- 1Wageningen Environmental Research, Wageningen University and Research, Wageningen, Netherlands
- 2Soil Biology Group, Wageningen University and Research, Wageningen, Netherlands
- 3Land Use Planning Group, Wageningen University and Research, Wageningen, Netherlands
Recycling of organic resources into agriculture has the potential to greatly increase nutrient use efficiency and improve soil carbon balance, but improper management can have adverse effects on the environment. Agriculture therefore faces large challenges to increase yields while decreasing these emissions to the environment. In this paper, we review (i) the availability and composition of organic resources, (ii) their agronomic value and risk of emissions, (iii) potential measures to reduce their emissions, and (iv) future challenges to support farmers and policy makers. The total amount of organic resource applied to soil amounted on average 41 kg nitrogen per ha agricultural land, 9 kg phosphorus per ha, and 456 kg carbon per ha in EU-27 + UK in 2017. Solid pig and cattle manures and cattle slurry are the most used organic resources. The availability of new organic resources from food processing, sewage sludge, municipal bio-wastes, and upcoming manure treatment techniques as fertilizer or soil conditioner is expected to strongly increase over the coming decade. Insight is needed into the composition of organic resources, the plant-availability of nutrients, the degradability of organic matter and the presence of contaminants. Measurement techniques become available to characterize soils, manures, crops, and emissions to the environment. However, the interpretation, and integration of data, and recommendations to farmers and policymakers using large amounts of data is expected to become more and more challenging. Many measures are available to improve nutrient and carbon management and to reduce emissions, including proper application, technological measures and structural changes in agriculture. For many measures, there is a risk of trade-offs that could lead to pollution swapping at different scales. We should focus on finding synergies between measures and no-regret management choices to develop effective mitigation strategies. The main future challenge for managing organic resources in agriculture is the development of an integrated nutrient management approach, including (i) the characterization of organic resources, their agronomic value and their environmental risks, (ii) knowledge of potential synergies and trade-offs between management measures, and (iii) implementation of this knowledge into decision support tools, models and legislation to support farmers and policy makers.
1 Introduction
Recycling of organic resources into agriculture has the potential to greatly increase nutrient use efficiency and soil quality. If done carelessly, however, it can lead to deterioration of groundwater and surface water quality through leaching and runoff of nutrients and polluting agents. Additionally, it can lead to other harmful consequences such as acidification and eutrophication of natural ecosystems resulting from ammonia (NH3) and nitrogen oxide (NOX) emissions and resulting N deposition, or enhanced global warming through emissions of the greenhouse gases nitrous oxide (N2O) and methane (CH4).
Since the 1990s, a series of policies have been implemented in the European Union (EU) to decrease agriculture-related nutrient emissions into the environment and improve the recycling of agricultural resources. Currently, emission reduction is mainly targeted by (i) the national emission ceilings for NH3 and NOX in the EU National Emission reduction Commitments Directive, and the United Nations (UN) Convention on Long-Range Transboundary Air Pollution, (ii) the water quality standards in the EU Water Framework Directive, and the EU Nitrates Directive, and (iii) the greenhouse gas emission reduction targets of the and the UN framework Convention on Climate Change (UNFCCC) (Oenema et al., 2011). Simultaneously, the European circular economy strategy and the Farm-to-Fork strategy aim to recycle resources, including nutrients (European Commission, 2020a). To close elemental cycles, the EU Waste Framework Directive sets ambitious targets of recycling and reuse of municipal waste to a minimum of 65% by 2035 (EU, 2018). Furthermore, with the implementation of the Fertilising Products Regulation no. 2019/1009 (FPR), the EU promotes the use of recycled and organic resources by providing harmonized conditions for the production and use of these materials (EU, 2019; Kurniawati et al., 2023).
Many measures are available to improve nutrient and carbon (C) management and to reduce emissions from organic resources used as fertilizer or soil conditioner. Measures can be categorized in management measures, technological measures, and structural changes. Management measures focus on the efficient use of nutrients and C, by incorporating composition, plant-availability, C stability, and risk of nutrients losses in fertilization strategies. Technological measures to reduce nutrient emissions include changes in livestock housing systems and manure storage, the use of manure treatment techniques, and the use of precision and low emission application techniques of manures (Chadwick, 2005; Hou et al., 2015; Bougouin et al., 2016; Jensen et al., 2020; Van der Weerden et al., 2021). Structural changes in agriculture, such as the reduction or reallocation of livestock numbers, are also options to improve nutrient and C management and reduce emissions from organic resources (Van Grinsven et al., 2018; Kim et al., 2023).
In this paper we review (i) the availability and composition of organic resources in the European Union, (ii) their agronomic value and environmental risks, (iii) the potential measures to reduce their emissions when applied to soils, and (iv) the future challenges to support farmers and policy makers.
2 Availability, composition and use of organic resources
2.1 Composition of organic resources
Organic resource composition can be variable, both within and among fertilizer types. In Table 1 the average dry matter, N, P and organic matter (OM) contents of a selection of the main organic resources used in Dutch agriculture are shown. The N:P ratio of an organic resource is an important parameter for balanced fertilization and to avoid excess application of N or P (see Section 6.1). The N:P ratio is generally higher for cattle manures than for pig and poultry manures (Table 1). The OM:nutrient ratio should be high when organic resources are mainly applied as soil improver and not as a source of nutrients. Vegetable, garden, and fruit-derived (VGF) compost has a higher OM:nutrient ratio than manures and this ratio is higher for solid manures than for slurries. Cattle slurry has the lowest N and P content on fresh product basis. Meat and bone meal is relatively rich in P and has a lower N:P ratio than the other organic resources. The UK Nutrient Management Guide (AHDB, 2023) also presents the composition of a range of organic resources. For cattle slurry, pig slurry, poultry manure, solid cattle manure and solid pig manure, they report values of 2.6; 3.6; 28; 6, and 7 g N kg−1 fresh product, and 0.52; 0.65; 7.4; 1.4; 2.6 g P kg−1 fresh product, respectively. Most of these values are somewhat lower than the Dutch, but the number of samples and the uncertainty level of the UK data is not available. These differences indicate however that the Dutch data -although based on a vast number of samples- may not necessarily be representative for other countries, and that average manure composition can depend on the common livestock system and level of intensity in each country.
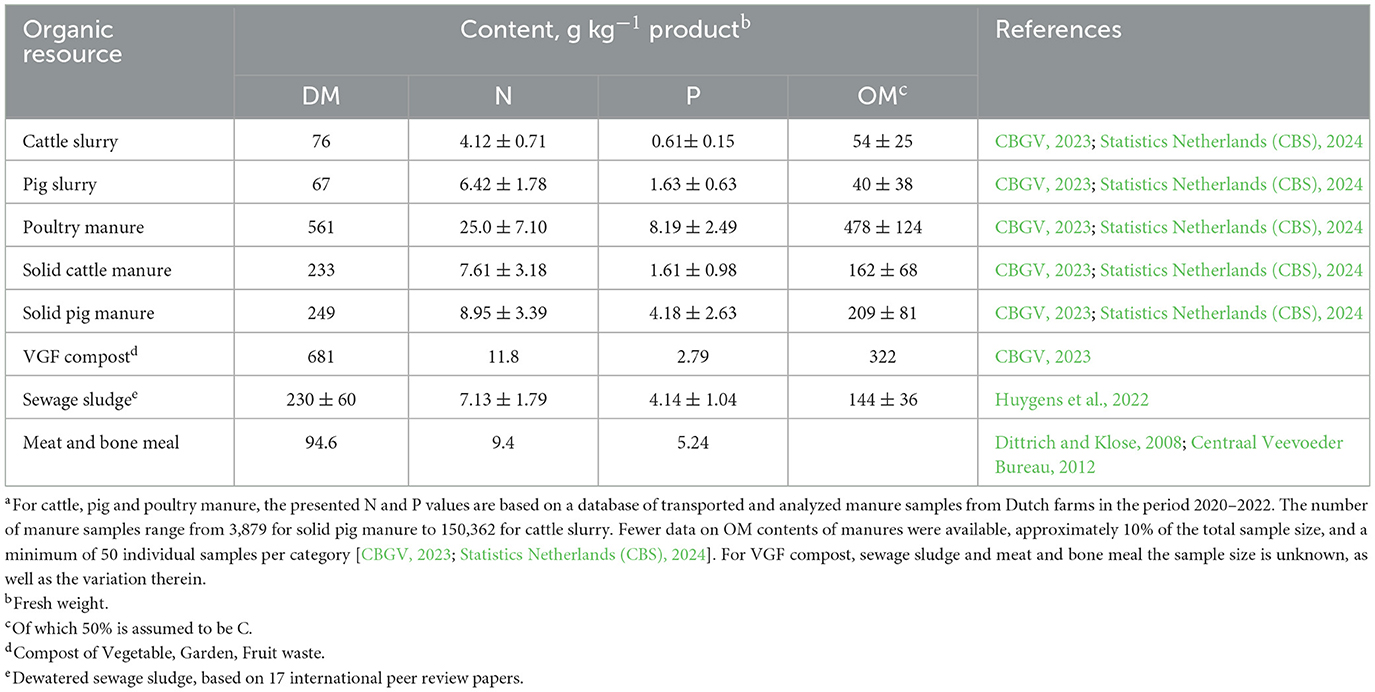
Table 1. Mean ± standard deviation of dry matter (DM), N, P and organic matter (OM) content of organic resources in the Netherlandsa.
2.2 Application of organic resources in EU-27 + UK
The MITERRA-EUROPE model was used to calculate the amount of carbon and nutrients that are applied on agricultural soils in the EU-27 + UK (Table 2). The results show that in total over 78,000 Gg of C is applied through organic resources annually in EU-27 + UK (Table 2). The total amount of organic resource applied to soil amounted 6,970 Gg N (on average 41 kg N ha−1 agricultural land, based on 171.7 million ha arable and grassland), 1,593 Gg P (9 kg P ha−1), and 78,294 Tg C (456 kg C ha−1) in EU-27 + UK in 2017 (Table 2). The amount of N is somewhat less and that of P somewhat higher than the with MITERRA-EUROPE estimated amounts of applied mineral N and P fertilizers, i.e., on average 66 kg N and 7 kg P per ha agricultural land. Solid pig and cattle manure and cattle slurry are the most used organic resources. More than half of the C applied to soils by organic resources in the EU-27 + UK is applied as solid manures. Relatively high amounts of cattle and pig slurries are applied in countries in NW Europe such as Belgium, Denmark, Netherlands, and Germany, whereas solid manures applied are more common in France, Spain, and part of central Europe (Figure 1). The application rate of VGF compost is relatively high in Denmark, Germany, and the Netherlands and that of sewage sludge in Spain, Denmark, Germany and Italy (Figure 1).
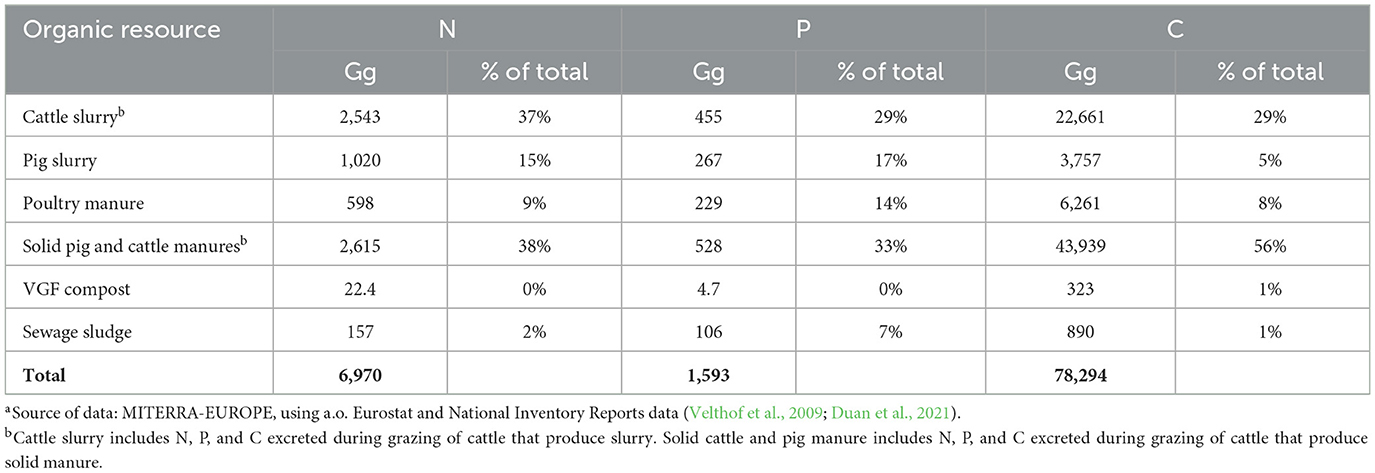
Table 2. Estimates of the amounts of organic resources applied to agricultural soil in EU-27 + UK in 2017a.
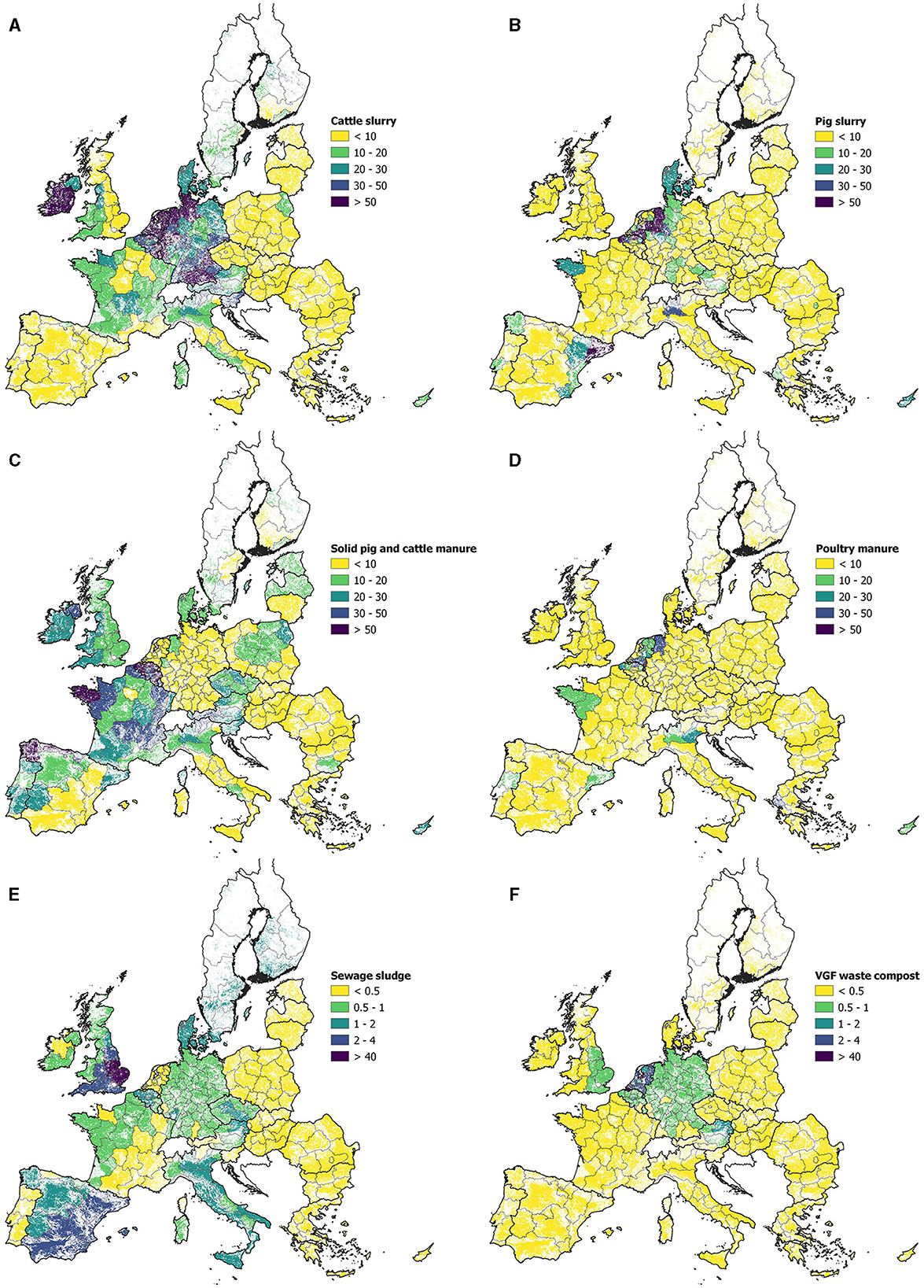
Figure 1. Nitrogen inputs into agricultural soils (expressed in kg N ha−1) via (A) cattle slurry including slurry deposits of grazing cattle, (B) pig slurry, (C) solid pig and cattle manure including solid manure deposits of grazing cattle, (D) poultry manure, (E) sewage sludge, and (F) VGF compost to agricultural land in EU-27 and UK. Notice that the scale is different for (E, F). Average application rates are calculated at NUTS2 level using MITERRA-EUROPE (Velthof et al., 2009; Duan et al., 2021).
Van Dijk et al. (2016) made an assessment of the P flows in Europe in 2005. Phosphorus excreted as manure by livestock was almost fully applied to agricultural land and amounted to about 1,749 Gg P. A relatively small part of bio-waste was composted and used in agriculture in 2005 (15 Gg P), most bio-waste was lost in landfills and incineration (212 Gg P). Part of the sewage sludge in Europe was used in agriculture (137 Gg P) while a larger part was lost by landfilling and incineration (234 Gg P). On average 35% of the produced sewage sludge is applied in agriculture in the EU (Huygens et al., 2022), with very large variations between countries (European Commission, 2022). From the late 1980s onwards, the risk of prion diseases has decreased the recycling of P from animal slaughter waste in Europe. A part (22% in 2022) of the total produced animal slaughter waste has since then been labeled as high risk material (category 1 in the regulations on Animal By-products; EU, 2009; Huygens et al., 2019; Dobbelaere, 2023). This part cannot be used as feed or fertilizer, and is landfilled after incineration or used in cement kilns. Therefore, only a relatively small portion of the waste of slaughtered animals was used as fertilizer (16 Gg P in 2005). The remaining part (281 Gg P) is lost by landfilling and incineration (Van Dijk et al., 2016), although these data could be outdated (Huygens et al., 2019). The total amount of P loss (727 Gg P) by landfilling and incineration of food waste (212 Gg P), sewage sludge (234 Gg P) and slaughtered animals (281 Gg P) in 2005, is high, i.e., more than half of the mineral P fertilizer use (Van Dijk et al., 2016). Phosphorus is a life-essential, irreplaceable element and the fossil P reserves are limited (USGS (U.S. Geological Survey), 2022). As Europe has no significant P mines, it is highly dependent on the import of phosphate ore to produce P fertilizers (De Ridder et al., 2012). Clearly, increased recycling of P from these waste streams can strongly reduce the use of mineral P fertilizers.
There is an ongoing trend in which less municipal waste is landfilled and more waste is recycled, composted and incinerated (Eurostat, 2023). The amount of food waste is currently estimated to be between 158 and 298 kg per person per year in Europe (Corrado and Sala, 2018), which is about 20% of the food produced (Stenmarck et al., 2016).
2.3 Developments in the availability of organic resources
The availability of organic resources for use in agriculture as fertilizer or soil conditioner from food processing, sewage sludge, and municipal bio-wastes is expected to strongly increase over the coming decade, because of the ambitious targets of recycling and reuse of municipal waste of the EU Waste Framework Directive sets (EU, 2018). The collection and recycling of municipal bio-waste is projected to increase in order to reach the EU's objective to reuse and recycle 65% of the municipal waste by 2035 (ECN, 2022). In comparison, in 2022, 48% of the municipal waste generated in the EU was recycled of which 18% organically, through composting and anaerobic digestion (Eurostat, 2023). A large fraction of the municipal waste (42%) is still incinerated or landfilled which offers potential for increasing recycling (Eurostat, 2023). The P recycling potential from waste water remains largely untapped in the EU (Serrano-Gomez et al., 2023). Some countries in Europe, such as Germany and Switzerland, have implemented measures to make the technical recovery of P from sewage sludge mandatory (Hušek et al., 2023), to ensure that P is recycled back into agricultural production. In addition, P recovery from slaughterhouse waste has become obligatory in Switzerland1 (ESPP (European Sustainable Phosphorous Platform), 2015). Further innovations in the development of waste water management, such as the use of urine source separation technologies, will increase the availability of nutrients for use in agriculture (Larsen et al., 2021). The circular economy action plan (European Commission, 2020b) has fostered many research initiatives, exploring a broad range of nutrient valorization pathways like the resource recovery from food-processing waste waters (Durkin et al., 2022). The overall recovery potential for N and P from currently untreated or unutilized waste streams, such as food chain waste, manure and waste water has been estimated to be 4.2 Tg of N and P in the EU, which represents about 35% of the currently used mineral fertilizers in EU agriculture (Chojnacka et al., 2019). The increased recycling of organic resources will decrease the dependence on mineral N and P fertilizers in the EU.
3 Developments in measurement methods to determine agronomic value and risk of emissions
3.1 Methods to characterize composition of organic resources
Data on the composition of organic resources are very important in order to increase nutrient use efficiency and to decrease the risk of emissions to the environment. Measurement techniques are developing, and new techniques become available to characterize soils, manures, crops, and emissions to the environment to improve nutrient management (Bühler et al., 2021; Horf et al., 2022; Reijneveld et al., 2022; Deru et al., 2023; Ge et al., 2023). These data can be used in decision support tools and fertilization recommendation to support farmers in management of nutrients (Section 7.2.1).
Analytical methods used to characterize fertilizing products have recently been standardized by the EU, as a result of the FPR that entered into force on 16 July 2022 (see CEN and ISO technical committees for the proposed and published methods).2 These methods are used for samples with varying composition due to the heterogeneity of organic resources. Furthermore, Member States are allowed to have national regulations for fertilizing products based on national standards and different methods than prescribed in the FPR. Comparison of samples from similar organic resources (e.g. from cattle slurry) and developing datasets about the composition of organic resources therefore remains difficult.
New techniques to determine C, and nutrients in organic resources are near-infrared spectroscopy (NIRS), low-field nuclear magnetic resonance for (NMR) and pyrolysis (Py-GC/MS) (He et al., 2020). Moreover, sensor-based techniques can analyze the total and ammonium N-, P-, K content of organic resources on site or on-board for precision farming with fast access to data and at a low cost (Sørensen et al., 2015; Evangelista et al., 2021; Morvan et al., 2021; Feng et al., 2022; Thiessen et al., 2022; Horf et al., 2024). However, the accuracy of these techniques is often less than classical chemical methods, and depends strongly on the number of reference samples of a specific material (Derikx et al., 2021). Specific techniques for compost, for example to test the stability or biodegradability of compost, such as Oxitop®, rottegrad (self heating test) (Binner et al., 2011), or the presence of plastic (Bläsing and Amelung, 2018), are rarely tested and used for other organic resources.
Besides analysis of their composition, properties related to decomposability of OM in organic resources are often characterized in incubation studies, in which organic resources are applied to soil. Examples are the determination of humification coefficients of organic resources (Section 4.2; Lashermes et al., 2009) and N mineralization. These incubation studies have similarities with pot and field studies to which they can be related (see Section 4.1), but also to measurements of plant-available nutrients such as citrate-extractable P (Delin, 2016), and Potential Mineralizable Nitrogen (PMN) (Westerik et al., 2023).
Many new analytical techniques are available, yet there are still many uncertainties involved in how the data obtained can be used to improve nutrient management. A combination of the aforementioned techniques can help to optimize the use of organic resources and decrease emissions to the environment. However, there are many challenges in the interpretation of the results and the handling of the large number of data generated with these techniques. Representative sampling of organic resources remains a challenge, even for these new analytical techniques.
3.2 Crop growth and yield
The effects of organic resource application on crop growth, yield, and quality are generally obtained by harvesting, weighing, and analyzing crops in a field experiment. Spectrum sensing techniques (e.g., handheld scanners using NIRS), from which information of the status of a crop in a field or field experiment can be rapidly obtained, are currently available and rapidly developing (Burkart et al., 2018; Luo et al., 2022). Crop images from satellites or unmanned aerial vehicles such as drones can also be used to get insight into the development of crops at a larger (field or farm) scale. This type of information can be used to predict crop response to (organic) fertilizers and, where necessary, adjust fertilization using precision farming techniques (Maresma et al., 2016; Zhang et al., 2020), as well as improve crop growth models (Dlamini et al., 2023). Data on crop growth and development in combination with data on nutrient input and availability of organic resources can be used in decision support tools for farmers to decide if a top dressing of a fertilizer is required to obtain the optimal yield (Section 7.2.1).
3.3 Gaseous emissions and water quality
3.3.1 Ammonia emission
There are several techniques currently used for measuring NH3 volatilization from soils after application of (organic) fertilizers (Van Andel et al., 2017); (i) micrometeorological methods such as Eddy Covariance and the upwind-downwind method (Cassel et al., 2005), (ii) static chamber enclosure with acid soaked sponges, or other forms of acid traps, placed within the chamber, (iii) dynamic enclosure by using wind tunnels, usually in combination with an acid trap (Lockyer, 1984; Sintermann et al., 2012), and (iv) satellite instruments such as the Infrared Atmospheric Sounding Interferometer (Dammers et al., 2016; Van Damme et al., 2021). Each technique has its own advantages and disadvantages and it depends on the research question what sampling method and technique are preferred. The micrometeorological method is considered to be the most accurate and reliable, but large field sites usually limit research to only one treatment without replications. On the other hand, methods with a static or dynamic enclosure can be used to investigate several treatments and replicates on a small scale, but there are concerns in terms of the effect of the chamber and consistent underestimation due to a lack of turbulence (Smith et al., 2007; Van Andel et al., 2017). The wind tunnel technique can also be used to investigate treatments with replicates and is usually considered to be more accurate than static chamber designs, yet standardizing air displacement and the costs of production and analytical analyses limit their application in research.
3.3.2 Nitrous oxide emission
Detection techniques of N2O emissions from the field can roughly be categorized in two groups: chamber methods and micrometeorological methods. Chamber methods are the most commonly used approach and measure the accumulation of gas in a known volume (Clough et al., 2020; Grace et al., 2020). Both manual (where the chamber is closed and opened by an operator) and automated chambers (where the chambers are opened and closed through a pneumatic system) exist with both their advantages and disadvantages (Rapson and Dacres, 2014). The second type of sampling technique comprise micrometeorological methods. The most widely used is the Eddy Covariance method in which the emission of a gas is defined as the covariance between the gas concentration and the vertical wind (Hensen et al., 2013). Other, similar techniques are the relaxed eddy accumulation method, mass balance and plume methods, and tall tower measurements (Hensen et al., 2013; Rapson and Dacres, 2014). Analytical techniques for gas measurements include chromatographic techniques, optical techniques and amperometric techniques. Optical techniques are based on the ability of a gas to absorb infrared light (IR) at unique wavelengths. Examples of used IR detectors are Fourier transform infrared spectrometers (FTIR), photo-acoustic instruments, laser-based systems such as tunable diode laser (TDL) and quantum cascade lasers (QCL) and cavity ring down spectrometers (CRDS). The amperometric method determines N2O concentrations by measuring the produced N2O from the reduction of N2O at an electrode (Hensen et al., 2013; Rapson and Dacres, 2014). The development of these different techniques have recently enabled reliable measuring N2O concentration at extremely low concentrations. Nonetheless, each technique has its own advantages and disadvantages and it really depends on the research question and site what sampling method and analytical technique is preferred or possible.
3.3.3 Water quality
The effect of fertilization on water quality is generally assessed by the occasional sampling of groundwater or surface water, followed by chemical analysis of the water sample in a laboratory. Sensor techniques for in-field analysis are currently available and will be further developed for direct and automatic determination of concentration of nutrients in the field at a high frequency (Rode et al., 2016; Jomaa et al., 2018). These sensors, sometimes in combination with software applications, can give researchers, farmers, water authorities and regional governments continuous insight into water quality and effects of specific events, such as fertilizer application or weather events, on water quality. Insight in the temporal and spatial variability of water quality in relation to the use of organic resources can be used to derive strategies to decrease leaching to groundwater and surface water.
4 Agronomic value of organic resources
4.1 Nutrient availability
Plant nutrient availability of organic resources can be quantified by their Nitrogen or Phosphorous Fertilizer Replacement Value (NFRV or PFRV, respectively), which specifies the amount of mineral fertilizer needed for a similar crop response as the organic resource (Schröder et al., 2007; Schils et al., 2020). Accurate FRVs are required to underpin fertilizer recommendations and maximize nutrient use efficiencies. FRVs are mostly applied to N, and NFRVs can be determined in a field or pot experiment in which the rates of organic and mineral fertilizers required to obtain equal crop N uptake are compared (Jensen, 2013; Westerik et al., 2023). NFRVs are commonly determined for the first year after application (i.e., short-term FRVs) and the variation in FRV is large, as is shown by two review papers in Table 3. Variation can be caused by variation in organic resources composition, time and method of application, climatic conditions, soil properties, reference fertilizer and test crop (Jensen, 2013). For example, the first year NFRV of cattle slurry can increase from 36 to 53% when injected instead of surface applied, most likely through a reduction in NH3 volatilization (Schröder et al., 2007). NFRVs are expected to increase over time because of mineralization of residual organic N in the years after application (Gutser et al., 2005; Schröder et al., 2007; Hijbeek et al., 2018).
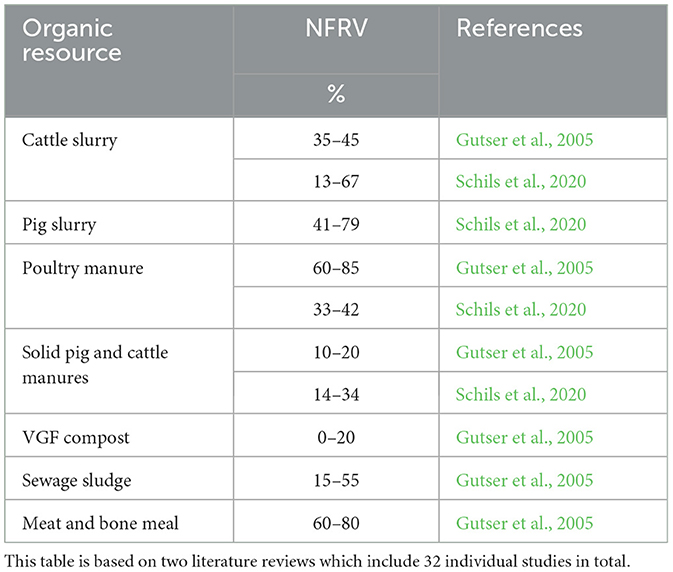
Table 3. Range (minimum–maximum) of Nitrogen Fertilizer Replacement Values (NFRVs) of different organic resources, expressed as % of mineral N fertilizer which can be replaced by N from the organic resource in the first year of application.
Since field experiments for the determination of FRVs are time intensive and costly, attempts are being made to predict FRVs based on easily obtainable chemical properties. Total N, C:N, mineral N and Potential Mineralizable Nitrogen (PMN) appear to be good predictors for NFRV in pot and field experiments, and citrate-extractable P can predict PFRVs (Stadler et al., 2006; Delin et al., 2012; Delin, 2016; De Notaris et al., 2018; Westerik et al., 2023). NFRVs generally increase with higher total and mineral N contents, lower C:N ratios and higher PMN (Tables 1, 3), but the strength of these correlations needs to be tested under a range of field conditions. This is especially relevant with the rapid emergence of new organic resources, and the need for product-specific recommendation rates and FRVs. These new products can in theory have improved plant nutrient availability, but adequate FRVs are required to underpin fertilizer recommendations and maximize the nutrient use efficiency of these products.
NFRVs of organic resources are often used in national fertilizer recommendations and decision support tools as well as action programs of the EU Nitrates Directive. Currently, there are striking differences of assumed NFRVs between European countries, therefore large differences exist in recommended or allowed rates of organic resources. For example, the NFRV of farm yard manure (FYM) is assumed to be 10% in the UK, whereas it is assumed to be 60% in Germany (Jordan-Meille et al., 2022). Such differences can result from alternative calculation methods, experimental setups and timeframes (Gutser et al., 2005; Jensen, 2013; Schils et al., 2020; Westerik et al., 2023). For example, some countries (Belgium, Switzerland, Italy and Germany) take into account long term effects (>1 year) of fertilizer application on yield, whereas other countries do not. There is a reasonable agreement between countries in the order of decreasing NFRV, i.e., pig slurry > cattle slurry > poultry manure > FYM (Webb et al., 2013). However, there are also differences in NFRV among countries which are, besides the calculation method and considered period, probably also due to differences in application time and method. Standardization of calculation methods should serve to improve N use efficiency of organic resources.
Besides their supply of N and P, organic resources also supply other nutrients and trace elements (Sager, 2007). Furthermore, repeated organic resources application can improve soil properties such as bulk density, aggregate stability, infiltration capacity and water retention, although these effects are largely dependent on soil and crop type (Fu et al., 2022). Organic resource application can therefore benefit soil fertility and improve yields beyond the supply of N and P (Hijbeek et al., 2018; Kok et al., 2023).
Although knowledge of NFRVs of different organic resources is rapidly emerging, insight in the chemical properties to explain and predict variability in NFRVs as well as standardization of determination methods of NFRVs is required for adequate fertilizer recommendations of new and existing products. Besides, data of supply of P, K and other macro- and micronutrients from organic resources to crops is still scarce.
4.2 Carbon stability
The addition of organic resources influences soil organic carbon (SOC) by the addition of organic material and by increasing aggregate stability and the organic C content of aggregates (Yilmaz and Sönmez, 2017). Generally, the potential to sequester C in the soil increases with increasing C:N rate of organic resources (Hijbeek et al., 2019).
The decomposability of organic resources varies largely between and within different groups of organic resources (Lashermes et al., 2009; Levavasseur et al., 2022). Levavasseur et al. (2022) found that for example the mineralization of C from animal manures varied between 0 and 500 mg C per g added C for incubation studies lasting an equivalent of 1 year under field conditions. To predict C mineralization in the field after organic resource application, Levavasseur et al. (2022) recommend to calibrate models per product and not per fertilizing product group. In addition, they recommend to also include product characteristics such as the Van Soest analysis of fiber (Van Soest and Wine, 1968).
The humification coefficient (HC) is the percentage of applied OM that is not decomposed after 1 year of soil application, and is used as an indicator for OM stability of fertilizing products. HCs are used in fertilizer recommendations and in simple models for estimation of C mineralization after application of OM (Janssen, 1984; De Neve et al., 2003; Egene et al., 2021). The HC is highly variable within and among different types of organic resources (Table 4). Several factors may influence the HC, such as climatic factors, soil characteristics, characteristics of the input material, and anthropogenic activities. Additionally, the relationship with initial SOC content and HC values was, based on a mathematical method, found to be non-linear: a higher SOC content leads to lower HC values (Tan et al., 2014). The wide ranges reported in Table 4 imply that using a single HC per fertilizer type for calculations of C build up, may lead to significant over- or underestimations (of up to 30%).
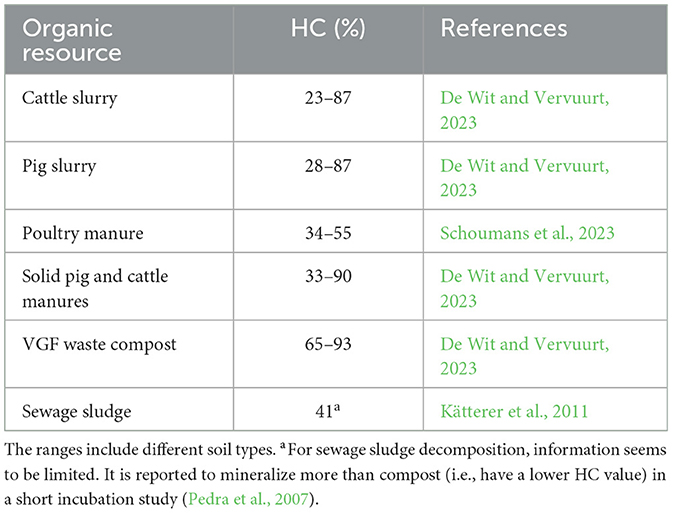
Table 4. Range (minimum–maximum) of humification coefficients (HC) of different organic resources, expressed as fraction of the applied organic matter that is not decomposed within the first year after soil application.
Other parameters used to describe C storage potential or mineralization include the indicator of remaining organic carbon (IROC) (Lashermes et al., 2009), the humus production capacity (VDLUFA, 2021), and the cumulative C-CO2 emissions over a chosen period of time (Mondini et al., 2017). All of these terms point toward decomposability trends with C from animal by-products including meat and bone meal being most decomposable and C from compost being least decomposable. For Lashermes et al. (2009), VDLUFA (2021) as well as Mondini et al. (2017), the fact remains that these decomposability terms vary strongly within product groups.
In short, commonly, animal by-products are most easily decomposed, followed by sewage sludge, manure and compost, but the variation in C mineralization is very high within the categories.
5 Environmental consequences
5.1 Ammonia emission
Ammonia emissions after application of manures or other organic resources can constitute a major N loss pathway from animal farms. Loss of NH3 through volatilization reduces N use efficiency of the fertilizer, contributes to the formation of airborne particulate matter, and may induce acidification, eutrophication, and indirect N2O emissions after deposition (Behera et al., 2013; Räbiger et al., 2020). Emission patterns differ for various fertilizer types. For liquid fertilizers, such as slurry and digestates, volatilization typically occurs during the first hours or days after application, whereas for solid manures, composts, and plant residues NH3 emissions are typically lower and can be spread out over a longer timeframe, as the OM in the fertilizer breaks down. Generally, emissions are higher for liquid manures and slurries with high levels of available N, such as slurry digestates or liquid poultry manure, than for solid manures and composts (Table 5). In an elaborate screening of over 30 biobased fertilizers, Wester-Larsen et al. (2022) reported a high NH3 volatilization potential for digestate products, and a low potential for solid products like composts.
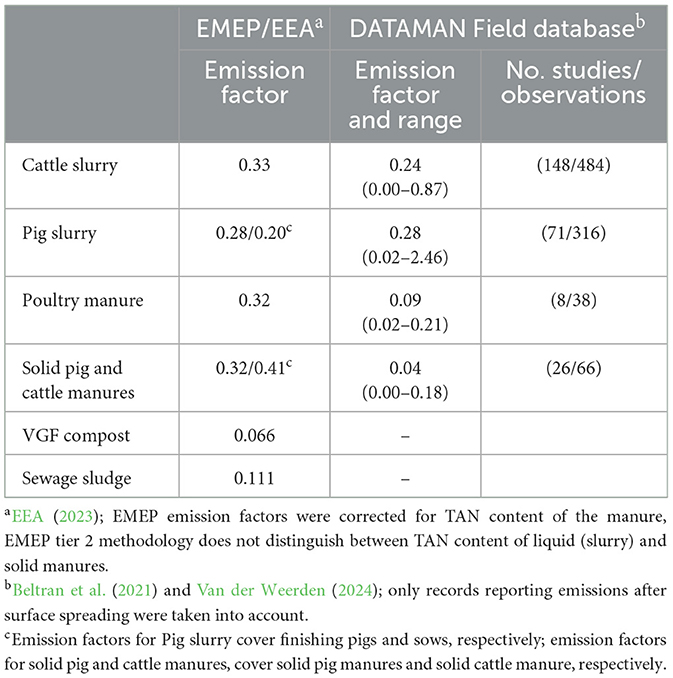
Table 5. EMEP/EEA NH3 emission factors of different surface applied organic resources and averages (and range) for the same fertilizer types derived from the DATAMAN field database, expressed as fraction of total N applied.
Composition strongly affects the magnitude of NH3 losses from organic resources, especially the total N and ammoniacal N contents and the fertilizer pH. Moreover, as NH3 volatilization strongly depends on the chemical equilibrium between ammonium, dissolved NH3, and gaseous NH3, environmental factors such as temperature, wind speed, soil moisture, and contact surface between the fertilizer and air play a crucial role. Mitigation strategies can therefore be aimed either at altering the composition or chemical properties of the fertilizer, e.g., by acidifying manures (Wagner et al., 2021; Silva et al., 2022; Pedersen and Nyord, 2023) or treating them with plasma (i.e., acidifying slurry and adding NO2 and NO3 by N fixation) (Graves et al., 2019), or at ensuring that the environmental conditions are unconducive for NH3 volatilization. The latter strategy includes applying liquid fertilizers shortly before rainfall events and applying water after slurry application (Webb et al., 2014a), by injecting or incorporating fertilizers into the soil (Thompson and Meisinger, 2002; Powell et al., 2011; Dell et al., 2012; Webb et al., 2014a; Wester-Larsen et al., 2022), or by covering the fertilizer with a foamlike substance to reduce air contact (Park et al., 2006; Lee et al., 2007; Bajagain et al., 2022). Generally, assuring quick infiltration of liquid organic resources into the soil and minimizing their exposure to air may reduce NH3 volatilization after application. However, the aforementioned measures may in turn lead to pollution swapping to e.g., enhanced NO3 losses or N2O emissions (see also Section 7.1).
National inventories for reporting NH3 emissions from agriculture often make use of emission factors that describe the cumulative emissions from a source as a fraction of total or ammoniacal N (Table 5). There are examples of national Tier 2 or Tier 3 emission calculation methodology that include emission factors for specific NH3 abatement measures, e.g., by differentiating for various application methods in The Netherlands (Van der Zee et al., 2021), but generally national inventories do not include the option to account for these measures. Emission factors for new organic resources in combination with abatement measures are desirable for accurate calculation of NH3 emissions and for providing farmers and governments with a means of accountability for management actions taken. Deriving these emission factors will be a major challenge, complicated by the increasing number of products and measures, and by the inherent difficulty of accurately determining NH3 emissions. Recent efforts to synthesize information on NH3 emission in databases with information from lab, field and model studies that describe emission data (Hafner et al., 2018; Beltran et al., 2021) can provide context for newly derived emission factors.
5.2 Nitrous oxide emission
Application of organic resources to soil can result in N2O emissions through several processes, of which the most important are nitrification—the oxidation of NH to NO and NO with N2O as an intermediate product, and denitrification—the reduction of NO3 to N2 with N2O as a product of incomplete denitrification (Chadwick et al., 2011). In the last decades many studies focused on N2O emissions following manure application (Chadwick et al., 2011; Thorman et al., 2020). The emission factors of manures (i.e., the cumulative N2O-N loss as a proportion of total N applied to soil via manure) can range from 0.1 to 3.3% (Chadwick et al., 2011; Thorman et al., 2020; Van der Weerden et al., 2021), reflecting differences in manure type and composition, soil type, management and climate (Table 6). The emergence of manure processing techniques leads to new organic resources (Section 6.2.1). The composition of these products varies strongly (Chadwick et al., 2011), and so do the N2O emissions following application to soil. However, many countries rely on the IPCC tier 1 default N2O emission factors, in which the type of organic resource, soil type and application method is ignored. This leads to large uncertainties in the quantification of N2O emission at local to global scales (Tian et al., 2020). The default aggregated IPCC-2019 N2O emission factors are 0.3–0.4% of N applied for manures and 1.0% for other organic resources (IPCC, 2019).
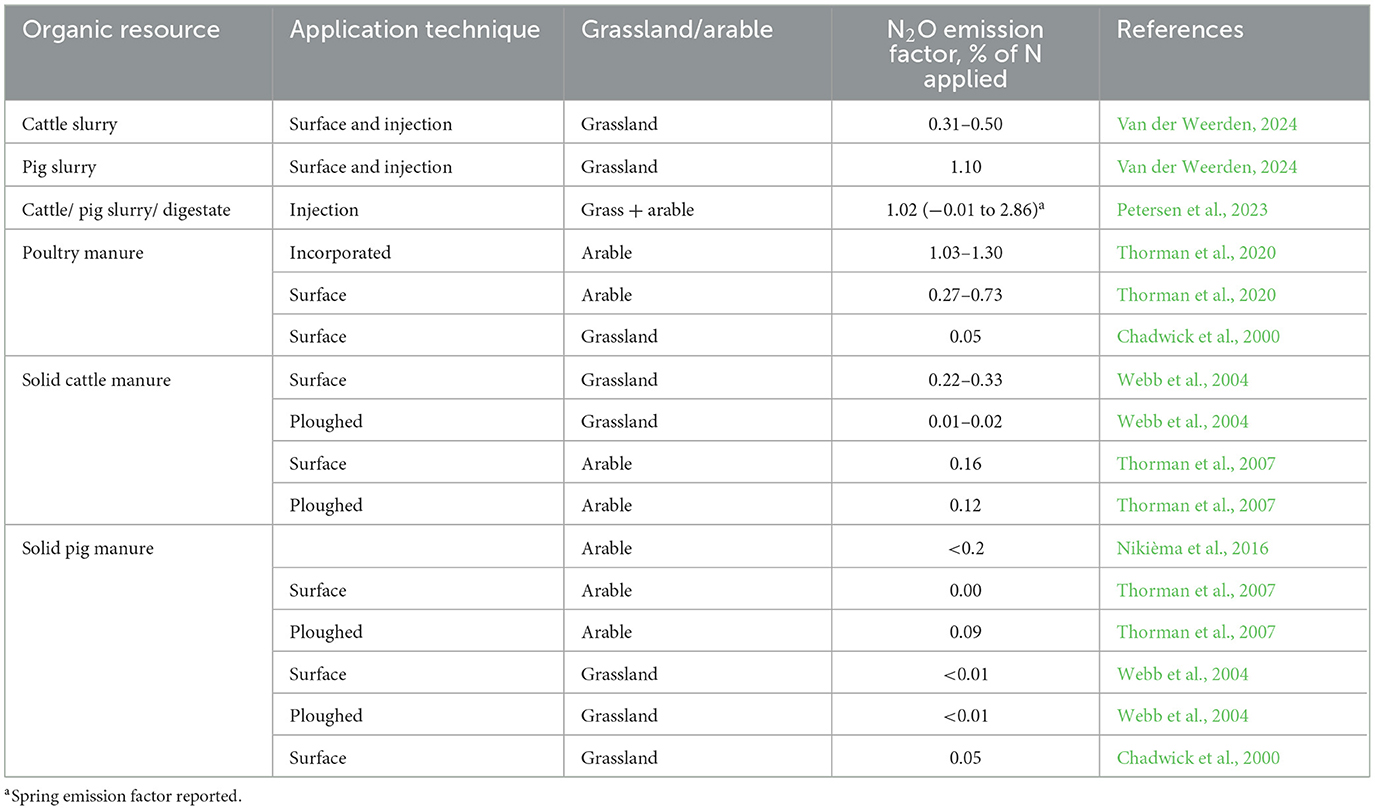
Table 6. Average or range (minimum–maximum) of N2O emission factors of different organic resources and application techniques, expressed as % of N applied.
Incorporation of slurry into the soil has already been shown to be an effective method to reduce NH3 emission (Section 5.1), however some studies have shown that soil injection increases the risk of N2O emission, pointing to a risk of pollution swapping (Velthof and Mosquera, 2011). Others report similar N2O emissions between broadcast and soil injection (Vallejo et al., 2005). The 4R nutrient management strategy (Section 6.1), including a lower application rate, splitting of N doses, and the application of nitrification inhibitors (Herr et al., 2020; Thorman et al., 2020) could balance the input and output of N and subsequently reduce the N2O emission. Organic resource application should furthermore be timed to avoid application during wet conditions, actively support crop growth, and reduce excess N in the soil as it could result in large quantities of N lost by NO3, which is in turn an indirect source of N2O emission.
It is assumed that the risk of N2O emissions from VGF compost, sewage sludge and meat- and bone meal are low, because of the generally lower mineral N content of these fertilizers.
5.3 Methane emission
Methane is produced by methanogenic bacteria under strict anaerobic conditions (Zeeman, 1991). Agricultural soils are generally well-aerated and are therefore not a source CH4, but can rather act as a sink of CH4 (Hansen et al., 2024). During storage of liquid manure, however, CH4 may be produced from easily degradable C components in the manure, such as volatile fatty acids (Kupper et al., 2020). After application to soil, part of the CH4 produced during storage and which is dissolved in the slurry can be released (Chadwick et al., 2011). However, this is not CH4 produced in soils. This does not hold for wetland rice soils, which are a large source of CH4. Application of organic resources to wetland rice may increase CH4 emission (Hu et al., 2023). The surface area in Europe used for wetland rice production is extremely low, thus risk of high CH4 emissions from agricultural soils in EU remains negligible.
5.4 N and P leaching and runoff
It has been hypothesized that N from organic resources has a larger risk of being lost by leaching than mineral N due to the untimely mineralization of fertilizer-derived organic N outside the growing season. This idea has likely also been taken into account when setting the specific limit for animal manure of 170 kg N ha−1 in the Nitrates Directive (EEC, 1991) to prevent nitrate leaching from agriculture. However, use of labeled N shows that only a few percent of leached N in 1 year originates from the N fertilizer applied in that year, both for mineral N and organic resource N (Powlson et al., 1986; Choi et al., 2004; Frick et al., 2022). In the long term, buildup of soil organic N by crop residues and organic organic resources is the most important source of N leaching (Goulding et al., 2000). Nitrate leaching increases with long-term N application (Blicher-Mathiesen et al., 2014; Wang et al., 2019), and wrong timing of application, for example the application use of pig manure in autumn (Shepherd and Newell-Price, 2016). A meta-analysis by Ren et al. (2022) shows lower N leaching at higher SOC contents. A meta-analysis by Wei et al. (2021) shows that substitution of a part of the applied mineral N by animal manure N results in limited yield loss and decreases N leaching on average with 30%, while yields do decrease at large substitution rates (Ren et al., 2022). Another study showed that organic inputs in addition to mineral N did not affect leaching and runoff, but also did not improve yield (Wei et al., 2021). Application of easily available C via organic amendments can decrease N leaching by enhancing denitrification, but this might also decrease overall nutrient use efficiency (Qin et al., 2017). A meta-analysis showed a 13% decrease of nitrate leaching due to biochar addition although the mechanisms behind this remain unclear (Borchard et al., 2019).
Phosphorus leaching from soil due to various P fertilizers is mainly driven by the P surplus in the long-term and not by fertilizer type or composition (Blake et al., 2003; Eichler-Löbermann et al., 2007; Messiga et al., 2015; Lemming et al., 2019). In the case of long-term use of certain fertilizers, such as calcium-rich poultry manure (Lehmann et al., 2005), iron-rich sewage sludges, or organic P rich sources, specific P forms can be formed in soil with different leaching susceptibility (Liu et al., 2020). A long term experiment with animal manure resulted in a lower P sorption to the soil in comparison to six other types of organic resources (composts, sewage sludges) (Nest et al., 2016), implying a higher risk of P leaching of manure than other organic resources. For runoff, risk assessment tools such as P indices often rely on landscape and management factors to estimate the risk of losses (Osmond et al., 2017; Ros et al., 2020). Distance to the closest waterways, slope, the use of cover crops and the timing and method of (organic) fertilizer application are examples. Additionally, weather conditions like heavy rainfall after application of manure increases risk of surface runoff of N and P toward surface water and these events may contribute to a large extent to annual N and P leaching from applied manure (Van der Salm et al., 2012).
5.5 Contaminants
Application of biodegradable waste material is challenged with avoidance of contamination of the soil, especially with “emerging contaminants”, such as microplastics, nanoparticles and pharmaceuticals (Ng et al., 2018; Kacprzak et al., 2022). Therefore, the research on waste processing to produce safe and sustainable organic resources is still ongoing (Kurniawati et al., 2023), while at the same time recent EU legislation has banned synthetic polymer microparticles from various products (REACH EU 1907/2006) and fertilizing products (EU 2019/1009) within a transitional period of 5 years.
In Table 7 an overview of heavy metals in selected organic resources is presented. The heavy metal content is generally very low in struvite and meat and bone meal (Möller and Schultheiss, 2015), and higher in manure, compost and sewage sludge (Dach and Starmans, 2005; Smith, 2009; Kupper et al., 2014). The contents of Cu and Zn in animal manures are generally relatively high compared to those of other heavy metals. Cu and Zn in animal manure often originate from additives in feed (Adamse et al., 2017). The composition of these additives is controlled in the EU since 1970 (70/524/EEC) by limit values for Cu and Zn specific for each animal type, which have been reduced since then (EU, 2009). However, the limit values for Cu and Zn in the FPR are sometimes still exceeded, making these manures unsuitable for marketing as EU fertilizer. In contrast to Cu and Zn, the Cd and Pb contents in animal manure are low in comparison to compost and sewage sludge (Table 7).
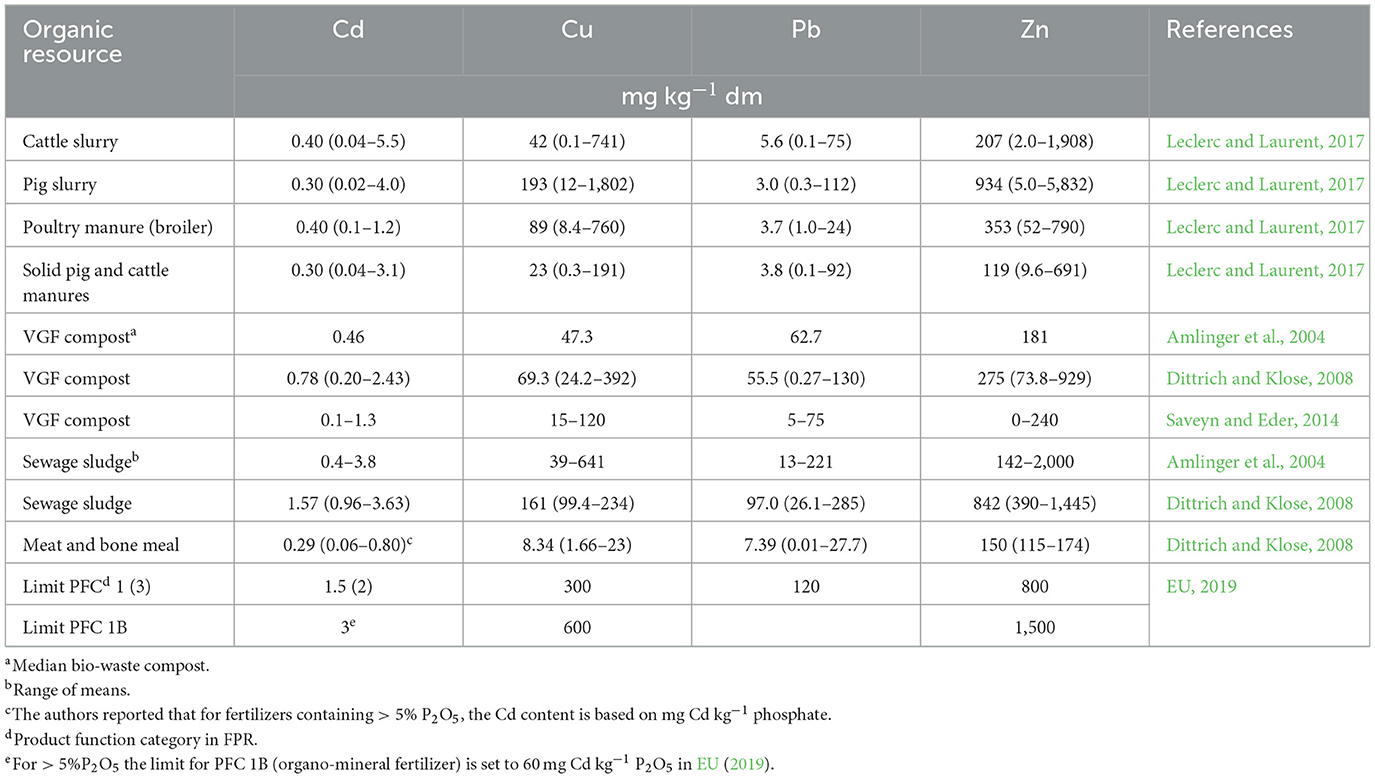
Table 7. Average and/or range (minimum–maximum) of a selection of heavy metals (Cd, Cu, Pb, and Zn) of some organic resources in mg kg−1 dry matter.
Levels of persistent organic contaminants, such as per- and polyfluoroalkyl substances (PFAS), polycyclic aromatic hydrocarbons (PAH), poly chlorinated biphenyls (PCB), dioxine-like PCBs (dl-PCB) and dioxines and dibenzofurans (PCDD/F), are normally very low in animal manure (Berset and Holzer, 1995; Munoz et al., 2021), but can be elevated in products such as sewage sludge (Munoz et al., 2021; Huygens et al., 2022) and compost (Brändli et al., 2007a,b; Saveyn and Eder, 2014; Huygens et al., 2020; Munoz et al., 2021). Limit values of organic contaminants have been set in the FPR for certain input categories (Component Material Categories: CMC) for the production of fertilizers (Table 8). There are concerns about the bioaccumulation and toxicity of pharmaceuticals from both manure and sewage sludge (Gworek et al., 2021). In some cases the use of pyridine herbicides that have a relative high toxicity for plants but not for grazing animals can result in herbicide levels in manure from grazing animals that are too high for use as a general fertilizer. This has resulted in proposed limit values for EU fertilizer products from animal manure for clopyralid and aminopyralid (Huygens, 2023). Some animals receive antibiotics that end up in animal manure. These may potentially leach from the soil or be harmful for soil biota (Rietra et al., 2023) if degradation is slow and sorption is low. Additionally, they may pose a threat to insects living in dung (Bruinenberg et al., 2023) and birds living on cattle farms (Buijs et al., 2022).
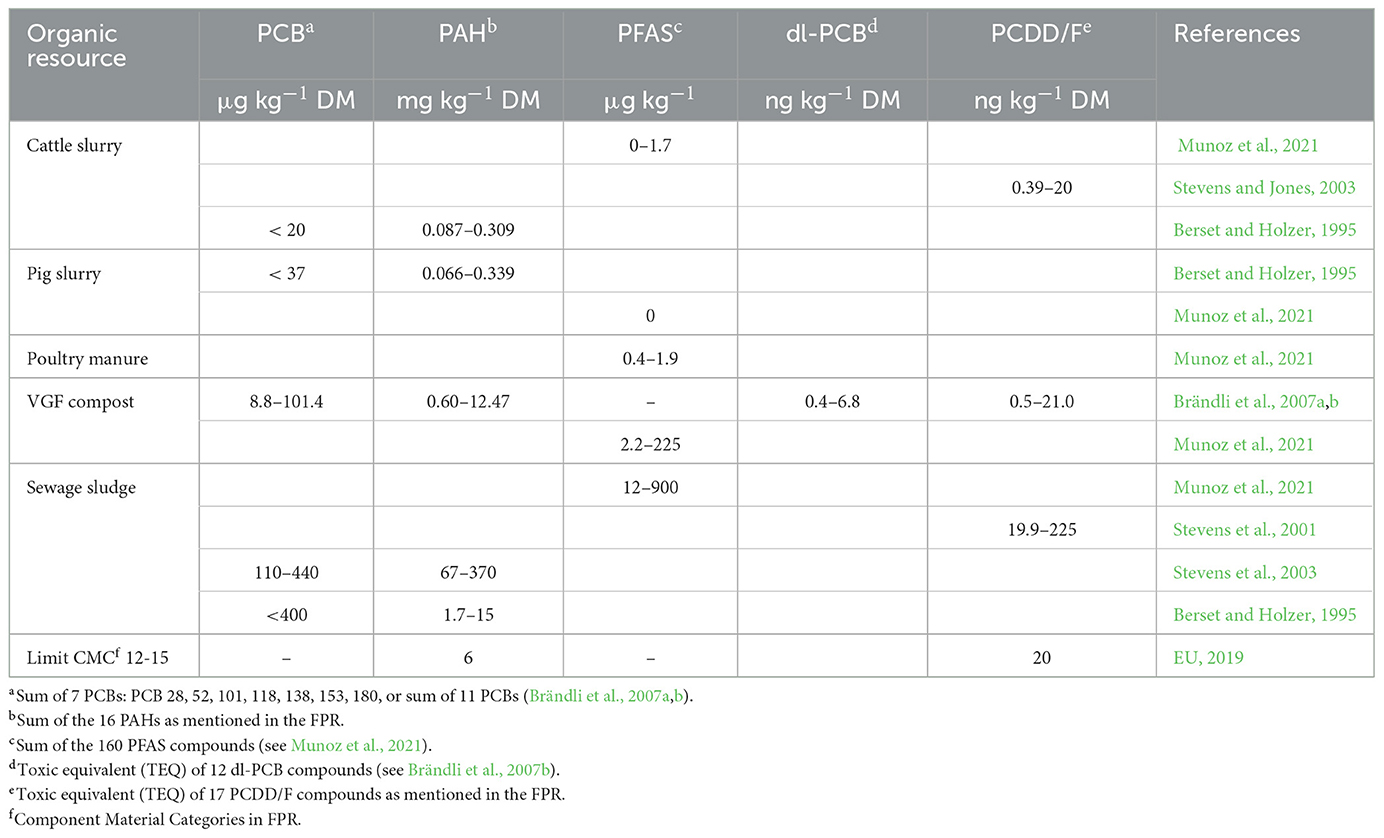
Table 8. Average and/or range (minimum–maximum) of a selection of organic contaminants (PCB, PAH, PFAS, PCDD/F) of some organic resources.
It has been estimated that 67 and 83% of sewage sludges (on the short- and long term, respectively) fails to comply with current limit values, if no pollution prevention is implemented (Aubain et al., 2002). For sewage sludge (ashes), techniques to extract phosphate are promoted by research (Canziani et al., 2023), which seems especially relevant for sewage sludge that does not comply with the limit values.
In summary, many types of contaminants exist and their prevalence and concentrations vary with the type of fertilizing product, e.g., levels heavy metals and pharmaceuticals are higher in manure and other contaminants are more concentrated in e.g., sewage sludge.
6 Potential mitigation measures to reduce emissions from organic resources
6.1 Management measures
The 4R nutrient stewardship guidelines are a framework that describes practices for the application of fertilizers to increase nutrient use efficiency and reduce environmental footprints (Johnston and Bruulsema, 2014; Flis, 2017, 2018). The practices focus on fertilizer application using the Right source, the Right rate, at the Right time, in the Right place. Management of organic resources according to these principles has the potential to increase nutrient use efficiencies and mitigate emissions to the environment. According to a synthesis of several meta-analyses by Young et al. (2021), 4R strategies generally positively impacted crop yield, crop N uptake, and soil organic carbon (SOC), while reducing N2O emissions, NH3 emissions and N surplus. Replacing mineral fertilizers with organic resources increases the SOC content and results in reductions of N losses. Moreover, nutrient management generally has a larger effect on sustainability indicators compared to crop, soil and water measures (Young et al., 2021). The 4R nutrient stewardship guidelines can be based on a decision support tool approach using combinations of soil, crop, organic resource, and weather data (Section 7.2.1.) Decision tools can also include the risk of emissions and measures to mitigate these emissions, although currently most decision support tools only focus on fertilizer recommendations (Nicholson et al., 2020).
For animal manures and other organic resources, however, it is likely that proper 4R nutrient stewardship is more difficult to attain compared to mineral fertilizers. Animal manures, for instance, usually contain multiple nutrients in different ratios, which requires careful consideration of the right source to meet crop demand of certain nutrients without under- or overapplication of others. Herein lies an increased risk of pollution swapping, for instance between NH3 volatilization, N leaching, and N2O emissions, compared to mineral fertilizers.
Since nutrients are often (partly) in organic forms and mineralize over time, control of nutrient release from organic resources remains a challenge, as it is partly governed by external factors such as moisture and temperature. Repeated applications of organic resources increases N mineralization in following years (Schröder et al., 2007; Jensen, 2013; Bhogal et al., 2016; Sorensen et al., 2017). The right time of application is essential to limit the mineralization and loss of nutrients outside of the crop growing season. For manures however, the window of application may be limited by on-farm storage capacity. The right placement of organic resources (near the crop roots) can be more challenging since regulations or available techniques do not always allow this.
Proper implementation of 4R strategies in combination with new measurement and processing techniques have great potential to reduce losses and increase nutrient use efficiency for organic resources, especially if they partly replace mineral fertilizers. Despite the universality of the 4R principles, optimizing strategies for application of organic resources requires tailoring them to specific environmental and management contexts (climate, soil type, cropping system, etc.). Herein still lies a big challenge.
6.2 Technological measures
6.2.1 Manure treatment
There is a wide array of available technologies to process manure (Figure 2). The development of anaerobic digestion for biogas production has given rise to digestate which is commonly used as fertilizer (Saveyn and Eder, 2014). In addition, innovative technologies were developed such as (physico-)chemical treatment, stripping, and membrane filtration to recover N and P and to produce soil conditioners (Lemmens et al., 2007; Foged et al., 2011; Ehlert and Schoumans, 2015). Separation technologies such as screw press, centrifuge, belt press with and without flocculants/polymers are used to separate manures into a liquid and solid fraction with different compositions (Aguirre-Villegas et al., 2019; Guilayn et al., 2019; Grell et al., 2023). Ammonium sulfate and ammonium nitrate are derived by stripping of ammonia with sulfuric acid or nitric acid, respectively (Lemmens et al., 2007; Foged et al., 2011; Ehlert and Schoumans, 2015). From the liquid fraction of manure, mineral NP(K) concentrates are produced using reversed osmosis technologies (Aguirre-Villegas et al., 2019; Guilayn et al., 2019; Grell et al., 2023). Evaporators are used to produce ammonia solutions. From the solid fraction, the main recycled P products are struvite and sometimes calcium phosphate (Sigurnjak et al., 2019; Brienza et al., 2020). Recycled C-rich products such as biochars and other soil improvers are produced through drying, gasification or pyrolysis of the solid fraction (Rathnayake et al., 2023).
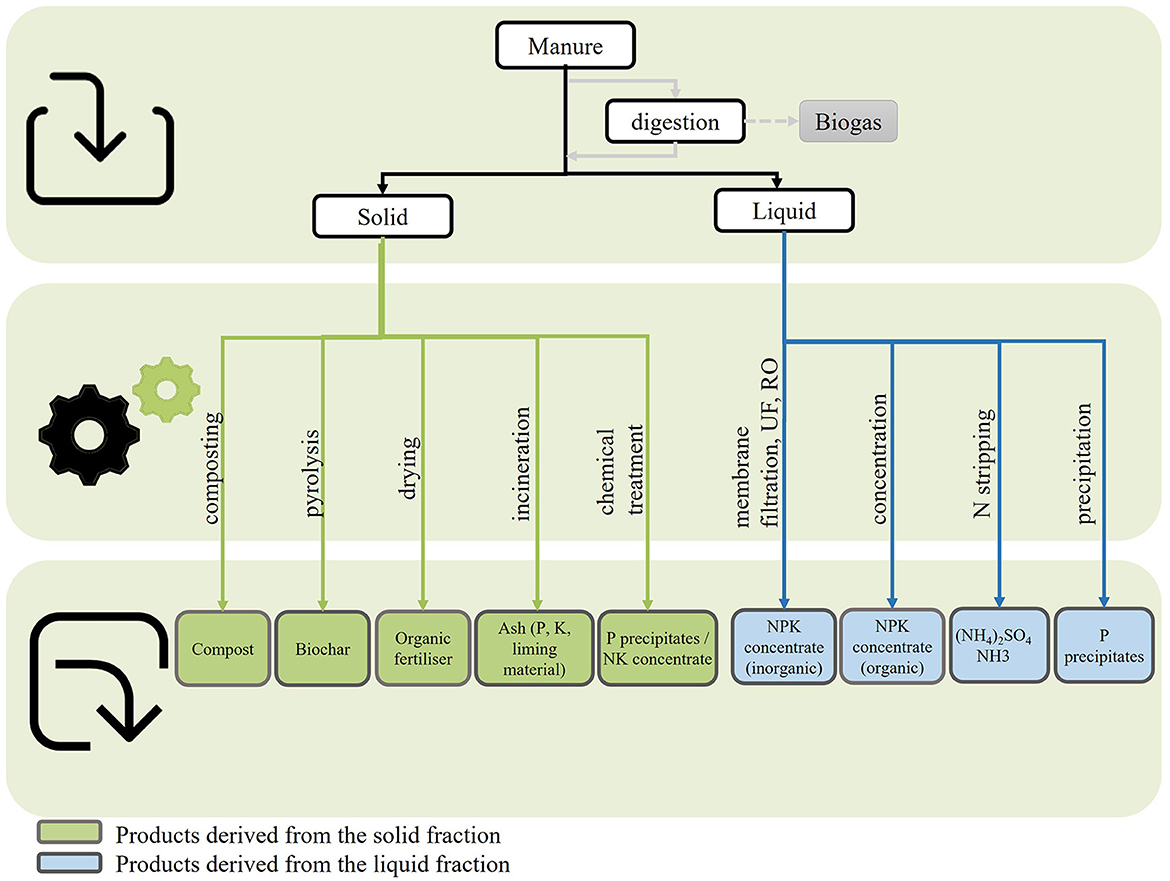
Figure 2. Overview of the main technologies of manure processing (adapted from Ehlert and Schoumans, 2015), with the different input products (top), the various processing techniques (middle), and the corresponding fertilizers or soil improvers (bottom).
In addition to the aforementioned processes, innovative technologies are developed to alter the manure composition, such as permeable membranes, bipolar membrane electrodialyse, forward osmosis, biological reactors, ion exchange with selective resins and plasma technology (Graves et al., 2019). With these developments ultimately a palette of tailor-made fertilizers can be created that can be used to increase nutrient efficiency and reduce nutrient losses to the environment. Recovered ammonium nitrates and ammonium sulfates have higher NFRVs than pig manure and pig urine (Saju et al., 2023), and the P availability of separated solid fractions of manure is high as long as no metal salts such as iron salts are used during processing (Regelink et al., 2021). A constraint in the application of recovered products in EU is that according to the Nitrates Directive (EEC, 1991) all N products recovered from processed manure are still considered as manure and fall under the manure application standard of 170 kg N ha−1 (Huygens et al., 2020).
Table 9 shows examples of manure treatment products. The liquid fraction is a N fertilizer, low in P and OM. Reversed osmosis increases the nutrient concentration in the liquid fraction. The solid fraction is rich in OM and P, but also contains N. The fraction of total N that is present as mineral N was 71% in untreated pig slurry, 92% in the concentrated liquid fraction, and 46% in the solid fraction of this slurry in a study of Hoeksma and De Buisonjé (2020). This indicates that the N in the concentrated liquid fraction is significantly higher and more plant-available than the N of the solid fraction. Ammonium sulfate recovered via air scrubbing is a liquid N fertilizer without P and OM.
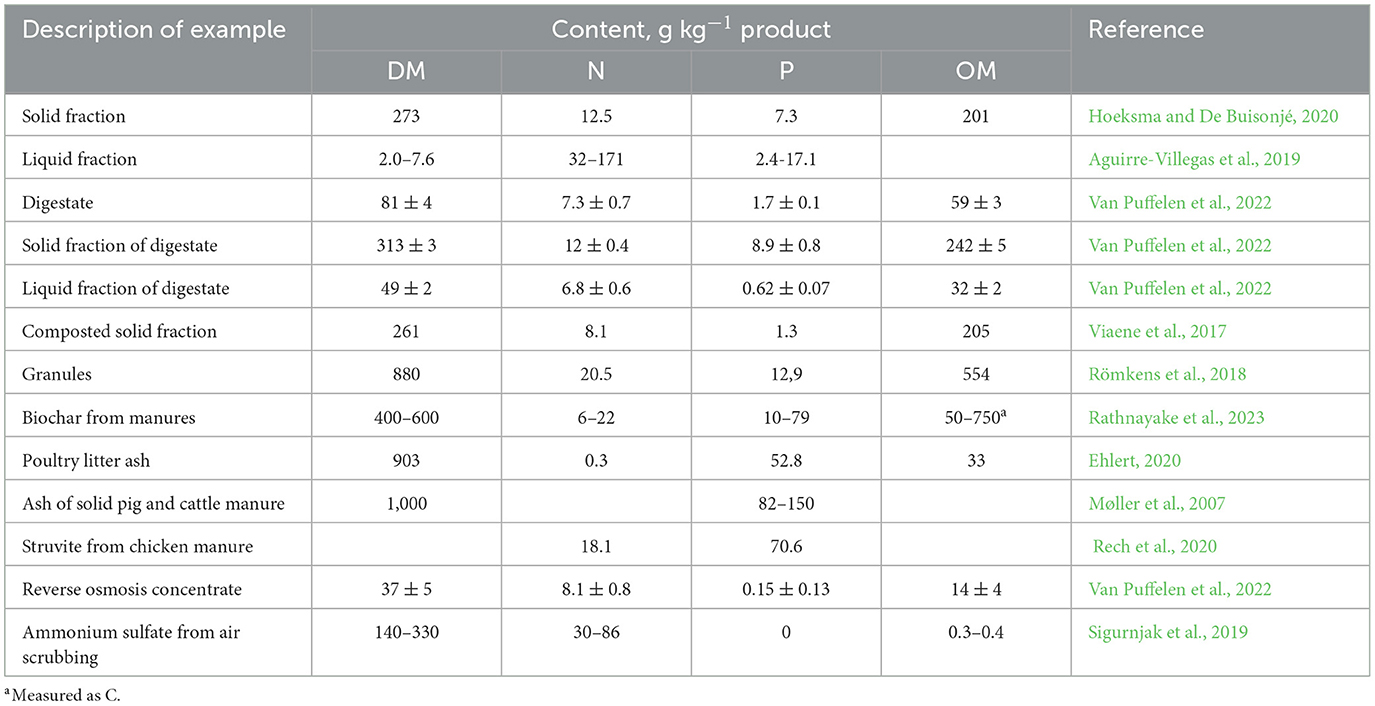
Table 9. Examples of composition of products derived from manure treatment, as indicated in Figure 2 (incl. standard deviation).
Incineration of poultry manure to generate energy produces ashes that can be used as PK fertilizer. Results of pot experiments with a crop show that in the short term the PFRV of poultry ash is about half that of regular mineral phosphate fertilizers, but that over a longer period, the value is similar to the value of regular fertilizers (Ehlert, 2020).
Biochar contains both C and nutrients. Rathnayake et al. (2023) concluded that pyrolysis of manure to create biochar would significantly reduce greenhouse gas (GHG) emissions from soils and create long-term soil C sinks. However, they also indicated that on basis of available studies it is difficult to draw conclusions about the value of biochar as a fertilizer.
6.2.2 Application methods
Solid manures and composts are surface applied and are either left on top of the soil or incorporated. Livestock slurries are also surface applied to a large extent, although this results in high NH3 losses. The risk of NH3 losses are highest in the period shortly after surface-application of livestock manures. Surface application directly followed by plowing is an effective method to reduce emissions (Webb et al., 2014b). The longer the time between application and plowing, the larger the N loss as NH3.
In addition to rapid incorporation after surface application, there are several application techniques available to reduce the risk of NH3 emissions, including methods in which (i) slurries are band-placed on top of the soils, such as trailing hose and trailing show, (ii) slurries are injected a few centimeters into the soil, such as shallow or sod injection, and (iii) slurries are injected deeper below the soil surface (Bittman et al., 2014; Van der Weerden et al., 2021). Generally, the risk of NH3 emission will decrease when slurries are injected (Section 5.1), but risk of N2O emission may increase (Section 5.2). The N losses through NH3 volatilization are larger than those via N2O emission, and often also larger than those through total denitrification to N2, implying that applying manures with low NH3 emission techniques may lead to higher nutrient use efficiency than surface application (Huijsmans et al., 2016).
Diluted slurries or liquid fractions of treated manure can be applied via irrigation, using irrigation or fertigation systems (Misselbrook et al., 2004; Guido et al., 2020). Injection reduces the risk of NH3 emission from application of N concentrates derived from manure treatment. However, these products have a much higher N content than livestock slurries (Table 9), which means that less volume has to be applied to obtain the required nutrient application rate. Development of new precision injection techniques, based on mineral fertilizer application techniques, is needed for adequate application of N concentrates (Van Middelkoop and Holshof, 2017). Besides increasing nutrient use efficiency, precision application of organic resources may also reduce odor nuisance and crop contamination.
Developments in soil and crop sensing data and rapid manure analytical methods (Section 3) in combination with GPS techniques can be used in site-specific precision N fertilization techniques (e.g., Corti et al., 2023). In addition to the differentiation of rates and sources of the application of organic resources among different fields, within-field establishment of zones with different yield potential may help increase the nutrient use efficiency from organic resources (Kharel et al., 2019) if it can be combined with accurate application of the fertilizer.
6.2.3 Inhibitors and additives
Urease inhibitors (UI) delay urea hydrolysis and may therefore have the potential to reduce NH3 emission from manure (Li et al., 2017). Yet, addition of UI shortly after excretion in livestock housing systems is practically challenging, and as most urea in manure is hydrolyzed during storage, adding UI at application is unlikely to result in large reductions in NH3 emission and N losses from soil-applied manures. An exception may be dried poultry manures, because of the relatively large part N present as uric acid (Nicholson et al., 1996). Nitrification inhibitors (NI) inhibit the activity of nitrifying bacteria, thereby reducing the risk of both NO leaching and gaseous N2O emissions. A meta-analysis has shown positive effects of inhibitors on crop yields, and a decrease in N losses of 32.9, 14.5, 37.6%, respectively by UI, NI or combinations of UI and NI, in mineral and organic resources (Sha et al., 2020). Addition of the nitrification inhibitor 3,4-dimethyl pyrazole phosphate (DMPP) to cattle slurry reduced N2O emission with 30–40% (Dittert et al., 2001; Herr et al., 2020). Studies indicate that the inhibitors have no impact on non-target microbial composition or abundance (Duff et al., 2022), but might affect free living N fixation (Liu et al., 2024). Studies on potential adverse effects of inhibitors on the soil microbial community are mostly short-term, and assessments over longer timeframes are still lacking.
It is often suggested that the use of biological or chemical manure additives, such as minerals, microorganisms, charcoal, or plant extracts may reduce NH3 and GHG emissions while research often shown very limited effects (Wheeler et al., 2010), and sometime even increased emissions (Van der Stelt et al., 2007).
A meta-analysis of slurry acidification has shown that acidification can decrease emissions of NH3, NOX, CH4 by 69, 21, 86%, respectively, while various other management strategies such as biological treatment, separation strategies, different storage types can decrease NH3 emissions but increase emissions of a least one other GHG (Emmerling et al., 2020). Trade-offs (Section 7.1.2) may occur as acidification of slurry with sulfuric acid may increase the risk of sulfate leaching to groundwater and acidification of slurry reduces NH3 emissions but may increase risk of N2O emission (Velthof and Oenema, 1993; Loide et al., 2020).
Clearly, there is scope to reduce N losses and increase nutrient use efficiency from organic resources by adding inhibitors and additives. These products are not always effective and may have negative side-effects that have to be considered.
6.3 Structural measures
Structural measures such as the reduction or relocation of livestock and crop production are an option for improving nutrient and C management and to reduce emissions from agriculture. In comparison to technical and management measures, structural measures generally refer to more drastic changes in the agricultural system, e.g., through transitioning toward lower livestock numbers, relocating or shifting livestock types, or changing land uses and cropping systems. Structural measures will need to be taken if large scale implementation of technical and management measures as shown in Sections 6.1 and 6.2 are not sufficient to reach the environmental targets. Besides their relevance for reaching environmental targets, structural measures will impact the nutrient demand (e.g., for fertilizing cropland) and nutrient supply (e.g., from livestock manure), which need to be considered in the future management of organic resources. The examples of studies exploring reduction potentials at the national or European scale in Table 10 show large ranges but also a high reduction potential for structural measures.
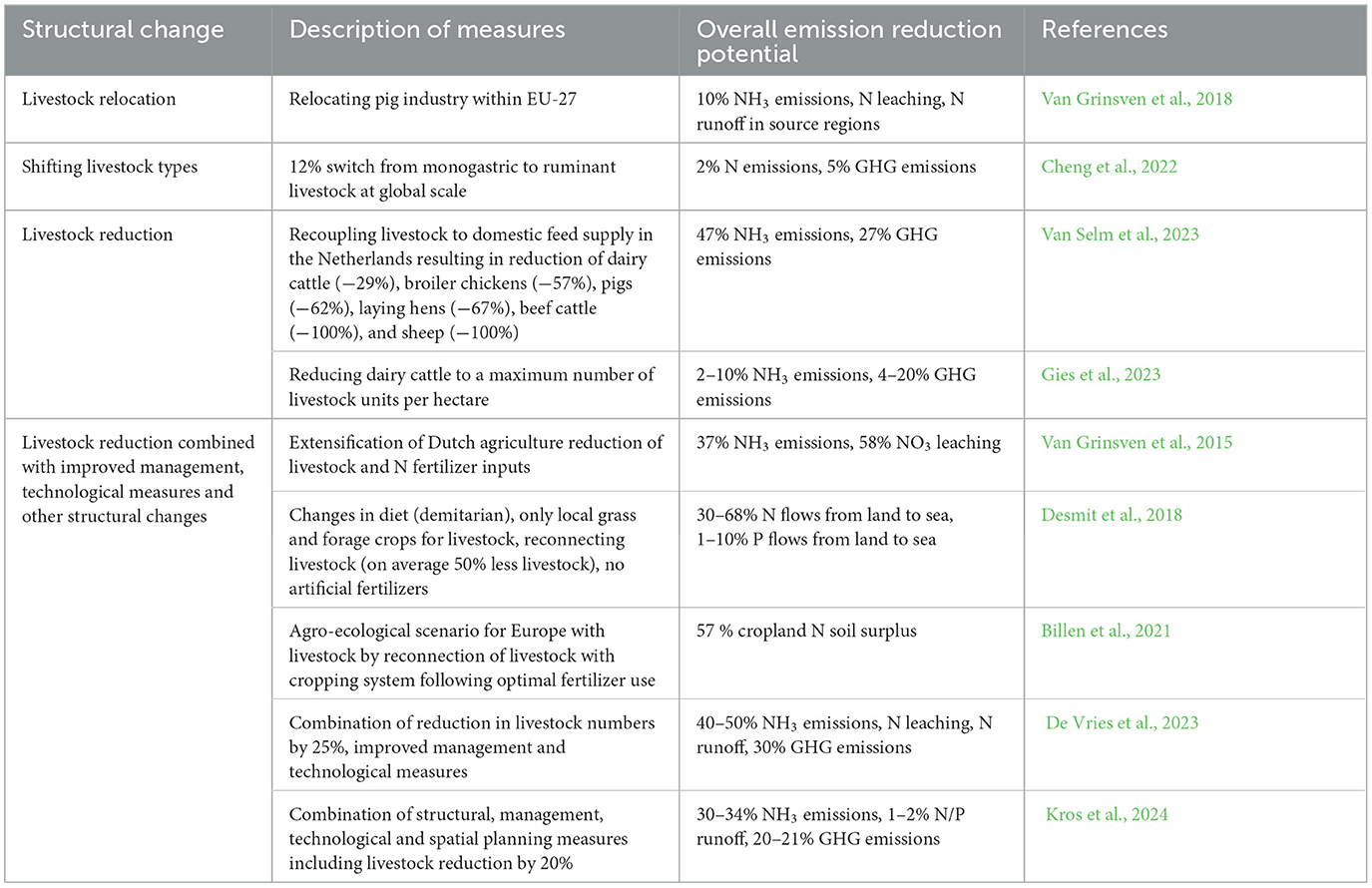
Table 10. Examples of studies that examined emission reduction potentials associated with structural changes in agriculture.
6.3.1 Changing livestock numbers
Various studies have explored the mitigation potentials of structural changes in livestock numbers. Studies on livestock relocation focused on the effects of redistributing livestock to areas where they cause lower environmental and human health impacts. Figure 1 shows large differences in livestock manure application due to variation in livestock density across the EU. In their model study, Van Grinsven et al. (2018) show that relocating the pig industry within the EU-27 can reduce emissions of NH3 and N leaching by ~10% in source regions, but may simultaneously increase emissions in receiving regions by 40 and 20%, respectively, for NH3 and N leaching. Although the authors found that relocation resulted in an overall decrease of N emissions and external N costs at EU level, they also noted that relocation comes with socio-economic barriers and redistribution of pollution.
Another example of structural measures that can have a positive impact on reducing emissions is shifting livestock types. For instance, one study found that shifting from monogastric livestock to ruminants led to a decrease in N and GHGs by 2 and 5% GHG emissions given the lower demand for cropland areas and lower fertilizer inputs in ruminant production systems (Cheng et al., 2022). In contrast, other studies found that shifting away from ruminant livestock production led to reductions of GHG emissions (Aleksandrowicz et al., 2016; Grummon et al., 2023).
Larger mitigation potentials were found through measures that significantly reduce livestock numbers the livestock density in the Netherlands is high, resulting in high manure inputs (Figure 1) and resulting in a manure surplus and exceedance of environmental targets of emissions of atmospheric pollutants and water quality. Van Selm et al. (2023) estimated that reducing livestock numbers to the level that pigs, poultry and cattle can be fed with domestic feed grown in the Netherlands, would require a large reduction in livestock ranging from 29 to 100%, depending on livestock types (Table 10). This reduction would significantly reduce NH3 and GHG emissions in the Netherlands by 47 and 27% respectively (Table 10). Gies et al. (2023) found that a reduction in Dutch dairy cattle of 7–34%, reduced the NH3, and GHG emissions from the agricultural sector in the Netherlands by 2–9.5%, and 4–19%, respectively.
6.3.2 Changing land use and cropping systems
A few studies investigated mitigation potential through land use changes and changes in cropping systems. One option to mitigate GHG emissions is the rewetting of peat soils, e.g., through taking peatland out of agricultural production or using shallower water tables (Boonman et al., 2022). Another structural measure is the spatial relocation of crop production, e.g., from areas where biodiversity and potential C stocks are high in order to reduce environmental impacts and biodiversity loss in more sensitive areas (Beyer et al., 2022). Furthermore, local interventions such as the introduction of buffer zones or the construction of wetlands can help prevent nutrient losses from agricultural soils to surface waters (Van den Broek et al., 2007). In the Netherlands, N application standards in sandy soil regions, prone to leaching, is stricter than in other regions (Van Grinsven et al., 2016), which may cause that some cropping systems will move from one region to another.
6.3.3 Combined interventions
Structural changes and technical improvements are not mutually exclusive and multiple studies explored their combined effects (Table 10). For instance, Van Grinsven et al. (2015) found that an extensification of the Dutch agricultural system through both reducing livestock numbers by 20–50% and reducing N fertilizer inputs by 40% led to significantly lower national ammonia emissions and nitrate leaching (Table 10). De Vries et al. (2023) showed that the combination of technical measures (e.g., low emission housing, application techniques), improved crop, soil and nutrient management, and a reduction in livestock in the Netherlands by 25% reduced N emissions and GHG by 40–50% and 30%, respectively. A more recent study showed that reducing livestock, with additional structural measures such as buffer zones along waterways, and combined with management and technical measures, can reduce emissions by 30–40% and GHG emissions by 20% (Kros et al., 2024). The latter studies highlighted that a combination of technical and management improvements, together with livestock reduction and spatial interventions (e.g., buffer strips) are needed to significantly reduce emissions and reach environmental targets in the Netherlands. The studies also emphasized that improved management alone was not sufficient to mitigate GHG emissions and nutrient losses from livestock production in order to reach long-term climate goals which was also suggested by Weishaupt et al. (2020). Therefore, implementation of structural changes will be crucial in order to reach emission targets and effectively mitigate agricultural pollution.
These examples show that in regions with a high livestock density and manure surplus, the implementation of structural changes next to technological and management measures will be needed in order to effectively mitigate agricultural pollution in Europe.
7 Future challenges to support farmers and policy makers
7.1 Risk of trade-offs between measures
A combination of measures have to be taken to increase nutrient use efficiency, improve soil quality and reduce gaseous emissions and leaching from organic resources so that the targets of the different environmental policies can be met. Combinations of measures may have synergies and strengthen their effects; however there is also a risk of trade-offs or pollution swapping. Different types of pollution swapping between measures can take place (Figure 3). Examples of the different types of pollution swapping risks are:
• Between management strategies, e.g., grazing decreases the risk of NH3 and CH4 emissions, but increases risk on NO3 leaching and N2O emission (Bussink, 1994; Velthof et al., 1996; Corré et al., 2014);
• Between technical measures; e.g., slurry injection reduces NH3 but increases N2O emission (Velthof and Mosquera, 2011; Goedhart et al., 2020) and acidification of slurry reduces NH3 emissions but may increase sulfate leaching or N2O emission (Velthof and Oenema, 1993; Loide et al., 2020);
• Within a farm; e.g., low NH3 emission housing increases the ammoniacal N content in manures and because of that increases the risk of NH3 emission when the manure is applied (Bittman et al., 2014);
• Within a country; e.g., in the sandy regions in the South of the Netherlands strict N application standards are applied for crops with a high nitrate leaching risk (Van Grinsven et al., 2016). This may stimulate the growth of these crops in the sandy regions in the North with higher N application rates and because that may increase leaching in these regions;
• Between countries; e.g., high environmental pressure and strict environmental rules for pig production in one country (e.g., the Netherlands) may increase the number of pig farms in another countries (e.g., Spain).
Clearly, avoiding pollution swapping and finding synergies in the implementation of combinations of measures is challenging. If sufficient information on organic resources is available, potential trade-offs can be taken into account. For example, Velthof and Mosquera (2011) calculated the total effect of application method on N2O emissions, taking into account indirect emissions from NH3 volatilization and the need for extra mineral fertilizers due to loss in NFRV after surface application. Based on their results and IPCC emission factors, they showed that, although injection of cattle slurry on grassland can lead to higher direct N2O emissions than surface spreading, the indirect emissions from NH3 volatilization and the additional mineral fertilizer need result in higher total N2O emissions for surface application (Velthof and Mosquera, 2011). This effect was not the same for pig slurry on arable land due to the high emission factor for injection of pig slurry, which shows that trade-offs are often heavily context dependent. In-depth knowledge on the composition, agronomic value and risks on the emissions of organic resources to the environment is therefore needed to derive effective nutrient management strategies. This especially holds for new waste products from the circular economy and new products from manure treatment (Figure 2), as the agronomic and environmental consequences of the use of many of these products is not yet clear.
7.2 Challenges for farmers
Fertilizer recommendations and decision support tools are important sources of information for farmers to increase nutrient use efficiency and decrease nutrient losses. Recent papers show that there is a large diversity in recommendations and tools between countries in the EU and that harmonization, shared learning, and a collective approach is recommended to tackle environmental problems (Nicholson et al., 2020; Higgins et al., 2023). The EU FAST tool (https://fastplatform.eu/) is developed for European Commission's DG Agriculture and Rural Development as nutrient management tool to support EU farmers, farm advisors, developers of digital solutions, and policy makers. This tool contains different modules, including a fertilizer module. Besides nutrient management tools, tools are also developed to calculate soil C sequestration and GHG emissions from farms (Arulnathan et al., 2020; Alexandropoulos et al., 2023), such as the Cool Farm Tool (Hillier et al., 2011) (https://coolfarm.org/). There is also an increasing interest of the food industry in these type of tools, as many industries have made commitments to decrease their GHG emissions. In the coming decade, farmers might be subject to an increasing level of accountability for the environmental footprint of their farm and stimulated to use aforementioned tools, by both governments and industry partners. This can give rise to new challenges because of the extra administration and record keeping these tools demand or because of management changes required to achieve set targets.
Fertilizer recommendations and decision support tools require information about the composition (Sections 2.1 and 3.1), the plant-availability of nutrients (Section 4.1) and degradability of OM of organic resources (Section 4.2). Ideally, analyses of the composition and nutrient availability of organic resources in combination with (real-time) data on soils, crop and weather are used, but at least standard values for a range of different organic resources have to be available for reliable recommendations (Nicholson et al., 2013). If tools are also used for estimating the risk of gaseous emissions and leaching, information on emission factors of NH3 and GHG for organic resources and coefficients for calculation of leaching are required (Section 5). Emission factors can be derived from default values of the EMEP/EEA air pollutant emission inventory guidebook, and IPPC guidelines, or from country specific emission factors. Farmers should also be aware about the potential risks of contaminants present in the organic resources, especially when they use these fertilizers frequently (Section 5.5). The use of farm specific emission factors and coefficients would improve the accuracy of emission calculations, using for example process-based soils models (Heinen et al., 2020).
The rapid development of analytical techniques for composition of organic resources, soils, crops, weather, and emissions to the environment, will further improve farm specific nutrient management strategies and DSS-tools. However, there are large challenges regarding the interpretation and integration of the huge amount of data that will be derived from these new techniques. Ultimately, they have to lead to scientifically underpinned and clear recommendations to farmers about daily field operations.
Lastly, the implementation of measures, that for example promote manure treatment or change of animal housing systems, is challenged with financial and practical barriers. Building manure processing installations or new stables is expensive and requires permits; all in all, it usually takes several years before such a change is implemented. For the biogas sector the key barriers are the lack of financial incentives and policy frameworks that ensure profitable operation until at least the investment is paid back (Kampman et al., 2017).
7.3 Challenges for policy makers
7.3.1 Monitoring of emissions
EU countries are obliged to report gaseous emissions of atmospheric pollutants and GHGs to the European Commission and United Nations. Emissions of NH3, NOX, fine particles and non-methane volatile organic compounds (NMVOC) have to be reported to the EU (NEC; EU, 2016) and United Nations [UNECE; Convention on long-range transboundary air pollution/Gothenborg protocol (UN, 2013)]. The emission of GHGs have to be reported as part of the Paris Climate Agreement to the United Nations Framework Convention on Climate Change (UNFCCC; UN, 2015). Moreover, EU countries have to write a progress report about implementation of the Nitrates Directive every 4 years, which includes data on water quality and nutrient use, and implementation of nutrient management measures.
The calculation methods for monitoring of atmospheric pollutants are presented in the EMEP/EEA air pollutant emission inventory guidebook (EEA, 2023). The calculation methods of GHG emissions are presented in the IPCC guidelines (IPCC, 2019). Both guidelines include different methodologies for calculation of emissions: Tier 1 (default method and emission factors), Tier 2 (default method and country specific or technology-specific emission factors), and Tier 3 (country specific calculation method). The EMEP/EEA guidebook differentiates Tier 1 emission factors of NH3 and NOX for application of manures, sewage sludge and other organic N resources (including composts and digestates). The Tier 1 and Tier 2 emission factors for NH3 are differentiated for livestock and manure types (in total 20 categories). The IPPC guidelines include one default aggregated N2O emission factor for all N sources and disaggregated emission factors for all organic N fertilizers. For accurate emission estimates and effective mitigation, country-specific, management-specific, and organic resource-specific emission factors for production, storage and application are needed. Studies aiming to derive emission factors for soil application have to follow protocols or general rules accepted by scientists, e.g., the VERA protocol for NH3 and the Global Research Alliance N2O chamber methodology guidelines (Vera, 2009; De Klein et al., 2020). There are large challenges to obtain emission factors for organic resources. These challenges will further increase because of the increasing number of organic resource types from manure treatment and new waste streams, with different product-specific emissions.
Combining data sources of different countries in large databases will improve estimates of emission factors (Beltran et al., 2021; Van der Weerden et al., 2021). Moreover, such databases create perspectives for the derivation of emission factors that take more controlling factors into account than the current simple emission factors based on N input and can be used to improve emission estimates from organic resources with models, such as the FAN, ALFAM-2, and DNDC (Li et al., 2012; Hafner et al., 2019; Vira et al., 2020).
7.3.2 New organic resources
It is expected that (new) organic resources from manure treatment, food processing, sewage sludge, and municipal bio-wastes will become available for use in agriculture as fertilizer or soil conditioner. Similar approaches to those described in the previous section to obtain emission factors for gaseous emissions are also needed for agronomic value of fertilizers, e.g., the NFRV (Section 4.1) and HC (Section 4.2). Policies often use these indicators for measures, such as the N application standards in the Nitrates Directive or calculations for carbon farming. These indicators were also used for setting up criteria for the use of treated manure above the “170 kg N per ha” threshold for manure application in the Nitrates Directive (Huygens et al., 2020)
Besides the agronomic value and risk of emissions of new organic resources, it is also very important to get insight into the possible presence of contaminants in these fertilizers to avoid soil pollution. The EU FPR provides rules for the use of new fertilizers.
8 Conclusions
There are huge challenges in maximizing the use of organic resources and meanwhile meeting all the targets on emissions of atmospheric pollutants, GHGs, water quality, and contaminants. In EU27+UK, nutrient (N, P) inputs in soil through organic resources roughly equal those of mineral fertilizers, but there are large regional differences in the EU in use of these fertilizers (Figure 1). The availability of organic resources from food processing, sewage sludge, and municipal bio-wastes is expected to strongly increase over the coming decade (Section 6.2.1). Also manure treatment will further develop, resulting in organic resources with different agronomic value and environmental impact (Section 6.2.1).
The rapid development of analytical techniques can be applied to enhance the knowledge of the use of organic resources and the presence of contaminants (Sections 3 and 5.5). There are, however, many challenges in the accurate sampling of the fertilizers and the handling/interpretation data generated. There is a need to link easily obtainable parameters to agronomic performance (FRVs and HCs) and environmental risk of losses. Insight in the chemical properties to explain and predict variability in the coefficients as well as standardization of determination methods is required for adequate fertilizer recommendations of new and existing products (Sections 4.1 and 4.2).
Both effective management and technological measures are available and often tested (Sections 6.1 and 6.2). Structural measures will have a large social and economic impact on farmers and regional communities, but can solve environmental issues at local and regional scales (Section 6.3). Synergies and trade-offs between measures may occur at different scale and between different types of emissions (Figure 3).
There are many EU policies dealing with the use of organic resources, but with a different focus, i.e., on atmospheric pollutants, GHGs, water quality, and contaminants. The implementation of these fragmented policies on national to local scale is often only partly effective. An integrated nutrient management approach addressing all the objectives of the single policies is needed. Such an approach (Integrated Nutrient Management Action Plan; INMAP) is already proposed by the European Commission, as part of the Farm-to-Fork strategy.
The main future challenge for organic resources in agriculture is an integrated nutrient management approach, including (i) the characterization of organic resources, their agronomic value and their environmental risks, (ii) knowledge of potential synergies and the risk of trade-offs between nutrient management measures, and (iii) implementation of this knowledge into (emission) models, inventories, and legislation to support farmers and policy makers.
Author contributions
GV: Conceptualization, Writing—original draft, Writing—review & editing. TC: Writing—original draft. JH: Writing—original draft. JL: Software, Writing—review & editing. ML: Writing—original draft. RP: Writing—original draft. MR: Writing—original draft. RR: Writing—original draft. OS: Writing—original draft. LV: Visualization, Writing—original draft. DW: Writing—original draft.
Funding
The author(s) declare that no financial support was received for the research, authorship, and/or publication of this article.
Conflict of interest
The authors declare that the research was conducted in the absence of any commercial or financial relationships that could be construed as a potential conflict of interest.
Publisher's note
All claims expressed in this article are solely those of the authors and do not necessarily represent those of their affiliated organizations, or those of the publisher, the editors and the reviewers. Any product that may be evaluated in this article, or claim that may be made by its manufacturer, is not guaranteed or endorsed by the publisher.
Footnotes
1. ^https://phosphorusplatform.eu/scope-in-print/news/1061-switzerland-makes-phosphorus-recycling-obligatory
2. ^https://standards.cencenelec.eu/dyn/www/f?p=205:105:0:::::
References
Adamse, P., Van der Fels-Klerx, H., and De Jong, J. (2017). Cadmium, lead, mercury and arsenic in animal feed and feed materials–trend analysis of monitoring results. Food Addit. Contam. Part A 34, 1298–1311. doi: 10.1080/19440049.2017.1300686
Aguirre-Villegas, H. A., Larson, R. A., and Sharara, M. A. (2019). Anaerobic digestion, solid-liquid separation, and drying of dairy manure: measuring constituents and modeling emission. Sci. Total Environ. 696:134059. doi: 10.1016/j.scitotenv.2019.134059
AHDB (2023). Nutrient Management Guide (RB209) - Section 2 Organic Materials. Conventry: Agriculture and Horticulture Development Board, Conventry.
Aleksandrowicz, L., Green, R., Joy, E. J. M., Smith, P., and Haines, A. (2016). The impacts of dietary change on greenhouse gas emissions, land use, water use, and health: a systematic review. PLoS ONE 11:e0165797. doi: 10.1371/journal.pone.0165797
Alexandropoulos, E., Anestis, V., Dragoni, F., Hansen, A., Cummins, S., O'Brien, D., et al. (2023). Decision support systems based on gaseous emissions and their impact on the sustainability assessment at the livestock farm level: an evaluation from the user's side. Sustainability 15:13041. doi: 10.3390/su151713041
Amlinger, F., Pollak, M., and Favoino, E. (2004). Heavy Metals and Organic Compounds From Wastes usEd as Organic Fertilisers. Brussels: European Commission DG Environment.
Arulnathan, V., Heidari, M. D., Doyon, M., Li, E., and Pelletier, N. (2020). Farm-level decision support tools: a review of methodological choices and their consistency with principles of sustainability assessment. J. Clean. Prod. 256:120410. doi: 10.1016/j.jclepro.2020.120410
Aubain, P., Gazzo, A., Moux, J. L., Brunet, H., and Landrea, B. (2002). Disposal and Recycling Routes for Sewage Sludge-Synthesis Report 22 February 2002. Brussels: European Commission DG Environment.
Bajagain, R., Gautam, P., Le, T. T. N., Dahal, R. H., Kim, J., and Jeong, S. W. (2022). Isolation and screening of odor-reducing microbes from swine manure and its role in reducing ammonia release in combination with surfactant foam. Appl. Sci. 12:1806. doi: 10.3390/app12041806
Behera, S. N., Sharma, M., Aneja, V. P., and Balasubramanian, R. (2013). Ammonia in the atmosphere: a review on emission sources, atmospheric chemistry and deposition on terrestrial bodies. Environ. Sci. Pollut. Res. 20, 8092–8131. doi: 10.1007/s11356-013-2051-9
Beltran, I., Van der Weerden, T., Alfaro, M., Amon, B., De Klein, C., Grace, P., et al. (2021). DATAMAN: a global database of nitrous oxide and ammonia emission factors for excreta deposited by livestock and land-applied manure. J. Environ. Qual. 50, 513–527. doi: 10.1002/jeq2.20186
Berset, J. D., and Holzer, R. (1995). Organic micropollutants in swiss agriculture: distribution of polynuclear aromatic hydrocarbons (PAH) and polychlorinated biphenyls (PCB) in soil, liquid manure, sewage sludge and compost samples; a comparative study. Int. J. Environ. Anal. Chem. 59, 145–165. doi: 10.1080/03067319508041324
Beyer, R. M., Hua, F., Martin, P. A., Manica, A., and Rademacher, T. (2022). Relocating croplands could drastically reduce the environmental impacts of global food production. Commun. Earth Environ. 3:49. doi: 10.1038/s43247-022-00360-6
Bhogal, A., Williams, J. R., Nicholson, F. A., Chadwick, D. R., Chambers, K. H., and Chambers, B. J. (2016). Mineralization of organic nitrogen from farm manure applications. Soil Use Manag. 32, 32–43. doi: 10.1111/sum.12263
Billen, G., Aguilera, E., Einarsson, R., Garnier, J., Gingrich, S., Grizzetti, B., et al. (2021). Reshaping the European agro-food system and closing its nitrogen cycle: the potential of combining dietary change, agroecology, and circularity. One Earth 4, 839–850. doi: 10.1016/j.oneear.2021.05.008
Binner, E., Smidt, E., Tintner, J., Böhm, K., and Lechner, P. (2011). How to enhance humification during composting of separately collected biowaste: impact of feedstock and processing. Waste Manag. Res. 29, 1153–1163. doi: 10.1177/0734242X11413954
Bittman, S., Dedina, H. C., Oenema, O., and Sutton, M. (2014). Options for Ammonia Mitigation: Guidance from the UNECE Task Force on Reactive Nitrogen, Centre for Ecology and Hydrology. Edinburgh.
Blake, L., Johnston, A., Poulton, P., and Goulding, K. (2003). Changes in soil phosphorus fractions following positive and negative phosphorus balances for long periods. Plant Soil 254, 245–261. doi: 10.1023/A:1025544817872
Bläsing, M., and Amelung, W. (2018). Plastics in soil: analytical methods and possible sources. Sci. Total Environ. 612, 422–435. doi: 10.1016/j.scitotenv.2017.08.086
Blicher-Mathiesen, G., Andersen, H. E., and Larsen, S. E. (2014). Nitrogen field balances and suction cup-measured N leaching in Danish catchments. Agric. Ecosyst. Environ. 196, 69–75. doi: 10.1016/j.agee.2014.06.022
Boonman, J., Hefting, M. M., Van Huissteden, C. J. A., Van Den Berg, M., Van Huissteden, J., Erkens, G., et al. (2022). Cutting peatland CO2 emissions with water management practices. Biogeosciences 19, 5707–5727. doi: 10.5194/bg-19-5707-2022
Borchard, N., Schirrmann, M., Cayuela, M. L., Kammann, C., Wrage-Mönnig, N., Estavillo, J. M., et al. (2019). Biochar, soil and land-use interactions that reduce nitrate leaching and N2O emissions: a meta-analysis. Sci. Total Environ. 651, 2354–2364. doi: 10.1016/j.scitotenv.2018.10.060
Bougouin, A., Leytem, A., Dijkstra, J., Dungan, R. S., and Kebreab, E. (2016). Nutritional and environmental effects on ammonia emissions from dairy cattle housing: a meta-analysis. J. Environ. Qual. 45, 1123–1132. doi: 10.2134/jeq2015.07.0389
Brändli, R. C., Bucheli, T. D., Kupper, T., Furrer, R., Stahel, W. A., Stadelmann, F. X., et al. (2007a). Organic pollutants in compost and digestate.: Part 1.: polychlorinated biphenyls, polycyclic aromatic hydrocarbons and molecular markers. J. Environ. Monit. 9, 456–464. doi: 10.1039/B617101J
Brändli, R. C., Kupper, T., Bucheli, T. D., Zennegg, M., Huber, S., Ortelli, D., et al. (2007b). Organic pollutants in compost and digestate.: Part 2.: Polychlorinated dibenzo-dioxins, and -furans, dioxin-like polychlorinated biphenyls, brominated flame retardants, perfluorinated alkyl substances, pesticides, and other compounds. J. Environ. Monit. 9, 465–472. doi: 10.1039/B617103F
Brienza, C., Sigurnjak, I., Michels, E., and Meers, E. (2020). “Ammonia stripping and scrubbing for mineral nitrogen recovery,” in Biorefinery of Inorganics, eds E. Meers, G. Velthof, E. Michels, and R. Rietra (Hoboken, NJ: Wiley), 95–106.
Bruinenberg, M., Van Agtmaal, M., Hoekstra, N., and Van Eekeren, N. (2023). Residues of pesticides in dairy cow rations and fly treatments reduce the number of Coleoptera in dung. Agric. Ecosyst. Environ. 344:108307. doi: 10.1016/j.agee.2022.108307
Bühler, M., Häni, C., Ammann, C., Mohn, J., Neftel, A., Schrade, S., et al. (2021). Assessment of the inverse dispersion method for the determination of methane emissions from a dairy housing. Agric. For. Meteorol. 307:108501. doi: 10.1016/j.agrformet.2021.108501
Buijs, J., Ragas, A., and Mantingh, M. (2022). Presence of pesticides and biocides at Dutch cattle farms participating in bird protection programs and potential impacts on entomofauna. Sci. Total Environ. 838:156378. doi: 10.1016/j.scitotenv.2022.156378
Burkart, A., Hecht, V., and Kraska, T. (2018). Phenological analysis of unmanned aerial vehicle based time series of barley imagery with high temporal resolution. Precis. Agric. 19, 134–147. doi: 10.1007/s11119-017-9504-y
Bussink, D. W. (1994). Relationships between ammonia volatilization and nitrogen-fertilizer application rate, intake and excretion of herbage nitrogen by cattle on grazed swards. Fertil. Res. 38, 111–121. doi: 10.1007/BF00748771
Canziani, R., Boniardi, G., and Turolla, A. (2023). “Phosphorus recovery—recent developments and case studies,” in Sustainable and Circular Management of Resources and Waste Towards a Green Deal Deal, eds. M. N. Vara Prasad and M. Smol (Elsevier), 269–281.
Cassel, T., Ashbaugh, L., Meyer, D., and Flocchini, R. (2005). Ammonia flux from open-lot dairies: development of measurement methodology and emission factors. Air Waste Manag. Assoc. 55, 816–825. doi: 10.1080/10473289.2005.10464659
CBGV (2023). Bemestingsadvies Commissie Bemesting Grasland en Voedergewassen: Versie 2023, Commissie Bemesting Grasland en Voedergewassen. Wageningen.
Centraal Veevoeder Bureau (2012). Tabellenboek Veevoeding, voedernormen landbouwhuisdieren en voederwaarde veevoeders. The Hague: Productschap Diervoeder.
Chadwick, D., Sommer, S., Thorman, R., Fangueiro, D., Cardenas, L., Amon, B., et al. (2011). Manure management: Implications for greenhouse gas emissions. Anim. Feed Sci. Technol. 166–167, 514–531. doi: 10.1016/j.anifeedsci.2011.04.036
Chadwick, D. R. (2005). Emissions of ammonia, nitrous oxide and methane from cattle manure heaps: effect of compaction and covering. Atmos. Environ. 39, 787–799. doi: 10.1016/j.atmosenv.2004.10.012
Chadwick, D. R., Pain, B. F., and Brookman, S. K. E. (2000). Nitrous oxide and methane emissions following application of animal manures to grassland. J. Environ. Qual. 29, 277–287. doi: 10.2134/jeq2000.00472425002900010035x
Cheng, L., Zhang, X., Reis, S., Ren, C., Xu, J., and Gu, B. (2022). A 12% switch from monogastric to ruminant livestock production can reduce emissions and boost crop production for 525 million people. Nat. Food 3, 1040–1051. doi: 10.1038/s43016-022-00661-1
Choi, W.-J., Ro, H.-M., and Chang, S. X. (2004). Recovery of fertilizer-derived inorganic-15 N in a vegetable field soil as affected by application of an organic amendment. Plant Soil 263, 191–201. doi: 10.1023/B:PLSO.0000047726.09394.d3
Chojnacka, K., Gorazda, K., Witek-Krowiak, A., and Moustakas, K. (2019). Recovery of fertilizer nutrients from materials - contradictions, mistakes and future trends. Renew. Sustain. Energy Rev. 110, 485–498. doi: 10.1016/j.rser.2019.04.063
Clough, T. J., Rochette, P., Thomas, S. M., Pihlatie, M., Christiansen, J. R., and Thorman, R. E. (2020). Global Research Alliance N2O chamber methodology guidelines: design considerations. J. Environ. Qual. 49, 1081–1091. doi: 10.1002/jeq2.20117
Corrado, S., and Sala, S. (2018). Food waste accounting along global and European food supply chains: state of the art and outlook. Waste Manag. 79, 120–131. doi: 10.1016/j.wasman.2018.07.032
Corré, W. J., Van Beek, C. L., and Van Groenigen, J. W. (2014). Nitrate leaching and apparent recovery of urine-N in grassland on sandy soils in the Netherlands. Wageningen J. Life Sci. 70–71, 25–32. doi: 10.1016/j.njas.2014.02.001
Corti, M., Cavalli, D., Pricca, N., Ferrè, C., Comolli, R., Marino Gallina, P., et al. (2023). Site-specific recommendations of cattle manure nitrogen and urea for silage maize. Nutrient Cycling Agroecosyst. 127, 155–169. doi: 10.1007/s10705-023-10302-z
Dach, J., and Starmans, D. (2005). Heavy metals balance in Polish and Dutch agronomy: actual state and previsions for the future. Agric. Ecosyst. Environ. 107, 309–316. doi: 10.1016/j.agee.2005.02.017
Dammers, E., Palm, M., Van Damme, M., Vigouroux, C., Smale, D., Conway, S., et al. (2016). An evaluation of IASI-NH3 with ground-based Fourier transform infrared spectroscopy measurements. Atmos. Chem. Phys. 16, 10351–10368. doi: 10.5194/acp-16-10351-2016
De Klein, C. A. M., Harvey, M. J., Clough, T. J., Petersen, S. O., Chadwick, D. R., and Venterea, R. T. (2020). Global Research Alliance N2O chamber methodology guidelines: introduction, with health and safety considerations. J. Environ. Qual. 49, 1073–1080. doi: 10.1002/jeq2.20131
De Neve, S., Sleutel, S., and Hofman, G. (2003). Carbon mineralization from composts and food industry wastes added to soil. Nutr. Cycl. Agroecosyst. 67, 13–20. doi: 10.1023/A:1025113425069
De Notaris, C., Sørensen, P., Møller, H. B., Wahid, R., and Eriksen, J. (2018). Nitrogen fertilizer replacement value of digestates from three green manures. Nutr. Cycl. Agroecosyst. 112, 355–368. doi: 10.1007/s10705-018-9951-5
De Ridder, M., De Jong, S., Polchar, J., and Lingemann, S. (2012). Risks and Opportunities in the Global Phosphate Rock market: Robust Strategies in Times of Uncertainty. The Hague: The Hague Centre for Strategic Studies (HCSS).
De Vries, W., Kros, J., Voogd, J. C., and Ros, G. H. (2023). Integrated assessment of agricultural practices on large scale losses of ammonia, greenhouse gases, nutrients and heavy metals to air and water. Sci. Total Environ. 857:159220. doi: 10.1016/j.scitotenv.2022.159220
De Wit, D., and Vervuurt, W. (2023). Effect wijzigingen HC-waarde, C/N-orgratio en OS-gehalte van verschillende veelgebruikte mestsoorten op de koolstofopbouw en N-mineralisatie op bouwplanniveau: deskstudie in het kader van de PPS Beter BodemBeheer. Wageningen: Wageningen Plant Research.
Delin, S. (2016). Fertilizer value of phosphorus in different residues. Soil Use Manag. 32, 17–26. doi: 10.1111/sum.12227
Delin, S., Stenberg, B., Nyberg, A., and Brohede, L. (2012). Potential methods for estimating nitrogen fertilizer value of organic residues. Soil Use Manag. 28, 283–291. doi: 10.1111/j.1475-2743.2012.00417.x
Dell, C. J., Kleinman, P. J. A., Schmidt, J. P., and Beegle, D. B. (2012). Low-disturbance manure incorporation effects on ammonia and nitrate loss. J. Environ. Qual. 41, 928–937. doi: 10.2134/jeq2011.0327
Derikx, P., Van de Kooi, B., Heskamp, H., and Rozijn, M. (2021). NIRS and Manure Composition (NIMACO), Wageningen Food Safety Research, Report 2021.012. Wageningen: Wageningen Food Safety Research.
Deru, J. G. C., Bloem, J., De Goede, R., Brussaard, L., and Van Eekeren, N. (2023). Effects of organic and inorganic fertilizers on soil properties related to the regeneration of ecosystem services in peat grasslands. Appl. Soil Ecol. 187:104838. doi: 10.1016/j.apsoil.2023.104838
Desmit, X., Thieu, V., Billen, G., Campuzano, F., Duli?re, V., Garnier, J., et al. (2018). Reducing marine eutrophication may require a paradigmatic change. Sci. Total Environ. 635, 1444–1466. doi: 10.1016/j.scitotenv.2018.04.181
Dittert, K., Bol, R., King, R., Chadwick, D., and Hatch, D. (2001). Use of a novel nitrification inhibitor to reduce nitrous oxide emission from 15N-labelled dairy slurry injected into soil. Rapid Commun. Mass Spectrom. 15, 1291–1296. doi: 10.1002/rcm.335
Dittrich, B., and Klose, R. (2008). Schwermetalle in Düngemitteln-Bestimmung und Bewertung von Schwermetallen in Düngemitteln, Bodenhilfsstoffen und Kultursubstraten, Sächsische Landesanstalt für Landwirtschaft. Leipzig.
Dlamini, L., Crespo, O., Van Dam, J., and Kooistra, L. (2023). A global systematic review of improving crop model estimations by assimilating remote sensing data: implications for small-scale agricultural systems. Remote Sens. 15:4066. doi: 10.3390/rs15164066
Dobbelaere, D. (2023). Statistical Overview of the Animal by-Products Industry in the EU in 2022. Naples: EFPRA.
Duan, Y. F., Bruun, S., Jensen, L. S., van Gerven, L. P. A., Hendriks, C. M. J., Veenemans, L., et al. (2021). Mapping and Characterization of CNP Flows and Their Stoichiometry in Main Farming Systems in Europe. Ghent: Nutri2Cycle, Ghent University.
Duff, A. M., Forrestal, P., Ikoyi, I., and Brennan, F. (2022). Assessing the long-term impact of urease and nitrification inhibitor use on microbial community composition, diversity and function in grassland soil. Soil Biol. Biochem. 170:108709. doi: 10.1016/j.soilbio.2022.108709
Durkin, A., Guo, M., Wuertz, S., and Stuckey, D. C. (2022). Resource recovery from food-processing wastewaters in a circular economy: a methodology for the future. Curr. Opin. Biotechnol. 76:102735. doi: 10.1016/j.copbio.2022.102735
ECN (2022). ECN Data Report 2022- Compost and Digestate for a Circular Economy. Bochum: European Compost Network (ECN) e.V.
EEA (2023). EMEP/EEA Air Pollutant Emission Inventory Guidebook 2023. Technical Guidance to Prepare National Emission Inventories. Copenhagen: European Environment Agency.
EEC (1991). Council Directive 91/676/EEC of 12 December 1991 concerning the protection of waters against pollution caused by nitrates from agricultural sources. Offi. J. Eur. Commun. 34, 1–8.
Egene, C. E., Sigurnjak, I., Regelink, I. C., Schoumans, O. F., Adani, F., Michels, E., et al. (2021). Solid fraction of separated digestate as soil improver: implications for soil fertility and carbon sequestration. J. Soils Sedim. 21, 678–688. doi: 10.1007/s11368-020-02792-z
Ehlert, P. (2020). “Agronomic effectivity of hydrated poultry litter ash,” in Biorefinery of Inorganics, eds E. Meers, G. Velthof, E. Michels, and R. Rietra (Hoboken, NJ: Wiley), 147–160.
Ehlert, P. A. I., and Schoumans, O. F. (2015). Products, By-Products an Recovered Secondary Materials From Processed Animal Manure. Alterra, Report 2668. Wageningen: Alterra.
Eichler-Löbermann, B., Köhne, S., and Köppen, D. (2007). Effect of organic, inorganic, and combined organic and inorganic P fertilization on plant P uptake and soil P pools. J. Plant Nutr. Soil Sci. 170, 623–628. doi: 10.1002/jpln.200620645
Emmerling, C., Krein, A., and Junk, J. (2020). Meta-analysis of strategies to reduce NH3 emissions from slurries in European agriculture and consequences for greenhouse gas emissions. Agronomy 10:1633. doi: 10.3390/agronomy10111633
ESPP (European Sustainable Phosphorous Platform) (2015). Switzerland Makes Phosphorus Recycling Obligatory. Available online at: https://www.phosphorusplatform.eu/scope-in-print/news/1061-switzerland-makes-phosphorus-recycling-obligatory?highlight=WyJzd2l0emVybGFuZCIsInNsYXVnaHRlcmhvdXNlIl0= (accessed Dec 5, 2023).
EU (2009). Regulation (EC) No 1069/2009 of the European Parliament and of the Council of 21 October 2009 laying down health rules as regards animal by-products and derived products not intended for human consumption and repealing Regulation (EC) No 1774/2002 (Animal by-products Regulation). Official Journal of the European Union, L300.
EU (2016). Directive (EU) 2016/2284 of the European Parliament and the council of 14 December 2016 on the reduction of national emissions of certain atmospheric pollutants, amending Directive 2003/35/EC and repealing Directive 2001/81/EC. Official Journal of the European Union, L 322/1.
EU (2018). Directive (EU) 2018/851 of the European parliament and the council of 30 May 2018 amending Directive 2008/98/EC on waste. Official Journal of the European Union, L 150/109.
EU (2019). Regulation (EU) 2019/1009 of the European Parliament and of the Council of 5 June 2019 laying down rules on the making available on the market of EU fertilising products and amending Regulations (EC) No 1069/2009 and (EC) No 1107/2009 and repealing Regulation (EC) No 2003/2003. Official Journal of the European Union, L 170, 1-114.
European Commission (2020a). A Farm to Fork Strategy for a Fair, Healthy and Environmentally-Friendly Food System. COM/2020/381/Final, Brussels.
European Commission (2020b). A New Circular Economy Action Plan for Cleaner and More Competitive Europe. Brussel: European Commission.
European Commission (2022). Support to the Evaluation of the Sewage Sludge Directive – Exploratory Study – Final Report. Publications Office of the European Union.
Evangelista, C., Basiricò, L., and Bernabucci, U. (2021). An overview on the use of near infrared spectroscopy (NIRS) on farms for the management of dairy cows. Agriculture 11:296. doi: 10.3390/agriculture11040296
Feng, X., Larson, R. A., and Digman, M. F. (2022). Evaluating the feasibility of a low-field nuclear magnetic resonance (NMR) sensor for manure nutrient prediction. Sensors 22:2438. doi: 10.3390/s22072438
Flis, S. (2017). The 4Rs in crop nitrogen research. Crops Soils 50, 18–20. doi: 10.2134/cs2017.50.0209
Flis, S. (2018). 4R history and recent phosphorus research. Crops Soils 51, 36–47. doi: 10.2134/cs2018.51.0207
Foged, H. L., Xavier, F., and Bonmati Blasi, A. (2011). Future Trends on Manure Processing Activities in Europe. Technical Report No. V concerning “Manure Processing Activities in Europe” to the European Commission. Brussels: EC Directorate-General Environment.
Frick, H., Oberson, A., Frossard, E., and Bünemann, E. K. (2022). Leached nitrate under fertilised loamy soil originates mainly from mineralisation of soil organic N. Agric. Ecosyst. Environ. 338:108093. doi: 10.1016/j.agee.2022.108093
Fu, Y., De Jonge, L. W., Moldrup, P., Paradelo, M., and Arthur, E. (2022). Improvements in soil physical properties after long-term manure addition depend on soil and crop type. Geoderma 425:116062. doi: 10.1016/j.geoderma.2022.116062
Ge, X., Schaap, M., and De Vries, W. (2023). Improving spatial and temporal variation of ammonia emissions for the Netherlands using livestock housing information and a Sentinel-2-derived crop map. Atmos. Environ. X 17:100207. doi: 10.1016/j.aeaoa.2023.100207
Gies, E., Cals, T., Kros, H., Kuindersma, W., and Voogd, J.-C. (2023). Aanvullende generieke stikstof-en klimaatbeleidsmaatregelen: een verkenning naar optionele generieke maatregelen om stikstof-en broeikasgasemissies te reduceren, aanvullend op de huidige en voorgenomen beleidsmaatregelen op rijksniveau. Wageningen: Wageningen Environmental Research, Report No. 3261.
Goedhart, P. W., Mosquera, J., and Huijsmans, J. F. M. (2020). Estimating ammonia emission after field application of manure by the integrated horizontal flux method: a comparison of concentration and wind speed profiles. Soil Use Manag. 36, 338–350. doi: 10.1111/sum.12564
Goulding, K., Poulton, P., Webster, C., and Howe, M. (2000). Nitrate leaching from the Broadbalk Wheat Experiment, Rothamsted, UK, as influenced by fertilizer and manure inputs and the weather. Soil Use Manag. 16, 244–250. doi: 10.1111/j.1475-2743.2000.tb00203.x
Grace, P. R., Van Der Weerden, T. J., Rowlings, D. W., Scheer, C., Brunk, C., Kiese, R., et al. (2020). Global Research Alliance N2O chamber methodology guidelines: considerations for automated flux measurement. J. Environ. Qual. 49, 1126–1140. doi: 10.1002/jeq2.20124
Graves, D. B., Bakken, L. B., Jensen, M. B., and Ingels, R. (2019). Plasma activated organic fertilizer. Plasma Chem. Plasma Process. 39, 1–19. doi: 10.1007/s11090-018-9944-9
Grell, T., Marchuk, S., Williams, I., McCabe, B. K., and Tait, S. (2023). Resource recovery for environmental management of dilute livestock manure using a solid-liquid separation approach. J. Environ. Manage. 325:116254. doi: 10.1016/j.jenvman.2022.116254
Grummon, A. H., Lee, C. J. Y., Robinson, T. N., Rimm, E. B., and Rose, D. (2023). Simple dietary substitutions can reduce carbon footprints and improve dietary quality across diverse segments of the US population. Nat. Food 4, 966–977. doi: 10.1038/s43016-023-00864-0
Guido, V., Finzi, A., Ferrari, O., Riva, E., Quílez, D., Herrero, E., et al. (2020). Fertigation of maize with digestate using drip irrigation and pivot systems. Agronomy 10:1453. doi: 10.3390/agronomy10101453
Guilayn, F., Jimenez, J., Rouez, M., Crest, M., and Patureau, D. (2019). Digestate mechanical separation: efficiency profiles based on anaerobic digestion feedstock and equipment choice. Bioresour. Technol. 274, 180–189. doi: 10.1016/j.biortech.2018.11.090
Gutser, R., Ebertseder, T., Weber, A., Schraml, M., and Schmidhalter, U. (2005). Short-term and residual availability of nitrogen after long-term application of organic fertilizers on arable land. J. Plant Nutr. Soil Sci. 168, 439–446. doi: 10.1002/jpln.200520510
Gworek, B., Kijeńska, M., Wrzosek, J., and Graniewska, M. (2021). Pharmaceuticals in the soil and plant environment: a review. Water Air Soil Pollut. 232, 1–17. doi: 10.1007/s11270-020-04954-8
Hafner, S. D., Pacholski, A., Bittman, S., Burchill, W., Bussink, W., Chantigny, M., et al. (2018). The ALFAM2 database on ammonia emission from field-applied manure: description and illustrative analysis. Agric. For. Meteorol. 258, 66–79. doi: 10.1016/j.agrformet.2017.11.027
Hafner, S. D., Pacholski, A., Bittman, S., Carozzi, M., Chantigny, M., Génermont, S., et al. (2019). A flexible semi-empirical model for estimating ammonia volatilization from field-applied slurry. Atmos. Environ. 199, 474–484. doi: 10.1016/j.atmosenv.2018.11.034
Hansen, L. V., Braendholt, A., Tariq, A., Jensen, L. S., Peixoto, L. E. K., Petersen, S. O., et al. (2024). Methane uptake rates across different soil types and agricultural management practices in Denmark. Agric. Ecosyst. Environ. 363:108878. doi: 10.1016/j.agee.2023.108878
He, S., Cao, C., Wang, J., Yang, J., Cheng, Z., Yan, B., et al. (2020). Pyrolysis study on cattle manure: from conventional analytical method to online study of pyrolysis photoionization time-of-flight mass spectrometry. J. Anal. Appl. Pyrolysis 151:104916. doi: 10.1016/j.jaap.2020.104916
Heinen, M., Assinck, F. B. T., Groenendijk, P., and Schoumans, O. F. (2020). “Soil dynamic models: predicting the behavior of fertilizers in the soil,” in Biorefinery of Inorganics, eds E. Meers, G. Velthof, E. Michels, and R. Rietra (Hoboken, NJ: Wiley).
Hensen, A., Skiba, U., and Famulari, D. (2013). Low cost and state of the art methods to measure nitrous oxide emissions. Environ. Res. Lett. 8:025022. doi: 10.1088/1748-9326/8/2/025022
Herr, C., Mannheim, T., Müller, T., and Ruser, R. (2020). Effect of nitrification inhibitors on N2O emissions after cattle slurry application. Agronomy 10:1174. doi: 10.3390/agronomy10081174
Higgins, S., Keesstra, S., Kadziuliene, Z., Jordan-Meille, L., Wall, D., Trinchera, A., et al. (2023). Stocktake study of current fertilisation recommendations across Europe and discussion towards a more harmonised approach. Eur. J. Soil Sci. 74:13422. doi: 10.1111/ejss.13422
Hijbeek, R., Loon, M. P. V., and Ittersum, M. K. V. (2019). “Fertiliser use and soil carbon sequestration: trade-offs and opportunities,” in CCAFS Working Paper. (Wageningen).
Hijbeek, R., Ten Berge, H. F. M., Whitmore, A. P., Barkusky, D., Schröder, J. J., and Van Ittersum, M. K. (2018). Nitrogen fertiliser replacement values for organic amendments appear to increase with N application rates. Nutr. Cycl. Agroecosyst. 110, 105–115. doi: 10.1007/s10705-017-9875-5
Hillier, J., Walter, C., Malin, D., Garcia-Suarez, T., Mila-i-Canals, L., and Smith, P. (2011). A farm-focused calculator for emissions from crop and livestock production. Environ. Model. Softw. 26, 1070–1078. doi: 10.1016/j.envsoft.2011.03.014
Hoeksma, P., and De Buisonjé, F. (2020). “Mineral concentrates from membrane filtration,” in Biorefinery of Inorganics, eds E. Meers, G. Velthof, E. Michels, and R. Rietra (Hoboken, NJ: Wiley), 121–131.
Horf, M., Gebbers, R., Olfs, H.-W., and Vogel, S. (2024). Determining nutrients, dry matter, and pH of liquid organic manures using visual and near-infrared spectrometry. Sci. Total Environ. 908:168045. doi: 10.1016/j.scitotenv.2023.168045
Horf, M., Vogel, S., Drücker, H., Gebbers, R., and Olfs, H.-W. (2022). Optical spectrometry to determine nutrient concentrations and other physicochemical parameters in liquid organic manures: a review. Agronomy 12:514. doi: 10.3390/agronomy12020514
Hou, Y., Velthof, G. L., and Oenema, O. (2015). Mitigation of ammonia, nitrous oxide and methane emissions from manure management chains: a meta-analysis and integrated assessment. Glob. Chang. Biol. 21, 1293–1312. doi: 10.1111/gcb.12767
Hu, M., Wade, A. J., Shen, W., Zhong, Z., Qiu, C., and Lin, X. (2023). Effects of organic fertilizers produced by different production processes on nitrous oxide and methane emissions from double-cropped rice fields. Pedosphere 34, 52–62. doi: 10.1016/j.pedsph.2023.03.006
Huijsmans, J. F. M., Schröder, J. J., Mosquera, J., Vermeulen, G. D., Ten Berge, H. F. M., and Neeteson, J. J. (2016). Ammonia emissions from cattle slurries applied to grassland: should application techniques be reconsidered? Soil Use Manag. 32, 109–116. doi: 10.1111/sum.12201
Hušek, M., Homma, R., Moško, J., Pohorelý, M., and Oshita, K. (2023). P-recovery versus current sewage sludge treatment policy in the Czech Republic and Japan. Clean Technol. Environ. Policy. doi: 10.1007/s10098-023-02679-w
Huygens, D. (2023). Technical Proposals for Processed Manure as a Component Material for EU Fertilising Products. Brussel: JRC (Joint Research Centre, European Commission).
Huygens, D., Garcia-Gutierrez, P., Orveillon, G., Schillaci, C., Delre, A., Orgiazzi, A., et al. (2022). Screening Risk Assessment of Organic Pollutants and Environmental Impacts From Sewage Sludge Management. Seville: Joint Research Centre.
Huygens, D., Orveillon, G., Lugato, E., Tavazzi, S., Comero, S., Jones, A., et al. (2020). Technical Proposals for the Safe Use of Processed Manure Above the Threshold Established for Nitrate Vulnerable Zones by the Nitrates Directive (91/676/EEC). Luxembourg: Publications Office of the European Union, JRC12163.
Huygens, D., Saveyn, H., Tonini, D., Eder, P., and Delgado Sancho, L. (2019). Technical Proposals for Selected New Fertilising Materials Under the Fertilising Products Regulation (Regulation (EU) 2019/1009). Process and Quality Criteria, and Assessment of Environmental and Market Impacts for Precipitated Phosphate Salts and Derivates, Thermal Oxidation Materials and Derivates and Pyrolysis and Gasification Materials. Luxembourg: Publications Office of the European Union, JRC117856.
IPCC (2019). 2019 Refinement to the 2006 IPCC Guidelines for National Greenhouse Gas Inventories. Switzerland: IPCC.
Janssen, B. H. (1984). A simple method for calculating decomposition and accumulation of 'young' soil organic matter. Plant Soil 76, 297–304. doi: 10.1007/BF02205588
Jensen, L., Oelofse, M., Ten Hoeve, M., and Bruun, S. (2020). “Environmental impact assessment on the production and use of biobased fertilizers,” in Biorefinery of Inorganics, eds E. Meers, G. Velthof, E. Michels, and R. Rietra (Hoboken, NJ: Wiley), 329–362.
Jensen, L. S. (2013). “Animal manure fertiliser value, crop utilisation and soil quality impacts,” in Animal Manure Recycling: Treatment and Management, eds S. G. Sommer, M. L. Christensen, T. Schmidt, and L. S. Jensen (Chichester: Wiley).
Johnston, A. M., and Bruulsema, T. W. (2014). 4R nutrient stewardship for improved nutrient use efficiency. Proc. Eng. 83, 365–370. doi: 10.1016/j.proeng.2014.09.029
Jomaa, S., Aboud, I., Dupas, R., Yang, X., Rozemeijer, J., and Rode, M. (2018). Improving nitrate load estimates in an agricultural catchment using event response reconstruction. Environ. Monit. Assess. 190, 1–14. doi: 10.1007/s10661-018-6700-9
Jordan-Meille, L., Higgins, S., Dittert, K., and Cugnon, T. (2022). “Comparison of nitrogen fertiliser recommendations in different West European countries,” in Proceedings 868, IFS 2022. Cambridge: International Fertiliser Society, 33.
Kacprzak, M., Kupich, I., Jasinska, A., and Fijalkowski, K. (2022). Bio-based waste' substrates for degraded soil improvement - Advantages and challenges in European context. Energies 15:385. doi: 10.3390/en15010385
Kampman, B., Leguijt, C., Scholten, T., Tallat-Kelpsaite, J., Br?ckmann, R., Maroulis, G., et al. (2017). Optimal Use of Biogas From Waste Streams: An Assessment of the Potential of Biogas From Digestion in the EU Beyond 2020. Brussel: European Commission.
Kätterer, T., Bolinder, M. A., Andrén, O., Kirchmann, H., and Menichetti, L. (2011). Roots contribute more to refractory soil organic matter than above-ground crop residues, as revealed by a long-term field experiment. Agric. Ecosyst. Environ. 141, 184–192. doi: 10.1016/j.agee.2011.02.029
Kharel, T. P., Maresma, A., Czymmek, K. J., Oware, E. K., and Ketterings, Q. M. (2019). Combining spatial and temporal corn silage yield variability for management zone development. Agron. J. 111, 2703–2711. doi: 10.2134/agronj2019.02.0079
Kim, D.-G., Kirschbaum, M. U. F., Eichler-Löbermann, B., Gifford, R. M., and Liáng, L. L. (2023). The effect of land-use change on soil C, N, P, and their stoichiometries: a global synthesis. Agric. Ecosyst. Environ. 348:108402. doi: 10.1016/j.agee.2023.108402
Kok, D. J. D., Scherer, L., De Vries, W., and Van Bodegom, P. M. (2023). Temporal variability in organic amendment impacts on hydro-physical properties of sandy agricultural soils. Soil Sci. Soc. Am. J. 87, 963–984. doi: 10.1002/saj2.20547
Kros, H., Cals, T., Gies, E., Groenendijk, P., Lesschen, J. P., Voogd, J. C., et al. (2024). Region oriented and integrated approach to reduce emissions of nutrients and greenhouse gases from agriculture in the Netherlands. Sci. Total Environ. 909:168501. doi: 10.1016/j.scitotenv.2023.168501
Kupper, T., Bürge, D., Bachmann, H. J., Güsewell, S., and Mayer, J. (2014). Heavy metals in source-separated compost and digestates. Waste Manag. 34, 867–874. doi: 10.1016/j.wasman.2014.02.007
Kupper, T., Häni, C., Neftel, A., Kincaid, C., Bühler, M., Amon, B., et al. (2020). Ammonia and greenhouse gas emissions from slurry storage - a review. Agric. Ecosyst. Environ. 300:106963. doi: 10.1016/j.agee.2020.106963
Kurniawati, A., Stankovics, P., Hilmi, Y. S., Toth, G., Smol, M., and Toth, Z. (2023). Understanding the future of bio-based fertilisers: The EU's policy and implementation. Sustain. Chem. Clim. Act. 3:100033. doi: 10.1016/j.scca.2023.100033
Larsen, T. A., Gruendl, H., and Binz, C. (2021). The potential contribution of urine source separation to the SDG agenda – a review of the progress so far and future development options. Environ. Sci. 7, 1161–1176. doi: 10.1039/D0EW01064B
Lashermes, G., Nicolardot, B., Parnaudeau, V., Thuriès, L., Chaussod, R., Guillotin, M. L., et al. (2009). Indicator of potential residual carbon in soils after exogenous organic matter application. Eur. J. Soil Sci. 60, 297–310. doi: 10.1111/j.1365-2389.2008.01110.x
Leclerc, A., and Laurent, A. (2017). Framework for estimating toxic releases from the application of manure on agricultural soil: national release inventories for heavy metals in 2000–2014. Sci. Total Environ. 590, 452–460. doi: 10.1016/j.scitotenv.2017.01.117
Lee, S. R., Han, J. K., Choi, Y. J., and Nam, K. (2007). Reduction of ammonia and hydrogen sulfide emission from swine manure using aqueous foams amended with microorganisms and chemical additives. Clean Soil Air Water 35, 230–234. doi: 10.1002/clen.200700017
Lehmann, J., Lan, Z., Hyland, C., Sato, S., Solomon, D., and Ketterings, Q. M. (2005). Long-term dynamics of phosphorus forms and retention in manure-amended soils. Environ. Sci. Technol. 39, 6672–6680. doi: 10.1021/es047997g
Lemmens, B., Ceulemans, J., Elslander, H., Vanassche, S., Brauns, E., and Vrancken, K. (2007). Beste Beschikbare Technieken (BBT) voor mestverwerking. Gent.
Lemming, C., Oberson, A., Magid, J., Bruun, S., Scheutz, C., Frossard, E., et al. (2019). Residual phosphorus availability after long-term soil application of organic waste. Agric. Ecosyst. Environ. 270, 65–75. doi: 10.1016/j.agee.2018.10.009
Levavasseur, F., Lashermes, G., Mary, B., Morvan, T., Nicolardot, B., Parnaudeau, V., et al. (2022). Quantifying and simulating carbon and nitrogen mineralization from diverse exogenous organic matters. Soil Use Manag. 38, 411–425. doi: 10.1111/sum.12745
Li, C., Salas, W., Zhang, R., Krauter, C., Rotz, A., and Mitloehner, F. (2012). Manure-DNDC: a biogeochemical process model for quantifying greenhouse gas and ammonia emissions from livestock manure systems. Nutr. Cycl. Agroecosyst. 93, 163–200. doi: 10.1007/s10705-012-9507-z
Li, Q. Q., Cui, X. Q., Liu, X. J., Roelcke, M., Pasda, G., Zerulla, W., et al. (2017). A new urease-inhibiting formulation decreases ammonia volatilization and improves maize nitrogen utilization in North China Plain. Sci. Rep. 7:43853. doi: 10.1038/srep43853
Liu, J., Han, C., Zhao, Y., Yang, J., Cade-Menun, B. J., Hu, Y., et al. (2020). The chemical nature of soil phosphorus in response to long-term fertilization practices: implications for sustainable phosphorus management. J. Clean. Prod. 272:123093. doi: 10.1016/j.jclepro.2020.123093
Liu, Y., Xu, Z., Bai, S. H., Fan, H., Zuo, J., Zhang, L., et al. (2024). Non-targeted effects of nitrification inhibitors on soil free-living nitrogen fixation modified with weed management. Sci. Total Environ. 912:169005. doi: 10.1016/j.scitotenv.2023.169005
Lockyer, D. R. (1984). A system for the measurement in the field of losses of ammonia through volatilisation. J. Sci. Food Agric. 35, 837–848. doi: 10.1002/jsfa.2740350805
Loide, V., Saue, T., Võsa, T., and Tamm, K. (2020). The effect of acidified slurry on crop uptake and leaching of nutrients from a loamy topsoil. Acta Agric. Scand. Sect. B Soil Plant Sci. 70, 31–38. doi: 10.1080/09064710.2019.1665705
Luo, H., Dewitte, K., Landschoot, S., Sigurnjak, I., Robles-Aguilar, A. A., Michels, E., et al. (2022). Benefits of biobased fertilizers as substitutes for synthetic nitrogen fertilizers: Field assessment combining minirhizotron and UAV-based spectrum sensing technologies. Front. Environ. Sci. 10:988932. doi: 10.3389/fenvs.2022.988932
Maresma, A., Ariza, M., Martínez, E., Lloveras, J., and Martínez-Casasnovas, J. A. (2016). Analysis of vegetation indices to determine nitrogen application and yield prediction in maize (L.) from a standard UAV service. Remote Sens. 8:973. doi: 10.3390/rs8120973
Messiga, A. J., Ziadi, N., Jouany, C., Virkajärvi, P., Suomela, R., Sinaj, S., et al. (2015). Soil test phosphorus and cumulative phosphorus budgets in fertilized grassland. Ambio 44, 252–262. doi: 10.1007/s13280-015-0628-x
Misselbrook, T. H., Smith, K. A., Jackson, D. R., and Gilhespy, S. L. (2004). ammonia emissions from irrigation of dilute pig slurries. Biosyst. Eng. 89, 473–484. doi: 10.1016/j.biosystemseng.2004.08.015
Møller, H., Jensen, H. S., Tobiasen, L., and Hansen, M. (2007). Heavy metal and phosphorus content of fractions from manure treatment and incineration. Environ. Technol. 28, 1403–1418. doi: 10.1080/09593332808618900
Möller, K., and Schultheiss, U. (2015). Chemical characterization of commercial organic fertilizers. Arch. Agron. Soil Sci. 61, 989–1012. doi: 10.1080/03650340.2014.978763
Mondini, C., Cayuela, M. L., Sinicco, T., Fornasier, F., Galvez, A., and Sánchez-Monedero, M. A. (2017). Modification of the RothC model to simulate soil C mineralization of exogenous organic matter. Biogeosciences 14, 3253–3274. doi: 10.5194/bg-14-3253-2017
Morvan, T., Gogé, F., Oboyet, T., Carel, O., and Fouad, Y. (2021). A dataset of the chemical composition and near-infrared spectroscopy measurements of raw cattle, poultry and pig manure. Data Brief 39:107475. doi: 10.1016/j.dib.2021.107475
Munoz, G., Michaud, A. M., Liu, M., Vo Duy, S., Montenach, D., Resseguier, C., et al. (2021). Target and nontarget screening of PFAS in biosolids, composts, and other organic waste products for land application in France. Environ. Sci. Technol. 56, 6056–6068. doi: 10.1021/acs.est.1c03697
Nest, T. V., Ruysschaert, G., Vandecasteele, B., Houot, S., Baken, S., Smolders, E., et al. (2016). The long term use of farmyard manure and compost: effects on P availability, orthophosphate sorption strength and P leaching. Agric. Ecosyst. Environ. 216, 23–33. doi: 10.1016/j.agee.2015.09.009
Ng, E.-L., Lwanga, E. H., Eldridge, S. M., Johnston, P., Hu, H.-W., Geissen, V., et al. (2018). An overview of microplastic and nanoplastic pollution in agroecosystems. Sci. Total Environ. 627, 1377–1388. doi: 10.1016/j.scitotenv.2018.01.341
Nicholson, F., Krogshave Laursen, R., Cassidy, R., Farrow, L., Tendler, L., Williams, J., et al. (2020). How can decision support tools help reduce nitrate and pesticide pollution from agriculture? A literature review and practical insights from the EU FAIRWAY Project. Water 12:768. doi: 10.3390/w12030768
Nicholson, F. A., Bhogal, A., Chadwick, D., Gill, E., Gooday, R. D., Lord, E., et al. (2013). An enhanced software tool to support better use of manure nutrients MANNER-NPKl. Soil Use Manag. 29, 473–484. doi: 10.1111/sum.12078
Nicholson, F. A., Chambers, B. J., and Smith, K. A. (1996). Nutrient composition of poultry manures in England and Wales. Bioresour. Technol. 58, 279–284. doi: 10.1016/S0960-8524(97)86087-7
Nikièma, P., Akinremi, O. O., and Tenuta, M. (2016). Nitrous oxide emissions as affected by liquid and solid pig manures applied to annual and perennial forage crops on a sandy loam soil. Can. J. Soil Sci. 96, 361–371. doi: 10.1139/cjss-2015-0052
Oenema, O., Bleeker, A., Braathen, N. A., Budnákov,á, M., Bull, K., Cermák, P., et al. (2011). “Nitrogen in current European policies,” in The European Nitrogen Assessment: Sources, Effects and Policy Perspectives, eds A. Bleeker, B. Grizzetti, C. M. Howard, G. Billen, H. van Grinsven, J. W. Erisman, et al. (Cambridge: Cambridge University Press), 62–81.
Osmond, D., Bolster, C., Sharpley, A., Cabrera, M., Feagley, S., Forsberg, A., et al. (2017). Southern phosphorus indices, water quality data, and modeling (APEX, APLE, and TBET) results: a comparison. J. Environ. Qual. 46, 1296–1305. doi: 10.2134/jeq2016.05.0200
Park, J., Lee, S. R., Han, I. K., and Nam, K. (2006). Mitigation of ammonia and hydrogen sulfide emissions by stable aqueous foam-microbial media. Environ. Sci. Technol. 40, 3030–3035. doi: 10.1021/es051810j
Pedersen, J., and Nyord, T. (2023). Effect of low-dose acidification of slurry digestate on ammonia emissions after field application. Atmos. Environ. X 17:100205. doi: 10.1016/j.aeaoa.2023.100205
Pedra, F., Polo, A., Ribeiro, A., and Domingues, H. (2007). Effects of municipal solid waste compost and sewage sludge on mineralization of soil organic matter. Soil Biol. Biochem. 39, 1375–1382. doi: 10.1016/j.soilbio.2006.12.014
Petersen, S. O., Peixoto, L. E. K., Sorensen, H., Tariq, A., Braendholt, A., Hansen, L. V., et al. (2023). Higher N2O emissions from organic compared to synthetic N fertilisers on sandy soils in a cool temperate climate. Agric. Ecosyst. Environ. 358:108718. doi: 10.1016/j.agee.2023.108718
Powell, J. M., Jokela, W. E., and Misselbrook, T. H. (2011). Dairy slurry application method impacts ammonia emission and nitrate leaching in no-till corn silage. J. Environ. Qual. 40, 383–392. doi: 10.2134/jeq2010.0082
Powlson, D., Johnston, A., and Jenkinson, D. (1986). The nitrogen cycle in the Broadbalk Wheat Experiment: recovery and losses of 15N-labelled fertilizer applied in spring and inputs of nitrogen from the atmosphere. J. Agric. Sci. 107, 591–609. doi: 10.1017/S0021859600069768
Qin, S., Hu, C., Clough, T. J., Luo, J., Oenema, O., and Zhou, S. (2017). Irrigation of DOC-rich liquid promotes potential denitrification rate and decreases N2O/(N2O+ N2) product ratio in a 0–2 m soil profile. Soil Biol. Biochem. 106, 1–8. doi: 10.1016/j.soilbio.2016.12.001
Räbiger, T., Andres, M., Hegewald, H., Kesenheimer, K., Köbke, S., Quinones, T. S., et al. (2020). Indirect nitrous oxide emissions from oilseed rape cropping systems by NH3 volatilization and nitrate leaching as affected by nitrogen source, N rate and site conditions. Eur. J. Agron. 116:126039. doi: 10.1016/j.eja.2020.126039
Rapson, T. D., and Dacres, H. (2014). Analytical techniques for measuring nitrous oxide. Trends Anal. Chem. 54, 65–74. doi: 10.1016/j.trac.2013.11.004
Rathnayake, D., Schmidt, H.-P., Leifeld, J., Mayer, J., Epper, C., Bucheli, T., et al. (2023). Biochar from animal manure: a critical assessment on technical feasibility, economic viability, and ecological impact. GCB Bioenergy 15, 1078–1104. doi: 10.1111/gcbb.13082
Rech, I., Kamogawa, M. Y., Jones, D. L., and Pavinato, P. S. (2020). Synthesis and characterization of struvite derived from poultry manure as a mineral fertilizer. J. Environ. Manage. 272:111072. doi: 10.1016/j.jenvman.2020.111072
Regelink, I. C., Egene, C. E., Tack, F. M., and Meers, E. (2021). Speciation of P in solid organic fertilisers from digestate and biowaste. Agronomy 11:2233. doi: 10.3390/agronomy11112233
Reijneveld, J. A., van Oostrum, M. J., Brolsma, K. M., Fletcher, D., and Oenema, O. (2022). Empower innovations in routine soil testing. Agronomy 12:191. doi: 10.3390/agronomy12010191
Ren, F., Sun, N., Misselbrook, T., Wu, L., Xu, M., Zhang, F., et al. (2022). Responses of crop productivity and reactive nitrogen losses to the application of animal manure to China's main crops: a meta-analysis. Sci. Total Environ. 850:158064. doi: 10.1016/j.scitotenv.2022.158064
Rietra, R., Berendsen, B., Mi-Gegotek, Y., Römkens, P., and Pustjens, A. (2023). Prediction of the mobility and persistence of eight antibiotics based on soil characteristics. Heliyon 10:e23718. doi: 10.2139/ssrn.4455170
Rode, M., Wade, A. J., Cohen, M. J., Hensley, R. T., Bowes, M. J., Kirchner, J. W., et al. (2016). Sensors in the stream: the high-frequency wave of the present. Environ. Sci. Technol. 50, 10297–10307. doi: 10.1021/acs.est.6b02155
Römkens, P., Rietra, R., Kros, H., Voogd, J. C., and De Vries, W. (2018). Impact of Cadmium Levels in Fertilisers on Cadmium Accumulation in Soil and Uptake by Food Crops. Wageningen Environmental Research Report 2889. Wageningen: Wageningen Environmental Research Report 2889.
Ros, M. B. H., Czymmek, K. J., and Ketterings, Q. M. (2020). Combining field phosphorus runoff risk assessments with whole-farm phosphorus balances to guide manure management decisions. J. Environ. Qual. 49, 496–508. doi: 10.1002/jeq2.20043
Sager, M. (2007). Trace and nutrient elements in manure, dung and compost samples in Austria. Soil Biol. Biochem. 39, 1383–1390. doi: 10.1016/j.soilbio.2006.12.015
Saju, A., Van De Sande, T., Ryan, D., Karpinska, A., Sigurnjak, I., Dowling, D., et al. (2023). Exploring the short-term in-field performance of recovered nitrogen from manure (RENURE) materials to substitute synthetic nitrogen fertilisers. Clean. Circ. Bioecon. 5:100043. doi: 10.1016/j.clcb.2023.100043
Saveyn, H., and Eder, P. (2014). End-of-Waste Criteria for Biodegradable Waste Subjected to Biological Treatment (Compost and Digestate): Technical Proposals. Luxembourg: Publications Office of the European Union.
Schils, R., Schröder, J., and Velthof, G. (2020). “Fertilizer replacement value,” in Biorefinery of Inorganics, eds E. Meers, G. Velthof, E. Michels, and R. Rietra (Hoboken, NJ: Wiley), 189–214.
Schoumans, O. F., Veenemans, L., Vervuurt, W., Verhoeven, J. T. W., De Koeijer, T. J., Manshanden, M., et al. (2023). Conceptual Framework to Evaluate Organic Fertilisers on C and N minerAlisation and Economic Aspects, Wageningen Environmental Research, Report 3309. Wageningen: Wageningen Environmental Research, Report 3309.
Schröder, J. J., Uenk, D., and Hilhorst, G. J. (2007). Long-term nitrogen fertilizer replacement value of cattle manures applied to cut grassland. Plant Soil 299, 83–99. doi: 10.1007/s11104-007-9365-7
Serrano-Gomez, J., Metson, G. S., Neset, T.-S., Santner, J., Hermann, L., and Zessner, M. (2023). EU-compliant wastewater recycled phosphorus: How much national cereal demand can it meet? J. Clean. Prod. 429:139482. doi: 10.1016/j.jclepro.2023.139482
Sha, Z., Ma, X., Wang, J., Lv, T., Li, Q., Misselbrook, T., et al. (2020). Effect of N stabilizers on fertilizer-N fate in the soil-crop system: a meta-analysis. Agric. Ecosyst. Environ. 290:106763. doi: 10.1016/j.agee.2019.106763
Shepherd, M., and Newell-Price, J. (2016). The effects of pig manure type and application timing and frequency on nitrate leaching from a seven-course arable rotation on a retentive alluvial soil. Soil Use Manag. 32, 117–126. doi: 10.1111/sum.12223
Sigurnjak, I., Brienza, C., Snauwaert, E., De Dobbelaere, A., De Mey, J., Vaneeckhaute, C., et al. (2019). Production and performance of bio-based mineral fertilizers from agricultural waste using ammonia (stripping-)scrubbing technology. Waste Manag. 89, 265–274. doi: 10.1016/j.wasman.2019.03.043
Silva, A. A., Fangueiro, D., and Carvalho, M. (2022). Slurry acidification as a solution to minimize ammonia emissions from the combined application of animal manure and synthetic fertilizer in no-tillage. Agronomy 12:265. doi: 10.3390/agronomy12020265
Sintermann, J., Neftel, A., Ammann, C., Häni, C., Hensen, A., Loubet, B., et al. (2012). Are ammonia emissions from field-applied slurry substantially over-estimated in European emission inventories? Biogeosciences 9, 1611–1632. doi: 10.5194/bg-9-1611-2012
Smith, E., Gordon, R., Bourque, C., and Campbell, A. (2007). Comparison of three simple field methods for ammonia volatilization from manure. Can. J. Soil Sci. 87, 469–477. doi: 10.4141/CJSS06038
Smith, S. R. (2009). A critical review of the bioavailability and impacts of heavy metals in municipal solid waste composts compared to sewage sludge. Environ. Int. 35, 142–156. doi: 10.1016/j.envint.2008.06.009
Sørensen, M. K., Jensen, O., Bakharev, O. N., Nyord, T., and Nielsen, N. C. (2015). NPK NMR sensor: online monitoring of nitrogen, phosphorus, and potassium in animal slurry. Anal. Chem. 87, 6446–6450. doi: 10.1021/acs.analchem.5b01924
Sorensen, P., Thomsen, I. K., and Schröder, J. J. (2017). Empirical model for mineralisation of manure nitrogen in soil. Soil Res. 55, 500–505. doi: 10.1071/SR17018
Stadler, C., Von Tucher, S., Schmidhalter, U., Gutser, R., and Heuwinkel, H. (2006). Nitrogen release from plant-derived and industrially processed organic fertilizers used in organic horticulture. J. Plant Nutr. Soil Sci. 169, 549–556. doi: 10.1002/jpln.200520579
Statistics Netherlands (CBS) (2024). Data of Samples of Manure Transports in the Netherlands in the Period 2020-2022. Den Haag.
Stenmarck, Â., Jensen, C., Quested, T., Moates, G., Buksti, M., Cseh, B., et al. (2016). Estimates of European Food Waste Levels. Stockholm: IVL Swedish Environmental Research Institute.
Stevens, J., Green, N. J., and Jones, K. C. (2001). Survey of PCDD/Fs and non-ortho PCBs in UK sewage sludges. Chemosphere 44, 1455–1462. doi: 10.1016/S0045-6535(00)00474-4
Stevens, J. L., and Jones, K. C. (2003). Quantification of PCDD/F concentrations in animal manure and comparison of the effects of the application of cattle manure and sewage sludge to agricultural land on human exposure to PCDD/Fs. Chemosphere 50, 1183–1191. doi: 10.1016/S0045-6535(02)00704-X
Stevens, J. L., Northcott, G. L., Stern, G. A., Tomy, G. T., and Jones, K. C. (2003). PAHs, PCBs, PCNs, organochlorine pesticides, synthetic musks, and polychlorinated n-alkanes in U.K. Sewage sludge: survey results and implications. Environ. Sci. Technol. 37, 462–467. doi: 10.1021/es020161y
Tan, B., Fan, J., He, Y., Luo, S., and Peng, X. (2014). Possible effect of soil organic carbon on its own turnover: a negative feedback. Soil Biol. Biochem. 69, 313–319. doi: 10.1016/j.soilbio.2013.11.017
Thiessen, E., Eslamifar, M., Kock, R., Lausen, P., and Hartung, E. (2022). On farm validation of different NIR sensors for manure sensing. VDI Ber. 2406, 9–16. doi: 10.51202/9783181024065-9
Thompson, R. B., and Meisinger, J. J. (2002). Management factors affecting ammonia volatilization from land-applied cattle slurry in the mid-Atlantic USA. J. Environ. Qual. 31, 1329–1338. doi: 10.2134/jeq2002.1329
Thorman, R. E., Chadwick, D. R., Harrisona, R., Boyles, L. O., and Matthews, R. (2007). The effect on N2O emissions of storage conditions and rapid incorporation of pig and cattle farmyard manure into tillage land. Biosyst. Eng. 97, 501–511. doi: 10.1016/j.biosystemseng.2007.03.039
Thorman, R. E., Nicholson, F. A., Topp, C. F. E., Bell, M. J., Cardenas, L. M., Chadwick, D. R., et al. (2020). Towards country-specific nitrous oxide emission factors for manures applied to arable and grassland soils in the UK. Front. Sustain. Food Syst. 4:62. doi: 10.3389/fsufs.2020.00062
Tian, H. Q., Xu, R. T., Canadell, J. G., Thompson, R. L., Winiwarter, W., Suntharalingam, P., et al. (2020). A comprehensive quantification of global nitrous oxide sources and sinks. Nature 586, 248–+. doi: 10.1038/s41586-020-2780-0
UN (2013). 1999 Protocol to Abate Acidification, Eutrophication and Ground-level Ozone to the Convention on Longrange Transboundary Air Pollution, as Amended on 4 May 2012. Geneva: Economic and Social Concil.
UN (2015). Report of the Conference of the Parties on its twenty-first session, held in Paris From 30 November to 13 December 2015. Decision 1/CP.21 Adoption of the Paris Agreement.
USGS (U.S. Geological Survey) (2022). Mineral Commodity Summaries. Phosphate Rock. Available online at: https://pubs.usgs.gov/periodicals/mcs2022/mcs2022-phosphate.pdf (accessed May 5, 2024).
Vallejo, A., García-Torres, L., Díez, J. A., Arce, A., and López-Fernández, S. (2005). Comparison of N losses (NO3, N2O, NO) from surface applied, injected or amended (DCD) pig slurry of an irrigated soil in a Mediterranean climate. Plant Soil 272, 313–325. doi: 10.1007/s11104-004-5754-3
Van Andel, M., Warland, J., Zwart, P. D., Van Heyst, B., and Lauzon, J. (2017). Development of a simple and affordable method of measuring ammonia volatilization from land applied manures. Can. J. Soil Sci. 97, 541–551. doi: 10.1139/CJSS-2016-0103
Van Damme, M., Clarisse, L., Franco, B., Sutton, M. A., Erisman, J. W., Wichink Kruit, R., et al. (2021). Global, regional and national trends of atmospheric ammonia derived from a decadal (2008–2018) satellite record. Environ. Res. Lett. 16:055017. doi: 10.1088/1748-9326/abd5e0
Van den Broek, J. A., Van Hofwegen, G., Beekman, W., and Woittiez, M. (2007). Options for Increasing Nutrient Use Efficiency in Dutch Dairy and Arable Farming Towards 2030. Wageningen: Statutory Research Tasks Unit for Nature and the Environment.
Van der Salm, C., Van den Toorn, A., Chardon, W. J., and Koopmans, G. F. (2012). Water and nutrient transport on a heavy clay soil in a fluvial plain in The Netherlands. J. Environ. Qual. 41, 229–241. doi: 10.2134/jeq2011.0292
Van der Stelt, B., Temminghoff, E., Van Vliet, P., and Van Riemsdijk, W. (2007). Volatilization of ammonia from manure as affected by manure additives, temperature and mixing. Bioresour. Technol. 98, 3449–3455. doi: 10.1016/j.biortech.2006.11.004
Van der Weerden, T. J., Noble, A., De Klein, C. A. M., Hutchings, N., Thorman, R. E., Alfaro, M. A., et al. (2021). Ammonia and nitrous oxide emission factors for excreta deposited by livestock and land-applied manure. J. Environ. Qual. 50, 1005–1023. doi: 10.1002/jeq2.20259
Van der Zee, T., Bannink, A., Van Bruggen, C., Groenestein, K., Huijsmans, J., Van der Kolk, J., et al. (2021). Methodology for Estimating Emissions From Agriculture in the Netherlands. Calculations for CH4, NH3, N2O, NOx, NMVOC, PM10, PM2.5 and CO2 Using the National Emission Model for Agriculture (NEMA) – Update 2021, RIVM, Report 2021-0008. Bilthoven: RIVM.
Van Dijk, K. C., Lesschen, J. P., and Oenema, O. (2016). Phosphorus flows and balances of the European Union Member States. Sci. Total Environ. 542, 1078–1093. doi: 10.1016/j.scitotenv.2015.08.048
Van Grinsven, H., Van Dam, J. D., Lesschen, J., Timmers, M., Velthof, G., and Lassaletta, L. (2018). Reducing external costs of nitrogen pollution by relocation of pig production between regions in the European Union. Reg. Environ. Change 18, 1–13. doi: 10.1007/s10113-018-1335-5
Van Grinsven, H. J. M., Erisman, J. W., De Vries, W., and Westhoek, H. (2015). Potential of extensification of European agriculture for a more sustainable food system, focusing on nitrogen. Environ. Res. Lett. 10:025002. doi: 10.1088/1748-9326/10/2/025002
Van Grinsven, H. J. M., Tiktak, A., and Rougoor, C. W. (2016). Evaluation of the Dutch implementation of the nitrates directive, the water framework directive and the national emission ceilings directive. Wageningen J. Life Sci. 78, 69–84. doi: 10.1016/j.njas.2016.03.010
Van Middelkoop, J. C., and Holshof, G. (2017). nitrogen fertilizer replacement value of concentrated liquid fraction of separated pig slurry applied to grassland. Commun. Soil Sci. Plant Anal. 48, 1132–1144. doi: 10.1080/00103624.2017.1323101
Van Puffelen, J. L., Brienza, C., Regelink, I. C., Sigurnjak, I., Adani, F., Meers, E., et al. (2022). Performance of a full-scale processing cascade that separates agricultural digestate and its nutrients for agronomic reuse. Separat. Purif. Technol. 297:121501. doi: 10.1016/j.seppur.2022.121501
Van Selm, B., Hijbeek, R., Van Ittersum, M. K., Van Hal, O., Van Middelaar, C. E., and De Boer, I. J. M. (2023). Recoupling livestock and feed production in the Netherlands to reduce environmental impacts. Sci. Total Environ. 899:165540. doi: 10.1016/j.scitotenv.2023.165540
Van Soest, P., and Wine, R. (1968). Determination of lignin and cellulose in acid-detergent fiber with permanganate. J. Assoc. Off. Anal. Chem. 51, 780–785. doi: 10.1093/jaoac/51.4.780
Velthof, G., and Oenema, O. (1993). Nitrous oxide flux from nitric-acid-treated cattle slurry applied to grassland under semi-controlled conditions. Netherlands J. Agric. Sci. 41, 81–93. doi: 10.18174/njas.v41i2.624
Velthof, G. L., Brader, A. B., and Oenema, O. (1996). Seasonal variations in nitrous oxide losses from managed grasslands in The Netherlands. Plant Soil 181, 263–274. doi: 10.1007/BF00012061
Velthof, G. L., and Mosquera, J. (2011). The impact of slurry application technique on nitrous oxide emission from agricultural soils. Agric. Ecosyst. Environ. 140, 298–308. doi: 10.1016/j.agee.2010.12.017
Velthof, G. L., Oudendag, D., Witzke, H. P., Asman, W. A. H., Klimont, Z., and Oenema, O. (2009). Integrated assessment of nitrogen losses from agriculture in EU-27 using MITERRA-EUROPE. J. Environ. Qual. 38, 402–417. doi: 10.2134/jeq2008.0108
Vera (2009). Test Protocol for Measurement of Gaseous Emissions From Land Applied Manure, Verification of Environmental Technologies for Agricultural Production, Version 1, 2009-12-09. Delft.
Viaene, J., Nelissen, V., Reubens, B., Willekens, K., Driehuis, F., De Neve, S., et al. (2017). Improving the product stability and fertilizer value of cattle slurry solid fraction through co-composting or co-ensiling. Waste Manag. 61, 494–505. doi: 10.1016/j.wasman.2016.12.037
Vira, J., Hess, P., Melkonian, J., and Wieder, W. R. (2020). An improved mechanistic model for ammonia volatilization in Earth system models: flow of Agricultural Nitrogen version 2 (FANv2). Geosci. Model Dev. 13, 4459–4490. doi: 10.5194/gmd-13-4459-2020
Wagner, C., Nyord, T., Vestergaard, A. V., Hafner, S. D., and Pacholski, A. S. (2021). Acidification effects on in situ ammonia emissions and cereal yields depending on slurry type and application method. Agriculture 11:1053. doi: 10.3390/agriculture11111053
Wang, Y., Ying, H., Yin, Y., Zheng, H., and Cui, Z. (2019). Estimating soil nitrate leaching of nitrogen fertilizer from global meta-analysis. Sci. Total Environ. 657, 96–102. doi: 10.1016/j.scitotenv.2018.12.029
Webb, J., Chadwick, D., and Ellis, S. (2004). Emissions of ammonia and nitrous oxide following incorporation into the soil of farmyard manures stored at different densities. Nutr. Cycl. Agroecosyst. 70, 67–76. doi: 10.1023/B:FRES.0000045985.32440.27
Webb, J., Lalor, S. T. J., Bittman, S., Misselbrook, T., Sutton, M. A., Menzi, H., et al. (2014a). “Manure application techniques,” in Options for Ammonia Mitigation: Guidance from the UNECE Task Force on Reactive Nitrogen, eds S. Bittman, M. Dedina, C. M. Howard, O. Oenema, and M. A. Sutton (Edinburgh: Centre for Ecology and Hydrology), 29–40.
Webb, J., Sørensen, P., Velthof, G., Amon, B., Pinto, M., Rodhe, L., et al. (2013). “Chapter seven - an assessment of the variation of manure nitrogen efficiency throughout europe and an appraisal of means to increase manure-N efficiency,” in Advances in Agronomy, ed. D. L. Sparks (Academic Press), 371–442. doi: 10.1016/B978-0-12-407247-3.00007-X
Webb, J., Thorman, R. E., Fernanda-Aller, M., and Jackson, D. R. (2014b). Emission factors for ammonia and nitrous oxide emissions following immediate manure incorporation on two contrasting soil types. Atmos. Environ. 82, 280–287. doi: 10.1016/j.atmosenv.2013.10.043
Wei, Z., Hoffland, E., Zhuang, M., Hellegers, P., and Cui, Z. (2021). Organic inputs to reduce nitrogen export via leaching and runoff: a global meta-analysis. Environ. Pollut. 291:118176. doi: 10.1016/j.envpol.2021.118176
Weishaupt, A., Ekardt, F., Garske, B., Stubenrauch, J., and Wieding, J. (2020). Land use, livestock, quantity governance, and economic instruments—Sustainability beyond big livestock herds and fossil fuels. Sustainability 12:2053. doi: 10.3390/su12052053
Westerik, D., Hoffland, E., and Hijbeek, R. (2023). Nitrogen fertilizer replacement values of organic amendments: determination and prediction. Nutr. Cycl. Agroecosyst. doi: 10.1007/s10705-023-10316-7
Wester-Larsen, L., Müller-Stöver, D. S., Salo, T., and Jensen, L. S. (2022). Potential ammonia volatilization from 39 different novel biobased fertilizers on the European market - A laboratory study using 5 European soils. J. Environ. Manage. 323:116249. doi: 10.1016/j.jenvman.2022.116249
Wheeler, E. F., Adviento-Borbe, M. A. A., Brandt, R. C., Topper, P. A., Topper, D. A., Elliott, H. A., et al. (2010). Amendments for Mitigation of Dairy Manure Ammonia and Greenhouse Gas Emissions: Preliminary Screening. Pittsburgh, PA: American Society of Agricultural and Biological Engineers, 1.
Yilmaz, E., and Sönmez, M. (2017). The role of organic/bio–fertilizer amendment on aggregate stability and organic carbon content in different aggregate scales. Soil Till. Res. 168, 118–124. doi: 10.1016/j.still.2017.01.003
Young, M. D., Ros, G. H., and De Vries, W. (2021). Impacts of agronomic measures on crop, soil, and environmental indicators: a review and synthesis of meta-analysis. Agric. Ecosyst. Environ. 319:107551. doi: 10.1016/j.agee.2021.107551
Zeeman, G. (1991). Mesophilic and Psychrophilic Digestion of Liquid Manure. Wageningen: Landbouwuniversiteit Wageningen.
Keywords: organic fertilizer, manure, compost, nitrogen, phosphorus, carbon, emission, soil
Citation: Velthof GL, Cals TCA, van 't Hull JP, Lesschen JP, Lessmann M, Porre RJ, Ros MBH, Rietra RPJJ, Schoumans OF, Veenemans L and Westerik D (2024) Managing organic resources in agriculture: future challenges from a scientific perspective. Front. Sustain. Food Syst. 8:1393190. doi: 10.3389/fsufs.2024.1393190
Received: 28 February 2024; Accepted: 30 April 2024;
Published: 17 May 2024.
Edited by:
Rachel E. Thorman, Agricultural Development Advisory Service, United KingdomReviewed by:
John Williams, Agricultural Development Advisory Service, United KingdomLars Stoumann Jensen, University of Copenhagen, Denmark
Copyright © 2024 Velthof, Cals, van 't Hull, Lesschen, Lessmann, Porre, Ros, Rietra, Schoumans, Veenemans and Westerik. This is an open-access article distributed under the terms of the Creative Commons Attribution License (CC BY). The use, distribution or reproduction in other forums is permitted, provided the original author(s) and the copyright owner(s) are credited and that the original publication in this journal is cited, in accordance with accepted academic practice. No use, distribution or reproduction is permitted which does not comply with these terms.
*Correspondence: Gerard L. Velthof, gerard.velthof@wur.nl