently large enough channel generated by porins in the outer membrane (Seow et al., 2014).
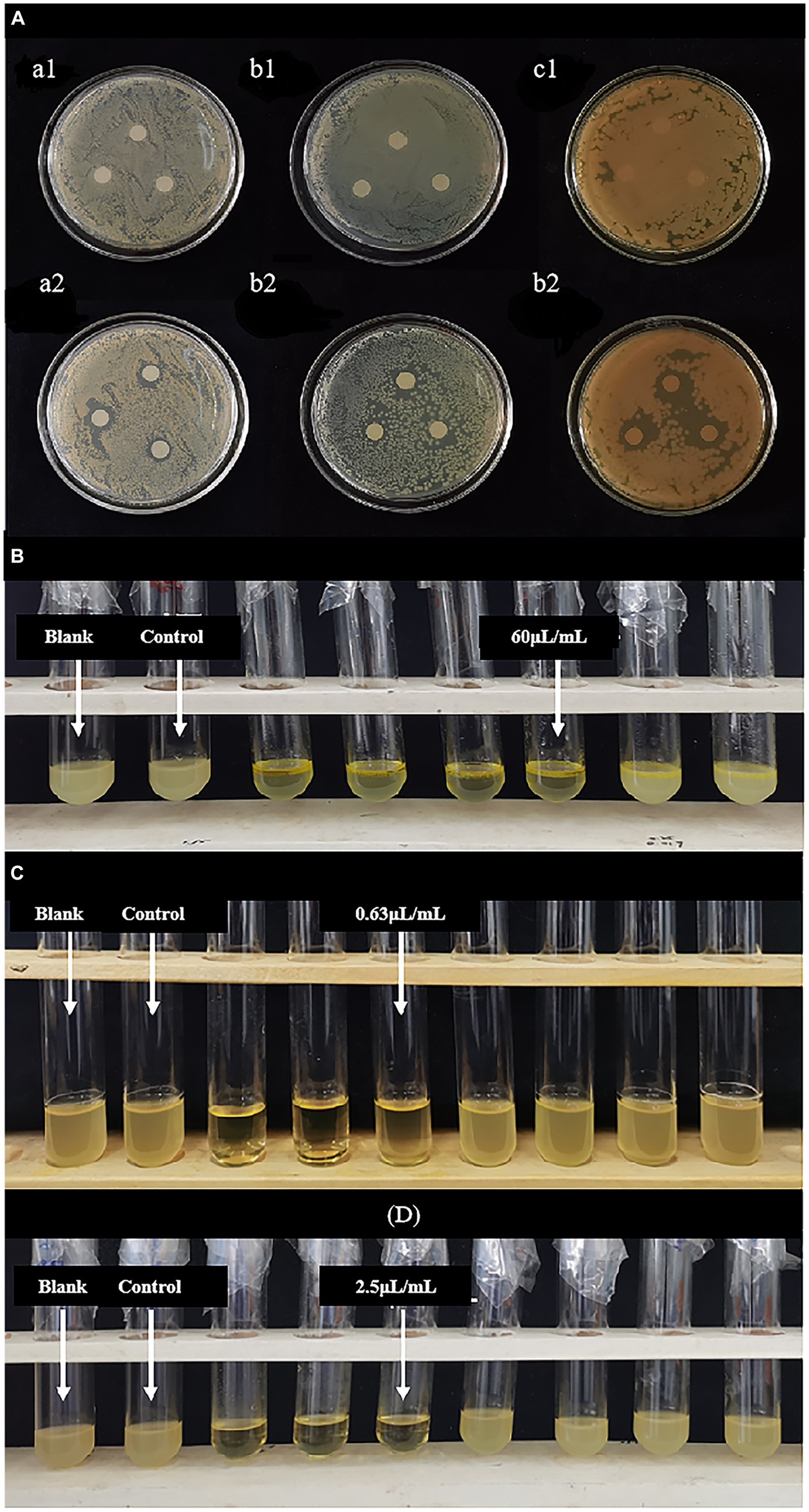
Figure 1. (A) Inhibitory zone of LEO. (a1,a2) Blank group against Staphylococcus aureus and LEO group against Staphylococcus aureus; (b1,b2) blank group against Escherichia coli and LEO group against Escherichia coli; (c1,c2) blank group against Listeria monocytogenes and LEO group against Listeria monocytogenes; (B) MIC and MBC of LEO against Escherichia coli; (C) MIC and MBC of LEO against Staphylococcus aureus; (D) MIC and MBC of LEO against Listeria monocytogenes.
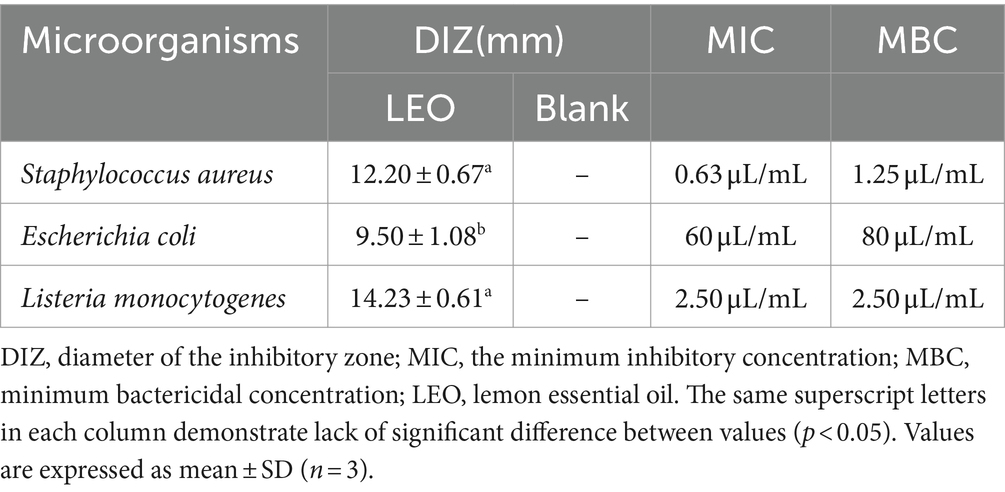
Table 1. Diameter (mm) of the inhibitory zone and the MIC, MBC of LEO against Staphylococcus aureus, Escherichia coli, and Listeria monocytogenes.
3.1.2 MIC and MBC of LEO
As shown in Figures 1B–D and Table 1, the MIC and MBC of LEO against S. aureus were determined to be 0.63 μL/mL and 1.25 μL/mL, respectively. For E. coli, the MIC and MBC of LEO were found to be 60 μL/mL and 80 μL/mL, respectively. LEO exhibited an MIC and an MBC of 2.50 μL/mL against L. monocytogenes. In this study, compared to the gram-negative bacteria (E. coli), the gram-positive bacteria (S. aureus and L. monocytogenes) exhibited greater sensitivity and lower concentrations required for inhibition by LEO. The findings are consistent with the results of the agar diffusion test and the conclusions of He et al. (2022).
3.1.3 Growth curve
Although the MIC and MBC are important for evaluating the antibacterial activity of LEO, the results of these analyses are static. Therefore, it is necessary to dynamically assess the activity over time. As shown in Figure 2A, the bacterial counts in the blank and control groups rapidly increased logarithmically with extended cultivation time, and the bacteria entered a stable phase after 12 h. LEO exhibited bactericidal activity against S. aureus at 2 MIC (1.25 μL/mL) and significant growth inhibition at the MIC (0.63 μL/mL). The lag phase of the growth curve of S. aureus treated with LEO was observed, with a lag phase of 6 h for MIC-treated S. aureus, and 4 h for the 1/2 MIC, 1/4 MIC, control, and blank groups.
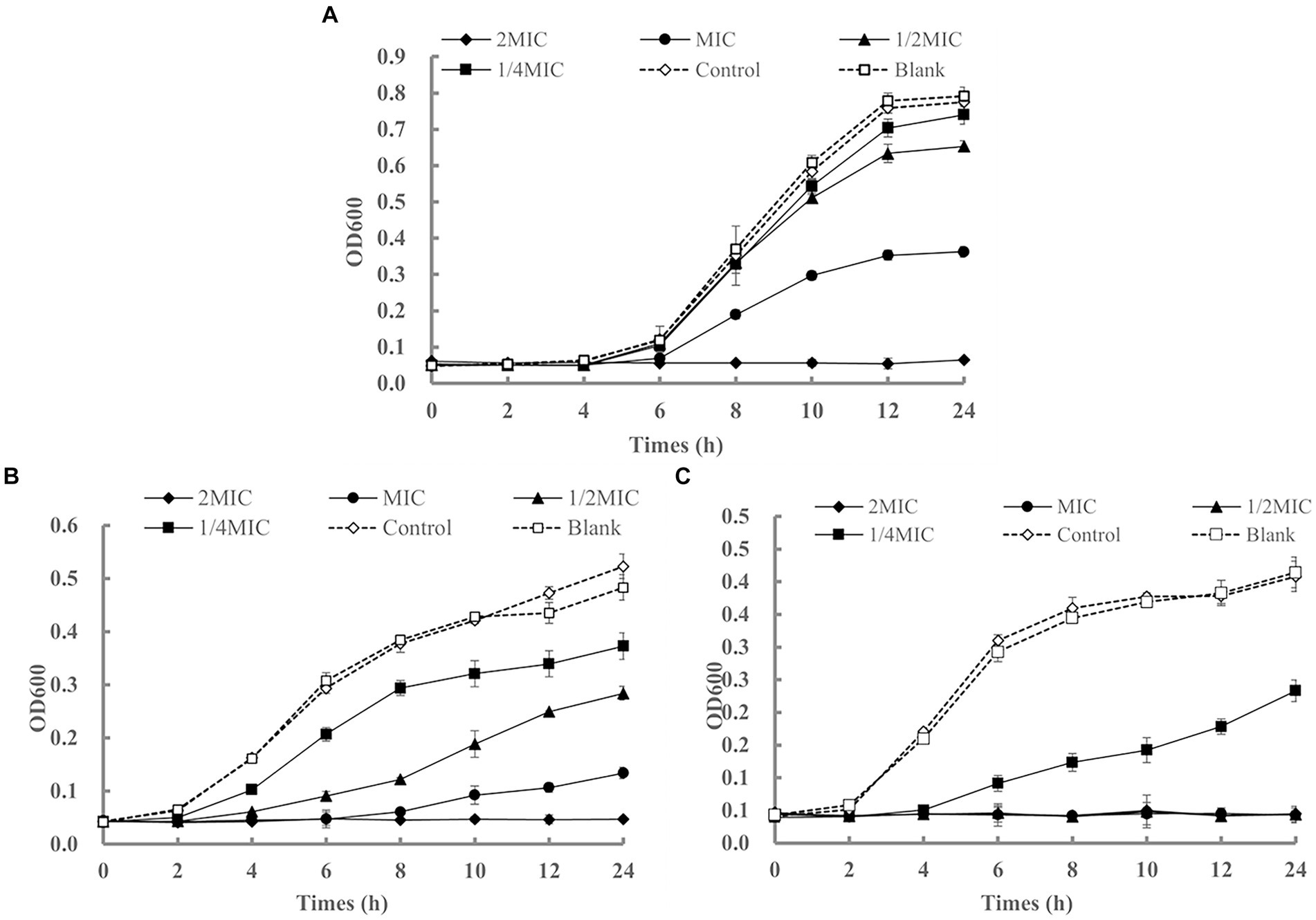
Figure 2. The growth curves of Staphylococcus aureus (A), Escherichia coli (B), and Listeria monocytogenes (C) affected by LEO.
As shown in Figure 2B, LEO exhibited bactericidal activity against E. coli at 2 MIC (120 μL/mL) and significant growth inhibition at the MIC (60 μL/mL) and 1/2 MIC (30 μL/mL). The lag phase of the growth curve of E. coli treated with LEO was observed, with a lag phase of 8 h for MIC-treated Escherichia coli, 4 h for the 1/2 MIC, and 2 h for the 1/4 MIC, control, and blank groups.
As shown in Figure 2C, LEO completely inhibited the growth of L. monocytogenes at 2 MIC (5.00 μL/mL), MIC (2.50 μL/mL), and 1/2 MIC (1.25 μL/mL), and exhibited significant growth inhibition at 1/4 MIC (0.63 μL/mL). The lag phase of the growth curve of L. monocytogenes treated with LEO was observed, with a lag phase of 4 h for 1/4 MIC-treated L. monocytogenes, and 2 h for the control and blank groups.
After 24 h of treatment, compared to those in the blank group, the inhibition rates of LEO at the MIC, 1/2 MIC, and 1/4 MIC against S. aureus were 54.19, 17.47, and 6.53%, respectively. The inhibition rates of LEO at the MIC, 1/2 MIC, and 1/4 MIC against E. coli were 72.26, 41.27, and 22.84%, respectively. The inhibition rate of 1/4 MIC LEO against L. monocytogenes was 43.72%.
3.2 The DPPH and ABTS radical scavenging ability of LEO
As shown in Figures 3A,B, the radical scavenging ability of DPPH and ABTS increased significantly from 60.54 to 84.88%, and from 42.76 to 83.01%, respectively, indicating a positive correlation between the free radical scavenging ability and the LEO concentration.
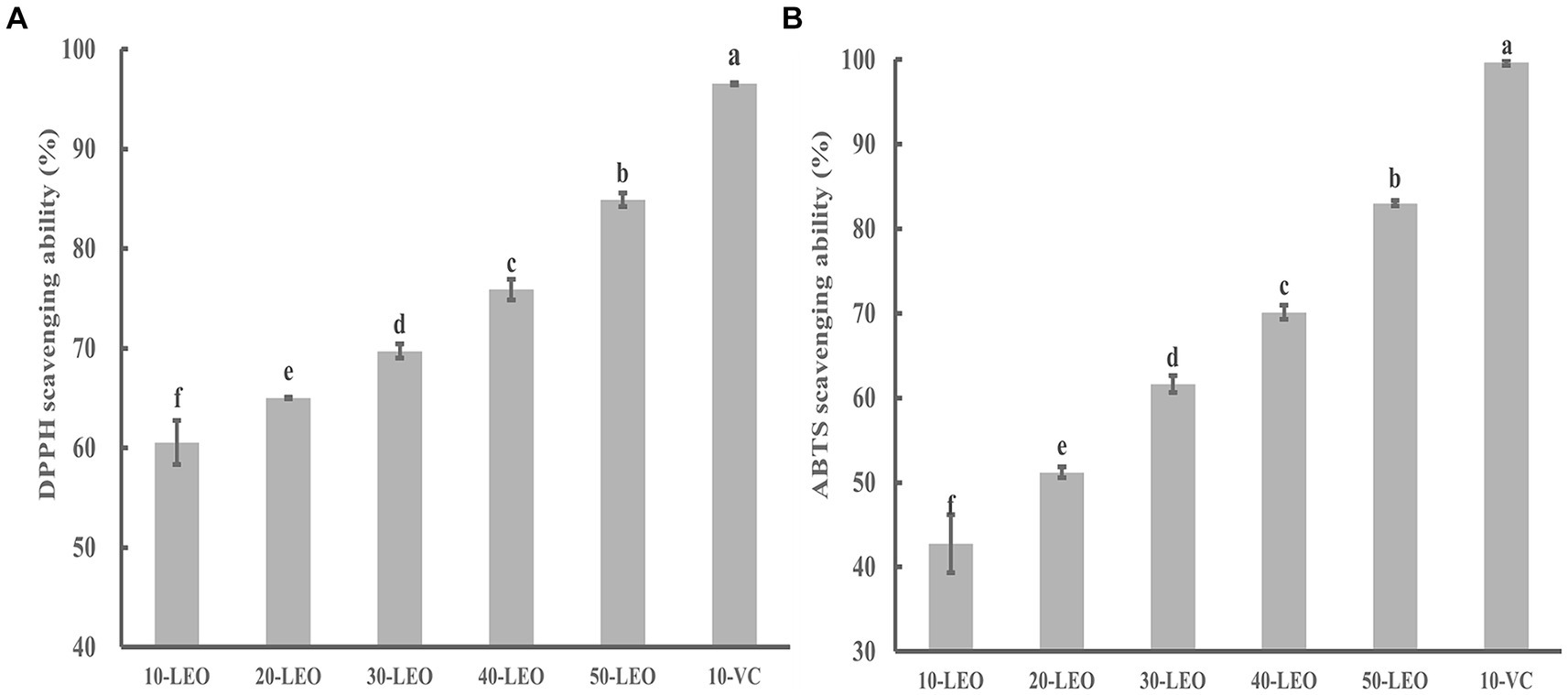
Figure 3. DPPH (A) and ABTS (B) radical scavenging ability of LEO. 10-LEO. 20-LEO, 30-LEO, 40-LEO, and 50-LEO indicated different concentration of LEO (10, 20, 30, 40, and 50 mg/mL), 10-VC indicated 10 mg/mL vitamin C. The same superscript letters in each column demonstrate lack of significant difference between values (p < 0.05).
Although the DPPH and ABTS radical scavenging abilities of LEO were significantly lower than those of VC, as the concentration of LEO increased, its scavenging rate approached that of VC, indicating that LEO possesses certain antioxidant activity. The volatile components detected in LEO mainly consisted of limonene, β-pinene, γ-terpinene, and other terpenes, and aldehydes (citronellal). Previous studies have shown that these substances have strong antioxidant activity, suggesting the feasibility of further application of LEO (da Silva et al., 2012; Victoria et al., 2014; Rezaeinia et al., 2019).
3.3 Average particle size, polydispersity index, zeta potential, and state of LEO nanoemulsion
As shown in Table 2, the average particle size of the nanoemulsion without LEO was 291.20 ± 14.89 nm, with a polydispersity index (PDI) of 0.515 ± 0.049, which was greater than that of the LEO treatment group. These findings indicate that the method used in this study can be used to prepare stable LEO nanoemulsion dispersions with relatively narrow size distributions (Kang et al., 2019). It has been reported that smaller particle sizes contribute to greater stability against gravity separation or coagulation, and smaller PDIs indicate better stability of the emulsion in a monodisperse state. Smaller particle sizes increase the surface area-to-volume ratio, allowing for better contact between the emulsion and microorganisms and leading to enhanced antibacterial effects of LEO (Falleh et al., 2021).
According to a study by Komaiko and McClements (2016), the diameter of nanoemulsion droplets should be within the range of 20 nm to 500 nm, and the stability should increase when the diameter decreases. Among the different concentrations of LEO nanoemulsion, 2.00% of the LEO nanoemulsion group exhibited an average particle size of 220.80 ± 14.37 nm and a PDI of 0.238 ± 0.024, indicating that the smallest particle size distribution range and the best dispersibility of the droplets, resulted in making the most stable system. After 15 days of storage at room temperature, the average particle size of the nanoemulsion without LEO increased to 444.17 ± 52.39 nm, and the PDI increased to 0.639 ± 0.091. A PDI higher than 0.5 indicates instability in the nanoemulsion system, leading to coagulation, Ostwald ripening, sedimentation, or flocculation (Pascual-Mathey et al., 2022). However, after 15-days storage, the PDIs of all emulsions were increased, which may be attributed to the volatility of lemon essential oil leads to a reduction in the oil phase of the emulsion and consequently causes instability. However, the PDIs of the LEO nanoemulsions were all lower than 0.5, indicating that the addition of LEO helps maintain the nanoemulsion in a stable monodisperse state. Specifically, the 2% LEO nanoemulsion had an average particle size of 344.13 ± 24.62 nm, a PDI of 0.443 ± 0.012, and a zeta potential of-18.57 ± 0.84 mV, exhibiting the best stability among the emulsions after 15 days of storage.
The zeta potential of the nanoemulsion without LEO was - 19.70 ± 0.70 mV, while the zeta potentials of the different concentrations of LEO nanoemulsion were all lower than that of the blank group. With increasing LEO concentration, the absolute value of the zeta potential also increased, indicating greater stability of the nanoemulsion system. In particular, the 2.00% LEO nanoemulsion had a zeta potential of - 27.83 ± 0.87 mV. After 15 days of storage at room temperature, the zeta potential of the nanoemulsion decreased, with the nanoemulsion without LEO having a zeta potential of - 10.97 ± 4.09 mV, and the nanoemulsion with added LEO having zeta potentials ranging from-14.62 mV to-18.57 mV, which still fell within the stable range and could resist coagulation and flocculation (Hashim et al., 2019). The decrease in the absolute value of the zeta potential after 15-days storage may be attributed to the increase in droplet size, resulting in a decrease in specific surface area, thereby inhibiting the presence of fatty acid anions (RCOO−) at the interface and reducing the charge (Lou et al., 2024). As shown in Figure 4, the state of the nanoemulsion with different concentrations of LEO on Days 0 and 15 is depicted. On the 15th day, the nanoemulsion without LEO exhibited noticeable layering and a significant amount of flocculent sedimentation, consistent with previous results. In contrast, nanoemulsions containing 0.5, 1.0, and 1.5% LEO exhibited minimal sedimentation of flocculent particles, while the nanoemulsion with 2% LEO was free from any visible sedimentation, indicating good stability of the LEO nanoemulsion, which maintained a favorable emulsion state after 15 days.
3.4 Effects of LEO nanoemulsions on fresh-cut kiwifruit
3.4.1 Weight loss
Weight loss is a major factor affecting fruit quality and storage life and is primarily caused by water evaporation from the fruit surface, which leads to shrinkage and deterioration (Guerreiro et al., 2015). As shown in Figure 5A, with increasing storage time, the percentage of weight loss gradually increased in all the groups. Kiwifruit slices treated with LEO nanoemulsions exhibited less weight loss as the concentration of LEO increased. Beginning on the 6th day, there was a significant difference (p < 0.05) in weight loss between the kiwifruit slices treated with the nanoemulsion and those without the nanoemulsion. Additionally, there were significant differences (p < 0.05) in weight loss between kiwifruit slices without added LEO and those treated with 1.5 and 2% LEO nanoemulsions. On the 8th day, the weight loss rate of the 2% LEO nanoemulsion group was only 1.16%. This result is consistent with the application of nanoemulsions containing 1.0 and 2.0% oregano essential oil for papaya preservation (Tabassum et al., 2023). This difference may be attributed to the formation of nanoemulsions on the surface of the kiwifruit, which reduce water evaporation. Essential oils can also reduce the permeability of water vapor (Pardeike et al., 2009) and can be further utilized to improve the water resistance properties of edible coatings (Atarés and Chiralt, 2016).
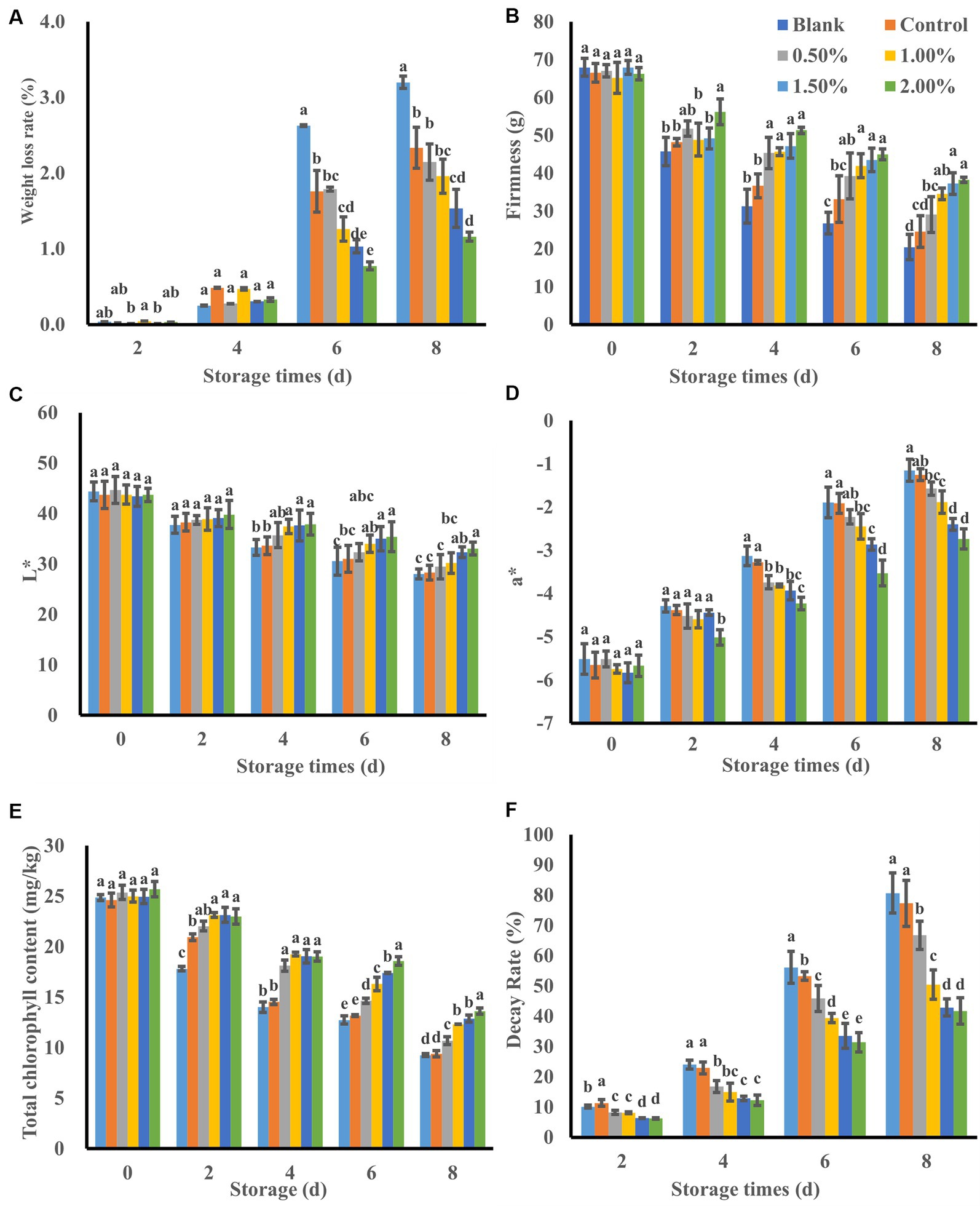
Figure 5. Effect of LEO nanoemulsion on weight loss rate (A), firmness (B), L* value (C), a* value (D), total chlorophyll content (E) and decay rate (F) of fresh-cut kiwifruit. The same superscript letters in each column demonstrate lack of significant difference between values (p < 0.05).
3.4.2 Firmness
Firmness, which is related to water content and metabolic changes (Saha et al., 2023), is an important quality parameter for consumer acceptance of fresh fruits and vegetables. As shown in Figure 5B, the increase in the storage period and the cell damage caused by cutting resulted in the release of cell wall-degrading enzymes (Ragaert et al., 2007). Under the catalysis of these enzymes, the polysaccharides in the cell wall are degraded, ultimately leading to a decrease in firmness. The blank group samples exhibited the maximum decrease in firmness, with a firmness value of only 20.459 g on the 8th day. Although the samples with nanoemulsion coatings showed a slight decrease in firmness, this decrease was relatively lower than that in the blank group at the end of storage, which is consistent with the results of previous research (Hu et al., 2022). This difference may be attributed to the addition of KGM to the nanoemulsion, which acts as a polysaccharide gas barrier, hindering the exchange of water vapor between the fruit and the external environment (Miranda et al., 2022).
The kiwifruit slices treated with the LEO nanoemulsion had greater firmness values than that did those in the emulsion-coated group, and decrease in firmness was also slighter than that in the blank group. Starting on the 2nd day, significant differences (p < 0.05) were observed between the kiwifruit slices treated with 2.0% LEO nanoemulsion and the untreated kiwifruit slices, indicating that the LEO nanoemulsion was beneficial for maintaining the firmness of the kiwifruit slices. This difference may be due to the continuous release of some active ingredients (including limonene) from the nanoemulsion by LEO during storage. These ingredients play a role in inhibiting bacteria, thus reducing the firmness decrease in firmness of kiwifruit slices caused by microbial deterioration (Ben Ghnaya et al., 2016). On the other hand, it is possible that the nanoemulsion coating disrupts the balance of O2/CO2 in the kiwifruit flesh, reducing the activity of pectin-related glycosidases involved in the hydrolysis of glycosidic bonds in kiwifruit (Huang et al., 2023), thereby slowing down cell wall degradation and alleviating the decrease in kiwifruit firmness.
3.4.3 Color
The color of fruits and vegetables is closely related to their quality during storage and directly influences consumer choices. The L* value represents the brightness of the sample color. As shown in Figure 5C, the L* values of all the kiwifruit slices decreased throughout the entire storage period, possibly due to browning on the surface of kiwifruit, resulting in a gradual decrease in brightness during storage (Yu et al., 2023). By the 8th day of storage, the L* value of the blank group decreased from 44.38 on the 1st day to 28.02, indicating a darker color. In contrast, the L* value of kiwifruit slices immersed in LEO nanoemulsions decreased at a slower rate, indicating better maintenance of color brightness. Among them, the L* value of the 2% LEO nanoemulsion group was 33.07, which was significantly different from that of the blank group and the control group (p < 0.05). This may be attributed to the smaller particle size and antioxidant properties of the nanoemulsion containing LEO, which can slowly release into the kiwifruit pulp, reduce gas exchange between the pulp and the external environment, inhibit the activity of enzymes that cause browning, and decrease the oxidation of phenolic substances catalyzed by enzymes, thus reducing the formation of brown pigments (Toivonen and Brummell, 2008).
The a* value represents the color from green to red (Passafiume et al., 2020) As shown in Figure 5D, the a* values of kiwifruit slices in all groups increased during the entire storage period, which was attributed to the degradation of chlorophyll. The a* values of the blank group and the control group on the 8th day were-1.15 and-1.25, respectively, with chlorophyll contents of 9.26 and 9.41 mg/kg, respectively (Figure 5E). In contrast, the a* value of the 2% LEO nanoemulsion sample group was −2.74, with a chlorophyll content of 13.64 mg/kg, indicating the highest green color retention ability. This finding suggested that the 2% LEO nanoemulsion had the greatest effect on preserving green color, which was similar to what was observed in fresh-cut kiwifruit treated with shortwave ultraviolet radiation on the 7th day (Hu et al., 2022). The changes in L* and a* values are consistent with the color of kiwifruit in Figure 6. These results demonstrated that immersion treatment with LEO nanoemulsions can maintain the typical bright green color of kiwifruit slices by preventing the oxidation and degradation of chlorophyll.
3.4.4 Decay rate
As shown in the Figure 5F, all the samples exhibited an increase in decay rate during storage. However, the samples in the nanoemulsion group exhibited a significantly lower decay rate than did those in the blank group. On the 2nd day, the decay rate of the blank samples was 10.57%, and after 8 days of storage, 80.42% of the kiwifruit slices were infected and decayed. With an increase in LEO concentration, the decay rate of the kiwifruit slices gradually decreased. On the 8th day of storage, when the LEO concentration increased from 0 to 2.0%, the decay rate of the kiwifruit slices significantly decreased from 73.24 to 41.55%. This indicates that the nanoemulsion coating with LEO can effectively reduce the decay of kiwifruit slices during storage, and that a higher concentration of LEO results in a better inhibitory effect on decay. LEO has antimicrobial activity, inhibits the growth of foodborne pathogens by forming a uniform surface on kiwifruit plants. By being uniformly dispersed in the nanoemulsion, the volatilization of the effective antimicrobial components of LEO can be minimized, thereby enhancing the antimicrobial properties of the LEO nanoemulsion.
3.4.5 Soluble solids content
The soluble solid content (SSC) is an important indicator for evaluating the quality of kiwifruit, and it is positively correlated with the sugar content. Additionally, SSC is closely related to metabolism, where stronger respiratory activity leads to greater consumption of its own substances and lower SSC. As shown in Figure 7A, the blank group, control group, and 0.5% group exhibited an initial increase followed by a decrease in SSC. This trend may be attributed to the respiratory activity of kiwifruit, where polysaccharides are hydrolyzed into sugars and other soluble compounds during metabolism, leading to an increase in soluble solids content. As the kiwifruit deteriorates, the converted sugars continue to hydrolyze into reducing sugars (Tian et al., 2023), and simple sugars further degrade and transform into acidic substances (Tirkey et al., 2014), resulting in a decrease in soluble solids content. In addition, the remaining treatment groups demonstrated a gradual increase in soluble solids content, possibly due to the ability of LEO concentrations exceeding 0.5% to inhibit the reproduction of microorganisms and reduce their consumption of carbohydrates. In addition, LEO reduced the respiration intensity of kiwifruit, thereby slowing its physiological metabolic activity and facilitating the accumulating of soluble sugars, thus better preserving the flavor of freshly cut kiwifruit.
3.4.6 Titratable acid
TA is an important factor that affects product flavor and directly influences consumer preference. As shown in Figure 7B, all treatment groups exhibited a decreasing trend in TA content during storage, possibly due to the respiratory activity of fresh-cut kiwifruit, which consumes some of its organic acids as substrates during respiration, resulting in a decrease in titratable acidity (Yu et al., 2021). On the 2nd day of storage, the TA content of all the treatment groups decreased rapidly, which may be attributed to external damage to the fresh fruit and respiratory consumption. Compared to those of the control group and the blank group, the soaking treatment with the LEO nanoemulsion reduced the decline in TA. The sustained-release effect of LEO helps maintain TA at a relatively high level, possibly due to the antimicrobial activity of LEO, which inhibits microbial growth and reduces the oxygen content on the surface of kiwifruit, thereby reducing the consumption of organic acids (Yan et al., 2020).
3.5 pH
As shown in Figure 7C, the pH values of all the samples increased continuously throughout the storage period. By the 8th day of storage, the pH values of the blank group and the control group were significantly greater than that of the LEO nanoemulsion group, with the lowest pH value observed in the 2% LEO nanoemulsion group. However, the differences among the different concentrations were not significant. Considering the trend of TA changes, it can be concluded that the immersion treatment with LEO nanoemulsions effectively inhibited the decomposition of organic acids in fresh-cut kiwifruit, maintained TA, and slowed down the increase in pH value.
3.5.1 VC content and DPPH radical scavenging ability of fresh-cut kiwifruit
As shown in Figure 8A, on the 0th day, kiwifruit slices treated with the LEO nanoemulsion exhibited greater DPPH radical scavenging ability than did those in the blank and control groups. During storage, this activity gradually decreased, beginning on the 6th day and then significantly decreased compared to that on 4th day (p < 0.05). Compared to those of the blank and control groups, the kiwifruit slices treated with the LEO nanoemulsion demonstrated improved DPPH scavenging ability, indicating the ability of the LEO nanoemulsion to enhance the antioxidant capacity of freshly cut kiwifruit. As shown in Figure 8B, during storage, the VC content in the blank group gradually decreased. In comparison to those in the blank and control groups, kiwifruit slices treated with LEO nanoemulsions exhibited a slower degradation rate of VC. Beginning on the 2nd day, the VC content in the blank group samples significantly differed (p < 0.05) from that on Day 0, while VC content did not significantly differ between the samples treated with the different concentrations of LEO nanoemulsion compared to those on Day 0. This difference may be due to the superior oxygen barrier ability of the LEO nanoemulsion, which reduces the oxidation of ascorbic acid by oxygen compared to that of the nanoemulsion without lemon essential oil. Considering the trend of DDPH scavenging ability changes, this may also be attributed to the superior oxygen isolation capability of the nanoemulsion containing LEO. Moreover, some terpenes in essential oils, for instance, γ-terpinene in LEO (Nunes-Damaceno et al., 2013) have synergistic antioxidant effects (Guo et al., 2021) with phenolic substances in kiwifruit and are gradually released from the nanoemulsions during storage, contributing to the maintenance of VC content and DPPH radical scavenging ability.
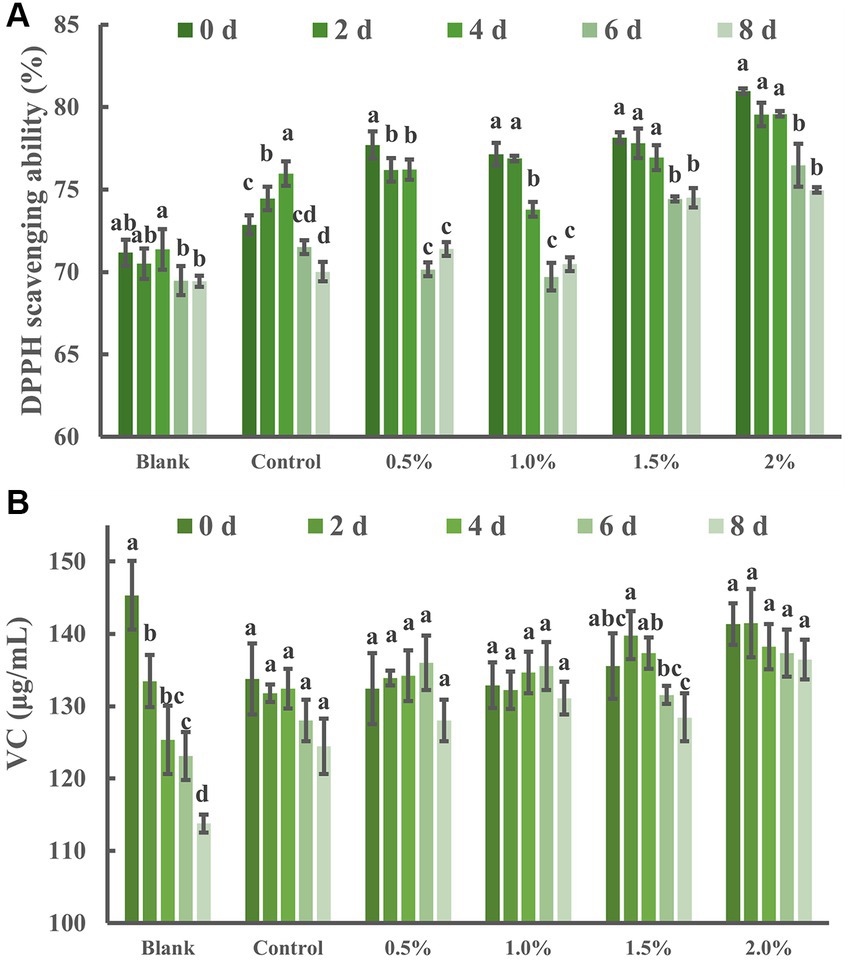
Figure 8. Effects of LEO nanoemulsion on DPPH radical scavenging ability (A) and VC (B) of fresh-cut kiwifruit. The same superscript letters in each column demonstrate lack of significant difference between values (p < 0.05).
3.5.2 Antioxidant enzyme activity
Fresh-cut kiwifruit undergo mechanical damage, such as peeling and cutting, leading to the production of a large amount of reactive oxygen species (ROS), which disrupts the balance of free radical metabolism in kiwifruit and accelerates membrane peroxidation (Zeng et al., 2019), severely affecting product quality. CAT and POD are important antioxidant enzymes in fruits that can scavenge ROS and reduce oxidative damage. As shown in Figures 9A,B the CAT and POD activities exhibited an increasing trend followed by a decrease in all treatment groups. The CAT and POD activities of the blank group and the control group peaked on the 4th day of storage, while those of the LEO nanoemulsion-treated group peaked on the 6th day and were greater than those of the blank group and the control group, indicating that immersion in LEO nanoemulsions can enhance the CAT and POD activities of fresh-cut kiwifruit.
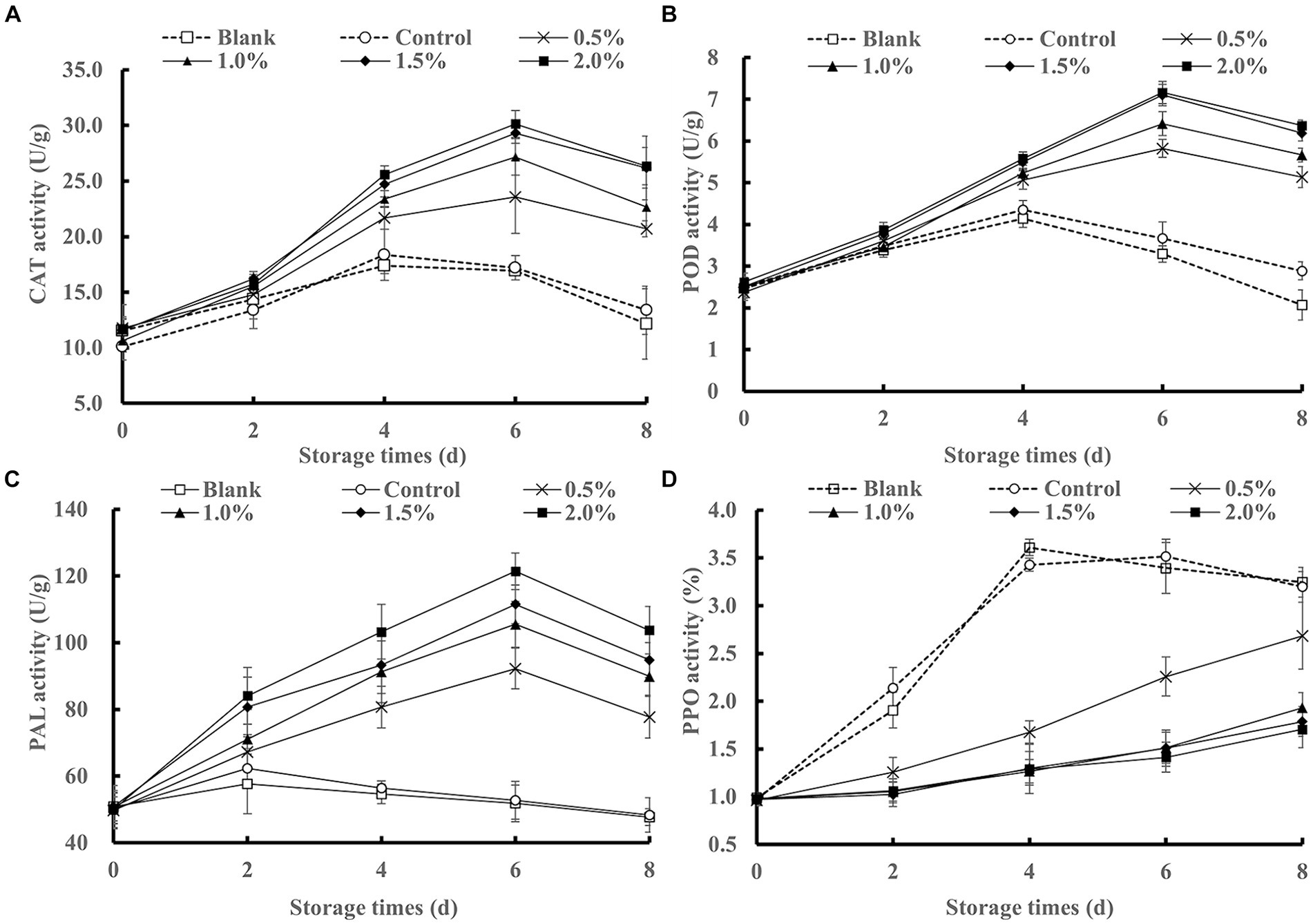
Figure 9. Effects of LEO nanoemulsion on CAT activity (A), POD activity (B), PAL activity (C) and PPO activity (D) of fresh-cut kiwifruit.
As shown in Figure 9C, PAL activity increased followed by a decrease in all treatment groups. The PAL activity in the blank group and the control group remained relatively low throughout the storage period, with a slight increase on the 2nd day. In contrast, the PAL activity in the LEO nanoemulsion group was consistently greater than that in the blank group and the control group, peaking on the 6th day and slightly decreasing on the 8th day. Kiwifruit plants are susceptible to chilling injury and sensitive to temperature. Storage at 4°C can lead to chilling injury in kiwifruit, resulting in browning and lignification, which affects the storage quality and edibility of kiwifruit. PAL is a key enzyme in phenylpropane metabolism and is involved in the synthesis of phenolic substances (Yu et al., 2021), which can enhance the antioxidant capacity of fresh-cut kiwifruit. Through the treatment with LEO nanoemulsions, the active components in LEO are continuously released, inhibiting microbial growth, enhancing PAL activity, and delaying the senescence of fresh-cut kiwifruit.
PPO is a key enzyme involved in the browning of fruits and vegetables. This process oxidizes phenolic substrates to quinones, resulting in the formation of brown pigments. Therefore, inhibiting PPO activity is an important measure for fruit and vegetable preservation. As shown in Figure 9D, the PPO activity of the samples treated with the LEO nanoemulsion was lower than that of the control group and the blank group throughout the entire storage period. This may be because VC has an inhibitory effect on PPO activity, and LEO inhibits PPO activity by reducing the loss of VC, thereby alleviating browning caused by the oxidation of phenolic substances. This finding is consistent with the previous results for kiwifruit color.
3.5.3 Microbiological analysis
After peeling and cutting, fresh-cut kiwifruit undergoes mechanical damage, losing the protection of the peel and increasing the exposed surface area, increasing susceptibility to microbial contamination. Additionally, during storage, the leakage of fruit juice provides an excellent environment for microbial growth and reproduction. As shown in Figure 10, there were significant differences in the total colony count between the group treated with the LEO nanoemulsion and the blank group and control group (p < 0.05). The values for both the blank group and the control group exceeded the edible safety standard [6 log10 (CFU/g)]. In contrast, the samples treated with the LEO nanoemulsion had total colony count within 6 log10 (CFU/g), and the 1.0, 1.5, and 2.0% of the LEO nanoemulsion groups had total colony count within 4 log10 (CFU/g). This indicates that the LEO nanoemulsion effectively inhibits microbial activity during storage. On the one hand, the immersion treatment with LEO nanoemulsions results in the formation of a protective film on the surface of fresh-cut kiwifruit, which helps to prevent microbial contamination. Moreover, LEO has certain antibacterial properties that induce bacterial cell membrane lysis, resulting in reduced total colony counts (Ghosh et al., 2024), and is gradually released from nanoemulsions, where it can achieve sustained antibacterial effects. These findings indicate that the coating fresh-cut kiwifruit with LEO nanoemulsion can extend their shelf life.
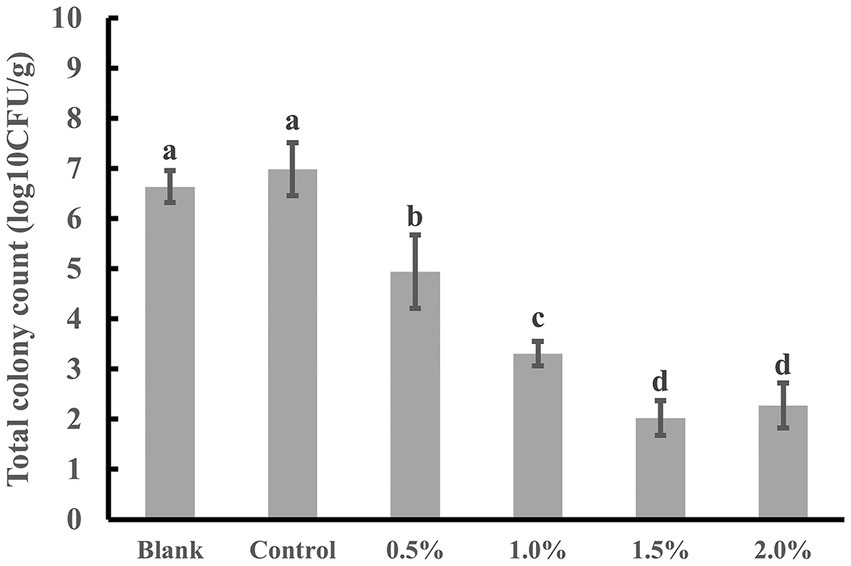
Figure 10. Total colony count of fresh-cut kiwifruit on the 8th day of storage. The same superscript letters in each column demonstrate lack of significant difference between values (p < 0.05).
4 Conclusion
This study confirmed the inhibitory effect of LEO on the growth of three tested bacteria (E. coli, S. aureus, and L. monocytogenes), with a significantly greater inhibitory effect on gram-positive bacteria than on gram-negative bacteria. Two naturally derived compounds were selected to prepare nanoemulsions containing LEO. Within a 15-day period, the nanoemulsion with a 2% LEO concentration demonstrated superior stability and had minimal impact on the sensory evaluation of freshly cut kiwifruit. The LEO nanoemulsion was applied to the surface of the kiwifruit slices through an immersion process. The LEO nanoemulsion could form a protective layer on the surface of fresh-cut kiwifruit, reducing water evaporation, modifying enzyme activity, mitigating firmness decline, and maintaining the original color of the kiwifruit. It also had positive effects on the changes in SSC, TA, pH, and other parameters of kiwifruit. Among them, the 2.0% LEO nanoemulsion exhibited the best preservation effect on kiwifruit, providing a feasible scheme for future exploration of synergistic formulas as a fruit and vegetable freshness-maintaining coatings.
Data availability statement
The original contributions presented in the study are included in the article/supplementary material, further inquiries can be directed to the corresponding author.
Author contributions
CC: Writing – original draft, Investigation, Formal analysis. F-BM: Writing – review & editing, Methodology, Data curation, Conceptualization. H-JL: Writing – original draft, Methodology, Investigation, Formal analysis. Z-ZG: Writing – original draft, Methodology, Investigation, Formal analysis. JQ: Writing – review & editing. Y-CL: Writing – review & editing, Validation, Project administration, Funding acquisition.
Funding
The author(s) declare that financial support was received for the research, authorship, and/or publication of this article. This work was supported by the National Key Research and Development Program of China (2022YFF0606800) and Sichuan Science and Technology Program (2023YFN0014).
Conflict of interest
The authors declare that the research was conducted in the absence of any commercial or financial relationships that could be construed as a potential conflict of interest.
Publisher’s note
All claims expressed in this article are solely those of the authors and do not necessarily represent those of their affiliated organizations, or those of the publisher, the editors and the reviewers. Any product that may be evaluated in this article, or claim that may be made by its manufacturer, is not guaranteed or endorsed by the publisher.
References
Ali, M., Batool, S., Khalid, N., Ali, S., Raza, M. A., Li, X., et al. (2024). Recent trends in hydrogen-associated treatments for maintaining the postharvest quality of fresh and fresh-cut fruits and vegetables: a review. Food Control 156:110114. doi: 10.1016/j.foodcont.2023.110114
Atarés, L., and Chiralt, A. (2016). Essential oils as additives in biodegradable films and coatings for active food packaging. Trends Food Sci. Technol. 48, 51–62. doi: 10.1016/j.tifs.2015.12.001
Ben Ghnaya, A., Amri, I., Hanana, M., Gargouri, S., Jamoussi, B., Romane, A., and Hamrouni, L. (2016). Tetraclinis articulata (Vahl.) masters essential oil from Tunisia: chemical characterization and herbicidal and antifungal activities assessment. Ind. Crop. Prod. 83, 113–117. doi: 10.1016/j.indcrop.2015.12.026
Costa, W. K., De Oliveira, A. M., Santos, I. B. D. S., Silva, V. B. G., Da Silva, E. K. C., Alves, J. V. D. O., et al. (2022). Antibacterial mechanism of Eugenia Stipitata Mcvaugh essential oil and synergistic effect against Staphylococcus Aureus. S. Afr. J. Bot. 147, 724–730. doi: 10.1016/j.sajb.2022.03.012
Da Silva, A. C. R., Lopes, P. M., De Azevedo, M. M. B., Costa, D. C. M., Alviano, C. S., and Alviano, D. S. (2012). Biological activities of Α-Pinene and Β-Pinene enantiomers. Molecules 17, 6305–6316. doi: 10.3390/molecules17066305
Falleh, H., Jemaa, M. B., Neves, M. A., Isoda, H., Nakajima, M., and Ksouri, R. (2021). Formulation, physicochemical characterization, and anti-E. coli activity of food-grade Nanoemulsions incorporating clove, cinnamon, and lavender essential oils. Food Chem. 359,:129963. doi: 10.1016/j.foodchem.2021.129963
Gardesh, A. S. K., Badii, F., Hashemi, M., Ardakani, A. Y., Maftoonazad, N., and Gorji, A. M. (2016). Effect of Nanochitosan based coating on climacteric behavior and postharvest shelf-life extension of apple cv. golab kohanz. LWT 70, 33–40. doi: 10.1016/j.lwt.2016.02.002
Ghosh, V., Chandwani, S. K., Lonhare, A., and Balan, N. S. (2024). Antibacterial Nanoemulsion of oregano oil for food preservation: in vitro and in situ evaluation against Escherichia coli. Bio Nano Sci. (In press). doi: 10.1007/S12668-024-01300-8
Gorzelany, J., Kapusta, I., Zardzewiały, M., and Belcar, J. (2022). Effects of ozone application on microbiological stability and content of sugars and bioactive compounds in the fruit of the Saskatoon berry (Amelanchier alnifolia Nutt.). Molecules, 27,:6446. doi: 10.3390/molecules27196446
Guerreiro, A. C., Gago, C. M. L., Faleiro, M. L., Miguel, M. G. C., and Antunes, M. D. C. (2015). The use of polysaccharide-based edible coatings enriched with essential oils to improve shelf-life of strawberries. Postharvest Biol. Technol. 110, 51–60. doi: 10.1016/j.postharvbio.2015.06.019
Guo, Y., Baschieri, A., Amorati, R., and Valgimigli, L. (2021). Synergic antioxidant activity of Γ-Terpinene with phenols and polyphenols enabled by Hydroperoxyl radicals. Food Chem. 345,:128468. doi: 10.1016/j.foodchem.2020.128468
Han, Y., Chen, W., and Sun, Z. (2021). Antimicrobial activity and mechanism of limonene against Staphylococcus aureus. J. Food Saf. 41,:E12918. doi: 10.1111/jfs.12918
Hashim, A. F., Hamed, S. F., Abdel Hamid, H. A., Abd-Elsalam, K. A., Golonka, I., Musiał, W., and El-Sherbiny, I. M. (2019). Antioxidant and antibacterial activities of Omega-3 rich oils/curcumin Nanoemulsions loaded in chitosan and alginate-based microbeads. Int. J. Biol. Macromol. 140, 682–696. doi: 10.1016/j.ijbiomac.2019.08.085
He, Q., Zhang, L., Yang, Z., Ding, T., Ye, X., Liu, D., and Guo, M. (2022). Antibacterial mechanisms of thyme essential oil nanoemulsions against Escherichia coli O157:H7 and Staphylococcus aureus: alterations in membrane compositions and characteristics. Innov. Food Sci. Emerg. 75,:102902. doi: 10.1016/j.ifset.2021.102902
Hu, X., Chen, Y., Wu, X., Liu, W., Jing, X., Liu, Y., et al. (2022). Combination of calcium lactate impregnation with Uv-C irradiation maintains quality and improves antioxidant capacity of fresh-cut kiwifruit slices. Food Chem. X 14:100329. doi: 10.1016/j.fochx.2022.100329
Huang, P., Ding, J., Liu, C., Li, H., Wang, C., Lin, Y., et al. (2023). Konjac Glucomannan/low-acyl Gellan gum edible coating containing thymol microcapsule regulates Cell Wall polysaccharides disassembly and delays postharvest softening of blueberries. Postharvest Biol. Technol. 204:112449. doi: 10.1016/j.postharvbio.2023.112449
Kang, J.-H., Park, S.-J., Park, J.-B., and Song, K. B. (2019). Surfactant type affects the washing effect of cinnamon leaf essential oil emulsion on kale leaves. Food Chem. 271, 122–128. doi: 10.1016/j.foodchem.2018.07.203
Katata-Seru, L., Lebepe, T. C., Aremu, O. S., and Bahadur, I. (2017). Application of Taguchi method to optimize garlic essential oil Nanoemulsions. J. Mol. Liq. 244, 279–284. doi: 10.1016/j.molliq.2017.09.007
Komaiko, J. S., and Mcclements, D. J. (2016). Formation of food-grade Nanoemulsions using low-energy preparation methods: a review of available methods. Compr. Rev. Food Sci. Food Saf. 15, 331–352. doi: 10.1111/1541-4337.12189
Lee, J. Y., Garcia, C. V., Shin, G. H., and Kim, J. T. (2019). Antibacterial and antioxidant properties of hydroxypropyl methylcellulose-based active composite films incorporating oregano essential oil nanoemulsions. LWT, 106, 164–171. doi: 10.1016/j.lwt.2019.02.061
Li, L., Li, C., Sun, J., Xin, M., Yi, P., He, X., et al. (2021). Synergistic effects of ultraviolet light irradiation and high-oxygen modified atmosphere packaging on physiological quality, microbial growth and lignification metabolism of fresh-cut carrots. Postharvest Biol. Technol. 173:111365. doi: 10.1016/j.postharvbio.2020.111365
Liu, D., Wang, F., Brennan, C., Benjakul, S., Xiao, G., Ying, X., and Ma, L. (2023). Combined melatonin and Uv-C treatment maintains the quality of fresh-cut bamboo shoots during storage by altering microbial diversity and metabolites. Postharvest Biol. Technol. 200:112327. doi: 10.1016/j.postharvbio.2023.112327
Lou, S., Ni, X., Xiao, W., Li, Y., and Gao, Z. (2024). Physical stability, microstructure and antimicrobial properties of Konjac Glucomannan coatings enriched with Litsea cubeba essential oil Nanoemulsion and its effect on citruses preservation. Int. J. Biol. Macromol. 256,:128306. doi: 10.1016/j.ijbiomac.2023.128306
Luo, Y., Li, Y., Wang, M., Zhou, L., Meng, F., and Jiang, L. (2023). Effects of grafting methods and raw materials on the physicochemical properties and biological activities of phenolic acids grafted oat Β-glucan. Food Res. Int. 173,:113250. doi: 10.1016/j.foodres.2023.113250
Manzoor, S., Gull, A., Wani, S. M., Ganaie, T. A., Masoodi, F. A., Bashir, K., et al. (2021). Improving the shelf life of fresh cut kiwi using Nanoemulsion coatings with antioxidant and antimicrobial agents. Food Biosci. 41:101015. doi: 10.1016/j.fbio.2021.101015
Meng, F., Gou, Z., Li, Y., Zou, L., Chen, W., and Liu, D. (2022a). The efficiency of lemon essential oil-based Nanoemulsions on the inhibition of Phomopsis Sp. and reduction of postharvest decay of kiwifruit. Food Secur. 11:1510. doi: 10.3390/foods11101510
Meng, F., Lei, Y., Zhang, Q., Li, Y., Chen, W., and Liu, D. (2022b). Encapsulation of Zanthoxylum Bungeanum essential oil to enhance flavor stability and inhibit lipid oxidation of Chinese-style sausage. J. Sci. Food Agr. 102, 4035–4045. doi: 10.1002/jsfa.11752
Meng, F., Zhang, Q., Li, Y., Li, J., Liu, D., and Peng, L. (2020). Konjac Glucomannan Octenyl succinate as a novel Encapsulation Wall material to improve curcumin stability and bioavailability. Carbohyd. Polym. 238,:116193. doi: 10.1016/j.carbpol.2020.116193
Miranda, M., Ribeiro, M. D. M. M., Spricigo, P. C., Pilon, L., Mitsuyuki, M. C., Correa, D. S., and Ferreira, M. D. (2022). Carnauba wax Nanoemulsion applied as an edible coating on fresh tomato for postharvest quality evaluation. Heliyon 8,:E09803. doi: 10.1016/j.heliyon.2022.e09803
Nunes-Damaceno, M., Muñoz-Ferreiro, N., Romero-Rodríguez, M. A., and Vázquez-Odériz, M. L. (2013). A comparison of kiwifruit from conventional, integrated and organic production systems. LWT 54, 291–297. doi: 10.1016/j.lwt.2013.05.002
Pardeike, J., Hommoss, A., and Müller, R. H. (2009). Lipid nanoparticles (Sln, Nlc) in cosmetic and pharmaceutical dermal products. Int. J. Pharm. 366, 170–184. doi: 10.1016/j.ijpharm.2008.10.003
Pascual-Mathey, L. I., Briones-Concha, J. A., Jiménez, M., Beristain, C. I., and Pascual-Pineda, L. A. (2022). Elaboration of essential oil Nanoemulsions of rosemary (Rosmarinus officinalis L.) and its effect on liver injury prevention. Food Bioprod. Process. 134, 46–55. doi: 10.1016/j.fbp.2022.04.006
Passafiume, R., Gaglio, R., Sortino, G., and Farina, V. (2020). Effect of three different Aloe vera gel-based edible coatings on the quality of fresh-cut “Hayward” kiwifruits. Food Secur., 9,:939. doi: 10.3390/foods9070939
Ragaert, P., Devlieghere, F., and Debevere, J. (2007). Role of microbiological and physiological spoilage mechanisms during storage of minimally processed vegetables. Postharvest Biol. Technol. 44, 185–194. doi: 10.1016/j.postharvbio.2007.01.001
Rezaeinia, H., Ghorani, B., Emadzadeh, B., and Tucker, N. (2019). Electrohydrodynamic atomization of Balangu (Lallemantia royleana) seed gum for the fast-release of Mentha longifolia L. essential oil: characterization of Nano-capsules and modeling the kinetics of release. Food Hydrocoll. 93, 374–385. doi: 10.1016/j.foodhyd.2019.02.018
Saha, T., Roy, D. K. D., Khatun, M. N., and Asaduzzaman, M. (2023). Quality and shelf life of fresh-cut pineapple (Ananas comosus) coated with aloe Vera and honey in the refrigerated condition. J. Agri. Food Res., 14,:100709. doi: 10.1016/j.jafr.2023.100709
Seow, Y. X., Yeo, C. R., Chung, H. L., and Yuk, H. G. (2014). Plant essential oils as active antimicrobial agents. Crit. Rev. Food Sci. 54, 625–644. doi: 10.1080/10408398.2011.599504
Shao, P., Liu, L., Yu, J., Zheng, L., and Sun, P. (2022). Novel aldehyde sensitive bio-based colorimetric film for kiwi fruit freshness monitoring. LWT 159:113177. doi: 10.1016/j.lwt.2022.113177
Song, L., Yang, H., Cheng, S., Zhang, Z., Zhang, L., Su, R., et al. (2024). Combination effects of ultrasound and Citral Nanoemulsion against Shigella flexneri and the preservation effect on fresh-cut carrots. Food Control 155:110069. doi: 10.1016/j.foodcont.2023.110069
Su, R., Guo, X., Cheng, S., Zhang, Z., Yang, H., Wang, J., et al. (2023). Inactivation of Salmonella using ultrasound in combination with Litsea cubeba essential oil Nanoemulsion and its bactericidal application on cherry tomatoes. Ultrason. Sonochem. 98:106481. doi: 10.1016/j.ultsonch.2023.106481
Tabassum, N., Aftab, R. A., Yousuf, O., Ahmad, S., and Zaidi, S. (2023). Application of Nanoemulsion based edible coating on fresh-cut papaya. J. Food Eng. 355,:111579. doi: 10.1016/j.jfoodeng.2023.111579
Tang, W., Zhang, Z., Nie, D., Liu, S., Li, Y., Liu, M., et al. (2023). Selective antibacterial activity of Citrus medica Limonum essential oil against Escherichia coli K99 and lactobacillus acidophilus and its antibacterial mechanism. LWT 186:115215. doi: 10.1016/j.lwt.2023.115215
Tapia, M. S., Rojas-Graü, M. A., Carmona, A., Rodríguez, F. J., Soliva-Fortuny, R., and Martin-Belloso, O. (2008). Use of alginate-and Gellan-based coatings for improving barrier, texture and nutritional properties of fresh-cut papaya. Food Hydrocoll. 22, 1493–1503. doi: 10.1016/j.foodhyd.2007.10.004
Tian, Y., Zhou, L., Liu, J., Yu, K., Yu, W., Jiang, H., et al. (2023). Effect of sustained-release tea tree essential oil solid preservative on fresh-cut pineapple storage quality in modified atmospheres packaging. Food Chem. 417:135898. doi: 10.1016/j.foodchem.2023.135898
Tirkey, B., Pal, U. S., Bal, L. M., Sahoo, N. R., Bakhara, C. K., and Panda, M. K. (2014). Evaluation of Physico-chemical changes of fresh-cut unripe papaya during storage. Food Packaging Shelf 1, 190–197. doi: 10.1016/j.fpsl.2014.02.002
Toivonen, P. M. A., and Brummell, D. A. (2008). Biochemical bases of appearance and texture changes in fresh-cut fruit and vegetables. Postharvest Biol. Technol. 48, 1–14. doi: 10.1016/j.postharvbio.2007.09.004
U. S. Food and Drug Administration (2019). CFR - code of federal regulations title 21. https://Www.Accessdata.Fda.Gov/Scripts/Cdrh/Cfdocs/Cfcfr/Cfrsearch.Cfm?Cfrpart=182 (Accessed December 22, 2023).
Victoria, F. N., Anversa, R., Penteado, F., Castro, M., Lenardão, E. J., and Savegnago, L. (2014). Antioxidant and antidepressant-like activities of semi-synthetic Α-Phenylseleno citronellal. Eur. J. Pharmacol. 742, 131–138. doi: 10.1016/j.ejphar.2014.09.005
Wang, M., Li, Y., Meng, F., Wang, Q., Wang, Z., and Liu, D. (2023). Effect of honeysuckle leaf extract on the physicochemical properties of Carboxymethyl Konjac Glucomannan/Konjac Glucomannan/gelatin composite edible film. Food Chem. X 18,:100675. doi: 10.1016/j.fochx.2023.100675
Yan, Y., Duan, S., Zhang, H., Liu, Y., Li, C., Hu, B., et al. (2020). Preparation and characterization of Konjac Glucomannan and pullulan composite films for strawberry preservation. Carbohyd. Polym. 243:116446. doi: 10.1016/j.carbpol.2020.116446
Yang, J., Goksen, G., and Zhang, W. (2023). Rosemary essential oil: chemical and biological properties, with emphasis on its delivery systems for food preservation. Food Control 154,:110003. doi: 10.1016/j.foodcont.2023.110003
Yang, F., Zhao, R., Suo, J., Ding, Y., Tan, J., Zhu, Q., and Ma, Y. (2024). Understanding quality differences between kiwifruit varieties during softening. Food Chem. 430,:136983. doi: 10.1016/j.foodchem.2023.136983
Yazgan, H. (2020). Investigation of antimicrobial properties of sage essential oil and its Nanoemulsion as antimicrobial agent. LWT 130:109669. doi: 10.1016/j.lwt.2020.109669
Yousuf, B., Wu, S., and Siddiqui, M. W. (2021). Incorporating essential oils or compounds derived thereof into edible coatings: effect on quality and shelf life of fresh/fresh-cut produce. Trends. Food. Sci. Technol. 108, 245–257. doi: 10.1016/j.tifs.2021.01.016
Yu, R., Song, H., Chen, Y., Shi, N., Shen, H., Shi, P., et al. (2023). Incorporation of ascorbic acid and L-cysteine in sodium Carboxymethyl cellulose coating delays color deterioration and extends the shelf-life of fresh-cut Asparagus lettuce (Lactuca SativaVar Angustata). Postharvest Biol. Technol. 204:112419. doi: 10.1016/j.postharvbio.2023.112419
Yu, K., Xu, J., Zhou, L., Zou, L., and Liu, W. (2021). Effect of chitosan coatings with cinnamon essential oil on postharvest quality of mangoes. Food Secur. 10,:3003. doi: 10.3390/foods10123003
Keywords: lemon essential oil, antibacterial, fresh-cut kiwifruit, nanoemulsion, preservation
Citation: Chen C, Meng F-B, Lv H-J, Gou Z-Z, Qiu J and Li Y-C (2024) Study on the bacteriostasis of lemon essential oil and the application of lemon essential oil nanoemulsion on fresh-cut kiwifruit. Front. Sustain. Food Syst. 8:1394831. doi: 10.3389/fsufs.2024.1394831
Edited by:
Raul Avila-Sosa, Benemérita Universidad Autónoma de Puebla, MexicoReviewed by:
Vijayalakshmi Ghosh, GD Rungta College of Science and Technology, IndiaBruno Dutra Da Silva, Federal University of Rio de Janeiro, Brazil
Copyright © 2024 Chen, Meng, Lv, Gou, Qiu and Li. This is an open-access article distributed under the terms of the Creative Commons Attribution License (CC BY). The use, distribution or reproduction in other forums is permitted, provided the original author(s) and the copyright owner(s) are credited and that the original publication in this journal is cited, in accordance with accepted academic practice. No use, distribution or reproduction is permitted which does not comply with these terms.
*Correspondence: Yun-Cheng Li, liyunchengs@126.com
†These authors have contributed equally to this work